- Department of Nephrology, Second Affiliated Hospital, Shantou University Medical College, Shantou, China
Diabetic kidney disease (DKD) is one of complications of diabetes mellitus with severe microvascular lesion and the most common cause of end-stage chronic kidney disease (ESRD). Controlling serum glucose remains the primary approach to preventing and slowing the progression of DKD. Despite considerable efforts to control diabetes, people with diabetes develop not only DKD but also ESRD. The pathogenesis of DKD is very complex, and current studies indicate that mesenchymal stromal cells (MSCs) regulate complex disease processes by promoting pro-regenerative mechanisms and inhibiting multiple pathogenic pathways. Extracellular vesicles (EVs) are products of MSCs. Current data indicate that MSC-EVs-based interventions not only protect renal cells, including renal tubular epithelial cells, podocytes and mesangial cells, but also improve renal function and reduce damage in diabetic animals. As an increasing number of clinical studies have confirmed, MSC-EVs may be an effective way to treat DKD. This review explores the potential efficacy and signaling pathways of MSC-EVs in the treatment of DKD.
Introduction
The global prevalence of diabetes is projected to rise from 9.3% in 2019 to 10.9% in 2045 (1). Diabetic kidney disease (DKD) is a serious complication caused by diabetes and occurs in 20-40% of people with diabetes (2, 3). Treatment of DKD has included blood pressure control with angiotensin receptor blockers or angiotensin-converting enzyme inhibitors and strict glycemic control (4). However, many patients still progress to the stage of end-stage renal disease (ESRD) (5). From 2000 to 2015, DKD as a percentage of chronic kidney disease increased from 22.1% to 31.3% (6) and is the most common cause of ESRD in many developed countries. When compared with other diabetic complications, the prevalence of DKD disease has not notably reduced over the past 20 years (7). There is an urgent need to develop effective therapeutic strategies to preserve the renal function and slow the progression of DKD.
Mesenchymal stromal cells (MSCs) are a group of cells with differentiation and proliferative potential (8). When treated with appropriate compounds, they can differentiate into cells of all mesodermal lineages, such as fibroblasts, myocytes, adipocytes, osteocytes, or chondrocytes (9, 10). Studies have shown that miR-124a can stimulate the differentiation of mesenchymal stem cells sourced bone marrow into islet-like cells and thus alleviate DKD (11). MSCs mainly used for DKD include adipose-derived (AD-MSCs), umbilical cord-derived (UC-MSCs), bone marrow-derived (BM-MSCs), and human urine-derived (HU-MSCs). MSCs protect the kidney in two ways. One is homing and differentiation, where MSCs recognize damaged tissue and then home and integrate into specific sites, and the other is through paracrine action. However, the main problem after direct administration of MSCs is that they do not target the target tissue. The infusion of MSCs via direct acute infusion or coupled with implanted continuous pumps directly into the kidney is the main way (12). After MSCs are injected into the tail vein of rats, most of the stem cells appear in the lungs (13). To determine whether MSCs exist in rat tissues, DNA was extracted from rat organs and a human Alu sequence was detected. Human Alu sequences were detected in the peritubular region, lung, and spleen in rats within 24 hours after injection of labeled MSCs, but rarely in the glomerulus and pancreas (14). Implanted continuous pumps directly into the kidney have the advantage of local tissue effect, but it is difficult to implement and difficult to popularize clinically. As only minimal numbers of donor MSCs are detected in the renal tissue, the therapeutic effect of MSCs on kidney injury appears to be attributable to lots of paracrine factors. These factors facilitate the renal repair by paracrine-mediated actions, including cell-cell interactions reactivating endogenous repair systems, and the release of extracellular vesicles (EVs) (15). This review explores the potential efficacy and signaling pathways of MSC-EVs in the treatment of DKD.
Characteristics of stem cell products
MSC-conditioned medium (MSC-CM) is rich in EVs secreted by MSCs. As stem cell products, EVs are generally classified into three categories: apoptotic bodies, microvesicles (MVs), and exosomes (Exos), which vary in size, origin, and release mechanism (16, 17). MVs are 50 nm-1000 nm in diameter and are shed directly from the cytoplasmic membrane (16, 18, 19). Their release is initiated by budding outward from the membrane surface (16, 19). Apoptotic bodies are released during apoptosis. The diameter of apoptotic vesicles is reported to range between 1000 nm and 5000 nm (20). The reported diameter of Exos is between 40 and 150 nm (21). Exos carry complex molecular cargoes such as proteins, lipids and nucleic acids (e.g., DNA, miRNA, circRNA) (Figure 1). A series of studies have shown that stem cell Exos protect the kidney from damage through multiple pathways involving anti-apoptotic, anti-inflammatory, anti-oxidative, anti-fibrotic roles and regulate podocyte autophagy (22, 23).
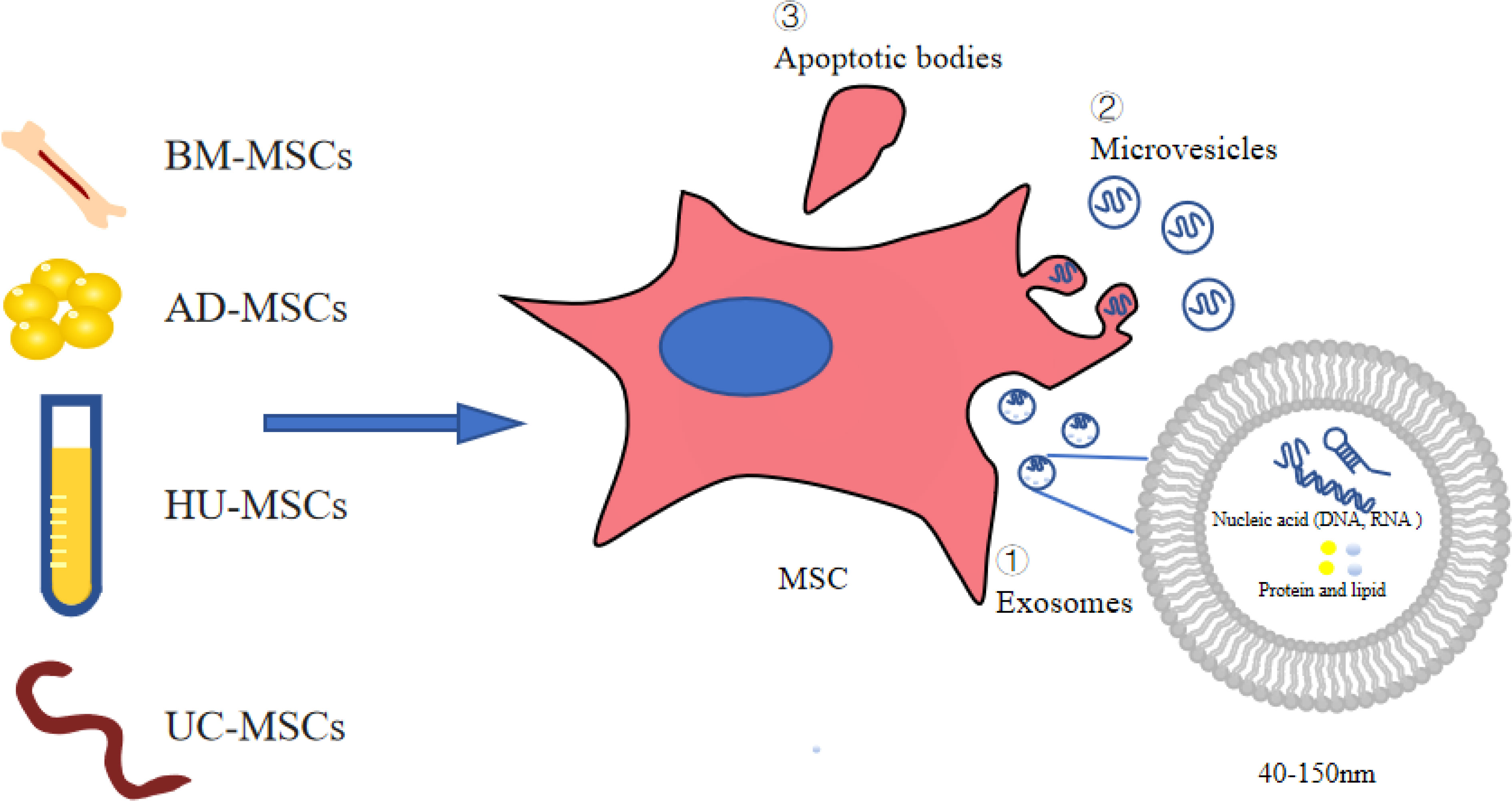
Figure 1 Extracellular vesicles derived from stem cells. AD-MSCs, adipose-derived MSCs; BM-MSCs, bone marrow-derived MSCs; UC-MSCs, umbilical cord-derived MSCs; HU-MSCs, human urine-derived MSCs; MSCs. mesenchymal stromal cells.
Amelioration of podocyte injury by stem cell products
Podocytes, important intrinsic cells of the glomerulus, are involved in protein filtration in the glomerulus and play an important role in the maintenance of kidney function (23, 24). Podocyte injury is an important characteristic of DKD. Podocyte loss contributes to the development of DKD (25). MSC-EVs are found that they can protect the podocytes in DKD in multiple ways, including inhibition of apoptosis and fibrosis and enhancement of autophagy, all perhaps due to the presence of a large number of growth factors and miRNAs in MSC-EVs (26).
High glucose (HG)-induced podocyte damage in vitro can mimic the state of podocytes in patients with DKD. Jiang et al. (27) conducted a study on human podocytes, and reported that HU-MSC-EVs reduced HG-induced podocyte apoptosis in vitro. UC-MSCs secrete EVs carrying bone morphogenetic protein-7 (BMP-7) and high levels of vascular endothelial growth factor (VEGF) (Table 1). The activation of cytokines such as BMP-7 or VEGF is important for the survival of podocyte. VEGFA is highly expressed in the podocytes and is required to maintain endothelial cell function (46). However, VEGFA is overexpressed in the early stage of DN, and blocking VEGFA reduces proteinuria in DN (47). Duan et al. (28) showed that silencing of VEGF attenuates podocyte inflammation and reduces apoptosis. Moreover, miR-16-5p encapsulated within HU-MSCs can inhibit VEGFA expression in podocytes induced by HG, promote podocyte viability and decrease the rate of apoptosis (Table 1). AD-MSC-EVs can transfer miR-26a-5p to mouse podocytes in vitro to inhibit podocyte apoptosis by downregulating nuclear factor kappa-B (NF-κB)/VEGFA and toll-like receptor 4 (TLR4) signaling pathways (29). Pyruvate dehydrogenase kinase 4 (PDK4) is a molecular target of miR-15b-5p (48). Zhao et al. (30) reported that AD-MSC-CM miR-15b-5p directly binds to PDK4 in podocytes from mouse and inhibits the expression of PDK4 mRNA and protein. Inhibition of PDK4 can reduce the activation of VEGFA and downregulate the inflammation and cell apoptosis (Table 1). The results of several studies showed that stem cell products inhibit VEGFA expression through multiple pathways, thereby attenuating HG-induced damage to podocytes.
Li et al. (31) reported that AD-MSC-CM reduced podocyte apoptosis induced by HG, downregulated activated caspase-3, increased epithelial growth factor (EGF), and prevented the rearrangement and downregulation of synaptopodin. However, it did not affect the levels of glial cell line-derived neurotrophic growth factor (GDNF), insulin-like growth factor binding protein and placental growth factor (Table 1). However, a study pointed out that GDNF promotes mouse podocyte survival in vitro and protects mouse podocytes from apoptosis (49). Zhang et al. (14) confirmed this and found that HG can reduce podocytic synaptopodin, and AD-MSC-CM can increase synaptopodin expression in podocytes. After blocking GDNF in AD-MSC-CM with GDNF-NtAb, the therapeutic effect of podocytic synaptopodin was partly abolished (Table 1). These studies demonstrated that stem cell products reduce podocytic apoptosis by modulating cytokines.
Autophagy is a lysosomal degradation pathway in cells for maintaining cellular homeostasis and cellular health under various stress conditions (50). Evidence suggests that podocytes have high levels of basal autophagy, which may be a mechanism to maintain cellular homeostasis (51). Jin et al. (32) showed that AD-MSC-EVs can inhibit p-mTOR/mTOR, Smad1, p62, and apoptosis, but they can increase Beclin1 and LC3 (Table 1). AD-MSC-EVs ameliorate podocyte damage by inhibiting the miR-486/Smad1/mTOR signaling pathway. This suggested that stem cell products can regulate the autophagy of podocytes.
HG may induce podocyte epithelial-mesenchymal transition (EMT) through multiple pathways (52). Jin et al. (33) showed that, with podocyte dysfunction, several EMT-related miRNAs, including miR-3066-5p, miR-879-5p, miR-251-5p, and miR-7a-5p, were increased by the addition of AD-MSC-EVs (Table 1). Potentially, AD-MSC-EVs can mediate the shuttling of miR-215-5p to podocytes, possibly through inhibiting the transcription of zinc finger E-box-binding homeobox 2 (ZEB2), thereby attenuating EMT of podocytes.
Stem cell products ameliorate fibrosis in mesangial cells
The signaling pathway of TGF-β can play an important role in the fibrogenesis, especially in DKD renal fibrosis, and can be activated by high glucose (53). Endothelin-1 (ET-1) promotes fibrosis and inflammation in DKD (54). ET-1 and TGF-β1 induce collagen I production by fibroblasts (55). Li et al. (34) found that blocking TGF-β1 by UC-CM inhibited the expression of collagen I and fibronectin in mesangial cells treated with HG. Antifibrotic effects of MSC paracrine in DN may be detected by EVs shed by MSCs. BM-MSC-CM treatment remarkably reduced expressions of TGF-β and TGF-β-induced glucose transporter 1, thus inhibiting fibrosis and oxidative stress. A large amount of hepatocyte growth factor (HGF) was detected in CM, and the effects of CM on TGF-β and TGF-β-induced glucose transporter expression could be blocked by the addition of neutralizing antibodies against HGF (Table 1) (35). This indicated that HGF in CM alleviates mesangial cells’ fibrosis and oxidative stress. Co-culture of BM-MSCs and mesangial cells alleviated cell fibrosis by targeting lipoxin A4 (LXA4) to modulate TGF-β/Smad signaling (36). STAT5A was identified as a transcriptional regulator of miR-21, which in turn affects collagen production and TGF-β expression in mesangial cells. MSC-EV-miR-222 regulates STAT5 expression and indirectly regulates TGF-β expression (37). Hao et al. (38) found that AD-MSC-EVs inhibit the histone deacetylase 1 (HDAC1)/ET1 axis by secreting miR-125a and suppressing IL6, collagen I and fibronectin levels in HG-treated mesangial cells. All these results suggested that MSC-EVs alleviate mesangial cell fibrosis by regulating the expression of TGF-β and ET-1 (Table 1).
Stem cell products ameliorate fibrosis in renal tubular epithelial cells
Renal tubulointerstitial fibrosis, characterized by EMT of renal tubular epithelial cells (RTEC), is a major cause of diabetic renal fibrosis (56). Important roles of different tubular responses, such as partial EMT, cell cycle arrest and metabolic defects are involved in renal fibrosis (57). Nagaishi et al. (39) found that MSC-EVs can reduce intracellular adhesion molecule-1 (ICAM-1) and TGF-β1 in RTEC isolated from streptozotocin (STZ)-induced diabetic rats, and zona occludens protein-1 (ZO-1) expression was increased in RTEC cultured with EVs or MSCs. UC-MSC-CM inhibited TGF-β1-induced EMT and extracellular matrix accumulation (ECM) in RTEC (NRK-52E) (Table 1) (40). Zhong et al. (41) further found that MSC-EV-miR-451a reverses EMT by inhibiting P15 and P19 to restart the blocked cell cycle (Table 1). Inhibition of fibrosis by regulating the cell cycle by miRNA may be a new therapeutic modality.
Advanced glycation end products (AGEs) induce apoptosis and increase the expression of inflammatory and fibrotic genes in renal tubular cells (58). Hyperglycemia-induced reactive oxygen species (ROS) generation activates mitogen-activated protein kinases (MAPKs), which are involved in the EMT of RTEC (HK2) (59, 60). Co-cultured stem cells from human exfoliated deciduous teeth inhibit AGE-induced EMT in HK-2 cells (Table 1) (42). Ali et al. (43) reported that the protein expression of Carboxyl terminus of HSP70 interacting protein (CHIP) was reduced under HG treatment, which can limit the therapeutic potential and survivability of Wharton’s jelly-derived MSCs (WJMSCs). CHIP-overexpressing WJMSCs attenuate fibrosis and cell apoptosis mediated by hyperglycemia-induced oxidative stress in HK-2 cells via activation of the MAPKs (Table 1). This proves that MSC-EVs can inhibit the production of AGEs and ROS and downregulate the fibrosis of renal tubular epithelial cells.
Mitochondria have been recognized as key regulators of inflammation, cell death, metabolism and ROS production (61). M1 macrophages express pro-inflammatory cytokines, and M2 macrophages are thought to regulate inflammatory responses and promote tissue repair (62). Arginase-1 (Arg1) is a marker of M2 macrophages, and co-culture of macrophages with MSCs increases Arg1 and decreases the expression of M1 markers. UC-MSCs reverse mitochondrial function in HK-2 cells by inducing Arg1 in macrophages (44). It should be noted that AD-MSCs cannot reverse mitochondrial dysfunction, which may indicate that the beneficial effect of MSCs is limited to umbilical cord blood. Konari et al. (45) showed that BM-MSCs also transfer their mitochondria to damaged RTEC when co-cultured in vitro, inhibiting apoptosis and reducing ROS production of damaged RTEC (Table 1).
MSC-EVs protect HG-induced damaged kidney cells through multiple pathways, suggesting that MSC-EVs may be a potential therapeutic modality for DKD (Figure 2).
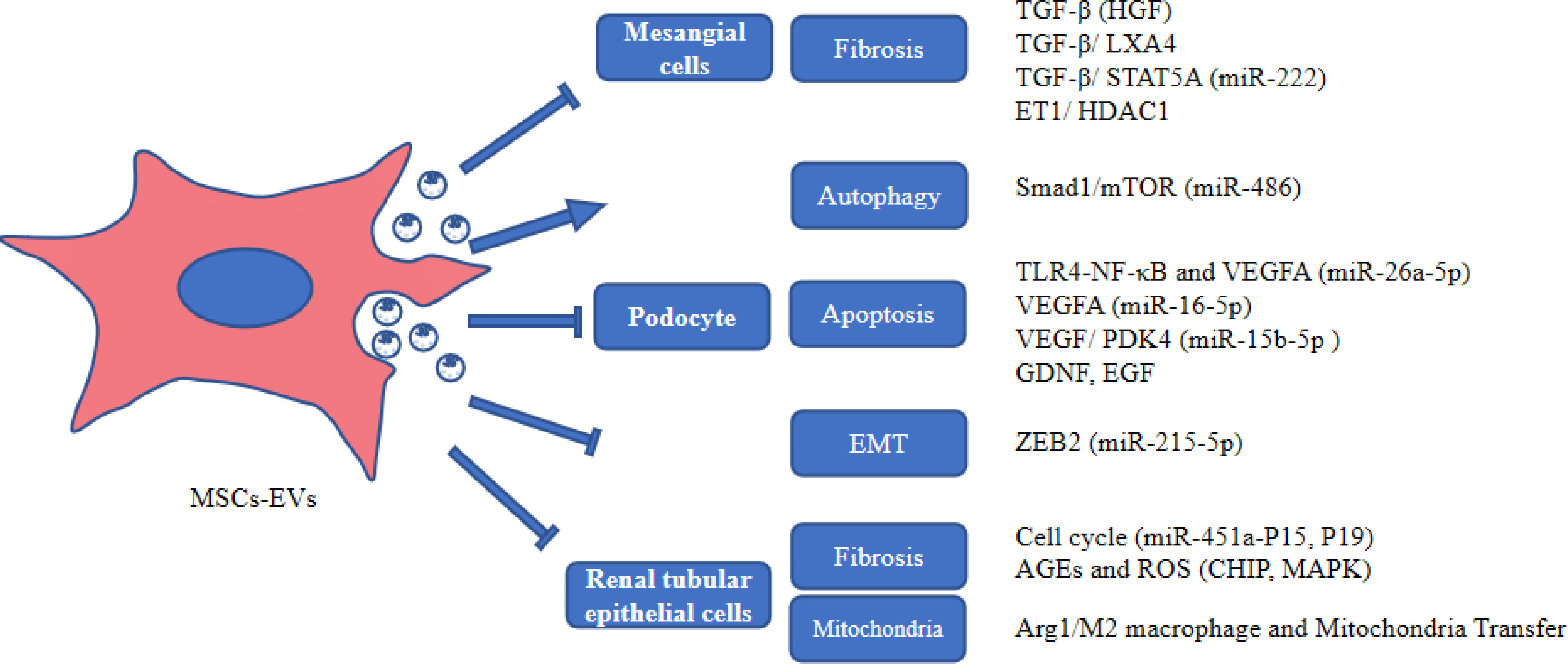
Figure 2 The role of MSC-EVs in protecting kidney cells. AGEs, advanced glycation end products; Arg1, arginase-1; EGF, epidermal growth factor; GDNF, glial cell-derived neurotrophic growth factor; HDAC1, histone deacetylase 1; HGF, hepatocyte growth factor; LXA4, lipoxin A4; MAPK, mitogen-activated protein kinase; PDK4, Pyruvate dehydrogenase kinase 4; ROS, reactive oxygen species; VEGF, vascular endothelial growth factor; ZEB2, Zinc finger E-box-binding homeobox 2.
Efficacy of MSC-EVs for DKD: A preclinical model
Intravenous injection of MSC-EVs can improve renal function and histological damage in diabetic animals. Hyperglycemia is a major factor in the occurrence of DKD. EVs secreted from AD-MSCs, BM-MSCs and UC-MSCs are able to reduce blood glucose. Furthermore, EVs can reduce serum creatinine (SCr), blood urea nitrogen (BUN), urinary protein (URPO), and urine albumin-to-creatinine ratio (UACR), and increase creatinine clearance (CCR) (28, 38, 63). Hyperlipidemia is an important risk factor for vascular complications of chronic kidney disease (64). BM-MSC-EVs reduced total cholesterol (TC) and triglycerides (TG) in a DKD mouse model (65, 66). Jiang et al. (27) found that HU-MSC-EVs could reduce the urinary microalbumin excretion and urine volume of DKD rats.
Duan et al. (29) showed that AD-MSC-EVs notably alleviated the histopathological changes associated with DKD, such as reducing ECM accumulation in their kidney tissues and the thickening of basement membrane. Jiang et al. (27)found that UC-MSC-EVs could prevent cell apoptosis in diabetic rats. Further, UC-MSC-EVs treatment significantly ameliorated mesangial expansion and promoted endothelial cell proliferation from glomerulus in the early stages of impairment in DKD kidney. Ebrahim et al. (63) found that injection of EVs in DKD rats reduced histological damage. EVs alleviated diffuse thickening of glomerular basement membrane and extensive fusion and disappearance of foot process.
Inflammation and fibrosis play a key role in the pathogenesis of DKD. Xiang et al. (67) found that UC-MSC-CM or UC-MSC-EVs suppressed IL-1β, TNF-α, IL-6, and TGF-β in HG-injured human renal glomerular endothelial cell line (hrGECs) and RTECs (HK2 and NRK-52E). Duan et al. (28) reported that HU-MSC-EVs can reduce the expression of VEGFA, monocyte chemoattractant protein-1 (MCP-1), TNF-α and TGF-β1 in diabetic mice. This means that the UC-MSC product can relieve inflammation in diabetic mice. MCP-1 is also known as the C-C motif chemokine ligand 2 (CCL2). CCL2 can stimulate the production of TGF-β1 in mesangial cells and macrophages, and TGF-β1 feeds back to increase the CCL2 expression in mesangial cells (68). Substantial evidence indicates that TGF-β/Smad signaling takes part in the development and progression of fibrosis of kidney (69). Hao et al. (38)found that AD-MSC-EVs reduced the protein expression of the Col-I (fibrosis-related marker) and suppressed mesangial hyperplasia in DKD rats (Table 2). Mao et al. (65) found that BM-MSC-EVs miR-let-7a inhibited the expression of N-cadherin and vimentin (Table 2). Furthermore, Nagaishi et al. (39) showed that BM-MSC-CM can reduce TNF-α, ICAM-1 and increase ZO-1 (Table 2). Zhong et al. (41) showed that UC-MSC-EVs reduced α-SMA, increased E-cadherin and prevented histological damages (Table 2). Grange et al. (70) reported that human liver stem-like cells (HLSC)-EVs and BM-MSC-EVs down-regulate genes involved in the pathogenesis of fibrosis, such as tissue inhibitor of metalloproteinases (TIMP), metalloproteinase 3 (MMP3), collagen I, TGF-β and α-SMA, the FAS ligand, CCL3, and Snail (Table 2). This indicates that injection of MSC-EVs from various sources can alleviate fibrosis in diabetic animals.
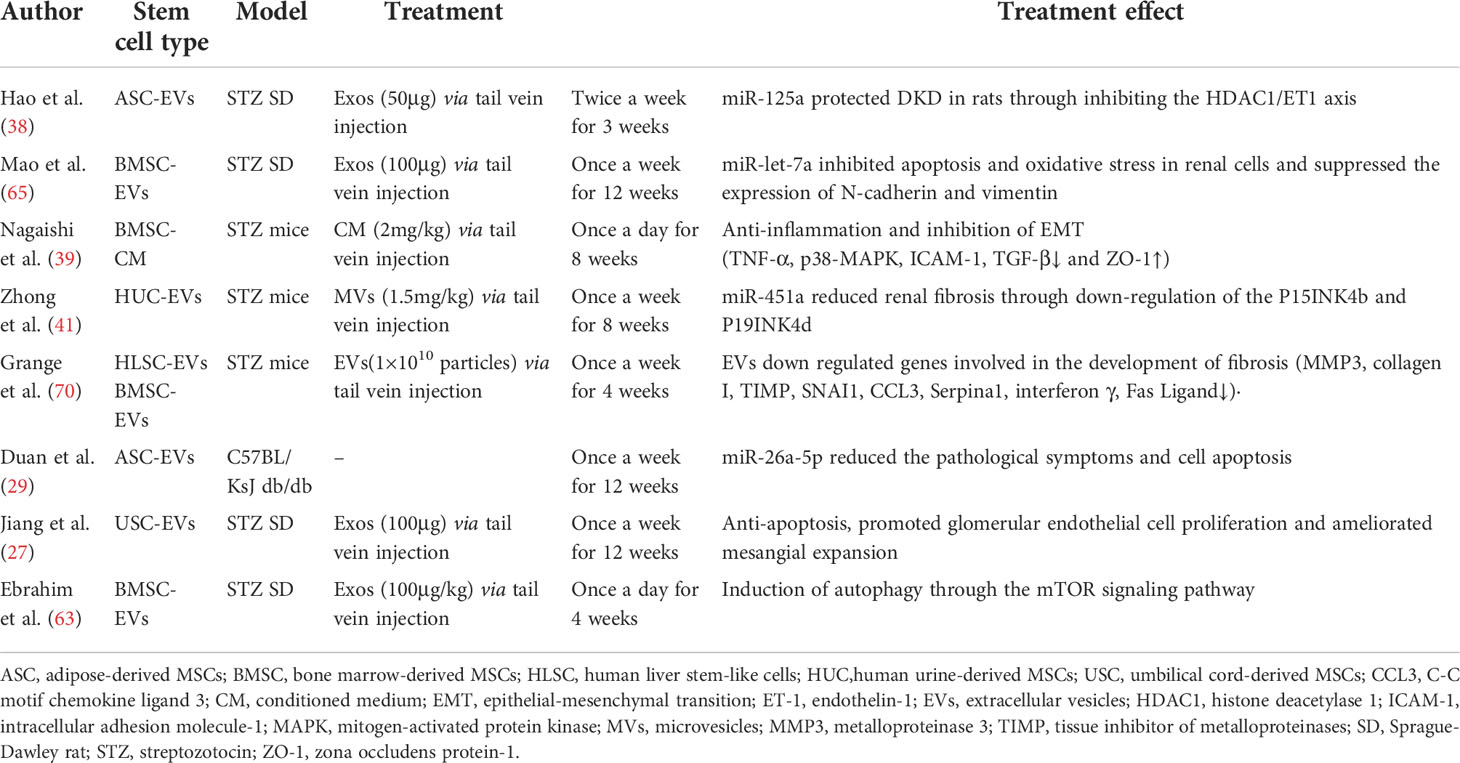
Table 2 Characteristics of preclinical studies assessing the efficacy of MSC products for DKD in vivo.
In addition to being anti-inflammatory and anti-fibrotic, MSC-EVs protect DKD animals by other means. With the effecting by increased oxidative stress and reduced NO bioavailability, endothelial dysfunction is a hallmark feature of type 2 diabetes mellitus and DKD (71). Mao et al. (65) found that BM-MSC-EVs suppress oxidative stress, based on decreases in NO and MDA content, and elevations of GSH-Px and SOD activities. EVs injection can alleviate the oxidative stress reaction in diabetic mice and play a protective role in DKD through down-regulation of USP22. Autophagy, a conserved and important “self-eating” pathway, is an important mechanism for maintaining glomerular and tubular homeostasis, and is involved in various aspects of renal injury, aging and disease (72). Ebrahim et al. (63) reported that BM-MSC-EVs can inhibit mTOR, and S6K1 protein expression, and increase Beclin-1, LC3-I, LC3-II, and p62 protein expression. MSC-EVs ameliorate DKD by modulating the mTOR-related autophagy pathway (Table 2). Duan et al. (29) reported that Bcl-2 protein was significantly increased by AD-MSC-EVs, whereas the protein expressions of cleaved caspase-3, caspase-3, and Bax were reduced. MiR-26a-5p produced by AD-MSC-EVs not only reduced podocyte apoptosis in vitro, but also reduced apoptosis in spontaneously diabetic mice.
Overall, through multiple studies injected with stem cell products from different sources, we found that stem cell products can alleviate kidney damage in animal models of DKD (Table 1).
Effect of stem cell extracellular vesicles on inflammatory factors and their potential signaling pathways
Phosphorylation of signal transducers and activators of transcription-1 (STAT1) is induced by multiple TLRs (TLR2, TLR4, TLR9). Tumor necrosis factor receptor-associated factor-6 (TRAF6) is a most important factor to activate TLR signaling. After activation of TRAF6, STAT1 is phosphorylated and translocated to the nucleus (73). Zhang et al. (74) found that UC-MSCs-derived miR-146a-5p can target the TRAF6 and significantly decreased the expression of p-STAT1. They identified that miR-146a-5p targeted the signaling pathway of TRAF6-STAT1 to inhibit renal inflammation and restore the function of kidney by promoting the polarization of M2 macrophage. Transcription factors, such as NF-κB, can regulate inflammation, immunological responses and cell proliferation (75). In DKD, the transposition of NF-κB into the nucleus can activate its target genes, including the inflammatory mediators of its downstream, such as nitric oxide synthase, TNF-β1, IL-1 and ICAM-1, which subsequently cause persistent and increased inflammation leading to overexpression of fibronectin and ECM accumulation in mesangial cells (76). Duan et al. (29) reported that AD-MSC-EV-miR-26a-5p alleviated both the ECM accumulation in kidney tissues and the thickening of basement membrane, and inhibited apoptosis in mouse podocytes in vitro by inhibiting signaling pathways of NF-κB/VEGFA and TLR4.
Autophagy promotes the degradation of excess or malfunctioning cellular components, including invasive microorganisms, misfolded proteins, damaged organelles and cells themselves (77). In DKD, autophagy represents the cooperation of related gene products of multiple autophagy (23). In general, mTORC1 is regarded as a negative regulator for the autophagy. Enhanced mTORC1 activity was observed in type 1 and type 2 DKD diseases from animal models. Treatment with rapamycin (an inhibitor of mTORC1) can suppress the progression of DKD disease induced by STZ in type 1 and type 2 diabetes from rats or mice (78, 79). Injection of MSC-EVs into the tail vein of DKD rats resulted in significant up-regulation of autophagy-related proteins (LC3II and Beclin-1), and significant down-regulation of mTOR gene expression. The results indicated that BM-MSC-EVs enhance autophagy by inhibiting mTOR (63). Jin et al. (32) found that AD-MSC-EVs inhibited p62/LC3 and mTOR signaling pathways, increased the levels of autophagy-related proteins, and ameliorated cell damage of podocyte by inhibiting the signaling pathway of miR-486/Smad1/mTOR. Cai et al. (80) showed that miR-125b from MSC-EVs could induce the cell autophagy to inhibit HKCs apoptosis induced by HG via signaling pathway of Akt. Collectively, multiple miRNAs in EVs inhibited mTORC1 expression by inhibiting AKT and finally upregulated autophagy.
It is well known that the signaling pathway of TGF-β can play an important role in fibrogenesis and also promotes fibrosis in DKD (81). Additionally, the signaling pathway of TGF-β/Smad also affects DKD through cross-talk with other pathways, such as the MAPK and PI3K/Akt signaling pathways (82). The TGF-β/Smad signaling pathway is notably activated in renal fibrosis. Smad3 exhibits a marked collagen deposition and contributes to the progression of renal fibrosis in db/db mice, and Smad3 knockout inhibits this process (83). Nagaishi et al. (39) reported that MSC-CM inhibits tubulointerstitial fibrosis in a model of DKD by reversing the endogenously elevated or ectopically expressed TGF-β. Furthermore, Bai et al. (36) demonstrated that MSC-CM reversed DKD via LXA4 by targeting the TGF-β/Smad pathway and pro-inflammatory cytokines. Liu et al. (84) found that MSCs modified with angiotensin-converting enzyme 2 (ACE2) to target the damaged kidney and enhance the expression of ACE2. The modified MSCs secreted soluble ACE2 protein into the culture medium. The upregulated ACE2 can degrade the Ang II into the Ang1-7, and MSCs-ACE2 is more beneficial when compared with MSCs alone in downregulating Ang II and upregulating Ang1-7. MSCs-ACE2 inhibited the deleterious effects of the accumulation of Ang II and inhibited the TGF-β/Smad pathway. Li et al. (34) found that MSC-CM alleviated renal fibrosis in a DKD model by blocking myofibroblast transdifferentiation mediated by the signaling pathway of TGF-β1/Smad2/3. MSC-CM also inhibited the proliferation of mesangial cell mediated by signaling pathways of PI3K/Akt and MAPK, and enhanced the expression of MMPs. Multiple investigations have indicated that MSC-EVs improve renal fibrosis which mediated TGF-β pathway and PI3K/Akt and MAPK signaling pathway by inhibiting the expression of TGF-β. (Figure 3).
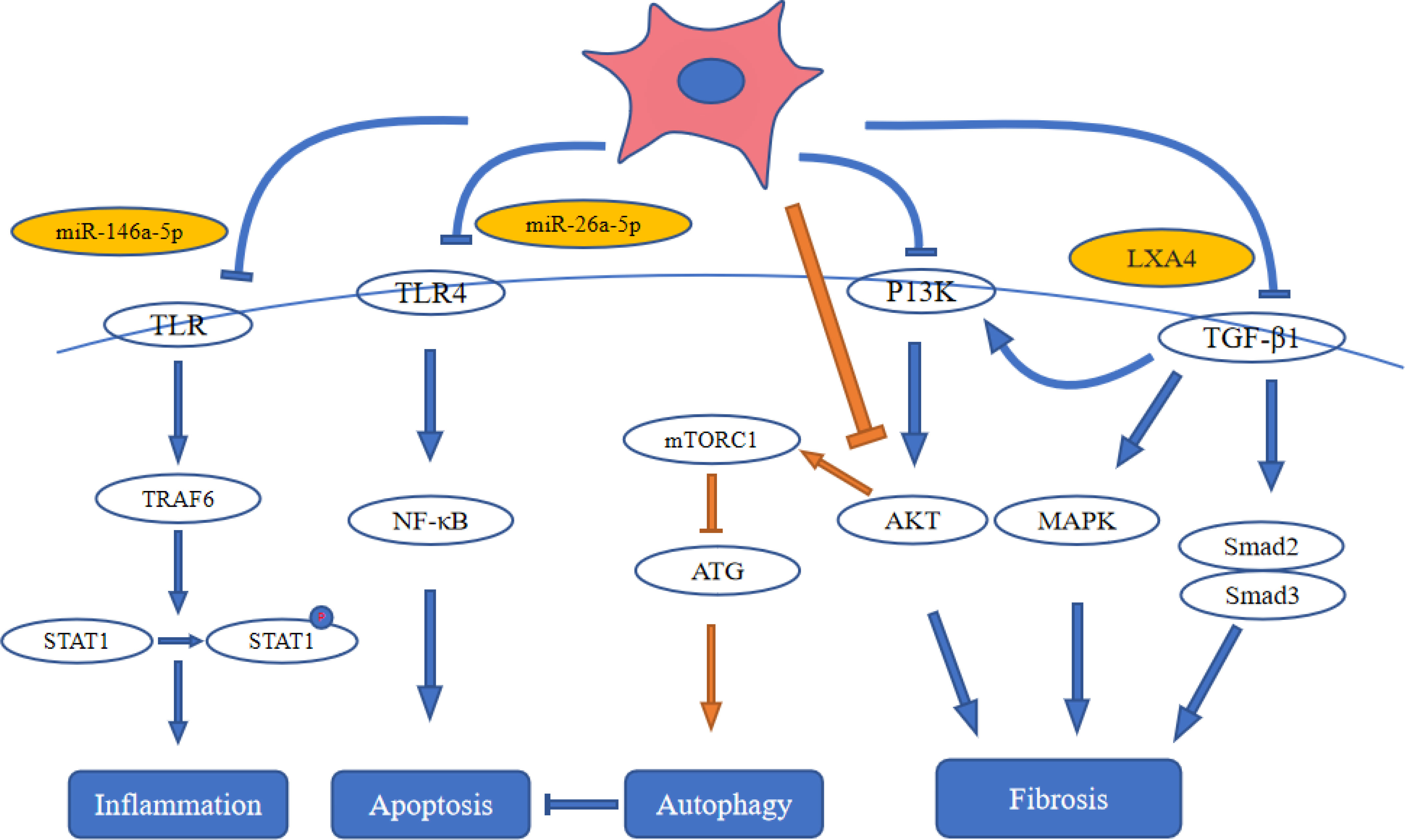
Figure 3 Stem cell therapy influences signal pathways in diabetic kidney disease. ATG: autophagy-related gene; LXA4: lipoxin A4; MAPK: mitogen-activated protein kinase; NF-κB: nuclear factor kappa-B; STAT1: Signal Transducers and Activators of Transcription-1; TLR4: toll-like receptor 4; TRAF6: tumor necrosis factor receptor-associated factor-6.
Conclusion
The importance of DKD has been increasingly acknowledged and treatment methods have been updated and improved. However, in the past 20 years, the incidence and prognosis of DKD have not been effectively controlled. MSC-based therapy brings prospective treatment to DKD. Various growth factors and miRNAs contained in MSC-EVs seem to play an important role in the therapeutic effect of MSCs. Some studies have pointed out that MSC-EVs not only protect podocytes, renal tubular epithelial cells and mesangial cells from HG-induced injury, but also protect against DKD in animals. Moreover, some studies have shown that the treatment efficacy of ACE2-modified MSCs is more effective than MSCs alone, and MSCs combined with miRNAs treatment can enhance the protective effect of MSCs. At the same time, MSC-EVs also alleviated the DKD in animals through various regulatory signaling pathways (NF-κB, TLR, mTOR, MAPK, PI3K/Akt, TGF-β/Smad). However, it is difficult to find a unified standard for the treatment of DKD with MSC extracellular vesicles. Different stem cell-derived extracellular vesicles, injection doses and frequencies are used in different experiments. Therefore, more research is needed to optimize the different MSC products, injection frequencies and doses to treat DKD.
Author contributions
YDL and TZ looked up a lot of pertinent papers and wrote the manuscript. QY, JW, XC and YPL reviewed and checked the article. TZ modified and polished the article, and reviewed the article. All authors contributed to the article and approved the submitted version.
Funding
This review was supported by Shantou Science and Technology Project (Shanfuke [2019] 106-4: 190606165268433), Guangdong Province Science and Technology Special Fund (shanfuke [2021]88-28: 210714086900312) and Shantou Youth Talent Project (no. 2020023).
Conflict of interest
The authors declare that the research was conducted in the absence of any commercial or financial relationships that could be construed as a potential conflict of interest.
Publisher’s note
All claims expressed in this article are solely those of the authors and do not necessarily represent those of their affiliated organizations, or those of the publisher, the editors and the reviewers. Any product that may be evaluated in this article, or claim that may be made by its manufacturer, is not guaranteed or endorsed by the publisher.
Glossary
References
1. Saeedi P, Petersohn I, Salpea P, Malanda B, Karuranga S, Unwin N, et al. Global and regional diabetes prevalence estimates for 2019 and projections for 2030 and 2045: Results from the international diabetes federation diabetes atlas, 9(th) edition. Diabetes Res Clin Pract (2019) 157:107843. doi: 10.1016/j.diabres.2019.107843
2. Jadawji C, Crasto W, Gillies C, Kar D, Davies MJ, Khunti K, et al. Prevalence and progression of diabetic nephropathy in south Asian, white European and African Caribbean people with type 2 diabetes: A systematic review and meta-analysis. Diabetes Obes Metab (2019) 21:658–73. doi: 10.1111/dom.13569.
3. Gao Z SA, Li XM, Li XL, Sui LN. Identification of key candidate genes and chemical perturbagens in diabetic kidney disease using integrated bioinformatics analysis. Front Endocrinol (2021) 12:721202. doi: 10.3389/fendo.2021.721202
4. Muskiet MHA, Wheeler DC, Heerspink HJL. New pharmacological strategies for protecting kidney function in type 2 diabetes. Lancet Diabetes Endocrinol (2019) 7:397–412. doi: 10.1016/S2213-8587(18)30263-8
5. Huang Y, Liu W, Liu J, Guo D, Zhang P, Liu D, et al. Association of urinary sodium excretion and diabetic kidney disease in patients with type 2 diabetes mellitus: A cross-sectional study. Front Endocrinol (2021) 12:772073. doi: 10.3389/fendo.2021.772073
6. Cheng HT, Xu X, Lim PS, Hung KY. Worldwide epidemiology of diabetes-related end-stage renal disease, 2000-2015. Diabetes Care (2021) 44:89–97. doi: 10.2337/dc20-1913
7. Gregg EW, Li Y, Wang J, Burrows NR, Ali MK, Rolka D, et al. Changes in diabetes-related complications in the united states, 1990-2010. N Engl J Med (2014) 370:1514–23. doi: 10.1056/NEJMoa1310799
8. Amable PR, Teixeira MV, Carias RB, Granjeiro JM, Borojevic R. Protein synthesis and secretion in human mesenchymal cells derived from bone marrow, adipose tissue and wharton's jelly. Stem Cell Res Ther (2014) 5:53. doi: 10.1186/scrt442
9. Kariminekoo S, Movassaghpour A, Rahimzadeh A, Talebi M, Shamsasenjan K, Akbarzadeh A. Implications of mesenchymal stem cells in regenerative medicine. Artif Cells Nanomed Biotechnol (2016) 44:749–57. doi: 10.3109/21691401.2015.1129620
10. Li FX, Lin X, Xu F, Shan SK, Guo B, Lei LM, et al. The role of mesenchymal stromal cells-derived small extracellular vesicles in diabetes and its chronic complications. Front Endocrinol (2021) 12:780974. doi: 10.3389/fendo.2021.780974
11. Sun J, Zhao F, Zhang W, Lv J, Lv J, Yin A. BMSCs and miR-124a ameliorated diabetic nephropathy via inhibiting notch signalling pathway. J Cell Mol Med (2018) 22:4840–55. doi: 10.1111/jcmm.13747
12. Kundu N, Nandula SR, Asico LD, Fakhri M, Banerjee J, Jose PA, et al. Transplantation of apoptosis-resistant endothelial progenitor cells improves renal function in diabetic kidney disease. J Am Heart Assoc (2021) 10:e019365. doi: 10.1161/JAHA.120.019365
13. Schrepfer S, Deuse T, Reichenspurner H, Fischbein MP, Robbins RC, Pelletier MP. Stem cell transplantation: The lung barrier. Transplant Proc (2007) 39:573–6. doi: 10.1016/j.transproceed.2006.12.019
14. Zhang L, Li K, Liu X, Li D, Luo C, Fu B, et al. Repeated systemic administration of human adipose-derived stem cells attenuates overt diabetic nephropathy in rats. Stem Cells Dev (2013) 22:3074–86. doi: 10.1089/scd.2013.0142
15. Hickson LJ, Abedalqader T, Ben-Bernard G, Mondy JM, Bian X, Conley SM, et al. A systematic review and meta-analysis of cell-based interventions in experimental diabetic kidney disease. Stem Cells Transl Med (2021) 10:1304–19. doi: 10.1002/sctm.19-0419
16. Théry C, Witwer KW, Aikawa E, Alcaraz MJ, Anderson JD, Andriantsitohaina R, et al. Minimal information for studies of extracellular vesicles 2018 (MISEV2018): A position statement of the international society for extracellular vesicles and update of the MISEV2014 guidelines. J Extracell Vesicles (2018) 7:1535750. doi: 10.1080/20013078.2018.1535750
17. Liao C, Chen G, Yang Q, Liu Y, Zhou T. Potential therapeutic effect and mechanisms of mesenchymal stem cells-extracellular vesicles in renal fibrosis. Front Cell Dev Biol (2022) 10:824752. doi: 10.3389/fcell.2022.824752
18. Gimona M, Brizzi MF, Choo ABH, Dominici M, Davidson SM, Grillari J, et al. Critical considerations for the development of potency tests for therapeutic applications of mesenchymal stromal cell-derived small extracellular vesicles. Cytotherapy (2021) 23:373–80. doi: 10.1016/j.jcyt.2021.01.001
19. Thery C, Ostrowski M, Segura E. Membrane vesicles as conveyors of immune responses. Nat Rev Immunol (2009) 9:581–93. doi: 10.1038/nri2567
20. van der Pol E, Boing AN, Harrison P, Sturk A, Nieuwland R. Classification, functions, and clinical relevance of extracellular vesicles. Pharmacol Rev (2012) 64:676–705. doi: 10.1124/pr.112.005983
21. Lotvall J, Hill AF, Hochberg F, Buzas EI, Di Vizio D, Gardiner C, et al. Minimal experimental requirements for definition of extracellular vesicles and their functions: A position statement from the international society for extracellular vesicles. J Extracell Vesicles (2014) 3:26913. doi: 10.3402/jev.v3.26913
22. Eirin A, Zhu XY, Puranik AS, Tang H, McGurren KA, van Wijnen AJ, et al. Mesenchymal stem cell-derived extracellular vesicles attenuate kidney inflammation. Kidney Int (2017) 92:114–24. doi: 10.1016/j.kint.2016.12.023
23. Sun B, Zhai S, Zhang L, Sun G. The role of extracellular vesicles in podocyte autophagy in kidney disease. J Cell Commun Signal (2021) 15:299–316. doi: 10.1007/s12079-020-00594-z
24. Zeng L, Szeto CC. Urinary podocyte markers in kidney diseases. Clin Chim Acta (2021) 523:315–24. doi: 10.1016/j.cca.2021.10.017
25. Pagtalunan ME, Miller PL, Jumping-Eagle S, Nelson RG, Myers BD, Rennke HG, et al. Podocyte loss and progressive glomerular injury in type II diabetes. J Clin Invest (1997) 99:342–8. doi: 10.1172/JCI119163
26. Deng H, Sun C, Sun Y, Li H, Yang L, Wu D, et al. Lipid, protein, and MicroRNA composition within mesenchymal stem cell-derived exosomes. Cell Reprogram (2018) 20:178–86. doi: 10.1089/cell.2017.0047
27. Jiang ZZ, Liu YM, Niu X, Yin JY, Hu B, Guo SC, et al. Exosomes secreted by human urine-derived stem cells could prevent kidney complications from type I diabetes in rats. Stem Cell Res Ther (2016) 7:24. doi: 10.1186/s13287-016-0287-2
28. Duan YR, Chen BP, Chen F, Yang SX, Zhu CY, Ma YL, et al. Exosomal microRNA-16-5p from human urine-derived stem cells ameliorates diabetic nephropathy through protection of podocyte. J Cell Mol Med (2021) 25:10798–813. doi: 10.1111/jcmm.14558
29. Duan Y, Luo Q, Wang Y, Ma Y, Chen F, Zhu X, et al. Adipose mesenchymal stem cell-derived extracellular vesicles containing microRNA-26a-5p target TLR4 and protect against diabetic nephropathy. J Biol Chem (2020) 295:12868–84. doi: 10.1074/jbc.RA120.012522
30. Zhao T, Jin Q, Kong L, Zhang D, Teng Y, Lin L, et al. microRNA-15b-5p shuttled by mesenchymal stem cell-derived extracellular vesicles protects podocytes from diabetic nephropathy via downregulation of VEGF/PDK4 axis. J Bioenergetics Biomembranes (2022) 54:17–30. doi: 10.1007/s10863-021-09919-y
31. Li D, Wang N, Zhang L, Hanyu Z, Xueyuan B, Fu B, et al. Mesenchymal stem cells protect podocytes from apoptosis induced by high glucose via secretion of epithelial growth factor. Stem Cell Res Ther (2013) 4:103. doi: 10.1186/scrt314
32. Jin J, Shi Y, Gong J, Zhao L, Li Y, He Q, et al. Exosome secreted from adipose-derived stem cells attenuates diabetic nephropathy by promoting autophagy flux and inhibiting apoptosis in podocyte. Stem Cell Res Ther (2019) 10:95. doi: 10.1186/s13287-019-1177-1
33. Jin J, Wang Y, Zhao L, Zou W, Tan M, He Q. Exosomal miRNA-215-5p derived from adipose-derived stem cells attenuates epithelial-mesenchymal transition of podocytes by inhibiting ZEB2. BioMed Res Int (2020) 2020:2685305. doi: 10.1155/2020/2685305
34. Li H, Rong P, Ma X, Nie W, Chen Y, Zhang J, et al. Mouse umbilical cord mesenchymal stem cell paracrine alleviates renal fibrosis in diabetic nephropathy by reducing myofibroblast transdifferentiation and cell proliferation and upregulating MMPs in mesangial cells. J Diabetes Res (2020) 2020:3847171. doi: 10.1155/2020/3847171
35. Lv S, Cheng J, Sun A, Li J, Wang W, Guan G, et al. Mesenchymal stem cells transplantation ameliorates glomerular injury in streptozotocin-induced diabetic nephropathy in rats via inhibiting oxidative stress. Diabetes Res Clin Pract (2014) 104:143–54. doi: 10.1016/j.diabres.2014.01.011
36. Bai Y, Wang J, He Z, Yang M, Li L, Jiang H. Mesenchymal stem cells reverse diabetic nephropathy disease via lipoxin A4 by targeting transforming growth factor β (TGF-β)/smad pathway and pro-inflammatory cytokines. Med Sci Monit Int Med J Exp Clin Res (2019) 25:3069–76. doi: 10.12659/MSM.914860
37. Gallo S, Gili M, Lombardo G, Rossetti A, Rosso A, Dentelli P, et al. Stem cell-derived, microRNA-carrying extracellular vesicles: A novel approach to interfering with mesangial cell collagen production in a hyperglycaemic setting. PloS One (2016) 11:e0162417. doi: 10.1371/journal.pone.0162417
38. Hao Y, Miao J, Liu W, Cai K, Huang X, Peng L. Mesenchymal stem cell-derived exosomes carry MicroRNA-125a to protect against diabetic nephropathy by targeting histone deacetylase 1 and downregulating endothelin-1. Diabetes Metab Syndr Obes (2021) 14:1405–18. doi: 10.2147/DMSO.S286191
39. Nagaishi K, Mizue Y, Chikenji T, Otani M, Nakano M, Konari N, et al. Mesenchymal stem cell therapy ameliorates diabetic nephropathy via the paracrine effect of renal trophic factors including exosomes. Sci Rep (2016) 6:34842. doi: 10.1038/srep34842
40. Park JH, Hwang I, Hwang SH, Han H, Ha H. Human umbilical cord blood-derived mesenchymal stem cells prevent diabetic renal injury through paracrine action. Diabetes Res Clin Pract (2012) 98:465–73. doi: 10.1016/j.diabres.2012.09.034
41. Zhong L, Liao G, Wang X, Li L, Zhang J, Chen Y, et al. Mesenchymal stem cells-microvesicle-miR-451a ameliorate early diabetic kidney injury by negative regulation of P15 and P19. Exp Biol Med (Maywood NJ) (2018) 243:1233–42. doi: 10.1177/1535370218819726
42. Rao N, Wang X, Xie J, Li J, Zhai Y, Li X, et al. Stem cells from human exfoliated deciduous teeth ameliorate diabetic nephropathy In vivo and In vitro by inhibiting advanced glycation end product-activated epithelial-mesenchymal transition. Stem Cells Int (2019) 2019:2751475. doi: 10.1155/2019/2751475
43. Ali A, Shibu MA, Kuo CH, Lo JF, Chen RJ, Day CH, et al. CHIP-overexpressing wharton's jelly-derived mesenchymal stem cells attenuate hyperglycemia-induced oxidative stress-mediated kidney injuries in diabetic rats. Free Radical Biol Med (2021) 173:70–80. doi: 10.1016/j.freeradbiomed.2021.07.026
44. Lee SE, Jang JE, Kim HS, Jung MK, Ko MS, Kim MO, et al. Mesenchymal stem cells prevent the progression of diabetic nephropathy by improving mitochondrial function in tubular epithelial cells. Exp Mol Med (2019) 51:1–14. doi: 10.1038/s12276-019-0299-y
45. Konari N, Nagaishi K, Kikuchi S, Fujimiya M. Mitochondria transfer from mesenchymal stem cells structurally and functionally repairs renal proximal tubular epithelial cells in diabetic nephropathy in vivo. Sci Rep (2019) 9:5184. doi: 10.1038/s41598-019-40163-y
46. Bailey E, Bottomley MJ, Westwell S, Pringle JH, Furness PN, Feehally J, et al. Vascular endothelial growth factor mRNA expression in minimal change, membranous, and diabetic nephropathy demonstrated by non-isotopic in situ hybridisation. J Clin Pathol (1999) 52:735–8. doi: 10.1136/jcp.52.10.735
47. Vriese AS, Tilton RG, Elger M, Stephan CC, Kriz W, Lameire NH. Antibodies against vascular endothelial growth factor improve early renal dysfunction in experimental diabetes. J Am Soc Nephrol (2001) 12:993–1000. doi: 10.1681/ASN.V125993
48. Weng Y, Shen Y, He Y, Pan X, Xu J, Jiang Y, et al. The miR-15b-5p/PDK4 axis regulates osteosarcoma proliferation through modulation of the warburg effect. Biochem Biophys Res Commun (2018) 503:2749–57. doi: 10.1016/j.bbrc.2018.08.035
49. Tsui CC, Shankland SJ, Pierchala BA. Glial cell line-derived neurotrophic factor and its receptor ret is a novel ligand-receptor complex critical for survival response during podocyte injury. J Am Soc Nephrol (2006) 17:1543–52. doi: 10.1681/ASN.2005080835
50. Kroemer G, Marino G, Levine B. Autophagy and the integrated stress response. Mol Cell (2010) 40:280–93. doi: 10.1016/j.molcel.2010.09.023
51. Hartleben B, Godel M, Meyer-Schwesinger C, Liu S, Ulrich T, Kobler S, et al. Autophagy influences glomerular disease susceptibility and maintains podocyte homeostasis in aging mice. J Clin Invest (2010) 120:1084–96. doi: 10.1172/JCI39492
52. Ying Q, Wu G. Molecular mechanisms involved in podocyte EMT and concomitant diabetic kidney diseases: an update. Ren Fail (2017) 39:474–83. doi: 10.1080/0886022X.2017.1313164
53. Qi W, Chen X, Zhang Y, Holian J, Mreich E, Gilbert RE, et al. High glucose induces macrophage inflammatory protein-3 alpha in renal proximal tubule cells via a transforming growth factor-beta 1 dependent mechanism. Nephrol Dial Transplant (2007) 22:3147–53. doi: 10.1093/ndt/gfm365
54. Fukui M, Nakamura T, Ebihara I, Osada S, Tomino Y, Masaki T, et al. Gene expression for endothelins and their receptors in glomeruli of diabetic rats. J Lab Clin Med (1993) 122:149–56.
55. Horstmeyer A, Licht C, Scherr G, Eckes B, Krieg T. Signalling and regulation of collagen I synthesis by ET-1 and TGF-beta1. FEBS J (2005) 272:6297–309. doi: 10.1111/j.1742-4658.2005.05016.x
56. Sun X, Xiao H, Li S, Chen R, Lin Z, Yang Y, et al. Connexin32 ameliorates epithelial-to-mesenchymal-transition in diabetic renal tubular via inhibiting NOX4. Pharmacol Res (2022) 176:106084. doi: 10.1016/j.phrs.2022.106084
57. Zhou D, Liu Y. Renal fibrosis in 2015: Understanding the mechanisms of kidney fibrosis. Nat Rev Nephrol (2016) 12:68–70. doi: 10.1038/nrneph.2015.215
58. Matsui T, Yamagishi S, Takeuchi M, Ueda S, Fukami K, Okuda S. Irbesartan inhibits advanced glycation end product (AGE)-induced proximal tubular cell injury in vitro by suppressing receptor for AGEs (RAGE) expression. Pharmacol Res (2010) 61:34–9. doi: 10.1016/j.phrs.2009.07.004
59. Lv ZM, Wang Q, Wan Q, Lin JG, Hu MS, Liu YX, et al. The role of the p38 MAPK signaling pathway in high glucose-induced epithelial-mesenchymal transition of cultured human renal tubular epithelial cells. PloS One (2011) 6:e22806. doi: 10.1371/journal.pone.0022806
60. Volpe CMO, Villar-Delfino PH, Dos Anjos PMF, Nogueira-Machado JA. Cellular death, reactive oxygen species (ROS) and diabetic complications. Cell Death Dis (2018) 9:119. doi: 10.1038/s41419-017-0135-z
61. Yu EP, Bennett MR. Mitochondrial DNA damage and atherosclerosis. Trends Endocrinol Metab (2014) 25:481–7. doi: 10.1016/j.tem.2014.06.008
62. Mills CD. M1 and M2 macrophages: Oracles of health and disease. Crit Rev Immunol (2012) 32:463–88. doi: 10.1615/CritRevImmunol.v32.i6.10
63. Ebrahim N, Ahmed IA, Hussien NI, Dessouky AA, Farid AS, Elshazly AM, et al. Mesenchymal stem cell-derived exosomes ameliorated diabetic nephropathy by autophagy induction through the mTOR signaling pathway. Cells (2018) 7:226. doi: 10.20944/preprints201809.0153.v1
64. Molitch ME. Management of dyslipidemias in patients with diabetes and chronic kidney disease. Clin J Am Soc Nephrol (2006) 1:1090–9. doi: 10.2215/CJN.00780306
65. Mao R, Shen J, Hu X. BMSCs-derived exosomal microRNA-let-7a plays a protective role in diabetic nephropathy via inhibition of USP22 expression. Life Sci (2021) 268:118937. doi: 10.1016/j.lfs.2020.118937
66. Wang S, Bao L, Fu W, Deng L, Ran J. Protective effect of exosomes derived from bone marrow mesenchymal stem cells on rats with diabetic nephropathy and its possible mechanism. Am J Transl Res (2021) 13:6423–30.
67. Xiang E, Han B, Zhang Q, Rao W, Wang Z, Chang C, et al. Human umbilical cord-derived mesenchymal stem cells prevent the progression of early diabetic nephropathy through inhibiting inflammation and fibrosis. Stem Cell Res Ther (2020) 11:336. doi: 10.1186/s13287-020-01852-y
68. Cheng J, Diaz Encarnacion MM, Warner GM, Gray CE, Nath KA, Grande JP. TGF-beta1 stimulates monocyte chemoattractant protein-1 expression in mesangial cells through a phosphodiesterase isoenzyme 4-dependent process. Am J Physiol Cell Physiol (2005) 289:C959–970. doi: 10.1152/ajpcell.00153.2005
69. Hills CE, Squires PE. The role of TGF-beta and epithelial-to mesenchymal transition in diabetic nephropathy. Cytokine Growth Factor Rev (2011) 22:131–9. doi: 10.1016/j.cytogfr.2011.06.002.
70. Grange C, Tritta S, Tapparo M, Cedrino M, Tetta C, Camussi G, et al. Stem cell-derived extracellular vesicles inhibit and revert fibrosis progression in a mouse model of diabetic nephropathy. Sci Rep (2019) 9:4468. doi: 10.1038/s41598-019-41100-9
71. Goligorsky MS, Chen J, Brodsky S. Workshop: endothelial cell dysfunction leading to diabetic nephropathy : Focus on nitric oxide. Hypertension (2001) 37:744–8. doi: 10.1161/01.HYP.37.2.744
72. Wang Z, Choi ME. Autophagy in kidney health and disease. Antioxid Redox Signal (2014) 20:519–37. doi: 10.1089/ars.2013.5363
73. Luu K, Greenhill CJ, Majoros A, Decker T, Jenkins BJ, Mansell A. STAT1 plays a role in TLR signal transduction and inflammatory responses. Immunol Cell Biol (2014) 92:761–9. doi: 10.1038/icb.2014.51
74. Zhang Y, Le X, Zheng S, Zhang K, He J, Liu M, et al. MicroRNA-146a-5p-modified human umbilical cord mesenchymal stem cells enhance protection against diabetic nephropathy in rats through facilitating M2 macrophage polarization. Stem Cell Res Ther (2022) 13:171. doi: 10.1186/s13287-022-02855-7
75. Barnes PJ, Karin M. Nuclear factor-kappaB: a pivotal transcription factor in chronic inflammatory diseases. N Engl J Med (1997) 336:1066–71. doi: 10.1056/NEJM199704103361506
76. Jiang Q, Liu P, Wu X, Liu W, Shen X, Lan T, et al. Berberine attenuates lipopolysaccharide-induced extracelluar matrix accumulation and inflammation in rat mesangial cells: Involvement of NF-kappaB signaling pathway. Mol Cell Endocrinol (2011) 331:34–40. doi: 10.1016/j.mce.2010.07.023
77. Xie W, Zhou J. Aberrant regulation of autophagy in mammalian diseases. Biol Lett (2018) 14:20170540. doi: 10.1098/rsbl.2017.0540
78. Lloberas N, Cruzado JM, Franquesa M, Herrero-Fresneda I, Torras J, Alperovich G, et al. Mammalian target of rapamycin pathway blockade slows progression of diabetic kidney disease in rats. J Am Soc Nephrol (2006) 17:1395–404. doi: 10.1681/ASN.2005050549
79. Mori H, Inoki K, Masutani K, Wakabayashi Y, Komai K, Nakagawa R, et al. The mTOR pathway is highly activated in diabetic nephropathy and rapamycin has a strong therapeutic potential. Biochem Biophys Res Commun (2009) 384:471–5. doi: 10.1016/j.bbrc.2009.04.136
80. Cai X, Zou F, Xuan R, Lai XY. Exosomes from mesenchymal stem cells expressing microribonucleic acid-125b inhibit the progression of diabetic nephropathy via the tumour necrosis factor receptor-associated factor 6/Akt axis. Endocr J (2021) 68:817–28. doi: 10.1507/endocrj.EJ20-0619
81. Border WA, Noble NA. Transforming growth factor beta in tissue fibrosis. N Engl J Med (1994) 331:1286–92. doi: 10.1056/NEJM199411103311907
82. Zhang Y, Jin D, Kang X, Zhou R, Sun Y, Lian F, et al. Signaling pathways involved in diabetic renal fibrosis. Front Cell Dev Biol (2021) 9:696542. doi: 10.3389/fcell.2021.696542
83. Xu BH, Sheng J, You YK, Huang XR, Ma RCW, Wang Q, et al. Deletion of Smad3 prevents renal fibrosis and inflammation in type 2 diabetic nephropathy. Metabolism (2020) 103:154013. doi: 10.1016/j.metabol.2019.154013
Keywords: diabetic kidney disease, mesenchymal stem cells, extracellular vesicles, signaling pathway, end-stage renal disease
Citation: Lin Y, Yang Q, Wang J, Chen X, Liu Y and Zhou T (2022) An overview of the efficacy and signaling pathways activated by stem cell-derived extracellular vesicles in diabetic kidney disease. Front. Endocrinol. 13:962635. doi: 10.3389/fendo.2022.962635
Received: 06 June 2022; Accepted: 06 July 2022;
Published: 28 July 2022.
Edited by:
Dr. Vinod Tiwari, Indian Institute of Technology (BHU), IndiaReviewed by:
Laureano D. Asico, George Washington University, United StatesMaria Felice Brizzi, University of Turin, Italy
Copyright © 2022 Lin, Yang, Wang, Chen, Liu and Zhou. This is an open-access article distributed under the terms of the Creative Commons Attribution License (CC BY). The use, distribution or reproduction in other forums is permitted, provided the original author(s) and the copyright owner(s) are credited and that the original publication in this journal is cited, in accordance with accepted academic practice. No use, distribution or reproduction is permitted which does not comply with these terms.
*Correspondence: Tianbiao Zhou, zhoutb@aliyun.com