- 1Department of Stomatology, 2nd Hospital, Shanxi Medical University, Taiyuan, Shanxi, China
- 2School of Basic Medicine, Shanxi Medical University, Taiyuan, Shanxi, China
- 3Stomatological Hospital of Shanxi Medical University, Taiyuan, Shanxi, China
- 4Department of Mental Health, Shanxi Medical University, Taiyuan, Shanxi, China
- 5Medical Imaging Department of Shanxi Medical University, Taiyuan, Shanxi, China
- 6Department of Radiology, 2nd Hospital, Shanxi Medical University, Taiyuan, Shanxi, China
Objectives: To observe the elongation of the axial tooth movement in the unopposed rodent molar model with type 1 diabetes mellitus and explore the pathological changes of periodontal ligament and alveolar bone, and their correlation with tooth axial movement.
Methods: The 80 C57BL/6J mice were randomly divided into the streptozotocin(STZ)-injected group (n = 50) and the control group (n = 30). Mice in the streptozotocin(STZ)-injected group were injected intraperitoneal with streptozotocin (STZ), and mice in the control group were given intraperitoneal injection of equal doses of sodium citrate buffer. Thirty mice were randomly selected from the successful models as the T1DM group. The right maxillary molar teeth of mice were extracted under anesthesia, and allowed mandibular molars to super-erupt. Mice were sacrificed at 0, 3, 6,9, and 12 days. Tooth elongation and bone mineral density (BMD) were evaluated by micro-CT analysis(0,and 12 days mice). Conventional HE staining, Masson staining and TRAP staining were used to observe the changes in periodontal tissue(0, 3, 6, 9, and 12 days mice). The expression differences of SPARC, FGF9, BMP4, NOGGIN, and type I collagen were analyzed by RT-qPCR.
Results: After 12 days of tooth extraction, our data showed significant super-eruption of mandibular mouse molars of the two groups. The amount of molar super-eruption in the T1DM group was 0.055mm( ± 0.014mm), and in the control group was 0.157( ± 0.017mm). The elongation of the T1DM mice was less than that of the control mice(P<0.001). It was observed that the osteoclasts and BMD increased gradually in both groups over time. Compared with the control group, the collagen arrangement was more disordered, the number of osteoclasts was higher (P<0.05), and the increase of bone mineral density was lower(2.180 ± 0.007g/cm3 vs. 2.204 ± 0.006g/cm3, P<0.001) in the T1DM group. The relative expression of SPARC, FGF9, BMP4, and type I collagen in the two groups increased with the extension of tooth extraction time while NOGGIN decreased. The relative expression of all of SPARC, FGF9, BMP4, and type I collagen in the T1DM group were significantly lower, and the expression of NOGGIN was higher than that in the control group (P<0.05).
Conclusion: The axial tooth movement was inhibited in type 1 diabetic mice. The result may be associated with the changes of periodontal ligament osteoclastogenic effects and alveolar bone remodeling regulated by the extracellular matrix and osteogenesis-related factors.
1 Introduction
Tooth movement is a multi-factor process involving the motion of existing tissues and the formation of new tissues coordinated by a set of genetic events (1), commonly occurring in tooth eruption, tooth loss, adaptation to mastication, orthodontic tooth movement, etc. (2). The alveolar bone surrounding the teeth is constantly remodeled throughout life (3) and presents a high turnover rate during tooth movement, including bone resorption and bone formation. The periodontal ligament(PDL) anchors the tooth in the alveolar socket. Extrinsic and intrinsic stimuli trigger the response of periodontal ligament fibroblasts to induce periodontal tissue reorganization, which is the biological basis of tooth movement (4). The tooth movement process involves high levels of elaborate interactions between growth factors, transcription factors, and structural proteins (5). Establishing the dynamic relationship among tooth movement, alveolar bone remodeling, and periodontal ligament biological responses is of great significance for orthodontics.
At present, studies of tooth movement have focused on tissue remodeling of horizontal tooth movement caused by orthodontic force (6). The horizontal movement during orthodontic treatment is affected by the traction force of the orthodontic device, and the mechanical stress or traction generated during orthodontic tooth movement also can cause tissue damage and triggers an inflammatory response (7), which cannot reflect the intrinsic physiological mechanism of tooth movement. The study of tooth axial movement is mainly related to tooth eruption, which is defined as the axial movement of the tooth from its site of development in the alveolar bone to its functional position in the oral cavity (8). Therefore, the research on axial tooth movement is of great significance to the study of the physiological mechanism of tooth movement and tooth eruption. However, there were few empirical researches on it. The research had demonstrated that after removing the upper molars unilaterally, the lower molars would erupt continuously without an antagonist, which was used to study the axial tooth movement (9).
In addition, tooth movement is also affected by medication or systemic pathological factors. Diabetes mellitus(DM) is a group of clinical syndromes, which will result in diminished bone-mineral density and osteoporosis (10–12). The study showed that DM not only induces higher alveolar bone resorption but also enhances fibroblast inflammation and osteoclastogenic effects of periodontal ligament (13). Some results suggested that tooth movement in T2DM animals was higher than in healthy animals with adapted orthodontic appliances (14), while another study observed that the amount of orthodontic tooth movement in T2DM was not compromised (15). However, relatively few data are available for type 1 diabetes mellitus (T1DM) (16). Studies have found that children with T1DM with age younger than 11.5 years had teeth eruption acceleration, while children older than 11.5 years had delayed teeth eruption process (17). Type 1 T1DM is becoming more prevalent among young individuals worldwide who is also the main population needing orthodontic treatment. Compared with T2DM, T1DM affects bone more severely and via a pathophysiological mechanism dependent on a decrease in bone mineral density (BMD) (18).The cellular and molecular related to T1DM that may affect the elongation movement of teeth in the axial direction are still unclear, and it is necessary to investigate these mechanisms.
The present study was performed to visualize the process of axial tooth movement by using T1DM the model of unopposed rodent molar with type 1 diabetes mellitus, and investigate the related changes of the periodontal ligament biological responses and alveolar bone remodeling.
2 Material and methods
2.1 Animals
C57BL/6J mice(six weeks old, male, 19~25g) were purchased from the Animal Laboratory Center of Shanxi Medical University(Animal Permit NO: DW2022038) and kept in a standard lab housing with a 12 h light/dark cycle at a temperature of 22 ± 2°C and 30% - 50% humidity with diet and water ad libitum. Study protocols were approved by the Animal Ethics Committee of the Second Hospital of Shanxi Medical University.
2.2 Induction of T1DM model
Eighty mice were divided into control(n=30) and streptozotocin(STZ)-injected groups(n=50) randomly. The mice were fasted for 8h before STZ injection. Injected mice were intraperitoneally injected a single dose of 150mg/kg STZ (Sigma S-0130) dissolved in 0.1 M citrate buffer at pH 4.2. The control group received the same volume of 0.1 M citrate buffer. 72h after the STZ injection, mice with consistent blood glucose levels ≥11.1 mmol/L for three consecutive days were regarded as successful diabetic models (19). T1DM mice were fed a diet high in fat and sugar. Plasma glucose concentration was recorded during the experimental period. The model was considered to be unsuccessful if blood glucose returned to normal or the mice died during feeding.
2.3 Induction of un-opposed molar model
The T1DM group(n=30) and the control group(n=30) were anesthetized with Ketamine (100mg/kg) and Xylazine (5mg/kg). All three right maxillary molars were extracted under anesthesia. The mice were intraperitoneally injected with buprenorphine (0.05 mg/kg) to manage pain after the operation.
2.4 Mandible morphometric analyses by microcomputerized
Mice were sacrificed using an excess of isoflurane anesthesia at 0 and 12 days after surgery. The mandibular alveolar bone was harvested, and bone specimens were scanned with a micro-CT scanner equipped with a custom software package (Micro-CTCH-8306, Scanco Medical, Basserdorf, Switzerland). Specimens were scanned at 70 kVp and 114 μA at high resolution (37 μm). The images were processed by three-dimensional reconstruction software (μCT Evaluation Program v6.0, Scanco Medical). Taking the line of the outermost tangent point above the bilateral mandibular foramen of mice as the reference line, the distance between the two parallel lines at 12 day after tooth extraction was measured from the highest point of the right mandibular first molar as the parallel line with the reference line, and the distance between the two parallel lines at 0 days after tooth extraction was subtracted as the eruption rate. Each sample was analyzed by two different investigators independently. The analysis software Mimics Medical 17.0 was used to measure bone mineral density (BMD).
2.5 Tissue processing
Mice were sacrificed using an excess of isoflurane anesthesia at 0, 3, 6, 9 and 12 days after surgery. Collected mandibles were fixed in 10% neutral formalin for 24h followed by decalcification for 30~40 days with 10% EDTA. After decalcification and paraffin embedding, the samples were cut in 4 μm sagittal sections along the long axis of the molar teeth, and the sections were stained with hematoxylin and eosin (H&E) Masson and Tartrate-resistant acid phosphatase (TRAP) staining. The results were observed under a microscope(Olympus, Tokyo, Japan). Scanscope scanning system was used to scan the stained sections, and Spectrum software was used to analyze the sections. Five high-magnification fields (×200) were randomly selected from the sections of each group of the diabetes group and the control group to count the osteoclasts. The results was analyzed by two different investigators independently.
2.6 RNA extraction and real-time quantitative PCR
Total RNA was extracted from the mandibles of three mice (per group). The expression of SPARC, FGF9, BMP4, type I collagen, and NOGGIN gene expression in the periodontal tissues were assessed by real-time quantitative PCR. RNAs underwent RT-PCR using Sprint RT Complete kit (Clontech). Real-time quantitative PCR was performed using Taqman Fast Universal PCR Master Mix (Applied Biosystems) with DLUX fluorogenic primers. Reaction conditions were as follows: 30s at 95°C (one cycle), 5s at 95°C, and 30s at 60°C (40 cycles). PCR products were continuously monitored with an ABI PRISM 7900 detection system (Applied Biosystems). Primer sequences used for real-time PCR analysis are shown in Table 1. The CT value of each group was computed and processed according to 2-ΔΔCt, and the results were plotted by the software GraphPad Prism.
2.7 Statistical analyses
SPSS27.0 statistical software was used for statistical analysis. The normality test was performed on measurement data. Quantitative data were presented as mean ± Standard Error of Mean (SEM), and the data were analyzed by Independent-Samples T-Test. Differences were considered significant at P < 0.05.
3 Results
3.1 T1DM animal model
A total of 46 mice were modeled, and all 30 mice in the control group survived. The body weight of mice in STZ-injected was significantly lower than that of the control mice(19.44 ± 1.92g vs. 22.76 ± 2.08g, P = 0.016). Mice in the STZ-injected group, the blood glucose levels were significantly higher than that of control mice(16.49 ± 2.75mmol/L vs. 6.94 ± 0.83 mmol/L, P < 0.001). The induction of the T1 T1DM model is characterized by a significant increase in fasting blood glucose and weight loss. These characteristics indicated that the T1DM model was successfully established (Figure 1).
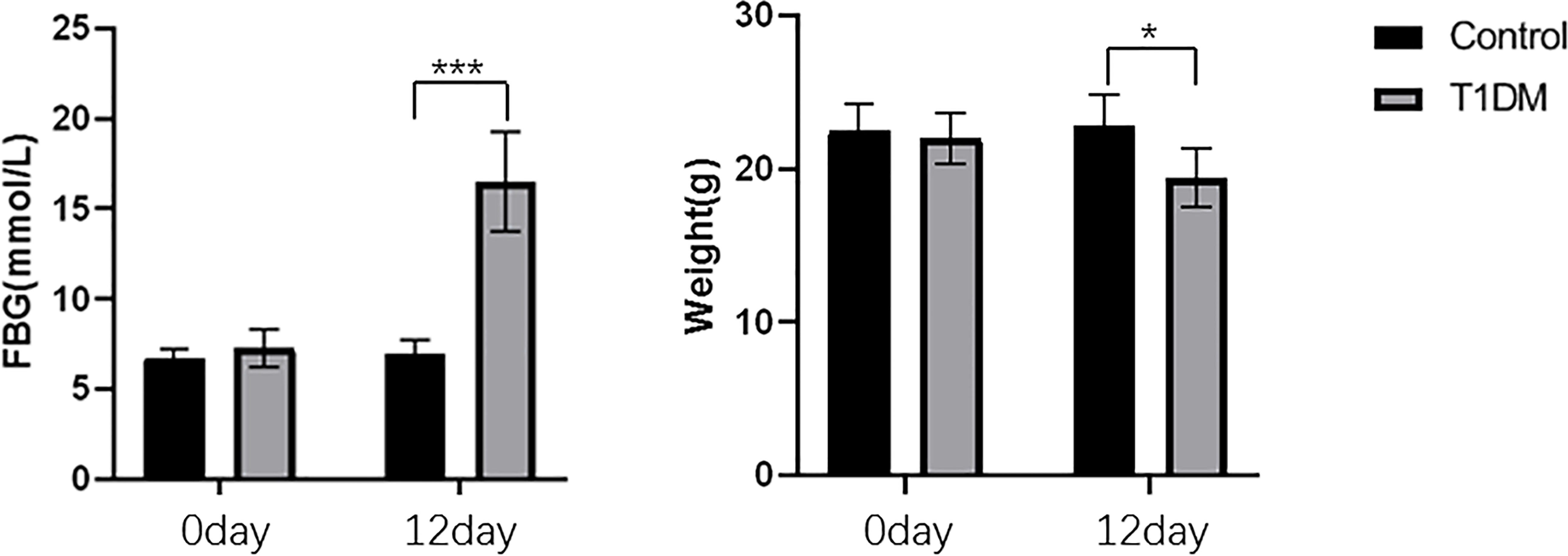
Figure 1 The changes of FBG and body weight in control and T1DM groups. Compared with the control group, the blood glucose was significantly increased, and the body weight was decreased in the T1DM group. *P < 0.05; ***P < 0.001.
3.2 Eruption rate
There was a significant amount of super-eruption on the unopposed side of both groups (Figure 2). The results demonstrated that the amount of tooth movement in the control group was significantly more than that in the T1DM group at 12 day after surgery(0.055 ± 0.014mm vs. 0.157 ± 0.017mm, P < 0.001).
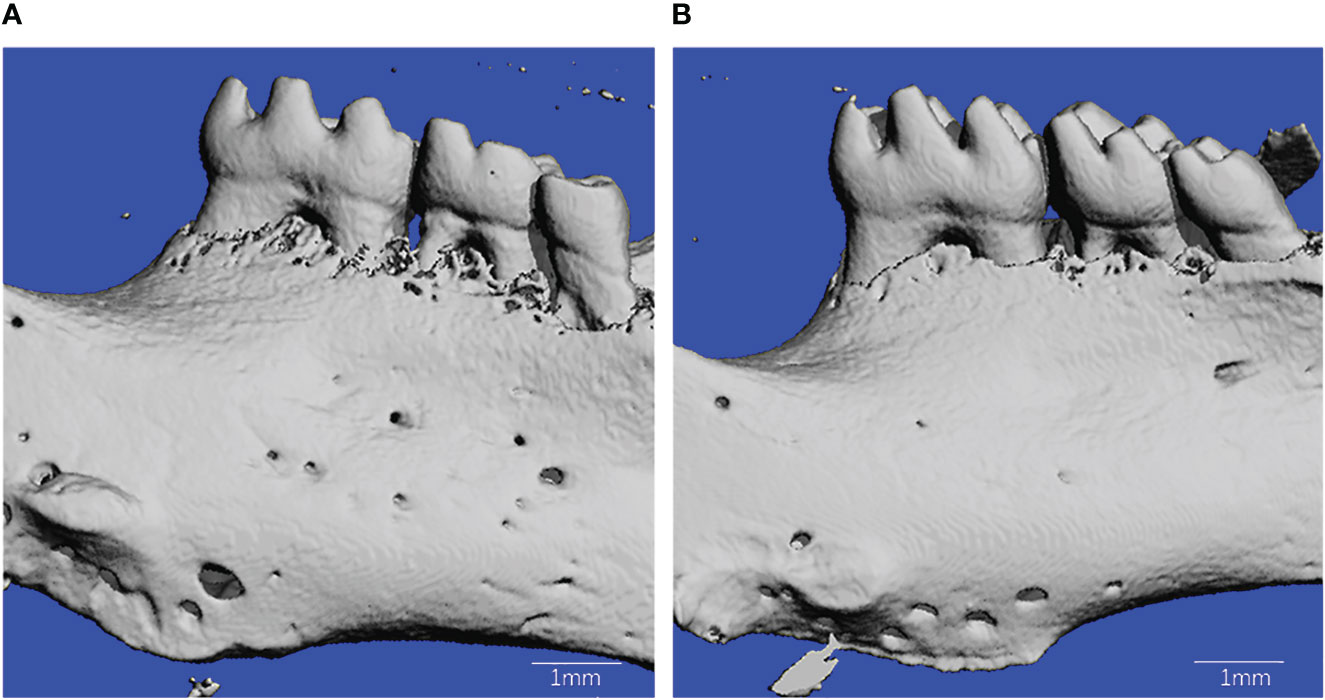
Figure 2 Microcomputed tomography reconstruction of mandibles in T1DM and control mice. (A) is from T1DM mice, and (B) is from control mice.
3.3 Bone mineral density of the right mandible
After 12 days of tooth extraction, the density of the right mandible increased in both groups (Figure 3A). Compared with control mice, the T1DM group showed less increase in bone mineral density (2.180 ± 0.006 g/cm3 vs. 2.204 ± 0.0061 g/cm3, P<0.001).
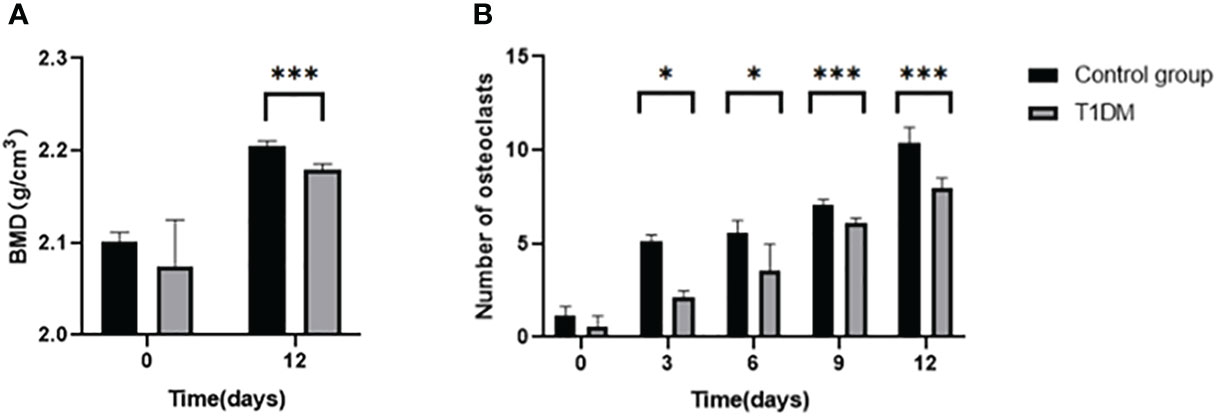
Figure 3 (A) is the bone mineral density(BMD) of the right mandible in control and T1DM groups on days 0 and 12 of the experiment.. After 12 days of tooth extraction, the bone mineral density in both groups increased during the axial tooth movement, and the increase of bone mineral density in the T1DM mice was significantly less than that in the control group. (B) is the number of right mandibular osteoclasts in both groups on days 0, 3, 6, 9, and 12 of the experiment. The number of osteoclasts in the two groups increased gradually. Compared with the control group, the number of osteoclasts in the T1DM mice was higher, which was particularly obvious at day 9 and day 12. *P < 0.05; ***P < 0.001.
3.4 Histological analysis
Histological analysis in all groups showed changes in the periodontal tissue structure of mice by HE staining (Figure 4) and Masson staining (Figure 5). TRAP staining (Figure 6) showed changes in the number of osteoclasts (Figure 3B). The histological trend of the T1DM group was consistent with that of the control group. In both groups of mice, the periodontal ligament was mainly composed of fibroblasts interspersed with collagen fibers and vascular and neural elements. Three days after the extraction of the right maxillary molars, cells were arranged irregularly, the periodontal collagen fiber bundles were arranged disorderly, and the cells on the surfaces of cementum and alveolar bone gradually increased. Collagen fibers also became more compact at 9~12days. In the control group, cells in the periodontal ligament were arranged in order, and the periodontal ligament fibers were orderly arranged. Compared with the control group, collagen arrangement was disordered and the number of osteoclasts (Figure 3B) was higher in the T1DM group (At days 3,6,9, and 12, p<0.05). There were fewer cellular components and sparse collagen fibers per unit area of alveolar bone in the T1DM mice.
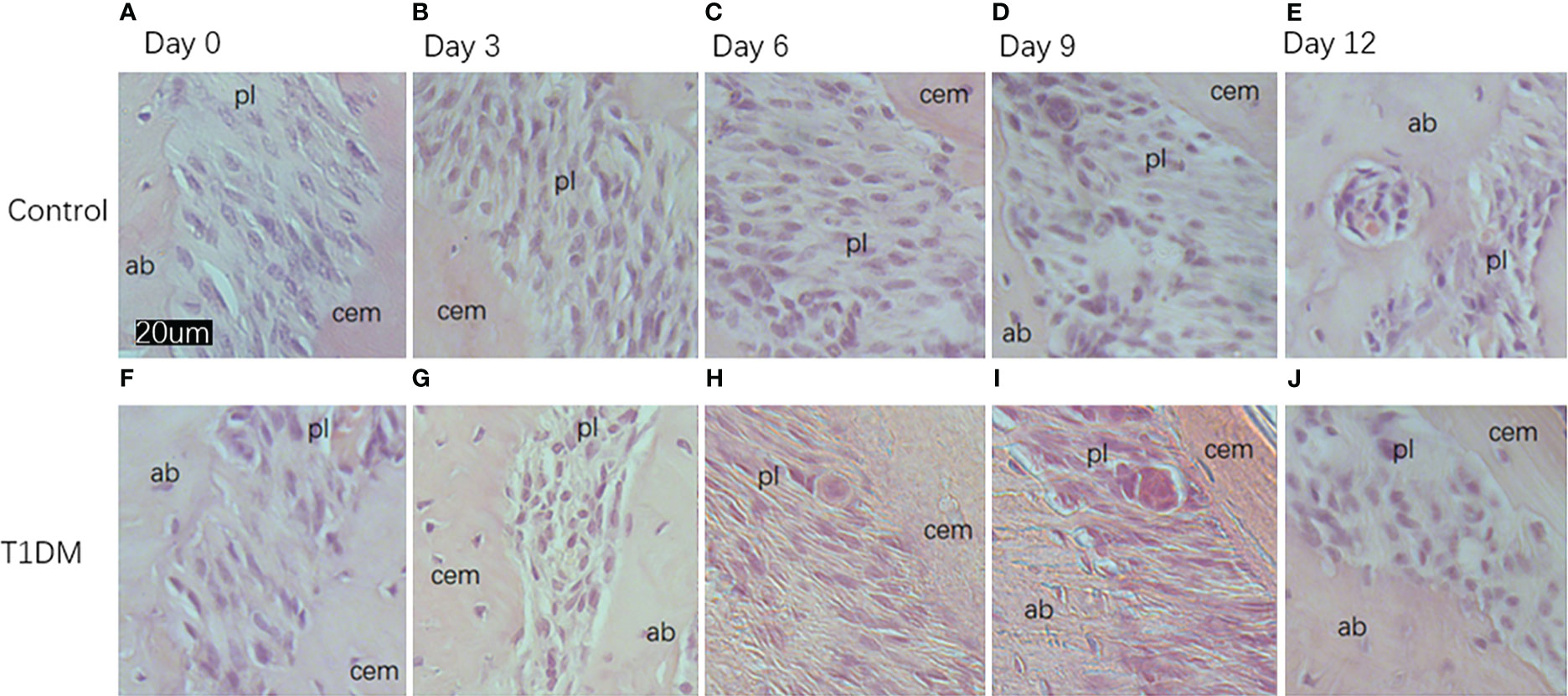
Figure 4 Histological appearance (HE staining)of the periodontium of mandibular molars in both groups on days 0, 3, 6, 9, and 12 of the experiment. (A–E) are from control mice, and (F–J) are from T1DM mice.3 to 12 days after the extraction of the surgery, the periodontal ligament of the corresponding mandibular molar showed irregular arrangement of cells. The cells in the periodontal ligament space, cementum, and alveolar bone surface gradually increased, and osteoclasts and osteoblasts could be observed in both groups of mice. In contrast, diabetic mice had fewer cellular components and a greater proportion of osteoclasts. cem, cementum; ab, alveolar bone; pl, periodontal ligament (×200).
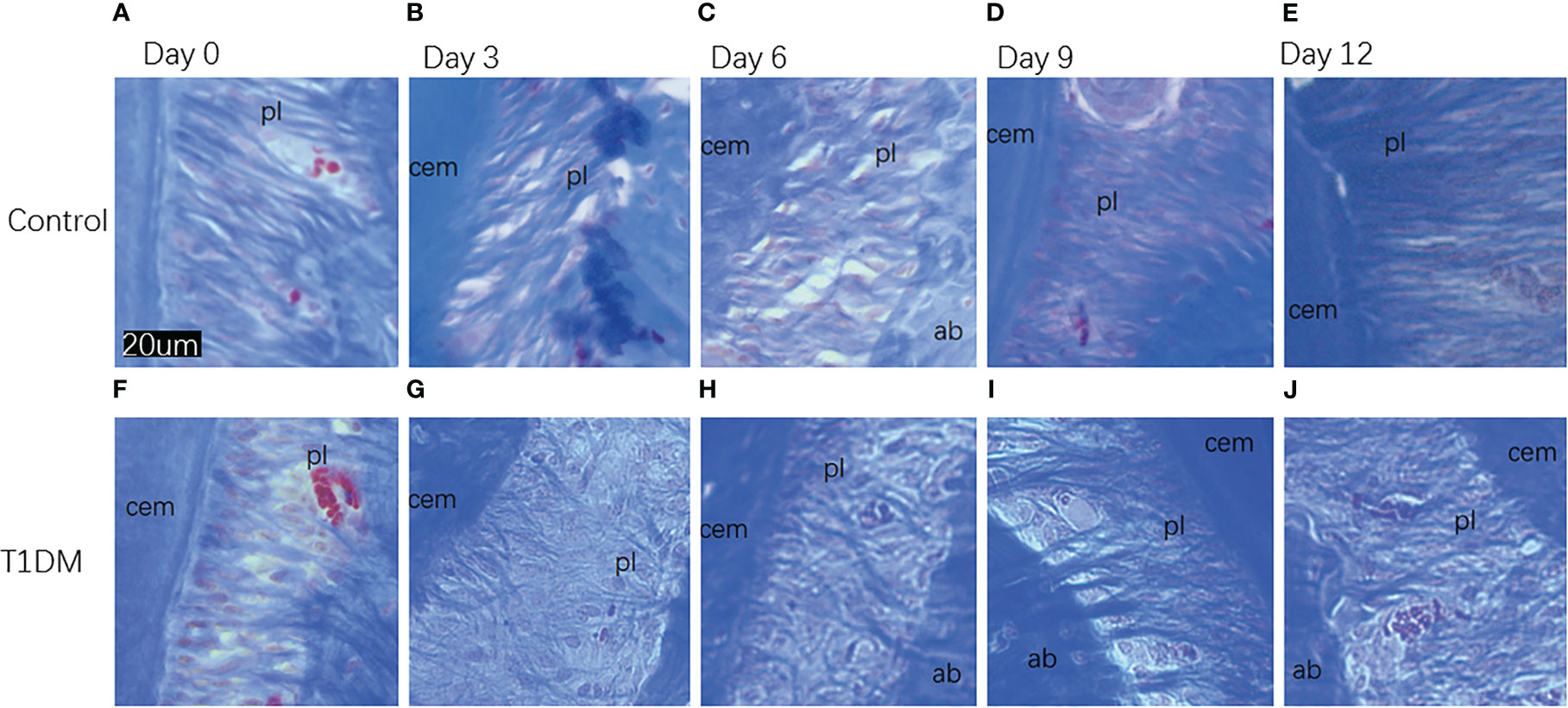
Figure 5 Histological appearance (Masson staining)of collagen fibers in the periodontal ligament in both groups on days 0, 3, 6, 9, and 12 of the experiment. (A–E) are from control mice, (F–J) are from T1DM mice.In both groups, at 3 and 6 days after the extraction of the right maxillary molar, the collagen fibers in the periodontal tissue of the mandibular molar began to arrange disorderly. At 9 and 12 days after the extraction, the collagen fibers became compact. Compared with the control group, the collagen fibers in the T1DM group were more sparse and disordered. cem, cementum; ab, alveolar bone; pl, periodontal ligament (×200).
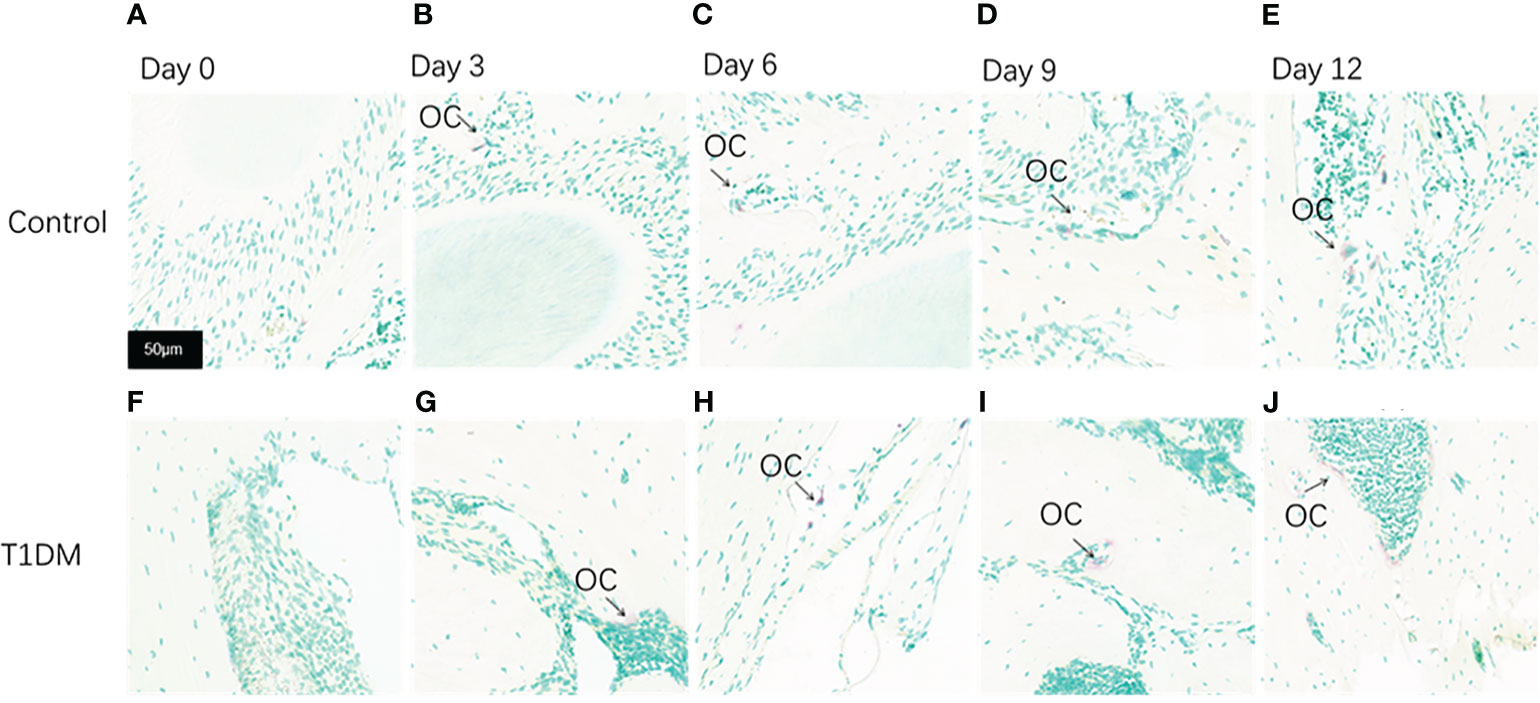
Figure 6 Tartrate-resistant acid phosphatase staining(TRAP staining) of the periodontium of mandibular molars in both groups on days 0, 3, 6, 9, and 12 of the experiment. (A–E) are from control mice, and (F–J) are from T1DM mice. 0 to 12 days after the extraction of the surgery, the number of osteoclasts increased gradually in both groups, however, the number of osteoclasts in the T1DM group was higher than that in the control group. The arrows indicate the site of positive osteoclast staining. OC, osteoclast (×200).
3.5 RT−qPCR analysis
The relative expression of SPARC, FGF9, BMP4, and type I collagen increased with the extension of tooth extraction time, while the relative expression of noggin decreased in the two groups. The relative expression of SPARC, FGF9, BMP4, and type I collagen in the T1DM mice were significantly lower than that in the control mice, and the relative expression of NOGGIN was higher (Figure 7)(At days 3,6,9, and 12, p<0.05).
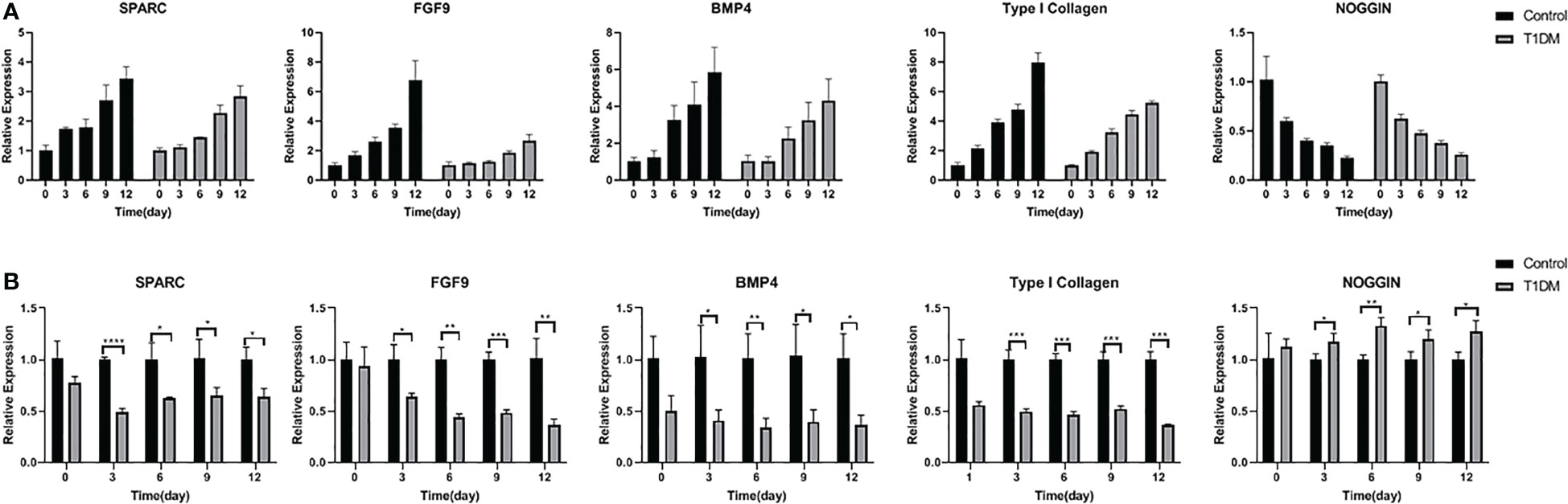
Figure 7 The relative expression of SPARC, FGF9, BMP4, Type I collagen and NOGGIN in control and T1DM group on days 0, 3, 6, 9, and 12 of the experiment. (A) The trend of relative expression levels in both groups at days o,3,6,9,12. Extraction of the opposing teeth resulted in a gradual increase in SPARC, FGF9, BMP4, and Type I collagen relative expression while the expression level of NOGGIN decreased, indicating that these osteogenesis-related factors were actively involved in bone remodeling during the axial tooth movement. (B) Comparison of the relative expression levels in two groups at different time points. The relative expression levels of SPARC, FGF9, BMP4, and Type I collagen were lower, and the NOGGIN expression was higher in T1DM mice compared with control mice, suggesting that osteogenesis was impaired in T1DM mice. *P < 0.05; **P < 0.01; ***P < 0.001; ****P < 0.0001.
4 Discussion
The axial tooth movement is the physiological mechanism of tooth eruption. The loss of antagonists results in varying degrees of axial tooth movement in the post-emergent phase of tooth eruption (20). Moreover, diabetes mellitus(DM) was a common disease which affected tooth movement. T1DM usually starts during adolescence, at a time of accelerated skeletal growth, and because the bone becomes compromised at a younger age, the adverse consequences are more severe relative to T2DM (21). In our study, the maxillary molars of mice were extracted to establish the model of the unopposed mouse molar, and we found that T1DM mice had a lower tendency of tooth super-eruption than normal mice(P<0.001). The periodontal ligament biological responses were inhibited, and the alveolar bone remodeling was weakened in type 1 diabetic T1DM mice.
Measurements of tooth movement were made through 3D reconstructed images and showed that the mandibular molars of T1DM mice in each group elongated after tooth extraction, and the elongation of type 1 diabetic T1DM mice was smaller. To find out whether external forces such as bite force or intrinsic genetic mechanisms worked for physiological tooth movement mechanisms, researchers developed the model of the unopposed mouse molar (22–24). Previous studies had established significant axial movement of first and second mandibular mouse molars following the complete extraction of antagonists (1). A study showed that diabetes significantly reduced orthodontic tooth movement (25). However, how type 1 diabetes T1DM affects axial tooth movement and its physiological mechanisms have not been studied.
In this study, bone mineral density BMD was measured during tooth elongation in both groups. The generation and functional regulation of osteoblasts and osteoclasts lead to changes in the bone mineral density BMD (26). The results showed that BMD increased in mandible of both groups, but the increment in diabetic group was smaller than that in control group. Previous studies had suggested that the decreased BMD in patients with type 1 diabetes T1DM was due to the retardation of osteoblast activity and the inhibition of bone remodeling (27). Uncontrolled blood glucose levels and insulin deficiency are thought to be the main causes of osteopenia in T1DM. Insulin can directly affect bone cells and may lead to low bone mineral density BMD in T1DM.
Our results showed that periodontal ligament collagen fibers and osteoclasts responded actively with increasing time, whereas T1DM mice had smaller responses and more osteoclast. Changes in the periodontal tissue were similar to those observed during physiological tooth development. It has been demonstrated in previous studies that axial tooth movement induced by unloading in mice was due to osteoclastic bone resorption on the distal aspect of the alveolar socket combined with alveolar bone and cementum formation on the mesial and apical parts of the alveolar socket (28). The changes of periodontal collagen and cells reflected the response of periodontal ligament during tooth movement with orthodontic treatment (29). Nevertheless, the detailed molecular mechanisms underlying the role of individual molecules in unloading-induced bone remodeling remain unclear.
The results of qPCR showed that the relative expression of extracellular matrix gene products SPARC and type I collagen in all mice increased with the extension of tooth extraction time, however, the relative expression of them in the T1DM group were significantly lower. Type I collagen is the main structural component of the PDL, which maintains periodontal health (30). In previous orthodontic experiments, the expression of type I collagen increased during tooth movement (31). SPARC (secreted protein, autogenous and rich in cysteine) could modulate osteoblasts and osteoclasts and was critical for normal bone remodeling. SPARC also could mediate the in vitro mineralization of type I collagen by binding firmly to type I collagen (32, 33). The regeneration capacity of PDL and bone formation were reduced in Sparc-null mice (34, 35). In previous studies, the relative expression of SPARC in the type 2 diabetes T2DM mice was higher than that in the control mice (36, 37), and this might be the result of SPARC promoting insulin resistance.
The relative expression of osteogenesis-related factors BMP4 and FGF9 were continuously increased while NOGGIN (Extracellular BMP antagonist, acts by binding BMP4 with high affinity, and as a consequence, blocks its biological effects) decreased. Compared with the control mice, the expression level of BMP4 and FGF9 was lower, while the NOGGIN expression level was higher in the T1DM mice. Bone morphogenetic proteins (BMPs) play a crucial role in regulating alveolar bone formation (38). BMP4 is identified as a bone-inducing factor, and injection of BMP4-transduced MSCs induced bone formation in mice (39). FGF9 has complex and essential roles in skeletal development and repair, inducing osteoblast proliferation and new bone formation (40). Some studies had demonstrated that Type 2 diabetes T2DM affected bone remodeling, leading to decreased bone regeneration. These effects could be reversed by the local application of FGF9 (41). Hyperglycemia is the main feature of T1DM. Hyperglycemia affects alveolar bone and periodontal ligament repair and reconstruction. Therefore, the unusual expression of these extracellular matrix gene products and osteogenesis-related factors in T1DM may be due to the impaired cells function and abnormal changes of bone protein matrix induced, leading to a chronic inflammatory state of bone (42).
However, this study had several limitations. First, our study only observed the expression changes of factors associated with tissue remodeling during tooth movement. The related genes should be knocked out in subsequent studies to determine whether the tissue remodeling ability is weakened or enhanced. Second, in this study, only the axial movement of mandibular teeth was observed, where the direction of gravity was opposite to the tooth elongation. The movement of maxillary teeth in the same direction of gravity should also be observed to research whether there was any influence in the next experiment. Finally, these experiments were currently limited to animal models, and further studies are needed to explore the data of axial tooth movement in type 1 diabetic T1DM patients.
In conclusion, the axial tooth movement was inhibited in type 1 diabetic T1DM mice. The mechanisms responsible for these pathological changes may be associated with enhanced periodontal ligament osteoclastogenic effects and reduced alveolar bone remodeling, based on the regulation of extracellular matrix and osteogenesis-related factors.
Data availability statement
The raw data supporting the conclusions of this article will be made available by the authors, without undue reservation.
Ethics statement
The animal study was reviewed and approved by Animal Ethics Committee of the Second Hospital of Shanxi Medical University.
Author contributions
Guarantor of integrity of entire study, JN; study concepts/study design or data acquisition or data analysis/interpretation, all authors; manuscript drafting or manuscript revision for important intellectual content, all authors; agrees to ensure any questions related to the work are appropriately resolved, all authors; literature research, JZ, YX, and WN; clinical studies, WL; experimental studies, WL and DG; statistical analysis, JZ; and manuscript editing, all authors. All authors contributed to the article and approved the submitted version.
Funding
This study was supported by the National Natural Science Fund of China (No. 82071898) and the Health Commission Fund of Shanxi Province(No. 2019042).
Conflict of interest
The authors declare that the research was conducted in the absence of any commercial or financial relationships that could be construed as a potential conflict of interest.
The reviewer XW declared a shared affiliation with the authors to the handling editor at the time of review.
Publisher’s note
All claims expressed in this article are solely those of the authors and do not necessarily represent those of their affiliated organizations, or those of the publisher, the editors and the reviewers. Any product that may be evaluated in this article, or claim that may be made by its manufacturer, is not guaranteed or endorsed by the publisher.
References
1. Luan X, Ito Y, Holliday S, Walker C, Daniel J, Galang TM, et al. Extracellular matrix-mediated tissue remodeling following axial movement of teeth. J Histochem Cytochem (2007) 55(2):127–40. doi: 10.1369/jhc.6A7018.2006
2. Yan ZQ, Wang XK, Zhou Y, Wang ZG, Wang ZX, Jin L, et al. H-type blood vessels participate in alveolar bone remodeling during murine tooth extraction healing. Oral Dis (2020) 26(5):998–1009. doi: 10.1111/odi.13321
3. Shoji-Matsunaga A, Ono T, Hayashi M, Takayanagi H, Moriyama K, Nakashima T. Osteocyte regulation of orthodontic force-mediated tooth movement via RANKL expression. Sci Rep (2017) 7(1):8753. doi: 10.1038/s41598-017-09326-7
4. Brockhaus J, Craveiro RB, Azraq I, Niederau C, Schröder SK, Weiskirchen R, et al. In vitro compression model for orthodontic tooth movement modulates human periodontal ligament fibroblast proliferation, apoptosis and cell cycle. Biomolecules (2021) 11(7):932. doi: 10.3390/biom11070932
5. Shimono M, Ishikawa T, Ishikawa H, Matsuzaki H, Hashimoto S, Muramatsu T, et al. Regulatory mechanisms of periodontal regeneration. Microsc Res Tech (2003) 60(5):491–502. doi: 10.1002/jemt.10290
6. Li Y, Zhan Q, Bao M, Yi J, Li Y. Biomechanical and biological responses of periodontium in orthodontic tooth movement: Up-date in a new decade. Int J Oral Sci (2021) 13(1):20. doi: 10.1038/s41368-021-00125-5
7. Koletsi D, Iliadi A, Papageorgiou SN, Konrad D, Eliades T. Evidence on the effect of uncontrolled diabetes mellitus on orthodontic tooth movement. A systematic review with meta-analyses in pre-clinical in- vivo research. Arch Oral Biol (2020) 115:104739. doi: 10.1016/j.archoralbio.2020.104739
8. Aldowsari M, Alsaif FS, Alhussain MS, AlMeshary BN, Alosaimi NS, Aldhubayb SM, et al. Prevalence of delayed eruption of permanent upper central incisors at a tertiary hospital in Riyadh, Saudi Arabia. Children (Basel) (2022) 9(11):1781. doi: 10.3390/children9111781
9. Walker CG, Dangaria S, Ito Y, Luan X, Diekwisch TG. Osteopontin is required for unloading-induced osteoclast recruitment and modulation of RANKL expression during tooth drift-associated bone remodeling, but not for super-eruption. Bone (2010) 47(6):1020–9. doi: 10.1016/j.bone.2010.08.025
10. Li Y, Ling J, Jiang Q. Inflammasomes in alveolar bone loss. Front Immunol (2021) 12:691013. doi: 10.3389/fimmu.2021.691013
11. DiMeglio LA, Evans-Molina C, Oram RA. Type 1 diabetes. Lancet (2018) 391(10138):2449–62. doi: 10.1016/S0140-6736(18)31320-5
12. Ferreira CL, da Rocha VC, da Silva Ursi WJ, De Marco AC, Santamaria M Jr, Santamaria MP, et al. Periodontal response to orthodontic tooth movement in diabetes-induced rats with or without periodontal disease. J Periodontol (2018) 89(3):341–50. doi: 10.1002/JPER.17-0190
13. Zheng J, Chen S, Albiero ML, Vieira GHA, Wang J, Feng JQ, et al. Diabetes activates periodontal ligament fibroblasts via NF-κB in vivo. J Dent Res (2018) 97(5):580–8. doi: 10.1177/0022034518755697
14. Gomes MF, Goulart MDGV, Giannasi LC, Hiraoka CM, Melo GFS, Zangaro RA, et al. Effects of the photobiomodulation using different energy densities on the periodontal tissues under orthodontic force in rats with type 2 diabetes mellitus. Braz Oral Res (2018) 32:e61. doi: 10.1590/1807-3107bor-2018.vol32.0061
15. Plut A, Sprogar Š, Drevenšek G, Hudoklin S, Zupan J, Marc J, et al. Bone remodeling during orthodontic tooth movement in rats with type 2 diabetes. Am J Orthod Dentofacial Orthop (2015) 148(6):1017–25. doi: 10.1016/j.ajodo.2015.05.031
16. Chakraborty P, Mukhopadhyay P, Bhattacharjee K, Chakraborty A, Chowdhury S, Ghosh S. Periodontal disease in type 1 diabetes mellitus: Influence of pubertal stage and glycemic control. Endocr Pract (2021) 27(8):765–8. doi: 10.1016/j.eprac.2021.01.010
17. Mandura RA, Meligy OAE, Attar MH, Alamoudi RA. Diabetes mellitus and dental health in children: A review of literature. Int J Clin Pediatr Dent (2021) 14(5):719–25. doi: 10.5005/jp-journals-10005-2006
18. Napoli N, Chandran M, Pierroz DD, Abrahamsen B, Schwartz AV, Ferrari SL, et al. Mechanisms of diabetes mellitus-induced bone fragility. Nat Rev Endocrinol (2017) 13(4):208–19. doi: 10.1038/nrendo.2016.153
19. Li Y, Li B, Wang B, Liu M, Zhang X, Li A, et al. Integrated pancreatic microcirculatory profiles of streptozotocin-induced and insulin-administrated type 1 diabetes mellitus. Microcirculation (2021) 28(5):e12691. doi: 10.1111/micc.12691
20. Dorotheou D, Bochaton-Piallat ML, Giannopoulou C, Kiliaridis S. Expression of α-smooth muscle actin in the periodontal ligament during post-emergent tooth eruption. J Int Med Res (2018) 46(6):2423–35. doi: 10.1177/0300060518769545
21. Cortet B, Lucas S, Legroux-Gerot I, Penel G, Chauveau C, Paccou J. Bone disorders associated with diabetes mellitus and its treatments. Joint Bone Spine (2019) 86(3):315–20. doi: 10.1016/j.jbspin.2018.08.002
22. Schneider BJ, Meyer J. Experimental studies on the interrelations of condylar growth and alveolar bone formation. Angle Orthod (1965) 35:187–99. doi: 10.1043/0003-3219(1965)035
23. Cohn SA. Disuse atrophy of the periodontium in mice following partial loss of function. Arch Oral Biol (1966) 11(1):95–105. doi: 10.1016/0003-9969(66)90120-8
24. Levy GG, Mailland ML. Histologic study of the effects of occlusal hypofunction following antagonist tooth extraction in the rat. J Periodontol (1980) 51(7):393–9. doi: 10.1902/jop.1980.51.7.393
25. Arita K, Hotokezaka H, Hashimoto M, Nakano-Tajima T, Kurohama T, Kondo T, et al. Effects of diabetes on tooth movement and root resorption after orthodontic force application in rats. Orthod Craniofac Res (2016) 19(2):83–92. doi: 10.1111/ocr.12117
26. Wang C, Cao L, Yang C, Fan Y. A novel method to quantify longitudinal orthodontic bone changes with In vivo micro-CT data. J Healthc Eng (2018) 2018:1651097. doi: 10.1155/2018/1651097
27. Dixit M, Liu Z, Poudel SB, Yildirim G, Zhang YZ, Mehta S, et al. Skeletal response to insulin in the naturally occurring type 1 diabetes mellitus mouse model. JBMR Plus (2021) 5(5):e10483. doi: 10.1002/jbm4.10483
28. Lira Dos Santos EJ, Salmon CR, Chavez MB, de Almeida AB, Tan MH, Chu EY, et al. Cementocyte alterations associated with experimentally induced cellular cementum apposition in hyp mice. J Periodontol (2021) 92(11):116–27. doi: 10.1002/JPER.21-0119
29. Ullrich N, Schröder A, Jantsch J, Spanier G, Proff P, Kirschneck C. The role of mechanotransduction versus hypoxia during simulated orthodontic compressive strain-an in vitro study of human periodontal ligament fibroblasts. Int J Oral Sci (2019) 11(4):33. doi: 10.1038/s41368-019-0066-x
30. Morimoto C, Takedachi M, Kawasaki K, Shimomura J, Murata M, Hirai A, et al. Hypoxia stimulates collagen hydroxylation in gingival fibroblasts and periodontal ligament cells. J Periodontol (2021) 92(11):1635–45. doi: 10.1002/JPER.20-0670
31. K S, Vijayaraghavan N, Krishnan V. Time-dependent variation in expression patterns of lysyl oxidase, type I collagen and tropoelastin mRNA in response to orthodontic force application. Arch Oral Biol (2019) 102:218–24. doi: 10.1016/j.archoralbio.2019.04.016
32. Rosset EM, Bradshaw AD. SPARC/osteonectin in mineralized tissue. Matrix Biol (2016) 52-54:78–87. doi: 10.1016/j.matbio.2016.02.001
33. Ribeiro N, Sousa SR, Brekken RA, Monteiro FJ. Role of SPARC in bone remodeling and cancer-related bone metastasis. J Cell Biochem (2014) 115(1):17–26. doi: 10.1002/jcb.24649
34. Trombetta-eSilva J, Rosset EA, Hepfer RG, Wright GJ, Baicu C, Yao H, et al. Decreased mechanical strength and collagen content in SPARC-null periodontal ligament is reversed by inhibition of transglutaminase activity. J Bone Miner Res (2015) 30(10):1914–24. doi: 10.1002/jbmr.2522
35. Delany AM, Hankenson KD. Thrombospondin-2 and SPARC/osteonectin are critical regulators of bone remodeling. J Cell Commun Signal (2009) 3(3-4):227–38. doi: 10.1007/s12079-009-0076-0
36. Atorrasagasti C, Onorato A, Gimeno ML, Andreone L, Garcia M, Malvicini M, et al. SPARC Is required for the maintenance of glucose homeostasis and insulin secretion in mice. Clin Sci (Lond) (2019) 133(2):351–65. doi: 10.1042/CS20180714
37. Wu D, Li L, Yang M, Liu H, Yang G. Elevated plasma levels of SPARC in patients with newly diagnosed type 2 diabetes mellitus. Eur J Endocrinol (2011) 165(4):597–601. doi: 10.1530/EJE-11-0131
38. Ou M, Zhao Y, Zhang F, Huang X. Bmp2 and Bmp4 accelerate alveolar bone development. Connect Tissue Res (2015) 56(3):204–11. doi: 10.3109/03008207.2014.996701
39. Chen Y, Ma B, Wang X, Zha X, Sheng C, Yang P, et al. Potential functions of the BMP family in bone, obesity, and glucose metabolism. J Diabetes Res (2021) 2021:6707464. doi: 10.1155/2021/6707464
40. Wang L, Roth T, Abbott M, Ho L, Wattanachanya L, Nissenson RA. Osteoblast-derived FGF9 regulates skeletal homeostasis. Bone (2017) 98:18–25. doi: 10.1016/j.bone.2016.12.005
41. Wallner C, Schira J, Wagner JM, Schulte M, Fischer S, Hirsch T, et al. Application of VEGFA and FGF-9 enhances angiogenesis, osteogenesis and bone remodeling in type 2 diabetic long bone regeneration. PLoS One (2015) 10(3):e0118823. doi: 10.1371/journal.pone.0118823
Keywords: tooth movement, periodontal ligament, type 1 diabetes, alveolar bone remodeling, osteogenesis
Citation: Li W, Zheng J, Xu Y, Niu W, Guo D, Cui J, Bian W, Wang X and Niu J (2023) Remodeling of the periodontal ligament and alveolar bone during axial tooth movement in mice with type 1 diabetes. Front. Endocrinol. 14:1098702. doi: 10.3389/fendo.2023.1098702
Received: 15 November 2022; Accepted: 09 January 2023;
Published: 23 January 2023.
Edited by:
Michela Rossi, Bambino Gesù Children’s Hospital (IRCCS), ItalyReviewed by:
Xianqi Li, Matsumoto Dental University, JapanBing Wu, GE Healthcare, China
Xiaochun Wei, Second Hospital of Shanxi Medical University, China
Wei Chen, Southwest Hospital, China
Rongjie Bai, Beijing Jishuitan Hospital, China
Zhengwei Huang, Shanghai Jiao Tong University, China
Copyright © 2023 Li, Zheng, Xu, Niu, Guo, Cui, Bian, Wang and Niu. This is an open-access article distributed under the terms of the Creative Commons Attribution License (CC BY). The use, distribution or reproduction in other forums is permitted, provided the original author(s) and the copyright owner(s) are credited and that the original publication in this journal is cited, in accordance with accepted academic practice. No use, distribution or reproduction is permitted which does not comply with these terms.
*Correspondence: Jinliang Niu, sxlscjy@163.com