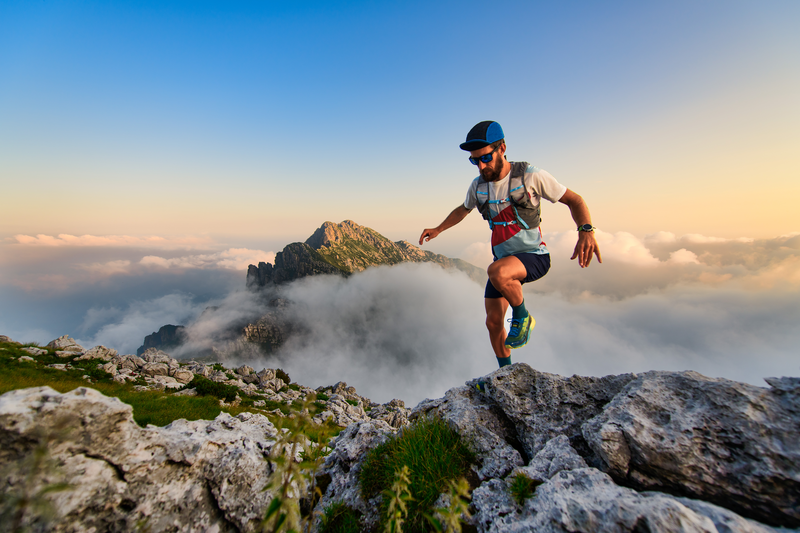
94% of researchers rate our articles as excellent or good
Learn more about the work of our research integrity team to safeguard the quality of each article we publish.
Find out more
ORIGINAL RESEARCH article
Front. Endocrinol. , 15 February 2023
Sec. Clinical Diabetes
Volume 14 - 2023 | https://doi.org/10.3389/fendo.2023.1111152
This article is part of the Research Topic Clinical and Genetic Determinants of Diabetes and Complications View all 31 articles
Background: Remnant cholesterol (RC) has been correlated with a higher risk of atherosclerosis. It has been confirmed that in the general population, an elevated RC level is related to a 5-fold higher risk of peripheral arterial disease (PAD). Diabetes is one of the strongest risk factors for PAD development. However, the association between RC and PAD in the specific population of type 2 diabetes mellitus (T2DM) has not been investigated. Herein, the correlation was investigated between RC and PAD in T2DM patients.
Methods: In the retrospective study, the hematological parameter data of 246 T2DM patients without PAD (T2DM - WPAD) and 270 T2DM patients with PAD (T2DM - PAD) was collected. Differences in RC levels between the two groups were compared, and the association between RC and PAD severity was examined. Multifactorial regression was used to determine whether RC was a significant contributor to the development of T2DM - PAD. The diagnostic potential of RC was tested using receiver operating characteristic (ROC) curve.
Results: The RC levels in T2DM - PAD individuals were considerably greater than in T2DM - WPAD individuals (P < 0.001). RC had a positive correlation with disease severity. Further, multifactorial logistic regression analyses found that elevated RC levels were a major contributor to T2DM - PAD (P < 0.001). The area under the curve (AUC) of the RC for T2DM - PAD patients was 0.727. The cut-off value of RC was 0.64 mmol/L.
Conclusion: The RC levels were higher in T2DM - PAD patients, and were independently linked with its severity. Diabetic patients with RC levels > 0.64 mmol/L had an elevated risk of developing PAD.
PAD is a chronic arterial occlusive disease of the lower limbs caused by atherosclerosis and is linked with substantial disability and death (1). T2DM is a main factor in the progression of atherosclerosis. The incidence of PAD rises in tandem with the occurrence of T2DM (2). In addition, diabetic people have a worse prognosis for PAD than non-diabetic ones (1). Thus, prompt diagnosis and treatment of PAD in diabetic subjects are necessary to reduce the danger of major adverse limb events (MALEs) (2).The ankle-brachial index (ABI) is currently recommended as the primary screening tool for PAD in diabetic patients and those with multiple risk factors (3). The ABI’s limited sensitivity in detecting PAD in its earliest stage highlights the critical need to discover new markers that may detect PAD in diabetics at an earlier stage.
RC is the cholesterol in triglyceride-rich lipoproteins and consists of very low-density lipoproteins (VLDL), intermediate-density lipoproteins (IDL), and chylomicron remnants (4). RC-level assessment can be easily calculated using established formulas, which are easy-to-access, and may provide valuable data for clinical management (5). Evidence from large prospective cohort studies based on the general population suggests a causal relationship between high remnant cholesterol levels and cardiovascular disease(CVD), and it is well established that lowering these lipoproteins reduces atherosclerotic cardiovascular events in humans (6–8). Recent studies have confirmed the atherogenic potential of RC, however, many of these studies focused on elevated RC levels in coronary arterial disease (CAD) and cerebrovascular disease, demonstrating an association between elevated RC levels and the risk of ischemic heart disease, myocardial infarction, and ischemic stroke (8–10). Interestingly, a recent investigation showed that in the general population, an elevated RC level was associated with a five-fold higher risk of PAD, greater than for myocardial infarction and ischemic stroke (10). High RC levels are common in diabetic individuals and has been linked to atherosclerosis through lipid metabolism and insulin resistance (11). It’s intriguing to speculate about whether or not RC also plays a part in the development of PAD in diabetics. Nevertheless, until now, there has been no study on whether there is a correlation between RC and PAD in T2DM population. The aim of the research was to examine whether higher RC levels were related to higher PAD risk among T2DM individuals.
The cross-sectional research involved 514 gender-matched diabetic patients consecutively admitted to the Department of Endocrinology and Metabolism of the Liyuan Hospital affiliated to Tongji Medical College, Huazhong University of Science and Technology (Wuhan, China), from 1 March 2018 to 30 October 2022. T2DM patients with or without PAD were recruited. T2DM was defined as a fasting plasma glucose(FPG) level ≥ 7.0 mmol/L and/or 2-h plasma glucose(PG) ≥11.1 mmol/L during OGTT and/or HbA1c level ≥ 6.5%, based on the T2DM international criteria (ADA) (12). The inclusion criteria were patients aged 18-79 years with a confirmed diagnosis of T2DM. The excluding criteria were: a) coronary artery disease (CAD); b) history of stoke; c) diabetic retinopathy; d) acute complications of diabetes mellitus (such as diabetic ketoacidosis, hyperglycemia hyperosmotic state, and lactic acidosis); e) chronic kidney disease with an estimated glomerular filtration rate (eGFR) less than 60 mL/min; f) documented liver cirrhosis with Child–Pugh C dysfunction; g) history of active solid or hematological malignancy or autoimmune diseases; h) ABI > 1.4; i)RC < 0; j) suspected or confirmed pregnancy; k) undefined type of diabetes or clinical suspicion of non-type 2 diabetes mellitus; l) previous non-traumatic lower limb amputation; m) incomplete clinical data.
Each patient included in the study was evaluated for a history of PAD symptoms. The ABI was measured in patients with PAD-like symptoms. ABI was calculated according to the Transatlantic Inter-Society Consensus Document II (TASC-II) guidelines for the management of peripheral arterial disease (13). ABI was calculated as the ratio of ankle-to-brachial artery systolic pressure. ABI was computed by dividing the highest systolic pressure recorded in either the right or left brachial arteries or the anterior or posterior tibial arteries in each limb (14). The physician evaluated the patients’ lower extremities using arterial Doppler-enhanced ultrasonography if they had symptoms in both legs. Patients with an ABI > 0.90 who were asymptomatic were not additionally evaluated for PAD.
Patients whose ABI < 0.9 underwent arterial Doppler-enhanced ultrasonography of the limb extremities. The common femoral artery, femoral artery bifurcation, popliteal artery, posterior tibial artery, and dorsalis pedis artery were examined. The evaluation and score of vascular pathology were as follows: a) Artery intima thickness: normal (< 1 mm), 0 point; moderately thickened (1 – 1.2 mm), 1 point; severely thickened (> 1.2 mm), 2 points. b) Hardening: normal, 0 point; mildly hardened (the intima was not thickened, the echo was increased, and with no plaque), 1 point; moderately to severely hardened (mildly hardened, accompanied with plaque or stenosis), 2 points. c) Plaque: normal (no plaque forming), 0 point; single plaque, 1 point; numerous plaques, 2 points; scattered plaques, 3 points. d) Stenosis: normal, 0 point; mild stenosis (narrowing by 30%–50%), 1 point; moderate or severe stenosis (narrowing by 50% – 75%), 2 points; occlusion (no blood flow), 3 points. The degree of PAD was categorized based on the total number of points: a) 0 point, normal; b) < 10 mild; c) 10 – 20 points, moderate; d) > 20 points, severe (15).
Demographic variables (age and gender), as well as laboratory results, such as blood count, total cholesterol (TC), high-density lipoprotein cholesterol (HDL-C), were obtained from the electronic medical record system in Liyuan hospital. On the second hospital morning, blood samples were collected from all patients’ peripheries. Laboratory personnel unaware of the patient’s diagnoses analyzed the blood samples.
RC levels were determined as TC (mmol/L) minus LDL-C (mmol/L) and HDL-C (mmol/L), as recommended by the dyslipidemia guidelines (16). The triglyceride glucose index (TyG index), neutrophil to lymphocyte ratio (NLR), monocyte to lymphocyte ratio (MLR), and platelet to HDL-C ratio (PHR) were calculated using the following formulas: TyG index = Ln [Triglyceride (TG, mg/dl) × FPG (mg/dl)/2]; NLR = neutrophil (109/L)/lymphocyte (109/L); MLR = monocyte (109/L)/lymphocyte (109/L); PHR = platelet (109/L)/HDL-C (mmol/L).
Statistical analyses were done using SPSS version 27.0 software (SPSS, Inc., Chicago, IL, United States). Graphs were created using Prism 9.0 (GraphPad Software). The normality of continuous variables was examined by the Shapiro-Wilk test. Continuous variables were described as means ± SDs and evaluated utilizing the Student’s t-test (two groups) or the One-way ANOVA (three groups). Non-normally distributed continuous variables were described as medians (interquartile ranges) and assessed using the Mann-Whitney U test (two groups) or Kruskal-Wallis test (three groups). Categorical variables were described as the numbers and percentages of patients. Chi-square tests were performed to assess categorical variables. The link between RC and PAD phases was analyzed by utilizing spearman correlation and partial correlation analysis. The relationship between RC and other variables in PAD patients was analyzed by Spearman correlation analysis. Covariates were excluded from the correlation analysis. Univariate and multivariate logistic regression analysis were used to examine the association between RC and PAD. The optimal value for identifying the risk of PAD in this sample was calculated using ROC curve analysis. The optimal cutoff value was determined by maximizing the Yoden index. Statistical significance was defined as a two-sided P value < 0.05.
The demographic and clinical data of T2DM - PAD group and T2DM - WPAD group are summarized in Table 1. Among the 516 diabetic patients enrolled, 270 had PAD, and 246 did not (WPAD). Compared to WPAD patients, PAD patients had a higher prevalence of hypertension (P < 0.05), and showed significantly increased levels of age, diabetes duration, systolic blood pressure (SBP), urea, creatinine (Cr), C-reactive protein (CRP), RC, neutrophils, monocytes, NLR, MLR, and PHR (P < 0.05), and showed significantly decreased levels of diastolic blood pressure (DBP), alanine aminotransferase (ALT), eGFR, HDL-C, and lymphocytes (P < 0.05). The two groups did not differ for gender, history of smoking, drinking, and dyslipidemia, aspartate aminotransferase (AST), uric acid, FPG, glycosylated hemoglobin (HbA1c), TG, TC, LDL-C, non-HDL-C(N-HDL-C), TyG index, and platelets (P > 0.05). Significant differences in glucose-lowering measures and statin use were found between the two groups (both P < 0.05). The incidence of mild, moderate, and severe PAD was 50.7, 23.3, and 25.9% in PAD patients, respectively.
The three groups did not differ regarding gender, duration of diabetes, history of smoking, drinking, and dyslipidemia, SBP, DBP, and laboratory parameters such as ALT, AST, urea, Cr, FPG, HbA1c, TC, LDL-C, N-HDL-C, TyG index, neutrophils, lymphocytes, monocytes, NLR, and MLR (P > 0.05) (Table 2). As disease severity increased, history of hypertension, eGFR, and HDL-C presented a decreasing trend (P < 0.05), but TG, RC, platelets, and PHR showed an increasing trend (P < 0.05). Moderate PAD patients had the highest levels of age, uric acid, and CRP (P < 0.05). Significant differences were found between the three groups using only oral medication or only insulin (P < 0.05).
The violin - plot in Figure 1 found that the RC levels showed an increasing relationship with disease extent.
Figure 1 The RC levels according to PAD severity based on ultrasound results. RC, remnant cholesterol. In the violin plot, the three horizontal lines from top to bottom represent the upper quartile, the median, and the lower quartile in order. P < 0.05 (two-sided) was defined as statistically significant. Bold values indicate statistically significance.
The correlations between RC and other lipid variables were assessed by utilizing spearman correlation analysis (including TG, TC, LDL-C, HDL-C, and N-HDL-C) in PAD patients. Based on the data in Table 3, RC (r = 0.387, P < 0.001), TG (r = 0.151, P = 0.013), and HDL-C (r = -0.197, P < 0.001) were associated with the PAD severity levels. RC still maintained connections with PAD stages after adjusting for TG and/or HDL-C using partial correlation analysis.
As univariate logistic regression analysis showed (Table 4), age, duration of diabetes, history of hypertension, SBP, DBP, ALT, urea, Cr, eGFR, HDL-C, CRP, RC, NLR, MLR, and PHR were independently associated with PAD occurrence in T2DM patients (P < 0.05). After excluding the effects of confounding factors for multivariate logistic regression, age, duration of diabetes, HDL-C, RC, NLR, MLR, and PHR were still statistically significant. RC, NLR, MLR, and PHR were considered independent risk factors for PAD occurrence in T2DM patients, while HDL-C was an independent protective factor.
The ability of RC to identify T2DM - PAD patients was evaluated by the ROC curve. Figure 2 showed that RC exhibited a high predicting value for T2DM – PAD (AUC = 0.727). The optimum RC cut-off value for predicting the occurrence of PAD in the group was 0.64 mmol/L (Sensitivity 71.9%, Specificity 64.6%).
Figure 2 ROC curve analysis of the ability of RC to predict T2DM – PAD. RC, remnant cholesterol. AUC = 0.727, 95% CI:0.683–0.770, P = 0.000, cut-off: 0.64, sensitivity 71.9%, specificity 64.6%.
Correlations between RC and other indicators in PAD patients were assessed using Spearman correlation analysis. The RC had a significant and positive correlation with gender (r = 0.330), fasting glucose (r = 0.125), TG (r = 0.641), TC (r = 0.342), N-HDL-C (r = 0.379), TyG index (r = 0.485), and PHR (r = 0.123) (all P < 0.05) (Table 5).
In this study, the relationship was first explored between RC and T2DM - PAD patients. The main conclusions were as follows: (1) RC levels had a positive association with the occurrence and severity of PAD, and RC was independently related to an increased risk of PAD in T2DM patients; (2) diabetic patients with RC levels > 0.64 mmol/L had an elevated risk of developing PAD.
Patients with T2DM and PAD have a cardiovascular mortality risk five times higher than patients with only one disease (17, 18). Hence, effective early screening and identification of T2DM - PAD individuals is crucial (19). Several potential biomarkers have been detected for PAD in diabetic patients, including HMGB 1, OPG, FGF 23, Omentin-1, Cyr61, and Sortilin (20–24). However, there are several limitations to obtaining these data in daily clinical practice. RC can be easily obtained using standard laboratory indices and may have substantial clinical use.
LDL-C is an established risk factor for atherosclerotic cardiovascular disease (ASCVD) (25). However, a high residual risk of CVD persists even in patients whose LDL-C levels meet therapeutic targets after statin therapy, as established by multiple recent meta-analyses (26, 27). RC may be an important contributor of this residual risk (28). In this study, RC levels were significantly higher in the PAD group than in the WPAD group, and LDL-C levels were not significantly different (Table 1). The 2019 European Society of Cardiology guidelines recommend that the goal level of LDL be below 1.8 mmol/L with an LDL-C reduction of ≥ 50% from baseline (29). Unfortunately, LDL-C levels failed to meet the established criteria in both groups of patients. In the Supplementary Material, the two groups were divided respectively based on the use of statins or not. In the subgroups, LDL-C levels decreased significantly, whereas there was no statistical difference in RC levels. The results indicated that statins did not have a substantial effect on RC levels in T2DM patients with or without PAD (See Supplementary Tables 1, 2). Previous clinical studies have shown that statins reduce RC levels in patients with CAD (30, 31). A prospective cohort with a larger sample size is necessary to see whether statins reduce RC levels in patients with PAD. Comparing the PAD and WPAD groups of patients with LDL-C at the target level, a significant difference in RC levels was found. Elevated RC levels might explain the residual risk of PAD in DM patients with LDL-C level < 1.8 mmol/L (See Supplementary Table 3).
In this study, the severity of PAD was graded based on ultrasound measurements, which showed a positive correlation between RC levels and severity (Figure 1). Patients were also classified according to the severity of their clinical symptoms using the Fontaine classification (32); however, there was no link between the RC levels and the Fontaine classification. This finding provided more evidence that RC should be promoted in clinical settings alongside ultrasonography results for patient evaluation (See Supplementary Figure 1). After adjusting for other factors in the lipid profile using partial correlation analysis (all P < 0.001), a significant connection was found between RC and ultrasound grading. (Table 3)
The multifactorial regression, excluding the effects of confounding factors, showed that RC was independently associated with T2DM - PAD. This study also demonstrated that age and duration of diabetes were independent risk factors, consistent with previous studies (19). The roles of lipid metabolism and inflammation in atherosclerosis are well-established. It is generally accepted that NLR and MLR can be evaluated as inflammatory markers (33, 34). The platelet to HDL-C ratio as a novel inflammatory index has also garnered attention (35). The research also showed that HDL-C was a protective factor, and that NLR, MLR, and PHR were independent risk factors for PAD (Table 4). The ability of RC to predict T2DM - PAD was examined by using ROC curve, and the AUC was 0.727. The cut-off value was 0.64 mmol/L, indicating that diabetic patients with RC > 0.64 mmol/L had an elevated risk of developing PAD.
The TyG index, a surrogate for insulin resistance, is significantly related to the gold standard hyperinsulinemic-orthoglycemic clamp (36) and can be a reliable assessment of insulin resistance in patients. RC has been explored to be linked to insulin resistance (37). TyG index showed a correlation with RC (r = 0.485, P < 0.001) (Table 5), so it could be speculated that elevated RC levels in T2DM - PAD patients might be mediated by insulin resistance. In addition, one of the key mechanisms of pathogenesis for T2DM - PAD is the hypo-inflammatory response (38). It is worth noting that RC can also cause an inflammatory response, resulting in vascular endothelial damage (5). As shown in Table 5, CRP, NLR, MLR, and PHR levels were elevated in the T2DM - PAD individuals, but only PHR was significantly linked to RC (r = 0.123, P = 0.044). The correlation between inflammation and RC needs to be further verified by a large-scale investigation.
The fact that RC leads to atherosclerosis is the most likely cause of the link between raised RC levels and an increased risk of PAD (39). As with LDL particles, RC may enter the endothelium, where they are predominantly trapped because of their relatively large size (40), leading to the development of atherosclerosis as a result of cholesterol levels (39). Elevated RC levels are considered a risk factor for endothelial vasodilator dysfunction and can upregulate endothelial expression of endothelial-derived proatherogenic thrombogenic molecules via redox mechanisms (41, 42).It was reported that at high glucose concentrations, endothelial cells showed increased expression of low-density lipoprotein receptor 1 (LOX-1), thereby increasing vascular dysfunction (43). Interestingly, RC stimulated NAD(P)H oxidase-dependent superoxide formation and induction of cytokines in human umbilical vein endothelial cells (HUVECs) via activation of LOX-1, thereby exacerbating atherosclerosis (44). Furthermore, LOX-1-mediated uptake of RC plays important roles in atherogenesis by inducing LOX-1 expression and vascular smooth muscle cell migration, especially in the context of postprandial hyperlipidemia, diabetes, and metabolic syndrome (45). It could be hypothesized that in patients with DM and PAD, RC might also impact the etiology of PAD by inducing LOX-1 expression. Further studies are needed to determine the specific mechanism of action.
However, this current study also has some limitations. First, this was a retrospective cross-sectional study conducted in a single center, unable to determine the causal relationship between disease and RC. Second, the data were collected from clinical databases, and direct measurement of RC has not yet become a routine test for clinical lipid testing. Therefore, only get the calculated RC levels could be obtained. Calculated and measured RC are closely related (46, 47). Previous studies have shown that calculated RC underestimates the risk of myocardial infarction compared to directly measured RC (48). Nevertheless, calculated RC can be easily obtained from available lipid measurements at no additional cost, and therefore has a strong clinical utility. Third, although the non-fasting RC is critical in the development of atherosclerosis (49), only fasting RC levels were considered, possibly ignoring the possible results of non-fasting RC levels (6).Further prospective studies are required to analyze whether RC accelerates atherosclerosis progression.
The original contributions presented in the study are included in the article/Supplementary Material. Further inquiries can be directed to the corresponding author.
The studies involving human participants were reviewed and approved by [2022] IEC CRYJ 0019. Written informed consent for participation was not required for this study in accordance with the national legislation and the institutional requirements.
SJ and YiS conceived the study plan and contributed to the revision of the final manuscript. YiS collected, analyzed the data, and finished the manuscript writing. YZ and XB participated in data collection and literature search. WC, LW, MS, YaS, and LZ contributed to the manuscript writing and data interpretation. All authors contributed to the article and approved the submitted version.
This work was supported by the National Key & R&D Program of China to SJ (No. 2020YFC2008900) and the grants from the National Natural Science Foundation of China to SJ (No. 82070862).
The authors declare that the research was conducted in the absence of any commercial or financial relationships that could be construed as a potential conflict of interest.
All claims expressed in this article are solely those of the authors and do not necessarily represent those of their affiliated organizations, or those of the publisher, the editors and the reviewers. Any product that may be evaluated in this article, or claim that may be made by its manufacturer, is not guaranteed or endorsed by the publisher.
The Supplementary Material for this article can be found online at: https://www.frontiersin.org/articles/10.3389/fendo.2023.1111152/full#supplementary-material
1. Barnes JA, Eid MA, Creager MA, Goodney PP. Epidemiology and risk of amputation in patients with diabetes mellitus and peripheral artery disease. Arterioscler Thromb Vasc Biol (2020) 40(8):1808–17. doi: 10.1161/ATVBAHA.120.314595
2. Nativel M, Potier L, Alexandre L, Baillet-Blanco L, Ducasse E, Velho G, et al. Lower extremity arterial disease in patients with diabetes: A contemporary narrative review. Cardiovasc Diabetol (2018) 17(1):138. doi: 10.1186/s12933-018-0781-1
3. American Diabetes Association. 1. improving care and promoting health in populations: Standards of medical care in diabetes-2020. Diabetes Care (2020) 43(Suppl 1):S7–13. doi: 10.2337/dc20-S001
4. Jørgensen AB, Frikke-Schmidt R, West AS, Grande P, Nordestgaard BG, Tybjærg-Hansen A. Genetically elevated non-fasting triglycerides and calculated remnant cholesterol as causal risk factors for myocardial infarction. Eur Heart J (2013) 34(24):1826–33. doi: 10.1093/eurheartj/ehs431
5. Bernelot Moens SJ, Verweij SL, Schnitzler JG, Stiekema LCA, Bos M, Langsted A, et al. Remnant cholesterol elicits arterial wall inflammation and a multilevel cellular immune response in humans. Arterioscler Thromb Vasc Biol (2017) 37(5):969–75. doi: 10.1161/ATVBAHA.116.308834
6. Varbo A, Freiberg JJ, Nordestgaard BG. Extreme nonfasting remnant cholesterol vs extreme LDL cholesterol as contributors to cardiovascular disease and all-cause mortality in 90000 individuals from the general population. Clin Chem (2015) 61(3):533–43. doi: 10.1373/clinchem.2014.234146
7. Varbo A, Nordestgaard BG. Remnant cholesterol and risk of ischemic stroke in 112,512 individuals from the general population. Ann Neurol (2019) 85(4):550–9. doi: 10.1002/ana.25432
8. Li W, Huang Z, Fang W, Wang X, Cai Z, Chen G, et al. Remnant cholesterol variability and incident ischemic stroke in the general population. Stroke (2022) 53(6):1934–41. doi: 10.1161/STROKEAHA.121.037756
9. Jepsen AMK, Langsted A, Varbo A, Bang LE, Kamstrup PR, Nordestgaard BG. Increased remnant cholesterol explains part of residual risk of all-cause mortality in 5414 patients with ischemic heart disease. Clin Chem (2016) 62(4):593–604. doi: 10.1373/clinchem.2015.253757
10. Wadström BN, Wulff AB, Pedersen KM, Jensen GB, Nordestgaard BG. Elevated remnant cholesterol increases the risk of peripheral artery disease, myocardial infarction, and ischaemic stroke: A cohort-based study. Eur Heart J (2022) 43(34):3258–69. doi: 10.1093/eurheartj/ehab705
11. Hattori S. Empagliflozin decreases remnant-like particle cholesterol in type 2 diabetes patients with insulin resistance. J Diabetes Investig (2018) 9(4):870–4. doi: 10.1111/jdi.12781
12. American Diabetes Association. 6. glycemic targets: Standards of medical care in diabetes–2021. Diabetes Care (2021) 44(Supplement_1):S73–84. doi: 10.1093/eurheartj/ehab705
13. Norgren L, Hiatt WR, Dormandy JA, Nehler MR, Harris KA, Fowkes FGR, et al. Inter-society consensus for the management of peripheral arterial disease (TASC II). Eur J Vasc Endovasc Surg (2007) 33(Suppl 1):S1–75. doi: 10.1111/jdi.12781
14. Demir O, Tasci I, Acikel C, Saglam K, Gezer M, Acar R, et al. Individual variations in ankle brachial index measurement among Turkish adults. Vascular (2016) 24(1):53–8. doi: 10.1177/1708538115584506
15. Jiang W, Tang Q, Zhang L, Chao Y, Hong B. Association of serum C1q tumour necrosis factor–related protein 9 with the severity of lower extremity peripheral arterial disease in type 2 diabetes patients. Diabetes Vasc Dis Res (2018) 15(3):270–3. doi: 10.1177/1479164118763254
16. Jacobson TA, Ito MK, Maki KC, Orringer CE, Bays HE, Jones PH, et al. National lipid association recommendations for patient-centered management of dyslipidemia: Part 1–full report. J Clin Lipidol (2015) 9(2):129–69. doi: 10.1016/j.jacl.2015.02.003
17. Jude EB, Oyibo SO, Chalmers N, Boulton AJM. Peripheral arterial disease in diabetic and nondiabetic patients. Diabetes Care (2001) 24(8):1433–7. doi: 10.2337/diacare.24.8.1433
18. Leibson CL, Ransom JE, Olson W, Zimmerman BR, O’Fallon WM, Palumbo PJ. Peripheral arterial disease, diabetes, and mortality. Diabetes Care (2004) 27(12):2843–9. doi: 10.2337/diacare.27.12.2843
19. American Diabetes Association. Peripheral arterial disease in people with diabetes. Diabetes Care (2003) 26(12):3333–41. doi: 10.2337/diacare.26.12.3333
20. Giovannini S, Tinelli G, Biscetti F, Straface G, Angelini F, Pitocco D, et al. Serum high mobility group box-1 and osteoprotegerin levels are associated with peripheral arterial disease and critical limb ischemia in type 2 diabetic subjects. Cardiovasc Diabetol (2017) 16(1):99. doi: 10.1186/s12933-017-0581-z
21. He X, Hu X, Ma X, Su H, Ying L, Peng J, et al. Elevated serum fibroblast growth factor 23 levels as an indicator of lower extremity atherosclerotic disease in Chinese patients with type 2 diabetes mellitus. Cardiovasc Diabetol (2017) 16(1):77. doi: 10.1186/s12933-017-0559-x
22. Biscetti F, Nardella E, Bonadia N, Angelini F, Pitocco D, Santoliquido A, et al. Association between plasma omentin-1 levels in type 2 diabetic patients and peripheral artery disease. Cardiovasc Diabetol (2019) 18(1):74. doi: 10.1186/s12933-019-0880-7
23. Feng B, Xu G, Sun K, Duan K, Shi B, Zhang N. Association of serum Cyr61 levels with peripheral arterial disease in subjects with type 2 diabetes. Cardiovasc Diabetol (2020) 19(1):194. doi: 10.1186/s12933-020-01171-9
24. Biscetti F, Bonadia N, Santini F, Angelini F, Nardella E, Pitocco D, et al. Sortilin levels are associated with peripheral arterial disease in type 2 diabetic subjects. Cardiovasc Diabetol (2019) 18(1):5. doi: 10.1186/s12933-019-0805-5
25. Expert Dyslipidemia Panel of the International Atherosclerosis Society Panel members. An international atherosclerosis society position paper: Global recommendations for the management of dyslipidemia–full report. J Clin Lipidol (2014) 8(1):29–60. doi: 10.1186/s12933-020-01171-9
26. Mills EJ, Wu P, Chong G, Ghement I, Singh S, Akl EA, et al. Efficacy and safety of statin treatment for cardiovascular disease: A network meta-analysis of 170,255 patients from 76 randomized trials. QJM (2011) 104(2):109–24. doi: 10.1093/qjmed/hcq165
27. Cholesterol Treatment Trialists’ (CTT) Collaboration, Baigent C, Blackwell L, Emberson J, Holland LE, Reith C, et al. Efficacy and safety of more intensive lowering of LDL cholesterol: A meta-analysis of data from 170,000 participants in 26 randomised trials. Lancet (2010) 376(9753):1670–81. doi: 10.1016/S0140-6736(10)61350-5
28. Sandesara PB, Virani SS, Fazio S, Shapiro MD. The forgotten lipids: Triglycerides, remnant cholesterol, and atherosclerotic cardiovascular disease risk. Endocr Rev (2019) 40(2):537–57. doi: 10.1210/er.2018-00184
29. Mach F, Baigent C, Catapano AL, Koskinas KC, Casula M, Badimon L, et al. 2019 ESC/EAS guidelines for the management of dyslipidaemias: Lipid modification to reduce cardiovascular risk. Eur Heart J (2020) 41(1):111–88. doi: 10.1093/eurheartj/ehz455
30. Mangili OC, Moron Gagliardi AC, Mangili LC, Mesquita CH, Machado Cesar LA, Tanaka A, et al. Favorable effects of ezetimibe alone or in association with simvastatin on the removal from plasma of chylomicrons in coronary heart disease subjects. Atherosclerosis (2014) 233(1):319–25. doi: 10.1016/j.atherosclerosis.2013.12.008
31. Elshazly MB, Mani P, Nissen S, Brennan DM, Clark D, Martin S, et al. Remnant cholesterol, coronary atheroma progression and clinical events in statin-treated patients with coronary artery disease. Eur J Prev Cardiol (2020) 27(10):1091–100. doi: 10.1177/2047487319887578
32. Hardman RL, Jazaeri O, Yi J, Smith M, Gupta R. Overview of classification systems in peripheral artery disease. Semin Intervent Radiol (2014) 31(4):378–88. doi: 10.1055/s-0034-1393976
33. Kurtul BE, Ozer PA. Neutrophil-to-lymphocyte ratio in ocular diseases: a systematic review. Int J Ophthalmol (2019) 12(12):1951–8. doi: 10.18240/ijo.2019.12.18
34. Puangsri P, Ninla-Aesong P. Potential usefulness of complete blood count parameters and inflammatory ratios as simple biomarkers of depression and suicide risk in drug-naive, adolescents with major depressive disorder. Psychiatry Res (2021) 305:114216. doi: 10.1016/j.psychres.2021.114216
35. Jialal I, Jialal G, Adams-Huet B. The platelet to high density lipoprotein -cholesterol ratio is a valid biomarker of nascent metabolic syndrome. Diabetes Metab Res Rev (2021) 37(6). doi: 10.1002/dmrr.3403
36. Zhao Q, Cheng YJ, Xu YK, Zhao ZW, Liu C, Sun TN, et al. Comparison of various insulin resistance surrogates on prognostic prediction and stratification following percutaneous coronary intervention in patients with and without type 2 diabetes mellitus. Cardiovasc Diabetol (2021) 20(1):190. doi: 10.1186/s12933-021-01383-7
37. Ohnishi H, Saitoh S, Takagi S, Ohata J, Isobe T, Kikuchi Y, et al. Relationship between insulin-resistance and remnant-like particle cholesterol. Atherosclerosis (2002) 164(1):167–70. doi: 10.1002/dmrr.3403
38. Aday AW, Matsushita K. Epidemiology of peripheral artery disease and polyvascular disease. Circ Res (2021) 128(12):1818–32. doi: 10.1161/CIRCRESAHA.121.318535
39. Nordestgaard BG. Triglyceride-rich lipoproteins and atherosclerotic cardiovascular disease: New insights from epidemiology, genetics, and biology. Circ Res (2016) 118(4):547–63. doi: 10.1161/CIRCRESAHA.115.306249
40. Nordestgaard BG, Wootton R, Lewis B. Selective retention of VLDL, IDL, and LDL in the arterial intima of genetically hyperlipidemic rabbits in vivo. molecular size as a determinant of fractional loss from the intima-inner media. Arterioscler Thromb Vasc Biol (1995) 15(4):534–42. doi: 10.1161/01.ATV.15.4.534
41. Nakamura T, Takano H, Umetani K, Kawabata Ki, Obata J, Kitta Y, et al. Remnant lipoproteinemia is a risk factor for endothelial vasomotor dysfunction and coronary artery disease in metabolic syndrome. Atherosclerosis (2005) 181(2):321–7. doi: 10.1016/j.atherosclerosis.2005.01.012
42. Doi H, Kugiyama K, Oka H, Sugiyama S, Ogata N, Koide Si, et al. Remnant lipoproteins induce proatherothrombogenic molecules in endothelial cells through a redox-sensitive mechanism. Circulation (2000) 102(6):670–6. doi: 10.1161/01.CIR.102.6.670
43. Li L, Sawamura T, Renier G. Glucose enhances endothelial LOX-1 expression: role for LOX-1 in glucose-induced human monocyte adhesion to endothelium. Diabetes (2003) 52(7):1843–50. doi: 10.2337/diabetes.52.7.1843
44. Shin HK, Kim YK, Kim KY, Lee JH, Hong KW. Remnant lipoprotein particles induce apoptosis in endothelial cells by NAD(P)H oxidase-mediated production of superoxide and cytokines via lectin-like oxidized low-density lipoprotein receptor-1 activation: Prevention by cilostazol. Circulation (2004) 109(8):1022–8. doi: 10.1161/01.CIR.0000117403.64398.53
45. Aramaki Y, Mitsuoka H, Toyohara M, Jinnai T, Kanatani K, Nakajima K, et al. Lectin-like oxidized LDL receptor-1 (LOX-1) acts as a receptor for remnant-like lipoprotein particles (RLPs) and mediates RLP-induced migration of vascular smooth muscle cells. Atherosclerosis (2008) 198(2):272–9. doi: 10.1016/j.atherosclerosis.2007.12.017
46. Duran EK, Aday AW, Cook NR, Buring JE, Ridker PM, Pradhan AD. Triglyceride-rich lipoprotein cholesterol, small dense LDL cholesterol, and incident cardiovascular disease. J Am Coll Cardiol (2020) 75(17):2122–35. doi: 10.1016/j.jacc.2020.02.059
47. Varbo A, Freiberg JJ, Nordestgaard BG. Remnant cholesterol and myocardial infarction in normal weight, overweight, and obese individuals from the Copenhagen general population study. Clin Chem (2018) 64(1):219–30. doi: 10.1373/clinchem.2017.279463
48. Varbo A, Nordestgaard BG. Directly measured vs. calculated remnant cholesterol identifies additional overlooked individuals in the general population at higher risk of myocardial infarction. Eur Heart J (2021) 42(47):4833–43. doi: 10.1016/j.jacc.2020.02.059
Keywords: type 2 diabetes, peripheral artery disease, remnant cholesterol, risk factor, lipid
Citation: Song Y, Zhao Y, Bai X, Cheng W, Wang L, Shu M, Shu Y, Zhang L and Jin S (2023) Remnant cholesterol is independently asssociated with an increased risk of peripheral artery disease in type 2 diabetic patients. Front. Endocrinol. 14:1111152. doi: 10.3389/fendo.2023.1111152
Received: 29 November 2022; Accepted: 06 February 2023;
Published: 15 February 2023.
Edited by:
Maurizio Delvecchio, Giovanni XXIII Children’s Hospital, ItalyReviewed by:
Arup Acharjee, Allahabad University, IndiaCopyright © 2023 Song, Zhao, Bai, Cheng, Wang, Shu, Shu, Zhang and Jin. This is an open-access article distributed under the terms of the Creative Commons Attribution License (CC BY). The use, distribution or reproduction in other forums is permitted, provided the original author(s) and the copyright owner(s) are credited and that the original publication in this journal is cited, in accordance with accepted academic practice. No use, distribution or reproduction is permitted which does not comply with these terms.
*Correspondence: Si Jin, Smluc2lAaHVzdC5lZHUuY24=
Disclaimer: All claims expressed in this article are solely those of the authors and do not necessarily represent those of their affiliated organizations, or those of the publisher, the editors and the reviewers. Any product that may be evaluated in this article or claim that may be made by its manufacturer is not guaranteed or endorsed by the publisher.
Research integrity at Frontiers
Learn more about the work of our research integrity team to safeguard the quality of each article we publish.