- 1Transplant Research Center, Shiraz University of Medical Sciences, Shiraz, Iran
- 2Cardiovascular research center, Shiraz University of Medical Sciences, Shiraz, Iran
- 3Gastroenterohepatology Research Center, Shiraz University of Medical Sciences, Shiraz, Iran
Background: Environmental pollution and infertility are two modern global challenges that agonize personal and public health. The causal relationship between these two deserves scientific efforts to intervene. It is believed that melatonin maintains antioxidant properties and may be utilized to protect the testicular tissue from oxidant effects caused by toxic materials.
Methods: A systematic literature search was conducted in PubMed, Scopus, and Web of Science to identify the animal trial studies that evaluated melatonin therapy’s effects on rodents’ testicular tissue against oxidative stress caused by heavy metal and non-heavy metal environmental pollutants. Data were pooled, and standardized mean difference and 95% confidence intervals were estimated using the random-effect model. Also, the risk of bias was assessed using the Systematic Review Centre for Laboratory animal Experimentation (SYRCLE) tool. (PROSPERO: CRD42022369872)
Results: Out of 10039 records, 38 studies were eligible for the review, of which 31 were included in the meta-analysis. Most of them showed beneficial effects of melatonin therapy on testicular tissue histopathology. [20 toxic materials were evaluated in this review, including arsenic, lead, hexavalent chromium, cadmium, potassium dichromate, sodium fluoride, cigarette smoke, formaldehyde, carbon tetrachloride (CCl4), 2-Bromopropane, bisphenol A, thioacetamide, bisphenol S, ochratoxin A, nicotine, diazinon, Bis(2-ethylhexyl) phthalate (DEHP), Chlorpyrifos (CPF), nonylphenol, and acetamiprid.] The pooled results showed that melatonin therapy increased sperm count, motility, viability and body and testicular weights, germinal epithelial height, Johnsen's biopsy score, epididymis weight, seminiferous tubular diameter, serum testosterone, and luteinizing hormone levels, testicular tissue Malondialdehyde, glutathione peroxidase, superoxide dismutase, and glutathione levels. On the other hand, abnormal sperm morphology, apoptotic index, and testicular tissue nitric oxide were lower in the melatonin therapy arms. The included studies presented a high risk of bias in most SYRCLE domains.
Conclusion: In conclusion, our study demonstrated amelioration of testicular histopathological characteristics, reproductive hormonal panel, and tissue markers of oxidative stress. Melatonin deserves scientific attention as a potential therapeutic agent for male infertility.
Systematic review registration: https://www.crd.york.ac.uk/PROSPERO, identifier CRD42022369872.
1 Introduction
Infertility is a universal public health issue with a dramatically increasing prevalence in recent decades (1). About 48 million couples worldwide suffer from fecundity problems (2), of which half have been implicated by male factors (3). Male infertility may result from various factors such as genetic, epigenetic, physical injuries, drugs, and environmental pollutants. Among these, pesticides, plasticizers, refrigerants, dry cleaners, and heavy metals are of great interest as they are widely used in industry. Environmental pollutants impair the function of male fecundity by altering hormonal, molecular, and histological characteristics. These pollutants mostly affect biomechanics through oxidative stress and increasing free radicals causing a shift in the equilibrium between the production of free-radical species and the antioxidant defense system in male reproductive cells (4, 5).
Reactive oxygen species (ROS) are highly reactive molecules generated by cellular metabolism. A physiological level of ROS is essential for the proper development of spermatozoa, including their production, maturation, morphological reshaping, and fertilization process (6). Redox reactions serve as cofactors for the spermatozoa maturation, free radicals also stimulate intracellular pathways resulting acrosome reaction, capacitation, motility, and condensation of chromatin (7–9). ROS concentration increases in excessive exposure to environmental pollutants (10); This excessive ROS overwhelms the cellular antioxidant defense system and accelerates oxidative stress (11). The elevated ROS attacks multiple cellular macromolecules leading to DNA damage, lipid peroxidation, and protein misfolding, resulting in mitochondrial dysfunction and sperms’ structural integrity impairment (12–16). The damage worsens by changes in the apoptotic index, cell vacuolization, and diminished capacity to proliferate, followed by a reduction in sperm viability and count (17, 18). Among the different cell types, spermatozoa are highly vulnerable to oxidation due to the abundance of unsaturated fatty acids in the membrane, lack of proper DNA repair mechanisms, and the absence of cytoplasmic antioxidant enzymes, with concomitant negative consequences on sperm quality (19–23).
Antioxidants have gained attention for their role in infertility (24–27). So far, various supplements with antioxidant capabilities, such as ginger, vitamin C, and vitamin E, have been shown to improve hormonal and histological parameters related to male reproduction (28–30). Melatonin also has exhibited potent protective activities against oxidative stress-induced testicular cell damage (31). Melatonin synthetic enzymes and melatonin membrane receptors are identified in testicular cells, indicating the importance of the melatonergic system in male reproduction. It also directly affects testosterone production from Leydig cells (32). Melatonin has recently gained scientific interest due to its protection against oxidative stress-induced testicular cell damage (33, 34). It protects testicular cells from elevated ROS through anti-apoptotic and antioxidant activities (34).
Despite the wealth of evidence, consensus and structured gathering of evidence are still needed. This systematic review and meta-analysis aims to congregate the evidence on melatonin as a protective agent against rodential testicular damages caused by environmental pollutants and toxic materials.
2 Material and methods
This systematic review of relevant studies was conducted according to the Preferred Reporting Items for Systematic Reviews and Meta-analyses guideline (PRISMA). The protocol is registered in the International Prospective Register of Systematic Reviews (PROSPERO: CRD42022369872). We systematically searched PubMed, Scopus, and Web of Science from January 1, 1970, until September 9, 2022, for “melatonin” and “reproductive indices” related terms (Supplementary Material 1). Also, we manually searched the reference list of the included papers for additional citations of interest.
2.1 Study selection and eligibility criteria
Firstly, duplicate records were removed automatically. All the records were uploaded to the Rayyan online tool for managing systematic reviews. Three reviewers (NDE, AS, and MAS) screened the records by title and abstract. Then, records were screened for eligibility criteria. Discrepancies were resolved with discussion. Studies were included if they fulfilled the following criteria (1): controlled animal studies (2), the subjects were rodents that were exposed to toxic environmental materials such as environmental pollutants and heavy metals to induce oxidative stress (3), at least one intervention group received melatonin regimen (4), at least one control group with similar oxidative stress that did not receive melatonin (with or without placebo), and (5) the study reported major hallmarks of testicular tissue (histopathologic, biochemical, and sperm analyses). We excluded the studies if they (1): designed as in-vitro and ex-vivo, (2) employed non-rodent animals, (3) studied other types of stressors such as physical, ischemic, heat, radiation, chemotherapy, and metabolic agents, (4) melatonin was administered in combination with other drugs or the study employed melatonin derivatives, (5) only evaluated healthy controls without oxidative stress, and (6) they failed to report favorable outcomes. Also, we excluded reviews, letters, and human trials.
2.2 Data extraction and assessment of risk of bias
Two reviewers (FN and NDE) independently extracted the data into Excel spreadsheets, and three reviewers (AS, MAS, and AM) rechecked the data for any mistakes. The following data were extracted from each study: (1) study characteristics (first author, publication year, and country), (2) population characteristics (species, age, and sample size), (3) toxic material, dose, administration route, and duration of exposure, (4) melatonin dose, duration, route, and setting of administration (before, simultaneous, or after oxidative stress), (5) sperm characteristics (count, motility, viability, and abnormal morphology), testicular parameters (height of germinal epithelium, Johnsen’s testicular biopsy score (JTBS), seminiferous tubular and luminal diameter, and apoptotic index), hormonal panel (serum testosterone, Follicle-Stimulating Hormone (FSH), Luteinizing Hormone (LH)), markers of oxidative stress (testicular tissue Superoxide dismutase (SOD), Catalase (CAT) activity, Malondialdehyde (MDA), glutathione peroxidase (GPx), glutathione (GSH), and nitric oxide (NO), and somatic characteristics (testis to body relative weight, total testis weight, epididymis weight, body weight, and body weight gain).
Two reviewers (NDE and AS) independently assessed the risk of bias using the Systematic Review Centre for Laboratory Animal Experimentation (SYRCLE) tool for animal intervention studies.
2.3 Data synthesis and statistical analyses
Meta-analysis was run via Stata 13 (College Station, TX, USA) using the DerSimonian-Laird random effect model. Standardized mean difference (SMD) was considered as the effect size for comparing the mean difference of variables between the control and intervention groups. The amount of heterogeneity in the studies was indicated by I-squared. Subgroup analyses were done where at least two studies were available in each subgroup to investigate the differences between heavy metal and non-heavy metal pollutants. Also, the forest plot was provided for each study, and pooled data publication bias was assessed by Egger’s test. In addition, sensitivity analysis was done to check for the robustness of our results.
3 Results
3.1 Search results
The PRISMA flow diagram of the literature search is presented in Figure 1. The systematic search resulted in 10,039 records while manual citation searching yielded 7 additional studies. The database searching included PubMed (n=1,375), Web of Science (n=3,838), and Scopus (n=4,826). 1,016 records were removed using automatic duplicate detection. Title and abstract screening was conducted on 9,023 records and 98 studies was sought for retrieval. With exclusion of two studies, which the full-texts were not found and our effort to communicate with the authors failed, 103 articles were assessed for eligibility. A total of 65 articles were excluded due to ineligible population (n=45), design (n=6), intervention (n=2), outcome (n=8), and publication type (n=4). Finally, a total of 38 articles were eligible for the study; among them, 7 (35–41) were only included in the narrative data synthesis.
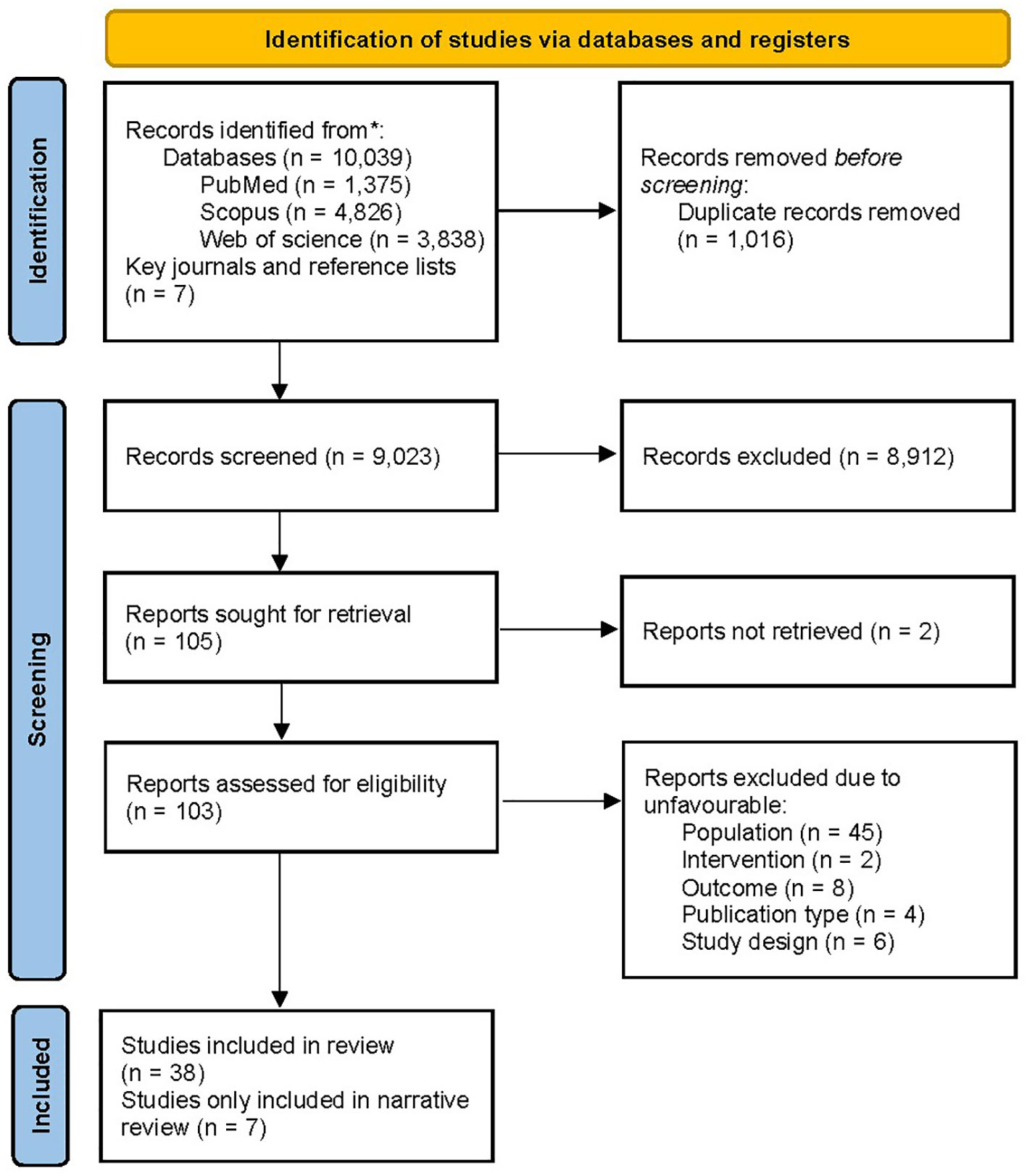
Figure 1 PRISMA flow chart of the literature screening. PRISMA, Preferred Reporting Items for Systematic Reviews and Meta-Analyses.
3.2 Study characteristics
Studies were published between 2007 and 2022 and all were published in English except one which was in Persian (42). Mice, rats, and hamsters were the subjects of 13 (35–38, 43–51), 24 (17, 39–42, 52–70), and one (71) studies, respectively. Studies have utilized heavy metals (n= 12) including arsenic (37, 45, 67), lead (58), cadmium (42, 47, 48, 55, 68, 69), Hexavalent chromium (38), and Potassium dichromate (52) and toxic materials (n= 26) including sodium fluoride (NaF) (61), 2-Bromopropane (54), Bisphenol A (17, 43, 46, 59, 62, 63, 70), thioacetamide (56), Bisphenol S (71), ochratoxin A (57), nicotine (49), Cigarette smoke (39), diazinon (35, 36, 50), formaldehyde (40, 60), bis(2-ethylhexyl) phthalate (DEHP) (44), carbon tetrachloride (CCl4) (41, 53), Chlorpyrifos (65, 66), nonylphenol (64), and acetamiprid (51) to induce oxidative stress. To administer the stressors, oral (n= 19) (37, 44–46, 51, 52, 57–59, 63–72), intraperitoneal (n= 14, IP) (35, 36, 38, 40–42, 47–50, 54, 56, 60, 62), and subcutaneous (n= 2, SQ) (55, 63) routes were used. To administer melatonin, oral (n= 9) (37, 45, 46, 57, 58, 63, 65, 66, 68), IP (n= 24) (35, 36, 38–42, 44, 47–52, 54, 56, 59, 60, 62, 64, 67, 70–72), and SQ (n= 2) (55, 63) routes were used. Melatonin was administered prior to (n= 18, preventive) (17, 35, 36, 41, 46–48, 50, 52, 54, 56, 62, 64–66, 70–72), simultaneously (n= 6) (38, 40, 42, 45, 59, 68), and after (n= 12, therapeutic) (37, 39, 43, 49, 53, 56–58, 60, 63, 67, 69) the start of stressor. The characteristics of the included studies are summarized in the Tables 1, 2.
3.3 Sperm and somatic characteristics
Sperm characteristics were reported in the included studies as abnormal morphology, count, motility, and viability. Melatonin therapy significantly improved all these parameters: abnormal morphology (SMD -3.59 with 95% CI -4.60, -2.59), count (SMD 3.56 with 95% CI 2.7, 4.42), motility (SMD 2.92 with 95% CI 2.16, 3.69), and viability (SMD 5.99 with 95% CI 4.19, 7.78), all with p-values <0.001.
Between-study heterogeneity was substantial for all these outcomes with I-squared ranging between 81% and 88% and p-values <0.001 for all outcomes. Also, Egger’s test showed statistically significant publication bias in all the outcomes with p-values <0.001. Forest plots of analyses for sperm parameter outcomes are presented in Figure 2.
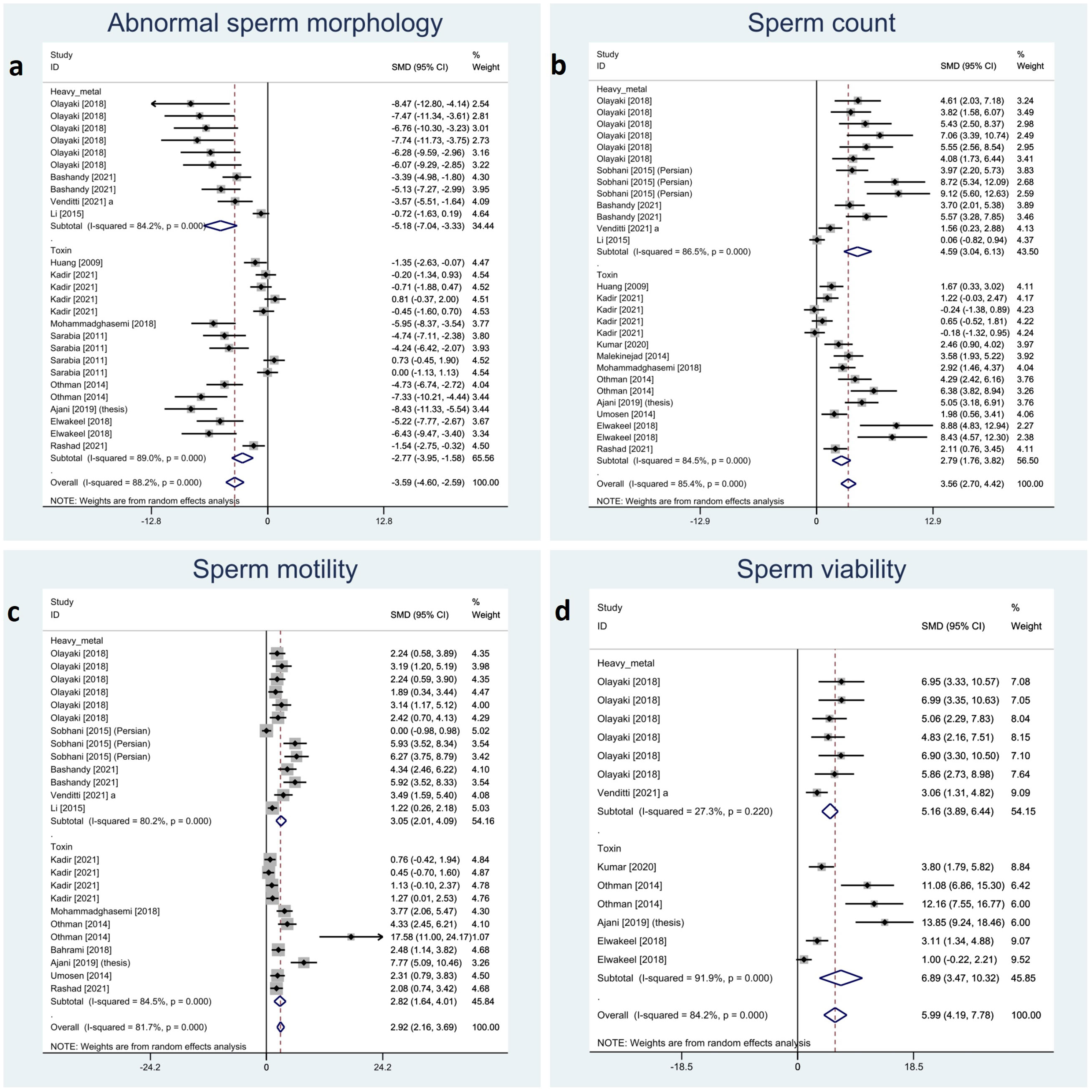
Figure 2 Forest plots for overall and subgroup effect measures on the impact of melatonin therapy on sperm characteristics including (A) abnormal sperm morphology, (B) count, (C) motility, and (D) viability.
We categorized relative testis to body, total testicular, and body weight and body weight gain as somatic indices. The meta-analyses showed a significant impact of melatonin therapy on total testicular and body weight (SMD 1.15 with 95%CI 0.56, 1.73 and p-value <0.001 and SMD 1.11 with 95%CI 0.42, 1.80 and p-value 0.002, respectively). Although, testis to body relative weight and body weight gain were not significantly affected by melatonin therapy (SMD 0.85 with 95%CI -0.05, 1.74 and p-value 0.064 and SMD -0.18 with 95%CI -1.62, 1.25 and p-value 0.803, respectively). Investigation of between-study variation revealed substantial heterogeneity with I-squared ranging between 72% and 83% (p-values <0.001 and 0.002). Assessment of publication bias was not feasible for body weight gain due to low sample size. Egger’s test showed statistically significant publication bias in testis to body relative weight and total testicular weight (p-value 0.003 and 0.012, respectively). Forest plots and detailed results of Egger’s test for sperm parameter outcomes is presented in Figure 3 and Supplementary Material 2.
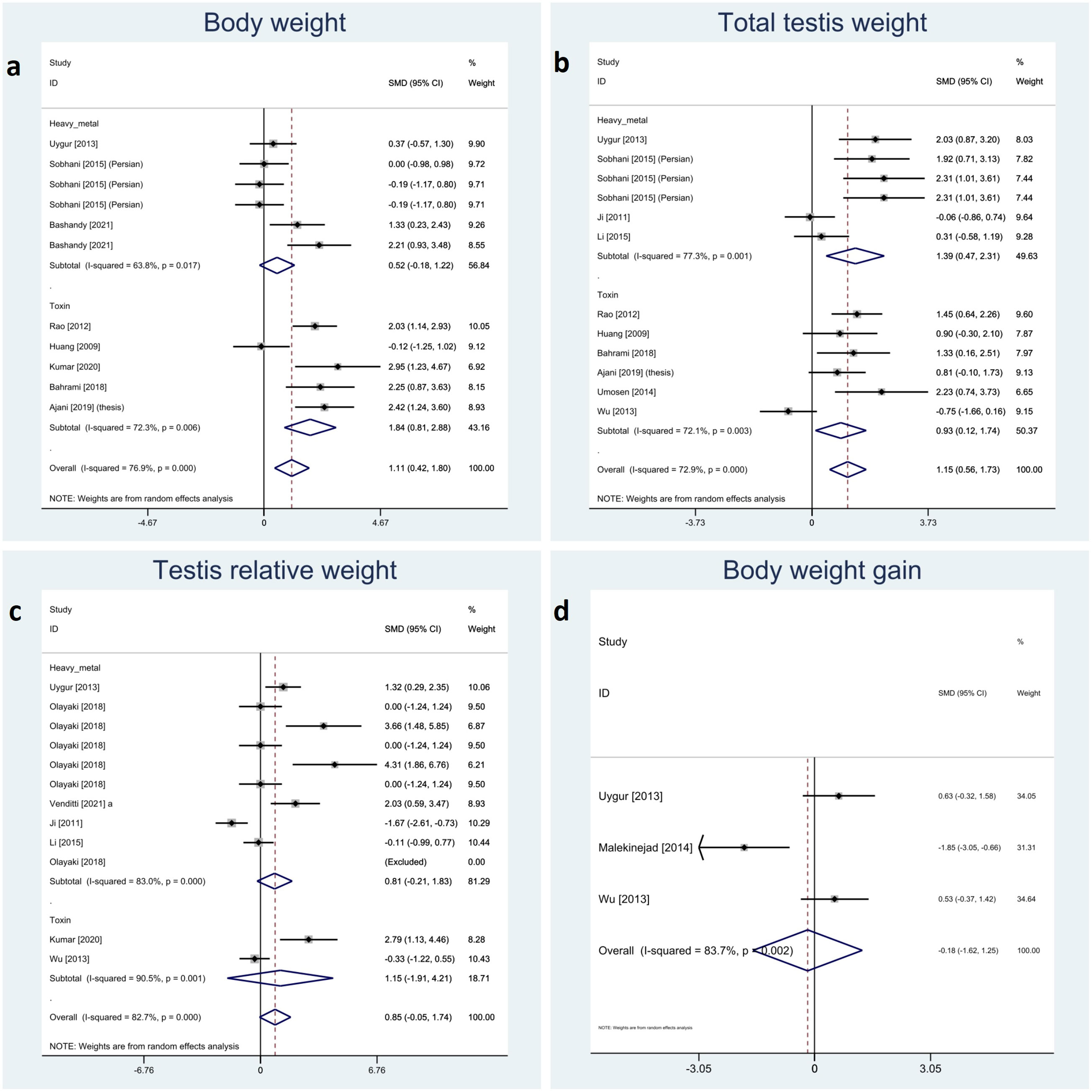
Figure 3 Forest plots for overall and subgroup effect measures on the impact of melatonin therapy on the (A) body, (B) total and (C) relative testicular weights and (D) body weight gain.
3.4 Testicular tissue parameters
Testicular parameters were reported in the included studies as height of germinal epithelium, JTBS, tubular diameter, luminal diameter, epididymis weight, and apoptotic index. Meta-analyses on these variables showed that melatonin therapy significantly increased height in germinal epithelium (SMD 3.63 with 95% CI 2.05, 5.21 and p-value <0.001), JTBS (SMD 4.13 with 95% CI 1.44, 6.81 and p-value <0.001), tubular diameter (SMD 2.44 with 95% CI 1.41, 3.47 and p-value <0.001), and epididymis weight (SMD 1.03 with 95% CI.014, 1.93 and p-value 0.024) and decreased apoptotic index (SMD -4.07 with 95% CI -7.23, -0.91 and p-value 0.012). Although not statistically significant, melatonin therapy increased luminal diameter (SMD 0.45 with 95% CI -0.90, 1.79 and p-value 0.515).
Between-study heterogeneity was considerable for all these outcomes with I-squared ranging between 76% and 91% and p-values <0.001 for all outcomes. Egger’s test showed statistically significant publication bias in all the outcomes with p-values <0.001 for tubular diameter, and epithelial height and 0.011, 0.026 and 0.008 for JTBS, luminal diameter, and epididymis, respectively. Forest plots and detailed results of Egger’s test for sperm parameter outcomes are presented in Figure 4 and Supplementary Material 2.
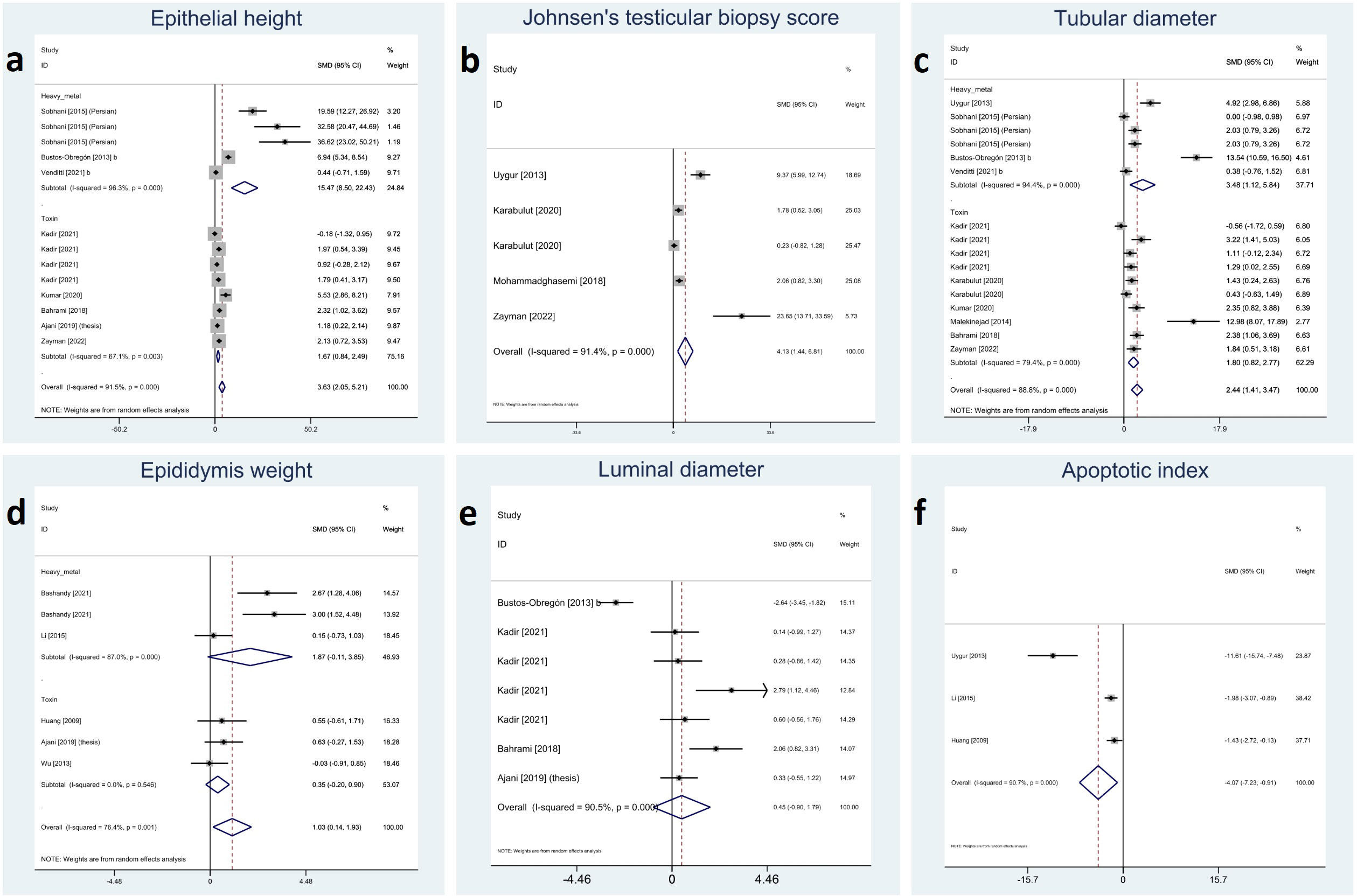
Figure 4 Forest plots for overall and subgroup effect measures on the impact of melatonin therapy on the parameters of testicular tissue including (A) epithelial height, (B) Johnsen’s biopsy score, (C) seminiferous tubular diameter, (D) epididymis weight, (E) seminiferous luminal diameter, and (F) apoptotic index.
3.5 Reproductive hormones
Included studies reported serum FSH, LH, and testosterone; among them, melatonin therapy increased serum LH and testosterone significantly (SMD 1.61 with 95% CI 0.59, 2.63 and p-value 0.002 and SMD 1.87 with 95% CI 1.14, 2.60 and p-value <0.001, respectively). On the other hand, changes in serum FSH were not statistically significant (SMD 0.55 with 95% CI -0.49, 1.60 and p-value 0.299). Between-study heterogeneity was substantial or considerable for reproductive hormones I-squared ranging between 85% and 88% with p-value <0.001. Egger’s test showed significant publication bias for serum LH with p-value <0.001. Forest plots and detailed results of Egger’s test for reproductive hormones outcomes are presented in Figure 5 and Supplementary Material 2.
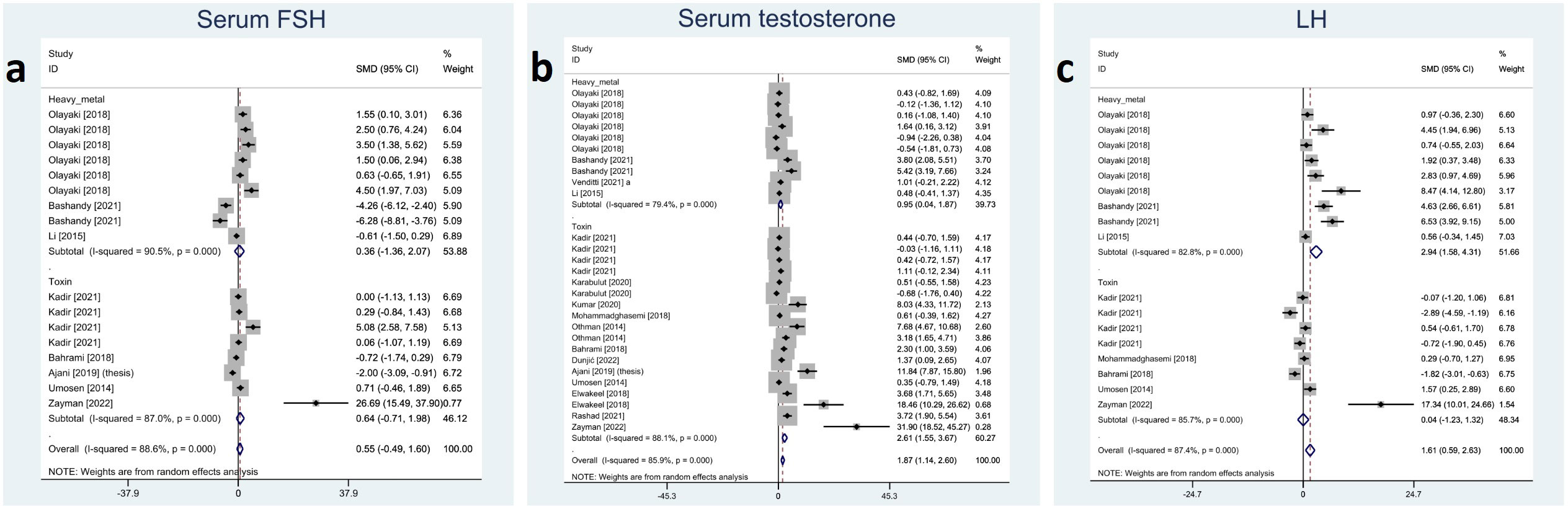
Figure 5 Forest plots for overall and subgroup effect measures on the impact of melatonin therapy on the serum level of reproductive hormones including (A) FSH, (B) testosterone, and (C) LH. FSH, Follicle-Stimulating Hormone; LH, Luteinizing Hormone.
3.6 Oxidative markers
All the reported oxidative markers showed significant changes with melatonin therapy: testicular tissue CAT (SMD 2.34 with 95%CI 1.51, 3.17), GSH (SMD 2.82 with 95%CI 1.46, 4.18), GPx (SMD 1.26 with 95%CI 0.51, 2.02), MDA (SMD -4.83 with 95%CI -6.05, -3.61), SOD (SMD 1.62 with 95%CI 0.81, 2.44), and NO (SMD -1.93 with 95%CI -2.97, -0.90) with all p-values <0.001. Between-study heterogeneity was substantial to considerable I-squared ranging between 60% to 90% with all p-values <0.001 except for NO (p-value 0.054). Using Egger’s regression model, all these outcomes suffered from publication bias (p-values <0.001) except GPx (p-value 0.992). Forest plots and detailed results of Egger’s test for sperm parameter outcomes is presented in Figure 6 Supplementary Material 2.
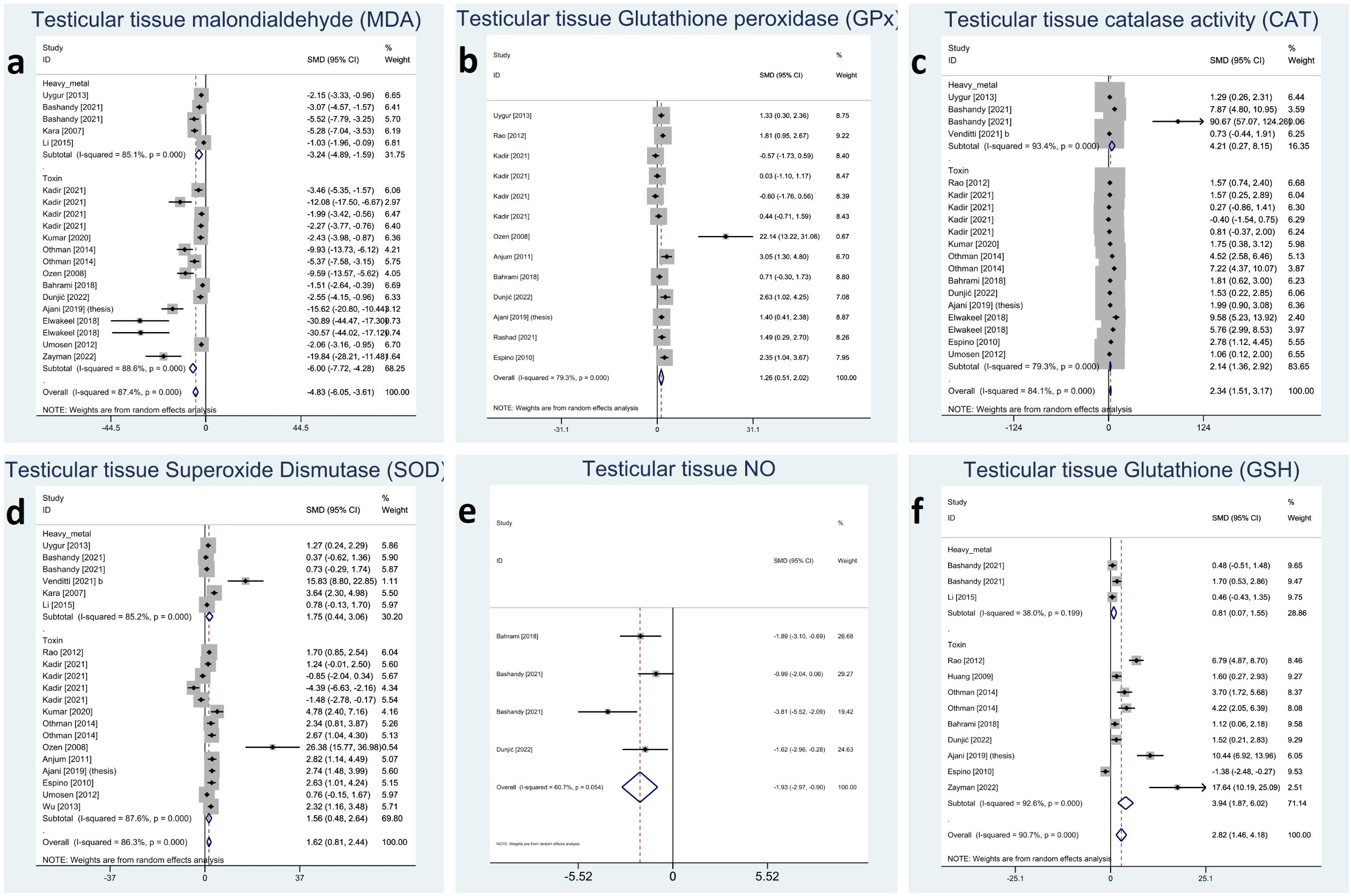
Figure 6 Forest plots for overall and subgroup effect measures on the impact of melatonin therapy on the testicular tissue level of oxidative stress markers including (A) MDA, (B) GPx, (C) CAT, (D) SOD, (E) NO, (F) GSH. MDA, Malondialdehyde; GPx, Glutathione Peroxidase; CAT, Catalase; SOD, Superoxide Dismutase; NO, Nitric Oxide; GSH, Glutathione.
3.7 Sensitivity analyses and risk of bias assessment
Sensitivity analyses were done with omitting one study each time to investigate robustness of our results. The leave-one-out plots are provided in the Supplementary Materials 3. After removing studies from the analyses individually, none substantially affected the pooled SMD estimates in the study.
For each domain, studies scored 1 if they were assessed as low risk. Studies scored between 2 and 4 for risk of bias assessment by SYRCKLE checklist. All the studies were labeled as unclear risk on random sequence generation, allocation concealment, random housing, blinding of investigators and outcome assessors, and random outcome assessment. For other sources of bias, all the studies were assessed as low risk. 21, 12, and 37 studies were labeled as low risk on baseline characteristics, incomplete outcome data, and selective outcome reporting, respectively. All the details are presented in the Figure 7 and Supplementary Material 4.
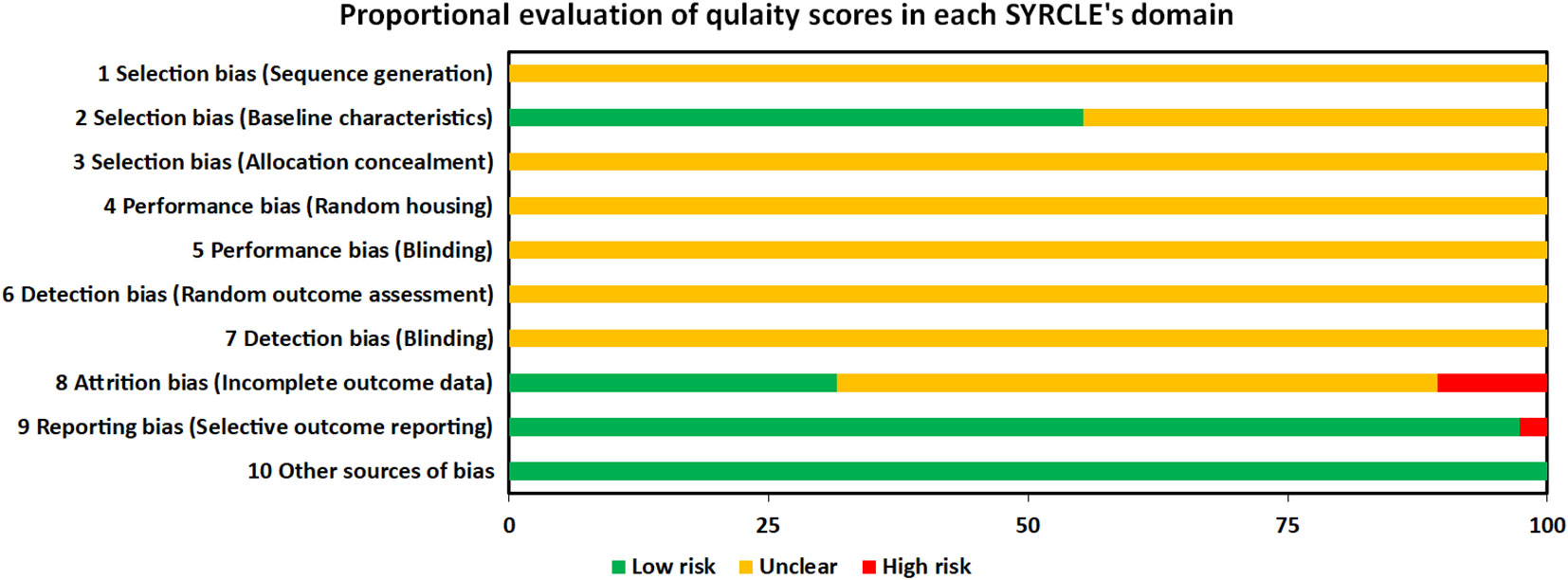
Figure 7 Proportional evaluation of quality score in each SYRCLE’s domain. SYRCLE, Systematic Review Centre for Laboratory Animal Experimentation.
3.8 Subgroup analyses
To investigate between study heterogeneity, subgroup analyses were done by categorizing the stressors as heavy metals and non-heavy metals. Abnormal sperm morphology, body and epididymis weight, epithelial height, serum LH, FSH, testosterone, sperm count, motility, viability, CAT, GSH, GPx, SOD, MDA, total and relative testicular weights, and tubular diameter were eligible for subgroup analysis. There was no significant difference between the protective effect of melatonin therapy against heavy metals and other toxins (non-heavy metals). Nonetheless, this method failed to reduce the heterogeneity within subgroups. Whenever feasible, subgroup analyses are demonstrated in the Figures 1-6.
4 Discussion
To the best of our knowledge, this is the first systematic review and meta-analysis examining how melatonin intake protects rodents’ male reproductive system in exposure to environmental pollutants. Environmental toxins, as potent oxidative stressors, damage male infertility by causing an imbalance between the cells’ free radical levels and the antioxidant defensive system. The following sections have gone through melatonin’s potential effects and related mechanisms to investigate its protective role on the male reproductive system.
4.1 Hormone parameters
The testosterone hormone mainly controls the spermatogenesis process in Sertoli cells, and LH regulates testosterone synthesis in Leydig cells (74, 75). As it is revealed by our data, melatonin intake increases serum testosterone and LH levels in male rodents injured by toxic components. These findings can be interpreted by antioxidants’ effects on reproductive hormones previously reported in reviews by Vecchio et al. and Banihani (28, 76).
Spermatogenesis, a process carried out in Sertoli cells in the testes, is mainly under testosterone control (74). Testosterone is synthesized in Leydig cells and is regulated by LH (75). Environmental pollutants may exert their effect as an endocrine disruption chemical in addition to their anti-oxidant effect (77). Some of the substances included in our study, such as Bisphenol A, Arsenic, and Cadmium, have an endocrine-disrupting effect (77, 78). Arsenic, for example, may interfere with gonadotropins’ function by suppressing their release and decreasing the transcription of androgen receptors, besides arsenic especially affects testosterone by decreasing its synthesis (79–81). Moreover, environmental pollutants by the accumulation of ROS could be accompanied by an over generation of reactive nitrogen species such as NO (82). High levels of ROS and NO generation in the testicles decrease the expression of biosynthetic enzymes, i.e., suppressing the steroidogenic acute regulatory protein (StAR) and cytochrome P450 side chain cleavage in Leydig cells (83). These cause a decrease in testosterone secretion, which is the primary hormone needed for optimal spermatogenesis (84).
Although it remains controversial, melatonin’s effect is likely to be reducing on serum testosterone levels in preclinical studies (32, 85–90). Melatonin acts directly on Leydig cells to reduce steroidogenesis and spermatogenic activity in the testes (86, 91). In our study, melatonin showed protective properties and relatively prevented the toxic effects of stressors on rodents’ serum testosterone levels in treatment arms. This effect can be explained by the protective effect of melatonin on Leydig cells against oxidative stress, increased NO, and pro-inflammatory factors (92). However, conducting more meticulous investigations in this regard is needed.
4.2 Oxidative stress parameters
Antioxidant defense system plays a crucial role in cells responding to environmental stresses (93). Numerous antioxidant responses are involved in antioxidant mechanisms. These responses include both non-enzymatic molecules (such as GSH) and enzymes (such as CAT, SOD, and GPx) (94). This system defends tissues and cells by scavenging free radicals against oxidative stress-related harm (72); however, it is not completely immune to free radicals (65).
As suggested by the results of this analysis, melatonin has been demonstrated to be generally essential in buffering oxidative stress. Regarding the effect of melatonin on MDA, GSH, and GPx levels, these results agree with recent meta-analyses conducted by Morvaridzade et al. and Sumsuzzman et al. (94, 95).
Environmental hazard components activate oxidative stress in testicular cells, causing damage to macromolecules involving membranes’ lipids. The testicular tissue MDA and NO levels increase by lipid peroxidation and endothelial damage, respectively. These environmental stressors also harm the pathways essential to GSH, CAT, and SOD synthesis as members of the antioxidant defense system. These changes in oxidative markers can be justified by ROS activity. ROS directly damages the macromolecules necessary for antioxidant production and overwhelms its capacity.
Melatonin plays its role by eliminating free and lipid peroxyl radicals before they act to damage macromolecules and membrane lipids (96, 97). Furthermore, it can improve CAT, GPx, SOD, and GSH expression and activity, possibly by interacting with nuclear or membrane receptors (98). Moreover, melatonin works complementary with CAT and GPx to keep the steady-state levels of intracellular H2O2, a more destructive form of free radicals with a longer half-life (96).
There are inconsistencies between our results and Sumsuzzman et al. reports regarding CAT and SOD levels (95), which are probably due to the varying types and numbers of melatonin receptors, bioavailability and concentration in different tissues, and the insufficient number of studies to support the results. Nevertheless, this concept remains controversial.
4.3 Sperm and somatic parameters
The results of this meta-analysis shows that melatonin significantly improves sperm parameters, including sperm count, viability, motility, and morphology. These findings align with the previous reviews regarding the ameliorating effects of antioxidants on semen qualities (28). Likewise, a systematic review by Wang et al. revealed that antioxidant treatment after varicocelectomy could significantly enhance the quality of sperm parameters (29).
In addition, findings from this systematic review and meta-analysis confirm that melatonin intake makes a marked enhancement in testicle tissue parameters, including histo-architecture, seminiferous tubular diameter, epithelial height, epididymis and total testis weight, and JTBS. In this regard, our data align with another systematic review by Tatar et al. showing the protective role of antioxidants on the weights of testes and epididymis (30).
Normal spermatogenesis is a specific determinant of semen quality (99). Toxic pollutants affect spermatogenesis by diminishing the cellular ability to proliferate and altering the apoptotic index (100). Elevated apoptosis causes a decrease in cell viability and count. Also, pollutants affect sperm motility by disturbing the function of proteins serving sperm movement as well as deterioration of the mitochondrial function to support sperm’s motion energy. Lowered testosterone levels, poor sperm quality, vacuolization in seminiferous tubules, disordered germinal epithelium, and high apoptotic index cause testicular dysfunction, leading to testicular atrophy and weight loss. As demonstrated by our data, melatonin decreases the apoptotic index, which can be justified by the free radical scavenging characteristics of melatonin. As a direct and indirect free radical scavenger, melatonin protects testis tissue/cells from dysfunctions and abnormal apoptosis.
Despite the effects of melatonin on the apoptosis index, germ cell maturation, and testosterone levels, the factors that total testis weight depends on, we did not observe any correlations in the relative testis weight. This might be due to a simultaneous modulation of body weight in melatonin-treated individuals.
The adverse effect of environmental pollutants on body weight is probably associated with their action as enzymatic toxins, eventually leading to disruption in metabolic processes that could be well modulated by melatonin administration. In this review, melatonin intervention is shown to be essential in buffering body weight against toxicity damage. This is in accordance with two other systematic reviews by Mostafavi et al. and Loloei et al. (101, 102). This observation is further supported by an earlier meta-analysis by Delpino et al., suggesting that supplemental melatonin highlighted a considerable decline in body weight after individuals experienced obesity (103).
5 Conclusions and future research directions
Melatonin had beneficial protective effects against oxidative stress caused by toxic materials in rodent animal models. Although included studies crucially suffered from low quality and methodological heterogeneity. Melatonin and stressor agents’ dose and duration of administration, rodents’ characteristics, and assessment strategies varied significantly across the studies. For more literature consolidation, meticulous future studies with less difference in methodology are needed.
Data availability statement
The original contributions presented in the study are included in the article/Supplementary Material. Further inquiries can be directed to the corresponding author.
Author contributions
NA and NDE conceptualized the study. AS and NDE designed the study. NE and AS searched databases. NDE and AS screened the records. NDE, FN, MS, AM, and AS extracted the data. NDE and AS performed quality assessment. AS and MS performed meta-analysis. NDE, AS, and SP provided the draft of the manuscript. NA supervised the work. All authors contributed to the article and approved the final version. AS and NDE have contributed equally to this work and share first authorship. All authors contributed to the article and approved the submitted version.
Acknowledgments
The present study was supported the Vice-chancellor for Research (code: 27117), Shiraz University of Medical Sciences, Shiraz, Iran. This study is a part of the thesis by the first author, Niloofar Dehdari Ebrahimi, for obtaining a medical doctor degree in Shiraz University of Medical Sciences. The authors also wish to express their sincere gratitude to Hossein Noroozpoor for sketching the visual abstract.
Conflict of interest
The authors declare that the research was conducted in the absence of any commercial or financial relationships that could be construed as a potential conflict of interest.
Publisher’s note
All claims expressed in this article are solely those of the authors and do not necessarily represent those of their affiliated organizations, or those of the publisher, the editors and the reviewers. Any product that may be evaluated in this article, or claim that may be made by its manufacturer, is not guaranteed or endorsed by the publisher.
Supplementary material
The Supplementary Material for this article can be found online at: https://www.frontiersin.org/articles/10.3389/fendo.2023.1119553/full#supplementary-material
References
1. Skakkebaek NE, Rajpert-De Meyts E, Buck Louis GM, Toppari J, Andersson AM, Eisenberg ML, et al. Male Reproductive disorders and fertility trends: Influences of environment and genetic susceptibility. Physiol Rev (2016) 96(1):55–97. doi: 10.1152/physrev.00017.2015
2. Organization WH. Infertility (2020). Available at: https://www.who.int/news-room/fact-sheets/detail/infertility.
3. Agarwal A, Mulgund A, Hamada A, Chyatte MR. A unique view on male infertility around the globe. Reprod Biol endocrinol: RB&E. (2015) 13:37. doi: 10.1186/s12958-015-0032-1
4. Sonne C, Torjesen PA, Fuglei E, Muir DC, Jenssen BM, Jørgensen EH, et al. Exposure to persistent organic pollutants reduces testosterone concentrations and affects sperm viability and morphology during the mating peak period in a controlled experiment on farmed Arctic foxes (Vulpes lagopus). Environ Sci Technol (2017) 51(8):4673–80. doi: 10.1021/acs.est.7b00289
5. Tvrdá E, Massanyi P, Lukáč N. Physiological and pathological roles of free radicals in male reproduction. In: Spermatozoa-facts and perspectives. IntechOpen London, UK (2017).
6. Du Plessis SS, Agarwal A, Halabi J, Tvrda E. Contemporary evidence on the physiological role of reactive oxygen species in human sperm function. J assist Reprod Genet (2015) 32(4):509–20. doi: 10.1007/s10815-014-0425-7
7. Leclerc P, de Lamirande E, Gagnon C. Regulation of protein-tyrosine phosphorylation and human sperm capacitation by reactive oxygen derivatives. Free Radical Biol Med (1997) 22(4):643–56. doi: 10.1016/S0891-5849(96)00379-6
8. Donà G, Fiore C, Tibaldi E, Frezzato F, Andrisani A, Ambrosini G, et al. Endogenous reactive oxygen species content and modulation of tyrosine phosphorylation during sperm capacitation. Int J androl (2011) 34(5 Pt 1):411–9. doi: 10.1111/j.1365-2605.2010.01097.x
9. Aitken RJ, Paterson M, Fisher H, Buckingham DW, van Duin M. Redox regulation of tyrosine phosphorylation in human spermatozoa and its role in the control of human sperm function. J Cell Sci (1995) 108(Pt 5):2017–25. doi: 10.1242/jcs.108.5.2017
10. Tipoe GL, Leung T-M, Hung M-W, Fung M-L. Green tea polyphenols as an anti-oxidant and anti-inflammatory agent for cardiovascular protection. Cardiovasc Haematol Disorders-Drug Targets (Formerly Curr Drug Targets-Cardiovascular Hematol Disorders). (2007) 7(2):135–44. doi: 10.2174/187152907780830905
11. Dias TR, Martin-Hidalgo D, Silva BM, Oliveira PF, Alves MG. Endogenous and exogenous antioxidants as a tool to ameliorate Male infertility induced by reactive oxygen species. Antioxid Redox Signaling (2020). doi: 10.1089/ars.2019.7977
12. Adwas AA, Elsayed A, Azab A, Quwaydir F. Oxidative stress and antioxidant mechanisms in human body. J Appl Biotechnol Bioeng (2019) 6(1):43–7. doi: 10.15406/jabb.2019.06.00173
13. De Lamirande E, Gagnon C. Reactive oxygen species and human spermatozoa: I. effects on the motility of intact spermatozoa and on sperm axonemes. J androl (1992) 13(5):368–78.
15. Li X, Fang EF, Scheibye-Knudsen M, Cui H, Qiu L, Li J, et al. Di-(2-ethylhexyl) phthalate inhibits DNA replication leading to hyperPARylation, SIRT1 attenuation and mitochondrial dysfunction in the testis. Sci Rep (2014) 4(1):1–9. doi: 10.1038/srep06434
16. Paoli D, Gallo M, Rizzo F, Baldi E, Francavilla S, Lenzi A, et al. Mitochondrial membrane potential profile and its correlation with increasing sperm motility. Fertil steril (2011) 95(7):2315–9. doi: 10.1016/j.fertnstert.2011.03.059
17. Othman AI, Edrees GM, El-Missiry MA, Ali DA, Aboel-Nour M, Dabdoub BR. Melatonin controlled apoptosis and protected the testes and sperm quality against bisphenol a-induced oxidative toxicity. Toxicol Ind Health (2016) 32(9):1537–49. doi: 10.1177/0748233714561286
18. Singh S, Singh SK. Chronic exposure to perfluorononanoic acid impairs spermatogenesis, steroidogenesis and fertility in male mice. J Appl Toxicol (2019) 39(3):420–31. doi: 10.1002/jat.3733
19. Agarwal A, Roychoudhury S, Sharma R, Gupta S, Majzoub A, Sabanegh E. Diagnostic application of oxidation-reduction potential assay for measurement of oxidative stress: clinical utility in male factor infertility. Reprod biomed online (2017) 34(1):48–57. doi: 10.1016/j.rbmo.2016.10.008
20. Aitken RJ, Baker MA. Oxidative stress, sperm survival and fertility control. Mol Cell Endocrinol (2006) 250(1-2):66–9. doi: 10.1016/j.mce.2005.12.026
21. Venkatesh S, Shamsi MB, Deka D, Saxena V, Kumar R, Dada R. Clinical implications of oxidative stress & sperm DNA damage in normozoospermic infertile men. Indian J Med Res (2011) 134(3):396–8.
22. Barati E, Nikzad H, Karimian M. Oxidative stress and male infertility: current knowledge of pathophysiology and role of antioxidant therapy in disease management. Cell Mol Life sci: CMLS (2020) 77(1):93–113. doi: 10.1007/s00018-019-03253-8
23. Koppers AJ, Mitchell LA, Wang P, Lin M, Aitken RJ. Phosphoinositide 3-kinase signalling pathway involvement in a truncated apoptotic cascade associated with motility loss and oxidative DNA damage in human spermatozoa. Biochem J (2011) 436(3):687–98. doi: 10.1042/BJ20110114
24. Martins da Silva SJ. Male Infertility and antioxidants: one small step for man, no giant leap for andrology? Reprod biomed Online (2019) 39(6):879–83. doi: 10.1016/j.rbmo.2019.08.008
25. Agarwal A, Leisegang K, Majzoub A, Henkel R, Finelli R, Panner Selvam MK, et al. Utility of antioxidants in the treatment of Male infertility: Clinical guidelines based on a systematic review and analysis of evidence. World J men’s Health (2021) 39(2):233–90. doi: 10.5534/wjmh.200196
26. Ross C, Morriss A, Khairy M, Khalaf Y, Braude P, Coomarasamy A, et al. A systematic review of the effect of oral antioxidants on male infertility. Reprod biomed online (2010) 20(6):711–23. doi: 10.1016/j.rbmo.2010.03.008
27. Busetto GM, Agarwal A, Virmani A, Antonini G, Ragonesi G, Del Giudice F, et al. Effect of metabolic and antioxidant supplementation on sperm parameters in oligo-astheno-teratozoospermia, with and without varicocele: A double-blind placebo-controlled study. Andrologia (2018) 50(3). doi: 10.1111/and.12927
28. Banihani SA. Effect of ginger (Zingiber officinale) on semen quality. Andrologia (2019) 51(6):e13296. doi: 10.1111/and.13296
29. Wang J, Wang T, Ding W, Wu J, Wu G, Wang Y, et al. Efficacy of antioxidant therapy on sperm quality measurements after varicocelectomy: A systematic review and meta-analysis. Andrologia (2019) 51(10):e13396. doi: 10.1111/and.13396
30. Tatar T, Akdevelioğlu Y. Effect of pollen, pit powder, and gemmule extract of date palm on male infertility: A systematic review. J Am Coll Nutr (2018) 37(2):154–60. doi: 10.1080/07315724.2017.1364183
31. Haghi-Aminjan H, Asghari MH, Farhood B, Rahimifard M, Hashemi Goradel N, Abdollahi M. The role of melatonin on chemotherapy-induced reproductive toxicity. J Pharm Pharmacol (2018) 70(3):291–306. doi: 10.1111/jphp.12855
32. Yang M, Guan S, Tao J, Zhu K, Lv D, Wang J, et al. Melatonin promotes male reproductive performance and increases testosterone synthesis in mammalian leydig cells†. Biol reprod (2021) 104(6):1322–36. doi: 10.1093/biolre/ioab046
33. Reiter RJ, Tan D-X, Fuentes-Broto L. Melatonin: a multitasking molecule. Prog Brain Res (2010) 181:127–51. doi: 10.1016/S0079-6123(08)81008-4
34. Guo Y, Sun J, Li T, Zhang Q, Bu S, Wang Q, et al. Melatonin ameliorates restraint stress-induced oxidative stress and apoptosis in testicular cells via NF-κB/iNOS and Nrf2/HO-1 signaling pathway. Sci Rep (2017) 7(1):1–13. doi: 10.1038/s41598-017-09943-2
35. Sarabia L, Maurer I, Bustos-Obregón E. Melatonin prevents damage elicited by the organophosphorous pesticide diazinon on mouse sperm DNA. Ecotoxicol Environ safety (2009) 72(2):663–8. doi: 10.1016/j.ecoenv.2008.04.023
36. Sarabia L, Maurer I, Bustos-Obregón E. Melatonin prevents damage elicited by the organophosphorous pesticide diazinon on the mouse testis. Ecotoxicol Environ safety (2009) 72(3):938–42. doi: 10.1016/j.ecoenv.2008.04.022
37. Bustos-Obregón E, Poblete D, Catriao R, del Sol M, Fernandes FH. Melatonin protective role in mouse cauda epipidymal spermatozoa damage induced by sodium arsenite. Int J Morphol (2013) 31:1251–6. doi: 10.4067/S0717-95022013000400017
38. Lv Y, Zhang P, Guo J, Zhu Z, Li X, Xu D, et al. Melatonin protects mouse spermatogonial stem cells against hexavalent chromium-induced apoptosis and epigenetic histone modification. Toxicol Appl Pharmacol (2018) 340:30–8. doi: 10.1016/j.taap.2017.12.017
39. Aslani H, Kesıcı H, Karaca Z, Özyurt B, Taş U, Ekıcı F, et al. Beneficial effects of melatonin and BQ-123 on the rat testis damage caused by cigarette smoke. Turkish J Med Sci (2015) 45(1):11–7. doi: 10.3906/sag-1312-66
40. abd el salam L. Light and electron microscopic study on the possible protective effect of melatonin on formaldehyde induced testicular damage in adult albino rats. Egyptian J Histol (2020) 43(4):1047–58. doi: 10.21608/ejh.2020.19282.1199
41. Wang T, Li Y, Wu X, Yang X, Wang Y, Wang DJ. Protective effects of melatonin on CCl4-induced acute liver damage and testicular toxicity in rats. Indian J Pharm Sci (2018) 80:1100–7. doi: 10.4172/pharmaceutical-sciences.1000461
42. Sobhani M, Rouzbehi A, Mahmoodi R, Sobhani Z. The protective effect of melatonin on sperm morphology and histology of the rat testis damage induced by cadmium chloride. J Mazandaran Univ Med Sci (2015) 25(123):32–44.
43. Anjum S, Rahman S, Kaur M, Ahmad F, Rashid H, Ansari RA, et al. Melatonin ameliorates bisphenol a-induced biochemical toxicity in testicular mitochondria of mouse. Food Chem Toxicol (2011) 49(11):2849–54. doi: 10.1016/j.fct.2011.07.062
44. Bahrami N, Goudarzi M, Hosseinzadeh A, Sabbagh S, Reiter RJ, Mehrzadi S. Evaluating the protective effects of melatonin on di(2-ethylhexyl) phthalate-induced testicular injury in adult mice. Biomed Pharmacother (2018) 108:515–23. doi: 10.1016/j.biopha.2018.09.044
45. Bustos-Obregón E, Poblete D, Catriao R, Fernandes FH. Protective role of melatonin in mouse spermatogenesis induced by sodium arsenite. Int J Morphol Int J Morphol (2013) 31:849. doi: 10.4067/S0717-95022013000300012
46. Elwakeel S, Abd El-Monem D. AMELIORATIVE EFFECT OF MELATONIN AND QUERCETIN AGAINST BISPHENOL a INDUCED REPRODUCTIVE TOXICITY IN MALE ALBINO MICE. Ciec e Técnica Vitivinícola. (2018) 33:2018:31–64.
47. Ji Y-L, Wang H, Meng C, Zhao X-F, Zhang C, Zhang Y, et al. Melatonin alleviates cadmium-induced cellular stress and germ cell apoptosis in testes. J Pineal Res (2012) 52(1):71–9. doi: 10.1111/j.1600-079X.2011.00921.x
48. Li R, Luo X, Li L, Peng Q, Yang Y, Zhao L, et al. The protective effects of melatonin against oxidative stress and inflammation induced by acute cadmium exposure in mice testis. Biol Trace Elem Res (2016) 170(1):152–64. doi: 10.1007/s12011-015-0449-6
49. Mohammadghasemi F, Jahromi SK. Melatonin ameliorates testicular damages induced by nicotine in mice. Iranian J Basic Med Sci (2018) 21(6):639–44. doi: 10.22038/IJBMS.2018.28111.6829
50. Sarabia L, Espinoza-Navarro O, Maurer I, Ponce C, Bustos-Obregón E. Protective effect of melatonin on damage in the sperm parameters of mice exposed to diazinon. Int J Morphol (2011) 29:1241–7. doi: 10.4067/S0717-95022011000400029
51. Zayman E, Gül M, Erdemli ME, Gül S, Bağ HG, Taşlıdere E. Biochemical and histopathological investigation of the protective effects of melatonin and vitamin e against the damage caused by acetamiprid in balb-c mouse testicles at light and electron microscopic level. Environ Sci pollut Res (2022) 29(31):47571–84. doi: 10.1007/s11356-022-19143-9
52. Bashandy SAE, Ebaid H, Al-Tamimi J, Ahmed-Farid OAH, Omara EA, Alhazza IM. Melatonin alleviated potassium dichromate-induced oxidative stress and reprotoxicity in Male rats. BioMed Res Int (2021) 2021:3565360. doi: 10.1155/2021/3565360
53. Dunjic M, Krstić D, Zivkovic J, Cvetković S, Dunjić K, Mirković M, et al. Acutely applied melatonin prevents CCl4-induced testicular lesions in rats: the involvement of the oxidative capacity and arginine metabolism. Braz J Pharm Sci (2022) 58. doi: 10.1590/s2175-97902022e19745
54. Huang F, Ning H, Xin Q-Q, Huang Y, Wang H, Zhang Z-H, et al. Melatonin pretreatment attenuates 2-bromopropane-induced testicular toxicity in rats. Toxicology (2009) 256(1):75–82. doi: 10.1016/j.tox.2008.11.005
55. Kara H, Cevik A, Konar V, Dayangac A, Yilmaz M. Protective effects of antioxidants against cadmium-induced oxidative damage in rat testes. Biol Trace Elem Res (2007) 120(1):205–11. doi: 10.1007/s12011-007-8019-1
56. Karabulut D, Akin AT, Sayan M, Kaymak E, Ozturk E, Yakan B. Effects of melatonin against thioacetamide-induced testicular toxicity in rats. Int J Morphol (2020) 38:1455–62. doi: 10.4067/S0717-95022020000501455
57. Malekinejad H, Mirzakhani N, Razi M, Cheraghi H, Alizadeh A, Dardmeh F. Protective effects of melatonin and glycyrrhiza glabra extract on ochratoxin a–induced damages on testes in mature rats. Hum Exp Toxicol (2011) 30(2):110–23. doi: 10.1177/0960327110368416
58. Olayaki LA, Alagbonsi IA, Abdulrahim AH, Adeyemi WJ, Bakare M, Omeiza N. Melatonin prevents and ameliorates lead-induced gonadotoxicity through antioxidative and hormonal mechanisms. Toxicol Ind Health (2018) 34(9):596–608. doi: 10.1177/0748233718773508
59. Olumide SA. Protective effects of melatonin on bisphenol a-induced reproductive toxicity in Male wistar rats. University of Ibadan (2019).
60. Ozen OA, Kus MA, Kus I, Alkoc OA, Songur A. Protective effects of melatonin against formaldehyde-induced oxidative damage and apoptosis in rat testes: An immunohistochemical and biochemical study. Syst Biol Reprod Med (2008) 54(4-5):169–76. doi: 10.1080/19396360802422402
61. Rao MV, Bhatt RN. Protective effect of melatonin on fluoride-induced oxidative stress and testicular dysfunction in rats. Fluoride (2012) 45(2):116–24.
62. Rashad S, Ahmed S, El-Sayed M, Ahmed D. The toxic effect of bisphenol a on albino rat testicles and the possible protective value of vitamin e and melatonin. Egyptian Soc Clin Toxicol J (2021) 9(2):1–12. doi: 10.21608/esctj.2021.63294.1001
63. Risikat Kadir E, Sheriff Ojulari L, Abdullah Gegele T, Adetayo Lawal I, Sulu-Gambari L, Ajoke Sulaimon F, et al. Altered testicular histomorphometric and antioxidant levels following In vivo bisphenol-a administration. IJT (2021) 15(3):165–74. doi: 10.32598/IJT.15.3.796.1
64. Tabassum H, Parvez S, Raisuddin S. Melatonin abrogates nonylphenol-induced testicular dysfunction in wistar rats. Andrologia (2017) 49(5). doi: 10.1111/and.12648
65. Umosen AJ, Ambali SF, Ayo JO, Mohammed B, Uchendu C. Alleviating effects of melatonin on oxidative changes in the testes and pituitary glands evoked by subacute chlorpyrifos administration in wistar rats. Asian Pacific J Trop biomed (2012) 2(8):645–50. doi: 10.1016/S2221-1691(12)60113-0
66. Umosen AJ, Chidiebere U. Effect of melatonin on chlorpyrifos-induced alterations in reproductive hormones and semen characteristics in wistar rats. Am J Phytomed Clin Ther (2014) 2:742–53.
67. Uygur R, Aktas C, Caglar V, Uygur E, Erdogan H, Ozen OA. Protective effects of melatonin against arsenic-induced apoptosis and oxidative stress in rat testes. Toxicol Ind Health (2016) 32(5):848–59. doi: 10.1177/0748233713512891
68. Venditti M, Ben Rhouma M, Romano MZ, Messaoudi I, Reiter RJ, Minucci S. Altered expression of DAAM1 and PREP induced by cadmium toxicity is counteracted by melatonin in the rat testis. Genes (2021) 12(7). doi: 10.3390/genes12071016
69. Venditti M, Ben Rhouma M, Romano MZ, Messaoudi I, Reiter RJ, Minucci S. Evidence of melatonin ameliorative effects on the blood-testis barrier and sperm quality alterations induced by cadmium in the rat testis. Ecotoxicol Environ safety (2021) 226:112878. doi: 10.1016/j.ecoenv.2021.112878
70. Wu HJ, Liu C, Duan WX, Xu SC, He MD, Chen CH, et al. Melatonin ameliorates bisphenol a-induced DNA damage in the germ cells of adult male rats. Mutat Res (2013) 752(1-2):57–67. doi: 10.1016/j.mrgentox.2013.01.005
71. Kumar J, Verma R, Haldar C. Melatonin ameliorates bisphenol s induced testicular damages by modulating nrf-2/HO-1 and SIRT-1/FOXO-1 expressions. Environ Toxicol (2021) 36(3):396–407. doi: 10.1002/tox.23045
72. Rao PS, Kalva S, Yerramilli A, Mamidi S. Free radicals and tissue damage: Role of antioxidants. Free radic antioxid (2011) 1(4):2–7.
73. Karbownik M, Gitto E, Lewinski A, Reiter RJ. Induction of lipid peroxidation in hamster organs by the carcinogen cadmium: Amelioration by melatonin. Cell Biol Toxicol (2001) 17(1):33–40. doi: 10.1023/A:1010903130693
74. Griswold MD. The central role of sertoli cells in spermatogenesis. Semin Cell Dev Biol (1998). 9(4):411–6. doi: 10.1006/scdb.1998.0203
75. Santi D, Spaggiari G, Casarini L, Fanelli F, Mezzullo M, Pagotto U, et al. Central hypogonadism due to a giant,”silent” FSH-secreting, atypical pituitary adenoma: effects of adenoma dissection and short-term leydig cell stimulation by luteinizing hormone (LH) and human chorionic gonadotropin (hCG). Aging Male (2017) 20(2):96–101. doi: 10.1080/13685538.2016.1276161
76. Vecchio M, Navaneethan SD, Johnson DW, Lucisano G, Graziano G, Saglimbene V, et al. Interventions for treating sexual dysfunction in patients with chronic kidney disease. Cochrane Database Syst Rev (2010) 12). doi: 10.1002/14651858.CD007747.pub2
77. Diamanti-Kandarakis E, Bourguignon JP, Giudice LC, Hauser R, Prins GS, Soto AM, et al. Endocrine-disrupting chemicals: An endocrine society scientific statement. Endocr Rev (2009) 30(4):293–342. doi: 10.1210/er.2009-0002
78. Anne B, Raphael R. Endocrine disruptor chemicals. In: Feingold KR, Anawalt B, Boyce A, Chrousos G, de Herder WW, Dhatariya K, et al, editors. Endotext. South Dartmouth (MA): MDText.com, Inc. (2000).
79. Jana K, Jana S, Samanta PK. Effects of chronic exposure to sodium arsenite on hypothalamo-pituitary-testicular activities in adult rats: possible an estrogenic mode of action. Reprod Biol endocrinol: RB&E. (2006) 4:9. doi: 10.1186/1477-7827-4-9
80. Rosenblatt AE, Burnstein KL. Inhibition of androgen receptor transcriptional activity as a novel mechanism of action of arsenic. Mol Endocrinol (Baltimore Md). (2009) 23(3):412–21. doi: 10.1210/me.2008-0235
81. Chiou TJ, Chu ST, Tzeng WF, Huang YC, Liao CJ. Arsenic trioxide impairs spermatogenesis via reducing gene expression levels in testosterone synthesis pathway. Chem Res toxicol (2008) 21(8):1562–9. doi: 10.1021/tx700366x
82. Lubos E, Handy DE, Loscalzo J. Role of oxidative stress and nitric oxide in atherothrombosis. Front biosci: J virtual library (2008) 13:5323. doi: 10.2741/3084
83. Kumar N, Singh AK. Impact of environmental factors on human semen quality and male fertility: a narrative review. Environ Sci Europe (2022) 34(1):1–13. doi: 10.1186/s12302-021-00585-w
84. Oduwole OO, Huhtaniemi IT, Misrahi M. The roles of luteinizing hormone, follicle-stimulating hormone and testosterone in spermatogenesis and folliculogenesis revisited. Int J Mol Sci (2021) 22(23). doi: 10.3390/ijms222312735
85. Qin F, Zhang J, Zan L, Guo W, Wang J, Chen L, et al. Inhibitory effect of melatonin on testosterone synthesis is mediated via GATA-4/SF-1 transcription factors. Reprod biomed online (2015) 31(5):638–46. doi: 10.1016/j.rbmo.2015.07.009
86. Ahmad R, Haldar C. Effect of intra-testicular melatonin injection on testicular functions, local and general immunity of a tropical rodent funambulus pennanti. Endocrine (2010) 37(3):479–88. doi: 10.1007/s12020-010-9331-7
87. Reiter RJ. Pineal melatonin: cell biology of its synthesis and of its physiological interactions. Endocr Rev (1991) 12(2):151–80. doi: 10.1210/edrv-12-2-151
88. Frungieri MB, Mayerhofer A, Zitta K, Pignataro OP, Calandra RS, Gonzalez-Calvar SI. Direct effect of melatonin on Syrian hamster testes: melatonin subtype 1a receptors, inhibition of androgen production, and interaction with the local corticotropin-releasing hormone system. Endocrinology (2005) 146(3):1541–52. doi: 10.1210/en.2004-0990
89. Maitra SK, Ray AK. Role of light in the mediation of acute effects of a single afternoon melatonin injection on steroidogenic activity of testis in the rat. J biosci (2000) 25(3):253–6. doi: 10.1007/BF02703932
90. Valenti S, Giusti M. Melatonin participates in the control of testosterone secretion from rat testis: an overview of our experience. Ann New York Acad Sci (2002) 966:284–9. doi: 10.1111/j.1749-6632.2002.tb04228.x
91. da Costa CF, Gobbo MG, Taboga SR, Pinto-Fochi ME, Góes RM. Melatonin intake since weaning ameliorates steroidogenic function and sperm motility of streptozotocin-induced diabetic rats. Andrology (2016) 4(3):526–41. doi: 10.1111/andr.12158
92. Olszowski T, Baranowska-Bosiacka I, Gutowska I, Chlubek D. Pro-inflammatory properties of cadmium. Acta Biochim Polonica (2012) 59(4):475–82. doi: 10.18388/abp.2012_2080
93. Li D, Ding Z, Du K, Ye X, Cheng S. Reactive oxygen species as a link between antioxidant pathways and autophagy. Oxid Med Cell longevity (2021) 2021:5583215. doi: 10.1155/2021/5583215
94. Morvaridzadeh M, Sadeghi E, Agah S, Nachvak SM, Fazelian S, Moradi F, et al. Effect of melatonin supplementation on oxidative stress parameters: a systematic review and meta-analysis. Pharmacol Res (2020) 161:105210. doi: 10.1016/j.phrs.2020.105210
95. Sumsuzzman DM, Khan ZA, Choi J, Hong Y. Differential role of melatonin in healthy brain aging: a systematic review and meta-analysis of the SAMP8 model. Aging (2021) 13(7):9373–97. doi: 10.18632/aging.202894
96. Tan D-X, Manchester LC, Reiter RJ, Plummer BF, Limson J, Weintraub ST, et al. Melatonin directly scavenges hydrogen peroxide: a potentially new metabolic pathway of melatonin biotransformation. Free Radical Biol Med (2000) 29(11):1177–85. doi: 10.1016/S0891-5849(00)00435-4
97. Reiter RJ, Tan D-X, Galano A. Melatonin reduces lipid peroxidation and membrane viscosity. Front Media SA; (2014) p:377. doi: 10.3389/fphys.2014.00377
98. Tomás-Zapico C, Coto-Montes A. A proposed mechanism to explain the stimulatory effect of melatonin on antioxidative enzymes. J pineal Res (2005) 39(2):99–104. doi: 10.1111/j.1600-079X.2005.00248.x
99. Sharpe RM. Environmental/lifestyle effects on spermatogenesis. philosophical transactions of the royal society of London series b. Biol Sci (2010) 365(1546):1697–712. doi: 10.1098/rstb.2009.0206
100. Shaha C, Tripathi R, Mishra DP. Male Germ cell apoptosis: Regulation and biology. Philos Trans R Soc London Ser B Biol Sci (2010) 365(1546):1501–15. doi: 10.1098/rstb.2009.0124
101. Mostafavi S-A, Akhondzadeh S, Reza Mohammadi M, Keshtkar A-A, Hosseini S, Reza Eshraghian M, et al. Role of melatonin in body weight: A systematic review and meta-analysis. Curr Pharm Design (2017) 23(23):3445–52. doi: 10.2174/1381612822666161129145618
102. Loloei S, Sepidarkish M, Heydarian A, Tahvilian N, Khazdouz M, Heshmati J, et al. The effect of melatonin supplementation on lipid profile and anthropometric indices: A systematic review and meta-analysis of clinical trials. Diabetes Metab Syndr: Clin Res Rev (2019) 13(3):1901–10. doi: 10.1016/j.dsx.2019.04.043
Keywords: melatonin, infertility, rodents, oxidative stress, environmental pollutants, heavy metals
Citation: Dehdari Ebrahimi N, Parsa S, Nozari F, Shahlaee MA, Maktabi A, Sayadi M, Sadeghi A and Azarpira N (2023) Protective effects of melatonin against the toxic effects of environmental pollutants and heavy metals on testicular tissue: A systematic review and meta-analysis of animal studies. Front. Endocrinol. 14:1119553. doi: 10.3389/fendo.2023.1119553
Received: 08 December 2022; Accepted: 17 January 2023;
Published: 30 January 2023.
Edited by:
Luna Samanta, Ravenshaw University, IndiaReviewed by:
Jun Wang, Jilin Agriculture University, ChinaSergio Minucci, Università della Campania Luigi Vanvitelli, Italy
Copyright © 2023 Dehdari Ebrahimi, Parsa, Nozari, Shahlaee, Maktabi, Sayadi, Sadeghi and Azarpira. This is an open-access article distributed under the terms of the Creative Commons Attribution License (CC BY). The use, distribution or reproduction in other forums is permitted, provided the original author(s) and the copyright owner(s) are credited and that the original publication in this journal is cited, in accordance with accepted academic practice. No use, distribution or reproduction is permitted which does not comply with these terms.
*Correspondence: Negar Azarpira, negarazarpira@yahoo.com
†These authors have contributed equally to this work and share first authorship