- 1Department of Biochemistry and Molecular Biology, University of the Basque Country UPV/EHU, Leioa, Spain
- 2Biocruces Bizkaia Health Research Institute, Barakaldo, Spain
- 3Department of Genetics, Physical Anthropology and Animal Physiology, University of the Basque Country, Leioa, Spain
- 4Institut d’Investigació Biomèdica de Girona, Girona, Spain
- 5CIBER Fisiopatología de la Obesidad y Nutrición (CIBERobn), Instituto de Salud Carlos III, Madrid, Spain
- 6ULB Center for Diabetes Research, Université Libre de Bruxelles, Brussels, Belgium
- 7Health Research Institute of the Principality of Asturias (ISPA), Oviedo, Spain
- 8University of Barcelona, Barcelona, Spain
- 9Endocrinology and Nutrition Department, Central University Hospital of Asturias (HUCA), Oviedo, Spain
- 10Department of Medicine, University of Oviedo, Oviedo, Spain
- 11Department of Clinical and Experimental Medicine, Cisanello University Hospital, Pisa, Italy
- 12Division of Endocrinology, Erasmus Hospital, Université Libre de Bruxelles, Brussels, Belgium
- 13Spanish Biomedical Research Network in Rare Diseases (CIBERER), Madrid, Spain
- 14Department of Medical Sciences, School of Medicine, University of Girona, Oviedo, Spain
- 15Diabetes and Associated Metabolic Diseases Networking Biomedical Research Centre, Madrid, Spain
- 16Ikerbasque - Basque Foundation for Science, Bilbao, Spain
Introduction: Most of the disease-associated single nucleotide polymorphisms (SNPs) lie in non- coding regions of the human genome. Many of these variants have been predicted to impact the expression and function of long non-coding RNAs (lncRNA), but the contribution of these molecules to the development of complex diseases remains to be clarified.
Methods: Here, we performed a genetic association study between a SNP located in a lncRNA known as LncTGM2 and the risk of developing type 2 diabetes (T2D), and analyzed its implication in disease pathogenesis at pancreatic beta cell level. Genetic association study was performed on human samples linking the rs2076380 polymorphism with T2D and glycemic traits. The pancreatic beta cell line EndoC-bH1 was employed for functional studies based on LncTGM2 silencing and overexpression experiments. Human pancreatic islets were used for eQTL analysis.
Results: We have identified a genetic association between LncTGM2 and T2D risk. Functional characterization of the LncTGM2 revealed its implication in the transcriptional regulation of TGM2, coding for a transglutaminase. The T2Dassociated risk allele in LncTGM2 disrupts the secondary structure of this lncRNA, affecting its stability and the expression of TGM2 in pancreatic beta cells. Diminished LncTGM2 in human beta cells impairs glucose-stimulated insulin release.
Conclusions: These findings provide novel information on the molecular mechanisms by which T2D-associated SNPs in lncRNAs may contribute to disease, paving the way for the development of new therapies based on the modulation of lncRNAs.
1 Introduction
Type 2 diabetes (T2D) is a complex metabolic disease that develops in genetically susceptible individuals (1). Indeed, the trigger of T2D development is presumed to be a combination of lifestyle and environmental factors working together with the genetic background (2). Genome-wide association studies (GWAS) have identified several genomic regions associated with the risk of T2D (3). Although these studies have provided a better understanding of T2D genetics, most of the genetic variants identified so far fall into non-coding regions of the genome. The molecular mechanism by which these variants increase risk of T2D remains to be clarified.
Transglutaminase 2 (TGM2) is a calcium-dependent multifunctional enzyme that can act as GTPase or transamidase, and that participates in several cellular processes, including apoptosis, cell adhesion or insulin release, among others (4). Disruption of TGM2 in mice has been associated with increased glucose levels, and reduced insulin release in response to glucose (5). In addition, missense mutations in TGM2 have been associated with early onset T2D and maturity onset diabetes of the young (MODY) (6).
A recent study identified a lncRNA (LOC107987281 or LncTMG2) located within the first intron of the TGM2 gene. The same study revealed that the expression of the lncRNA was tightly correlated with the expression of the TGM2 coding gene in several cell lines and tumor tissues, suggesting its role as a cis acting transcriptional regulatory lncRNA (7).
LncRNAs are non-coding RNA molecules of more than 200 nucleotides in lenght that participate in several cellular and biological processes, including transcriptional regulation (8). Most of the complex disease-associated variants are located in non-coding regions of the human genome, and more specifically, in lncRNAs. The presence of disease-associated single nucleotide polymorphism (SNPs) in exonic regions of lncRNAs usually disrupt their secondary structure, affecting their capacity to interact with other macromolecules, and eventually altering their function (9). Although the function of most lncRNAs has not been annotated yet, there is already accumulating evidence of their implication in the development of several diseases, including metabolic disorders (10–12).
In the present work, we have described a genetic association between a SNP located in the coding sequence of LncTGM2 and T2D and related traits. In addition, we have characterized the relation between LncTGM2 and TGM2 in pancreatic beta cells and unveiled the mechanisms by which LncTGM2 might induce beta cell dysfunction in T2D.
2 Materials and methods
2.1 Association study
Cohort 1 consisted of 725 individuals (47 ± 11 years, 54% men) recruited in the northwest of Spain, including general population, and obesity and diabetes outpatient clinics in which the percentage of obese individuals was 72% and the percentage of type 2 diabetic individuals was 11% (13). Cohort 2 included 616 Caucasian subjects selected for a study of non-classic cardiovascular risk factors performed in the northwest of Spain (Asturias) (14). Participants (52 ± 12 years, 45% men, 26% obesity, 11% T2D) were randomly identified from a census and invited to participate.
Clinical characterization of human cohorts included a standardized questionnaire, physical examination and the performance of routine laboratory tests. Height and weight were measured by trained personnel using calibrated scales and a wall-mounted stadiometer, respectively, and with the participant in light clothing and without shoes. Body mass index (BMI) was calculated by dividing weight in kilograms by the square of the height in meters (kg/m2). Obesity was set at BMI≥30 kg/m2. The waist of the subjects was measured with a soft tape midway between the lowest rib and the iliac crest, hip circumference was measured at the widest part of the gluteal region, and waist-to-hip ratio was then calculated. Together with clinically relevant information and subsidiary data, the number of cigarettes/day (if any) and the use of hormonal contraceptives were recorded. In those participants that agree (>75%), oral glucose tolerance test (OGTT) was performed to measure glucose tolerance. Blood samples from all the participants were collected, and after 15 minutes, tubes were centrifuged at 4,000 r.p.m. at room temperature. The serum and peripheral blood leukocytes were separated and immediately frozen at –80°C. Genomic DNA was extracted from blood samples following standard purification methods (QIAamp DNA Blood Mini Kit, Qiagen, Hilden, Germany) and DNA quantity and purity was determined using a spectrophotometer (GeneQuant, GE Health Care, Piscataway, USA). The targeted single nucleotide polymorphism (SNP) rs2076380 was genotyped by means of a predesigned rhAmp™ allelic discrimination assay (Hs.GT.rs2076380.A.1; Thermo Fisher Scientific, Massachusetts, USA) and the rhAmp Genotyping Master Mix (IDT, Coralville, USA), using a LightCycler 480 RT-qPCR System sequence detector (Roche Diagnostics, Barcelona, Spain). Replicates and positive and negative controls were included in all reactions.
2.2 Cell cultures and human cDNA samples
The EndoC-βH1 human pancreatic cell line (Univercell Biosolutions, Paris, France) was cultured in plates coated with Matrigel-fibronectin (100 mg/ml and 2 mg/ml, respectively; Sigma-Aldrich, Burlington, USA) in Opti-β1 medium (Univercell Biosolutions). DMEM containing 5.6 mmol/l glucose, 2% vol/vol Fetal Bovine Serum, 50 μmol/l 2-mercaptoethanol (Bio-Rad, Hercules, USA), 10 mmol/l nicotinamide (Calbiochem, Darmstadt, Germany), 5.5 μg/ml transferrin and 6.7 ng/ml selenite (Sigma-Aldrich) was used for transfection.
EndoC-βH1 cell line was Mycoplasma free as determined by the MycoAlert Mycoplasma Detection kit (Lonza). For the prevention of Mycoplasma contamination, Plasmocin Prophylactic (Invivogen, Toulouse, France) was added to the culture medium on a regular basis.
cDNA samples from human pancreatic islets were obtained from Cisanello University Hospital, Pisa, Italy. All the islets were isolated and cultured using the same experimental conditions and following established isolation procedures (15). Characteristics of islet preparations are described in Table S1. The Ethical Committee of Cisanello University Hospital approved experiments using human islets.
2.3 Silencing experiments
LncTGM2 silencing in the EndoC-βH1 cell line was performed by transfecting 30 nmol/l of a siRNA targeting LncTGM2 (CD.Ri.214258.13.13, IDT) using Lipofectamine RNAimax reagent (Thermo Fisher Scientific) following the manufacturer’s instructions.
2.4 Plasmid construction and transfection
For overexpressing plasmids, LncTGM2 was purchased as a gBlock (IDT) and cloned into a modified pCMV6 vector using KpnI and FseI restriction enzymes (New England Biolabs, Ipswich, USA). Plasmids were transfected using Lipofectamine 2000 Transfection Reagent (Invitrogen, Carlsbad, USA) following the manufacturer’s instructions.
2.5 Cell treatments
EndoC-βH1 cells were exposed to Actinomycin D (Sigma-Aldrich) at a final concentration of 5 μg/ml for 2, 4 or 6h. Palmitate treatment was performed by adding BSA-palmitic acid (0.5 mmol/l; 1:1) to DMEM/F-12, complemented with 0.25% vol/vol FBS, 50 μmol/l 2-mercaptoethanol (Bio-Rad), 10 mmol/l nicotinamide (Calbiochem), 5.5 μg/ml transferrin, 6.7 ng/ml selenite (Sigma-Aldrich), 100 units/ml penicillin and 100 μg/ml streptomycin (Lonza) for 4 or 8h.
2.6 Cellular fractionation
For LncTGM2 RNA quantification in subcellular fractions of EndoC-βH1 cells, nuclei were isolated using C1 lysis buffer (1.28 mol/l sucrose, 40 mmol/l Tris -HCl pH 7.5, 20 mmol/l MgCl2, 4% vol/vol Triton X-100). LncTGM2, MEG3 (nuclear control) and RPLP0 (cytoplasmic control) expression levels were measured by RT-qPCR and compared to the total amount of those RNAs in the whole cell lysate.
2.7 RNA isolation and RT-qPCR
RNA extraction was performed using the NucleoSpin RNA Kit (Macherey Nagel, Düren Germany) and expression values were determined by RT-qPCR using iTaq Universal SYBR Green Supermix (Bio-Rad) using specific primers for each target RNA (Table S2). All RT-qPCR measurements were performed in duplicates and expression levels were analyzed using the 2–ΔΔCt method. A commercially available RNA panel set (Human total RNA master panel II, Clontech, Saint-Germain-en-Laye, France) was used to assess LncTGM2 and TGM2 expression levels in different human tissues.
2.8 Western blot analysis
EndoC-βH1 cells were washed with cold PBS and lysed in Laemmli buffer (62 mmol/l Tris-HCl, 100 mmol/l dithiothreitol (DTT), 10% vol/vol glycerol, 2% wt/vol SDS, 0.2 mg/ml bromophenol blue, 5% vol/vol 2-mercaptoethanol). Proteins in the lysate were separated by SDS-PAGE. After electrophoresis, proteins were transferred to nitrocellulose membranes using a Transblot-Turbo Transfer System (Bio-Rad) and blocked in 5% wt/vol non-fatty milk diluted in TBST (20 mmol/l Tris, 150 mmol/l NaCl and 0.1% vol/vol Tween 20) at room temperature for 1h. The membranes were incubated overnight at 4°C with a primary antibody specific for TGM2 (15100-1-AP, Proteintech Group, Rosemont, USA) diluted 1:1000 in 5% wt/vol BSA or anti-α-tubulin (Cat #T9026, Sigma-Aldrich) diluted 1:5000 in 5% wt/vol BSA. Immunoreactive bands were revealed using the Clarity Max Western ECL Substrate (Bio-Rad) after incubation with a horseradish peroxidase-conjugated anti-rabbit (1:1000 dilution in 5% wt/vol non-fatty milk) or anti-mouse (1:5000 dilution in 5% wt/vol non-fatty milk) secondary antibody for 1h at room temperature. The immunoreactive bands were detected using a Bio-Rad Molecular Imager ChemiDoc XRS and quantified using ImageLab software (Bio-Rad).
2.9 TGM2 promoter reporter assay
TGM2 promoter sequence was cloned into an empty pBV-Luc plasmid (Addgene, Watertown, USA) using KpnI and EcoRI restriction enzymes. EndoC-βH1 cells were transfected with a control vector (ovCTRL) or a vector overexpressing LncTGM2 (ovLncTGM2), and co-transfected with the TGM2 promoter reporter vector plus a pRL-CMV plasmid (used as an internal control) using Lipofectamine 2000 Transfection Reagent (Invitrogen). Dual-Luciferase Reporter Assay System (Promega, Madison, USA), was used to measure bioluminescence following the manufacturer’s protocol.
2.10 In silico secondary structure prediction
Secondary structure of LncTGM2 harboring the different alleles of rs2076380, rs7275079 and rs2067027 SNPs was predicted using the RNAsnp Web Server tool (16).
2.11 RNA mobility shift assay
LncTGM2 harboring rs2076380-A or rs2076380-G alleles were in vitro transcribed using T7 RNA Polymerase kit (TaKaRa, Kusatsu, Japan). RNAs were run in a native TBE 2% wt/vol agarose gel and migration profile was analyzed in a ChemiDoc XRS apparatus (Bio-Rad).
2.12 Insulin release
For insulin release experiments, LncTGM2-silenced EndoC-βH1 were left in Opti-β2 (Univercell Biosolutions) starving medium for 24h. After glucose starvation, cells were incubated in KREBS medium (Univercell Biosolutions) for 1h, and consecutively exposed to 0 or 20 mmol/l glucose for 40 minutes. Supernatant and lysate were harvested and insulin release and content measured by a commercial human insulin ELISA kit (Mercodia, Uppsala, USA) according to the manufacturer’s instructions.
2.13 Statistics
The association between the rs2076380 single variation in the TGM2 gene, clinical parameters and the risk of T2D was assessed using SPSS Statistics (IBM). Departures from Hardy-Weinberg equilibrium were tested in all groups using a chi-square goodness of fit test with one degree of freedom. The risk of developing T2D under exposure to rs2076380 TGM2 genotypes was evaluated using logistic regression to estimate Odd Ratios (OR), considering a dominant model in which G-allele carriers (i.e., AG-heterozygotes plus GG-homozygotes) were the reference group. To compare groups with respect to continuous variables, one-way ANOVA for multiple comparisons was used. Other statistical tests and plots were performed using GraphPad Prism 8 software (Dotmatics). Significance-level was set at p-value <0.05. Results for in vitro functional studies are represented as means ± standard error of mean (S.E.M.).
3 Results
3.1 An exonic SNP in LncTGM2 is associated with T2D risk
In order to determine the potential association of LncTGM2 with T2D clinical parameters, we performed an association study by genotyping a SNP located in the exonic region of LncTGM2 (rs2076380; chr20:38,165,027-38,165,227, hg38). This SNP can be considered as a tagSNP since it is in high linkage disequilibrium (LD>0.8) with other SNPs in the region (Figure S1). The LncTGM2 SNP rs2076380 was tested in association with measures of T2D and other metabolic and clinical parameters in two independent cohorts (Table S3). In cohort 1, the frequency of AA-individuals for the LncTGM2 SNP was 8.6%, similarly to the observed frequency in Cohort 2 (8.3%). These frequencies are in line with the observed frequency of the minor allele (A) in Caucasian populations (1000 Genomes Europe; A allele frequency = 0.32) (17) and Spanish control individuals (Medical Genome Project healthy controls from Spanish population; A allele frequency = 0.225) (18).
As observed in Figure 1, the percentage of known type 2 diabetic individuals was increased in individuals harboring the rs2076380-AA genotype in both cohorts (Cohort 1: OR=1.13 [0.999-1.27], Pearson’s Chi-square p=0.006, two-sided Fisher’s exact test p=0.013); and Cohort 2: OR=1.08 [0.996-1.18], Pearson’s Chi-square p=0.018, two-sided Fisher’s exact test p=0.026). For both cohorts, regression analyses depicted the impact of the polymorphism in LncTGM2 on T2D incidence (ANOVA p-value of 0.026 in Cohort 1, and p=0.013 in Cohort 2) after correcting for sex and age. A codominant genetic model that included age, weight and sex effects was fitted to estimate the ORs between the exposure to the AA, AG and GG genotypes, the later as the reference group. The similar ORs for AG and GG genotypes obtained for the codominant model suggested the possibility of fitting a recessive model for AA-genotype carriers. This model allowed us to determine the OR between carriers of the AA genotype in relation to the G-allele porters. In this case, the residual deviance of the genotype, once age, weight and sex were added to the model, reached a p-value <0.05, indicating that the genotype effect was significant.
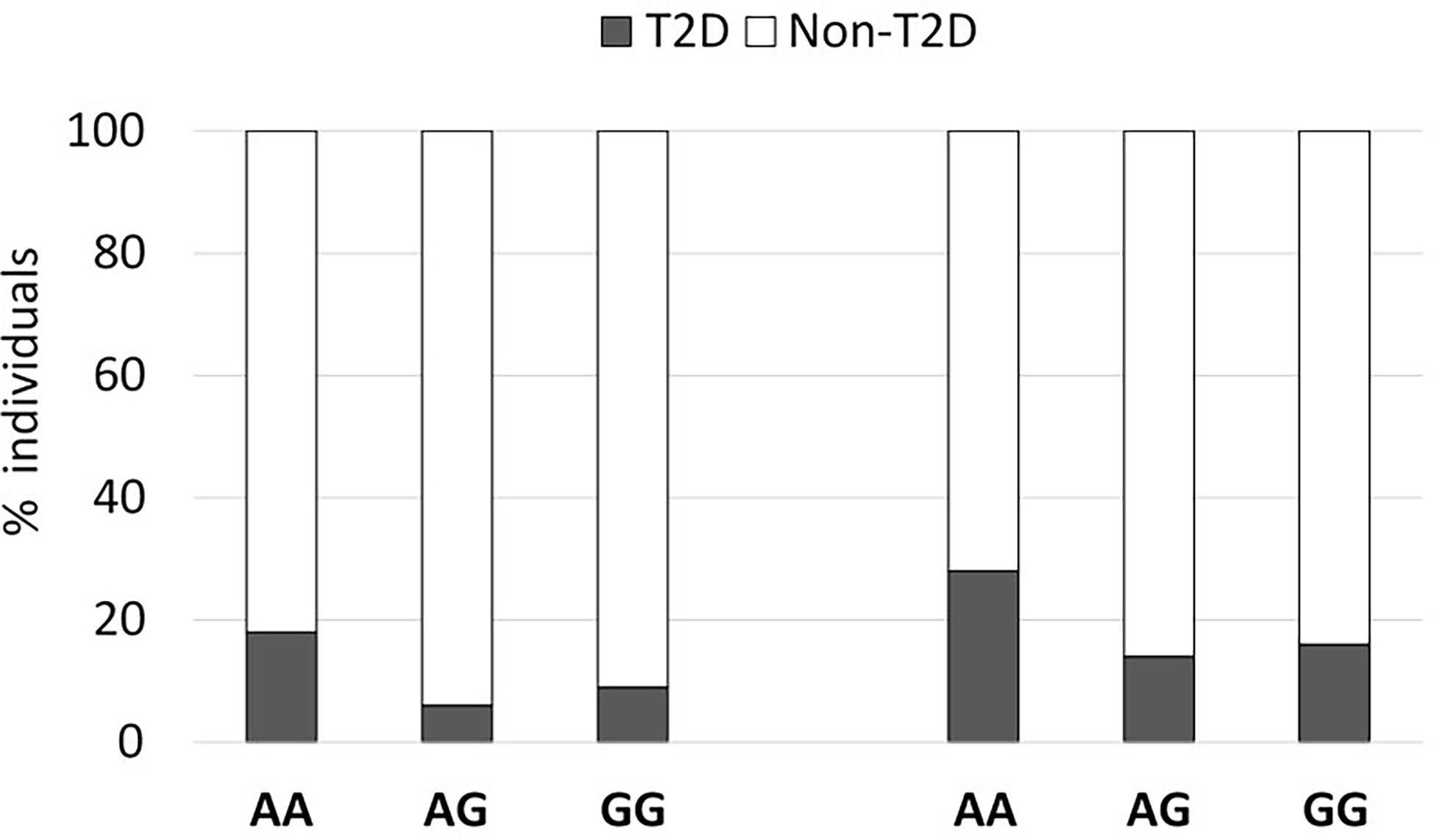
Figure 1 An exonic SNP in LncTGM2 is associated with T2D risk. The graph shows the percent of T2D in two independent cohorts segregated according to their rs2076380 genotype.
In addition, we observed that in Cohort 1, fasting glucose (p=0.005) and insulin levels (p=0.006) were increased compared to G-allele carriers (Table S3). However, in Cohort 2, association with fasting glucose only reached statistical significance in female participants (Table S3).
3.2 LncTGM2 expression is correlated with TGM2 expression in several human tissues and regulated by lipotoxicity in pancreatic beta cells
Previous studies have correlated LncTGM2 and TGM2 expression in tumor tissues and some human cell lines, including lymphoblast (K562), promyeoloblast (HL60) and monocyte (THP-1) cell lines (7). In order to clarify whether LncTGM2 and TGM2 expression was also correlated in healthy human tissues and in pancreatic beta cells, we first evaluated the expression of both genes in EndoC-βH1 cells and a set of human tissues. The highest expression of both, LncTGM2 and TGM2, was found in lung, placenta and heart, and the expression in the EndoC-βH1 cell line was similar to that of intestine and liver (Figure S2). Spearman’s correlation analysis showed a significant correlation between LncTGM2 and TGM2 expression across the tissues analyzed (R=0.87 (0.59-0.9); p<0.0001). Interestingly, a correlation was also seen in EndoC-βH1 cells using siRNA-driven inhibition of LncTGM2. As shown in Figure 2A, a 70% decrease of LncTGM2 expression reduced TGM2 mRNA expression by 20%, suggesting a potential implication of LncTGM2 in the transcriptional regulation of TGM2.
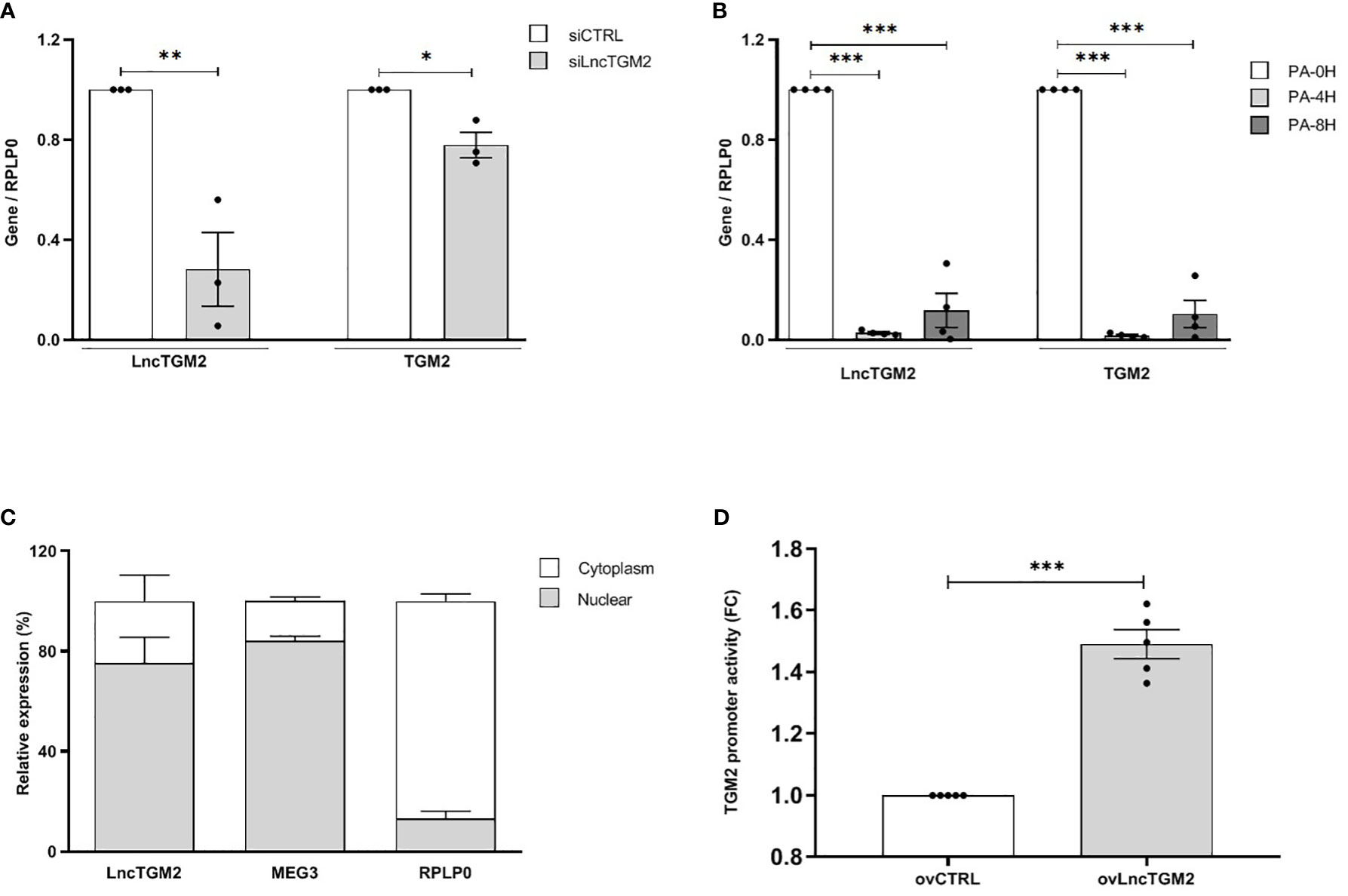
Figure 2 LncTGM2 co-expresses with TGM2 and regulates its transcriptional activity in pancreatic beta cells. (A) LncTGM2 was silenced in the EndoC-βH1 cell line using a siRNA, and (B) EndoC-βH1 cells were exposed to palmitate (0.5 mM) for 4 or 8h. LncTGM2 and TGM2 expression was assessed by RT-qPCR and normalized by the reference gene RPLP0. The results are means ± S.E.M. of 3-4 independent experiments; *p < 0.05, **p < 0.01, and ***p < 0.001 by Student’s t-test. (C) RT-qPCR analysis of LncTGM2, MEG3 (as nuclear marker) and RPLP0 (as cytoplasmic marker) in nuclear and cytoplasmic fractions in EndoC-βH1 cells. (D) HEK-293 cells were transfected with a control vector (ovCTRL) or a vector overexpressing LncTGM2 (ovLncTGM2), and co-transfected with a TGM2 promoter luciferase reporter construct plus a pRL-CMV plasmid (used as internal control). After 48h of recovery, bioluminescence was measured.
In order to simulate the pathophysiological conditions of T2D in pancreatic beta cells, we next exposed EndoC-βH1 cells to palmitate (PA) as an in vitro model of lipotoxicity (19). As shown in Figure 2B, 4 and 8h PA exposure decreased both LncTGM2 and TGM2 expression in EndoC-βH1 cells, suggesting that in the presence of a lipotoxic insult the expression of both genes is reduced.
3.3 LncTMG2 regulates the transcriptional activity of TGM2
Knowledge of the subcellular localization of lncRNAs is crucial to understand and characterize their function. In contrast to protein-coding mRNAs, lncRNA themselves should be located in their site of action, and thus, their location within the cell is crucial for their function. While nuclear lncRNAs are usually implicated in the regulation of transcriptional activity, cytoplasmic lncRNAs can participate for example, in the regulation of mRNA stability or in protein translation (20). Having this in mind, we next decided to analyze the subcellular localization of LncTGM2 in EndoC-βH1 cells. As shown in Figure 2C, LncTGM2 was detected in both nuclear and cytoplasmic fractions, but its expression level was significantly higher in the nuclear compartment, suggesting its potential implication in transcriptional regulation. Since expression of LncTGM2 and TGM2 was significantly correlated in pancreatic beta cells, we performed a promoter reporter assay to clarify whether LncTGM2 was directly regulating the promoter activation of TGM2 gene. To this aim, we constructed an expression vector coding for a luciferase under the control of the promoter of TGM2. The luciferase vector was then co-transfected in EndoC-βH1 cells with an empty overexpression plasmid (ovCTRL) or with the overexpression plasmid of LncTGM2 (ovLncTGM2) and the activation of TGM2 promoter was determined by measuring bioluminiscence. As shown in Figure 2D, the activation of the TGM2 promoter was 1.5-fold higher in LncTGM2-overexpressing cells than in control cells, pointing out a role of LncTGM2 in the activation of TGM2 promoter, and consequently in the transcriptional activation of TGM2.
3.4 The T2D-associated risk allele in LncTGM2 disrupts its secondary structure impacting on its stability, and correlates with decreased expression of TGM2 in beta cells
Disease-associated SNPs located within lncRNAs can affect their function through the disruption of their secondary structure (21, 22). As previously shown (Figure S1), the T2D-associated rs2076380 SNP is in high LD with other two SNPs located in the exonic region of LncTGM2 (rs7275079 and rs2067027). To assess whether these SNPs alter the secondary structure of LncTGM2, we performed an in silico prediction analysis using the RNAsnp webserver from the Center for non-coding RNA in Technology and Health (23). Interestingly, rs2076380 was predicted to significantly alter the secondary structure of LncTGM2 (p=0.0803), while the software did not predict any significant change in the structure of the lncRNA when the different alleles of rs7275079 or rs2067027 SNPs were present (p>0.2) (data not shown). As shown in Figure 3A, the predicted secondary structures of LncTGM2 carrying the T2D protective (rs2076380-G) or risk allele (rs2076380-A) were significantly different. Consistent with the prediction, in vitro–transcribed forms of T2D protective and risk allele–harboring LncTGM2 revealed different motilities on a native agarose gel (Figure 3B), suggesting a different conformation of the lncRNA in the presence of one or other allele in rs2076380.
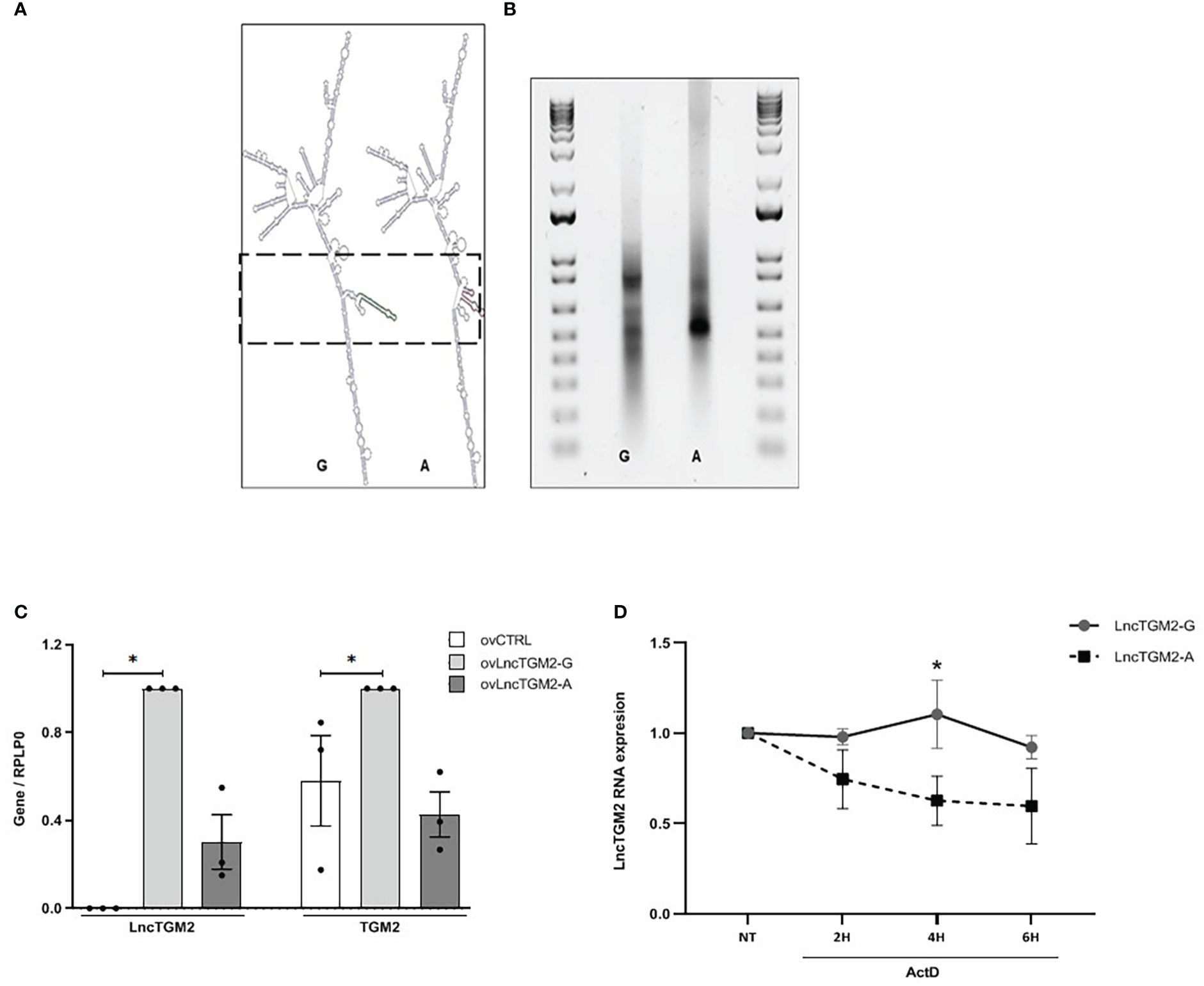
Figure 3 The T2D-associated risk allele in LncTGM2 disrupts its secondary structure impacting on its stability, and correlates with decreased expression of TGM2 in beta cells. (A) In silico prediction of the secondary structure of LncTGM2 harboring each allele for rs2076380; T2D protective allele (G) or T2D risk allele (A). (B) Electrophoretic mobility profiles of in vitro-transcribed LncTGM2 molecule harboring the T2D protective allele (rs2076380-G) or the risk allele (rs2076380-A). (C) EndoC-βH1 cells were transfected with overexpression plasmids of LncTGM2 harboring the protective (ovLncTGM2-G) or risk allele (ovLncTGM2-A) for T2D, and mRNA levels of LncTGM2 and TGM2 were determined by RT-qPCR and normalized to RPLP0. The results are means ± S.E.M. of 3 independent experiments; *p < 0.05 by Student’s t-test. (D) EndoC-βH1 cells were transfected with LncTGM2 overexpression plasmids harboring the protective (ovLncTGM2-G) or risk allele (ovLncTGM2-A) for T2D. EndoC-βH1 cells were exposed to Actinomycin D (ActD) (5 μg/ml) for 2, 4 or 6h and LncTGM2 mRNA level was determined by RT-qPCR. The results are means ± S.E.M. of 3 independent experiments. *p < 0.05 ovLncTGM2-G vs. ovLncTGM2-A at the same time-point.
Taking into account that the secondary structure of a lncRNA is crucial for its interaction with other macromolecules, and thus, for its function (9), we next decided to determine whether the genotype of the T2D-associated SNP in LncTGM2 affected TGM2 expression in human pancreatic islets. To this aim we genotyped rs2076380 SNP and measured TGM2 expression in 16 cDNA samples from human islets, and performed an eQTL analysis. As shown in Figure S3, there was a trend for higher expression of TGM2 in islets harboring the protective rs2076380-GG genotype compared to islets harboring the risk allele in heterozygosis (rs2076380-AG) or homozygosis (rs2076380-AA), although the differences did not reach statistical significance, probably due to the limited number of islets.
Next, to characterize the potential effect of each allele in rs2076380 SNP on the expression of both, LncTGM2 and TGM2, we constructed two LncTGM2 overexpression plasmids, one harboring the T2D risk allele (ovLncTGM2-A), and the other harboring the T2D protective allele (ovLncTGM2-G). Interestingly, allele-specific upregulation of LncTGM2 in beta cells revealed that the expression level reached by transfecting ovLncTGM2-G plasmid was higher than the expression level obtained with ovLncTGM2-A plasmid (Figure 3C), suggesting that the T2D risk allele might be affecting the stability of LncTGM2 RNA molecule.
In order to directly test whether the T2D-associated polymorphism affected LncTGM2 stability, we next performed an allele-specific overexpression of LncTGM2 and exposed the EndoCβ-H1 cells to Actinomycin D, a drug that inhibits transcription. As shown in Figure 3D, LncTGM2 harboring the protective allele (rs2076380-G) was more stable than the lncRNA harboring the risk allele (rs2076380-A) at all time-points, although the differences only reached statistical significance at 4h of Actinomycin D treatment (p<0.05). These results confirmed that the LncTGM2 risk allele in the T2D-associated rs2076380 SNP reduced the stability of the lncRNA.
To clarify whether the decreased stability of LncTGM2-A affected its capacity to regulate TGM2 expression, we next analyzed the expression of TGM2 in EndoC-βH1 cells overexpressing LncTGM2-A or LncTGM2-G. As observed in Figure 3C, only the upregulation of the lncRNA harboring the protective allele (ovLncTGM2-G) increased the expression of TGM2 mRNA. These results were also confirmed at the protein level (Figure S4).
In summary, these results suggested that the LncTGM2 harboring the T2D risk allele induced less TGM2 expression due to its reduced stability.
3.5 LncTGM2 downregulation affects glucose-stimulated insulin secretion
Previous studies have shown that TGM2 might be implicated in insulin release through different mechanisms, including cytoplasmic actin remodeling and regulation of the action of other proteins during granule movement (24). Taking into account that our present results suggest that the T2D risk allele in LncTGM2 might induce a decrease in TGM2 expression in pancreatic beta cells, we next decided to determine the potential contribution of LncTGM2 in insulin release.
To this aim, we silenced LncTGM2 with a specific siRNA in EndoC-βH1 cells and determined glucose-stimulated insulin release. As shown in Figure 4, high glucose stimulation in siCTRL-transfected EndoC-βH1 cells increased insulin secretion. In siLncTGM2-transfected beta cells, however, high glucose-induced insulin secretion (GSIS) was no longer statistically significant, suggesting that disruption of LncTGM2 in pancreatic beta cell might affect GSIS through diminished expression of TGM2.
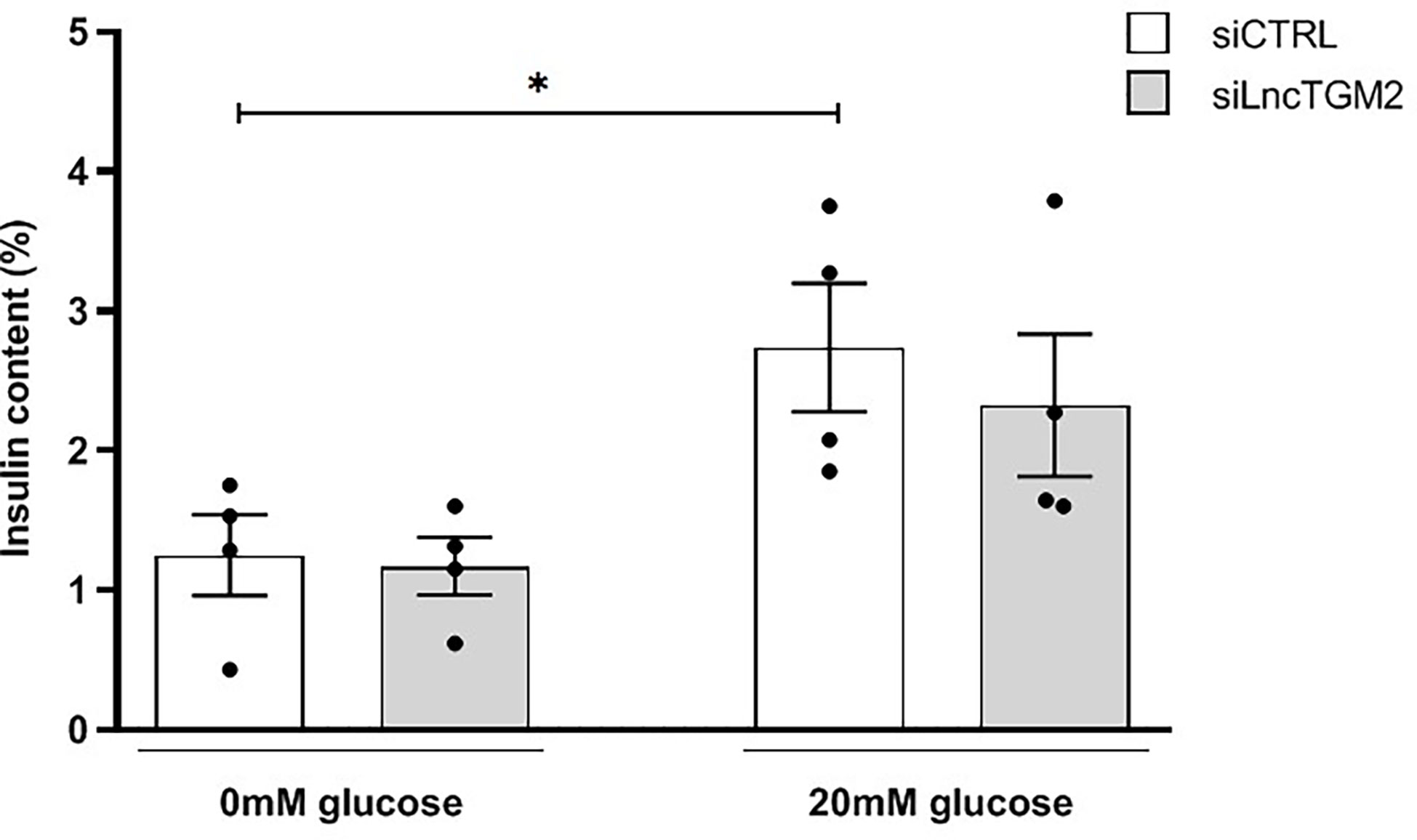
Figure 4 LncTGM2 downregulation affects glucose-stimulated insulin secretion. LncTGM2 was silenced using a siRNA and EndoC-βH1 cells were exposed to 0 or 20 mmol/l glucose. Insulin release was determined by ELISA. The results are means ± S.E.M. of 4 independent experiments; *p<0.05 by Student’s t-test.
4 Discussion
In the current study, we identified a genetic association between LncTGM2 and T2D and glycemic traits in two independent cohorts. Previous GWAS in larger Caucasian populations have not detected a genetic association between rs2076380 and T2D, however based on phenotype-wide association data (T2D knowledge portal), this polymorphism has been associated with T2D-related complications (e.g. microalbuminuria). Moreover, based on the T2D knowledge portal, the genomic region in which LncTGM2 is located (also containing TGM2, RPRD1B and KIAA1755 genes) has been associated with several metabolic and glycemic traits, including cardiovascular disease related parameters, cholesterol and type 2 diabetes. The main reason for the discordance between our findings and GWAS data may lie on the fact that our two cohorts are enriched for obese individuals (especially Cohort 1), and in our both cohorts, T2D incidence seem to be associated with obesity (data not shown). In this sense, several studies have described a link between TGM2 and obesity and associated glycemic traits. For example, a study found that loss of TGM2 sensitizes for diet-induced obesity-related inflammation and insulin resistance (25). Moreover, a network-based approach to assess the cellular processes associated with protein–protein interaction subnetworks of glycemic traits showed that TGM2 was associated with both, HOMA-β and HOMA-IR, suggesting a potential role of this protein in pancreatic beta cell function and insulin resistance (26). The same study concluded that HOMA-β-associated GWAS genes (which include TGM2) enriched pathways of fat metabolism, especially in adipose tissues, supporting the “lipotoxicity theory” of beta cell failure in T2D.
In line with this hypothesis, in the present study, we have observed a co-expression between LncTGM2 and the coding gene TGM2 in pancreatic beta cells under basal and lipotoxic conditions. Our data suggest that lipotoxicity, a typical feature of obesity-associated T2D, reduces LncTGM2, which in turn provokes a reduction of TGM2 in pancreatic beta cells. Indeed, lipotoxicity (e.g. high fat diet) has been previously associated with TGM2 expression reduction in other tissues, including liver (27).
Moreover, we propose a mechanism by which LncTGM2 may affect glucose-stimulated insulin release through TGM2 expression reduction in an allele-specific manner. The lncRNA LncTGM2 lies within the first intron of the TGM2 gene (9), which encodes a multifunctional enzyme that has been implicated in the pathogenesis of early onset T2D and MODY (6). Interestingly, early onset T2D and MODY-associated TGM2 mutants have altered enzymatic activities, such as reduced transamidation and kinase activity that impact in glucose-stimulated insulin release (28). Transcriptional regulation of TGM2 is controlled by several transcription factors, including nuclear factor-kappa B, RA receptor/retinoid X receptor, liver X receptor and Sp1 (4). Here, we show for the first time that LncTGM2 participates in the transcriptional regulation of TGM2 in pancreatic beta cells. We observed that the T2D-associated risk allele in LncTGM2 correlates with a reduction of TGM2 expression in pancreatic beta cells. Moreover, our results suggest that a reduction in TGM2 expression in human beta cells impair glucose-stimulated insulin release. These observations are in line with studies in rodents, in which reduced TGM2 activity has been linked to impaired glucose-stimulated insulin secretion (GSIS) (28), and also with data showing that naturally occurring mutations altering TGM2 enzymatic activities correlate with reduced insulin secretion (29). Interestingly, TGM2 has also been shown to interact with nuclear proteins (e.g. BAF and H3) immediately upon a glucose stimulus, suggesting that it may be involved not only in insulin secretion, but also in the regulation of glucose-induced gene transcription (30).
Although the molecular mechanisms by which LncTGM2 participates in the regulation of TGM2 transcription remain to be fully clarified, our results demonstrate that a T2D-associated polymorphism affects the secondary structure of the lncRNA, and, eventually, disrupts its function. Several other disease-associated SNPs that alter the secondary structure of lncRNAs affect the regulation of genes that participate in important pathways for disease pathogenesis, including type 1 diabetes and cardiovascular disease (31, 32). Here we demonstrate that the T2D risk allele in LncTGM2 reduces its stability, affecting TGM2 expression in pancreatic beta cells. Some studies have suggested that disease-associated SNPs in lncRNAs may affect RNA-turnover through disruption of the binding of proteins that regulate stability, and thus, affecting their biological function (33–35).
In conclusion, our results show that LncTGM2 is associated with T2D and suggest that it might be implicated in disease pathogenesis through an allele-specific downregulation of TGM2 in pancreatic beta cells. Our findings provide new information on the molecular mechanisms by which T2D-associated SNPs in lncRNAs cause disease and open the door to the development of novel diagnostic tools and therapeutic approaches based on lncRNA modulation.
Data availability statement
The original contributions presented in the study are included in the article/Supplementary Materials. Further inquiries can be directed to the corresponding authors.
Author contributions
IS and AC-R conceived and designed the study. FO and AL performed the genetic association studies. ED, JF-R, CL and JA-B coordinated human samples, clinical information, written consents and intellectual content collection. LM and PM provided the human pancreatic islet material. HR-M, IG-M, JM-S, MS-C, AO-G, FF, LM-M and MC designed and performed the experimental procedures. HR-M and IG-M wrote the paper. IS and AC-R reviewed the manuscript. All authors contributed to the article and approved the submitted version.
Funding
This work was supported by grants from the Ministerio de Ciencia, Innovación y Universidades (PID2019-104475GA-I00 to I.S, and PGC2018-097573-A-I00 to AC-R) and the European Foundation for the Study of Diabetes (EFSD) - EFSD/JDRF/Lilly Programme on Type 1 Diabetes Research to IS. FO (MS19/00109) is recipient of the Miguel Servet scheme, and AL (FI19/00045) was supported by the Instituto de Salud Carlos III (ISCIII); Ministerio de Ciencia, Innovación y Universidades, Gobierno de España (ES). HR-M (PRE2019-089350) is supported by predoctoral grant from the Ministerio de Ciencia, Innovacion y Universidades, Gobierno de España (ES) IG-M, MS-C, JM-S and AO-G were supported by Predoctoral Fellowship Grants from the UPV/EHU (Universidad del Pais Vasco/EuskalHerrikoUnibertsitatea) and the Basque Department of Education. MC is supported by the Fonds National de la RechercheScientifique (FNRS), the Francophone Foundation for Diabetes Research (sponsored by the French Diabetes Federation, Abbott, Eli Lilly, Merck Sharp & Dohme, and Novo Nordisk) and FF and MC by the EFSD/BoehringerIngelheim European Research Programme on Multi-System Challenges in Diabetes. The funders were not involved in the study design, collection, analysis, interpretation of data, the writing of this article, or the decision to submit it for publication.
Conflict of interest
The authors declare that this study received funding from the Francophone Foundation for Diabetes Research which is sponsored by Abbott, Eli Lilly, Merck Sharp & Dohme, and Novo Nordisk. The funders were not involved in the study design, collection, analysis, interpretation of data, the writing of this article, or the decision to submit it for publication.
Publisher’s note
All claims expressed in this article are solely those of the authors and do not necessarily represent those of their affiliated organizations, or those of the publisher, the editors and the reviewers. Any product that may be evaluated in this article, or claim that may be made by its manufacturer, is not guaranteed or endorsed by the publisher.
Supplementary material
The Supplementary Material for this article can be found online at: https://www.frontiersin.org/articles/10.3389/fendo.2023.1101934/full#supplementary-material
References
1. Dimas AS, Lagou V, Barker A, Knowles JW, Mägi R, Hivert M-F, et al. Impact of type 2 diabetes susceptibility variants on quantitative glycemic traits reveals mechanistic heterogeneity. Diabetes (2014) 63:2158–71. doi: 10.2337/db13-0949
2. Laakso M. Biomarkers for type 2 diabetes. Mol Metab (2019) 27:S139–46. doi: 10.1016/j.molmet.2019.06.016
3. Aguiari G, Crudele F, Taccioli C, Minotti L, Corrà F, Keillor JW, et al. Dysregulation of transglutaminase type 2 through GATA3 defines aggressiveness and doxorubicin sensitivity in breast cancer. Int J Biol Sci (2022) 18:1–14. doi: 10.7150/ijbs.64167
4. Tatsukawa H, Furutani Y, Hitomi K, Kojima S. Transglutaminase 2 has opposing roles in the regulation of cellular functions as well as cell growth and death. Cell Death Dis (2016) 7:e2244–4. doi: 10.1038/cddis.2016.150
5. Bernassola F, Federici M, Corazzari M, Terrinoni A, Hribal ML, De Laurenzi V, et al. Role of transglutaminase 2 in glucose tolerance: knockout mice studies and a putative mutation in a MODY patient. FASEB J (2002) 16:1371–8. doi: 10.1096/fj.01-0689com
6. Porzio O, Massa O, Cunsolo V, Colombo C, Malaponti M, Bertuzzi F, et al. Missense mutations in theTGM2 gene encoding transglutaminase 2 are found in patients with early-onset type 2 diabetes. Hum Mutat (2007) 28:1150–0. doi: 10.1002/humu.9511
7. Minotti L, Baldassari F, Galasso M, Volinia S, Bergamini CM, Bianchi N. A long non-coding RNA inside the type 2 transglutaminase gene tightly correlates with the expression of its transcriptional variants. Amino Acids (2018) 50:421–38. doi: 10.1007/s00726-017-2528-9
8. Ponting CP, Oliver PL, Reik W. Evolution and functions of long noncoding RNAs. Cell (2009) 136:629–41. doi: 10.1016/j.cell.2009.02.006
9. Graf J, Kretz M. From structure to function: Route to understanding lncRNA mechanism. BioEssays (2020) 42:12. doi: 10.1002/bies.202000027
10. Wang S-H, Zhu X-L, Wang F, Chen S-X, Chen Z-T, Qiu Q, et al. LncRNA H19 governs mitophagy and restores mitochondrial respiration in the heart through Pink1/Parkin signaling during obesity. Cell Death Dis (2021) 12:557. doi: 10.1038/s41419-021-03821-6
11. Chen Y-T, Yang Q-Y, Hu Y, Liu X-D, de Avila JM, Zhu M-J, et al. Imprinted lncRNA Dio3os preprograms intergenerational brown fat development and obesity resistance. Nat Commun (2021) 12:6845. doi: 10.1038/s41467-021-27171-1
12. Zhao X-Y, Xiong X, Liu T, Mi L, Peng X, Rui C, et al. Long noncoding RNA licensing of obesity-linked hepatic lipogenesis and NAFLD pathogenesis. Nat Commun (2018) 9:2986. doi: 10.1038/s41467-018-05383-2
13. Moreno-Navarrete JM, Ortega FJ, Bassols J, Castro A, Ricart W, Fernández-Real JM. Association of circulating lactoferrin concentration and 2 nonsynonymous LTF gene polymorphisms with dyslipidemia in men depends on glucose-tolerance status. Clin Chem (2008) 54:301–9. doi: 10.1373/clinchem.2007.095943
14. Valdés S, Botas P, Delgado E, Álvarez F, Cadórniga FD. Population-based incidence of type 2 diabetes in northern Spain. Diabetes Care (2007) 30:2258–63. doi: 10.2337/dc06-2461
15. Marchetti P, Bugliani M, Lupi R, Marselli L, Massini M, Boggi U, et al. The endoplasmic reticulum in pancreatic beta cells of type 2 diabetes patients. Diabetologia (2007) 50:2486–94. doi: 10.1007/s00125-007-0816-8
16. Sabarinathan R, Tafer H, Seemann SE, Hofacker IL, Stadler PF, Gorodkin J. The RNAsnp web server: predicting SNP effects on local RNA secondary structure. Nucleic Acids Res (2013) 41:W475–9. doi: 10.1093/nar/gkt291
17. The 1000 Genomes Project Consortium. A global reference for human genetic variation. Nature (2015) 526:68–74. doi: 10.1038/nature15393
18. García-Alonso L, Jiménez-Almazán J, Carbonell-Caballero J, Vela-Boza A, Santoyo-López J, Antiñolo G, et al. The role of the interactorme in the maintenance of deleterious variability in human populations. Mol Syst Biol (2014) 10:752. doi: 10.15252/msb.20145222
19. Ciregia F, Bugliani M, Ronci M, Giusti L, Boldrini C, Mazzoni MR, et al. Palmitate-induced lipotoxicity alters acetylation of multiple proteins in clonal β cells and human pancreatic islets. Sci Rep (2017) 7:13445. doi: 10.1038/s41598-017-13908-w
20. Cabili MN, Dunagin MC, McClanahan PD, Biaesch A, Padovan-Merhar O, Regev A, et al. Localization and abundance analysis of human lncRNAs at single-cell and single-molecule resolution. Genome Biol (2015) 16:20. doi: 10.1186/s13059-015-0586-4
21. Ransohoff JD, Wei Y, Khavari PA. The functions and unique features of long intergenic non-coding RNA. Nat Rev Mol Cell Biol (2018) 19:143–57. doi: 10.1038/nrm.2017.104
22. Castellanos-Rubio A, Fernandez-Jimenez N, Kratchmarov R, Luo X, Bhagat G, Green PHR, et al. A long noncoding RNA associated with susceptibility to celiac disease. Science (1979) 2016) 352:91–5. doi: 10.1126/science.aad0467
23. Sabarinathan R, Tafer H, Seemann SE, Hofacker IL, Stadler PF, Gorodkin J. RNAsnp: Efficient detection of local RNA secondary structure changes induced by SNPs. Hum Mutat (2013) 34:546–56. doi: 10.1002/humu.22273
24. Russo L, Marsella C, Nardo G, Massignan T, Alessio M, Piermarini E, et al. Transglutaminase 2 transamidation activity during first-phase insulin secretion: natural substrates in INS-1E. Acta Diabetol (2013) 50(1):61–72. doi: 10.1007/s00592-012-0381-6
25. Sághy T, Köröskényi K, Hegedűs K, Antal M, Bankó C, Bacsó Z, et al. Loss of transglutaminase 2 sensitizes for diet-induced obesity-related inflammation and insulin resistance due to enhanced macrophage c-src signaling. Cell Death Dis (2019) 10(6):439. doi: 10.1038/s41419-019-1677-z
26. Saxena A, Wahi N, Kumar A, Mathur SK. Functional interactomes of genes showing association with type-2 diabetes and its intermediate phenotypic traits point towards adipo-centric mechanisms in its pathophysiology. Biomolecules (2020) 10(4):601. doi: 10.3390/biom10040601
27. Liu H, Niu Q, Wang T, Dong H, Bian C. Lipotoxic hepatocytes promote nonalcoholic fatty liver disease progression by delivering microRNA-9-5p and activating macrophages. Int J Biol Sci (2021) 17(14):3745–59. doi: 10.7150/ijbs.57610
28. Sileno S, D’Oria V, Stucchi R, Alessio M, Petrini S, Bonetto V, et al. A possible role of transglutaminase 2 in the nucleus of INS-1E and of cells of human pancreatic islets. J Proteomics (2014) 96:314–27. doi: 10.1016/j.jprot.2013.11.011
29. Iismaa SE, Aplin M, Holman S, Yiu TW, Jackson K, Burchfield JG, et al. Glucose homeostasis in mice is transglutaminase 2 independent. PloS One (2013) 8:e63346. doi: 10.1371/journal.pone.0063346
30. Salter NW, Ande SR, Nguyen HK, Nyomba BLG, Mishra S. Functional characterization of naturally occurring transglutaminase 2 mutants implicated in early-onset type 2 diabetes. J Mol Endocrinol (2012) 48:203–16. doi: 10.1530/JME-11-0064
31. Gonzalez-Moro I, Olazagoitia-Garmendia A, Colli ML, Cobo-Vuilleumier N, Postler TS, Marselli L, et al. The T1D-associated lncRNA Lnc13 modulates human pancreatic β cell inflammation by allele-specific stabilization of STAT1 mRNA. Proc Natl Acad Sci (2020) 117:9022–31. doi: 10.1073/pnas.1914353117
32. Aguilo F, di Cecilia S, Walsh MJ. Long non-coding RNA ANRIL and polycomb in human cancers and cardiovascular disease. Current Topics in Microbiology and Immunology (2015) 394:29–39. doi: 10.1007/82_2015_455
33. Statello L, Guo C-J, Chen L-L, Huarte M. Gene regulation by long non-coding RNAs and its biological functions. Nat Rev Mol Cell Biol (2021) 22:96–118. doi: 10.1038/s41580-020-00315-9
34. Sebastian-delaCruz M, Gonzalez-Moro I, Olazagoitia-Garmendia A, Castellanos-Rubio A, Santin I. The role of lncRNAs in gene expression regulation through mRNA stabilization. Noncoding RNA (2021) 7:3. doi: 10.3390/ncrna7010003
Keywords: long non-coding RNA, type 2 diabetes, single nucleotide pholymorphism (SNP), pancreatic beta cell, transglutaminase 2
Citation: González-Moro I, Rojas-Márquez H, Sebastian-delaCruz M, Mentxaka-Salgado J, Olazagoitia-Garmendia A, Mendoza LM, Lluch A, Fantuzzi F, Lambert C, Ares Blanco J, Marselli L, Marchetti P, Cnop M, Delgado E, Fernández-Real JM, Ortega FJ, Castellanos-Rubio A and Santin I (2023) A long non-coding RNA that harbors a SNP associated with type 2 diabetes regulates the expression of TGM2 gene in pancreatic beta cells. Front. Endocrinol. 14:1101934. doi: 10.3389/fendo.2023.1101934
Received: 18 November 2022; Accepted: 24 January 2023;
Published: 07 February 2023.
Edited by:
Kavita Jadhav, AstraZeneca, United StatesReviewed by:
Mihaela Stefan Lifshitz, Albert Einstein College of Medicine, United StatesMohsen Ghanbari, Erasmus Medical Center, Netherlands
Copyright © 2023 González-Moro, Rojas-Márquez, Sebastian-delaCruz, Mentxaka-Salgado, Olazagoitia-Garmendia, Mendoza, Lluch, Fantuzzi, Lambert, Ares Blanco, Marselli, Marchetti, Cnop, Delgado, Fernández-Real, Ortega, Castellanos-Rubio and Santin. This is an open-access article distributed under the terms of the Creative Commons Attribution License (CC BY). The use, distribution or reproduction in other forums is permitted, provided the original author(s) and the copyright owner(s) are credited and that the original publication in this journal is cited, in accordance with accepted academic practice. No use, distribution or reproduction is permitted which does not comply with these terms.
*Correspondence: Izortze Santin, izortze.santin@ehu.eus; Ainara Castellanos-Rubio, ainara.castellanos@ehu.eus
†These authors have equally contributed to this work