- Department of Endocrinology and Metabolism, First Hospital of Jilin University, Changchun, Jilin, China
Obesity is increasingly becoming a global epidemic of concern and is considered a risk factor for several endocrine-related cancers. Moreover, obesity is associated with cancer development and poor prognosis. As a metabolic abnormality, obesity leads to a series of changes in insulin, IGF-1, sex hormones, IGFBPs, and adipokines. Among these factors, IGF-1 plays an important role in obesity-related endocrine cancers. This review describes the role of obesity in endocrine-related cancers, such as prostate cancer, breast cancer and pancreatic cancer, focusing on the mechanism of IGF-1 and the crosstalk with estrogen and adipokines. In addition, this review briefly introduces the current status of IGF-1R inhibitors in clinical practice and shows the prospect of IGF-1R inhibitors in combination with other anticancer drugs.
1 Introduction
Obesity is a risk factor for several chronic diseases, such as hypertension, type 2 diabetes, dyslipidemia and cardiovascular disease. Epidemiological studies have shown that obesity is also a risk factor for certain types of cancer, such as postmenopausal breast cancer, prostate cancer, endometrial cancer, pancreatic cancer and thyroid cancer. Furthermore, a growing number of studies indicate that obesity predicts unfavorable outcomes for cancers (1). Obesity, a clinical marker of insulin resistance and metabolic syndrome, is associated with multiple biological metabolic changes, such as hyperinsulinemia, an increase in free fatty acid levels and triglycerides and hypoHDL-cholesterol. Hyperinsulinemia induces a decrease in insulin-like growth factor binding protein-1 and 2 (IGFBP-1 and 2) and reduces sex hormone binding globulin (SHBG) levels, resulting in an increase in free estrogen and androgen and insulin-like growth factor-1 (IGF-1) levels (2). The IGF system plays an important role in normal growth and development as well as in a variety of pathological situations, particularly tumorigenesis. Obesity is associated with an increased incidence of cancers arising from tissues responsive to estrogenic stimulation, including the endometrium, breast, and prostate (3). In postmenopausal women, elevated bioavailable plasma estrogen levels are related to an increased risk of breast cancer and endometrial cancer (4). Sex steroid alterations are associated with prostate cancer development and progression. This review will focus on the effects of IGF-1 on the relationships between obesity and endocrine-related cancer, especially on prostate cancer, breast cancer and pancreatic cancer.
2 Insulin-like growth factor-1
Insulin-like growth factors (IGFs) were first described in the late 1950s as skeletal growth factors produced in the liver in response to pituitary growth hormone (GH), and these growth factors play a fundamental role in regulating somatic growth according to nutritional conditions. IGFs, a group of polypeptide substances with growth-promoting effects, consist of IGF-1 and IGF-2. IGF-1 is produced in the liver, secreted into the circulation and acts in target tissues. In addition to the liver, IGF-1 is also produced in most extrahepatic tissues and functions as an endocrine, autocrine and paracrine growth stimulator to regulate cell growth (5). IGF-1 is a major target gene of growth hormone, and its product mediates many of the actions of growth hormone on growth and development. IGF action is also important in the development of specific organs, such as in the nervous system, in which IGF signaling regulates neuronal proliferation, apoptosis and cell survival. However, the IGF system has been implicated in various pathophysiological conditions and plays a particularly prominent role in the development and progression of human cancer (6). A growing body of epidemiological data suggests that high levels of circulating IGF-1 constitute a risk factor for the development of breast, prostate, colon, and lung cancer.
IGF-1 receptor (IGF-1R) is not mutated in most cancers and has a high degree of structural homology with insulin receptor (INSR), particularly in the tyrosine domain, which can form a heterodimer with each other and signal through many common mediators, but the two receptor signaling axes exhibit marked functional variance (7, 8). In addition, the expression levels of IGF-1R and INSR are predictive of cancer outcome. Experimentally, the modulation of IGF-1R activity affects the growth of many types of tumor cells. As a result of these findings, intensive effort is being directed toward investigating the utility of the IGF system as both a diagnostic marker and a therapeutic target in cancer therapy (6).
Six high-affinity IGF-binding proteins (IGFBP) are described at present. They are synthesized by several cell types, mostly from the fibroblast lineage, which regulate IGF-1 and IGF-2. In this family, IGFBP-3 is the most abundant IGFBP in blood, and IGFBP-2 is the second most abundant IGFBP. The IGF-1-IGFBP-3 complex and IGF1-IGFBP-5 complex bind to a third protein termed the acid labile subunit (9, 10). IGFBP-4, 5 and 6 are present in lower concentrations and appear to be less important for the regulation of free IGF concentrations in serum (5). More than 99% IGF-1 is bound to IGFBPs, which can increase the half-life of IGF to some extent, and their binding affinity for IGF is nearly 10 times higher than that for IGF-1R (10, 11). Therefore, the function and concentration of IGFBPs are critical to regulate the biological actions of IGFs.
3 Endocrine-related cancer
Circulating insulin and IGF-1 increase in the obese state, and sex hormones, adipokines and other inflammatory factors also vary (12). Both IGF and insulin can increase the expression of IGF-1R and INSR, which forms a functional network of interactions. In addition, IGFBPs are produced at lower levels, which reduces their inhibitory effects on insulin/IGF. IGF-1 has been studied more frequently in prostate cancer. In addition to IGF-1, estrogen is critical in breast cancer, and adiponectin is receiving increasing attention in pancreatic cancer; leptin and adiponectin have been relatively less studied in endocrine-related cancers. To varying degrees, these biological factors play a role in endocrine-related cancers.
3.1 Prostate cancer
A correlation between obesity and prostate cancer risk has been reported in a number of studies, especially in abdominal obesity, with a linear relationship between increasing BMI and prostate cancer (13). Moreover, obesity is associated with an increased risk of recurrence after treatment, advanced cancer progression and prostate cancer-specific mortality for prostate cancer patients (14, 15) Obesity and chronic hyperinsulinemia are known to reduce the production of IGFBPs and increase IGF-1 biological activity (16). The insulin/IGF-1 axis is associated with obesity-induced prostate carcinogenesis via the phosphatidylinostitol-3 kinase (PI3K)/Akt/mTOR pathways. Using Hi-Myc/HIT mice, Wang et al. showed that IGF-1 promotes prostate cancer and that the IGF-1/AKT/FOXO3A/BIM pathway plays an important role (17). Prostate cancer cells overexpress IGF-1R and INSR (18). In vitro and in vivo experiments suggest that both IGF-1R and INSR promote angiogenesis in prostate cancer (19). Sayeed et al. showed that IGF-IR signaling strictly regulates prosurvival signaling in prostate cancer by controlling the expression of a5b1 integrin, which indicates that IGF-1R and INSR promote the growth and invasion of prostate cancer (20). Therefore, changes in IGFS in obese people may increase the potential risk of prostate cancer.
According to a recent study by Markers et al., the serum levels of steroid hormones were not associated with prostate cancer risk in obese men, which is consistent with most previous studies (21). However, many studies have indicated that low testosterone and elevated estrogen levels in obese men are correlated with the development of prostate cancer (22). To clarify these contradictory results, further investigation of the underlying mechanisms is still needed. Leptin has prostate cancer-promoting effects and is positively correlated with fat mass, while adiponectin has anticancer effects and is negatively correlated with BMI (23, 24). The ratio between leptin and adiponectin is imbalanced in obese individuals, leading to abnormalities in the AMPK and mTOR signaling pathways, which may influence prostate cancer development (25). In addition, adipose tissue itself is also associated with prostate cancer. Periprostatic adipose tissue secretes a variety of inflammatory factors and creates a tumor microenvironment that promotes the development of prostate cancer (26–29).
3.2 Breast cancer
Obesity is associated with the occurrence and progression of breast cancer, especially postmenopausal ER+/PR+ breast cancer (30, 31). According to a systematic evaluation, higher levels of IGF-1, IGFBP-3 and leptin and lower levels of adiponectin among obesity-associated protein biomarkers are correlated with an increased breast cancer risk (32). The association between IGFBP-3 and breast cancer risk is reportedly due to its interaction with IGF-1, and this interaction can be eliminated by the adjustment of IGF-1, suggesting that IGF-1 itself is an inducing factor of breast cancer (33).
In addition to the direct regulation of estrogen and these obesity-associated protein biomarkers on breast cancer cells, these biological factors exhibit crosstalk. The role of IGF-1 is mediated by IGF-1R, which is overexpressed in breast cancer (34, 35). IGF-1R promotes breast cancer by altering the expression of proliferation and survival genes through the Ras/Raf/MAPK and PI3K/Akt signaling pathways (36, 37). Insulin can also promote breast cancer via these signaling pathways (38). The estrogen-induced production of ROS leads to DNA damage, while estrogen itself inhibits the DNA damage response and can promote cell proliferation, which is the background of estrogen-induced breast cancer (39–41). Postmenopausal adipose tissue is an important site of estrogen production. The rate of estrogen conversion is higher in obese postmenopausal women, and obesity increases estrogen levels in ER receptor-positive breast cancer tissue, which increases the risk associated with breast cancer (42, 43). This risk is further increased by a reduction in SHBG in the liver of individuals with obesity and an increase in the concentration of non-SHBG-bound estradiol (E2, the most abundant and active estrogen) (44, 45). Similar to IGF-1, estrogen can also play a role in breast cancer through the MAPK and PI3K/Akt signaling pathways, and estrogen and IGF-1 exhibit crosstalk. E2 accelerates and enhances the binding of ERα to IGF-1R, and ERα, activated by the Ras-MAPK cascade of the growth factor signaling pathway, induces the phosphorylation of Akt and ERK1/2 after rapid binding to IGF-1R, thereby enlarging IGF-1R signaling (46). Leptin, which is associated with estrogen, also contributes to breast cancer. It may upregulate the PI3K/Akt pathway, MAPK pathway and STAT3 pathway, the downstream signaling pathways of Ob-R, which stimulate oncogenesis (47). Interestingly, elevated cAMP may inhibit leptin-induced migration of highly aggressive breast cancer cells MDA-MB-231 by suppressing ERK1/2 and STAT3 signaling pathway (48, 49). Leptin also promotes the proliferation of breast cancer cells by activating the Wnt/β-catenin pathway (50). Aromatase is the rate-limiting enzyme for estrogen synthesis, and leptin can induce aromatase expression through COX-2 expression in breast cancer cells and increase estrogen synthesis, promoting the progression of breast cancer (51, 52). Zahid et al. showed that the increase in aromatase expression in the obese state may be mediated by leptin via the P53-HIF1α/PKM2-aromatase axis (53). In addition, Morad et al. suggested that estrogen can in turn increase the expression of leptin and leptin receptors (54). In ERα-negative breast cancer, adiponectin inhibited IGF-1-induced cell migration, whereas in ERα-positive breast cancer, low concentrations of adiponectin promoted IGF-1R phosphorylation and thus enhanced IGF-1/IGF-1R signaling (55, 56). The concentration of adiponectin tends to decrease in obese states, which may reduce the protective effect and increase the risk of breast cancer. In conclusion, in obese women, especially postmenopausal women, the role of estrogen and obesity-associated protein biomarkers in breast cancer is complicated due to their own changes and crosstalk.
3.3 Pancreatic cancer
Epidemiological surveys show that obesity is associated with pancreatic cancer morbidity and mortality, and the morbidity of pancreatic cancer increases with increasing BMI (57, 58). Since approximately 90% of pancreatic cancers are pancreatic ductal carcinoma (PDAC), many models have been built with PDAC. Various events are considered to elevate the risk of pancreatic cancer in obesity, such as the increase in IGF-1. A survey including 105 patients showed that IGF-1R was overexpressed in more than half of the PDAC samples (59). Du et al. indicated that high IGF-1R expression was associated with shorter overall survival and relapse in patients (60). In PDAC, both insulin and IGF-1 are considered to play a role in cancer development and progression, with the PI3K and MAPK signaling pathways as its central pathways, and these signals are enhanced in obesity (61, 62). Tian et al. inhibited the PI3K/Akt signaling pathway by knocking down the IGF-1R gene, thereby inhibiting the proliferation of pancreatic cancer cells and increasing the sensitivity of anticancer drugs, which to some extent proves the role of IGF-1 in pancreatic cancer (63). Oncogenic KRAS mutation occurs in approximately 90% of PDAC, and MEK/ERK is one of the main effector pathways of KRAS signaling (64). MEK/ERK-stimulated IGF-1R signaling is required for murine pancreatic epithelial cell transformation, which suggests a critical role for IGF-1R signaling in pancreatic carcinogenesis (65). In addition, leptin, adiponectin and inflammatory factors also play an important role in the association between obesity and pancreatic cancer (66, 67). Adiponectin acts by binding to AdipoRs to activate downstream signaling pathways and AdipoRon is a synthetic small molecule AdipoR agonist that binds to the AdipoR1 and AdipoR2. AdipoRon induces pancreatic cancer cell death by activating ERK1/2, however, obesity weakens this anti-cancer effect (68, 69). Messaggio et al. demonstrated that leptin promotes pancreatic tumor growth through altered pathways of STAT3 and PI3K/AKT signaling, whereas AdipoRon inhibits leptin-induced STAT3 activation and pancreatic tumor growth in vivo (70, 71). Recently, Ragone et al. combined gemcitabine, a first-line agent for pancreatic cancer, with AdipoRon to enhance growth inhibition in human PDAC cell lines, which may be associated with the AdipoRon mediated p44/42 MAPK(ERK1/2) pathway (72).
3.4 Other endocrine-related cancers
Ovarian, endometrial and thyroid cancers are also associated with obesity. In ovarian cancer, epidemiological studies show that obesity may promote the peritoneal dissemination of ovarian cancer and increase patient mortality (73, 74). Ignacio et al. suggest that obesity may accelerate the peritoneal dissemination of ovarian cancer by producing more proinflammatory chemokines and recruiting macrophages (75). Leptin helps maintain the cancer stem cell-like properties of ovarian cancer cells and stimulates the migration and invasion of ovarian cancer cells (76). IGF-IR mediates TGFβ for the maintenance of EMT transformation, angiogenesis and extracellular matrix remodeling (77). Together, these factors contribute to the development of ovarian cancer. In endometrial cancer, epidemiological studies have shown that obesity is associated with an increased incidence of endometrial cancer and is correlated with recurrence and death in patients with endometrial cancer (78). Merritt et al. indicated that estrogen, insulin, and IGF-1 are associated with the multistage process of endometrial carcinogenesis (79). Estrogen can stimulate cell proliferation by increasing IGF-1 synthesis and IGF-IR expression in the uterus via ERα (80). In addition, in the absence of IGFBP-1 synthesis in obesity, IGF-1 may further induce the abnormal proliferation of endometrial cells, which is a possible pathway for endometrial cancer formation (81). In thyroid cancer, a cohort study collected more than 450,000 samples from 1995 to 2015 and suggested that being overweight and obesity may be important causes of the increased incidence of thyroid cancer (82). IGF-1 may play a role in the association between obesity and thyroid cancer (83). IGF-1 is positively associated with the risk of developing thyroid cancer (84). Yang et al. suggested that IGF-1 promotes the proliferation and invasion of thyroid-like carcinoma through the STAT3 pathway (85). Studies have also indicated a role for the IGF axis in thyroid tumorigenesis (86).
4 Therapies targeting the IGF family
In the face of increasing cancer incidence, researchers have targeted the IGF axis for therapeutic intervention due to its role in cancer.Three main approaches are used to target IGFs, including IGF-1R monoclonal antibodies, IGF-1R tyrosine kinase inhibitors (TKIs) and IGF-1/-2 blocking monoclonal antibodies. IGF-1R monoclonal antibodies (IGF-1R mAb, such as cixutumumab, figitumumab and ganitumab) function mainly by blocking ligand-receptor action, inducing the internalization/degradation of IGF-1R and partially downregulating IGF-1R/INSR hybrid receptors (87, 88). Among IGF-1R TKIs, ATP-competitive TKIs act by competing for the binding site of the IGF-1R kinase domain to ATP, which inhibits both IGF-1R and INSR. However, non-ATP competitive IGF-1R inhibitors do not influence INSR (89, 90). IGF-1/-2 blocking mAb blocks IGF-1R and INSR-A and their hybrid receptors from transducing proliferative/anti-apoptotic signals by binding IGF-1 and IGF-2 without affecting INSR-B and insulin function (91). For more than a decade, researchers have conducted numerous clinical trials on these three classes of inhibitors due to the role of IGF-1 in tumor development (Table 1). Unfortunately, the results of most clinical trials targeting single-agent activity were disappointing. Some IGF-1R inhibitors have been discontinued or abandoned from development; for example, Pfizer decided to discontinue figitumumab (CP-751871) in 2010, and Roche shifted to teprotumumab to treat ophthalmic disease.
One possible reason for the resistance of cancer cells to IGF-1R inhibitors is the compensatory activation of RTK signaling; therefore, several combination therapies have recently emerged to improve efficacy (104, 105), such as IGF-1R inhibitors in combination with other RTK inhibitors. EGFR is an important factor in the RTK signaling pathway, and the IGF-1/2 neutralizing antibody m708.5 exhibits a strong synergistic effect with gefitinib, which shows benefit in the treatment of neuroblastoma and breast cancer (106). IGF-1R inhibitors are valuable in endocrine-related tumors. For example, the activation of the IGF-1R pathway is associated with tamoxifen resistance, while the elimination of IGF-1R signaling with linsitinib could restore the sensitivity of endocrine therapy (107). Combined IGF-1R/mTOR inhibition also shows synergistic effects. In ACC, in vitro assays showed stronger antiproliferative activity in combination with sirolimus or everolimus than with linsitinib (108). A phase I clinical trial of ridaforolimus in combination with anti-IGF1R mAb dalozumab also demonstrated clinical activity in advanced cancer (109). Some experimental support also exists for the combination of IGF-1R inhibitors with therapeutic approaches to induce DNA damage. Most invasive breast cancers have insulin/IGF-1R signaling activation, and xentuzumab in combination with paclitaxel can effectively reduce metastasis incidence and metastatic burden in preclinical mouse models (110). IGF-1R is also involved in radiotherapy resistance. Low IGF-1R expression was demonstrated to increase the sensitivity of CRC to radiation therapy when treated with NVP-ADW742 in CRC cells (111, 112). Some anticancer drugs containing IGF-1R inhibitors have entered in phase III clinical trials, but clinical benefit is not significant. More clinical trials are needed to prove the effectiveness of the combination therapy.
5 Conclusion
Obesity has been called the disease of modern civilization and is a heavy disease burden for society. Disorders of insulin, IGF-1, sex hormones, adipokines and inflammatory factors have been observed in numerous studies in the presence of obesity. Obesity is also recognized as a factor associated with cancer, and adipose tissue, which is an active endocrine organ, strengthens the link between obesity and endocrine-related cancers. IGF-1 plays an important role in obesity-associated endocrine-related cancers, promoting cancer development mainly through the PI3K/AKT and MAPK pathways. Insulin, IGF-1, sex hormones and adipokines also exhibit crosstalk to synergistically function in this process. The relationships among them and their roles are shown in Figure 1. Investigators have conducted a series of clinical trials targeting IGFs; however, most of them failed to demonstrate single-agent efficacy. Although drug combinations perform better in preclinical studies, fewer patients benefit from clinical trials. The following issues remain in the study of obesity and endocrine-related cancers: 1. the mechanism of action of obesity-related biologic factors in cancer and the crosstalk among them still need to be studied further; 2. whether obesity-related biologic factors can be used as biomarkers to evaluate endocrine-related cancers has not been fully investigated; 3. suitable predictive biomarkers urgently need to be identified in clinical trials to design reasonable joint therapeutic strategies accordingly. If these problems are solved in the future, IGF inhibitors can play a larger role in clinical practice and provide more treatment options for cancer patients.
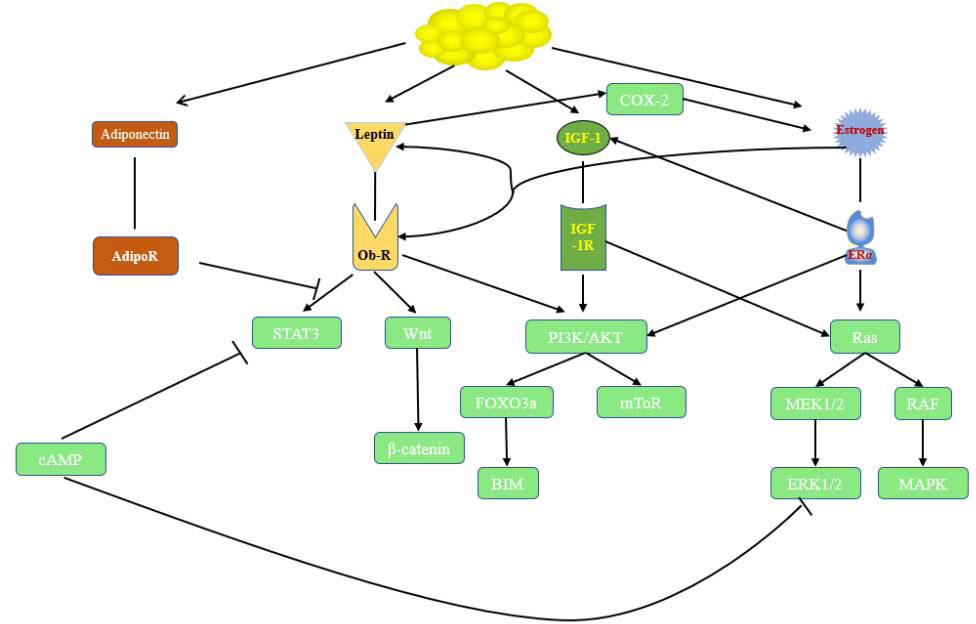
Figure1 Relationships and roles in endocrine-related cancer. cAMP, cyclic adenosine monophosphate; ObR, leptin receptor; STAT3, signal transducer and activator of transcription 3; FOXO3a, Forkhead box O3; IGF-1, insulin-like growth factor; IGF-1R, insulin-like growth factor receptor 1; P13K/Akt, phosphatidylinositol 3-kinase/protein kinase B; ERα, estrogen receptor α; MAPK,mitogen-activated protein kinase;MEK,mitogen-activated protein kinase;ERK, extractor signal regulated kinase.
Author contributions
WZ wrote and revised the manuscript. ZL wrote the outline and critically revised the manuscript. WZ, XW, YW, GS and JZ contributed to literature search. WZ and XW prepared the table and figure. All authors have read and given approval of the final manuscript.
Funding
This study was supported by the National Natural Science Foundation of China (82070871).
Conflict of interest
The authors declare that the research was conducted in the absence of any commercial or financial relationships that could be construed as a potential conflict of interest.
Publisher’s note
All claims expressed in this article are solely those of the authors and do not necessarily represent those of their affiliated organizations, or those of the publisher, the editors and the reviewers. Any product that may be evaluated in this article, or claim that may be made by its manufacturer, is not guaranteed or endorsed by the publisher.
References
1. Sung H, Siegel RL, Torre LA, Pearson-Stuttard J, Islami F, Fedewa SA, et al. Global patterns in excess body weight and the associated cancer burden. CA: Cancer J Clin (2019) 69(2):88–112. doi: 10.3322/caac.21499
2. Amin MN, Hussain MS, Sarwar MS, Rahman Moghal MM, Das A, Hossain MZ, et al. How the association between obesity and inflammation may lead to insulin resistance and cancer. Diabetes Metab Syndr (2019) 13(2):1213–24. doi: 10.1016/j.dsx.2019.01.041
3. Crujeiras AB, Casanueva FF. Obesity and the reproductive system disorders: Epigenetics as a potential bridge. Hum Reprod Update (2015) 21(2):249–61. doi: 10.1093/humupd/dmu060
4. Zhao H, Zhou L, Shangguan AJ, Bulun SE. Aromatase expression and regulation in breast and endometrial cancer. J Mol Endocrinol (2016) 57(1):R19–33. doi: 10.1530/JME-15-0310
5. Clemmons DR. Modifying Igf1 activity: An approach to treat endocrine disorders, atherosclerosis and cancer. Nat Rev Drug Discovery (2007) 6(10):821–33. doi: 10.1038/nrd2359
6. Cao J, Yee D. Disrupting insulin and igf receptor function in cancer. Int J Mol Sci (2021) 22(2):555. doi: 10.3390/ijms22020555
7. Maki RG. Small is beautiful: Insulin-like growth factors and their role in growth, development, and cancer. J Clin Oncol (2010) 28(33):4985–95. doi: 10.1200/JCO.2009.27.5040
8. Osher E, Macaulay VM. Therapeutic targeting of the igf axis. Cells (2019) 8(8):895. doi: 10.3390/cells8080895
9. Cai Q, Dozmorov M, Oh Y. Igfbp-3/Igfbp-3 receptor system as an anti-tumor and anti-metastatic signaling in cancer. Cells (2020) 9(5):1261. doi: 10.3390/cells9051261
11. Fettig LM, Yee D. Advances in insulin-like growth factor biology and -directed cancer therapeutics. Adv Cancer Res (2020) 147:229–57. doi: 10.1016/bs.acr.2020.04.005
12. Nimptsch K, Konigorski S, Pischon T. Diagnosis of obesity and use of obesity biomarkers in science and clinical medicine. Metabolism (2019) 92:61–70. doi: 10.1016/j.metabol.2018.12.006
13. Choi JB, Myong JP, Lee Y, Kim I, Kim JH, Hong SH, et al. Does increased body mass index lead to elevated prostate cancer risk? it depends on waist circumference. BMC Cancer (2020) 20(1):589. doi: 10.1186/s12885-020-07089-5
14. Freedland SJ, Branche BL, Howard LE, Hamilton RJ, Aronson WJ, Terris MK, et al. Obesity, risk of biochemical recurrence, and prostate-specific antigen doubling time after radical prostatectomy: Results from the search database. BJU Int (2019) 124(1):69–75. doi: 10.1111/bju.14594
15. Genkinger JM, Wu K, Wang M, Albanes D, Black A, van den Brandt PA, et al. Measures of body fatness and height in early and mid-to-Late adulthood and prostate cancer: Risk and mortality in the pooling project of prospective studies of diet and cancer. Ann Oncol (2020) 31(1):103–14. doi: 10.1016/j.annonc.2019.09.007
16. Uehara H, Kobayashi T, Matsumoto M, Watanabe S, Yoneda A, Bando Y. Adipose Tissue:Critical contributor to the development of prostate cancer. J Med Invest (2018) 65(1.2):9–17. doi: 10.2152/jmi.65.9
17. Wang S, Wang N, Yu B, Cao M, Wang Y, Guo Y, et al. Circulating igf-1 promotes prostate adenocarcinoma Via Foxo3a/Bim signaling in a double-transgenic mouse model. Oncogene (2019) 38(36):6338–53. doi: 10.1038/s41388-019-0880-9
18. Breen KJ, O'Neill A, Murphy L, Fan Y, Boyce S, Fitzgerald N, et al. Investigating the role of the igf axis as a predictor of biochemical recurrence in prostate cancer patients post-surgery. Prostate (2017) 77(12):1288–300. doi: 10.1002/pros.23389
19. Heidegger I, Kern J, Ofer P, Klocker H, Massoner P. Oncogenic functions of Igf1r and insr in prostate cancer include enhanced tumor growth, cell migration and angiogenesis. Oncotarget (2014) 5(9):2723–35. doi: 10.18632/oncotarget.1884
20. Sayeed A, Fedele C, Trerotola M, Ganguly KK, Languino LR. Igf-ir promotes prostate cancer growth by stabilizing Alpha5beta1 integrin protein levels. PloS One (2013) 8(10):e76513. doi: 10.1371/journal.pone.0076513
21. Chau CH, Till C, Price DK, Goodman PJ, Neuhouser ML, Pollak MN, et al. Serum markers, obesity and prostate cancer risk: Results from the prostate cancer prevention trial. Endocr Relat Cancer (2022) 29(2):99–109. doi: 10.1530/ERC-21-0107
22. Allott EH, Masko EM, Freedland SJ. Obesity and prostate cancer: Weighing the evidence. Eur Urol (2013) 63(5):800–9. doi: 10.1016/j.eururo.2012.11.013
23. Kamel HFM, Nassir AM, Al Refai AA. Assessment of expression levels of leptin and leptin receptor as potential biomarkers for risk of prostate cancer development and aggressiveness. Cancer Med (2020) 9(15):5687–96. doi: 10.1002/cam4.3082
24. Yoon YS, Kwon AR, Lee YK, Oh SW. Circulating adipokines and risk of obesity related cancers: A systematic review and meta-analysis. Obes Res Clin Pract (2019) 13(4):329–39. doi: 10.1016/j.orcp.2019.03.006
25. Hu X, Hu C, Zhang C, Zhang M, Long S, Cao Z. Role of adiponectin in prostate cancer. Int Braz J Urol (2019) 45(2):220–8. doi: 10.1590/S1677-5538.IBJU.2018.0261
26. La Civita E, Liotti A, Cennamo M, Crocetto F, Ferro M, Liguoro P, et al. Peri-prostatic adipocyte-released tgfbeta enhances prostate cancer cell motility by upregulation of connective tissue growth factor. Biomedicines (2021) 9(11):1692. doi: 10.3390/biomedicines9111692
27. Mangiola S, Stuchbery R, Macintyre G, Clarkson MJ, Peters JS, Costello AJ, et al. Periprostatic fat tissue transcriptome reveals a signature diagnostic for high-risk prostate cancer. Endocr Relat Cancer (2018) 25(5):569–81. doi: 10.1530/ERC-18-0058
28. Nassar ZD, Aref AT, Miladinovic D, Mah CY, Raj GV, Hoy AJ, et al. Peri-prostatic adipose tissue: The metabolic microenvironment of prostate cancer. BJU Int (2018) 121(S3):9–21. doi: 10.1111/bju.14173
29. Gucalp A, Iyengar NM, Zhou XK, Giri DD, Falcone DJ, Wang H, et al. Periprostatic adipose inflammation is associated with high-grade prostate cancer. Prostate Cancer Prostatic Dis (2017) 20(4):418–23. doi: 10.1038/pcan.2017.31
30. Neuhouser ML, Aragaki AK, Prentice RL, Manson JE, Chlebowski R, Carty CL, et al. Overweight, obesity, and postmenopausal invasive breast cancer risk: A secondary analysis of the women's health initiative randomized clinical trials. JAMA Oncol (2015) 1(5):611–21. doi: 10.1001/jamaoncol.2015.1546
31. Chan DSM, Vieira AR, Aune D, Bandera EV, Greenwood DC, McTiernan A, et al. Body mass index and survival in women with breast cancer-systematic literature review and meta-analysis of 82 follow-up studies. Ann Oncol (2014) 25(10):1901–14. doi: 10.1093/annonc/mdu042
32. Wu X, Zhang X, Hao Y, Li J. Obesity-related protein biomarkers for predicting breast cancer risk: An overview of systematic reviews. Breast Cancer (2021) 28(1):25–39. doi: 10.1007/s12282-020-01182-0
33. Endogenous H, Breast Cancer Collaborative G, Key TJ, Appleby PN, Reeves GK, Roddam AW. Insulin-like growth factor 1 (Igf1), igf binding protein 3 (Igfbp3), and breast cancer risk: Pooled individual data analysis of 17 prospective studies. Lancet Oncol (2010) 11(6):530–42. doi: 10.1016/S1470-2045(10)70095-4
34. Silva Rocha F, da Silva Maues JH, Brito Lins Pereira CM, Moreira-Nunes CA, Rodriguez Burbano RM. Analysis of increased egfr and igf-1r signaling and its correlation with socio-epidemiological features and biological profile in breast cancer patients: A study in northern Brazil. Breast Cancer (Dove Med Press) (2021) 13:325–39. doi: 10.2147/BCTT.S308554
35. Yerushalmi R, Gelmon KA, Leung S, Gao D, Cheang M, Pollak M, et al. Insulin-like growth factor receptor (Igf-1r) in breast cancer subtypes. Breast Cancer Res Treat (2012) 132(1):131–42. doi: 10.1007/s10549-011-1529-8
36. Bowers LW, Cavazos DA, Maximo IX, Brenner AJ, Hursting SD, deGraffenried LA. Obesity enhances nongenomic estrogen receptor crosstalk with the Pi3k/Akt and mapk pathways to promote in vitro measures of breast cancer progression. Breast Cancer Res (2013) 15(4):R59. doi: 10.1186/bcr3453
37. Zhang X, Lin M, van Golen KL, Yoshioka K, Itoh K, Yee D. Multiple signaling pathways are activated during insulin-like growth factor-I (Igf-I) stimulated breast cancer cell migration. Breast Cancer Res Treat (2005) 93(2):159–68. doi: 10.1007/s10549-005-4626-8
38. Yee LD, Mortimer JE, Natarajan R, Dietze EC, Seewaldt VL. Metabolic health, insulin, and breast cancer: Why oncologists should care about insulin. Front Endocrinol (Lausanne) (2020) 11:58. doi: 10.3389/fendo.2020.00058
39. Zhang S, Shang P, Gao K, Zhao G, Zhou J, Chen R, et al. Dynamics of estrogen-induced ros and DNA strand break generation in estrogen receptor alpha-positive breast cancer. Biochem Biophys Res Commun (2022) 602:170–8. doi: 10.1016/j.bbrc.2022.02.089
40. Rajan A, Nadhan R, Latha NR, Krishnan N, Warrier AV, Srinivas P. Deregulated estrogen receptor signaling and DNA damage response in breast tumorigenesis. Biochim Biophys Acta Rev Cancer (2021) 1875(1):188482. doi: 10.1016/j.bbcan.2020.188482
41. Wu CH, Chuang HY, Wang CL, Hsu CY, Long CY, Hsieh TH, et al. Estradiol induces cell proliferation in Mcf7 mammospheres through Her2/Cox2. Mol Med Rep (2019) 19(3):2341–9. doi: 10.3892/mmr.2019.9879
42. Kakugawa Y, Tada H, Kawai M, Suzuki T, Nishino Y, Kanemura S, et al. Associations of obesity and physical activity with serum and intratumoral sex steroid hormone levels among postmenopausal women with breast cancer: Analysis of paired serum and tumor tissue samples. Breast Cancer Res Treat (2017) 162(1):115–25. doi: 10.1007/s10549-016-4094-3
43. Hetemaki N, Savolainen-Peltonen H, Tikkanen MJ, Wang F, Paatela H, Hamalainen E, et al. Estrogen metabolism in abdominal subcutaneous and visceral adipose tissue in postmenopausal women. J Clin Endocrinol Metab (2017) 102(12):4588–95. doi: 10.1210/jc.2017-01474
44. Lipworth L, Adami HO, Trichopoulos D, Carlstrom K, Mantzoros C. Serum steroid hormone levels, sex hormone-binding globulin, and body mass index in the etiology of postmenopausal breast cancer. Epidemiology (1996) 7(1):96–100. doi: 10.1097/00001648-199601000-00017
45. Grasa MDM, Gulfo J, Camps N, Alcala R, Monserrat L, Moreno-Navarrete JM, et al. Modulation of shbg binding to testosterone and estradiol by sex and morbid obesity. Eur J Endocrinol (2017) 176(4):393–404. doi: 10.1530/EJE-16-0834
46. Yu Z, Gao W, Jiang E, Lu F, Zhang L, Shi Z, et al. Interaction between igf-ir and er induced by E2 and igf-I. PloS One (2013) 8(5):e62642. doi: 10.1371/journal.pone.0062642
47. Schmidt S, Monk JM, Robinson LE, Mourtzakis M. The integrative role of leptin, oestrogen and the insulin family in obesity-associated breast cancer: Potential effects of exercise. Obes Rev (2015) 16(6):473–87. doi: 10.1111/obr.12281
48. Naviglio S, Di Gesto D, Romano M, Sorrentino A, Illiano F, Sorvillo L, et al. Leptin enhances growth inhibition by camp elevating agents through apoptosis of mda-Mb-231 breast cancer cells. Cancer Biol Ther (2009) 8(12):1183–90. doi: 10.4161/cbt.8.12.8562
49. Naviglio S, Di Gesto D, Illiano F, Chiosi E, Giordano A, Illiano G, et al. Leptin potentiates antiproliferative action of camp elevation Via protein kinase a down-regulation in breast cancer cells. J Cell Physiol (2010) 225(3):801–9. doi: 10.1002/jcp.22288
50. Liang X, Wang S, Wang X, Zhang L, Zhao H, Zhang L. Leptin promotes the growth of breast cancer by upregulating the Wnt/Beta-catenin pathway. Exp Ther Med (2018) 16(2):767–71. doi: 10.3892/etm.2018.6212
51. Hosney M, Sabet S, El-Shinawi M, Gaafar KM, Mohamed MM. Leptin is overexpressed in the tumor microenvironment of obese patients with estrogen receptor positive breast cancer. Exp Ther Med (2017) 13(5):2235–46. doi: 10.3892/etm.2017.4291
52. Kim HG, Jin SW, Kim YA, Khanal T, Lee GH, Kim SJ, et al. Leptin induces creb-dependent aromatase activation through cox-2 expression in breast cancer cells. Food Chem Toxicol (2017) 106(Pt A):232–41. doi: 10.1016/j.fct.2017.05.058
53. Zahid H, Subbaramaiah K, Iyengar NM, Zhou XK, Chen IC, Bhardwaj P, et al. Leptin regulation of the P53-Hif1alpha/Pkm2-Aromatase axis in breast adipose stromal cells: A novel mechanism for the obesity-breast cancer link. Int J Obes (Lond) (2018) 42(4):711–20. doi: 10.1038/ijo.2017.273
54. Morad V, Abrahamsson A, Dabrosin C. Estradiol affects extracellular Leptin:Adiponectin ratio in human breast tissue in vivo. J Clin Endocrinol Metab (2014) 99(9):3460–7. doi: 10.1210/jc.2014-1129
55. Mauro L, Naimo GD, Gelsomino L, Malivindi R, Bruno L, Pellegrino M, et al. Uncoupling effects of estrogen receptor alpha on Lkb1/Ampk interaction upon adiponectin exposure in breast cancer. FASEB J (2018) 32(8):4343–55. doi: 10.1096/fj.201701315R
56. Mauro L, Pellegrino M, Giordano F, Ricchio E, Rizza P, De Amicis F, et al. Estrogen receptor-alpha drives adiponectin effects on cyclin D1 expression in breast cancer cells. FASEB J (2015) 29(5):2150–60. doi: 10.1096/fj.14-262808
57. Huang J, Lok V, Ngai CH, Zhang L, Yuan J, Lao XQ, et al. Worldwide burden of, risk factors for, and trends in pancreatic cancer. Gastroenterology (2021) 160(3):744–54. doi: 10.1053/j.gastro.2020.10.007
58. Carreras-Torres R, Johansson M, Gaborieau V, Haycock PC, Wade KH, Relton CL, et al. The role of obesity, type 2 diabetes, and metabolic factors in pancreatic cancer: A mendelian randomization study. J Natl Cancer Inst (2017) 109(9):djx012. doi: 10.1093/jnci/djx012
59. Valsecchi ME, McDonald M, Brody JR, Hyslop T, Freydin B, Yeo CJ, et al. Epidermal growth factor receptor and insulinlike growth factor 1 receptor expression predict poor survival in pancreatic ductal adenocarcinoma. Cancer (2012) 118(14):3484–93. doi: 10.1002/cncr.26661
60. Du C, da Silva A, Morales-Oyarvide V, Dias Costa A, Kozak MM, Dunne RF, et al. Insulin-like growth factor-1 receptor expression and disease recurrence and survival in patients with resected pancreatic ductal adenocarcinoma. Cancer Epidemiol Biomarkers Prev (2020) 29(8):1586–95. doi: 10.1158/1055-9965.EPI-19-1315
61. Chang ML. Fatty pancreas-centered metabolic basis of pancreatic adenocarcinoma: From obesity, diabetes and pancreatitis to oncogenesis. Biomedicines (2022) 10(3):692. doi: 10.3390/biomedicines10030692
62. Deng J, Guo Y, Du J, Gu J, Kong L, Tao B, et al. The intricate crosstalk between insulin and pancreatic ductal adenocarcinoma: A review from clinical to molecular. Front Cell Dev Biol (2022) 10:844028. doi: 10.3389/fcell.2022.844028
63. Tian X, Hao K, Qin C, Xie K, Xie X, Yang Y. Insulin-like growth factor 1 receptor promotes the growth and chemoresistance of pancreatic cancer. Dig Dis Sci (2013) 58(9):2705–12. doi: 10.1007/s10620-013-2673-2
64. Mann KM, Ying H, Juan J, Jenkins NA, Copeland NG. Kras-related proteins in pancreatic cancer. Pharmacol Ther (2016) 168:29–42. doi: 10.1016/j.pharmthera.2016.09.003
65. Appleman VA, Ahronian LG, Cai J, Klimstra DS, Lewis BC. Kras(G12d)- and Braf(V600e)-induced transformation of murine pancreatic epithelial cells requires Mek/Erk-stimulated Igf1r signaling. Mol Cancer Res (2012) 10(9):1228–39. doi: 10.1158/1541-7786.MCR-12-0340-T
66. Jiang J, Fan Y, Zhang W, Shen Y, Liu T, Yao M, et al. Adiponectin suppresses human pancreatic cancer growth through attenuating the beta-catenin signaling pathway. Int J Biol Sci (2019) 15(2):253–64. doi: 10.7150/ijbs.27420
67. Harbuzariu A, Rampoldi A, Daley-Brown DS, Candelaria P, Harmon TL, Lipsey CC, et al. Leptin-notch signaling axis is involved in pancreatic cancer progression. Oncotarget (2017) 8(5):7740–52. doi: 10.18632/oncotarget.13946
68. Takenaga K, Akimoto M, Koshikawa N, Nagase H. Obesity reduces the anticancer effect of adiporon against orthotopic pancreatic cancer in diet-induced obese mice. Sci Rep (2021) 11(1):2923. doi: 10.1038/s41598-021-82617-2
69. Akimoto M, Maruyama R, Kawabata Y, Tajima Y, Takenaga K. Antidiabetic adiponectin receptor agonist adiporon suppresses tumour growth of pancreatic cancer by inducing Ripk1/Erk-dependent necroptosis. Cell Death Dis (2018) 9(8):804. doi: 10.1038/s41419-018-0851-z
70. Mendonsa AM, Chalfant MC, Gorden LD, VanSaun MN. Modulation of the leptin receptor mediates tumor growth and migration of pancreatic cancer cells. PloS One (2015) 10(4):e0126686. doi: 10.1371/journal.pone.0126686
71. Messaggio F, Mendonsa AM, Castellanos J, Nagathihalli NS, Gorden L, Merchant NB, et al. Adiponectin receptor agonists inhibit leptin induced Pstat3 and in vivo pancreatic tumor growth. Oncotarget (2017) 8(49):85378–91. doi: 10.18632/oncotarget.19905
72. Ragone A, Salzillo A, Spina A, Naviglio S, Sapio L. Integrating gemcitabine-based therapy with adiporon enhances growth inhibition in human pdac cell lines. Front Pharmacol (2022) 13:837503. doi: 10.3389/fphar.2022.837503
73. Zamorano AS, Hagemann AR, Morrison L, Lee JA, Liao LM, Brinton LA, et al. Pre-diagnosis body mass index, physical activity and ovarian cancer mortality. Gynecol Oncol (2019) 155(1):105–11. doi: 10.1016/j.ygyno.2019.07.025
74. Iyoshi S, Sumi A, Yoshihara M, Kitami K, Mogi K, Uno K, et al. Obesity contributes to the stealth peritoneal dissemination of ovarian cancer: A multi-institutional retrospective cohort study. Obes (Silver Spring) (2022) 30(8):1599–607. doi: 10.1002/oby.23497
75. Ignacio RMC, Lee ES, Wilson AJ, Beeghly-Fadiel A, Whalen MM, Son DS. Obesity-induced peritoneal dissemination of ovarian cancer and dominant recruitment of macrophages in ascites. Immune Netw (2018) 18(6):e47. doi: 10.4110/in.2018.18.e47
76. Kato S, Abarzua-Catalan L, Trigo C, Delpiano A, Sanhueza C, Garcia K, et al. Leptin stimulates migration and invasion and maintains cancer stem-like properties in ovarian cancer cells: An explanation for poor outcomes in obese women. Oncotarget (2015) 6(25):21100–19. doi: 10.18632/oncotarget.4228
77. Alsina-Sanchis E, Figueras A, Lahiguera A, Vidal A, Casanovas O, Graupera M, et al. The tgfbeta pathway stimulates ovarian cancer cell proliferation by increasing Igf1r levels. Int J Cancer (2016) 139(8):1894–903. doi: 10.1002/ijc.30233
78. Kokts-Porietis RL, Elmrayed S, Brenner DR, Friedenreich CM. Obesity and mortality among endometrial cancer survivors: A systematic review and meta-analysis. Obes Rev (2021) 22(12):e13337. doi: 10.1111/obr.13337
79. Merritt MA, Strickler HD, Einstein MH, Yang HP, Sherman ME, Wentzensen N, et al. Insulin/Igf and sex hormone axes in human endometrium and associations with endometrial cancer risk factors. Cancer Causes Control (2016) 27(6):737–48. doi: 10.1007/s10552-016-0751-4
80. Hewitt SC, Winuthayanon W, Lierz SL, Hamilton KJ, Donoghue LJ, Ramsey JT, et al. Role of eralpha in mediating female uterine transcriptional responses to Igf1. Endocrinology (2017) 158(8):2427–35. doi: 10.1210/en.2017-00349
81. Majchrzak-Baczmanska D, Malinowski A. Does igf-1 play a role in the biology of endometrial cancer? Ginekol Pol (2016) 87(8):598–604. doi: 10.5603/GP.2016.0052
82. Kitahara CM, Pfeiffer RM, Sosa JA, Shiels MS. Impact of overweight and obesity on us papillary thyroid cancer incidence trends (1995-2015). J Natl Cancer Inst (2020) 112(8):810–7. doi: 10.1093/jnci/djz202
83. Franchini F, Palatucci G, Colao A, Ungaro P, Macchia PE, Nettore IC. Obesity and thyroid cancer risk: An update. Int J Environ Res Public Health (2022) 19(3):1116. doi: 10.3390/ijerph19031116
84. Qian F, Huo D. Circulating insulin-like growth factor-1 and risk of total and 19 site-specific cancers: Cohort study analyses from the uk biobank. Cancer Epidemiol Biomarkers Prev (2020) 29(11):2332–42. doi: 10.1158/1055-9965.EPI-20-0743
85. Yang L, Tan Z, Li Y, Zhang X, Wu Y, Xu B, et al. Insulin-like growth factor 1 promotes proliferation and invasion of papillary thyroid cancer through the Stat3 pathway. J Clin Lab Anal (2020) 34(12):e23531. doi: 10.1002/jcla.23531
86. Manzella L, Massimino M, Stella S, Tirro E, Pennisi MS, Martorana F, et al. Activation of the igf axis in thyroid cancer: Implications for tumorigenesis and treatment. Int J Mol Sci (2019) 20(13):3258. doi: 10.3390/ijms20133258
87. Zhang T, Shen H, Dong W, Qu X, Liu Q, Du J. Antitumor effects and molecular mechanisms of figitumumab, a humanized monoclonal antibody to igf-1 receptor, in esophageal carcinoma. Sci Rep (2014) 4:6855. doi: 10.1038/srep06855
88. Calzone FJ, Cajulis E, Chung YA, Tsai MM, Mitchell P, Lu J, et al. Epitope-specific mechanisms of Igf1r inhibition by ganitumab. PloS One (2013) 8(2):e55135. doi: 10.1371/journal.pone.0055135
89. Fuentes-Baile M, Ventero MP, Encinar JA, Garcia-Morales P, Poveda-Deltell M, Perez-Valenciano E, et al. Differential effects of igf-1r small molecule tyrosine kinase inhibitors bms-754807 and osi-906 on human cancer cell lines. Cancers (Basel) (2020) 12(12):3717. doi: 10.3390/cancers12123717
90. Girnita A, Girnita L, del Prete F, Bartolazzi A, Larsson O, Axelson M. Cyclolignans as inhibitors of the insulin-like growth factor-1 receptor and malignant cell growth. Cancer Res (2004) 64(1):236–42. doi: 10.1158/0008-5472.can-03-2522
91. Friedbichler K, Hofmann MH, Kroez M, Ostermann E, Lamche HR, Koessl C, et al. Pharmacodynamic and antineoplastic activity of bi 836845, a fully human igf ligand-neutralizing antibody, and mechanistic rationale for combination with rapamycin. Mol Cancer Ther (2014) 13(2):399–409. doi: 10.1158/1535-7163.MCT-13-0598
92. Gradishar WJ, Yardley DA, Layman R, Sparano JA, Chuang E, Northfelt DW, et al. Clinical and translational results of a phase ii, randomized trial of an anti-Igf-1r (Cixutumumab) in women with breast cancer that progressed on endocrine therapy. Clin Cancer Res (2016) 22(2):301–9. doi: 10.1158/1078-0432.CCR-15-0588
93. Abou-Alfa GK, Capanu M, O'Reilly EM, Ma J, Chou JF, Gansukh B, et al. A phase ii study of cixutumumab (Imc-A12, Nsc742460) in advanced hepatocellular carcinoma. J Hepatol (2014) 60(2):319–24. doi: 10.1016/j.jhep.2013.09.008
94. Weigel B, Malempati S, Reid JM, Voss SD, Cho SY, Chen HX, et al. Phase 2 trial of cixutumumab in children, adolescents, and young adults with refractory solid tumors: A report from the children's oncology group. Pediatr Blood Cancer (2014) 61(3):452–6. doi: 10.1002/pbc.24605
95. Rajan A, Carter CA, Berman A, Cao L, Kelly RJ, Thomas A, et al. Cixutumumab for patients with recurrent or refractory advanced thymic epithelial tumours: A multicentre, open-label, phase 2 trial. Lancet Oncol (2014) 15(2):191–200. doi: 10.1016/s1470-2045(13)70596-5
96. Schoffski P, Adkins D, Blay JY, Gil T, Elias AD, Rutkowski P, et al. An open-label, phase 2 study evaluating the efficacy and safety of the anti-Igf-1r antibody cixutumumab in patients with previously treated advanced or metastatic soft-tissue sarcoma or Ewing family of tumours. Eur J Cancer (2013) 49(15):3219–28. doi: 10.1016/j.ejca.2013.06.010
97. Juergens H, Daw NC, Geoerger B, Ferrari S, Villarroel M, Aerts I, et al. Preliminary efficacy of the anti-Insulin-Like growth factor type 1 receptor antibody figitumumab in patients with refractory Ewing sarcoma. J Clin Oncol (2011) 29(34):4534–40. doi: 10.1200/JCO.2010.33.0670
98. Reidy-Lagunes DL, Vakiani E, Segal MF, Hollywood EM, Tang LH, Solit DB, et al. A phase 2 study of the insulin-like growth factor-1 receptor inhibitor mk-0646 in patients with metastatic, well-differentiated neuroendocrine tumors. Cancer (2012) 118(19):4795–800. doi: 10.1002/cncr.27459
99. Bergqvist M, Holgersson G, Bondarenko I, Grechanaya E, Maximovich A, Andor G, et al. Phase ii randomized study of the igf-1r pathway modulator Axl1717 compared to docetaxel in patients with previously treated, locally advanced or metastatic non-small cell lung cancer. Acta Oncol (2017) 56(3):441–7. doi: 10.1080/0284186X.2016.1253866
100. Fassnacht M, Berruti A, Baudin E, Demeure MJ, Gilbert J, Haak H, et al. Linsitinib (Osi-906) versus placebo for patients with locally advanced or metastatic adrenocortical carcinoma: A double-blind, randomised, phase 3 study. Lancet Oncol (2015) 16(4):426–35. doi: 10.1016/s1470-2045(15)70081-1
101. Barata P, Cooney M, Tyler A, Wright J, Dreicer R, Garcia JA. A phase 2 study of osi-906 (Linsitinib, an insulin-like growth factor receptor-1 inhibitor) in patients with asymptomatic or mildly symptomatic (Non-opioid requiring) metastatic castrate resistant prostate cancer (Crpc). Invest New Drugs (2018) 36(3):451–7. doi: 10.1007/s10637-018-0574-0
102. de Bono J, Lin CC, Chen LT, Corral J, Michalarea V, Rihawi K, et al. Two first-in-Human studies of xentuzumab, a humanised insulin-like growth factor (Igf)-neutralising antibody, in patients with advanced solid tumours. Br J Cancer (2020) 122(9):1324–32. doi: 10.1038/s41416-020-0774-1
103. Doi T, Kuboki Y, Naito Y, Ishida M, Tanaka T, Takeuchi Y. A phase 1 trial of xentuzumab, an igf-neutralizing antibody, in Japanese patients with advanced solid tumors. Cancer Sci (2022) 113(3):1010–7. doi: 10.1111/cas.15231
104. Tsui J, Qi S, Perrino S, Leibovitch M, Brodt P. Identification of a resistance mechanism to igf-ir targeting in human triple negative mda-Mb-231 breast cancer cells. Biomolecules (2021) 11(4):527. doi: 10.3390/biom11040527
105. Iida M, Tsuboi K, Niwa T, Ishida T, Hayashi SI. Compensatory role of insulin-like growth factor 1 receptor in estrogen receptor signaling pathway and possible therapeutic target for hormone therapy-resistant breast cancer. Breast Cancer (2019) 26(3):272–81. doi: 10.1007/s12282-018-0922-0
106. Ma G, Tan C, Shan Y, Shao N, Wang F, Dimitrov DS, et al. An insulin growth factor-I/Ii-Neutralizing monoclonal antibody in combination with epidermal growth factor receptor inhibitors potently inhibits tumor cell growth. J Cancer (2022) 13(6):1830–6. doi: 10.7150/jca.69064
107. Kruger DT, Alexi X, Opdam M, Schuurman K, Voorwerk L, Sanders J, et al. Igf-1r pathway activation as putative biomarker for linsitinib therapy to revert tamoxifen resistance in er-positive breast cancer. Int J Cancer (2020) 146(8):2348–59. doi: 10.1002/ijc.32668
108. De Martino MC, van Koetsveld PM, Feelders RA, de Herder WW, Dogan F, Janssen J, et al. Igf and mtor pathway expression and in vitro effects of linsitinib and mtor inhibitors in adrenocortical cancer. Endocrine (2019) 64(3):673–84. doi: 10.1007/s12020-019-01869-1
109. Di Cosimo S, Sathyanarayanan S, Bendell JC, Cervantes A, Stein MN, Brana I, et al. Combination of the mtor inhibitor ridaforolimus and the anti-Igf1r monoclonal antibody dalotuzumab: Preclinical characterization and phase I clinical trial. Clin Cancer Res (2015) 21(1):49–59. doi: 10.1158/1078-0432.CCR-14-0940
110. Ireland L, Santos A, Campbell F, Figueiredo C, Hammond D, Ellies LG, et al. Blockade of insulin-like growth factors increases efficacy of paclitaxel in metastatic breast cancer. Oncogene (2018) 37(15):2022–36. doi: 10.1038/s41388-017-0115-x
111. Chen L, Zhu Z, Gao W, Jiang Q, Yu J, Fu C. Systemic analysis of different colorectal cancer cell lines and tcga datasets identified igf-1r/Egfr-Ppar-Caspase axis as important indicator for radiotherapy sensitivity. Gene (2017) 627:484–90. doi: 10.1016/j.gene.2017.07.003
Keywords: obesity, endocrine-related cancer, insulin-like growth factor-1(IGF-1R), insulin like growth factor-1 receptor (IGF-1R), mechanism
Citation: Zhong W, Wang X, Wang Y, Sun G, Zhang J and Li Z (2023) Obesity and endocrine-related cancer: The important role of IGF-1. Front. Endocrinol. 14:1093257. doi: 10.3389/fendo.2023.1093257
Received: 08 November 2022; Accepted: 04 January 2023;
Published: 23 January 2023.
Edited by:
Michaela Ruth Reagan, MaineHealth, United StatesReviewed by:
Paola Maroni, Ospedale Galeazzi S.p.A, ItalySilvio Naviglio, University of Campania Luigi Vanvitelli, Italy
Copyright © 2023 Zhong, Wang, Wang, Sun, Zhang and Li. This is an open-access article distributed under the terms of the Creative Commons Attribution License (CC BY). The use, distribution or reproduction in other forums is permitted, provided the original author(s) and the copyright owner(s) are credited and that the original publication in this journal is cited, in accordance with accepted academic practice. No use, distribution or reproduction is permitted which does not comply with these terms.
*Correspondence: Zhuo Li, zhuoli@jlu.edu.cn