- 1Liggins Institute, University of Auckland, Auckland, New Zealand
- 2Department of Health Sciences, Carleton University, Ottawa, ON, Canada
Introduction: Maternal periconceptional undernutrition (PCUN) alters fetal hypothalamic-pituitary-adrenal axis (HPAA) function and placental glucocorticoid metabolism in sheep. The effects of PCUN on HPAA function in adult life are not known. We investigated the effects of PCUN on fetal adrenal development across gestation and on cortisol regulation in adult offspring.
Methods: Ewes were undernourished from 61 days before to 30 days after conception (‘PCUN’) or fed ad libitum (‘N’). mRNA expression in the fetal adrenal gland of ACTH receptor (ACTHR), steroidogenic acute regulatory protein (STAR), cytochrome P450 17A1 (CYP17A1), 11beta-hydroxysteroid-dehydrogenase type 2 (11βHSD2), insulin-like growth factor-2 (IGF2), and in the fetal hippocampus of 11βHSD1, 11βHSD2, mineralocorticoid receptor (MR) and glucocorticoid receptor (GR) was determined at 50 (adrenal only), 85, 120 and 131 days of gestation (term=148 days). In adult offspring (≥ 3 years, N; 10 female, 5 male, PCUN; 10 female, 10 male) a combined arginine vasopressin (AVP, 0.1 μg/kg) and corticotropin-releasing hormone (CRH, 0.5 μg/kg) challenge and a metyrapone (40 mg/kg) challenge were undertaken. mRNA expression of ACTHR, STAR and CYP17A1 were determined in adult adrenals.
Results: Fetal adrenal STAR, CYP17A1 and IGF2 mRNA expression were not different between groups in early gestation but were higher in PCUN than N at 131 days’ gestation (all p<0.01). PCUN reduced fetal hippocampal MR and GR mRNA expression by 50% at 85 day, but not in later gestation. Adult offspring plasma cortisol responses to AVP+CRH or metyrapone were not different between groups. Plasma ACTH response to AVP+CRH was lower in PCUN males but ACTH response to metyrapone was not different between groups. Adult adrenal ACTHR, STAR, and CYP17A1 mRNA expression were not affected by PCUN.
Conclusions: We conclude that the effects of PCUN on fetal HPAA function that became apparent in late gestation, are not reflected in adrenal cortisol secretion in mid-adulthood.
1 Introduction
Poor maternal nutrition around the time of conception is associated with an increased risk of impaired glucose regulation, cardiovascular disease and obesity in the offspring (1). Sheep studies indicate that the effects of periconceptional maternal undernutrition (PCUN) may be mediated by altered maternal and fetal hypothalamo-pituitary-adrenal axis (HPAA) function (2, 3) and placental glucocorticoid metabolism (3–5) during pregnancy. Central to this phenomenon is the concept that in the face of an adverse maternal environment, adaptations made for maintenance of pregnancy and survival of offspring from birth to reproductive age may lead to a mismatch with later environmental influences during postnatal life (6). Longitudinal studies in large mammals, such as the sheep, have the potential to investigate potential mechanisms as well as effects of the exposure through to adulthood within a reasonable timeframe.
The periconceptional period is generally defined as that extending from oocyte maturation, follicular development, conception, and the embryonic blastocyst stage up until implantation (7). Maternal nutrition around this time may have powerful effects on hormonal axes such as the HPAA (4). The effects of PCUN may be found in tissues and organs of different germ line origin such as muscle (8), gonads (9) and regions of the brain such as the hypothalamus, and likely are mediated by epigenetic modifications of DNA (10), such as methylation (11).
Precocious activation of the fetal HPAA in response to PCUN has been reported in sheep (12); however, in the same paradigm, by young adulthood circulating plasma cortisol responses to AVP+CRH stimulation were depressed (13). The level of the HPAA at which this suppression of cortisol responses is regulated is not known, nor is it clear whether the postnatal suppression of cortisol response in PCUN offspring persists to later adulthood. Alterations on HPAA responsiveness may have very important implications for risk of disease in later life, for example via immune function (14, 15).
We aimed to characterize the effects of PCUN on the ontogeny of fetal HPAA development and on HPAA function in mid-adulthood, in response to stimulation by both corticotropic hormones and blockade of cortisol synthesis.
2 Materials and methods
2.1 Animals
All animal experimentation was approved by the University of Auckland Animal Ethics Committee (Approvals AEC/07/2004/R277, AEC/07/2005/R389, and AEC/02/2008/R628) and conducted in accordance with those approvals.
Multiparous 4- to 5-year-old Romney ewes were acclimatized to a custom-made total concentrate ration (Dunstan Nutrition, Hamilton, NZ) over 10 days on pasture prior to feedlot entry (16). Ewes were then randomly allocated to 2 treatment groups: controls (N; daily feed intake 3-4% of body weight) or undernutrition from 61 days before to 30 days after mating (PCUN). Undernutrition involved a 2-day fast then individual adjustment of feed to achieve and maintain 10% body weight reduction (16). In undernourished ewes, feed intake after fasting was 1-2% of body weight per day but increased to around 80% of controls before restriction was lifted. Representative maternal weight data during pregnancy are reported elsewhere (17).
Ewes were housed indoors in a photoperiod-controlled feedlot from 70 days before mating until 2 weeks after lambing. While undergoing nutritional restriction ewes were housed individually in 1.2 × 1.4 m pens with mesh sides to allow visual contact between ewes. All ewes were housed in individual pens from 2 weeks before, until 2 weeks after lambing (term gestation is ~148 days after mating).
Two weeks before mating with Dorset rams, estrus was synchronized using an intravaginal progesterone release device (Eazi-Breed CIDR; Zoetis, NZ, Ltd) (18). Ewes identified as carrying singleton lambs by ultrasonography 50 days after mating were retained for the studies. Separate cohorts of singleton-bearing ewes were euthanized at 50 (N=7, PCUN=3), 85 (N=7, PCUN=8), 120 (N=12, PCUN=10) and 131 (N=9, PCUN=11) days of pregnancy for collection of fetal adrenal and hippocampal (except day 50) samples that were stored at -85°C for later mRNA analysis.
Ewes and lambs for the postnatal studies were returned to pasture 2 weeks after birth. Weight and size measurements been reported previously (19, 20). Lambs were weaned at 12 weeks of age. Between 3 and 4 years of age offspring (N: 10 females, 5 males; PCUN: 10 females, 10 males) were brought indoors and acclimatized for a week to full ration feeding with the same concentrate feed used in the maternal manipulations (~3% of body weight per day). The procedures were the same as those described for HPAA tests undertaken at 10 months, in the same animals (13). Indwelling catheters (size 040, Critchley Electrical, Auburn, Australia) were inserted into both jugular veins under local anesthesia. Ewes were fitted with CIDR releasing devices to prevent estrus (18) occurring during endocrine testing. Two to 3 days after catheter insertion animals underwent AVP + CRH challenge. After a 3 mL baseline blood sample, equimolar doses of bovine CRH (0.5 μg/Kg) and AVP (0.1 μg/kg, both Sigma Chemical Co., St Louis, MO, USA) were given intravenously. Further 3 mL blood samples were taken 15, 30, 45, 60, 120 and 240 minutes after the dose. Two days later blockade of cortisol synthesis was induced using Metyrapone (40 mg/Kg intravenously), supplied by Novartis, Basel, Switzerland, 09-MTA-046). Three mL blood samples were taken before blockade and then 30, 60 and 120 minutes later. Samples were centrifuged at 3,000 g and plasma split into two aliquots and stored at -20°C until analysis. Three days after the conclusion of the endocrine studies sheep were euthanized by anesthetic overdose (20). Adrenal tissues were snap-frozen in liquid nitrogen and stored at -85°C until later analysis.
2.2 Hormonal analysis
Plasma ACTH was measured using a commercial radioimmunoassay kit (DiaSorin Inc., Stillwater, MN, USA) previously validated for sheep (21); inter- and intra-assay coefficients of variation were 13.0% and 7.6%, respectively. Plasma cortisol and its precursor 11-deoxycortisol were measured by liquid chromatography tandem mass-spectrometry as described previously (22) with mean inter- and intra-assay coefficients of variation of 11.0 and 4.3%, respectively.
2.3 RNA isolation and cDNA synthesis
Adult adrenal was pulverized into powder using 6870 Freezer Mill Cryogenic Grinder (SPEX SamplePrep, USA). Fetal hippocampus and fetal adrenal were ground into powder using mortar and pestle in liquid nitrogen. Total RNA was extracted using TRIZOL (Invitrogen, USA). RNA concentration was measured and the absorbance ratios 260/280 and 260/230 with ratios ≥1.9 considered acceptable purity. RNA gels were run to check the RNA integrity. Total RNA (2.5 µg) was treated with RNase-free DNase I (Invitrogen, USA) before reverse transcriptase polymerase chain reaction (RT-PCR) to eliminate potential genomic DNA. First-strand cDNA was synthesized using SuperScript® VILO™ cDNA Synthesis system (Invitrogen, USA).
2.4 Primers and Taqman probes
All primers and Taqman probes were designed using Primer Express Software (Applied Biosystems, USA). The following genes were studied, ACTH receptor (ACTHR), steroidogenic acute regulatory protein (STAR), cytochrome P450 17A1 (CYP17A1), 11beta-hydroxysteroid-dehydrogenase type 2 (11βHSD2), insulin-like growth factor-2 (IGF2), and in the fetal hippocampus 11βHSD1, 11βHSD2, mineralocorticoid receptor (MR) and glucocorticoid receptor (GR). A BLAST search ensured that primers and probes were not designed from homologous regions that would encode for genes other than our target genes (Table 1). Reference genes primer and probe sequences have been previously published (23).
2.5 Analysis and selection of stably expressed reference genes
For fetuses, real time PCR data were normalized with 18s; these studies were performed shortly after tissue collection to avoid any tissue deterioration from prolonged storage, and this was the method of normalization used at that time. For adult animals, analysis was ~4 years later and real-time PCR data were normalized with multiple stably expressed reference genes reflecting development in analytical approach over time. Using BestKeeper (24), RPL19, GAPDH and HPRT1 were found to be the most stably expressed HKGs (with SD<1) amongst our seven candidate reference genes: RPL19, GAPDH, HPRT1, YWHAZ, ACTB, CYPA, 18S.
2.6 Real time PCR and relative gene expression analysis
Real time PCR was carried out using ABI 7900HT Real-Time PCR system (Applied Biosystems). Samples were performed in triplicate and an average quantification cycle () value was obtained for each triplicate. Standard curves for the target genes and reference genes were included in the runs to calculate the amplification efficiencies (E) of the genes using E = 10(-1/slope) (24) value was obtained for each triplicate. Standard curves for the target genes and reference genes were included in the runs to calculate the amplification efficiencies (E) of the genes using E = 10(-1/slope) (24). The relative expression value (RE) in fetal tissue was calculated using mathematical model described by Pfaffl et al (25); the RE in adult tissue was calculated using a mathematical model that accounts for normalization against multiple reference genes (26, 27).
2.7 Statistical analysis
Basal hormonal and area under the curve (AUC) responses to challenge tests were analyzed initially by factorial ANOVA and then multiple regression with treatment group and sex as covariates (JMP software, version 15). Changes over time during the challenge tests were analyzed by repeated measures MANOVA with Tukey-Kramer correction for multiple comparisons. Significant differences were accepted at the 5% level. Data are presented as mean ± SEM. For mRNA, statistical analyses were conducted using R version 4.1.1. Gene expression results are presented in log2 fold change by log2 transformation of the RE value. Statistical differences in log2 fold change were analyzed using Student’s t-test. A p value < 0.05 was considered statistically significant.
Figure 1 shows how the current studies relate to other HPAA-related studies on this same cohort, previously undertaken (13, 28, 29)
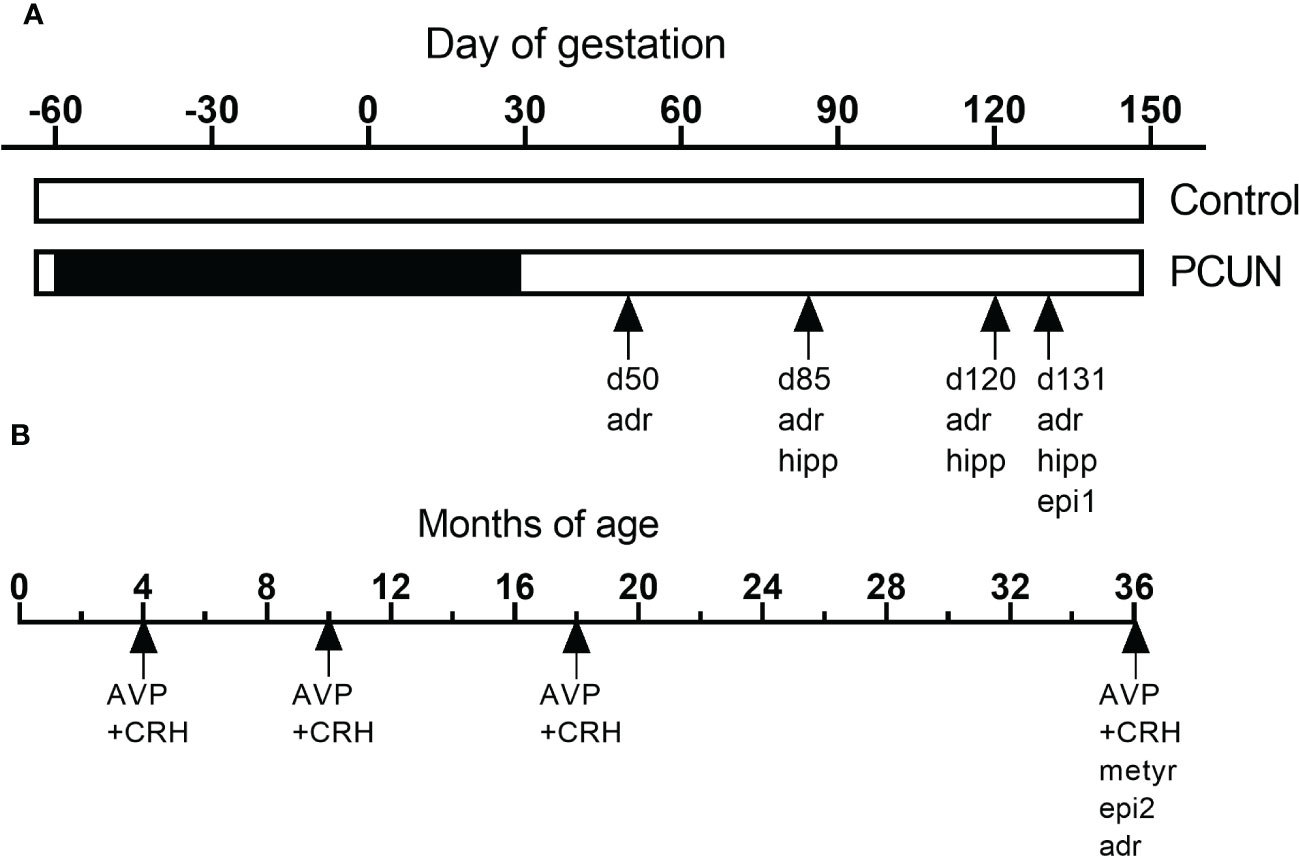
Figure 1 Schematic representation of the various studies using this cohort of animals during fetal (A) and postnatal life (B). ‘adr’, mRNA expression in adrenals. ‘hip’, mRNA expression in hippocampus. ‘epi1’, fetal epigenetic studies (28). ‘epi2, epigenetic studies in adult (29). ‘‘AVP+CRH’, corticotropin stimulation tests in previous (13) and the current study. ‘met’, metyrapone blockade.
3 Results
Growth and post-mortem morphometric data for both fetuses and adult offspring have been reported previously (17, 20).In brief, there were no effects of PCUN on body or adrenal weight in fetal life, at birth or in middle adulthood.
3.1 Fetal hippocampus
Hippocampal mRNA expression of both GR and MR mRNA was decreased in PCUN fetuses compared to their N counterparts at 85 days gestation (Figure 2, p < 0.05). There were no differences at the later ages examined. Expression of 11β-HSD1 and 11β-HSD2 in the fetal hippocampus were not affected by PCUN.
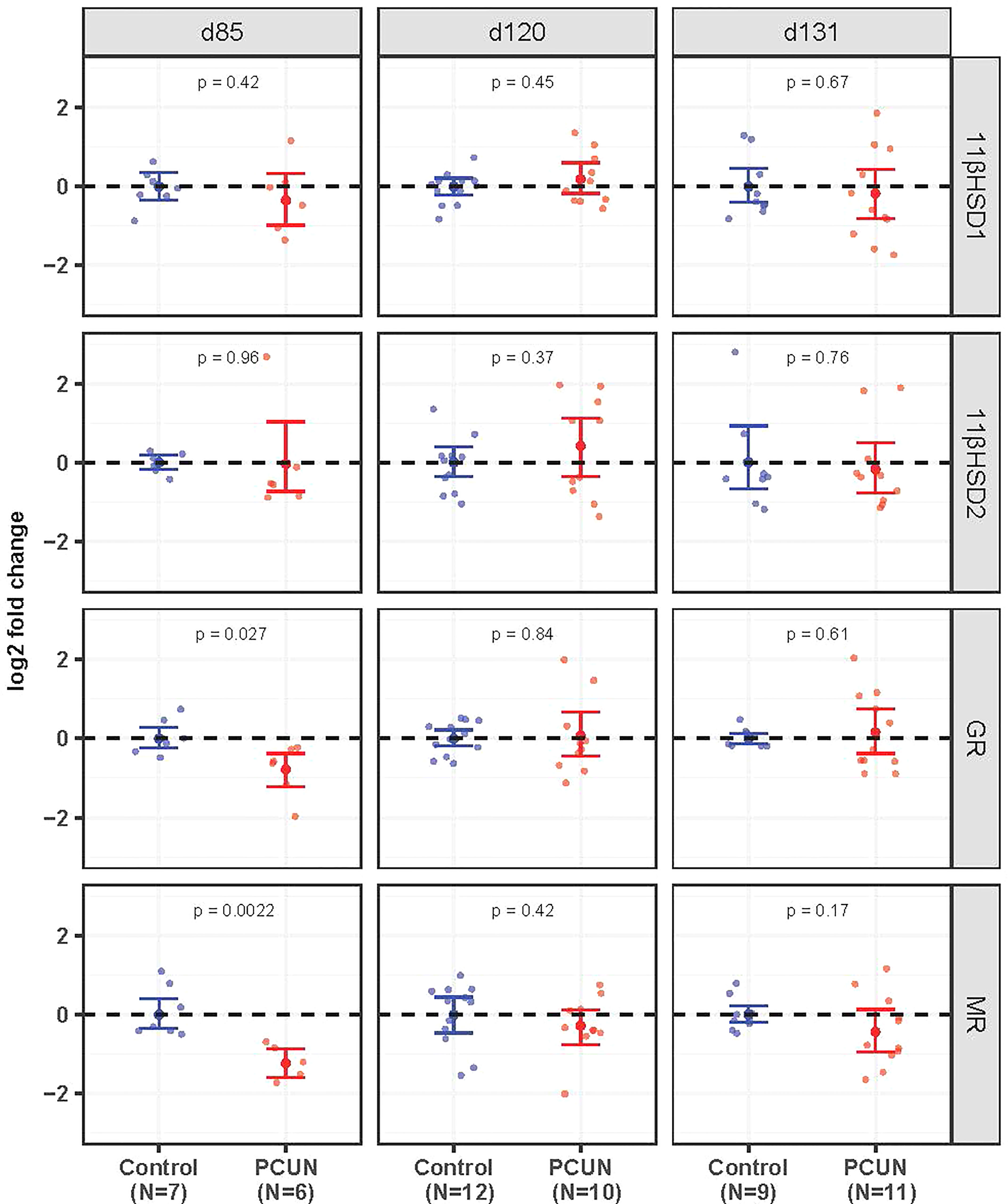
Figure 2 Fetal hippocampal mRNA expression. Log2 fold change of 11βHSD1, 11βHSD2, GR and MR mRNA expression levels in PCUN (symbol in red) in comparison to N (symbol in blue) based on real-time PCR analysis in the fetal hippocampus. Data are shown as mean ± 95% confidence interval. Symbols represent individual animals. Student’s t-tests were used to assess statistical difference, with significance defined as p < 0.05.
3.2 Fetal adrenal
Prior to 131 days of gestation there were no effects of PCUN on expression of mRNA for any of the fetal adrenal targets examined (Figure 3). At 131 days expression of STAR, CYP17A1, IGF2 and 11β-HSD2 mRNA were all higher in PCUN relative to N (all p<0.05), while ACTHR mRNA expression was unaffected.
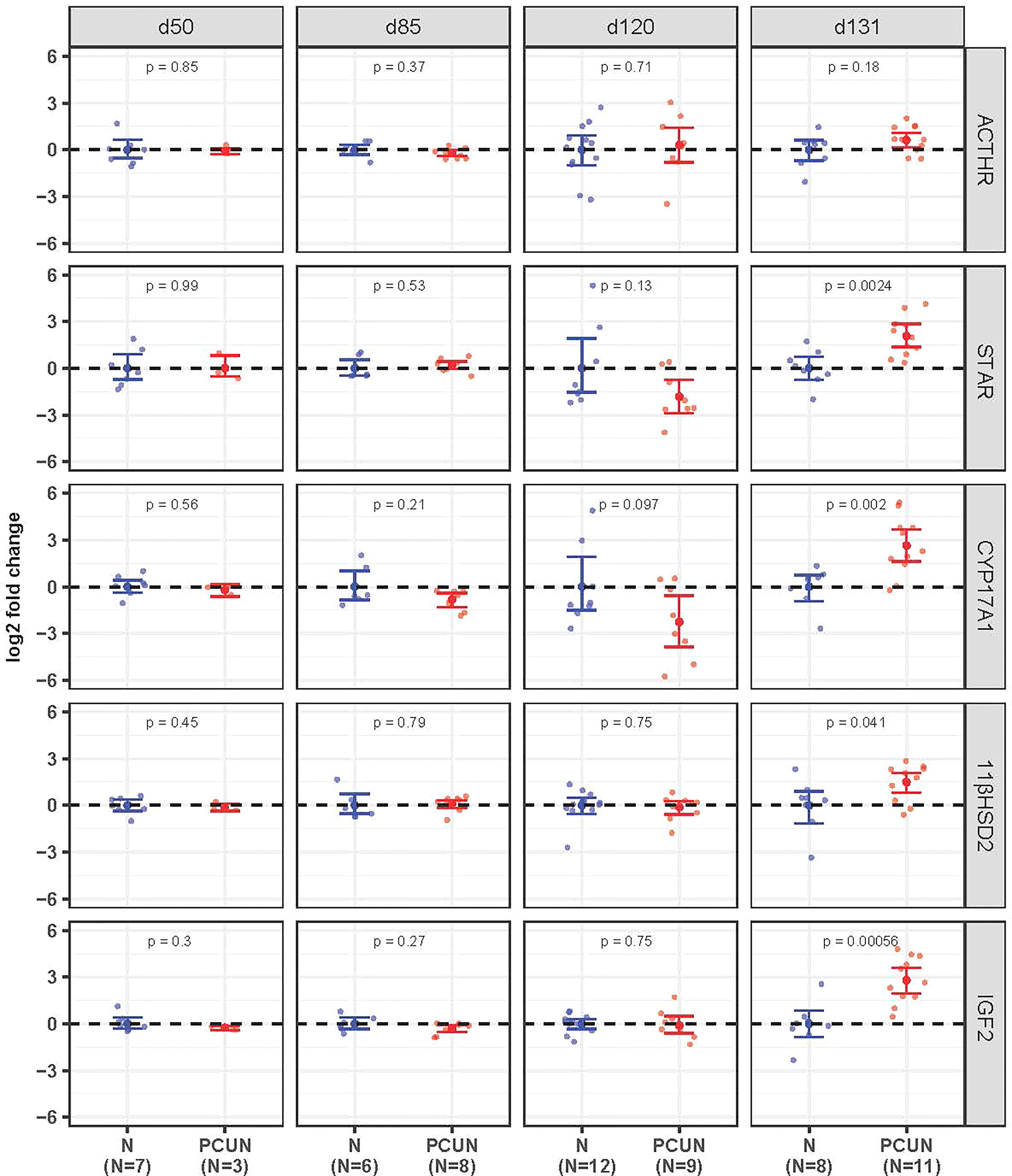
Figure 3 Fetal adrenal mRNA expression. Log2 fold change of ACTHR, STAR, CYP17A1, 11βHSD2, and IGF2 mRNA expression levels in PCUN (symbol in red) in comparison to N (symbol in blue) based on real-time PCR analysis in the fetal adrenal. Data are shown as mean ± 95% confidence interval. Symbols represent individual animals. Student’s t-tests were used to assess statistical difference, with significance defined as p < 0.05.
3.3 Adult adrenal stimulation tests
Plasma ACTH and cortisol concentrations before AVP+CRH challenge were not different between treatment groups, but baseline cortisol concentrations were 2-3-fold higher in females than males (Figure 4, N female, 12.1 ± 3.0; PCUN female, 9.5 ± 3.0 vs. N male, 4.3 ± 1.2; PCUN male, 3.6 ± 0.9 ng/mL, p < 0.05 for sex effect). Plasma ACTH AUC response (0-240 min) to AVP+CRH stimulation in females was not affected by PCUN (N, 22 ± 3 vs. 24 ± 3 ng/ml/min); in males, PCUN offspring had a greater AUC response than N males (22 ± 2 vs. 15 ± 3, ng/ml/min, p < 0.05, Figure 4). Cortisol AUC response to AVP+CRH stimulation was not affected by PCUN over 240 min (Figure 4); however, females had a greater cortisol AUC response than males (7861 ± 355 vs. 4485 ± 410 ng/ml/min, p < 0.0001). Plasma ACTH to cortisol AUC ratio (0-240 min) was not different between groups or between sexes (data not shown). At 240 minutes post AVP+CRH challenge plasma ACTH and cortisol concentrations were similar between groups, but cortisol concentrations remained higher in females than in males (N female, 19 ± 1; PCUN female, 16 ± 1 vs. N male, 9.8 ± 2.6; PCUN male, 10.3 ± 1.8 ng/mL, p < 0.0001).
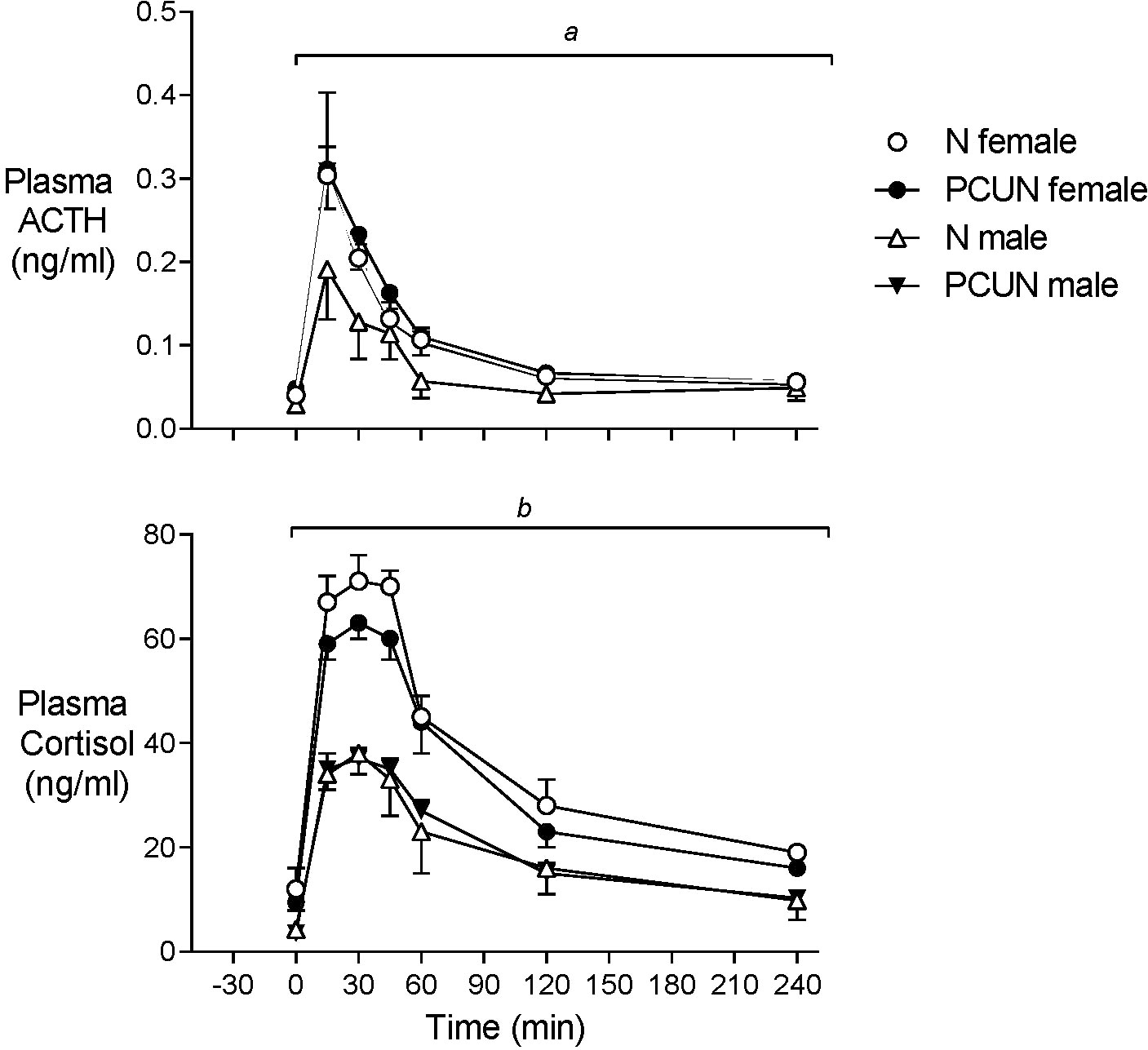
Figure 4 Adult plasma ACTH and cortisol responses to equimolar challenge of intravenous AVP+CRH (0.1 and 0.5 μg/kg respectively) given at time ‘0’. atreatment x time interaction in males. bsex effect (p < 0.0001). Values are mean ± SEM.
Prior to metyrapone blockade of cortisol synthesis plasma concentrations of ACTH were similar in males and females of both treatment groups (Figure 5). Basal plasma cortisol concentrations were higher in females than males in the N group (11.2 ± 2.5 vs. 1.1 ± 3.5 ng/ml, Figure 4, p < 0.05) but not the PCUN group (females, 3.7 ± 1.2 vs. males, 4.6 ± 1.2 ng/ml). Within 30 min of intravenous injection of metyrapone, plasma cortisol had fallen significantly in all animals except N males (group*time interaction, p < 0.001, Figure 4). However, plasma concentrations of both ACTH and 11-deoxycortisol increased in both sexes of both treatment groups (p < 0.0001), consistent with a feedback response to blockade of cortisol synthesis, and elevations of both ACTH and 11-deoxycortisol were greater in females than in males over the 120 minutes (repeated measures, p < 0.05). The subsequent increase in cortisol was greater over time in N than in PCUN females (p < 0.05) leading to higher values at 120 min (13.9 ± 3.9 vs. 5.4 ± 1.0 ng/ml, p <0.05).
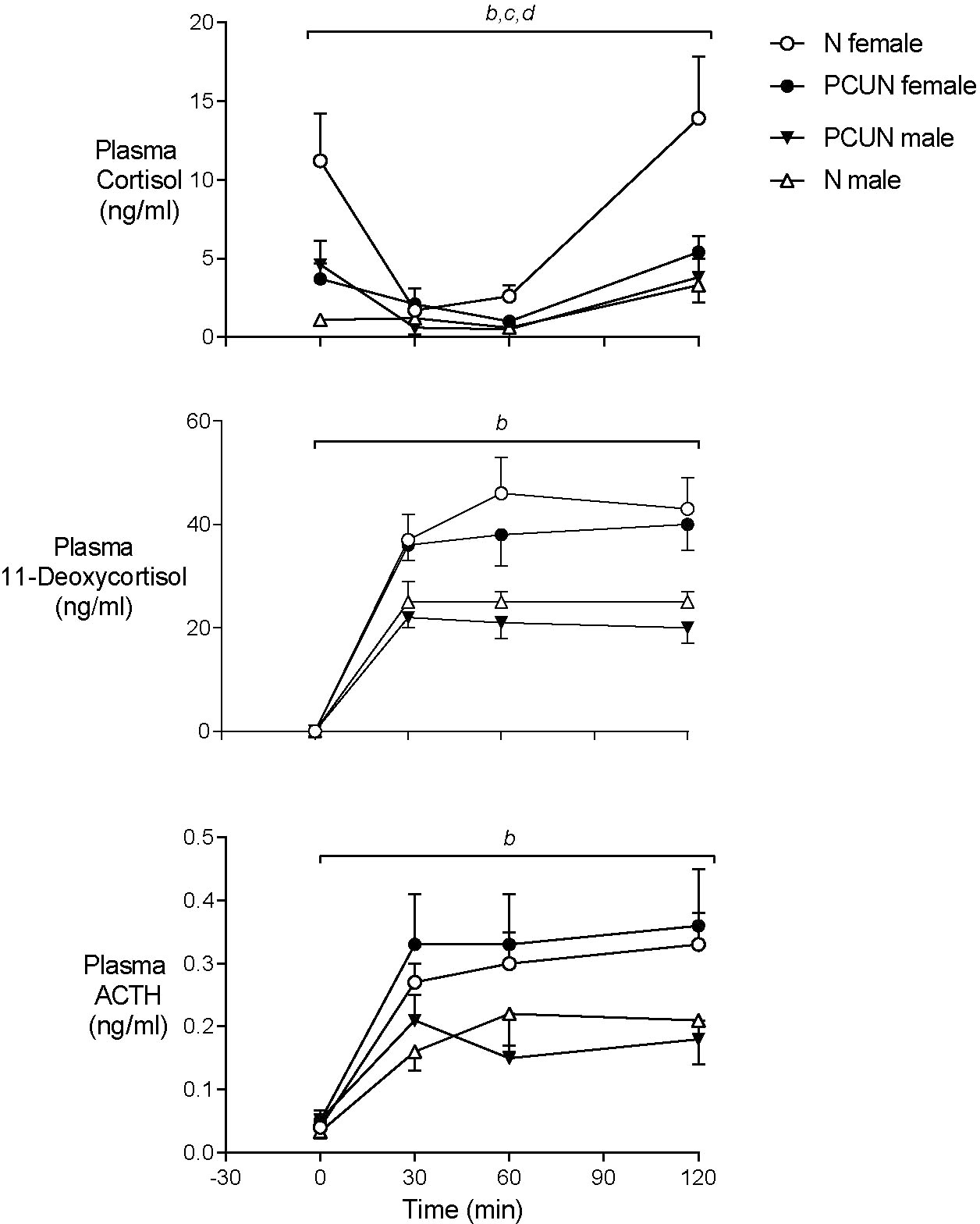
Figure 5 Adult plasma cortisol, 11-Deoxycortisol and ACTH responses to cortisol synthesis blockade with intravenous metyrapone (40 mg/kg) given at time ‘0’. bsex effect (p < 0.0001). ctreatment x sex interaction (p < 0.05). dtreatment effect in females (p < 0.05). Values are mean ± SEM.
3.4 Adult adrenal
Adrenal ACTHR, STAR, and CYP17A1 mRNA expression values were not different between adult PCUN and N offspring (Figure 6).
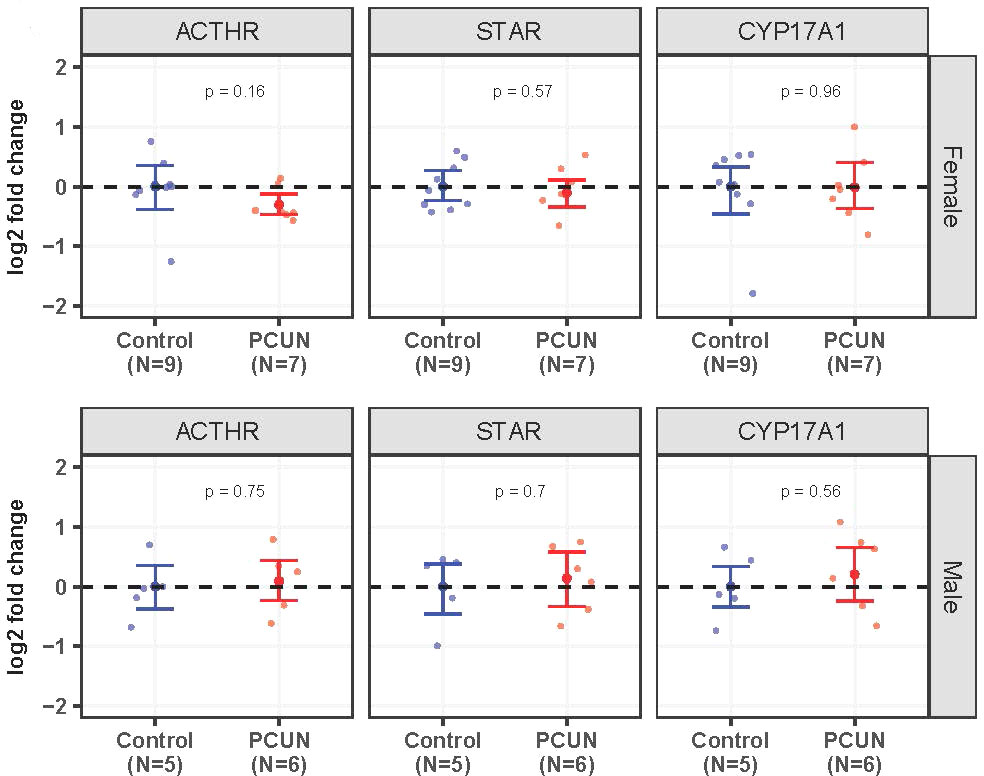
Figure 6 Adult adrenal mRNA expression. Log2 fold change of ACTHR, STAR, and CYP17A1 mRNA expression levels in PCUN (symbol in red) in comparison to N (symbol in blue) in female (A) and in male (B). Data are mean ± 95% confidence interval. Symbols represent individual animals. Student’s t-tests were used to assess statistical difference, with significance defined as p < 0.05.
4 Discussion
Our findings demonstrate that the effect of PCUN on the fetal adrenal steroidogenic pathway is not apparent until late in gestation, approximately 100 days after the end of the nutritional manipulation. In concert with increased fetal adrenal STAR and CYP17A1 mRNA expression we also report that IGF2 and 11β-HSD2 expression was increased in PCUN relative to N fetal adrenals at 131 days. Maturation and growth of the fetal adrenal steroidogenic pathway is influenced by a range of factors including exposure to ACTH and its precursors, and fetal growth factors like the IGFs (30). In previous studies at 131 days of gestation we have shown that PCUN leads to increased pituitary message for proopiomelanocortin (POMC) RNA and factors involved in post-translational processing of POMC to form functional ACTH, such as prohormone convertase-1 (2). The higher expression of 11β-HSD2 expression in PCUN adrenals may also indicate relative maturation as this enzyme is thought to be a fine-tuning modulator of cortisol secretion (31). Increased expression and activity could favor a feed forward loop favoring further development of cortisol secretion by the maturing fetal adrenal.
Previous studies in rats suggest maternal glucocorticoids mediate responses to maternal nutritional stress which in turn mediate effects on fetal HPAA development (32, 33). Studies in late-gestation sheep demonstrate that maternal exposure to synthetic glucocorticoids affects fetal HPAA development (34). We previously have reported that placental 11β-HSD2, the enzyme that inactivates cortisol to cortisone, had reduced activity at day 55, 25 days after ewes returned to a normal nutritional plane following PCUN (5). This decrease in activity was accompanied by a higher cortisol/cortisone ratio in the blood of PCUN fetuses compared to N fetuses. Glucocorticoids are known to counter-regulate mRNA expression of their own receptors (35). However, there may be crossover effects with evidence that aldosterone acting through the MR may influence both GR and MR expression in the hippocampus (36). In this study we report day 85 fetal hippocampal GR and MR mRNA expression were decreased in PCUN, relative to N fetuses, indicating that a long-term effect of PCUN had occurred. This change in fetal hippocampal GR and MR expression may indicate the start of a cascade of events that leads to earlier functional maturation of the HPAA during late gestation.
In the current studies we found no significant effect of PCUN on plasma cortisol response to AVP+CRH stimulation in female and male sheep at 3-4 years of postnatal age. Our studies at 18 months of age showed that the effect of PCUN on plasma cortisol was waning in males compared to studies at 10 months (13). Plasma ACTH responses to AVP+CRH stimulation were higher in PCUN, than in N males in the current studies. As cortisol response was not different, this suggests either adrenal ACTH receptor or post receptor mechanisms may be affected by PCUN. We do not have ACTH receptor protein or binding studies, but neither adrenal ACTHR mRNA nor that of STAR and CYP17A were affected by PCUN. Taken together, the AVP+CRH simulation test, cortisol synthesis blockade with metyrapone and adrenal mRNA data indicate that any effects of PCUN on cortisol secretion have largely disappeared by 3-4 years of postnatal age; approximate middle age in sheep.
Up until this investigation, studies on HPAA function in the sheep have been limited to early adulthood. Compared to the studies at 18 months, peak plasma cortisol responses to CRH+AVP had increased by 20-30% by 3-4 years of age, independent of any effect of maternal periconceptional nutrition. Studies in the human suggest that cortisol secretion increases with aging (37) so perhaps this increase in secretory activity overrides the influence of PCUN.
Although we have shown that there was a diminution of the effect of PCUN on cortisol secretory response, other studies indicate that effects of PCUN, possibly mediated by the central HPAA, do persist into mid-adulthood. For example, male PCUN offspring had increased fat mass 3-4 years of age (20) following decreased spontaneous locomotor activity at 18 months of postnatal age (38). Additional evidence comes from the observation made in animals from the current study where PCUN resulted in disturbance of hypothalamic GR and POMC epigenetic regulation, extending to both mRNA and protein expression that persisted from the late gestation fetal sheep (28) until at least 3-4 years of age (29). These persistent alterations in epigenetic and message expression are found in areas of the hypothalamus directly associated with food intake and energy balance. GR mRNA expression and protein were reported to be increased in male and female hypothalamus of adult PCUN sheep (29). This finding may be consistent with reports from rat studies where increased hypothalamic glucocorticoid was associated with decreased POMC mRNA expression (39, 40) and also obesity (41), whereas increased hypothalamic POMC mRNA expression following adrenalectomy in the rat led to increased hypophagic response to endotoxemia (42). Therefore, our observations of reduced locomotion and increased adiposity in adult offspring of PCUN ewes may have strong correlates in the epigenetic, mRNA and protein changes in hypothalamic GR.
The divergence in cortisol regulation between the sexes was observed at earlier postnatal ages (13) continued in the current study. Sex difference in postnatal plasma cortisol response to AVP+CRH is well reported (43–46). Similar effects of sex have been reported following the stress of tail docking lambs at 8 weeks of age (47) or other behavioral stressors (45, 48). In rats (49, 50) and humans (51) sex differences in HPAA regulation are well characterized. Sex steroids may influence this difference in HPAA regulation. For example, in the rat, acute activation of the HPAA by estrogen and inhibition by testosterone suggested as mechanisms (52). However, in human subjects a differential sex response to CRH stimulation persists during leuprolide-induced gonadal suppression (53). In our studies females were maintained in anestrus during the tests while male offspring remained uncastrated.
Strengths of this study include the longitudinal assessment from fetal life through to adulthood, with multiple fetal time-points, and the ability to manage ewe weight individually, accounting for variability in metabolism. Together with our previous studies of HPAA function in juvenile and early adult life (13), and of epigenetic modification of the glucocorticoid receptor in fetal and adult hippocampus, hypothalamus and pituitary (28, 29), these data provide a comprehensive description of maternal PCUN on the offspring HPAA. Studies in old-age sheep would have been ideal to encompass the full lifecycle but these studies already took 7 years due to breeding across years and extending them further was not viable. Similarly, additional assessments of HPAA to behavioral stress tests and investigation of immune and cardiovascular function would have been of great interest. Although the study design is relevant to the human situation, with mother and offspring kept together from conception until weaning, more mechanistic studies, such as embryo transfer (46) and/or cross fostering, are required to isolate the maternal/placental./fetal effects. From a human health perspective, the individualized nutritional management achieved a 10-15% reduction in maternal mass at mating with nutritional intake in the undernourished group approximately 80% of controls at this time (17), with no marked effect on birth size or postnatal growth (20). This is comparable with reductions in body weight that may be observed with dieting in humans and contrasts with more extreme paradigms often reported in rodent studies. The long gestation, singleton offspring and comparative fetal physiology in the ovine and human pregnancy, also are relevant for translational potential.
In conclusion, these studies further clarified the effect of PCUN on fetal HPAA development in late gestation and provide further evidence that maternal nutrition around the period of conception has important effects that last into middle-age offspring. We have shown in related studies that hypothalamic effects on central HPAA elements have remained present in 3–4-year-old sheep (29) and may be consistent with altered behavioral (38) and body composition phenotypes (20). However, the regulation of peripheral circulating cortisol concentrations in response to the corticotropic stimulation tests used, has normalized, in contrast with the findings in the same animals at earlier ages (13). A life stage-specific HPAA regulation by PCUN may represent an appropriate ‘trade off’ adaptation (54) given the central role the axis has on functional maturation in late gestation, in preparation for survival from birth and then later in life, on regulation of energy balance and fat mass. Effects of changed HPAA regulation with age on other systems, e.g., the immune, have yet to be determined within this PCUN paradigm. In addition, we confirm that changes in HPAA regulation with postnatal age interact with sex of the offspring. The summed information from our own and other studies, from late gestation to 3–4-year-old adult sheep, clearly show that related endocrine and metabolic axes may be affected differently at different times of life by PCUN and therefore isolated snap-shot observations at a specific age may not be representative of the complex biology resulting from restricted maternal nutrition during the periconceptional period.
Data availability statement
The datasets presented in this study can be found in online repositories. The names of the repository/repositories and accession number(s) can be found below: https://figshare.com/, 4148055972811865db05; https://figshare.com/, 83a677c9ee88b2989ad4.
Ethics statement
The animal study was reviewed and approved by the University of Auckland Animal Ethics Committee.
Author contributions
MO, AJ, KC, JH, and FB conceptualized the hypothesis and designed the experiment. MO and AJ performed the animal studies. KC and HP designed the molecular approaches used and performed that analysis. ET supervised the ACTH RIAs, designed the LQ tandem mass-spectroscopy approach and performed the analysis. MO and HP performed the statistical analysis. MO led the manuscript drafting and all authors contributed. All authors contributed to the article and approved the submitted version.
Funding
This long term project was funded by the HRC of NZ. The specific studies were funded by the grants listed below.
Acknowledgments
The authors would like to acknowledge funding by the Health Research Council of New Zealand, Gravida, Auckland Medical Research Foundation and the Maurice and Phyllis Paykel Trust. We also acknowledge the superb technical support of animal research staff of the Ngapouri Research Farm Laboratory, Reporoa, New Zealand.
Conflict of interest
The authors declare that the research was conducted in the absence of any commercial or financial relationships that could be construed as a potential conflict of interest.
Publisher’s note
All claims expressed in this article are solely those of the authors and do not necessarily represent those of their affiliated organizations, or those of the publisher, the editors and the reviewers. Any product that may be evaluated in this article, or claim that may be made by its manufacturer, is not guaranteed or endorsed by the publisher.
References
1. Roseboom T, de Rooij S, Painter R. The Dutch famine and its long-term consequences for adult health. Early Hum Dev (2006) 82(8):485–91. doi: 10.1016/j.earlhumdev.2006.07.001
2. Bloomfield FH, Oliver MH, Hawkins P, Holloway AC, Campbell M, Gluckman PD, et al. Periconceptional undernutrition in sheep accelerates maturation of the fetal hypothalamic-pituitary-adrenal axis in late gestation. Endocrinology (2004) 145(9):4278–85. doi: 10.1210/en.2004-0424
3. Jaquiery AL, Oliver MH, Bloomfield FH, Connor KL, Challis JR, Harding JE. Fetal exposure to excess glucocorticoid is unlikely to explain the effects of periconceptional undernutrition in sheep. J Physiol (2006) 572(Pt 1):109–18. doi: 10.1113/jphysiol.2006.105734
4. McMillen IC, Warnes KE, Adams MB, Robinson JS, Owens JA, Coulter CL. Impact of restriction of placental and fetal growth on expression of 11beta-hydroxysteroid dehydrogenase type 1 and type 2 messenger ribonucleic acid in the liver, kidney, and adrenal of the sheep fetus. Endocrinology (2000) 141(2):539–43. doi: 10.1210/endo.141.2.7338
5. Connor KL, Bloomfield FH, Oliver MH, Harding JE, Challis JR. Effect of periconceptional undernutrition in sheep on late gestation expression of mRNA and protein from genes involved in fetal adrenal steroidogenesis and placental prostaglandin production. Reprod Sci (2009) 16(6):573–83. doi: 10.1177/1933719109332827
6. Gluckman PD, Hanson MA, Beedle AS. Early life events and their consequences for later disease: A life history and evolutionary perspective. Am J Hum Biol (2007) 19(1):1–19. doi: 10.1002/ajhb.20590
7. MacLaughlin SM, McMillen IC. Impact of periconceptional undernutrition on the development of the hypothalamo-pituitary-adrenal axis: does the timing of parturition start at conception? Curr Drug Targets (2007) 8(8):880–7. doi: 10.2174/138945007781386848
8. Capra E, Toschi P, Del Corvo M, Lazzari B, Stella A, Williams JL, et al. Short communication: Maternal undernutrition during peri-conceptional period affects whole genome ovine muscle methylation in adult offspring. J Anim Sci (2022) 100:1–8. doi: 10.1093/jas/skac180
9. Toschi P, Capra E, Anzalone DA, Lazzari B, Turri F, Pizzi F, et al. Maternal peri-conceptional undernourishment perturbs offspring sperm methylome. Reproduction (2020) 159(5):513–23. doi: 10.1530/REP-19-0549
10. Zhang L, Lu Q, Chang C. Epigenetics in health and disease. Adv Exp Med Biol (2020) 1253:3–55. doi: 10.1007/978-981-15-3449-2_1
11. Stevens A, Begum G, White A. Epigenetic changes in the hypothalamic pro-opiomelanocortin gene: A mechanism linking maternal undernutrition to obesity in the offspring? Eur J Pharmacol (2011) 660(1):194–201. doi: 10.1016/j.ejphar.2010.10.111
12. Bloomfield FH, Oliver MH, Giannoulias CD, Gluckman PD, Harding JE, Challis JR. Brief undernutrition in late-gestation sheep programs the hypothalamic-pituitary-adrenal axis in adult offspring. Endocrinology (2003) 144(7):2933–40. doi: 10.1210/en.2003-0189
13. Oliver MH, Bloomfield FH, Jaquiery AL, Todd SE, Thorstensen EB, Harding JE. Periconceptional undernutrition suppresses cortisol response to arginine vasopressin and corticotropin-releasing hormone challenge in adult sheep offspring. J Dev Orig Health Dis (2012) 3(1):52–8. doi: 10.1017/S2040174411000626
14. Bauer ME, Jeckel CM, Luz C. The role of stress factors during aging of the immune system. Ann N Y Acad Sci (2009) 1153:139–52. doi: 10.1111/j.1749-6632.2008.03966.x
15. Buford TW, Willoughby DS. Impact of DHEA(S) and cortisol on immune function in aging: A brief review. Appl Physiol Nutr Metab (2008) 33(3):429–33. doi: 10.1139/H08-013
16. Oliver MH, Hawkins P, Harding JE. Periconceptional undernutrition alters growth trajectory and metabolic and endocrine responses to fasting in late-gestation fetal sheep. Pediatr Res (2005) 57(4):591–8. doi: 10.1203/01.PDR.0000155942.18096.9C
17. Rumball CW, Bloomfield FH, Oliver MH, Harding JE. Different periods of periconceptional undernutrition have different effects on growth, metabolic and endocrine status in fetal sheep. Pediatr Res (2009) 66(6):605–13. doi: 10.1203/PDR.0b013e3181bbde72
18. Wheaton JE, Windels HF, Johnston LJ. Accelerated lambing using exogenous progesterone and the ram effect. J Anim Sci (1992) 70(9):2628–35. doi: 10.2527/1992.7092628x
19. Jaquiery AL, Oliver MH, Bloomfield FH, Harding JE. Periconceptional events perturb postnatal growth regulation in sheep. Pediatr Res (2011) 70(3):261–6. doi: 10.1203/PDR.0b013e3182242deb
20. Jaquiery AL, Oliver MH, Honeyfield-Ross M, Harding JE, Bloomfield FH. Periconceptional undernutrition in sheep affects adult phenotype only in males. J Nutr Metab (2012) 2012:123610. doi: 10.1155/2012/123610
21. Jeffray TM, Matthews SG, Hammond GL, Challis JR. Divergent changes in plasma ACTH and pituitary POMC mRNA after cortisol administration to late-gestation ovine fetus. Am J Physiol (1998) 274(3 Pt 1):E417–25. doi: 10.1152/ajpendo.1998.274.3.E417
22. Rumball CW, Oliver MH, Thorstensen EB, Jaquiery AL, Husted SM, Harding JE, et al. Effects of twinning and periconceptional undernutrition on late-gestation hypothalamic-pituitary-adrenal axis function in ovine pregnancy. Endocrinology (2008) 149(3):1163–72. doi: 10.1210/en.2007-1306
23. Jaquiery AL, Park SS, Phua HH, Berry MJ, Meijler D, Harding JE, et al. Brief neonatal nutritional supplementation has sex-specific effects on glucose tolerance and insulin regulating genes in juvenile lambs. Pediatr Res (2016) 80(6):861–9. doi: 10.1038/pr.2016.168
24. Pfaffl MW, Tichopad A, Prgomet C, Neuvians TP. Determination of stable housekeeping genes, differentially regulated target genes and sample integrity: BestKeeper–excel-based tool using pair-wise correlations. Biotechnol Lett (2004) 26(6):509–15. doi: 10.1023/B:BILE.0000019559.84305.47
25. Pfaffl MW. A new mathematical model for relative quantification in real-time RT-PCR. Nucleic Acids Res (2001) 29(9):e45. doi: 10.1093/nar/29.9.e45
26. Vandesompele J, De Preter K, Pattyn F, Poppe B, Van Roy N, De Paepe A, et al. Accurate normalization of real-time quantitative RT-PCR data by geometric averaging of multiple internal control genes. Genome Biol (2002) 3(7):1–12. doi: 10.1186/gb-2002-3-7-research0034
27. Hellemans J, Mortier G, De Paepe A, Speleman F, Vandesompele J. qBase relative quantification framework and software for management and automated analysis of real-time quantitative PCR data. Genome Biol (2007) 8(2):R19. doi: 10.1186/gb-2007-8-2-r19
28. Stevens A, Begum G, Cook A, Connor K, Rumball C, Oliver M, et al. Epigenetic changes in the hypothalamic proopiomelanocortin and glucocorticoid receptor genes in the ovine fetus after periconceptional undernutrition. Endocrinology (2010) 151(8):3652–64. doi: 10.1210/en.2010-0094
29. Begum G, Davies A, Stevens A, Oliver M, Jaquiery A, Challis J, et al. Maternal undernutrition programs tissue-specific epigenetic changes in the glucocorticoid receptor in adult offspring. Endocrinology (2013) 154(12):4560–9. doi: 10.1210/en.2013-1693
30. Coulter CL, Ross JT, Owens JA, Bennett HP, McMillen IC. Role of pituitary POMC-peptides and insulin-like growth factor II in the developmental biology of the adrenal gland. Arch Physiol Biochem (2002) 110(1-2):99–105. doi: 10.1076/apab.110.1.99.894
31. Yang K, Matthews SG. Cellular localization of 11 beta-hydroxysteroid dehydrogenase 2 gene expression in the ovine adrenal gland. Mol Cell Endocrinol (1995) 111(2):R19–23. doi: 10.1016/0303-7207(95)03574-Q
32. Langley-Evans SC, Clamp AG, Grimble RF, Jackson AA. Influence of dietary fats upon systolic blood pressure in the rat. Int J Food Sci Nutr (1996) 47(5):417–25. doi: 10.3109/09637489609006955
33. Lesage J, Blondeau B, Grino M, Breant B, Dupouy JP. Maternal undernutrition during late gestation induces fetal overexposure to glucocorticoids and intrauterine growth retardation, and disturbs the hypothalamo-pituitary adrenal axis in the newborn rat. Endocrinology (2001) 142(5):1692–702. doi: 10.1210/endo.142.5.8139
34. Sloboda DM, Moss TJ, Li S, Doherty D, Nitsos I, Challis JR, et al. Prenatal betamethasone exposure results in pituitary-adrenal hyporesponsiveness in adult sheep. Am J Physiol Endocrinol Metab (2007) 292(1):E61–70. doi: 10.1152/ajpendo.00270.2006
35. Rosewicz S, McDonald AR, Maddux BA, Goldfine ID, Miesfeld RL, Logsdon CD. Mechanism of glucocorticoid receptor down-regulation by glucocorticoids. J Biol Chem (1988) 263(6):2581–4. doi: 10.1016/S0021-9258(18)69104-5
36. Chao HM, Ma LY, McEwen BS, Sakai RR. Regulation of glucocorticoid receptor and mineralocorticoid receptor messenger ribonucleic acids by selective agonists in the rat hippocampus. Endocrinology (1998) 139(4):1810–4. doi: 10.1210/endo.139.4.5896
37. Van Cauter E, Leproult R, Kupfer DJ. Effects of gender and age on the levels and circadian rhythmicity of plasma cortisol. J Clin Endocrinol Metab (1996) 81(7):2468–73. doi: 10.1210/jcem.81.7.8675562
38. Donovan EL, Hernandez CE, Matthews LR, Oliver MH, Jaquiery AL, Bloomfield FH, et al. Periconceptional undernutrition in sheep leads to decreased locomotor activity in a natural environment. J Dev Orig Health Dis (2013) 4(4):296–9. doi: 10.1017/S2040174413000214
39. Beaulieu S, Gagne B, Barden N. Glucocorticoid regulation of proopiomelanocortin messenger ribonucleic acid content of rat hypothalamus. Mol Endocrinol (1988) 2(8):727–31. doi: 10.1210/mend-2-8-727
40. Wardlaw SL, McCarthy KC, Conwell IM. Glucocorticoid regulation of hypothalamic proopiomelanocortin. Neuroendocrinology (1998) 67(1):51–7. doi: 10.1159/000054298
41. Zakrzewska KE, Cusin I, Stricker-Krongrad A, Boss O, Ricquier D, Jeanrenaud B, et al. Induction of obesity and hyperleptinemia by central glucocorticoid infusion in the rat. Diabetes (1999) 48(2):365–70. doi: 10.2337/diabetes.48.2.365
42. Rorato R, Castro M, Borges BC, Benedetti M, Germano CM, Antunes-Rodrigues J, et al. Adrenalectomy enhances endotoxemia-induced hypophagia: Higher activation of corticotrophin-releasing-factor and proopiomelanocortin hypothalamic neurons. Horm Behav (2008) 54(1):134–42. doi: 10.1016/j.yhbeh.2008.02.011
43. Gardner DS, Van Bon BW, Dandrea J, Goddard PJ, May SF, Wilson V, et al. Effect of periconceptional undernutrition and gender on hypothalamic-pituitary-adrenal axis function in young adult sheep. J Endocrinol (2006) 190(2):203–12. doi: 10.1677/joe.1.06751
44. Chadio SE, Kotsampasi B, Papadomichelakis G, Deligeorgis S, Kalogiannis D, Menegatos I, et al. Impact of maternal undernutrition on the hypothalamic-pituitary-adrenal axis responsiveness in sheep at different ages postnatal. J Endocrinol (2007) 192(3):495–503. doi: 10.1677/JOE-06-0172
45. Poore KR, Boullin JP, Cleal JK, Newman JP, Noakes DE, Hanson MA, et al. Sex- and age-specific effects of nutrition in early gestation and early postnatal life on hypothalamo-pituitary-adrenal axis and sympathoadrenal function in adult sheep. J Physiol (2010) 588(Pt 12):2219–37. doi: 10.1113/jphysiol.2010.187682
46. Wallace JM, Milne JS, Green LR, Aitken RP. Postnatal hypothalamic-pituitary-adrenal function in sheep is influenced by age and sex, but not by prenatal growth restriction. Reprod Fertil Dev (2011) 23(2):275–84. doi: 10.1071/RD10103
47. Turner AI, Hosking BJ, Parr RA, Tilbrook AJ. A sex difference in the cortisol response to tail docking and ACTH develops between 1 and 8 weeks of age in lambs. J Endocrinol (2006) 188(3):443–9. doi: 10.1677/joe.1.06328
48. Hernandez CE, Matthews LR, Oliver MH, Bloomfield FH, Harding JE. Effects of sex, litter size and periconceptional ewe nutrition on offspring behavioural and physiological response to isolation. Physiol Behav (2010) 101(5):588–94. doi: 10.1016/j.physbeh.2010.08.020
49. Kitay JI. Sex differences in adrenal cortical secretion in the rat. Endocrinology (1961) 68:818–24. doi: 10.1210/endo-68-5-818
50. Rivier C. Gender, sex steroids, corticotropin-releasing factor, nitric oxide, and the HPA response to stress. Pharmacol Biochem Behav (1999) 64(4):739–51. doi: 10.1016/S0091-3057(99)00148-3
51. Uhart M, Chong RY, Oswald L, Lin PI, Wand GS. Gender differences in hypothalamic-pituitary-adrenal (HPA) axis reactivity. Psychoneuroendocrinology (2006) 31(5):642–52. doi: 10.1016/j.psyneuen.2006.02.003
52. Viau V, Bingham B, Davis J, Lee P, Wong M. Gender and puberty interact on the stress-induced activation of parvocellular neurosecretory neurons and corticotropin-releasing hormone messenger ribonucleic acid expression in the rat. Endocrinology (2005) 146(1):137–46. doi: 10.1210/en.2004-0846
53. Roca CA, Schmidt PJ, Deuster PA, Danaceau MA, Altemus M, Putnam K, et al. Sex-related differences in stimulated hypothalamic-pituitary-adrenal axis during induced gonadal suppression. J Clin Endocrinol Metab (2005) 90(7):4224–31. doi: 10.1210/jc.2004-2525
Keywords: programming, long-term, outcomes, adrenal, cortisol
Citation: Oliver MH, Jaquiery AL, Connor KL, Phua HH, Harding JE, Thorstensen EB and Bloomfield FH (2023) Effect of maternal periconceptional undernutrition in sheep on cortisol regulation in offspring from mid-late gestation, through to adulthood. Front. Endocrinol. 14:1122432. doi: 10.3389/fendo.2023.1122432
Received: 12 December 2022; Accepted: 20 January 2023;
Published: 02 February 2023.
Edited by:
Takahiro Nemoto, Nippon Medical School, JapanCopyright © 2023 Oliver, Jaquiery, Connor, Phua, Harding, Thorstensen and Bloomfield. This is an open-access article distributed under the terms of the Creative Commons Attribution License (CC BY). The use, distribution or reproduction in other forums is permitted, provided the original author(s) and the copyright owner(s) are credited and that the original publication in this journal is cited, in accordance with accepted academic practice. No use, distribution or reproduction is permitted which does not comply with these terms.
*Correspondence: Mark H. Oliver, bS5vbGl2ZXJAYXVja2xhbmQuYWMubno=