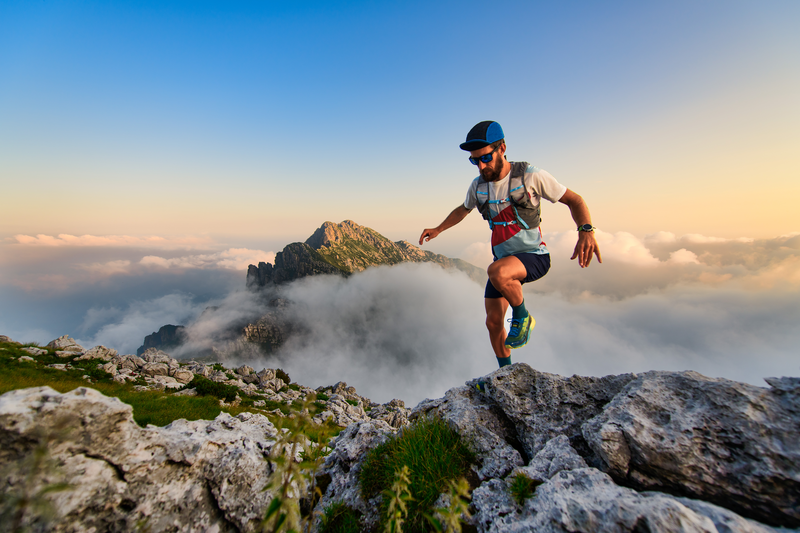
94% of researchers rate our articles as excellent or good
Learn more about the work of our research integrity team to safeguard the quality of each article we publish.
Find out more
REVIEW article
Front. Endocrinol. , 04 October 2022
Sec. Systems Endocrinology
Volume 13 - 2022 | https://doi.org/10.3389/fendo.2022.989135
The physiological functions of organs are intercommunicated occurring through secreted molecules. That exercise can improve the physiological function of organs or tissues is believed by secreting myokines from muscle to target remote organs. However, the underlying mechanism how exercise regulates the inter-organ communications remains incompletely understood yet. A recently identified myokine–irisin, primarily found in muscle and adipose and subsequently extending to bone, heart, liver and brain, provides a new molecular evidence for the inter-organ communications. It is secreted under the regulation of exercise and mediates the intercommunications between exercise and organs. To best our understanding of the regulatory mechanism, this review discusses the recent evidence involving the potential molecular pathways of the inter-organ communications, and the interactions between signalings and irisin in regulating the impact of exercise on organ functions are also discussed.
It has long been suspected that physiological functions are mediated through the inter-organ communications (1–3), and such communications mainly occur through secreted molecules. The muscle, for example, is known as a motor organ, but compelling evidence has implied that it is also an endocrine organ, secreting hundred proteins (known as myokines) during exercise that enter circulatory system and function as endocrine modulators mediating the adaption of organs and tissues to exercise (4). Obesity and insulin resistance are regarded as chronic inflammation diseases; endurance exercise is effective in improving obesity and insulin resistance by stimulating muscle to secret myokines promoting energy metabolism and alleviating inflammation status (2, 5, 6). Similarly, brain, heart, bone, and liver also are responded to exercise training via secreted myokines (7–12).
Since the identification of the novel myokine, fibronectin type III domain-containing protein 5 (FNDC5), this link between organs gains its new molecular evidence (5, 12–14). The expression of Fndc5 is regulated by peroxisome proliferator-activated receptor γ coactivator 1α (PGC1α) that acts as a transcriptional co-regulator produced by exercising muscle (5). It has gained great attention in regulating the physiological and pathological processes of organs in the condition of health and diseases recently. It involves the process of inter-organ crosstalk between muscle, bone, liver, heart, and brain, and new evidence on the molecular signalings engaged in the communications has been proposed recently (1, 10, 12, 13, 15, 16). The primary goal of this review is to best our understanding of the signaling pathways for irisin regulating the inter-organ communications in health and diseases, and also to provide an approach to understanding the adaptations of organs to exercise
About twenty years ago, two research groups independently reported a novel gene that was expressed in rodent skeletal muscle, heart, brain, and other tissues (17, 18). One decade later, this gene was named Fndc5, one of the target genes of PGC1α, a transcriptional co-regulator usually secreted by exercising muscles (5). FNDC5 is highly expressed in excising or genetically overexpressing PGC1α (Ppargc1a) mice, and the medium of cultured myocytes form mice overexpressing Fndc5 induces the upregulation of uncoupling protein (Ucp) 1 mRNA in adipocytes, which is a pivotal gene promoting brown adipose tissues (BAT). Moreover, irisin also promotes mitochondrial biogenesis, another hallmark of fat browning, subsequently upregulating mitochondrial DNA contents (mtDNA) and mitochondrial biogenesis-associated genes, including mitochondrial transcription factor A (TFAM), nuclear respiratory factors 1 and 2 (NRF1 and NRF2) and UCP1 in human adipocytes (19, 20). So far, numerous studies have confirmed the role of FNDC5 in regulating energy metabolism (5, 12, 14). In the meantime, a novel myokine–irisin has been found; it comprises of 112 amino acid (aa) and is derived from FNDC5 by cleaving at the 30th and 142th aa sites by proteolytic enzymes (Figure 1) (5). It is proposed that the secreted irisin involves in the positive effect of exercise on metabolism through browning of adipose tissue. Thereafter, thousand papers have been published to explore the associations of FNDC5/irisin with a variety of exercise protocols and pathophysiological situations, as well as ex vivo and in vivo influence of recombinant irisin (r-irisin) treatment on various cells and different rodent models.
Figure 1 Structure of irisin. (A) Schematic structure of mouse fibronectin type III domain-containing protein 5 (FNDC5) protein. (B) A model of transmembrane FNDC5 and the linker that locates between the FNIII domain and the transmembrane helix. The N-terminal signal sequence is proposed to be cleaved. (C) Structure of irisin. It is a proteolytic product of FNDC5. The irisin domain is connected to a short transmembrane region, which is followed by the cytosolic region. (D) The diagram of the irisin dimer. The C’ strands pair to form an eight-strand beta sheet in the dimer.
The construct of irisin contains the classical FNIII region and the linker (Figure 1) (21). Albrecht et al. (22) measured non-glycosylated r-irisin by performing a band with the molecular weight (MW) of 13 000, close to 12 300 for this molecule, and glycosylated irisin running a band with the MW of 20 000. There are mainly 3 methods to measure secreted irisin, i.e. Enzyme-Linked Immunosorbent Assays (ELISA), Western Blot, and Mass Spectrometry (MS). Among those approaches, MS is recognized as “gold standard” for measuring the protein levels (23). To date, the widely recognized reference levels for blood irisin were 3~4 ng/mL (24) and 0.3 ng/mL for humans and mice (14), respectively.
However, for measurement of irisin with Western blot methods, only a small number of studies reported the bands approximate to the predicated value for deglycosylated irisin (13 000) in rodent and human circulation (24–26). The most confusion concerning the detection of irisin with Western blot methods is that the reporting of bands of MW shows much divergent values, indicating that the anti-body used are different between the reports. Huh and colleagues (27) first determined circulating irisin using ELISA and reported a level of 113.1 ± 20.6 ng/mL among a population of common women. Afterward, De Meneck et al. (28) reported that children with overweight/obesity showed higher circulating irisin levels (143.1 ng/mL) than those with normal weight (75.2 ng/mL). This began a period of testing circulating irisin in various conditions (29–31). The most hallmark of those studies is the extreme values reported, varying from 40 pg/mL (30) to 14.78 µg/mL (32). No specific antibodies for irisin may account for the divergent reports.
Quantitative MS is conducted via a novel approach with the combination of detecting specific peptides for irisin and adding quantified dose of marked peptides to the sample which is applied to measure the concentrations of unmarked irisin within the sample by comparing the peak heights or areas. By labeling both FIQEVNTTTR and DSPSAPVNTVR (N-terminus), Jedrychowski et al. (24) reported the circulating irisin levels about 4 ng/mL in human samples. They also reported a circulating concentration of 4.3 ng/mL for irisin in the group with aerobic exercise training. In a murine serum sample, Albrecht et al. (33) measured irisin levels about 0.6 ~ 0.9 ng/mL. Before conducting MS measurement, proteins, such as albumins and immunoglobulins, in the blood samples must be removed that results in divergent amount of proteins left for measurement, which could lead to different irisin levels reported between studies.
Irisin is expressed almost in whole tissues and organs of rodents and humans; it presents in the liver, heart, kidney, brain, skin, muscle, retina, and pineal gland in rodents (17, 34–38), and also appears in human liver, pancreas, stomach, cerebrospinal fluid, serum, saliva and urine (39–42). Varied human tissues express diverse concentrations of irisin, with the highest amounts of irisin detected in skeletal muscle, proceeded behind by other muscle-contained tissues. Adipose tissue expressed a level of irisin almost hundred times lower than that of muscle (27, 43, 44). The secretion of irisin is affected by a number of factors, including exercise, cold, diet, drugs, and pathological situations (45), among which exercise is the strongest stimulator for promoting irisin expression. Exercise led to about threefold elevation of Fndc5 mRNA levels in murine muscle compared with that of controls. Additionally, the expression of FNDC5 mRNA in muscle was approximately twofold higher in adult receiving endurance training than that without exercising individuals (46). White adipose tissue (WAT) constitutes an alternative source of irisin contributing approximately one third total levels of circulating irisin, and the secretion of irisin in white adipose tissue significantly increases in response to exercise training (47).
Exercise has many positive effects on brain health, such as reducing the risks of dementia, depression and stress, restoring or maintaining cognitive function and metabolic control (1, 48, 49). The facts that brain not only controls the action of muscle during physical exercise, but it senses and responds to proteins secreted from muscle during exercise, indicating a direct crosstalk between muscle and brain. Accumulating evidence has confirmed muscle as an endocrine organ, releasing myokines (such as cathepsin B and PGC1α) into blood to mediate the function of remote organs (4, 9). Since identified as a novel myokine, irisin has gained a lot of interests for its beneficial effects in regulating neuronal generation, synaptic plasticity, inflammation, as well as cognition. In this section, we will discuss the evidence on the mechanism that modulates the communication between irisin and brain function in physical and pathological conditions.
The expression of irisin in the cerebrospinal fluid (CSF) of humans has been detected by both Western blot and MS (17, 50). Moreover, many regions of brain express FNDC5, such as cerebellar Purkinje cells (5, 17, 18), the hypothalamus (51), and hippocampus (52). FNDC5 mRNA is found to be up-regulated in differentiated human embryonic stem cells (hESCs) and reaches the highest expression level in neural cells (50, 52). Additionally, during the formation of neuronal precursors, Fndc5 overexpression resulted in elevated glial fibrillary acidic protein (GFAP), brain-derived neurotrophic factor (BDNF), Neurocan, and Microtubule-associated protein 2 (Map2). Contrarily, Fndc5 knockout (KO) in neuronal precursor cells impaired their maturation (53). Taken together, the evidence suggests that presence of FNDC5 is essential for the differentiation, proliferation, and maturation of neuronal cells.
Fndc5 gene expression in neurons is under the regulation of PGC1α. Up-regulating Ppargc1a gene expression by adding forskolin (10 mM) into primary cortical neurons was accompanied by a significant elevation of Fndc5 gene expression, whereas down-regulating Ppargc1a gene expression by nifedipine (5 mM) administration decreased Fndc5 expression levels (52). Furthermore, forced Ppargc1a expression in primary cortical neurons led to a fourfold increase in Fndc5 mRNA levels. Conversely, blocking of Ppargc1a gene expression with lentiviral-mediated shRNA KO decreased Fndc5 mRNA expression by more than 40% (52). Additionally, the expression of Bdnf in brain is partly regulated by FNDC5. Wrann et al. (52) found that overexpression of Fndc5 markedly increased Bdnf mRNA levels by fourfold, whereas Fndc5 KO via lentiviral delivery of shRNA significantly reduced Bdnf expression in primary cortical neurons. Primary cortical neuronal cells cultivated with BDNF remarkably deceased Fndc5 mRNA levels in a dose-repose pattern (52). More importantly, peripherally forced expression of Fndc5 in the liver led to increased circulating irisin and up-regulated hippocampal expression of Bdnf (52). To determine the role of peripheral FNDC5/irisin in the brain, Lourenco et al. injected intravenously AdFNDC5 in mice to increase FNDC5/irisin overexpression (13). Intravenous administration of AdFNDC5 led to increment of both plasma and hippocampal FNDC5/irisin expression, and restored novel object recognition (NOR) memory defects in Alzheimer’s disease (AD) mice (13). These data indicate the capacity of circulating FNDC5/irisin entering into the brain to elevate FNDC5/irisin expression, and providing protection against memory impairment.
However, one concern about the beneficial effects of irisin on brain health is that it remains unclear how circulating irisin may pass through the blood-brain barrier (BBB). The BBB prevents the transport of big molecules from circulation to CSF, with only some small proteins capable of entering the central nervous system by passive diffusion (54). It is assumed that if peripheral irisin has effects on brain function, it needs a selective BBB transporter and a receptor on brain cells. It is known that irisin is only one of the exercise-stimulated proteins capable of promoting brain functions (9). Recently, a substitutive mechanism connecting exercise and improvement of learning and memory in rodents has been proposed (55). Lactate levels were elevated during exercise, which subsequently cross BBB and increase Bdnf expression through monocarboxylate transporter (MCT) 2 and upon the activation of Sirtuin 1 (SIRT1)/PGC1α/FNDC5/BDNF signalings (55).
To date, a vast body of literatures have demonstrated that irisin generates beneficial effects on neurodegenerative diseases. Lourenco et al. (13) investigated the capable of FNDC5/irisin alleviating synapse failure and memory impairment in AD. FNDC5/irisin levels were decreased in AD hippocampi and CSF, whereas there had no significant changes in plasma irisin. They knocked down Fndc5 via injecting brain with lentiviruses harboring two types of shRNA constructs. This caused impairment of hippocampal long-term potentiation (LTP) and memory during NOR task. Conversely, elevating Fndc5 expression in hippocampus restored synaptic plasticity and memory in AD mice (13). Similar to the work of Wrann and colleagues, peripherally up-regulating FNDC5/irisin restored memory deficit, whereas blocking FNDC5/irisin expression in peripheral and brain inhibited the protective effects of exercise on synaptic plasticity and memory in AD murine models (13). Those data further supported the beneficial effects of peripheral FNDC5/irisin in improving brain function. Moreover, this link between irisin and its positive effects on brain function provides therapeutic implications of this protein and exercise in alleviating cognitive impairment in neurodegenerative diseases.
Wang et al. (56) cultivated astrocyte-conditioned medium with r-irisin for twelve hours, finding that r-irisin treatment protected the neurons from the toxicity of Amyloid-β peptides (Aβ). Moreover, irisin decreased the production of interleukin (IL)-6 and IL-1β from astrocyte culture and reduced the expression of cyclooxygenase 2 (Cox2) and phosphorylated protein kinase B (AKT) (56). Additionally, irisin attenuated nuclear factor kappa B (NF-κB) expression in astrocytes subjected to Aβ exposure by inhibiting phosphorylation and degradation of IκBα (56). A recent in vitro study investigated the potential role of FNDC5/irisin in the process of Aβ formation (57). Amyloid precursor protein (APP)-FNDC5 communication that occurs via connecting FNDC5 with a specific region between β- and α- secretase cleavage locations of APP decreased Aβ generation, resulting in a reduced production of Aβ40 and Aβ42 peptides in the cultured system (57). Other studies demonstrated that irisin were related to episodic memory and global cognition in individuals with high risk of dementia (58), and affected cognitive degradation in obese subjects at risk of AD (59).
Irisin benefits brain health partly due to its ability of inhibiting the inflammatory pathway. Both irisin and its precursor protein FNDC5 expression were significantly down-regulated whereas oxidative stress and reactive oxygen species (ROS)-NLR family pyrin domain containing 3 (NLRP3) inflammasome signaling were up-regulated in PC12 neuronal cells stimulated with oxygen-glucose deprivation (OGD) (60). The r-irisin treatment reversed OGD-induced oxidative stress and inflammation, but the irisin-related beneficial effects were inhibited by overexpression Nlrp3, suggesting that irisin alleviated OGD-caused neuronal impairment partly through blocking ROS-NLRP3 signalings (60).
Myocardial infarction (MI) occurs when blood supply reduces or ceases to coronary artery of heart, resulting in injuries of heart muscle (61, 62). In US, approximately 550,000 adults experience first heart attack and 200,000 suffer recurrence of MI each year (63); it become a major source of the burden of diseases worldwide. The expressions of Fndc5 mRNA and irisin protein have been detected in cardiomyocytes (35, 64), and irisin treatment increased cardiac regeneration and neovascularization (65) and enhanced diastolic volume, heart rate, and cardiac output (66). Current evidence suggests that irisin production is linked with the occurrence of MI and likely to become a potentially therapeutic target for MI. Cardiac ischemia frequently results in insufficient energy production, and lower irisin levels might benefit the maintenance of energy homeostasis by the suppression of browning white adipose tissue. In accordance with this notion, irisin production were found gradually reducing within 24 hours in a rat model of MI (67), and decreased expression of skeletal muscle Fndc5 and reduced serum irisin levels were detected in rats with MI or cardiac ischemia-related heart failure (68–71).
Current evidence supports irisin as a therapeutic target for MI. Ischemia and reperfusion (I/R) and hypoxia-reoxygenation (HR) are usually applied as common models of MI in vivo and in vitro studies. Wang et al. (72) performed experiments with a rat model of heart I/R to determine whether systemic administration of irisin could protect against I/R-induced tissue injury. The treatment of r-irisin protected rat heart from I/R-induced injury in a dose-dependent manner. They further showed that r-irisin treatment reduced apoptosis of cardiomyocytes and oxidative stress by increasing superoxide dismutase (SOD) activity and restoring the mitochondria localization of SOD2 in cardiomyocytes (72). Liao and colleagues (73) demonstrated that, in a mice MI model, two-week r-irisin administration increased cardiac function, alleviated ventricular dilation, and decreased infarct size and fibrosis at four weeks after MI. The therapeutic effects are associated with the pro-angiogenic function and suppression of cardiomyocyte apoptosis via triggering extracellular signal-regulated kinase (ERK) pathways (73). Zhao et al. (74) reported a protective effect of irisin on H9C2 cells exposed to hypoxia/reoxygenation lesions partially via regulating histone deacetylase 4 (HDAC4). The dynamin-like GTPase optic atrophy1 (Opa1) overexpression could protect myocardial cells against hypoxia-linked impairment and increased cell survival through triggering autophagy. Irisin treatment stimulated Opa1-related autophagy, and protected cardiomyocytes from further damage following MI (75). Additionally, as a treatment strategy, r-irisin administration reduced myocardial infarct size, attenuated cell apoptosis, and preserved mitochondria function, as well as promoting SOD1 and p38 phosphorylation in an in vitro model of myocardial I/R injury (76).
Cardiomyocyte hypertrophy is a common outcome of long-term pressure/volume overload in a variety of cardiovascular diseases (77). Pathological situations, e.g. hypertension and heart attack, continuedly initiate cardiomyocyte hypertrophy and ultimately cause heart failure, meanwhile myocardial cell necrosis, fibrosis, fetal gene activation, disturbance of mitochondria function, and structurally sarcomeric alterations may occur (78). Among the signaling mechanisms that induce these responses, reprogramming of systemic and heart metabolism precede the progress of cardiomyocyte hypertrophy. Given the important role of irisin in regulation of metabolism, it is not surprised when several studies found the associations of irisin expression and the occurrence of cardiac hypertrophy (69, 71, 79). The changes in irisin may imply a damaged metabolic adaptation during the hypertrophic process and are associated with cardiomyocyte hypertrophy-induced cardiomyocyte death.
Both in vivo and ex vivo evidence indicates that FNDC5/irisin is involved in the overload/obesity-induced cardiomyocyte hypertrophy by affecting autophagy, inflammation, fibrosis, and oxidative stress (71, 79, 80). Several signaling pathways are suggested to involve the irisin-associated improvement of cardiomyocyte hypertrophy. Irisin prevents overloading-caused cardiac hypertrophy mainly via triggering defensive autophagy by stimulating Adenosine Monophosphate Activated Protein Kinase (AMPK)-Unc-51 like autophagy activating kinase 1 (ULK1) pathway (71). Moreover, irisin administration could improve overloading-caused cardiac dysfunction and myocardial hypertrophy via suppressing oxidative stress through downregulating AKT signaling activation (81). Yu and colleagues reported that cardiac and circulatory expressions of irisin were increased in cardiac hypertrophy mice, and the treatment of irisin is able to inhibit pathological cardiac hypertrophy and fibrosis through AMPK-mammalian target of rapamycin (mTOR) signaling pathways (79). In addition, Zhao et al. suggested that cardiomyocytes secreted irisin in an ADAM family dependent manner, and irisin administration improved overloading-caused cardiac hypertrophy and fibrosis and enhanced cardiac function by activating AMPK-mTOR signaling pathways (82).
Liver is one target organ of the physiological action of irisin. Liver plays an important role in regulating gluconeogenesis, lip metabolism, inflammation, oxidation, etc. which are associated with hepatic diseases, including hepatitis, cirrhosis, non-alcoholic fatty liver disease (NAFLD), steatohepatitis (NASH), and liver cancer.
Liver regulates blood glucose concentrations by controlling glucose production, uptake, storage, and release via glycogen synthesis and degradation (83), and it is one major organ involving insulin resistance, a hallmark of metabolic syndrome and many of those patients eventually result in the onset of type 2 diabetes mellitus (T2DM) (84). In cultured hepatocytes with insulin resistance, r-irisin treatment (20nM) decreases gluconeogenesis via decreasing phosphoenolpyruvate carboxykinase (PEPCK) and glucose-6-phosphatase (G6Pase) expression through the phosphatidylinositol 3-kinase (PI3K)/AKT/Forkhead box protein O1 (FOXO1) signalings, and increases glycogenesis through PI3K/AKT/glycogen synthase kinase 3 (GSK3)/glycogen synthase (GS) signaling cascades (12, 85). Additionally, in diabetic obese C57BL/6 mice, the injection of r-irisin (0.5 µg/g body weight) caused a reduction in Pepck and G6pase expressions in the liver by stimulating the AMPK signaling pathway, and blocking of AMPK signaling decreased the influence of irisin on hepatocytic expressions of Pepck and G6pase (86).
Moreover, irisin is involved in hepatic cholesterol synthesis. Subcutaneous injection of r-irisin (12 nmol/d·kg body weight) induced a reduction in hepatic cholesterol in obese mice, and, in primary hepatocytes from lean and obese mice, r-irisin treatment remarkably reduced cholesterol concentrations by the inhibition of sterol regulatory element-binding transcription factor 2 (SREBP2) (87). The r-irisin administration prevented the palmitic acid (PA)-caused lipid deposition and lipogenic factors, acetyl CoA carboxylase (ACC) and fatty acid synthase (FAS), via inhibiting protein arginine methyltransferase-3 (PRMT3) in hepatocytes (37). Mo et al. has confirmed FNDC5/irisin to be a direct transcriptional target of constitutive androstane receptor (CAR), and the activation of CAR upregulates Fndc5 expression in the liver (88).
Irisin can act as anti-inflammatory factor in the adipose tissue. Irisin alleviates adipose tissue inflammation partly via inhibiting macrophage polarization (89, 90). More interestingly, recent studies have proposed an anti-inflammatory action of irisin against SARS-CoV-2 infection. RNAseq-based data have revealed that irisin affects multiple genes related to the SARS-CoV-2 infection in human subcutaneous adipocytes (91), suggesting that the potential beneficial effects of irisin on COVID-19 outcomes are beyond its anti-inflammatory action on macrophages. In this regard, irisin inhibits SARS-CoV-2 entry points and the spike glycoprotein subunit S1-induced inflammatory cell death in human visceral adipocytes (92). Irisin also inhibits oxidative stress through reducing inflammatory biomarkers, including NF−κB, COX2, TNF and IL−6, and downregulating hepatocytic ROS generation. The effects are regulated via downregulating PRMT3 (37). Exogenous r-irisin treatment (250 μg/kg) promoted hepatic functions, decreased hepatocytic necrosis and apoptosis, and alleviated inflammation after liver I/R (93). Additionally, r-irisin treatment increased mitochondria function related Ppargc1a and Tfam expressions, and reduced oxidative stress via elevating UCP2 levels in liver I/R (93). Irisin ignited autophagy and promoted mitochondrial action by up-regulating telomerase function in aged liver cells, and then decreased the inflammatory responses, oxidative stress, apoptosis, and hepatic impairment in an aged murine liver I/R model (94). The effects of irisin on the increased telomerase activity is regulated by the inhibition of the phosphorylation of c-Jun N-terminal kinases (JNKs) during hepatic I/R (94).
Additionally, irisin is also involved in the pathological process of human hepatocellular carcinoma (HCC). The levels of Fndc5 mRNA in liver were upregulated in HCC patients compared to that in donors, and positively associated with the transcription factor sterol regulatory element-binding factor-1 (SREBF1), stearoyl-CoA desaturase (SCD1), TNFα, and Il6 mRNA expressions in HCC patients (95). Another study observed a reduction of Fndc5 expression in HCC tissues, and lower circulating irisin levels were related to high comprehensive complication index (CCI) marks after hepatectomy (96). Similarly, Shi and colleagues reported that irisin expression significantly increased in cancerous livers compared with those in the controls. And it upregulated cell proliferation, invasion, and migration partly via activating PI3K/AKT signalings, and decreased the cytotoxicity of doxorubicin in HepG2 cells (97).
The mechanical coupling of skeletal muscle and bone is obvious. Bone continually adjusts its mass and architecture to the changes of mechanical strain, and muscle contractions are essential for applying load to the bone. This mechanical perspective implies that muscle function declines resulting in reduced loading on bone and causing a reduced bone mass subsequently. Moreover, the two tissues also communicate in an endocrine manner in which muscle secretes myokines during exercise and regulates the physiological functions and pathological processes of bone (8, 15, 98). The discovery of irisin receptor–αV/β5 in bone cells provides further molecular evidence for the muscle-bone interaction through irisin signalings in a variety of physiological and pathological conditions (14).
Some studies have reported more interesting findings about injection of r-irisin enhancing bone mass and microstructure in mice. Colaianni et al. (99) measured the tibia bone of mice received four-time injections of 100 µg/kg r-irisin per week. They detected a significant increment of cortical bone mineral density (BMD) and strength. Applying X-ray imaging, r-irisin treatment elevated femoral and tibia bone density and bone circumference. Mice received r-irisin intervention showed elevated polar moment of inertia and bending strength. Moreover, the cortical bone expressed an elevated mRNA levels for osteopontin (Osn) (involved in bone formation) and a decreased sclerostin (SOST) (99). In another study, Levene et al. reported the administration of r-irisin increased BMD and restored impaired microstructure in ovariectomized rats via relieving inflammatory cytokines (TNF-α, IL-6, etc.) and up-regulating bone formation marker genes [such as Runt-related transcription factor 2 (RUNX2), B-cell lymphoma 2 (BCL2) and Nuclear factor erythroid 2-related factor 2 (NRF2)] (100). It is indicated that the positive effects of r-irisin on bone density and microstructure partly via enhancing osteogenic gene expression.
Fndc5 KO is an important approach to the investigation of the effects of irisin on bone remodeling. Luo et al. (101) generated a global Fndc5 KO murine model. They found that the lacking of irisin reduced bone mass and bone strength, and increased osteoclast numbers and RANKL cell surface expression in mice. The levels of IL-6 and tumor necrosis factor-α (TNF-α) were also enhanced in irisin-deficient mice (101). Taken together, it is suggested that irisin seem to involve in the mediation of bone homeostasis. In another study, Zhu and colleagues generated FNDC5/irisin specific ablation mice in osteoblastic lineage (Osx-Cre:FNDC5/irisin KO mice). It led to decreased Fndc5 mRNA and irisin protein expressions in bone, reduced bone mass, and retarded bone formation and mineralization (102). These Fndc5 KO mice also exhibited lower cortical BMD and trabecular bone volume in contrast to controls. However, the cortical bone surface/volume ratio accretion indicated thinner cortical bones (102). Fndc5 KO mice exhibited decreased osteoblastic genes [Runx2, bone sialoprotein (Bsp), osterix (Osx), and alkaline phosphatase (Alp)] and enhanced osteoclastic genes [tartrate-resistant acid phosphatase (Trap), Mmp9, and cathepsin K]. Additionally, exercise did not benefit Fndc5 KO mice by increasing bone strength and body weight loss, but treatment of r-irisin restored the exercise-related beneficial effects (102). However, Kim et al. reported that the Fndc5 KO mice displayed elevated femoral trabecular BMD and better micro-structure (14). More interesting, Fndc5 KO mice protected against ovariectomy exhibiting maintenance of bone mass and changed receptor activator of nuclear factor kappa-B ligand (RANKL) expression. In this study, the authors firstly demonstrated that irisin acted via the αV/β5 integrin receptor in osteocytes (14). Finally, they assumed that the action of irisin might like that of Parathyroid Hormone (PTH), acting to initiate both resorption and formation upon the pattern of r-irisin administration.
Hindlimb unloaded animals were frequently used as a paradigm to investigate the microgravity impact on bone intravitally. The hindlimb-suspended mice showed accelerated femur bone loss, and decreased mineral deposition rates of trabecular and cortical bone (103). Fndc5 together with the osteogenic marker mRNA levels were decreased. The activity of ALP and mRNA expressions of Alp, Colia1 and Fndc5 in primary osteoblasts were decreased under the condition of microgravity. The r-irisin administration (1 nM) for primary osteoblasts in the condition of simulated microgravity up-regulated gene expression of osteogenic markers, ALP activity, and mineralization (103). In a study by Kawao et al., hindlimb unloading decreased muscle cross-section area around tibia, lower leg muscle weights, and tibial trabecular bone density in mice (104). Hindlimb unloading also decreased Fndc5 mRNA expression in the soleus muscle of mice, and it was positively related to trabecular BMD in the tibia and negatively correlated with Rankl mRNA levels in the tibia of mice (104). On the contrary, mechanical loading elevated Fndc5 mRNA levels, which could be inhibited by blocking bone morphogenetic protein (BMP) and PI3K signalings. It is suggested that irisin may involve in the mechanical unloading-caused muscle loss and bone mass reduction, and BMP and PI3K pathways probably involve in those pathophysiological conditions. Metzger et al. reported that weekly three times of r-irisin treatment (18 ng/mL) increased bone homeostasis and bone formation rate in hindlimb unloaded rats, and decreased osteoclast differentiation and production of TNF-α, IL-17, RANKL and SOST in the suspended hindlimb (105). These data suggested that r-irisin treatment protect unloaded rats from disuse-induced bone loss through reducing pro-inflammatory cytokines and bone turnover.
Irisin can also inhibit bone cell apoptosis and then improves ovariectomy-induced bone loss. Xu and colleagues showed that r-irisin administration significantly enhanced trabecular thickness, number, and BMD in estrogen-deficient rats (106). The expressions of Runx2, osteocalcin (OC), Bcl2 and Nrf2 were higher whereas those of caspase 3 and Nlrp3 were lower in the r-irisin intervention group. Irisin increases Nrf2, decreases NLRP3 inflammasome, and reduces inflammatory cytokines, thus mitigating osteoblast apoptosis in estrogen-deficient rats (106).
Inflammatory bowel disease (IBD) is a group of inflammatory conditions of the colon and small intestine that not only leads to gastrointestinal dysfunction but inflammation-related bone loss (107). Twice weekly treatment of r-irisin (18 ng/mL) increase gut and bone properties through alleviating inflammation and reconstituting structure. In bone, IBD increased osteoclast surface and decreased bone formation, and r-irisin treatment reversed this phenotype (107). Furthermore, r-irisin treatment also decreased the levels of TNF-α and RANKL in osteocytes in bone (107). Sprague–Dawley rats with severe IBD received twice weekly r-irisin treatment for 3 weeks showing increased osteogenic rate, reduced osteoclastic resorption, and down-regulated pro-inflammatory cytokines (108).
Circulating irisin concentrations were positively associated with Oc and cyclophosphamide (CTX), and negatively related to Dickkopf-related protein 1 (DKK-1) circulating concentrations (109). Circulating irisin levels were inversely associated with age, PTH, and creatinine levels (110). The evidence implies that irisin might act as a predictor for bone statues and plays a determinant role in bone metabolism. Irisin is correlated to low bone mass in postmenopausal women. Circulating irisin concentrations were markedly decreased in osteoporotic postmenopausal females (111). Serum irisin concentrations were decreased whereas c-reactive protein levels were increased in osteoporotic women, indicating that irisin may act as a modulator in the pathogenesis of osteoporosis. Colaianni et al. reported that circulating irisin concentrations were lower in osteopenic/osteoporotic patients in contrast to healthy individuals, and positively associated with femoral and vertebral BMD in patients with total hip or knee replacement (112).
Zhang et al. reported that older Chinese men with low bone mass had decreased circulating irisin concentrations compared with age-matched controls, and circulating irisin concentrations were an independent predictor for BMD (113). Yan et al. demonstrated that, as an independent factor of bone density, irisin account for approximately 17.8% and 22.5% of femoral neck and lumbar spine BMD, respectively (114). In multiple linear regression, Anastasilakis et al. found that the risk of osteoporotic fracture and serum PTH levels were inversely correlated with irisin concentrations (110). Irisin was positively correlated with FNDC5 gene expression in muscle biopsies as well as Oc mRNA in bone biopsies, and FNDC5 positive fibers were closely linked to BMD of total femur and femoral neck, respectively (112). Furthermore, irisin directly affected the senescence marker p21, which was highly expressed in the bone biopsies of osteoporotic patients, by down-regulating the p21 mRNA expression in osteoblasts (112). Those data suggest that irisin is an independent factor for bone density, bone biomarkers, and the occurrence of fracture.
Irisin is involved in the neuroprotective effects of exercise against ischemic attack. Li and colleagues (115) reported that circulating irisin concentrations and muscular FNDC5 protein levels reduced after ischemic attack, and its concentrations were negatively correlated to cerebral infarction size and the neurological function deficient scores. Interestingly, irisin levels in skeletal muscle increased after physical exercise, and 2-week exercise training significantly reduced brain infarction volume (115). This beneficial impact of exercise was weakened by irisin-blocking agents. Similarly, exercise also decreased TNFα and Il6 expressions in the peripheral infarct area of cerebral tissues in the middle cerebral artery occlusion mice, which was inhibited by administration of irisin antibody (115). Injecting 6-hydroxydopamine into the medial forebrain bundle (MFB) in rats induced impairments in behavioral and a remarked decrease in the expressions of Ppargc1a, Fndc5, and Bdnf mRNA in both the striatum and the hippocampus compared with the sedentary controlled rats, whereas those negative changes were reversed by 16-week exercise intervention (116). It is indicated that physical exercise can alleviate neurodegeneration and learning deficiency by promoting the cerebral expression of certain effective and relevant factors.
Increasing evidence from in vivo and ex vivo experimental animal models has suggested that the favorable impact of exercise on memory and neural plasticity is mediated by irisin. A daily swimming programme (1h per day, 5days per week for 5weeks) protected mice from amyloid-β oligomers (AβOs)-induced memory impairment and decrease in the expressions of Fndc5 mRNA and irisin protein in mouse hippocampus (13). However, exercise-induced improvement of LTP was inhibited by intracerebroventricular injection of a lentiviral vector harboring Fndc5 shRNA (13). More intriguingly, intraperitoneal injection of FNDC5-inhibiting antibody blocked the neuroprotective effects of exercise against the synaptic plasticity and memory damage induced by AβOs. Similarly, exercise protected against the reduction of hippocampal Fndc5 expression induced by AβOs, but the positive effects were blocked by peripheral administration of anti-FNDC5 (13). Additionally, elevating serum irisin levels via overexpression of irisin in liver via adenoviral vectors led to increment in cerebral expression of irisin sufficient to protect against cognitive impairment and neural pathology in AD mice (117). Those data suggest that circulating irisin could cross the BBB and modulate the neural protective effects of exercise on neural plasticity and memory in AD.
Exercise-related improvement of brain function is linked to the irisin-induced Bdnf expression. Choi et al. demonstrated that the exercise-stimulated neurogenesis in adult hippocampus was correlated with the increment of cerebral Fndc5 and Bdnf expressions, subsequently promoting cognitive function in AD mice (118). As one of target genes of Ppargc1a, the expression of Fndc5 is regulated by Ppargc1a. Wrann et al. (52) reported that exercise induced increased Ppargc1a expression leading to increment in hippocampal Fndc5 gene expression in mice. Subsequently, the changes in Fndc5 gene expression affect Bdnf expression; overexpressing Fndc5 in primary cortical neurons stimulated increment of BDNF levels, whereas KO of Fndc5 via siRNA led to decrease in Bdnf expression. Moreover, adenoviral delivery of FNDC5 to liver led to elevation of circulating irisin and increased hippocampal Bdnf gene (52). Those data suggest the existence of muscle–brain crosstalk during exercise, which is regulated partly through the activation of PGC1α/FNDC5/BDNF signaling pathway (Figure 2).
Figure 2 Irisin regulating the communications between organs. Exercise upregulates the expression of peroxisome proliferator-activated receptor γ coactivator 1α (PGC1α) in skeletal muscle in mice and humans by upregulating Sirtuin 1 (SIRT1). Ppargc1a expression in muscle induces an increased expression of fibronectin type III domain-containing protein 5 (FNDC5), which is released as irisin into the circulation after cleavage. Some organs such as brain and heart also produce irisin in response to exercise. Peripherally treated recombinant irisin (r-irisin) can enter multiple organs and exert physiological effects. Irisin can lead to browning of white adipose tissue (WAT) increasing energy expenditure. In brain, irisin upregulates brain-derived neurotrophic factor (BDNF) expression and increase the plasticity of dendrite. Irisin promotes osteoblast differentiation by decreasing sclerostin (SOST) signalings. In liver, irisin decreases gluconeogenesis through adenosine monophosphate activated protein kinase (AMPK) signaling and increases glycogenesis via phosphatidylinositol 3-kinases (PI3K)/protein kinase B (AKT) pathways. Irisin also decreases inflammation, mitigates cardiac hypotrophy and fibrosis, and increase vasorelaxation. BAT, brown adipose tissues. BBB, brain-blood barrier. ROS, reactive oxygen species. NF-κB, nuclear factor kappa B. eNOS, endothelial NO synthase.
As an energy metabolism modulator, irisin is engaged in the impact of exercise on insulin action and glucose metabolism. Six-week treadmill training significantly increased serum concentrations of irisin and decreased serum insulin and glucose levels in mice (119). Serum irisin concentrations were negatively correlated to homeostatic model assessment of insulin resistance (HOMA-IR) (119). Korkmaz et al. (120) determined the relationship between irisin and glucose metabolism in exercised overweight/obese subjects (n = 144) with impairment of glucose regulation. Compared to the sedentary group, a program of aerobic type Nordic walking showed elevated plasma irisin concentrations. Training-related changes in muscular Fndc5 gene were negatively associated with the change in HB-A1c-IR, and circulating irisin levels were positively correlated with insulin concentrations following a 2-hour oral glucose tolerance test (120).
More interestingly, secretion of irisin during exercise might be up-regulated by cold exposure to increase brown fat thermogenesis. Ulupinar et al. (121) showed that aerobic exercise in a cold environment induced greater irisin secretion. Lee et al. (122) reported that cold exposure significantly elevated circulating irisin levels and irisin increment was proportional to shivering magnitude, similar to exercise-induced secretion. The authors pointed out that exercise-stimulated irisin secretion might represent a thermogenic, cold-activated endocrine axis, serving to augment brown fat thermogenesis (122). Those findings suggested that the combination of cold exposure with exercise increased irisin secretion to promote energy expenditure.
The ability of exercise increasing glucose uptake in skeletal muscles is regulated by increasing expression of glucose transporter 4 (GLUT4) (123). In a programme of 45-minute treadmill running (30 m/min, 15% grade), the levels of Glut4 gene expressed on the membrane of skeletal muscle cells in rats increased by 2.5 times (124). GLUT4 is one of the most essential glucose transporters, and its action is closely correlated to insulin action (125). Exercise increases the content and trafficking of GLUT4 in muscle cells in part through up-regulating irisin production. Eight-week interventions of high-intensity interval training (HIIT) or moderate-intensity continuous training (MICT) remarkably increased the expression of Fndc5 gene in the soleus muscle of rats (126). In the meantime, the Glut4 gene expression in soleus muscle were also remarkably increased, and MICT rats exhibited greater effects than those of HIIT rats (126). The capacity of irisin up-regulating Glut4 expression in muscle is assumed partly via activating p38/mitogen-activated protein kinase (MAPK) signalings. Exercise significantly up-regulated the expressions of Ppargc1a mRNA and Fndc5 mRNA in murine skeletal muscle (46, 127), and simultaneously p38 and Mapk mRNA levels were also markedly elevated (128). Irisin can promote the translocation of GLUT4 to the muscle cell membrane by phosphorylation of p38/MAPK, and subsequently increase up-taking glucose via skeletal muscle (129). Taken together, it is suggested that exercise improves insulin resistance partly via activating irisin/p38/MAPK/GLUT4 signaling pathway (Figure 2).
Exercise is a strong stimulator for the production of FNDC5/irisin in cardiac tissue (130–132), and the exercise-induced changes in irisin are associated with heart diseases (10, 133). Aydin et al. reported that irisin is highly presented in myocardium of both sedentary and exercise young rats, and it increased particularly in the connective tissues of heart after acute exercise (134). In accordance with this, irisin concentrations increased both in the supernatants from cardiac tissues of exercised rats and in circulation after exercise intervention (134). Serum irisin levels significantly increased in infarct rats received exercise training compared with those without exercise intervention (44.28 ± 2.20 vs. 30.70 ± 2.66 ng/mL) (135). Meanwhile, the levels of caspase-3 expression and the percentage of collagen deposition were increased in infarct rates whereas those negative outcomes were alleviated after exercise intervention. Furthermore, the changes in caspase-3 expression and collagen deposition were negatively correlated with serum concentrations of irisin (135). Twelve weeks of daily1-h treadmill training induced greater increase in serum irisin levels and murine cardiac function, including the ejection fraction (12%), fractional shortening (17%), cardiac output (29%), stroke volume (21%), end-diastole volume (34%), and end-systolic volume (58%) (136). Those data suggest that the exercise-stimulated irisin secretion helps to improve cardiac function and ameliorate the severity of heart diseases.
Exercises enhanced remarkably cardiac Fndc5 expression, and meantime PTEN-induced kinase 1 (PINK1)/Parkin pathway and mitophagy activity were also up-regulated which led to increased antioxidant function and improved cardiac function (137). PINK1/Parkin signaling is a key regulatory pathway for mediating mitophagy action, and the up-regulation of PINK1/Parkin activates mitophagy activity via stimulating mitochondrial microtubule-associated protein light chain 3 (LC3II/I) and SQSTM1/p62 (P62) pathway (138). Consequently, increased mitophagy activity helps to alleviate MI via improving hypoxia, metabolic disturbance, and cardiac dysfunction (139). It is indicated that exercise decreased oxidative stress and promoted heart function in part through triggering FNDC5/Irisin-PINK1/Pakin-LC3II/I-P62 signalings (Figure 2).
The efficacy of physical activity to increase bone health is due to the capacity of continuously generating mechanical loading upon bone tissues (140). Mechanical strain could be sensed by osteocytes and is transferred from physical stimuli into electronic signalings to regulate osteoblast and osteoclast activity. SOST, one master protein secreted by osteocytes, acts as an inhibitor of osteoblast differentiation via blocking Wnt/β-catenin signalings (141, 142). People who have more physical activities shows lower level of SOST, and regular exercise intervention induced a significant reduction of SOST level (143, 144). Circulating irisin and SOST are highly negatively correlated (145). Four-week treatment of r-irisin induced a significant increment in cortical BMD (7%), and the cortical bone contained higher level of Osn mRNA and lower level of Sost expression (99). In another study, Colaianni and colleagues (145) suspended the hindlimb of mice, which led to bone density reduction. Four-week unloading induced ~4% and ~40% reduction of cortical and trabecular BMD in femurs (145). Weekly administration of r-irisin (100µg/kg) protected against the cortical and trabecular bone loss via decreasing Sost expression and increasing osteoprotegerin expression (145) (Figure 2). However, Kim and colleagues (14) reported that r-irisin treatment up-regulated Sost expression both in vivo and ex vivo experiments. Given the fact that each dose (1 mg/kg per day) is nearly 10 times higher than the dose used by Colaianni and colleagues (100µg/kg per week), the amount of irisin and durations or frequencies of intervention may account for the controversies. Taken together, the current studies suggested the beneficial effects of exercise on bone metabolism partly via irisin/SOST pathway.
To date, compelling studies have suggested interesting roles for irisin, initially in muscle and adipose, and subsequently extending to bone, heart and brain. It is obvious that irisin lies on a center of the intercommunications between organs or tissues. As an “exercise hormone”, irisin promotes energy consumption by browning of white fat tissues during exercise. However, there remains some concerns needing to be addressed by future studies. Although αVβ5 integrin has been identified as the irisin receptor in osteocytes, adipocytes, and enterocytes (14, 146), it needs to be confirmed in other organs or tissues, such as brain, liver, heart, etc. Any progress in this field will improve our understanding of the signalings of irisin regulating the interactions between exercise and organs or tissues. However, the findings of some studies have been questioned for using questionable antibody-based assays; therefore, it will help improve the accuracy in determination of circulating irisin using the valid ones. Fortunately, in vivo or ex vivo exposure to irisin in cell culture or rodent models has provided a reliable approach to determine its physiological and pathological roles in health and diseases. The use of gain-of-function or loss-of-function models by injection of r-irisin or Fndc5 deletion provides a powerful tool to address controversies and to determine the mechanism of irisin-induced beneficial effects.
RZ reviewed the clinical evidence, performed the data synthesis, interpreted findings, wrote the manuscript, and led the work as a whole.
This work was supported by Natural Science Foundation of Jiangsu Province (BK20201435). The funder has no role in writing manuscript and determining the submission of manuscript.
The author declares that the research was conducted in the absence of any commercial or financial relationships that could be construed as a potential conflict of interest.
All claims expressed in this article are solely those of the authors and do not necessarily represent those of their affiliated organizations, or those of the publisher, the editors and the reviewers. Any product that may be evaluated in this article, or claim that may be made by its manufacturer, is not guaranteed or endorsed by the publisher.
1. Pedersen BK. Physical activity and muscle-brain crosstalk. Nat Rev Endocrinol (2019) 15(7):383–92. doi: 10.1038/s41574-019-0174-x
2. Pedersen BK, Febbraio MA. Muscles, exercise and obesity: skeletal muscle as a secretory organ. Nat Rev Endocrinol (2012) 8(8):457–65. doi: 10.1038/nrendo.2012.49
3. Karsenty G, Eric N. Olson: Bone and muscle endocrine functions: Unexpected paradigms of inter-organ communication. Cell (2016) 164(6):1248–56. doi: 10.1016/j.cell.2016.02.043
4. Fukumoto S, Martin TJ. Bone as an endocrine organ. Trends Endocrinol Metab (2009) 20(5):230–6. doi: 10.1016/j.tem.2009.02.001
5. Bostrom P, Wu J, Jedrychowski MP, Korde A, Ye L, Lo JC, et al. A PGC1-alpha-dependent myokine that drives brown-fat-like development of white fat and thermogenesis. Nature (2012) 481(7382):463–8. doi: 10.1038/nature10777
6. Kwak HB. Exercise and obesity-induced insulin resistance in skeletal muscle. Integr Med Res (2013) 2(4):131–8. doi: 10.1016/j.imr.2013.09.004
7. Brotto M, Isaacson J, Abreu EL. The muscle-bone connection. In: Duque G, Kiel D. editors Osteoporosis Older Persons. (2016) Cham: Springer (2016).
8. Karsenty G, Mera P. Molecular bases of the crosstalk between bone and muscle. Bone (2018) 115:43–9. doi: 10.1016/j.bone.2017.04.006
9. Delezie J, Handschin C. Endocrine crosstalk between skeletal muscle and the brain. Front Neurol (2018) 9:698. doi: 10.3389/fneur.2018.00698
10. Ma C, Ding H, Deng Y, Liu H, Xiong X, Yang Y. Irisin: A new code uncover the relationship of skeletal muscle and cardiovascular health during exercise. Front Physiol (2021) 12:620608. doi: 10.3389/fphys.2021.620608
11. Maak S, Norheim F, Drevon CA, Erickson HP. Progress and challenges in the biology of FNDC5 and irisin. Endocrine Rev (2021) 42(4):436–56. doi: 10.1210/endrev/bnab003
12. Perakakis N, Triantafyllou GA, Fernandez-Real JM, Huh JY, Park KH, Seufert J, et al. Physiology and role of irisin in glucose homeostasis. Nat Rev Endocrinol (2017) 13(6):324–37. doi: 10.1038/nrendo.2016.221
13. Lourenco MV, Frozza RL, de Freitas GB, Zhang H, Kincheski GC, Ribeiro FC, et al. Exercise-linked FNDC5/irisin rescues synaptic plasticity and memory defects in alzheimer's models. Nat Med (2019) 25(1):165–75. doi: 10.1038/s41591-018-0275-4
14. Kim H, Wrann CD, Jedrychowski M, Vidoni S, Kitase Y, Nagano K, et al. Irisin mediates effects on bone and fat via alphaV integrin receptors. Cell (2018) 175(7):1756–1768 e17. doi: 10.1016/j.cell.2018.10.025
15. Colaianni G, Sanesi L, Storlino G, Brunetti G, Colucci S, Grano M. Irisin and bone: From preclinical studies to the evaluation of its circulating levels in different populations of human subjects. Cells (2019) 8(5):451. doi: 10.3390/cells8050451
16. Pinkowska A, Podhorska-Okołów M, Dzięgiel P, Nowińska K. The role of irisin in cancer disease. Cells (2021) 10(6):1479. doi: 10.3390/cells10061479
17. Ferrer-Martinez A, Ruiz-Lozano P, Chien KR. Mouse PeP: a novel peroxisomal protein linked to myoblast differentiation and development. Dev Dyn (2002) 224(2):154–67. doi: 10.1002/dvdy.10099
18. Teufel A, Malik N, Mukhopadhyay M, Westphal H. Frcp1 and Frcp2, two novel fibronectin type III repeat containing genes. Gene (2002) 297(1-2):79–83. doi: 10.1016/s0378-1119(02)00828-4
19. Huh JY, Dincer F, Mesfum E, Mantzoros CS. Irisin stimulates muscle growth-related genes and regulates adipocyte differentiation and metabolism in humans. Int J Obes (Lond) (2014) 38(12):1538–44. doi: 10.1038/ijo.2014.42
20. Frühbeck G, Fernández-Quintana B, Paniagua M, Hernández-Pardos AW, Valentí V, Moncada R, et al. FNDC4, a novel adipokine that reduces lipogenesis and promotes fat browning in human visceral adipocytes. Metabolism (2020) 108:154261. doi: 10.1016/j.metabol.2020.154261
21. Schumacher MA, Chinnam N, Ohashi T, Shah RS, Erickson HP. The structure of irisin reveals a novel intersubunit beta-sheet fibronectin type III (FNIII) dimer: implications for receptor activation. J Biol Chem (2013) 288(47):33738–44. doi: 10.1074/jbc.M113.516641
22. Albrecht E, Norheim F, Thiede B, Holen T, Ohashi T, Schering L, et al. Irisin - a myth rather than an exercise-inducible myokine. Sci Rep (2015) 5:8889. doi: 10.1038/srep08889
23. Polyzos SA, Mathew H, Mantzoros CS. Irisin: A true, circulating hormone. Metabolism (2015) 64(12):1611–8. doi: 10.1016/j.metabol.2015.09.001
24. Jedrychowski MP, Wrann CD, Paulo JA, Gerber KK, Szpyt J, Robinson MM, et al. Detection and quantitation of circulating human irisin by tandem mass spectrometry. Cell Metab (2015) 22(4):734–40. doi: 10.1016/j.cmet.2015.08.001
25. Loffler D, Muller U, Scheuermann K, Friebe D, Gesing J, Bielitz J, et al. Serum irisin levels are regulated by acute strenuous exercise. J Clin Endocrinol Metab (2015) 100(4):1289–99. doi: 10.1210/jc.2014-2932
26. Brenmoehl J, Albrecht E, Komolka K, Schering L, Langhammer M, Hoeflich A, et al. Irisin is elevated in skeletal muscle and serum of mice immediately after acute exercise. Int J Biol Sci (2014) 10(3):338–49. doi: 10.7150/ijbs.7972
27. Huh JY, Panagiotou G, Mougios V, Brinkoetter M, Vamvini MT, Schneider BE, et al. FNDC5 and irisin in humans: I. predictors of circulating concentrations in serum and plasma and II. mRNA expression and circulating concentrations in response to weight loss and exercise. Metabolism (2012) 61(12):1725–38. doi: 10.1016/j.metabol.2012.09.002
28. De Meneck F, Victorino de Souza L, Oliveira V, do Franco MC. High irisin levels in overweight/obese children and its positive correlation with metabolic profile, blood pressure, and endothelial progenitor cells. Nutr Metab Cardiovasc Dis (2018) 28(7):756–64. doi: 10.1016/j.numecd.2018.04.009
29. El-Mottaleb Abd NA, Galal HM, El Maghraby KM, Gadallah AI. Serum irisin level in myocardial infarction patients with or without heart failure. Can J Physiol Pharmacol (2019) 97(10):932–8. doi: 10.1139/cjpp-2018-0736
30. Choi YK, Kim MK, Bae KH, Seo HA, Jeong JY, Lee WK, et al. Serum irisin levels in new-onset type 2 diabetes. Diabetes Res Clin Pract (2013) 100(1):96–101. doi: 10.1016/j.diabres.2013.01.007
31. Zhang ZP, Zhang XF, Li H, Liu TJ, Zhao QP, Huang LH, et al. Serum irisin associates with breast cancer to spinal metastasis. Med (Baltimore) (2018) 97(17):e0524. doi: 10.1097/MD.0000000000010524
32. Tang L, Tong Y, Zhang F, Chen G, Zhang YC, Jobin J, et al. The association of circulating irisin with metabolic risk factors in Chinese adults: a cross-sectional community-based study. BMC Endocr Disord (2019) 19(1):147. doi: 10.1186/s12902-019-0479-8
33. Albrecht E, Schering L, Buck F, Vlach K, Schober HC, Drevon CA, et al. Irisin: Still chasing shadows. Mol Metab (2020) 34:124–35. doi: 10.1016/j.molmet.2020.01.016
34. Aydin S, Kuloglu T, Ozercan MR, Albayrak S, Aydin S, Bakal U, et al. Irisin immunohistochemistry in gastrointestinal system cancers. Biotech Histochem (2016) 91(4):242–50. doi: 10.3109/10520295.2015.1136988
35. Dun SL, Lyu RM, Chen YH, Chang JK, Luo JJ, Dun NJ. Irisin-immunoreactivity in neural and non-neural cells of the rodent. Neuroscience (2013) 240:155–62. doi: 10.1016/j.neuroscience.2013.02.050
36. Gencer Tarakci B, Girgin A, Timurkaan S, Yalcin MH, Gur FM, Karan M. Immunohistochemical localization of irisin in skin, eye, and thyroid and pineal glands of the crested porcupine (Hystrix cristata). Biotech Histochem (2016) 91(6):423–7. doi: 10.1080/10520295.2016.1183820
37. Park MJ, Kim DI, Choi JH, Heo YR, Park SH. New role of irisin in hepatocytes: The protective effect of hepatic steatosis in vitro. Cell Signal (2015) 27(9):1831–9. doi: 10.1016/j.cellsig.2015.04.010
38. Tastekin E, Palabiyik O, Ulucam E, Uzgur S, Karaca A, Vardar SA, et al. The effect of high protein diet and exercise on irisin, eNOS, and iNOS expressions in kidney. Ren Fail (2016) 38(7):1107–14. doi: 10.1080/0886022X.2016.1194163
39. Aydin S, Kuloglu T, Aydin S, Kalayci M, Yilmaz M, Cakmak T, et al. A comprehensive immunohistochemical examination of the distribution of the fat-burning protein irisin in biological tissues. Peptides (2014) 61:130–6. doi: 10.1016/j.peptides.2014.09.014
40. Piya MK, Harte AL, Sivakumar K, Tripathi G, Voyias PD, James S, et al. The identification of irisin in human cerebrospinal fluid: influence of adiposity, metabolic markers, and gestational diabetes. Am J Physiol Endocrinol Metab (2014) 306(5):E512–8. doi: 10.1152/ajpendo.00308.2013
41. Bakal U, Aydin S, Sarac M, Kuloglu T, Kalayci M, Artas G, et al. Serum, saliva, and urine irisin with and without acute appendicitis and abdominal pain. Biochem Insights (2016) 9:11–7. doi: 10.4137/BCI.S39671
42. Kuloglu T, Celik O, Aydin S, Hanifi Ozercan I, Acet M, Aydin Y, et al. Irisin immunostaining characteristics of breast and ovarian cancer cells. Cell Mol Biol (Noisy-le-grand)(2016) 62(8)40–4. doi: 10.14715/cmb/2016.62.8.7
43. Kurdiova T, Balaz M, Vician M, Maderova D, Vlcek M, Valkovic L, et al. Effects of obesity, diabetes and exercise on Fndc5 gene expression and irisin release in human skeletal muscle and adipose tissue: in vivo and in vitro studies. J Physiol (2014) 592(5):1091–107. doi: 10.1113/jphysiol.2013.264655
44. Moreno-Navarrete JM, Ortega F, Serrano M, Guerra E, Pardo G, Tinahones F, et al. Irisin is expressed and produced by human muscle and adipose tissue in association with obesity and insulin resistance. J Clin Endocrinol Metab (2013) 98(4):E769–78. doi: 10.1210/jc.2012-2749
45. Novelle MG, Contreras C, Romero-Pico A, Lopez M, Dieguez C. Irisin, two years later. Int J Endocrinol (2013) 2013:746281. doi: 10.1155/2013/746281
46. Norheim F, Langleite TM, Hjorth M, Holen T, Kielland A, Stadheim HK, et al. The effects of acute and chronic exercise on PGC-1alpha, irisin and browning of subcutaneous adipose tissue in humans. FEBS J (2014) 281(3):739–49. doi: 10.1111/febs.12619
47. Roca-Rivada A, Castelao C, Senin LL, Landrove MO, Baltar J, Belen Crujeiras A, et al. FNDC5/irisin is not only a myokine but also an adipokine. PloS One (2013) 8(4):e60563. doi: 10.1371/journal.pone.0060563
48. Di Liegro CM, Schiera G, Proia P, Di Liegro I. Physical activity and brain health. Genes (2019) 10(9):720. doi: 10.3390/genes10090720
49. Liu-Ambrose T, Barha CK, Best JR. Physical activity for brain health in older adults. Appl Physiol Nutr Metab (2018) 43(11):1105–12. doi: 10.1139/apnm-2018-0260
50. Ahmadi Ghahrizjani F, Ghaedi K, Salamian A, Tanhaei S, Shoaraye Nejati A, Salehi H, et al. Enhanced expression of FNDC5 in human embryonic stem cell-derived neural cells along with relevant embryonic neural tissues. Gene (2015) 557(2):123–9. doi: 10.1016/j.gene.2014.12.010
51. Varela-Rodríguez BM, Pena-Bello L, Juiz-Valiña P, Vidal-Bretal B, Cordido F, Sangiao-Alvarellos S. FNDC5 expression and circulating irisin levels are modified by diet and hormonal conditions in hypothalamus, adipose tissue and muscle. Sci Rep (2016) 6(1):29898. doi: 10.1038/srep29898
52. Wrann CD, White JP, Salogiannnis J, Laznik-Bogoslavski D, Wu J, Ma D, et al. Exercise induces hippocampal BDNF through a PGC-1alpha/FNDC5 pathway. Cell Metab (2013) 18(5):649–59. doi: 10.1016/j.cmet.2013.09.008
53. Hashemi MS, Ghaedi K, Salamian A, Karbalaie K, Emadi-Baygi M, Tanhaei S, et al. Fndc5 knockdown significantly decreased neural differentiation rate of mouse embryonic stem cells. Neuroscience (2013) 231:296–304. doi: 10.1016/j.neuroscience.2012.11.041
54. Banks WA. Leptin transport across the blood-brain barrier: implications for the cause and treatment of obesity. Curr Pharm Des (2001) 7(2):125–33. doi: 10.2174/1381612013398310
55. El Hayek L, Khalifeh M, Zibara V, Abi Assaad R, Emmanuel N, Karnib N, et al. Lactate mediates the effects of exercise on learning and memory through SIRT1-dependent activation of hippocampal brain-derived neurotrophic factor (BDNF). J Neurosci (2019) 39(13):2369–82. doi: 10.1523/JNEUROSCI.1661-18.2019
56. Wang K, Li H, Wang H, Wang J-h, Song F, Sun Y. Irisin exerts neuroprotective effects on cultured neurons by regulating astrocytes. Mediators Inflammation (2018) 2018:1–7. doi: 10.1155/2018/9070341
57. Noda Y, Kuzuya A, Tanigawa K, Araki M, Kawai R, Ma B, et al. Fibronectin type III domain-containing protein 5 interacts with APP and decreases amyloid β production in alzheimer’s disease. Mol Brain (2018) 11(1):61. doi: 10.1186/s13041-018-0401-8
58. Küster OC, Laptinskaya D, Fissler P, Schnack C, Zügel M, Nold V, et al. Novel blood-based biomarkers of cognition, stress, and physical or cognitive training in older adults at risk of dementia: Preliminary evidence for a role of BDNF, irisin, and the kynurenine pathway. J Alzheimer's Dis (2017) 59(3):1097–111. doi: 10.3233/jad-170447
59. Tsai C-L, Pai M-C. Circulating levels of irisin in obese individuals at genetic risk for alzheimer’s disease: Correlations with amyloid-β, metabolic, and neurocognitive indices. Behav Brain Res (2021) 400:113013. doi: 10.1016/j.bbr.2020.113013
60. Peng J, Deng X, Huang W, Yu J-h, Wang J-x, Wang J-p, et al. Irisin protects against neuronal injury induced by oxygen-glucose deprivation in part depends on the inhibition of ROS-NLRP3 inflammatory signaling pathway. Mol Immunol (2017) 91:185–94. doi: 10.1016/j.molimm.2017.09.014
61. Thygesen K, Alpert JS, Jaffe AS, Simoons ML, Chaitman BR, White HD. Third universal definition of myocardial infarction. Circulation (2012) 126(16):2020–35. doi: 10.1161/CIR.0b013e31826e1058
62. Anderson JL, Campion EW, Morrow DA. Acute myocardial infarction. New Engl J Med (2017) 376(21):2053–64. doi: 10.1056/NEJMra1606915
63. Writing Group M, Mozaffarian D, Benjamin EJ, Go AS, Arnett DK, Blaha MJ, et al. Heart disease and stroke statistics-2016 update: A report from the American heart association. Circulation (2016) 133(4):e38–360. doi: 10.1161/CIR.0000000000000350
64. Gur FM, Timurkaan S, Yalcin MH, Girgin A, Gencer Tarakci B. Immunohistochemical localization of irisin in mole rats (Spalax leucodon). Biotech Histochem (2017) 92(4):245–51. doi: 10.1080/10520295.2017.1303194
65. Zhao YT, Wang J, Yano N, Zhang LX, Wang H, Zhang S, et al. Irisin promotes cardiac progenitor cell-induced myocardial repair and functional improvement in infarcted heart. J Cell Physiol (2019) 234(2):1671–81. doi: 10.1002/jcp.27037
66. Sundarrajan L, Yeung C, Hahn L, Weber LP, Unniappan S. Irisin regulates cardiac physiology in zebrafish. PloS One (2017) 12(8):e0181461. doi: 10.1371/journal.pone.0181461
67. Kuloglu T, Aydin S, Eren MN, Yilmaz M, Sahin I, Kalayci M, et al. Irisin: a potentially candidate marker for myocardial infarction. Peptides (2014) 55:85–91. doi: 10.1016/j.peptides.2014.02.008
68. Matsuo Y, Gleitsmann K, Mangner N, Werner S, Fischer T, Bowen TS, et al. Fibronectin type III domain containing 5 expression in skeletal muscle in chronic heart failure-relevance of inflammatory cytokines. J Cachexia Sarcopenia Muscle (2015) 6(1):62–72. doi: 10.1002/jcsm.12006
69. Abd El-Mottaleb NA, Galal HM, El Maghraby KM, Gadallah AI. Serum irisin level in myocardial infarction patients with or without heart failure. Can J Physiol Pharmacol (2019) 97(10):932–8. doi: 10.1139/cjpp-2018-0736
70. Aydin S, Aydin S, Kobat MA, Kalayci M, Eren MN, Yilmaz M, et al. Decreased saliva/serum irisin concentrations in the acute myocardial infarction promising for being a new candidate biomarker for diagnosis of this pathology. Peptides (2014) 56:141–5. doi: 10.1016/j.peptides.2014.04.002
71. Li RL, Wu SS, Wu Y, Wang XX, Chen HY, Xin JJ, et al. Irisin alleviates pressure overload-induced cardiac hypertrophy by inducing protective autophagy via mTOR-independent activation of the AMPK-ULK1 pathway. J Mol Cell Cardiol (2018) 121:242–55. doi: 10.1016/j.yjmcc.2018.07.250
72. Wang Z, Chen K, Han Y, Zhu H, Zhou X, Tan T, et al. Irisin protects heart against ischemia-reperfusion injury through a SOD2-dependent mitochondria mechanism. J Cardiovasc Pharmacol (2018) 72(6):259–69. doi: 10.1097/FJC.0000000000000608
73. Liao Q, Qu S, Tang LX, Li LP, He DF, Zeng CY, et al. Irisin exerts a therapeutic effect against myocardial infarction via promoting angiogenesis. Acta Pharmacol Sin (2019) 40(10):1314–21. doi: 10.1038/s41401-019-0230-z
74. Zhao YT, Wang H, Zhang S, Du J, Zhuang S, Zhao TC. Irisin ameliorates Hypoxia/Reoxygenation-induced injury through modulation of histone deacetylase 4. PloS One (2016) 11(11):e0166182. doi: 10.1371/journal.pone.0166182
75. Xin T, Lu C. Irisin activates Opa1-induced mitophagy to protect cardiomyocytes against apoptosis following myocardial infarction. Aging (2020) 12(5):4474–88. doi: 10.18632/aging.102899
76. Wang H, Zhao YT, Zhang S, Dubielecka PM, Du J, Yano N, et al. Irisin plays a pivotal role to protect the heart against ischemia and reperfusion injury. J Cell Physiol (2017) 232(12):3775–85. doi: 10.1002/jcp.25857
77. Schiattarella GG, Hill JA. Inhibition of hypertrophy is a good therapeutic strategy in ventricular pressure overload. Circulation (2015) 131(16):1435–47. doi: 10.1161/CIRCULATIONAHA.115.013894
78. Nakamura M, Sadoshima J. Mechanisms of physiological and pathological cardiac hypertrophy. Nat Rev Cardiol (2018) 15(7):387–407. doi: 10.1038/s41569-018-0007-y
79. Yu Q, Kou W, Xu X, Zhou S, Luan P, Xu X, et al. FNDC5/Irisin inhibits pathological cardiac hypertrophy. Clin Sci (2019) 133(5):611–27. doi: 10.1042/cs20190016
80. Geng Z, Fan W-Y, Zhou B, Ye C, Tong Y, Zhou Y-B, et al. FNDC5 attenuates obesity-induced cardiac hypertrophy by inactivating JAK2/STAT3-associated inflammation and oxidative stress. J Trans Med (2019) 17(1):107. doi: 10.1186/s12967-019-1857-8
81. Peng Q, Ding R, Wang X, Yang P, Jiang F, Chen X. Effect of irisin on pressure overload–induced cardiac remodeling. Arch Med Res (2021) 52(2):182–90. doi: 10.1016/j.arcmed.2020.10.006
82. Zhao Y, Xu Y, Peng W. Abstract 298: Irisin is an endogenous negative regulator of pathological cardiac hypertrophy. Circ Res (2018) 123(Suppl):A298. doi: 10.1161/res.123.suppl_1.298
83. Nordlie RC, Foster JD, Lange AJ. Regulation of glucose production by the liver. Annu Rev Nutr (1999) 19:379–406. doi: 10.1146/annurev.nutr.19.1.379
84. Moller DE, Kaufman KD. Metabolic syndrome: a clinical and molecular perspective. Annu Rev Med (2005) 56:45–62. doi: 10.1146/annurev.med.56.082103.104751
85. Liu TY, Shi CX, Gao R, Sun HJ, Xiong XQ, Ding L, et al. Irisin inhibits hepatic gluconeogenesis and increases glycogen synthesis via the PI3K/Akt pathway in type 2 diabetic mice and hepatocytes. Clin Sci (Lond) (2015) 129(10):839–50. doi: 10.1042/CS20150009
86. Xin C, Liu J, Zhang J, Zhu D, Wang H, Xiong L, et al. Irisin improves fatty acid oxidation and glucose utilization in type 2 diabetes by regulating the AMPK signaling pathway. Int J Obes (Lond) (2016) 40(3):443–51. doi: 10.1038/ijo.2015.199
87. Tang H, Yu R, Liu S, Huwatibieke B, Li Z, Zhang W. Irisin inhibits hepatic cholesterol synthesis via AMPK-SREBP2 signaling. EBioMedicine (2016) 6:139–48. doi: 10.1016/j.ebiom.2016.02.041
88. Mo L, Shen J, Liu Q, Zhang Y, Kuang J, Pu S, et al. Irisin is regulated by CAR in liver and is a mediator of hepatic glucose and lipid metabolism. Mol Endocrinol (2016) 30(5):533–42. doi: 10.1210/me.2015-1292
89. Mazur-Bialy AI, Bilski J, Pochec E, Brzozowski T. New insight into the direct anti-inflammatory activity of a myokine irisin against proinflammatory activation of adipocytes. implication for exercise in obesity. J Physiol Pharmacol (2017) 68(2):243–51.
90. Xiong XQ, Geng Z, Zhou B, Zhang F, Han Y, Zhou YB, et al. FNDC5 attenuates adipose tissue inflammation and insulin resistance via AMPK-mediated macrophage polarization in obesity. Metabolism (2018) 83:31–41. doi: 10.1016/j.metabol.2018.01.013
91. de Oliveira M, De Sibio MT, Mathias LS, Rodrigues BM, Sakalem ME, Nogueira CR. Irisin modulates genes associated with severe coronavirus disease (COVID-19) outcome in human subcutaneous adipocytes cell culture. Mol Cell Endocrinol (2020) 515:110917. doi: 10.1016/j.mce.2020.110917
92. Fruhbeck G, Catalan V, Valenti V, Moncada R, Gomez-Ambrosi J, Becerril S, et al. FNDC4 and FNDC5 reduce SARS-CoV-2 entry points and spike glycoprotein S1-induced pyroptosis, apoptosis, and necroptosis in human adipocytes. Cell Mol Immunol (2021) 18(10):2457–9. doi: 10.1038/s41423-021-00762-0
93. Bi J, Zhang J, Ren Y, Du Z, Li Q, Wang Y, et al. Irisin alleviates liver ischemia-reperfusion injury by inhibiting excessive mitochondrial fission, promoting mitochondrial biogenesis and decreasing oxidative stress. Redox Biol (2019) 20:296–306. doi: 10.1016/j.redox.2018.10.019
94. Bi J, Yang L, Wang T, Zhang J, Li T, Ren Y, et al. Irisin improves autophagy of aged hepatocytes via increasing telomerase activity in liver injury. Oxid Med Cell Longevity (2020) 2020:1–13. doi: 10.1155/2020/6946037
95. Gaggini M, Cabiati M, Del Turco S, Navarra T, De Simone P, Filipponi F, et al. Increased FNDC5/Irisin expression in human hepatocellular carcinoma. Peptides (2017) 88:62–6. doi: 10.1016/j.peptides.2016.12.014
96. Zhang J, Ke M, Ren Y, Bi J, Du Z, Zhang M, et al. Serum irisin predicts posthepatectomy complications in patients with hepatocellular carcinoma. Dis Markers (2019) 2019:1–7. doi: 10.1155/2019/9850191
97. Shi G, Tang N, Qiu J, Zhang D, Huang F, Cheng Y, et al. Irisin stimulates cell proliferation and invasion by targeting the PI3K/AKT pathway in human hepatocellular carcinoma. Biochem Biophys Res Commun (2017) 493(1):585–91. doi: 10.1016/j.bbrc.2017.08.148
98. Colaianni G, Mongelli T, Colucci S, Cinti S, Grano M. Crosstalk between muscle and bone Via the muscle-myokine irisin. Curr Osteoporosis Rep (2016) 14(4):132–7. doi: 10.1007/s11914-016-0313-4
99. Colaianni G, Cuscito C, Mongelli T, Pignataro P, Buccoliero C, Liu P, et al. The myokine irisin increases cortical bone mass. Proc Natl Acad Sci USA (2015) 112(39):12157–62. doi: 10.1073/pnas.1516622112
100. Levene NA, Varon D, Shtalrid M, Berrebi A. Chronic active hepatitis following splenectomy for autoimmune thrombocytopenia. Isr J Med Sci (1991) 27(4):199–201.
101. Luo Y, Qiao X, Ma Y, Deng H, Xu CC, Xu L. Disordered metabolism in mice lacking irisin. Sci Rep (2020) 10(1):17368. doi: 10.1038/s41598-020-74588-7
102. Zhu X, Li X, Wang X, Chen T, Tao F, Liu C, et al. Irisin deficiency disturbs bone metabolism. J Cell Physiol (2020) 236(1):664–76. doi: 10.1002/jcp.29894
103. Chen Z, Zhang Y, Zhao F, Yin C, Yang C, Wang X, et al. Recombinant irisin prevents the reduction of osteoblast differentiation induced by stimulated microgravity through increasing β-catenin expression. Int J Mol Sci (2020) 21(4):1259. doi: 10.3390/ijms21041259
104. Kawao N, Moritake A, Tatsumi K, Kaji H. Roles of irisin in the linkage from muscle to bone during mechanical unloading in mice. Calcified Tissue Int (2018) 103(1):24–34. doi: 10.1007/s00223-018-0387-3
105. Metzger CE, Anand Narayanan S, Phan PH, Bloomfield SA. Hindlimb unloading causes regional loading-dependent changes in osteocyte inflammatory cytokines that are modulated by exogenous irisin treatment. NPJ Microgravity (2020) 6:28. doi: 10.1038/s41526-020-00118-4
106. Xu L, Shen L, Yu X, Li P, Wang Q, Li C. Effects of irisin on osteoblast apoptosis and osteoporosis in postmenopausal osteoporosis rats through upregulating Nrf2 and inhibiting NLRP3 inflammasome. Exp Ther Med (2020) 19(2):1084–90. doi: 10.3892/etm.2019.8313
107. Narayanan SA, Metzger CE, Bloomfield SA, Zawieja DC. Inflammation-induced lymphatic architecture and bone turnover changes are ameliorated by irisin treatment in chronic inflammatory bowel disease. FASEB J (2018) 32(9):4848–61. doi: 10.1096/fj.201800178R
108. Metzger CE, Narayanan SA, Elizondo JP, Carter AM, Zawieja DC, Hogan HA, et al. DSS-induced colitis produces inflammation-induced bone loss while irisin treatment mitigates the inflammatory state in both gut and bone. Sci Rep (2019) 9(1):15144. doi: 10.1038/s41598-019-51550-w
109. Colaianni G, Faienza MF, Sanesi L, Brunetti G, Pignataro P, Lippo L, et al. Irisin serum levels are positively correlated with bone mineral status in a population of healthy children. Pediatr Res (2019) 85(4):484–8. doi: 10.1038/s41390-019-0278-y
110. Anastasilakis AD, Polyzos SA, Makras P, Gkiomisi A, Bisbinas I, Katsarou A, et al. Circulating irisin is associated with osteoporotic fractures in postmenopausal women with low bone mass but is not affected by either teriparatide or denosumab treatment for 3 months. Osteoporos Int (2014) 25(5):1633–42. doi: 10.1007/s00198-014-2673-x
111. Engin-Üstün Y, Çağlayan EK, Göçmen AY, Polat MF. Postmenopausal osteoporosis is associated with serum chemerin and irisin but not with apolipoprotein m levels. J Menopausal Med (2016) 22(2):76–9. doi: 10.6118/jmm.2016.22.2.76
112. Colaianni G, Errede M, Sanesi L, Notarnicola A, Celi M, Zerlotin R, et al. Irisin correlates positively with BMD in a cohort of older adult patients and downregulates the senescent marker p21 in osteoblasts. J Bone Miner Res (2021) 36(2):305–14. doi: 10.1002/jbmr.4192
113. Zhang J, Huang X, Yu R, Wang Y, Gao C. Circulating irisin is linked to bone mineral density in geriatric Chinese men. Open Med (Wars) (2020) 15(1):763–8. doi: 10.1515/med-2020-0215
114. Yan J, Liu HJ, Guo WC, Yang J. Low serum concentrations of irisin are associated with increased risk of hip fracture in Chinese older women. Joint Bone Spine (2018) 85(3):353–8. doi: 10.1016/j.jbspin.2017.03.011
115. Li DJ, Li YH, Yuan HB, Qu LF, Wang P. The novel exercise-induced hormone irisin protects against neuronal injury via activation of the akt and ERK1/2 signaling pathways and contributes to the neuroprotection of physical exercise in cerebral ischemia. Metabolism (2017) 68:31–42. doi: 10.1016/j.metabol.2016.12.003
116. Rezaee Z, Marandi SM, Alaei H, Esfarjani F. The effect of preventive exercise on the neuroprotection in 6-hydroxydopamine-lesioned rat brain. Appl Physiology Nutrition Metab (2019) 44(12):1267–75. doi: 10.1139/apnm-2018-0545
117. Islam MR, Valaris S, Young MF, Haley EB, Luo R, Bond SF, et al. Exercise hormone irisin is a critical regulator of cognitive function. Nat Metab (2021) 3(8):1058–70. doi: 10.1038/s42255-021-00438-z
118. Choi SH, Bylykbashi E, Chatila ZK, Lee SW, Pulli B, Clemenson GD, et al. Combined adult neurogenesis and BDNF mimic exercise effects on cognition in an alzheimer's mouse model. Science (2018) 361(6406):eaan8821. doi: 10.1126/science.aan8821
119. Bastu E, Zeybek U, Gurel Gurevin E, Yüksel Ozgor B, Celik F, Okumus N, et al. Effects of irisin and exercise on metabolic parameters and reproductive hormone levels in high-fat diet-induced obese female mice. Reprod Sci (2017) 25(2):281–91. doi: 10.1177/1933719117711264
120. Korkmaz A, Venojärvi M, Wasenius N, Manderoos S, Deruisseau KC, Gidlund E-K, et al. Plasma irisin is increased following 12 weeks of Nordic walking and associates with glucose homoeostasis in overweight/obese men with impaired glucose regulation. Eur J Sport Sci (2018) 19(2):258–66. doi: 10.1080/17461391.2018.1506504
121. Ulupinar S, Ozbay S, Gencoglu C, Altinkaynak K, Sebin E, Oymak B. Exercise in the cold causes greater irisin release but may not be enough for adropin. Chin J Physiol (2021) 64(3):129–34. doi: 10.4103/cjp.cjp_2_21
122. Lee P, Linderman JD, Smith S, Brychta RJ, Wang J, Idelson C, et al. Irisin and FGF21 are cold-induced endocrine activators of brown fat function in humans. Cell Metab (2014) 19(2):302–9. doi: 10.1016/j.cmet.2013.12.017
123. Hussey SE, McGee SL, Garnham A, McConell GK, Hargreaves M. Exercise increases skeletal muscle GLUT4 gene expression in patients with type 2 diabetes. Diabetes Obes Metab (2012) 14(8):768–71. doi: 10.1111/j.1463-1326.2012.01585.x
124. Douen AG, Ramlal T, Rastogi S, Bilan PJ, Cartee GD, Vranic M, et al. Exercise induces recruitment of the "insulin-responsive glucose transporter". evidence for distinct intracellular insulin- and exercise-recruitable transporter pools in skeletal muscle. J Biol Chem (1990) 265(23):13427–30.
125. Moraes-Vieira PM, Saghatelian A, Kahn BB. GLUT4 expression in adipocytes regulates De novo lipogenesis and levels of a novel class of lipids with antidiabetic and anti-inflammatory effects. Diabetes (2016) 65(7):1808–15. doi: 10.2337/db16-0221
126. Rahmati-Ahmadabad S, Rostamkhani F, Meftahi GH, Shirvani H. Comparative effects of high-intensity interval training and moderate-intensity continuous training on soleus muscle fibronectin type III domain-containing protein 5, myonectin and glucose transporter type 4 gene expressions: a study on the diabetic rat model. Mol Biol Rep (2021) 48(8):6123–9. doi: 10.1007/s11033-021-06633-1
127. Cho E, Jeong DY, Kim JG, Lee S. The acute effects of swimming exercise on PGC-1alpha-FNDC5/Irisin-UCP1 expression in Male C57BL/6J mice. Metabolites (2021) 11(2):111. doi: 10.3390/metabo11020111
128. Ye X, Shen Y, Ni C, Ye J, Xin Y, Zhang W, et al. Irisin reverses insulin resistance in C2C12 cells via the p38-MAPK-PGC-1alpha pathway. Peptides (2019) 119:170120. doi: 10.1016/j.peptides.2019.170120
129. Xian HM, Che H, Qin Y, Yang F, Meng SY, Li XG, et al. Coriolus versicolor aqueous extract ameliorates insulin resistance with PI3K/Akt and p38 MAPK signaling pathways involved in diabetic skeletal muscle. Phytother Res (2018) 32(3):551–60. doi: 10.1002/ptr.6007
130. Belviranlı M, Okudan N. Exercise training increases cardiac, hepatic and circulating levels of brain-derived neurotrophic factor and irisin in young and aged rats. Hormone Mol Biol Clin Invest (2018) 36(3):20180053. doi: 10.1515/hmbci-2018-0053
131. Hosseinzadeh M, Rashid Lamir A, Hejazi SM. Are heart muscle irisin levels and FNDC5 gene expression regulated by endurance and resistance exercises? Ann Appl Sport Sci (2018) 6(1):21–8. doi: 10.29252/aassjournal.6.1.21
132. Hassaan PS, Nassar SZ, Issa Y, Zahran N. Irisin vs. treadmill exercise in post myocardial infarction cardiac rehabilitation in rats. Arch Med Res (2019) 50(2):44–54. doi: 10.1016/j.arcmed.2019.05.009
133. Ho M-Y, Wang C-Y. Role of irisin in myocardial infarction, heart failure, and cardiac hypertrophy. Cells (2021) 10(8):2103. doi: 10.3390/cells10082103
134. Aydin S, Kuloglu T, Aydin S, Eren MN, Celik A, Yilmaz M, et al. Cardiac, skeletal muscle and serum irisin responses to with or without water exercise in young and old male rats: Cardiac muscle produces more irisin than skeletal muscle. Peptides (2014) 52:68–73. doi: 10.1016/j.peptides.2013.11.024
135. Bashar SM, Samir El-sherbeiny SM, Boraie MZ. Correlation between the blood level of irisin and the severity of acute myocardial infarction in exercise-trained rats. J Basic Clin Physiol Pharmacol (2018) 30(1):59–71. doi: 10.1515/jbcpp-2018-0090
136. Seo DY, Bae JH, Kim TN, Kwak H-B, Kha PT, Han J. Exercise-induced circulating irisin level is correlated with improved cardiac function in rats. Int J Environ Res Public Health (2020) 17(11):3863. doi: 10.3390/ijerph17113863
137. Li H, Qin S, Liang Q, Xi Y, Bo W, Cai M, et al. Exercise training enhances myocardial mitophagy and improves cardiac function via Irisin/FNDC5-PINK1/Parkin pathway in MI mice. Biomedicines (2021) 9(6):701. doi: 10.3390/biomedicines9060701
138. Ivankovic D, Chau KY, Schapira AHV, Gegg ME. Mitochondrial and lysosomal biogenesis are activated following PINK 1/parkin-mediated mitophagy. J Neurochemistry (2015) 136(2):388–402. doi: 10.1111/jnc.13412
139. Madrigal-Matute J, Sciarretta S, Tsansizi L, Semenzato M, Sebti S, Saito T, et al. Comprehensive autophagy evaluation in cardiac disease models. Cardiovasc Res (2020) 116(3):483–504. doi: 10.1093/cvr/cvz233
140. Zhao R. Exercise protocols for osteoporosis. In: Zhao R, editor. Oteoporosis and exercise intevention: From molecules to clinic. Changchun: Jilin University Press (2021).
141. van Bezooijen RL, Roelen BAJ, Visser A, van der Wee-Pals L, de Wilt E, Karperien M, et al. Sclerostin is an osteocyte-expressed negative regulator of bone formation, but not a classical BMP antagonist. J Exp Med (2004) 199(6):805–14. doi: 10.1084/jem.20031454
142. Singh A, Gupta MK, Mishra SP. Study of correlation of level of expression of wnt signaling pathway inhibitors sclerostin and dickkopf-1 with disease activity and severity in rheumatoid arthritis patients. Drug Discov Ther (2019) 13(1):22–7. doi: 10.5582/ddt.2019.01011
143. Cheung AM, Giangregorio L. Mechanical stimuli and bone health. Curr Opin Rheumatol (2012) 24(5):561–6. doi: 10.1097/BOR.0b013e3283570238
144. Janik M, Stuss M, Michalska-Kasiczak M, Jegier A, Sewerynek E. Effects of physical activity on sclerostin concentrations. Endokrynologia Polska (2018) 69(2):142–9. doi: 10.5603/EP.a2018.0008
145. Colaianni G, Mongelli T, Cuscito C, Pignataro P, Lippo L, Spiro G, et al. Irisin prevents and restores bone loss and muscle atrophy in hind-limb suspended mice. Sci Rep (2017) 7(1):2811. doi: 10.1038/s41598-017-02557-8
Keywords: irisin, exercise, brain function, heart diseases, bone, liver
Citation: Zhao R (2022) Irisin at the crossroads of inter-organ communications: Challenge and implications. Front. Endocrinol. 13:989135. doi: 10.3389/fendo.2022.989135
Received: 08 July 2022; Accepted: 20 September 2022;
Published: 04 October 2022.
Edited by:
Rita De Matteis, University of Urbino Carlo Bo, ItalyReviewed by:
Amaia Rodríguez, University of Navarra, SpainCopyright © 2022 Zhao. This is an open-access article distributed under the terms of the Creative Commons Attribution License (CC BY). The use, distribution or reproduction in other forums is permitted, provided the original author(s) and the copyright owner(s) are credited and that the original publication in this journal is cited, in accordance with accepted academic practice. No use, distribution or reproduction is permitted which does not comply with these terms.
*Correspondence: Renqing Zhao, cmVucWluZy56aGFvQHl6dS5lZHUuY24=
Disclaimer: All claims expressed in this article are solely those of the authors and do not necessarily represent those of their affiliated organizations, or those of the publisher, the editors and the reviewers. Any product that may be evaluated in this article or claim that may be made by its manufacturer is not guaranteed or endorsed by the publisher.
Research integrity at Frontiers
Learn more about the work of our research integrity team to safeguard the quality of each article we publish.