- 1Faculdade Israelita de Ciências da Saúde Albert Einstein, Hospital Israelita Albert Einstein, São Paulo, Brazil
- 2Laboratório de Endocrinologia Molecular e Translacional (LEMT), Escola Paulista de Medicina (EPM), Universidade Federal de São Paulo (UNIFESP), São Paulo, Brazil
- 3Instituto de Ciências Ambientais, Químicas e Farmacêuticas (ICAQF), Departamento de Ciências Biológicas, Universidade Federal de São Paulo (UNIFESP), Diadema, Brazil
Introduction: DEHP is an endocrine disruptor widely used in the production of malleable plastics. DEHP exposure was associated with altered hypothalamic-pituitary-thyroid (HPT) axis function. Although previous studies reported deleterious effects of DEHP exposure during the intrauterine period, few studies have evaluated the direct effects triggered by this endocrine disruptor on the offspring animals' thyroid function. This study aimed to investigate the impact of intrauterine exposure to DEHP on the HPT axis function programming of the offspring animals during adulthood.
Methods: Pregnant Wistar rats were orally treated with corn oil or corn oil supplemented with DEHP (0.48 or 4.8 mg/kg/day) throughout the gestational period. The offspring rats were euthanized on the 90th postnatal day. Hypothalamus, pituitary, thyroid, and liver were collected to analyze gene expression and protein content through qPCR and Western Blot. Blood was collected to determine TSH and thyroid hormone levels through fluorometric or chemiluminescence immunoassays.
Results: In the adult F1 female rats, the highest dose of DEHP decreased TSH serum levels. In the thyroid, DEHP reduced the gene expression and/or protein content of NIS, TSHR, TG, TPO, MCT8, NKX2.1, PAX8, and FOXE1. These data are consistent with the reduction in T4 serum levels of the F1 DEHP-exposed female rats. In the liver, DEHP exposure increased the mRNA expression of Dio1 and Ttr, while the highest dose of DEHP reduced the mRNA expression of Ugt1a1 and Ugt1a6. Conversely, in the F1 male adult rats, TSHB expression and TSH serum levels were increased in DEHP-exposed animals. In the thyroid, except for the reduced protein content of TSHR, none of the evaluated genes/proteins were altered by DEHP. TH serum levels were not changed in the DEHP-exposed F1 male rats compared to the control group. Additionally, there were no significant alterations in the expression of hepatic enzymes in these animals.
Discussion/Conclusions: Our results demonstrated, for the first time, that intrauterine exposure to DEHP disrupts the HPT axis function in male and female offspring rats and strongly suggest that DEHP exposure increases the susceptibility of the offspring animals to develop thyroid dysfunctions during adulthood.
1 Introduction
Phthalates are plasticizers used in producing polyvinyl chloride plastics and several manufactured goods, such as toys, food packaging, and medical devices. Phthalates are not covalently bound as plasticizes, and thus can easily leach into the water, food, and environment (1, 2).
Di(2-ethylhexyl) phthalate (DEHP) is the most common member of the class of phthalates, and several studies indicated that humans are highly exposed to DEHP (3, 4). Indeed, DEHP exposure was considered a potential risk to human health (5–7). The estimated daily intake of DEHP for adult humans was 0.5-30 μg/kg/day, although some studies have reported higher levels of exposure to this phthalate (8–10). Once in the body, DEHP is metabolized to mono(2-ethylhexyl) phthalate (MEHP), a highly toxic compound (11).
DEHP and MEHP are compounds that interfere with the endocrine function in humans and other animals (12). It is worth noting that the thyroid hormones control metabolic processes essential for normal development and growth (13–15). Moreover, the thyroid hormones are transferred through the placenta to the fetal compartment during the intrauterine period, and the thyroid hormones’ actions are crucial to the development of the central nervous system (16–18).
Although it has been suggested in several epidemiological studies, the negative association between thyroid hormone serum levels and DEHP exposure is still controversial in humans (19–22). On the contrary, the disruptive effect of DEHP exposure on the thyroid function of the most susceptible individuals, such as newborns, children, and pregnant women, was extensively reported in the literature (23–25).
In animal models, the direct and chronic exposure to high doses of DEHP (250 to 750 mg/kg/day) was related to increased serum levels of TRH and TSH, decreased thyroid hormones serum concentration, altered expression of genes/proteins involved in the thyroid hormone synthesis, altered peripheral metabolism and impaired plasma transport of thyroid hormones to the target tissues (26–28).
It is well known that the intrauterine period is essential for programming health or disease during adulthood (29–31). Nevertheless, although it has been described that in-utero exposure to DEHP was related to neurobehavioral and neurodevelopment impairments due to alterations in thyroid homeostasis (32, 33), limited studies investigated the effects of DEHP early-life exposure directly in the thyroid function of the offspring animals. Therefore, this study aimed to evaluate the impact of intrauterine exposure to DEHP in the programming of the HPT axis of the adult F1 rat offspring.
2 Material and methods
2.1 Animals and experimental protocol
Virgin female and male Wistar rats were obtained from the Animal Breeding Centre at the Institute of Biomedical Sciences, University of Sao Paulo. The animals were maintained in polysulfone rat cages in the Experimental and Training Center of the Hospital Israelita Albert Einstein at constant temperature (23 ± 1°C), 12:12-h light-dark cycle schedule, and fed with rat chow (NUVLAB, CR1; Nuvital, Brazil) and water (polysulfone bottles) ad libitum. The female and male rats were mated, and the presence of spermatozoa in the vaginal smear was defined as the first day of gestation. Thereafter, each pregnant rat was randomly assigned to one of 3 treatment groups: control, 0.48 mg/kg/day (DEHP 0.48), or 4.8 mg/kg/day (DEHP 4.8). Four pregnant rats were used in each experimental group. Each pregnant rat had one litter of 10-12 animals per group. The number of offspring animals did not vary among the groups. DEHP was dissolved in corn oil (Figure 1). The treatment doses were based on the NOAEL dose of DEHP, which was defined as 4.8 mg/kg/day (34, 35).
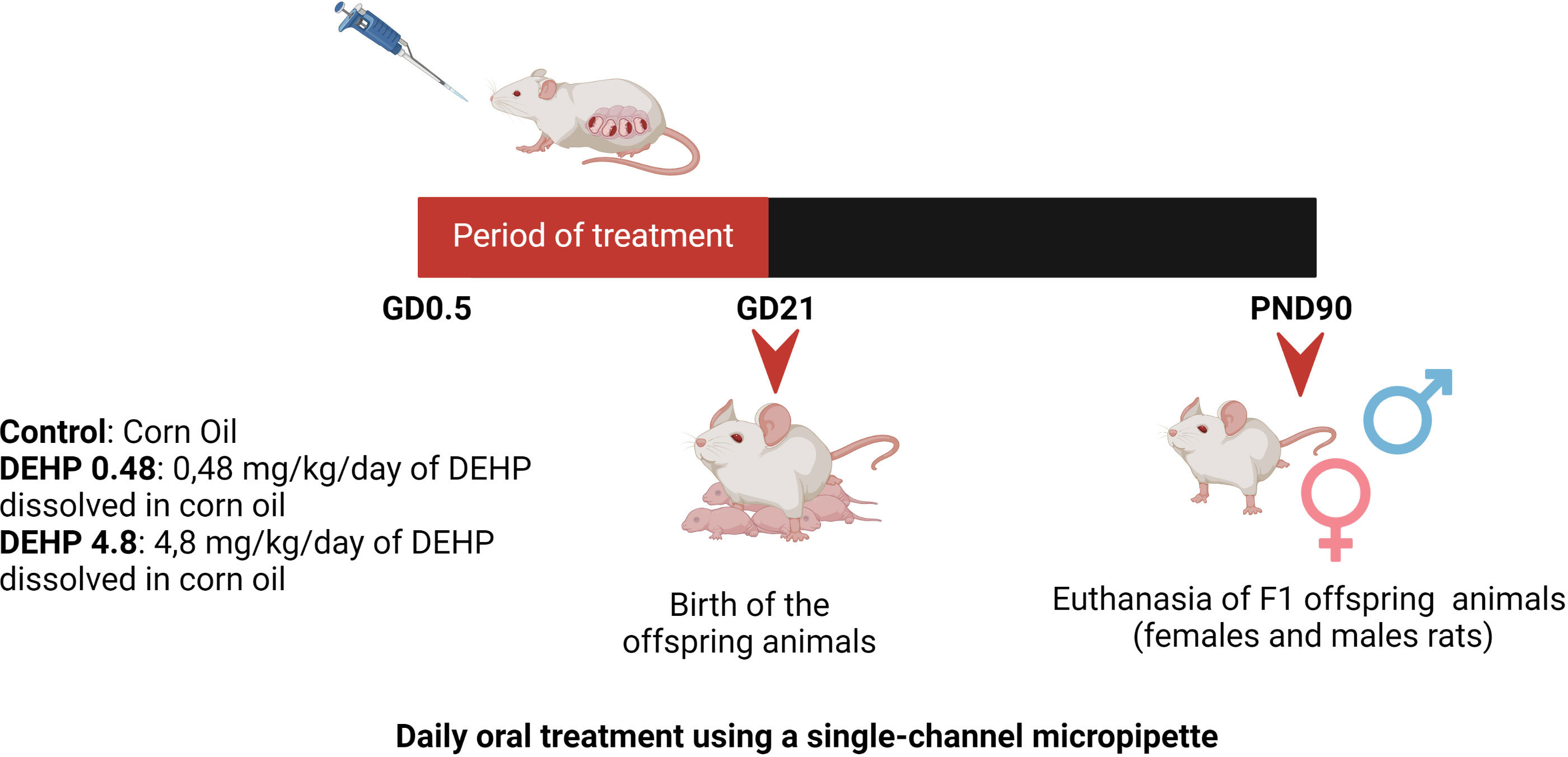
Figure 1 Schematic representation of the experimental protocol. Pregnant Wistar rats were randomly divided into 3 experimental groups: Control, DEHP 0.48 mg/kg/day and DEHP 4.8 mg/kg/day. Pregnant rats orally received corn oil (control) or corn oil supplemented with DEHP (0.48 or 4.8 mg/kg/day) throughout pregnancy (GD0.5 – GD21). After birth, F1 offspring male and female rats were maintained with normal rat chow and filtered water. The offspring animals were anesthetized and euthanized in the PND90. Created with BioRender.com.
From the first day of gestation until the birth of the pups, pregnant dams were orally dosed once a day with corn oil (vehicle control) or the doses mentioned above of DEHP by placing a pipette tip containing the dosing solution into the mouth (Figure 1). This method of drug administration was chosen to mimic oral exposure in humans (35). The volume of each dosing mixture in corn oil was adjusted daily based on changes in the dam’s weight.
The DEHP treatment was interrupted after the birth of the offspring rats. After weaning, the rats were separated by sex and kept on standard chow and filtered water until the 90th postnatal day (PND90). Then, the animals were weighed, anesthetized, and euthanized by decapitation. The euthanasia was performed in the morning between 8h00 and 11h00AM. The female offspring were in the metestrus and diestrus phases of the estrous cycle on the day of the euthanasia. Blood, hypothalamus, pituitary, thyroid, and liver were collected, stored at -80°C, and processed as described below.
The experimental protocol was approved by the Ethics Committee on Animal Experimentation of the Hospital Israelita Albert Einstein (CEUA 3326/2018), following the ethical principles in animal research adopted by the National Council for the Control of Animal Experimentation.
2.2 Evaluation of gene expression
Hypothalamus, pituitary, thyroid, and liver were homogenized in TRIzol® Reagent (Invitrogen Life Technologies Carlsbad, CA, USA), using Polytron® equipment (KINEMATIC), following the manufacturer’s recommendations (36). In addition, gene expression of the hypothalamus and pituitary (Tshb, Cga, Trh), thyroid transcription factors (Nkx2.1, Pax8, Foxe1), thyroid differentiation markers (Slc5a5, Tshr, Tpo, Tg, Mct8), deiodinases (Dio1, Dio3), hepatic enzymes (Ttr, Ugt1a1, Ugt1a6) was evaluated through RT-qPCR assays. The relative mRNA expression was calculated according to the 2−ΔΔCt method using Rpl19 mRNA expression as the internal control. Primer sequences are described in Supplementary Table 1.
2.3 Evaluation of protein content
Hypothalamus, pituitary, and thyroid were homogenized in RIPA lysis buffer (50 mM Tris, pH 7.5; 150 mM NaCl, 1% Nonidet P-40; 0.5% sodium deoxycholate; 1 mM EDTA and 0.1% SDS) supplemented with protease inhibitors (Protease Inhibitor Tablets, Thermo Scientific Pierce™), using Polytron® equipment (KINEMATIC). Western Blotting was performed as previously described (36). Briefly, nitrocellulose membranes were blocked with 3% BSA solution and incubated with specific primary and secondary antibodies, described in Supplementary Table 2. Blots were developed using the enhanced chemiluminescence (ECL) kit (Bio-Rad). Densitometric analyses were performed using Image J. 1.4 software (National Institutes of Health). Ponceau staining was used for thyroid total protein normalization, as previously described (37).
2.4 Determination of TSH, T3, and T4 serum levels
T4 and T3 rat serum concentrations were determined by a chemiluminescent immunoassay (Roche). TSH serum levels were determined by a fluorometric immunoassay, as previously described (37).
2.5 Statistical analysis
All data are reported as means ± SEM. The number of animals used in the study is indicated in the legends of the figures. Statistical analysis was performed using the GraphPad Prism Software – Version: 6.0. Data was subjected to a normality test (Kolmogorov-Smirnov) and then to unpaired One-Way ANOVA followed by Student-Newman-Keuls post hoc test and/or Dunnet's multiple comparisons test post hoc test. Differences were considered statistically significant at P < 0.05.
3 Results
3.1 Intrauterine exposure to DEHP alters TSH and thyroid hormones serum levels in the F1 female rats
As presented in Table 1, the exposure of F1 female rats in utero to DEHP did not alter body weight but altered the serum levels of the TSH and thyroid hormones during adulthood. Indeed, the female rats exposed to the highest dose of DEHP treatment during the intrauterine period presented lower serum TSH levels than the control group. Moreover, in-utero exposure to DEHP reduced T4 serum levels in the F1 female rats. Interestingly, there was a significant increase in the T3 total serum levels in the animals exposed to the lowest dose of DEHP during the intrauterine period.
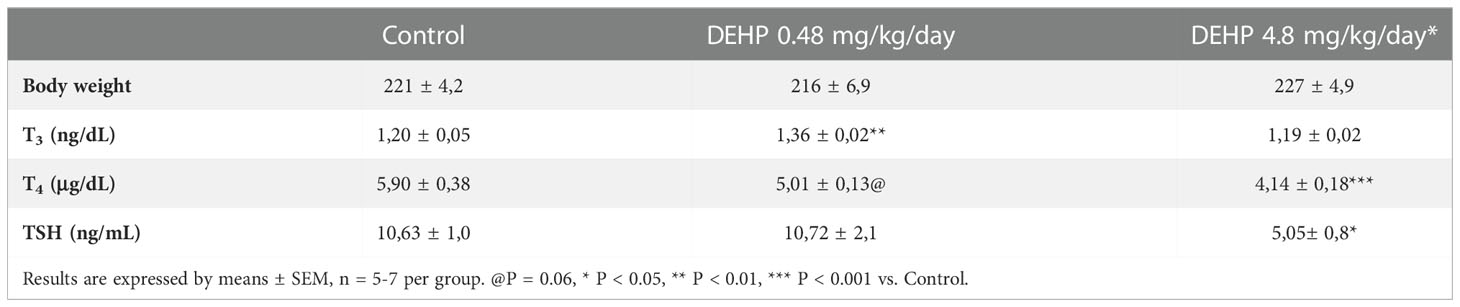
Table 1 Body weight, thyroid hormones and TSH serum levels in adult F1 female rats that were exposed or not to DEHP during intrauterine period.
3.2 Intrauterine exposure to DEHP alters the gene expression and protein content of the F1 female rats’ hypothalamus and pituitary
As demonstrated in Figure 2, the intrauterine exposure to DEHP has not altered TRH’s gene and protein expression in the hypothalamus of the F1 female rats during adulthood. Interestingly, exposure to the lowest dose of DEHP significantly increased the gene expression and protein content of the alpha and beta subunits of TSH in the pituitary of the F1 female rats. However, there were no significant alterations in these subunits’ gene/protein content in the animals exposed to the highest dose of DEHP (Figure 3).
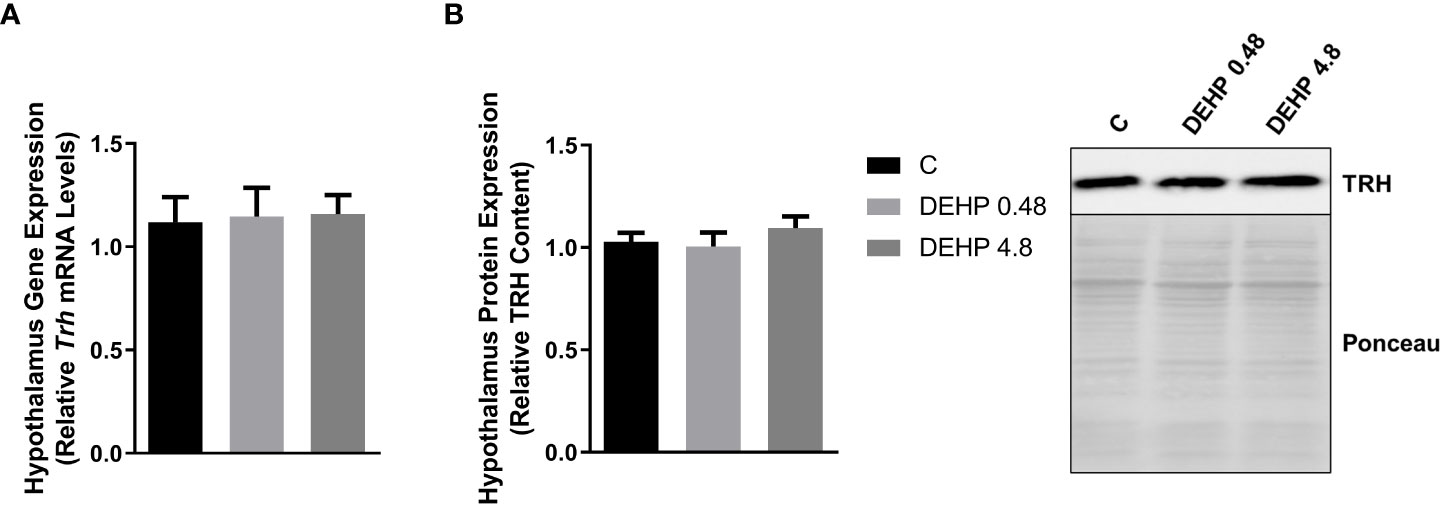
Figure 2 Impact of DEHP exposure during the intrauterine period on TRH expression in the hypothalamus of adult F1 female rats. (A) Gene expression (Trh) was evaluated by Real-Time PCR and normalized by the expression of the constitutive gene Rpl19. (B) The protein content of thyrotropin-releasing hormone (TRH) was evaluated by Western Blot and normalized by Western Blot and normalized by Ponceau staining of the membranes. Representative western blots are shown in the right panel. Results are expressed as means ± SEM as fold change or in arbitrary units (AU). Values are expressed as mean ± SEM, in arbitrary units, n = 8-9 animals. P > 0.05 (One-Way ANOVA).
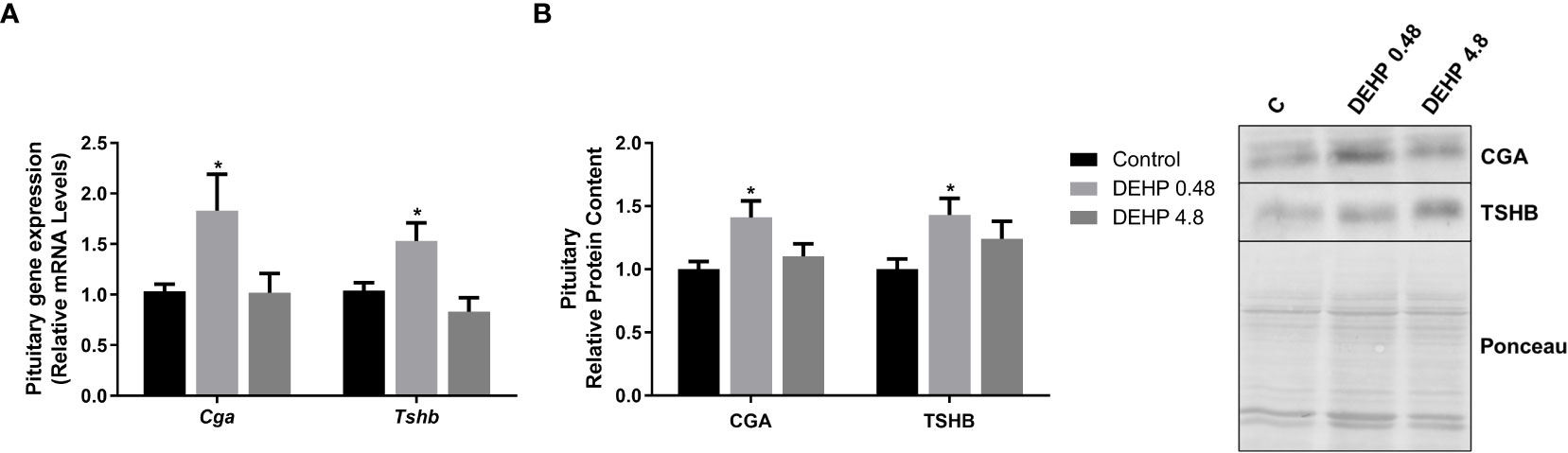
Figure 3 Impact of DEHP exposure during intrauterine period in the expression of alpha and beta subunits of TSH in the pituitary of adult F1 female rats. (A) Gene expression (Cga, Tshb) was evaluated by Real-Time PCR and normalized by the expression of the constitutive gene Rpl19. (B) The protein content of CGA and TSHB was assessed by Western Blot and normalized by Ponceau staining of the membranes. Representative western blots are shown in the right panel. Values are expressed as mean ± SEM in arbitrary units, n=10-14 animals. * p < 0.05 vs. Control (One-Way ANOVA).
3.3 Intrauterine exposure to DEHP reduces the gene expression and protein content of the F1 female rats’ thyroid gland
Intrauterine exposure to both doses of DEHP has also reduced the expression of genes/proteins involved in the synthesis and secretion of thyroid hormones (Figure 4). Indeed, there was a significant decrease in the gene expression of Foxe1 and Nkx2.1 transcription factors in both treatment doses, while the Pax8 gene expression was reduced only in the animals exposed to the highest dose of DEHP. In addition, both doses of DEHP exposure reduced the thyroid expression of Slc5a5, Tpo, Tshr, and Mct8 mRNAs compared to the control animals (Figure 4A). In agreement, the in-utero DEHP-exposed F1 female rats presented a significant reduction in the content of NIS, TPO, and TSHR, critical proteins involved in synthesizing thyroid hormones (Figure 4B).
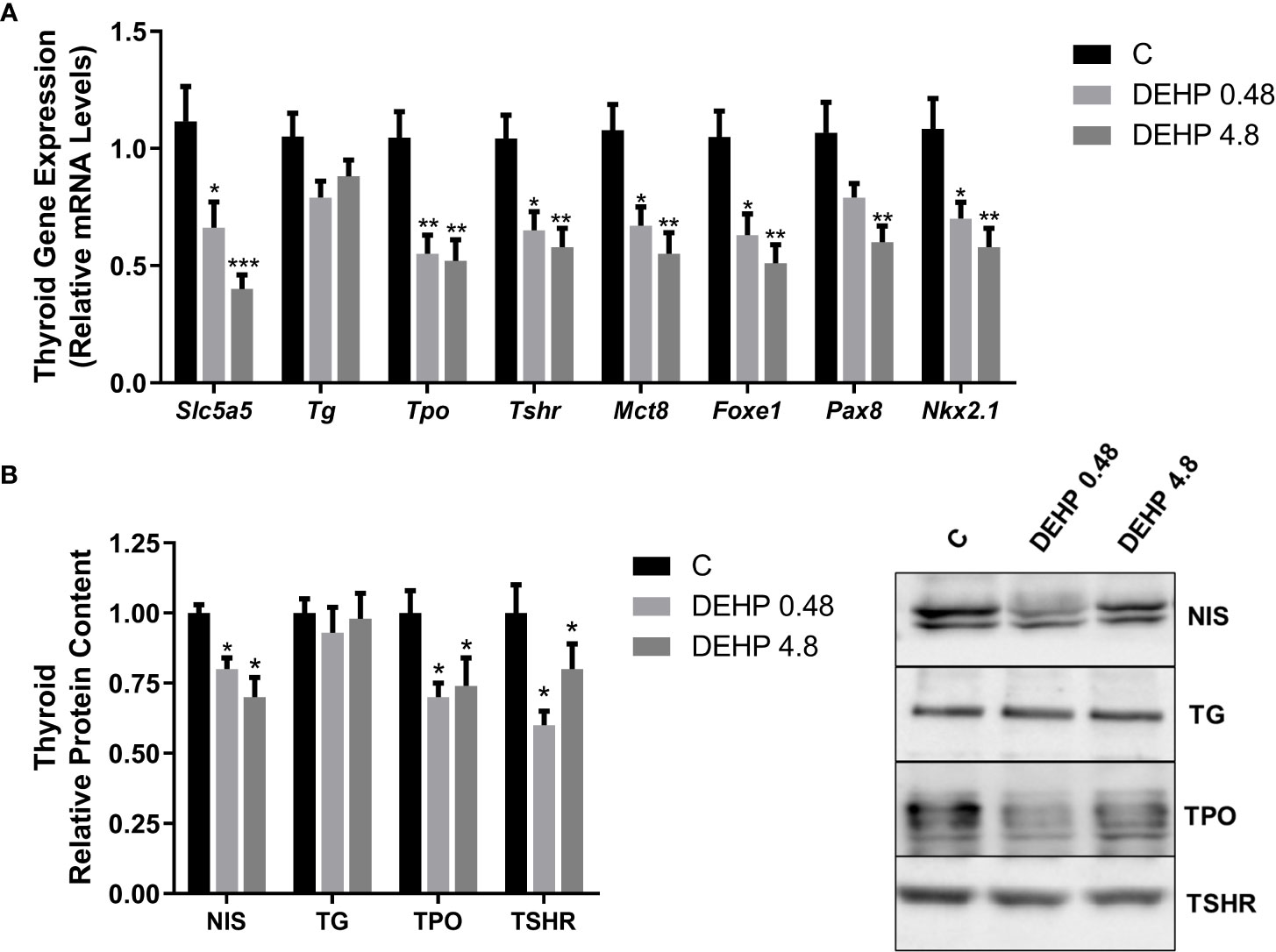
Figure 4 Impact of DEHP exposure during the intrauterine period on thyroid gene and protein expression in adult F1 female rats. (A) Gene expression (Slc5a5, Tg, Tpo, Tshr, Mct8, Foxe1, Pax8, Nkx2.1) was evaluated by Real-Time PCR and normalized by the expression of the constitutive gene Rpl19. (B) The protein content of NIS, TG, TPO, and TSHR was evaluated by Western Blot and normalized by Ponceau staining of the membranes (Supplementary Figure 1). Representative western blots are shown in the right panel. Values are expressed as mean ± SEM, in arbitrary units, n=8-10. * p < 0.05, ** p < 0.01, *** p < 0.001 vs Control. (One-Way ANOVA).
3.4 Intrauterine exposure to DEHP alters the gene expression of the F1 female rats’ liver
Finally, the gene expression of type I deiodinase (Dio1) was significantly increased in the liver of female rats exposed to both doses of DEHP. There was no significant alteration in the expression of type 3 deiodinase (Dio3). Moreover, intrauterine exposure to the highest dose of DEHP reduced the mRNA expression of Ugt1a and Ugt1a6, glucuronosyltransferases involved in the depuration of thyroid hormones. The mRNA expression of the transthyretin (Ttr), an essential plasma protein transporter of thyroid hormones, was increased in the liver of DEHP-exposed adult F1 female rats (Figure 5).
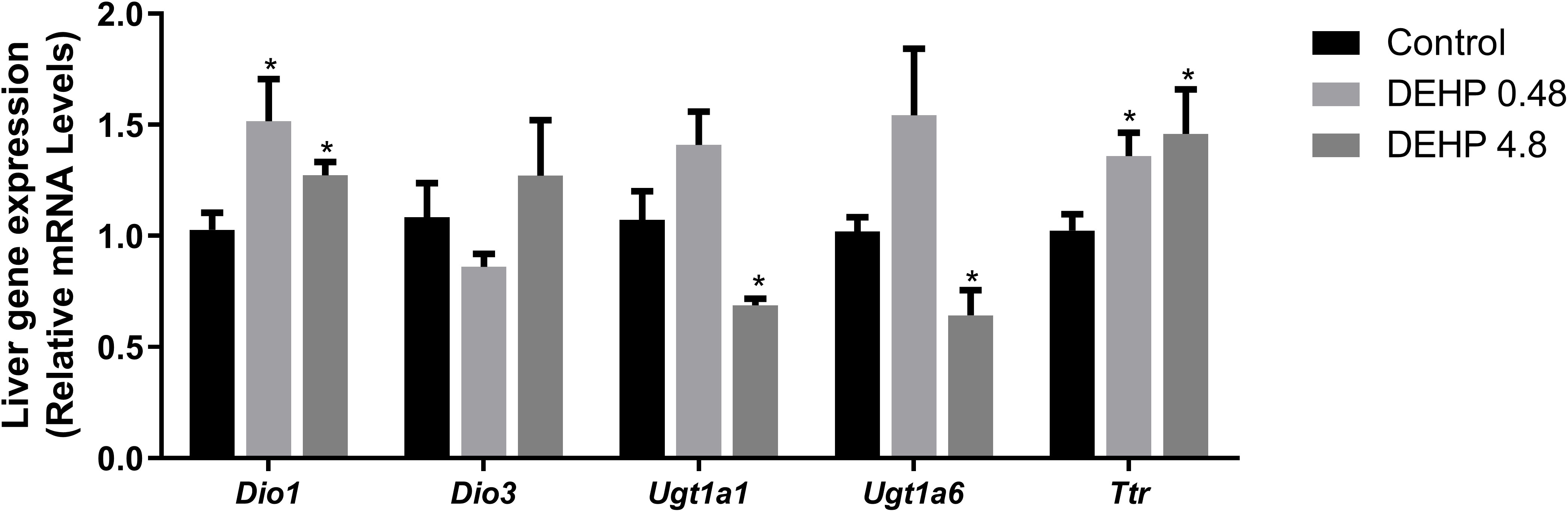
Figure 5 Impact of DEHP exposure during intrauterine period in the liver gene expression in adult F1 female rats. Gene expression (Dio1, Dio3, Ugt1a, Ugt1a6, Ttr) was evaluated by Real-Time PCR and was normalized by the expression of the constitutive gene Rpl19. Values are expressed as mean ± SEM in arbitrary units, n=7-10 animals. * p < 0.05, vs. Control (One-Way ANOVA).
3.5 Intrauterine exposure to DEHP alters TSH serum levels but doesn’t change thyroid hormones serum levels in the F1 male rats
In contrast to the results obtained in the F1 female rats, adult F1 male rats have not presented alterations in the thyroid hormones serum levels. However, both studied doses significantly elevated the TSH serum levels in the DEHP-exposed animals. Notably, the offspring’s body weight did not vary in the DEHP-exposed animals (Table 2).
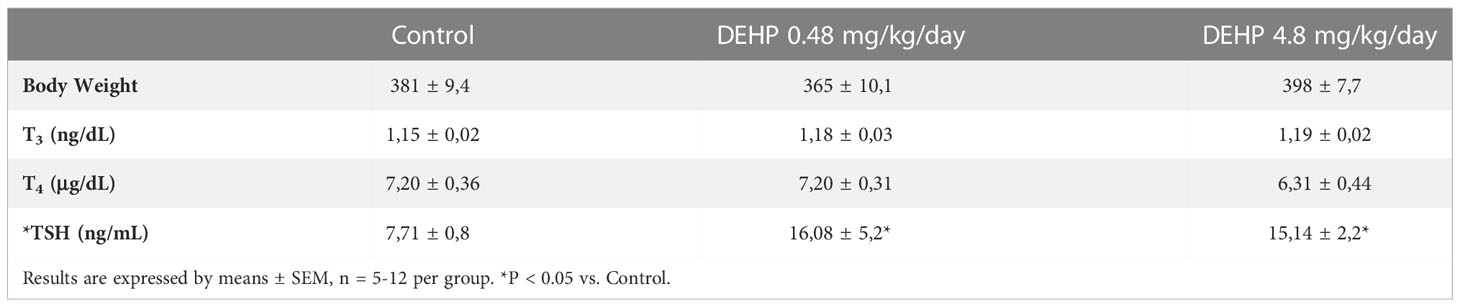
Table 2 Body weight, thyroid hormones and TSH serum levels in adult F1 male rats that were exposed or not to DEHP during intrauterine period.
3.6 Intrauterine exposure to DEHP alters the gene expression and protein content of the F1 male rats’ hypothalamus and pituitary
Interestingly, the in-utero exposure to the lowest dose of DEHP increased the mRNA expression of Trh in the hypothalamus of adult F1 male rats, even though there were no significant alterations in the TRH protein content in these animals (Figure 6). Furthermore, as demonstrated in Figure 7, DEHP exposure has not altered the alpha subunit of TSH expression in the pituitary of the F1 male rats. In contrast, the animals exposed to the lowest dose of DEHP during the intrauterine period presented increased mRNA expression and protein content of the beta subunit of TSH in the pituitary.
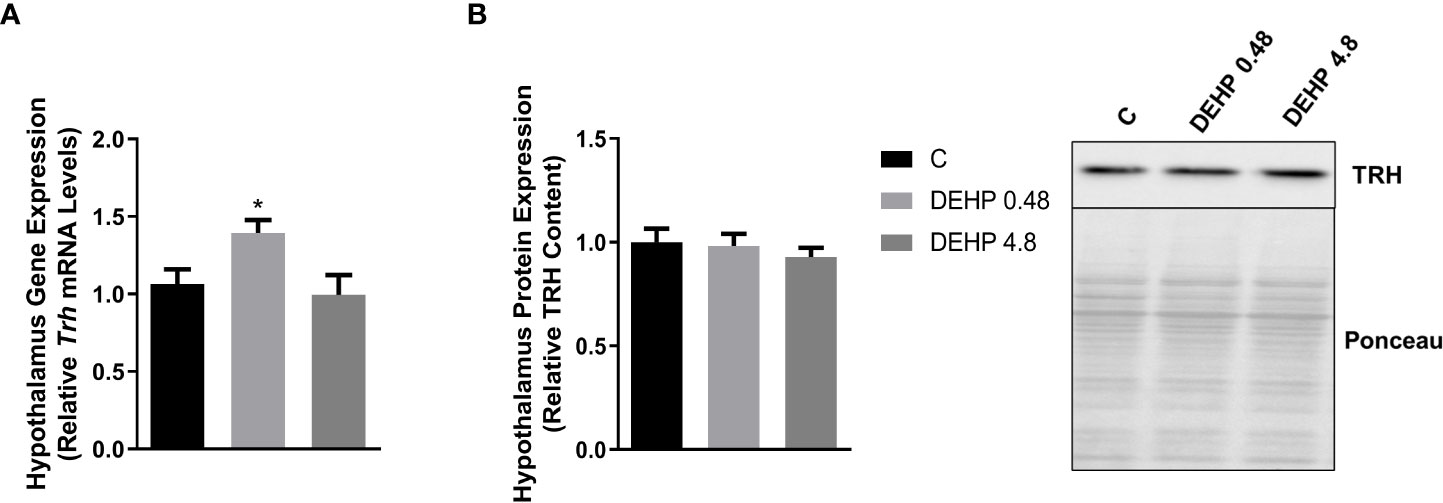
Figure 6 Impact of DEHP exposure during intrauterine period in the TRH expression in the hypothalamus of adult F1 male rats. (A) Gene expression (Trh) was evaluated by Real-Time PCR and normalized by the expression of the constitutive gene Rpl19. (B) The protein content of thyrotropin-releasing hormone (TRH) was evaluated by Western Blot and normalized by Ponceau staining of the membranes. Representative western blots are shown in the right panel. Values are expressed as mean ± SEM, in arbitrary units, n = 8-10 animals. * p < 0.05 vs. Control (One-Way ANOVA).
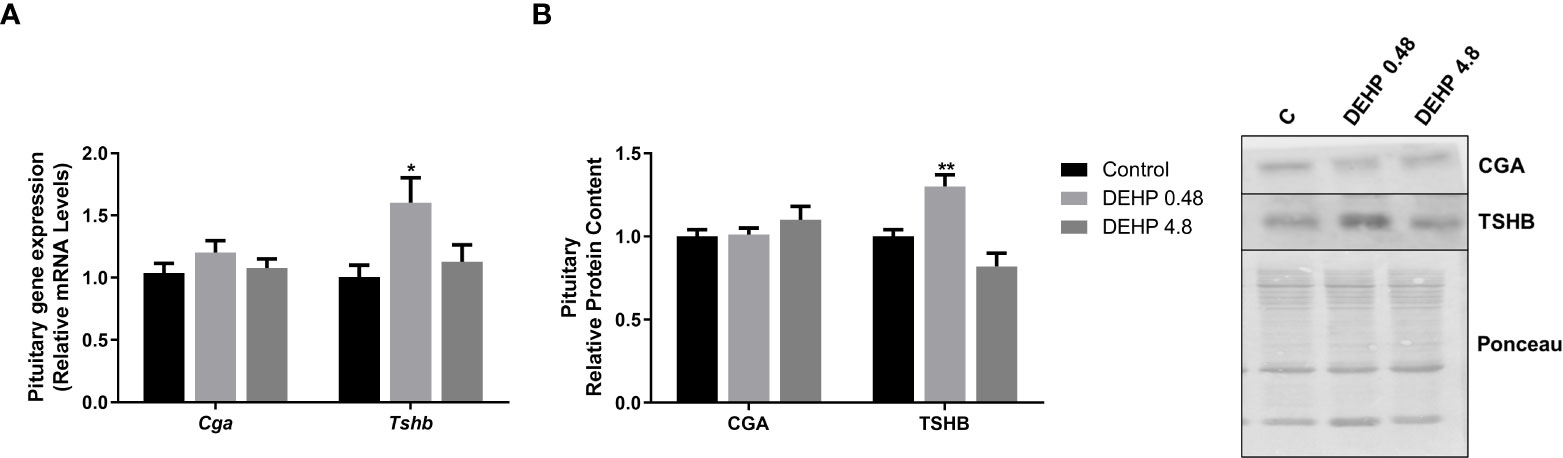
Figure 7 Impact of DEHP exposure during intrauterine period in the expression of alpha and beta subunits of TSH in the pituitary of adult F1 male rats. (A) Gene expression (Cga, Tshb) was evaluated by Real-Time PCR and normalized by the expression of the constitutive gene Rpl19. (B) The protein content of CGA and TSHB was evaluated by Western Blot and normalized by Ponceau staining of the membranes.. Representative western blots are shown in the right panel. Values are expressed as mean ± SEM, in arbitrary units, n= 13-14 animals. * p < 0.05, ** p < 0.01 vs. Control (One-Way ANOVA).
3.7 Intrauterine exposure to DEHP affects the gene expression and protein content of the F1 male rats’ thyroid gland
Interestingly, the exposure of F1 male rats to DEHP in utero has not significantly altered the mRNA expression of Slc5a5, Tg, Tpo, Tshr, Mct8, Foxe1, Pax8, and Nkx2.1 (Figure 8A). However, DEHP-exposed animals presented a significant decrease in the TSRH protein content in the thyroid (Figure 8B).
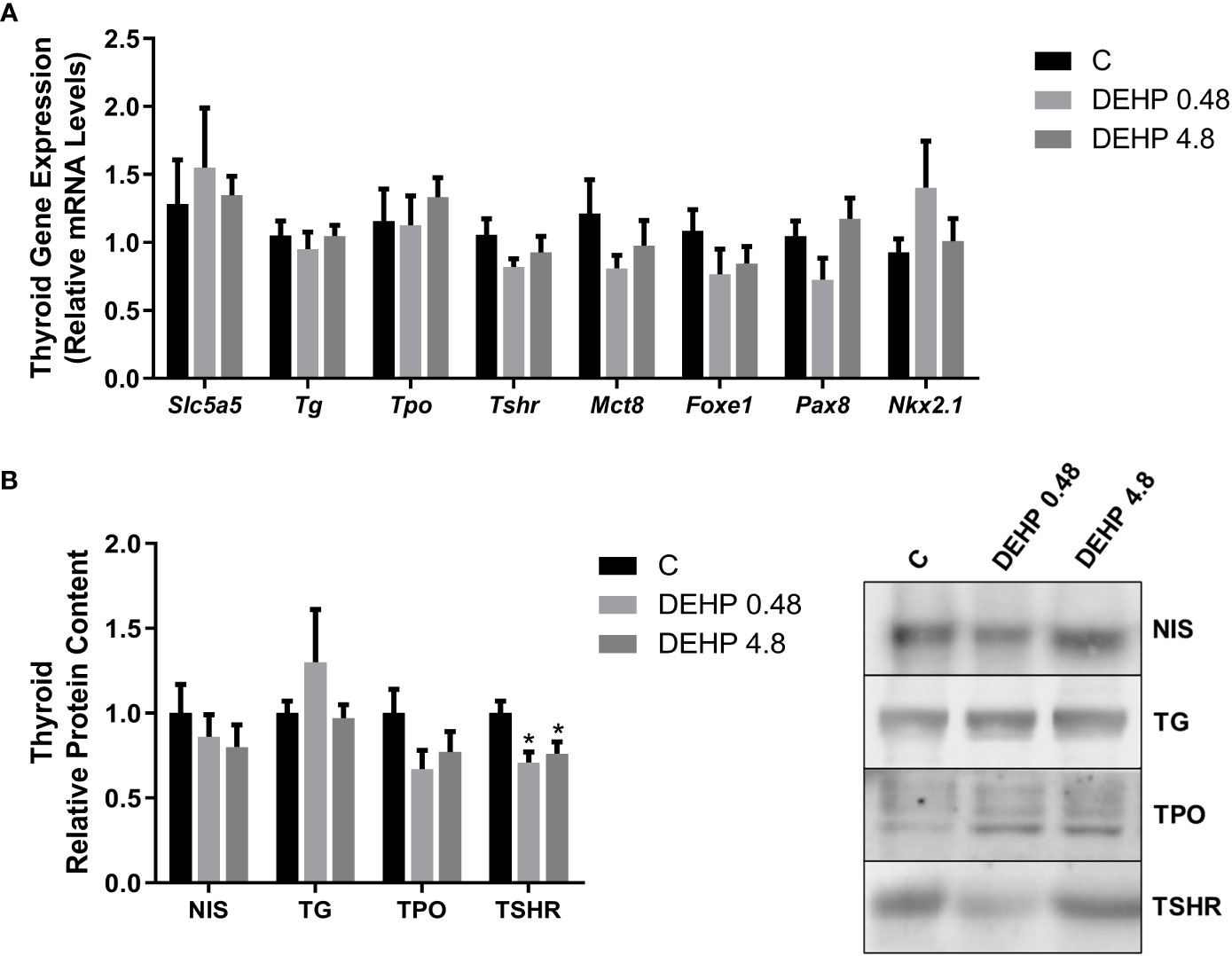
Figure 8 Impact of DEHP exposure during the intrauterine period on thyroid gene and protein expression in the adult F1 male rats. (A) Gene expression (Slc5a5, Tg, Tpo, Tshr, Mct8, Foxe1, Pax8, Nkx2.1) was evaluated by Real-Time PCR and normalized by the expression of the constitutive gene Rpl19. (B) The protein content of NIS, NIS, TG, TPO, and TSHR was evaluated by Western Blot and normalized by Ponceau staining of the membranes (Supplementary Figure 2). Representative western blots are shown in the right panel. Values are expressed as mean ± SEM, in arbitrary units, n=8-10. * p < 0.05 vs. Control. (One-Way ANOVA).
3.8 Intrauterine exposure to DEHP doesn’t alter the gene expression of the F1 male rats’ liver
The mRNA expression of Dio1, Dio3, Ugt1a, Ugt1a6, and Ttr were not altered in the liver of adult F1 DEHP-exposed male rats compared to the control group (Figure 9).
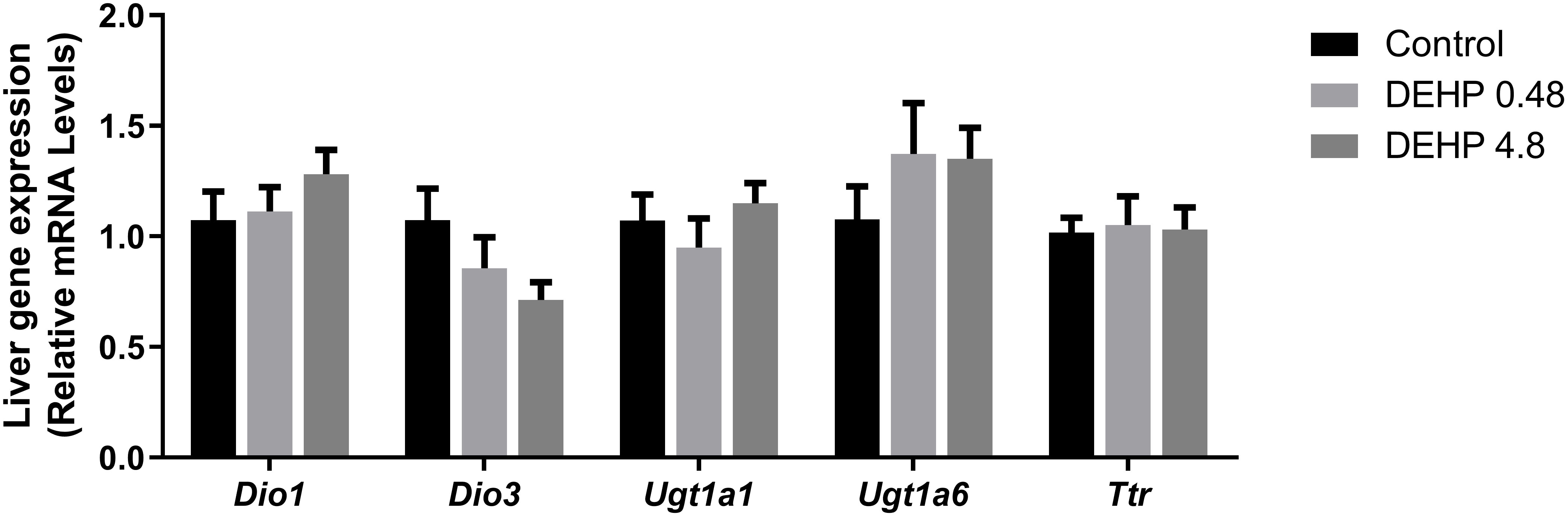
Figure 9 Impact of DEHP exposure during intrauterine period in the liver gene expression in adult F1 male rats. Gene expression (Dio1, Dio3, Ugt1a, Ugt1a6, Ttr) was evaluated by Real-Time PCR and was normalized by the expression of the constitutive gene Rpl19. Values are expressed as mean ± SEM in arbitrary units, n=7-10 animals. p > 0.05 (One-Way ANOVA).
In summary, Figure 10 presents a schematic representation of the deleterious effects of intrauterine exposure to DEHP in the HPT axis function of F1 offspring during adulthood.
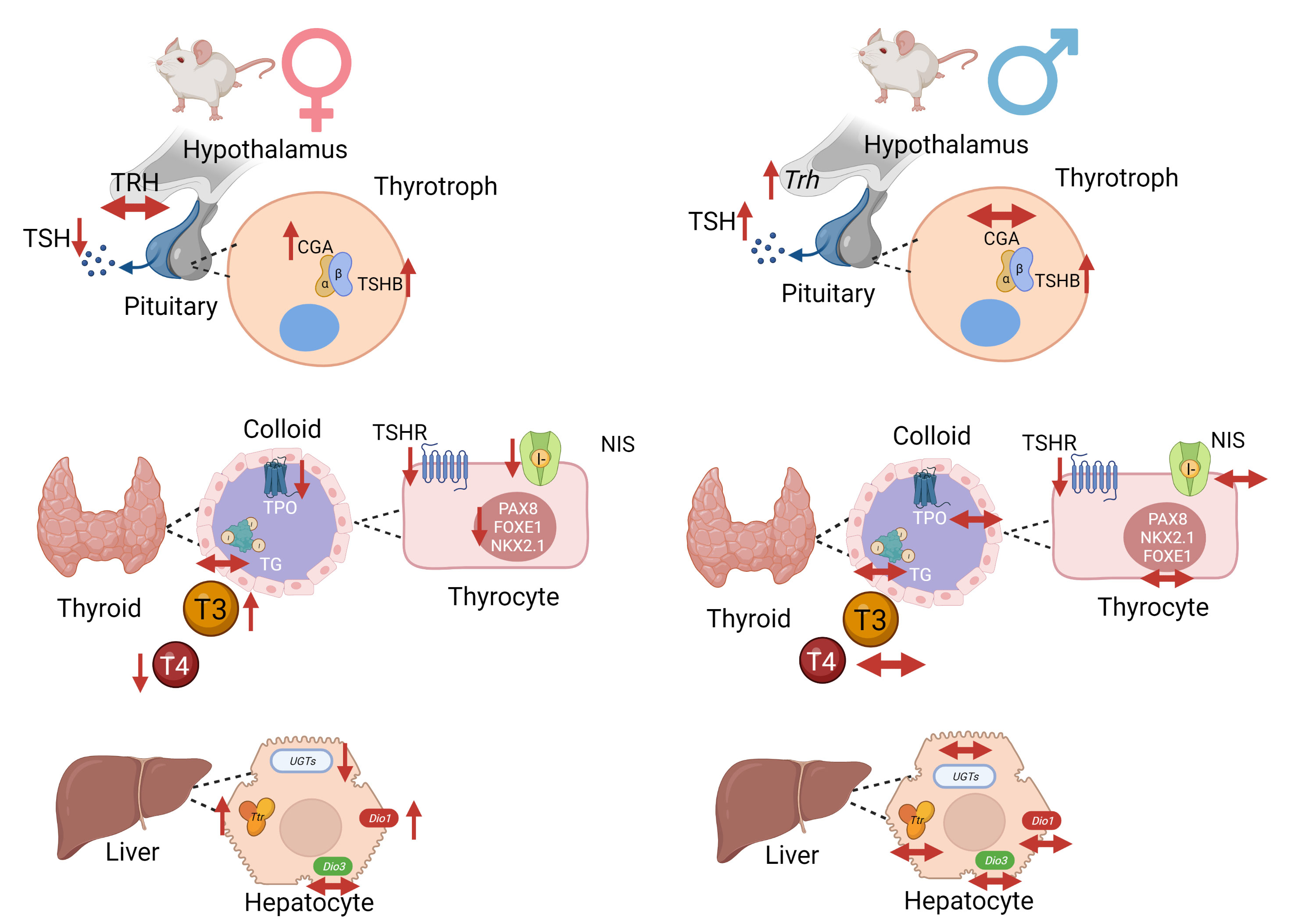
Figure 10 Schematic representation of DEHP effects in the HPT axis of adult F1 female and male offspring animals. In summary, intrauterine exposure to DEHP induced hypothyroidism in adult female offspring rats and subclinical hypothyroidism in adult male offspring rats. Created with BioRender.com.
4 Discussion
The data presented in this study demonstrated that intrauterine exposure to DEHP disrupts the HPT axis of the offspring rats during adulthood. Our results also suggest that the male and female offspring present different sensitivity to DEHP exposure.
The disruptive effects of DEHP exposure in the HPT axis were previously reported in the literature (38–40). However, although these studies were very elucidative about the deleterious effects of DEHP on thyroid function, the studied doses were much higher than those used herein. Moreover, most of the previous studies were performed in male rats. It is well known that the prevalence of thyroid diseases is higher in women than in men (41). For that reason, we aimed to evaluate the consequences of DEHP exposure in both genders. Therefore, our study was the first to describe the effects of intrauterine exposure to DEHP in the programming of the HPT axis both in male and female offspring.
The adverse effects triggered by intrauterine exposure to DEHP were previously reported in the reproductive function and neurobehavioral of the offspring (32, 42). However, the impact of this exposure directly on thyroid function is poorly described (33, 43). Therefore, this is a limitation of the present study since it is difficult to compare the obtained results with those presented in the literature.
In the hypothalamus of the adult F1 female rats, there was no alteration in the gene/protein expression of TRH. Contrary to these results, the male offspring rats presented increased Trh mRNA expression in the lowest treatment dose without significant alterations in the TRH protein content. Sun et al. also observed increased TRH content in the hypothalamus of male rats directly exposed to high doses of DEHP (50 to 500 mg/kg/day) (39).
It is well described that thyroid hormones exert a negative feedback loop in the hypothalamus and pituitary (44). Therefore, our data suggest an impairment in the HPT axis function both in the male and female offspring since there was an increased expression of Trh in the hypothalamus of the male rats even in the absence of thyroid hormones alterations, and the Trh expression was not upregulated in the females with reduced T4 levels.
In the pituitary, the adult F1 female rats presented increased gene/protein expression of CGA, especially in the lowest dose of DEHP treatment. It is well known that the glycoprotein hormones, such as TSH, FSH, and LH, are composed of a common alpha-subunit and a specific beta-subunit, which confers biological specificity to these hormones. (45). Therefore, the increased expression of CGA in the female offspring rats’ pituitary suggests that the synthesis and secretion of FSH and LH are potentially altered in the DEHP-exposed animals. These results follow previous data about the disruptive effects of DEHP exposure, including during the intrauterine period, in the hypothalamus-pituitary-ovary axis function and the reproductive outcomes in female rats (35, 42). Interestingly, the exposure to DEHP during the intrauterine period decreased TSH levels only in the F1 female offspring exposed to the highest dose of treatment
The exposure to DEPH increased the content of the TSHB in the adult F1 male rats. This result was consistent with the increased TSH serum levels that were observed in the DEHP-exposed animals. Even though the effects of DEHP exposure on the expression/secretion of TSH are still controversial, Dong et al. demonstrated that the perinatal exposure to DEHP (30 to 750 mg/kg/day) increased the TSH serum levels of the pups at PND 7, PND 14, and PND 21 (43). The results also agree with previous results that demonstrated increased TSH levels in the male offspring rats of rat dams exposed to 600 mg/kg/day of DEHP (33). Moreover, our results are consonant with previous studies carried out in zebrafish that reported a significant increase in the Tshb expression MEHP-exposed animals (40 and 200 μg/L) (46). Nevertheless, studies performed with male rats chronically exposed to DEHP (600 mg/kg/day) presented decreased levels of TSH. (40). These discordant results indicate that the exposure period is crucial to determine the DEHP-triggered effects on the TSH expression in the pituitary.
TSH is the primary regulator of thyroid morphology and all the steps involved in thyroid hormone synthesis. TSH triggers its effects through the binding in the TSHR expressed in the basolateral membrane of thyrocytes (47). As described herein, the intrauterine exposure to DEHP triggered different results in the thyroid of the adult F1 female and male offspring rats. In the F1 female rats, there was a significant reduction in the expression of genes/proteins involved in the synthesis and secretion of thyroid hormones in both studied doses of DEHP, which was consistent with the reduced serum levels of T4 in these animals. However, in the males, thyroid gene expression was not altered by DEHP exposure, although TSHR expression was reduced in these animals. Moreover, the thyroid hormone levels were not changed in the adult F1 males, although TSH levels were significantly increased in both studied doses.
The disruptive effect of DEHP on TSH, TSH signaling pathway, and thyroid hormone levels have been previously reported, especially in male rats. Indeed, it has been demonstrated that the exposure of rats to high doses of DEHP (50 and 500 mg/kg/day) for two weeks reduced T3 and T4 levels and the Tshr mRNA expression in the thyroid gland (48). Rats chronically and directly exposed to DEHP (250, 500, or 750 mg/kg/day) presented reduced levels of thyroid hormones or increased levels of TSH (26, 27, 49).
Indeed, thyroid transcription factors are regulated by TSH and are critical to maintaining thyroid function and controlling the expression of thyroid differentiation genes (50, 51). Thus, the significant reduction of Pax8, Nkx2.1, and Foxe1 mRNA in the thyroid of F1 female rats could justify the decrease in the expression of the other thyroid genes. Additionally, the reduced TSH serum levels observed in the females exposed to the highest dose of DEHP could also contribute to the reduced thyroid transcriptional activity. Therefore, in the males, the absence of thyroid gene expression regulation, even with high serum circulating levels of TSH, suggests that the thyroid gland is hypofunctional in these animals. This hypothesis is strengthened by the reduced expression of TSHR in the thyroid of DEHP-exposed animals.
The impact of DEHP exposure on thyroid gene expression and function is still inconclusive. In fact, previous studies demonstrated that chronic exposure to high doses of DEHP (150, 300, 600 mg/kg/day) induced a stimulatory effect on Slc5a5, Tpo, Tshr, and Tg mRNA expression in rats (40). In agreement, rats perinatally exposed to DEHP also presented increased expression of genes/proteins involved in the biosynthesis of thyroid hormones (43). On the other hand, Kim et al. demonstrated reduced expression of TSHR in male rats exposed to DEHP (50 and 500mg/kg/day) for 14 days (48). These results are in accordance with previous studies that demonstrated reduced expression of NIS and TPO in rats chronically treated with high doses of DEHP (500 and 750 mg/kg/day) (27). Although this is the first study to evaluate the impact of in utero DEHP exposure in the thyroid gene/protein expression of the offspring during adulthood, previous studies have clearly demonstrated impairment in the thyroid hormones secretion and action in DEHP-exposed animals during critical windows of susceptibility (32, 33, 52). These data reinforce that gender, the treatment dose, and the period of exposure are crucial to determining the effects of DEHP on the HPT axis.
Although the female rats presented decreased expression of genes/proteins involved in thyroid hormone production, T3 levels were increased in the lowest treatment dose. Previous data indicated increased T3 serum levels and decreased T4 levels in animals exposed to DEHP (300 mg of DEHP/kg/day) (40, 53).
Thyroid hormone serum levels depend on the thyroid secretion rate and the expression/activity of deiodinases in the peripheral tissues (54). Therefore, the increased expression of Dio1 mRNA in the liver of F1 female rats could contribute to the increased circulating levels of T3. Conversely, there were no significant alterations in the Dio1 mRNA expression in the liver of adult F1 male rats, suggesting a sexually different response in the liver of DEHP-exposed animals.
The regulation of deiodinases expression by DEHP has already been reported in the literature. Indeed, the exposure of male rats to DEHP (600 mg/kg/day) for six months increased the Dio1 mRNA expression in the liver (55). Perinatal exposure to DEHP was also related to increased Dio1 mRNA levels in PND14 and PND21 rats (43). Furthermore, zebrafish exposed to significant environmental concentrations of DEHP presented increased expression of Dio1 and Dio 2 transcripts (46).
Transthyretin (Ttr) is the main transport protein of thyroid hormones in rats (56). In the present study, in-utero exposure to DEHP increased the Ttr mRNA expression in the liver of female offspring but not in the male one. In contrast, previous studies have demonstrated a suppressive effect of chronic DEHP exposure in the TTR expression, which was associated with decreased thyroid hormone serum levels (27). Future studies are needed to unravel the sexually dimorphic response of Ttr mRNA expression in DEHP-exposed animals and elucidate the molecular mechanisms involved in the differential regulation of Ttr mRNA expression in animals exposed during critical periods of development or during adulthood.
Finally, UDP-glucuronyltransferases (UGTs) enzymes catalyze the glucuronidation and peripheral degradation of thyroid hormones (56). The regulation of the expression of these enzymes presented a different response in the DEHP-exposed male and female offspring. Future studies might elucidate the molecular pathways in regulating these crucial enzymes in DEHP-exposed animals.
This study has some limitations. First, although we reported several alterations in the gene/protein expression in the HPT axis glands, we couldn’t wholly elucidate the molecular mechanisms involved in this disruption. We have preliminary data suggesting the participation of epigenetic mechanisms in regulating thyroid transcriptional activity, but additional studies will be needed to elucidate their involvement in the disruption of thyroid gene transcription. Moreover, the morphological analysis of the thyroid gland would contribute to understanding if the disruption of the thyroid function in the offspring animals is related to the impairment of the follicle’s structure.
In summary, the data presented herein indicated, for the first time, that intrauterine exposure to DEHP causes long-term damage in the HPT axis function both in the male and female offspring. Furthermore, the results suggest that exposure to DEHP programs the offspring’s thyroid function, increasing their susceptibility to developing thyroid dysfunctions during adulthood.
Data availability statement
The raw data supporting the conclusions of this article will be made available by the authors, without undue reservation.
Ethics statement
The animal study was reviewed and approved by Ethics Committee on Animal Experimentation of the Hospital Israelita Albert Einstein (CEUA 3326/2018).
Author contributions
ES-V performed the treatments and experiments, processed the tissues, collected the data, performed the analysis, and wrote the paper. GH processed the tissues, collected the data, and performed the analysis. RS collected the data, performed the analysis. CS-N conceived and designed the analysis, performed the treatments, and wrote the paper. All authors contributed to the article and approved the submitted version.
Funding
This study was supported by a grant from Fundação de Amparo à Pesquisa do Estado de São Paulo (FAPESP) to CSN (16/18517-8).
Conflict of interest
The authors declare that the research was conducted in the absence of any commercial or financial relationships that could be construed as a potential conflict of interest.
Publisher’s note
All claims expressed in this article are solely those of the authors and do not necessarily represent those of their affiliated organizations, or those of the publisher, the editors and the reviewers. Any product that may be evaluated in this article, or claim that may be made by its manufacturer, is not guaranteed or endorsed by the publisher.
Supplementary material
The Supplementary Material for this article can be found online at: https://www.frontiersin.org/articles/10.3389/fendo.2022.995491/full#supplementary-material
References
1. Bergé A, Cladière M, Gasperi J, Coursimault A, Tassin B, Moilleron R. Meta-analysis of environmental contamination by phthalates. Environ Sci pollut Res Int (2013) 20:8057–76. doi: 10.1007/s11356-013-1982-5
2. Hongjun Y, Wenjun X, Qing L, Jingtao L, Hongwen Y, Zhaohua L. Distribution of phthalate esters in topsoil: A case study in the yellow river delta, China. Environ Monit Assess (2013) 185:8489–500. doi: 10.1007/s10661-013-3190-7
3. Dickson-Spillmann M, Siegrist M, Keller C, Wormuth M. Phthalate exposure through food and consumers’ risk perception of chemicals in food. Risk Anal (2009) 29:1170–81. doi: 10.1111/j.1539-6924.2009.01233.x
4. Rowdhwal SSS, Chen J. Toxic effects of di-2-ethylhexyl phthalate: an overview. BioMed Res Int (2018) 22(2018):1750368. doi: 10.1155/2018/1750368
5. Trnka B, Polan M, Zigmont VA. Exposure to di-2-ethylhexyl phthalate (DEHP) and infertility in women, NHANES 2013-2016. Reprod Toxicol (2021) 103:46–50. doi: 10.1016/j.reprotox.2021.05.010
6. Zou Q-Y, Hong S-L, Kang H-Y, Ke X, Wang X-Q, Li J, et al. Effect of di-(2-ethylhexyl) phthalate (DEHP) on allergic rhinitis. Sci Rep (2020) 10:14625. doi: 10.1038/s41598-020-71517-6
7. Crobeddu B, Ferraris E, Kolasa E, Plante I. Di(2-ethylhexyl) phthalate (DEHP) increases proliferation of epithelial breast cancer cells through progesterone receptor dysregulation. Environ Res (2019) 173:165–73. doi: 10.1016/j.envres.2019.03.037
8. Clark KE, David RM, Guinn R, Kramarz KW, Lampi MA, Staples CA. Modeling human exposure to phthalate esters: A comparison of indirect and biomonitoring estimation methods. Hum Ecol Risk Assess (2011) 17:923–65. doi: 10.1080/10807039.2011.588157
9. Serrano SE, Braun J, Trasande L, Dills R, Sathyanarayana S. Phthalates and diet: a review of the food monitoring and epidemiology data. Environ Health (2014) 13:1–14. doi: 10.1186/1476-069X-13-43
10. Huang C-F, Wang I-J. Changes in urinary phthalate metabolite levels before and after the phthalate contamination event and identification of exposure sources in a cohort of Taiwanese children. Int J Environ Res Public Health (2017) 14:935. doi: 10.3390/ijerph14080935
11. Erkekoglu P, Kocer-Gumusel B. Genotoxicity of phthalates. Toxicol Mech Methods (2014) 24:616–26. doi: 10.3109/15376516.2014.960987
12. Martinez-Arguelles DB, Campioli E, Culty M, Zirkin BR, Papadopoulos V. Fetal origin of endocrine dysfunction in the adult: The phthalate model. J Steroid Biochem Mol Biol (2013) 137:5–17. doi: 10.1016/j.jsbmb.2013.01.007
13. Mantzouratou P, Lavecchia AM, Xinaris C. Thyroid hormone signalling in human evolution and disease: A novel hypothesis. J Clin Med (2022) 11:43. doi: 10.3390/jcm11010043
14. Gereben B, Zavacki AM, Ribich S, Kim BW, Huang SA, Simonides WS, et al. Cellular and molecular basis of deiodinase-regulated thyroid hormone signaling. Endocr Rev (2008) 29:838–98. doi: 10.1210/er.2008-0019
15. Mullur R, Liu Y-Y, Brent GA. Thyroid hormone regulation of metabolism. Physiol Rev (2014) 94:355–82. doi: 10.1152/physrev.00030.2013
16. Zuñiga LFF, Muñoz YS, Pustovrh MC. Thyroid hormones: Metabolism and transportation in the fetoplacental unit. Mol Reprod Dev (2022) 89:526–39. doi: 10.1002/mrd.23647
17. Patel J, Landers K, Li H, Mortimer RH, Richard K. Thyroid hormones and fetal neurological development. J Endocrinol (2011) 209:1–8. doi: 10.1530/JOE-10-0444
18. Bernal J. Thyroid hormone regulated genes in cerebral cortex development. J Endocrinol (2017) 232:R83–97. doi: 10.1530/JOE-16-0424
19. Tsai H-J, Wu C-F, Tsai Y-C, Huang P-C, Chen M-L, Wang S-L, et al. Intake of phthalate-tainted foods and serum thyroid hormones in Taiwanese children and adolescents. Sci Rep (2016) 6:30589. doi: 10.1038/srep30589
20. Zhao Y, Song X, Ding S, Qi W, Zhang Y, Xu Q, et al. The associations of urinary DEHP metabolite levels, serum thyroid hormones, and thyroid-related genes among the adolescent students from China: a cross-sectional study. Environ Sci pollut Res (2022) 29:19081–97. doi: 10.1007/s11356-021-16909-5
21. Huang P-C, Waits A, Chen H-C, Chang W-T, Jaakkola JJK, Huang H-B. Mediating role of oxidative/nitrosative stress biomarkers in the associations between phthalate exposure and thyroid function in Taiwanese adults. Environ Int (2020) 140:105751. doi: 10.1016/j.envint.2020.105751
22. Derakhshan A, Shu H, Broeren MAC, Lindh CH, Peeters RP, Kortenkamp A, et al. Association of phthalate exposure with thyroid function during pregnancy. Environ Int (2021) 157:106795. doi: 10.1016/j.envint.2021.106795
23. Huang P-C, Tsai C-H, Liang W-Y, Li S-S, Huang H-B, Kuo P-L. Early phthalates exposure in pregnant women is associated with alteration of thyroid hormones. PloS One (2016) 11:e0159398. doi: 10.1371/journal.pone.0159398
24. Huang P-C, Chang W-H, Wu M-T, Chen M-L, Wang I-J, Shih S-F, et al. Characterization of phthalate exposure in relation to serum thyroid and growth hormones, and estimated daily intake levels in children exposed to phthalate-tainted products: A longitudinal cohort study. Environ pollut (2020) 264:114648. doi: 10.1016/j.envpol.2020.114648
25. Yao H, Han Y, Gao H, Huang K, Ge X, Xu Y, et al. Maternal phthalate exposure during the first trimester and serum thyroid hormones in pregnant women and their newborns. Chemosphere (2016) 157:42–8. doi: 10.1016/j.chemosphere.2016.05.023
26. Ye H, Ha M, Yang M, Yue P, Xie Z, Liu C. Di2-ethylhexyl phthalate disrupts thyroid hormone homeostasis through activating the Ras/Akt/TRHr pathway and inducing hepatic enzymes. Sci Rep (2017) 7:40153. doi: 10.1038/srep40153
27. Liu C, Zhao L, Wei L, Li L. DEHP reduces thyroid hormones via interacting with hormone synthesis-related proteins, deiodinases, transthyretin, receptors, and hepatic enzymes in rats. Environ Sci pollut Res (2015) 22:12711–9. doi: 10.1007/s11356-015-4567-7
28. Kim S, Park G-Y, Yoo YJ, Jeong JS, Nam KT, Jee S-H, et al. Di-2-ethylhexylphthalate promotes thyroid cell proliferation and DNA damage through activating thyrotropin-receptor-mediated pathways in vitro and in vivo. Food Chem Toxicol (2019) 124:265–72. doi: 10.1016/j.fct.2018.12.010
29. Gluckman PD, Hanson MA, Cooper C, Thornburg KL. Effect of in utero and early-life conditions on adult health and disease. New Engl J Med (2008) 359:61–73. doi: 10.1056/NEJMra0708473
30. Hocher B. More than genes: The advanced fetal programming hypothesis. J Reprod Immunol (2014) 104–105:8–11. doi: 10.1016/j.jri.2014.03.001
31. Bianco-Miotto T, Craig JM, Gasser YP, van Dijk SJ, Ozanne SE. Epigenetics and DOHaD: from basics to birth and beyond. J Dev Orig Health Dis (2017) 8:513–9. doi: 10.1017/S2040174417000733
32. Lv J, Li Y, Chen J, Li R, Bao C, Ding Z, et al. Maternal exposure to bis(2-ethylhexyl) phthalate during the thyroid hormone-dependent stage induces persistent emotional and cognitive impairment in middle-aged offspring mice. Food Chem Toxicol (2022) 163:112967. doi: 10.1016/j.fct.2022.112967
33. Zhang Y, Zhang W, Fu X, Zhou F, Yu H, Na X. Transcriptomics and metabonomics analyses of maternal DEHP exposure on male offspring. Environ Sci pollut Res (2018) 25:26322–9. doi: 10.1007/s11356-018-2596-8
34. Lyche JL, Gutleb AC, Bergman Å, Eriksen GS, Murk AJ, Ropstad E, et al. Reproductive and developmental toxicity of phthalates. J Toxicol Environ Health Part B (2009) 12:225–49. doi: 10.1080/10937400903094091
35. Blystone CR, Kissling GE, Bishop JB, Chapin RE, Wolfe GW, Foster PMD. Determination of the di-(2-ethylhexyl) phthalate NOAEL for reproductive development in the rat: Importance of the retention of extra animals to adulthood. Toxicological Sci (2010) 116:640–6. doi: 10.1093/toxsci/kfq147
36. Serrano-Nascimento C, Calil-Silveira J, Dalbosco R, Zorn TT, Nunes MT. Evaluation of hypothalamus-pituitary-thyroid axis function by chronic perchlorate exposure in male rats. Environ Toxicol (2018) 33:209–19. doi: 10.1002/tox.22509
37. Sander H, Wallace S, Plouse R, Tiwari S, Gomes AV. Ponceau s waste: Ponceau s staining for total protein normalization. Anal Biochem (2019) 575:44–53. doi: 10.1016/j.ab.2019.03.010
38. Xu Q, Zhao T, Ri H, Ye J, Zhao W, Zhang Y, et al. Di(2-ethylhexyl) phthalate induced thyroid toxicity via endoplasmic reticulum stress: In vivo and in vitro study. Environ Toxicol (2022) 37:2924–36. doi: 10.1002/tox.23648
39. Sun D, Zhou L, Wang S, Liu T, Zhu J, Jia Y, et al. Effect of di-(2-ethylhexyl) phthalate on the hypothalamus-pituitary-thyroid axis in adolescent rat. Endocr J (2022) 69:EJ17–0272r. doi: 10.1507/endocrj.EJ17-0272r
40. Wu H, Zhang W, Zhang Y, Kang Z, Miao X, Na X. Novel insights into di‐(2‐ethylhexyl)phthalate activation: Implications for the hypothalamus‐pituitary‐thyroid axis. Mol Med Rep (2021) 23:290. doi: 10.3892/mmr.2021.11930
41. Okosieme OE, Khan I, Taylor PN. Preconception management of thyroid dysfunction. Clin Endocrinol (Oxf) (2018) 89:269–79. doi: 10.1111/cen.13731
42. Niermann S, Rattan S, Brehm E, Flaws JA. Prenatal exposure to di-(2-ethylhexyl) phthalate (DEHP) affects reproductive outcomes in female mice. Reprod Toxicol (2015) 53:23–32. doi: 10.1016/j.reprotox.2015.02.013
43. Dong J, Cong Z, You M, Fu Y, Wang Y, Wang Y, et al. Effects of perinatal di (2-ethylhexyl) phthalate exposure on thyroid function in rat offspring. Environ Toxicol Pharmacol (2019) 67:53–60. doi: 10.1016/j.etap.2019.01.012
44. Chiamolera MI, Wondisford FE. Minireview: Thyrotropin-releasing hormone and the thyroid hormone feedback mechanism. Endocrinology (2009) 150:1091–6. doi: 10.1210/en.2008-1795
45. Pierce JG. Eli Lilly lecture: The subunits of pituitary thyrotropin–their relationship to other glycoprotein hormones. Endocrinology (1971) 89:1331–44. doi: 10.1210/endo-89-6-1331
46. Zhai W, Huang Z, Chen L, Feng C, Li B, Li T. Thyroid endocrine disruption in zebrafish larvae after exposure to mono-(2-ethylhexyl) phthalate (MEHP). PloS One (2014) 9:e92465. doi: 10.1371/journal.pone.0092465
47. Vassart G, Dumont JE. The thyrotropin receptor and the regulation of thyrocyte function and growth. Endocr Rev (1992) 13:596–611. doi: 10.1210/edrv-13-3-596
48. Kim MJ, Kim HH, Song YS, Kim O-H, Choi K, Kim S, et al. DEHP down-regulates tshr gene expression in rat thyroid tissues and FRTL-5 rat thyrocytes: A potential mechanism of thyroid disruption. Endocrinol Metab (2021) 36:447. doi: 10.3803/EnM.2020.920
49. Zhang P, Guan X, Yang M, Zeng L, Liu C. Roles and potential mechanisms of selenium in countering thyrotoxicity of DEHP. Sci Total Environ (2018) 619–620:732–9. doi: 10.1016/j.scitotenv.2017.11.169
50. Fernandez LP, Lopez-Marquez A, Santisteban P. Thyroid transcription factors in development, differentiation and disease. Nat Rev Endocrinol (2015) 11:29–42. doi: 10.1038/nrendo.2014.186
51. Kambe F, Nomura Y, Okamoto T, Seo H. Redox regulation of thyroid-transcription factors, pax-8 and TTF-1, is involved in their increased DNA-binding activities by thyrotropin in rat thyroid FRTL-5 cells. Mol Endocrinol (1996) 10:801–12. doi: 10.1210/mend.10.7.8813721
52. Zhao L-L, Shen R, Sun C-C, Zhang S-Y, Wang B, Sun M-F, et al. Prenatal di-(2-ethylhexyl) phthalate maternal exposure impairs the spatial memory of adult mouse offspring in a phase- and gender-dependent manner. Environ Sci pollut Res (2020) 27:28267–75. doi: 10.1007/s11356-020-09181-6
53. Sherif NAE-H, El-Banna A, Abdel-Moneim RA, Sobh ZK, Balah MIF. The possible thyroid disruptive effect of di-(2-ethyl hexyl) phthalate and the potential protective role of selenium and curcumin nanoparticles: A toxicological and histological study. Toxicol Res (Camb) (2022) 11:108–21. doi: 10.1093/toxres/tfab122
54. Bianco AC, da Conceição RR. The deiodinase trio and thyroid hormone signaling. Thyroid Hormone Nucl Receptor (2018), 1801:67–83. doi: 10.1007/978-1-4939-7902-8_8
55. Dong X, Dong J, Zhao Y, Guo J, Wang Z, Liu M, et al. Effects of long-term in vivo exposure to di-2-ethylhexylphthalate on thyroid hormones and the TSH/TSHR signaling pathways in wistar rats. Int J Environ Res Public Health (2017) 14:44. doi: 10.3390/ijerph14010044
Keywords: phthlates, DEHP, endocrine disruptors, hypothalamus-pituitary-thyroid axis, intrauterine period, DOHaD
Citation: Sousa-Vidal ÉK, Henrique G, Silva RECd and Serrano-Nascimento C (2023) Intrauterine exposure to di(2-ethylhexyl) phthalate (DEHP) disrupts the function of the hypothalamus-pituitary-thyroid axis of the F1 rats during adult life. Front. Endocrinol. 13:995491. doi: 10.3389/fendo.2022.995491
Received: 15 July 2022; Accepted: 07 December 2022;
Published: 13 January 2023.
Edited by:
Maaike Van Gerwen, Icahn School of Medicine at Mount Sinai, United StatesReviewed by:
Sakina Mhaouty-Kodja, Centre National de la Recherche Scientifique (CNRS), FranceLin Ye, Jilin University, China
Jing-Cai Liu, Nanjing Agricultural University, China
Copyright © 2023 Sousa-Vidal, Henrique, Silva and Serrano-Nascimento. This is an open-access article distributed under the terms of the Creative Commons Attribution License (CC BY). The use, distribution or reproduction in other forums is permitted, provided the original author(s) and the copyright owner(s) are credited and that the original publication in this journal is cited, in accordance with accepted academic practice. No use, distribution or reproduction is permitted which does not comply with these terms.
*Correspondence: Caroline Serrano-Nascimento, Y2Fyb2xpbmUuc2VycmFub0B1bmlmZXNwLmJy