- National Clinical Research Center for Metabolic Diseases, Key Laboratory of Diabetes Immunology (Central South University), Ministry of Education, and Department of Metabolism and Endocrinology, The Second Xiangya Hospital of Central South University, Changsha, China
Latent autoimmune diabetes in adults (LADA) is a heterogeneous disease sharing some phenotypic, genetic, and immunological features with both type 1 and 2 diabetes. Patients with LADA have a relatively slow autoimmune process and more residual islet β-cell function at onset, allowing a time window to protect residual islet β cells and delay or inhibit disease progression. It is crucial to discover various heterogeneous factors affecting islet β-cell function for precise LADA therapy. In this review, we first describe the natural history of LADA. Thereafter, we summarize β-cell function-related heterogeneous factors in LADA, including the age of onset, body mass index, genetic background, and immune, lifestyle, and environmental factors. In parallel, we evaluate the impact of current hypoglycemic agents and immune intervention therapies for islet β-cell protection. Finally, we discuss the opportunities and challenges of LADA treatment from the perspective of islet β-cell function protection.
1 Introduction
Diabetes is generally regarded as a disease spectrum ranging from classic insulin-resistant type 2 diabetes to classic insulin-dependent type 1 diabetes (1). Latent autoimmune diabetes in adults (LADA) is a heterogeneous form of diabetes with common clinical features of both type 1 and 2 diabetes (2, 3). It is also known as type 1.5 diabetes or slowly progressive insulin-dependent type 1 diabetes. LADA accounts for approximately 2%–14% of all diabetes patients (4). Latest data have shown that the number of LADA among the Chinese population has exceeded 10 million (5, 6), being the largest number of LADA patients in the world.
There is no universal agreement on LADA classification, diagnosis, and management. It is called slowly evolving immune-mediated diabetes and is classified as a type of hybrid forms of diabetes in the Classification of Diabetes Mellitus 2019 of the World Health Organization (7). However, it is also classified as a subtype of type 1 diabetes because of its autoimmune destruction of islet β cells according to the Diagnosis and Classification of Diabetes Mellitus of the American Diabetes Association in 2022 (8). Currently, the exact underlying mechanisms of LADA are poorly understood, and specific etiological treatment of LADA remains an issue.
Loss of islet β-cell function due to autoimmunity is the key factor in LADA occurrence and progression, and individuals with LADA have more residual islet β cells at onset compared with classic type 1 diabetes (9, 10). After diagnosis, the ability to retain residual β-cell function is heterogeneous, being influenced by the age of onset, genetics, and immune mechanisms. A comprehensive understanding of the influencing factors and different protection methods of islet β-cell function is clinically significant to further explore how to effectively protect islet β cells and delay disease progression. Herein, we summarize related heterogeneous factors for islet β-cell protection. Then, we evaluate the impact of current hypoglycemic agents and immune intervention therapies for islet β cells. Finally, we discuss the opportunities and challenges of LADA treatment from the perspective of islet β-cell function protection.
2 Natural history of LADA
The traditional natural history of type 1 diabetes can conceptually be divided into six stages. The stages are genetic predisposition; an action of the precipitating event; overt immunologic abnormalities with normal insulin release; progressive loss of insulin release with normal glucose level; overt diabetes with present C-peptide; and complete β-cell destruction (no C-peptide) (11). LADA, as a subtype of type 1 diabetes, has a similar natural course (Figure 1). However, because of the heterogeneity of β-cell function in LADA patients, the course has two special features. One, pancreatic β-cell function declines faster in LADA patients than in those with type 2 diabetes but slower than that of classic type 1 diabetes (12). Another, several previous studies have shown that levels of C-peptide in LADA patients continued to decline rapidly when followed up for 3 or 6 years (13, 14). The decline pattern of β-cell function in LADA was biphasic, showing an initial rapid progression followed by a stable mode (15).
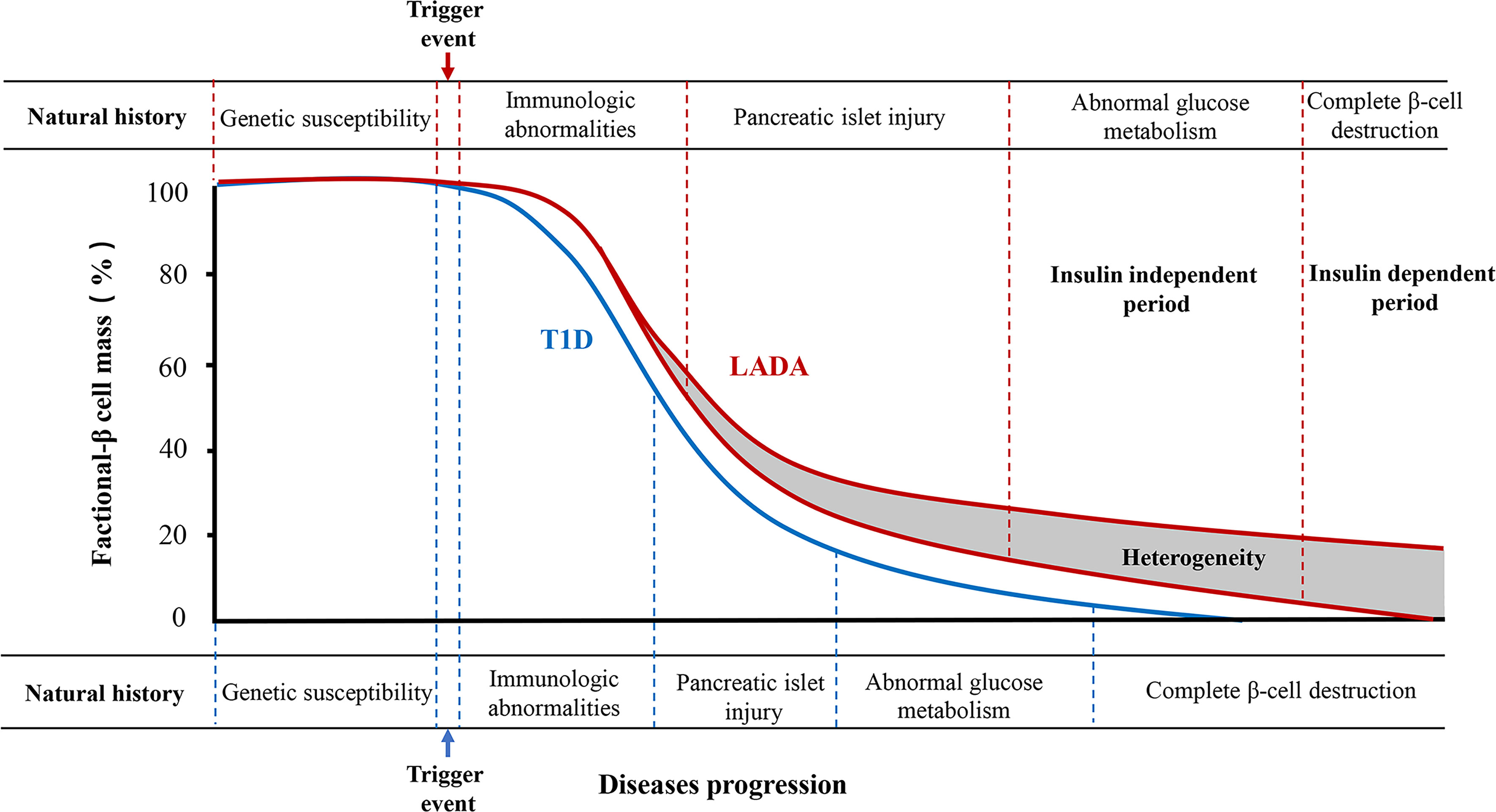
Figure 1 The natural history of type 1 diabetes and LADA. Type 1 diabetes can be divided into six stages. LADA has a similar natural course to type 1 diabetes as its autoimmune subtype. However, the rate of decline of pancreatic β-cell function is lower than that of type 1 diabetes. Moreover, the natural history of most LADA patients can be divided into insulin-independent and insulin-dependent periods according to the degree of pancreatic β-cell destruction. There is great heterogeneity in the progression to insulin dependence in patients with LADA, which is associated with the age of onset, body mass index, genetic background, and immune, lifestyle, and environmental factors.
The clinical course of most LADA patients can be divided into insulin-independent and insulin-dependent stages (16). The insulin-independent stage is the early clinical stage of LADA, with similar clinical features to type 2 diabetes. No typical clinical symptoms are present in this stage. Oral hypoglycemic agents are capable of controlling blood glucose at this stage. Due to presence of heterogeneity, there are LADA patients whose diabetes can also be controlled with diet alone, weight loss, and an exercise program, without any oral medications. These are stable forms that can last for decades and require insulin therapy only very late, when LADA patients’ islet β-cell function becomes significantly impaired and leads to diabetic ketosis or acidosis. Additionally, insulin therapy becomes necessary, and patients thereafter enter the insulin-dependent stage.
3 Heterogeneity of LADA and islet β-cell function
LADA exhibits significant clinical heterogeneity. Patients with LADA have different clinical, immunological, and genetic characteristics when compared with type 1 and 2 diabetes. The main parameter differences among them are summarized in Table 1 (2, 14). These factors of clinical heterogeneity are also related to islet β-cell function in patients with LADA.
3.1 Age of onset
LADA patients with different ages of onset show different clinical features and islet β-cell function. Accumulating evidence shows that individuals with LADA that are younger at disease onset have a lower level of insulin resistance and worse residual β-cell function than those with an older age of onset (16, 17). A recent study has indicated that LADA patients with the old age of onset (≥60 years) tend to have higher fasting C-peptide levels and 2-h postprandial C-peptide levels compared with those with a young age of onset (<60 years) (17). Therefore, the age of LADA onset may be associated with islet β-cell destruction, with patients with the old age of onset having more residual β-cell mass and better islet β-cell function.
3.2 Body mass index
Overweight/obesity is a risk indicator for patients with LADA (18, 19). Data indicate that 66% of LADA falls into the overweight/obesity category (20). Although patients with LADA are leaner compared with type 2 diabetes patients, LADA-China study has shown that the median body mass index (BMI) was 24.5 kg/m2 in LADA patients, and the proportion of overweight and obese patients was up to 41.7% (9). Emerging evidence has demonstrated a significant correlation between BMI and islet β-cell demise and dysfunction. Compared with classic type 1 diabetes, LADA patients are more obese and have a larger waist circumference, lower low-density lipoprotein cholesterol levels, and more residual islet β cells (21). Moreover, obese LADA patients show a lower frequency of insulin dependence and have better β-cell function (22, 23). The reason why β-cell depletion is slower in LADA patients with higher waist circumference is unclear. Perhaps, adipose tissue-induced insulin resistance increases fasting glucose levels earlier compared to lean LADA patients, leading to prompt management of the disease (24). Additionally, diabetic obese mice with leptin receptor knockout causing hyperleptinemia show that obesity may delay the onset of insulin dependence (25). This concept diverges from the “accelerator hypothesis,” which refers to the onset of the autoimmune reaction itself. The “accelerator hypothesis” argues that intrinsic nature, insulin resistance, and autoimmunity can accelerate islet β-cell loss through apoptosis in diabetes patients (26). Obesity may enhance insulin resistance and islet autoantigen exposure, contributing to the initiation of autoimmune destructive processes (27).
3.3 Genetic background
Susceptibility genes, as a predictor and player of the disease, play a vital role in the islet β-cell failure in diabetes. Recent studies have verified the genetic overlap between LADA and both type 1 and 2 diabetes (28). Human leukocyte antigen (HLA) class II genes are the most susceptible genes in LADA patients and can mediate the autoimmune response (29, 30). Most type 1 diabetes-associated HLA haplotypes also confer LADA susceptibility (31). A study has revealed that DR9/DR9 is the Chinese-specific LADA risk genotype, while DR3/DR4 is the major risk factor in Caucasians (32). A study of Caucasians has indicated that the DRB1/DQB1 genotype in LADA was associated with the age of onset, and LADA patients with HLA DRB1 and DQB1 developed the disease at a younger age and had severe islet β-cell function (33). Additionally, previous studies have shown that HLA-DQB1 genotypes are most frequent in early-onset classic type 1 diabetes patients who were diagnosed at <20 years, followed by late-onset classic type 1 diabetes patients who were diagnosed at >35 years and LADA patients (34). This further suggests that HLA genes are related to islet β-cell function. Japanese LADA subjects with insulin dependence more often had DRB1*0405-DQB1*0401, DRB1*0802-DQB1*0302, and DRB1*0901-DQB1*0303 haplotypes, whereas only the DRB1*0405-DQB1*0401 haplotype occurred more often in non-insulin-dependence LADA patients, additionally reinforcing the stated point (35).
Some non-HLA genes, including type 1 diabetes-associated variants and type 2 diabetes-associated variants, also have a role in LADA. Insulin (INS) (36), cytotoxic T lymphocyte-associated protein 4 (CTLA4), and SH2B adapter protein 3 (SH2B3) are implicated in LADA pathogenesis (37). PFKFB3 has been linked to immune and metabolic diabetes (30). A previous study has indicated that the PFKFB3 product can regulate glycolysis and insulin pathway (38). A further experiment in mice has revealed that insulin resistance exacerbation and increased adipose tissue inflammation occurred if PFKFB3 expression in adipose tissue was disturbed (39). Additionally, targeted overexpression of the gene can protect against diet-induced insulin resistance and inflammatory responses (40). Moreover, genome-scale in vivo CRISPR screening has identified RNLS as a target for β-cell protection in type 1 diabetes. By deleting RNLS, β cells can become resistant to autoimmune killing in type 1 diabetes mice (41). However, its specific function in LADA remains unclear. Briefly, it can be concluded that LADA patients share a genetic predisposition with type 1 and 2 diabetes.
3.4 Immune mechanisms
LADA is a heterogeneous disease characterized by immune-mediated β-cell destruction. In recent years, multiple studies have reported that innate, cellular, and humoral immunity plays an important role in LADA pathogenesis (42–44).
3.4.1 Innate immunity
A cross talk is believed to exist between β cells and various immune cells from adaptive and innate immunity during the initiation and progression of autoimmune diabetes (45). In LADA occurrence and progression, macrophages, natural killer (NK) cells, dendritic cells (DCs), and neutrophils (NEs) play a crucial role. First, macrophages are present in the pancreas of LADA patients and a rat model, and an increase in interleukin-1β (IL-1β) has been able to recruit more macrophages to infiltrate the pancreas, leading to apoptosis of β cells (46). Other studies have also shown that macrophages infiltrate pancreatic islets and participate in the destruction of β cells (47, 48). A further study has shown that macrophages can produce more IL-1β, and IL-1β may contribute to β-cell destruction (49).
Second, NK cells may also be involved in LADA development. Wang et al. (50) have found a higher number of inducible interferon (IFN)-γ (+) NK cells in newly diagnosed Chinese LADA patients compared to controls. IFN-γ released by NK cells may promote LADA development by affecting islet β cells. The role of NK cells in LADA is not well understood, with some studies reporting a decrease in the number of NK cells, while other studies have indicated an increase in the number of NK cells (51, 52). In a Caucasian population with LADA, the number of CD3-CD56+ NK cells has decreased, unlike that in healthy individuals (52), whereas in Chinese LADA patients, the number of activated NKp46(+) NK cells has significantly increased (50). Interestingly, the expression of NKG2D, a surface-activated receptor for NK cells, has been reduced in type 1 diabetes patients and an animal model (53, 54). In contrast, in patients with LADA, NKG2D expression has been increased, while the expression of killer cell immunoglobulin-like receptor 3DL1 has been decreased (52). This might imply a distinction between LADA patients with slower progression of β-cell injury (52).
DCs are involved in pancreatitis along with macrophages and activate CD4+ T cells via IL-12 secretion (55). Evidence has shown that the proportion of CD123-CD11c+ DCs is lower in patients with LADA than that in patients with type 1 diabetes, implying a slower progression of LADA, unlike in acute type 1 diabetes patients (56). However, to date, few studies have been performed on the importance of DCs in LADA.
Finally, NE counts have shown a gradual increase from type 1 diabetes and LADA to type 2 diabetes and correlated with the number and level of islet β-cell autoantibodies (57). Data have shown that patients positive for glutamic acid decarboxylase antibodies (GADA), protein tyrosine phosphatase 2 antibodies (IA2A), and selective zin transporter 8 (ZnT8A) all had minimal NE counts, suggesting an association between NE and autoimmune dysfunction and β-cell failure in diabetic patients (57). In conclusion, although the exact mechanism of β-cell failure is still unknown, the cross talk between immune cells is slowly becoming elucidated.
3.4.2 Cellular immunity
Insulitis is a hallmark of β-cell immune-mediated dysfunction and is characterized by various immune cell infiltrates, consisting of CD8+ cytotoxic T cells, CD4+ T cells, and B cells (58, 59). Insulitis exists in the pancreas of LADA patients, as indicated by pancreatic scintigraphy (60), and it has been verified in isolated pancreatic tissue and LADA animal model (46, 61). Rolandsson et al. (62) noted that type 2 diabetes patients with antigen-specific reactive T cells and no autoantibodies were termed as T-LADA (Ab-T+). T-LADA (Ab-T+) patients have worse β-cell function distinct from classic type 2 diabetes (Ab-T-) (63). The latest study also indicated that T cell-mediated autoimmunity plays an important role in β-cell destruction and worse glycemic control (64), implying that altered proportions and functional defects of T-cell subsets are an important cause of autoimmunity in LADA. Studies found that decreased regulatory T cells in LADA mediate β-cell damage (65). Further experiments have revealed that the expression level of forkhead box protein 3 (FOXP3) is downregulated in peripheral CD4+ T cells, and this FOXP3 expression is regulated by STAT3-mediated epigenetic silencing through HDAC5 and DNA methyltransferase 3b (66, 67). In addition to T-cell subsets, B-cell subsets also have an alteration, in which the size of the marginal zone B is increased while the number of follicular B and IL-10-producing regulatory B (B10) cells is decreased. This change has been associated with the loss of self-tolerance and the destruction of islet β cells (68). A previous study has suggested that B10 cells can exert anti-inflammatory effects and maintain peripheral tolerance by secreting IL-10 in type 1 diabetes patients (69).
3.4.3 Humoral immunity
Humoral immunity is primarily reflected in the presence of islet autoantibodies in the serum of patients with LADA. In fact, autoimmunity to islet β cells precedes the onset of LADA by several years. A prospective study has verified this phenomenon in nearly 60% of LADA patients (70). GADA, as one of the most potent autoantibodies and sensitive diagnostic markers, is involved in β cell-specific autoimmunity (71). The discussion on whether GADA levels can predict the functional exhaustion of β cell has lasted for years without reaching definitive conclusions. Some believe that GADA antibodies have high predictive power (72), while others do not (73). Furthermore, a transient increase in circulating GADA levels may result from body weight gain or β-cell stress, not necessarily indicating an underlying ongoing autoimmune process. Quantitative data on antibody levels should make it easier to decide whether patients have true LADA or a mere surge in antibody levels due to metabolic decompensation. Additionally, the affinity and identifying specific epitopes of islet autoantibodies help improve the prediction of insulin deficiency (74). The electrochemiluminescence (ECL) assay is a promising islet autoantibody detection method that can precisely discriminate between high-affinity and high-risk specific autoantibodies, leading to an earlier identification of islet autoimmunity initiation before clinically evident diabetes (75). According to ECL analysis, ECL-GAD65 antibody-positive participants resemble type 1 diabetes patients with slender body size and poor β-cell function, whereas ECL-GAD65 antibody-negative patients have a similar phenotype to type 2 diabetes individuals with good β-cell mass and a slow rate of insulin insufficiency (74). The presence of IA-2A is also effective in predicting the future need for insulin therapy (76). A report indicated that among seven IA-2 constructs, the IA-2 (256–760) fragment was analyzed as the most sensitive marker for detecting humoral IA-2 immunoreactivity in patients with LADA (77). Moreover, as verified in an animal model, the reduced activity of pancreatic β cells ZnT8 can contribute to impaired β-cell function and accelerate diabetes related to islet amyloidosis (78). Recently, a new autoantibody of LADA, tetraspanin 7 autoantibodies (TSPAN7A), has been investigated. TSPAN7A is a valid islet autoantibody for use in East Asian populations suffering from LADA and can discriminate against individuals with LADA who have a lower β-cell function after disease progression (79).
3.5 Environmental factors
Environmental factors may also contribute to LADA occurrence and progression. Numerous studies have indicated that physical activity, smoking, drinking, sweetened beverages, coffee intake, viruses, and gut microbiota are related to disorders in glucose metabolism, insulin sensitivity, and autoimmune destruction of LADA (80–83). High physical activity can regulate metabolism and increase immunity (80). Individuals who exercise daily have a three-fold lower risk of developing LADA compared to those who exercise less than once a week (81). Notably, the association between smoking and LADA incidence has been contradictory. A 22-year follow-up study found that heavy smoking can suppress autoimmunity in a Norwegian population with LADA (84), while some data from a Swedish case–control study indicated that heavy smoking increases the risk of LADA compared with never-smokers who have high levels of homeostatic model assessment of insulin resistance and β-cell function and lower levels of GADA (85). Additionally, certain evidence has suggested that smoking or nicotine has a biphasic action, which depends on patients’ different smoking statuses (86, 87). Further studies have indicated that former and current smoking is associated with high or low islet β-cell function, respectively (88).
Recently, an emerging study has demonstrated a significant link between gut microbiota and autoimmune targeting of islet β cells (83). LADA patients exhibit various gut microbiota and metabolic profiles compared with healthy subjects and classic type 1 and 2 diabetes patients. Furthermore, data have suggested that patients with LADA have a decrease in short-chain fatty acid (SCFA)-producing bacteria. SCFA-producing bacteria can regulate intestinal hormones, increase insulin sensitivity, and attenuate islet β-cell destruction (89, 90). Additionally, acetate and butyrate derived from non-obese diabetic mice gut microbes have been demonstrated to serve as protective factors to inhibit insulitis development. In contrast, acetate and butyrate in the diet decrease the proportion of autoreactive T cells, increase regulatory T-cell counts, enhance the function of regulatory T cells, and boost gut integrity (91). A further study has shown that the transfer of microbiota to pancreatic lymph nodes triggered the intracellular protein receptor nucleotide-binding oligomerization domain-containing 2 (NOD2) activation and contributed to the onset of type 1 diabetes (92). Remarkably, this suggests that gut dysbiosis in patients with autoimmune diabetes may contribute to the onset and progression of the disease, but this requires further high-quality evidence to confirm it.
4 Hypoglycemic agents and islet β-cell protection
Currently, for LADA drug treatment, some hypoglycemic agents can prolong the duration of the insulin-independent stage progression to the insulin-dependent stage. Achieving strict glycemic management is the basis of preventing or postponing preserved β-cell destruction and inhibiting the development of diabetic complications in LADA patients.
4.1 Insulin
Certain studies have reported that insulin therapy is rational and essential for intervention in progressive β-cell deterioration in LADA patients, especially in patients with the early onset and more preserved β-cell function (93–95). The possible reason for protecting β-cell function using insulin in LADA patients may be due to a decrease in antigen expression and autoimmune attack on β cells as a result of exogenous insulin inhibition of β-cell activity (94). Furthermore, exogenous insulin may lead to immune tolerance and inhibit subsequent immune pathways, thereby protecting residual β cells (94). Additionally, exogenous insulin prevents the accumulation of amylin-positive amyloid in β cells, which is considered one of the most important pathogenic mechanisms of β-cell failure (94). One study reported that therapy with intensive insulin in newly diagnosed type 2 diabetic patients has a great significance for the recovery and maintenance of β-cell function (96). We speculate that intensive insulin therapy can protect islet β-cell function in LADA patients. However, to date, studies on insulin intensification in patients with LADA have not been reported.
4.2 Insulin sensitizer
Metformin, an insulin sensitizer, is the most commonly used hypoglycemic drug. Patients with LADA who have not yet progressed to the insulin-dependent stage often utilize metformin to control their blood glucose levels. However, it has been shown that metformin does not delay or halt the progressive deterioration of β-cell function in youth with impaired glucose tolerance or newly diagnosed type 2 diabetes (97). In patients with LADA, this is unclear, and further studies are needed.
Thiazolidinediones (TZDs), as a stronger insulin sensitizer, have anti-inflammatory activity on β cells and can increase their survival during the non-insulin-dependent stage of LADA. For example, Brooks-Worrell et al. (98) treated T-LADA patients with rosiglitazone for 3 years and found that the levels of IFN-γ and IL-12 secreted by autoreactive T cells in the intervention group were lower than those in the control group, suggesting that rosiglitazone can delay islet failure by inhibiting autoreactive T cells. Moreover, clinical studies in China showed that rosiglitazone alone or in combination with insulin can maintain C-peptide levels and protect islet β-cell function in LADA patients (99). In animal models, TZDs have protected the structure and tissue integrity of pancreatic islet cells, ameliorated oxidative stress, reduced apoptosis stimulation, and promoted the proliferation of islet β cells, thereby improving insulin secretion and regulating blood glucose levels (71). Regretfully, the side effects of the cardiovascular risks of this drug have extremely limited its use in patients with LADA.
4.3 Dipeptidyl-peptidase-4 inhibitors
Dipeptidyl-peptidase-4 inhibitors (DPP-4is) have exhibited a promising role in β-cell function protection in LADA patients. A randomized controlled trial has observed the changes in T-cell phenotype, downregulation of messenger RNA expression, and improved glycemic control in LADA patients after 12 months of treatment with sitagliptin (100). Similarly, other studies have concluded that saxagliptin increases islet β-cell function in patients with LADA (101–103). Moreover, recent studies have shown that saxagliptin combined with vitamin D has a beneficial effect on islet β cells (104, 105). Mechanistically, DPP-4is may influence the control of glucose metabolism in patients with LADA. Further, DPP-4is increase glucagon-like peptide-1 levels and inhibit glucagon levels, thereby boosting insulin secretion after glucose loading through the activation of DPP-4 receptors. Additionally, DPP-4 receptors have also been expressed on the surface of T lymphocytes, and studies have suggested that they are associated with immune regulation, which might have a significant role in delaying and stopping β-cell immune destruction in LADA (101, 106–108).
4.4 Glucagon‐like peptide-1 receptor agonists
Glucagon-like peptide-1 receptor agonists (GLP-1RAs), including liraglutide and dulaglutide, have been approved to treat type 2 diabetes and obesity. They elicit robust improvements in glycemic control and weight loss, combined with cardioprotection in individuals at risk of or with preexisting cardiovascular disease (109). GLP-1RAs seem to have a potential role in the treatment of patients with overweight or obese LADA. A post-hoc analysis of the AWARD-2, -4, and -5 trials has found that dulaglutide can reduce glycated hemoglobin (HbA1c) levels in patients with LADA (110). Recently, liraglutide combined with an anti-IL-21 antibody has been shown to preserve islet β cells in adults with recent-onset type 1 diabetes (111). A further study has found that continuous infusion of GLP-1RA in non-obese diabetic mice can reduce β-cell apoptosis, promote β-cell regeneration, and delay disease development (112). In vitro, GLP-1RA can suppress cell apoptosis and enhance glucose response in freshly isolated human islets (113). However, whether GLP-1RAs can preserve islet β-cell function in LADA patients requires further confirmation by a randomized controlled clinical trial.
4.5 Sodium-glucose cotransporter 2 inhibitors
Sodium-glucose cotransporter 2 inhibitors (SGLT2is) are a new class of antidiabetic drugs. Research has clearly demonstrated that SGLT2is, such as empagliflozin, canagliflozin, and dapagliflozin, have pleiotropic effects in preventing cardiovascular and chronic kidney diseases beyond their favorable impact on hyperglycemia (114). Studies have reported that SGLT2is can decrease HbA1c levels in patients with type 1 diabetes, and the European Union has approved SGLT2is for the treatment of adult type 1 diabetes patients with poor blood glucose control and obesity (115, 116). However, the roles of SGLT2is in LADA have not been well studied. Recent data have suggested that the cardiorenal protective properties of these new therapies are present even in people without diabetes; thus, the extrapolation of their results on LADA individuals seems reasonable (117). SGLT2is and GLP-1RAs should be considered for the management of people with preserved insulin production and at high cardiovascular risk. The risk of diabetic ketoacidosis with SGLT2i yet requires increased vigilance by clinicians.
5 Immune intervention and islet β-cell protection
Several immune interventions, as an emerging and promising therapeutic approach, aimed to counteract autoimmune responses against β cells and preserve β-cell function are currently being investigated. Glutamic acid decarboxylase (GAD) is a major autoantigen in the process leading to LADA with both a clear cell-mediated immune response to GAD and autoantibodies to GAD. Administration of the GAD65 isoform can prevent autoimmune destruction of pancreatic β cells in non-obese diabetic mice and the subsequent need for exogenous insulin replacement (118–124). In phase I and II studies, an alum-formulated vaccine (Diamyd) has shown to be safe, and in a dose-finding study in LADA patients, 20 mcg of Diamyd has been given subcutaneously 1 month apart, indicating the preservation of residual insulin secretion (125, 126). Additionally, a double-blind, multicenter study has found a good safety and tolerability profile for multiple subcutaneous doses of HSP60 peptide (DiaPep277) in LADA patients (71). With this promising background, further studies are coming.
There is increasing attention to the non-mineral metabolism function of vitamin D. Evidence has shown that the vitamin D system may be involved in autoimmunity pathogenesis (127). Our previous research has shown the protective effects of 1-alpha-hydroxyvitamin D3 on residual β-cell function in patients with LADA (128). The use of immunotherapeutic agents in a combination therapy appears to be a valid approach to obtaining better results in terms of β-cell mass and function preservation. DPP-4is combined with vitamin D3 can delay the loss of β cells and improve endogenous insulin production in patients with new-onset type 1 diabetes and LADA (104, 129, 130). The main findings on the use of combination therapy with vitamin D and DPP-4is in patients with autoimmune diabetes have been summarized elsewhere (131). Vitamin D may boost anti-inflammatory response, enhance immunomodulatory effects, induce immune tolerance, and potentially stimulate insulin synthesis and secretion. Additionally, DPP-4is have been shown to develop similar actions on the innate and adaptive immune systems. The co-administration of both vitamin D and DPP-4is may substantially strengthen the efficacy of each compound as immunomodulators.
Over the past two decades, research has identified multiple immune cell types and soluble factors destroying insulin-producing β cells in type 1 diabetes. Effective immunotherapies to treat type 1 diabetes are currently under development. Bluestone et al. (132) have summarized current results for targeting complementary nodes in immunotherapies that have shown efficacy in patients with type 1 diabetes, as well as additional efforts in next-generation immune therapeutics. These immune therapeutics included cytokine antagonists, cytokine agonists, T-cell activation inhibitors, T effector cell depletion and exhaustion therapy, checkpoint agonists, regulatory cell-based therapy, B-cell antagonists, microbiome therapy, and autoantigen therapy. Whether these immunotherapies can be used for LADA and their effect of protecting β-cell function are questions expected to be answered in future research. LADA represents an ideal model for exploring immunotherapy of autoimmunity and β-cell function because it is typically associated with a lower and less severe immune-mediated β-cell destruction compared to type 1 diabetes. Therefore, LADA offers a wider window to test immune interventions that may slow down a β-cell failure.
6 Conclusions and future perspectives
Currently, there is an urgent need for an optimal treatment scheme for LADA patients. This scheme should not only achieve good blood glucose control but also actively protect islet β cell cells and their function and delay and prevent complications as long as possible. The importance of therapeutic methods in protecting islet β-cell function in LADA is attracting significant attention. This article highlighted the risk factors and protection aspects of islet β cells in LADA according to the age of onset, BMI, environmental factors, genetic background, immune mechanisms, hypoglycemic agents, and immune therapeutics (Figure 2). To date, delaying islet β-cell exhaustion and precision treatment of LADA still face many challenges. Over the past two decades, research has identified multiple immune cell types and soluble factors that destroy insulin-producing β cells. These insights into disease pathogenesis have enabled the development of therapies to prevent and modify LADA. Furthermore, research concentrating on β-cell protection treatment options can provide potential opportunities for β-cell protection and reversal of diabetes, such as β-cell dedifferentiation, regeneration, and β-cell replacement.
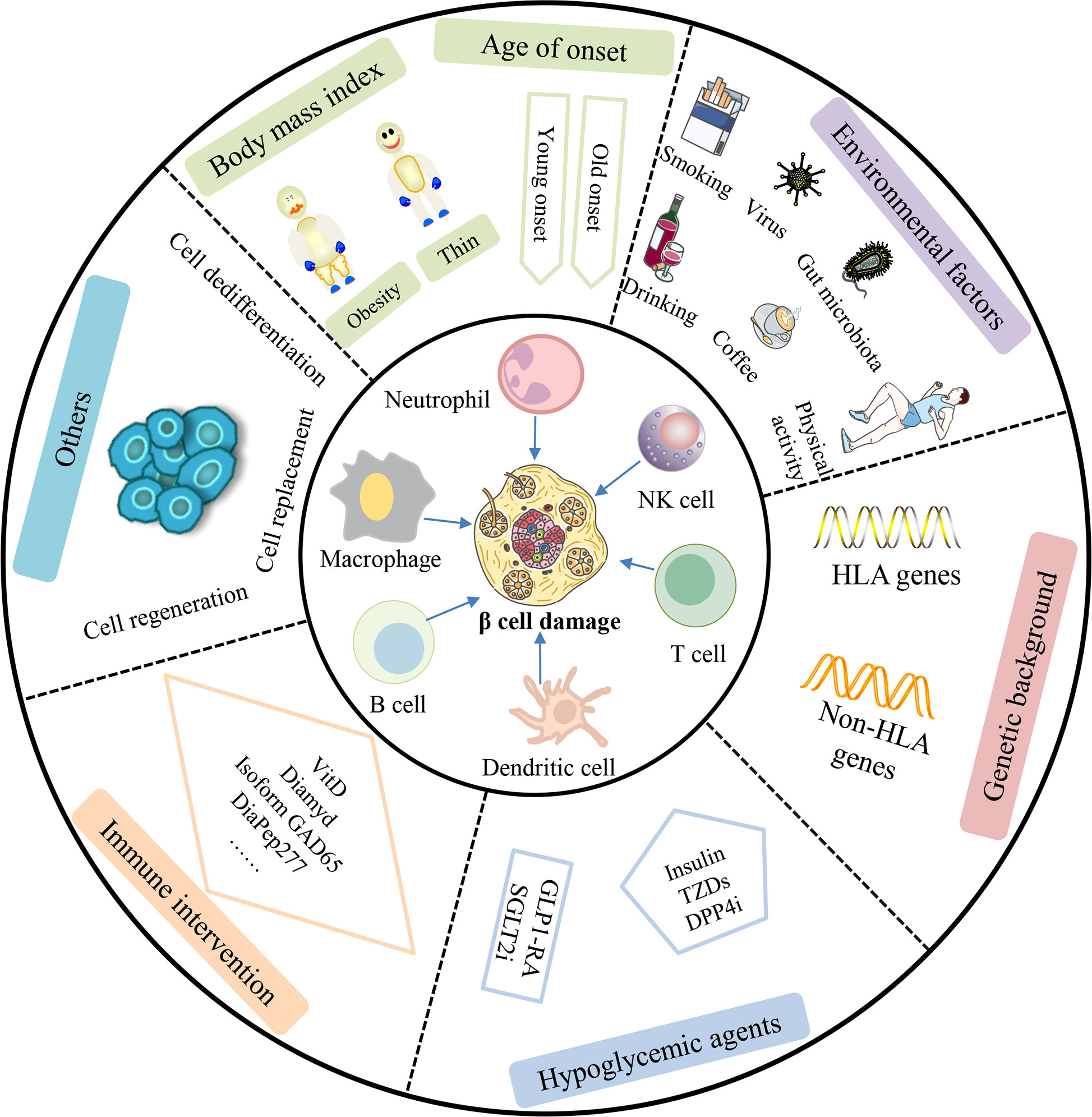
Figure 2 Comprehensive factors affecting β-cell protection and therapy in LADA patients. β-cell function in LADA patients can be affected by cellular, humoral, and innate immunity. Additionally, studies have shown that age of onset, body mass index, environmental factors, genetic background, hypoglycemic agents, and immune intervention can also play an important role in β-cell damage in patients with LADA. In the future, β-cell dedifferentiation, regeneration, and β-cell replacement may be a promising approach to cure LADA patients. NK cell, natural killer cell; TZDs, thiazolidinediones; DPP4i, dipeptidyl-peptidase-4 inhibitor; GLP1-RA, glucagon-like peptide-1 receptor agonist; SGLT2i, sodium-glucose cotransporter 2 inhibitor.
There is a great need for additional studies exploring the protection of islet β cells in LADA prevention and treatment. Several studies have supported the development of β-like cells by transplantation in vitro (133). Human pluripotent stem cell-derived pancreatic islets have received widespread attention as a promising cellular resource for diabetes therapy. A recent study has indicated that transoral injection of human chemo-induced pluripotent stem cells into non-human primates with diabetes can significantly recover the secretion of endogenous insulin and improve glycemic management (134). Utilizing current evolving and extensive technologies and platforms to study human β cells will permit a more elaborate investigation of the underlying mechanisms and will also stimulate the further advancement of therapeutic methods dedicated to human β-cell quality and function (135). The progress in stem cell biology and transplant site engineering has provided both innovative sources of cellular material and improved transplantation approaches. Frontier methods to guard against xenograft rejection and relapsing autoimmunity are also included (136). However, there is still a long distance to cross to the application of these to the therapy of β cells in diabetic patients.
Author contributions
WY performed the paper search and wrote the first draft of the manuscript. SL, ZX, ZZW, and BL critically revised the text and provided substantial scientific contributions. SL and ZGZ proposed the project and revised the manuscript. SL is the guarantor of this work; thus, SL had full access to all study data and took responsibility for the integrity of the data and the accuracy of the data analysis. All authors contributed to the article and approved the submitted version.
Funding
This study was supported by the Hunan Province Natural Science Funds for Distinguished Young Scholars (Grant No. 2020JJ2053), the key projects supported by the Hunan Health Commission (Grant No. 202103060904), and the independent exploration and innovation projects for postgraduate of Central South University (Grant Nos. 2021zzts1052, 2021zzts0365).
Conflict of interest
The authors declare that the research was conducted in the absence of any commercial or financial relationships that could be construed as a potential conflict of interest.
Publisher’s note
All claims expressed in this article are solely those of the authors and do not necessarily represent those of their affiliated organizations, or those of the publisher, the editors and the reviewers. Any product that may be evaluated in this article, or claim that may be made by its manufacturer, is not guaranteed or endorsed by the publisher.
References
1. Association Diabetes Association. 2. classification and diagnosis of diabetes: Standards of medical care in diabetes-2021. Diabetes Care (2021), S15–s33, 44(Suppl 1). doi: 10.2337/dc21-S002
2. Buzzetti R, Zampetti S, Maddaloni E. Adult-onset autoimmune diabetes: Current knowledge and implications for management. Nat Rev Endocrinology (2017) 13(11):674–86. doi: 10.1038/nrendo.2017.99
3. Buzzetti R, Tuomi T, Mauricio D, Pietropaolo M, Zhou Z, Pozzilli P, et al. Management of latent autoimmune diabetes in adults: A consensus statement from an international expert panel. Diabetes (2020) 69(10):2037–47. doi: 10.2337/dbi20-0017
4. Liu B, Xiang Y, Liu Z, Zhou Z. Past, present and future of latent autoimmune diabetes in adults. Diabetes/metabolism Res Rev (2020) 36(1):e3205. doi: 10.1002/dmrr.3205
5. Li Y, Teng D, Shi X, Qin G, Qin Y, Quan H, et al. Prevalence of diabetes recorded in mainland China using 2018 diagnostic criteria from the American diabetes association: National cross sectional study. Bmj (2020) 369:m997. doi: 10.1136/bmj.m997
6. Tang X, Yan X, Zhou H, Yang X, Niu X, Liu J, et al. Prevalence and identification of type 1 diabetes in Chinese adults with newly diagnosed diabetes. Diabetes Metab syndrome Obes (2019) 12:1527–41. doi: 10.2147/DMSO.S202193
7. Organization WH. Classification of diabetes mellitus. Geneva: World Health Organization (2019). Licence: CC BY-NC-SA 3.0 IGO. 2019.
8. Draznin B, Aroda VR, Bakris G, Benson G, Brown FM, Freeman R, et al. 2. classification and diagnosis of diabetes: Standards of medical care in diabetes-2022. Diabetes Care (2022) 45(Suppl 1):S17–s38. doi: 10.2337/dc22-S002
9. Zhou Z, Xiang Y, Ji L, Jia W, Ning G, Huang G, et al. Frequency, immunogenetics, and clinical characteristics of latent autoimmune diabetes in China (LADA China study): A nationwide, multicenter, clinic-based cross-sectional study. Diabetes (2013) 62(2):543–50. doi: 10.2337/db12-0207
10. Katsarou A, Gudbjörnsdottir S, Rawshani A, Dabelea D, Bonifacio E, Anderson BJ, et al. Type 1 diabetes mellitus. Nat Rev Dis Primers (2017) 3:17016. doi: 10.1038/nrdp.2017.16
11. Eisenbarth GS. Type I diabetes mellitus. a chronic autoimmune disease. N Engl J Med (1986) 314(21):1360–8. doi: 10.1056/NEJM198605223142106
12. Yang L, Zhou ZG, Huang G, Ouyang LL, Li X, Yan X. Six-year follow-up of pancreatic beta cell function in adults with latent autoimmune diabetes. World J Gastroenterol (2005) 11(19):2900–5. doi: 10.3748/wjg.v11.i19.2900
13. Johansen OE, Boehm BO, Grill V, Torjesen PA, Bhattacharya S, Patel S, et al. C-peptide levels in latent autoimmune diabetes in adults treated with linagliptin versus glimepiride: Exploratory results from a 2-year double-blind, randomized, controlled study. Diabetes Care (2014) 37(1):e11–2. doi: 10.2337/dc13-1523
14. Liu L, Li X, Xiang Y, Huang G, Lin J, Yang L, et al. Latent autoimmune diabetes in adults with low-titer GAD antibodies: Similar disease progression with type 2 diabetes: A nationwide, multicenter prospective study (LADA China study 3). Diabetes Care (2015) 38(1):16–21. doi: 10.2337/dc14-1770
15. Li X, Chen Y, Xie Y, Xiang Y, Yan X, Huang G, et al. Decline pattern of beta-cell function in adult-onset latent autoimmune diabetes: An 8-year prospective study. J Clin Endocrinol Metab (2020) 105(7):2331–40. doi: 10.1210/clinem/dgaa205
16. Turner R, Stratton I, Horton V, Manley S, Zimmet P, Mackay IR, et al. UKPDS 25: Autoantibodies to islet-cell cytoplasm and glutamic acid decarboxylase for prediction of insulin requirement in type 2 diabetes. UK prospective diabetes study group. Lancet (1997) 350(9087):1288–93. doi: 10.1016/S0140-6736(97)03062-6
17. Niu X, Luo S, Li X, Xie Z, Xiang Y, Huang G, et al. Identification of a distinct phenotype of elderly latent autoimmune diabetes in adults: LADA China study 8. Diabetes/metabolism Res Rev (2019) 35(1):e3068. doi: 10.1002/dmrr.3068
18. Maddaloni E, Moretti C, Mignogna C, Buzzetti R. Adult-onset autoimmune diabetes in 2020: An update. Maturitas (2020) 137:37–44. doi: 10.1016/j.maturitas.2020.04.014
19. Hjort R, Ahlqvist E, Carlsson PO, Grill V, Groop L, Martinell M, et al. Overweight, obesity and the risk of LADA: Results from a Swedish case-control study and the Norwegian HUNT study. Diabetologia (2018) 61(6):1333–43. doi: 10.1007/s00125-018-4596-0
20. Adeleye OO, Ogbera AO, Fasanmade O, Ogunleye OO, Dada AO, Ale AO, et al. Latent autoimmune diabetes mellitus in adults (LADA) and it's characteristics in a subset of nigerians initially managed for type 2 diabetes. Int Arch Med (2012) 5(1):23. doi: 10.1186/1755-7682-5-23
21. Hawa MI, Kolb H, Schloot N, Beyan H, Paschou SA, Buzzetti R, et al. Adult-onset autoimmune diabetes in Europe is prevalent with a broad clinical phenotype: Action LADA 7. Diabetes Care (2013) 36(4):908–13. doi: 10.2337/dc12-0931
22. Zaharia OP, Bobrov P, Strassburger K, Bódis K, Karusheva Y, Scholz M, et al. Metabolic characteristics of recently diagnosed adult-onset autoimmune diabetes mellitus. J Clin Endocrinol Metab (2018) 103(2):429–37. doi: 10.1210/jc.2017-01706
23. Hernandez M, Mollo A, Marsal JR, Esquerda A, Capel I, Puig-Domingo M, et al. Insulin secretion in patients with latent autoimmune diabetes (LADA): Half way between type 1 and type 2 diabetes: Action LADA 9. BMC endocrine Disord (2015) 15:1. doi: 10.1186/1472-6823-15-1
24. Castelblanco E, Hernández M, Castelblanco A, Gratacòs M, Esquerda A, Molló À, et al. Low-grade inflammatory marker profile may help to differentiate patients with LADA, classic adult-onset type 1 diabetes, and type 2 diabetes. Diabetes Care (2018) 41(4):862–8. doi: 10.2337/dc17-1662
25. Suriano F, Vieira-Silva S, Falony G, Roumain M, Paquot A, Pelicaen R, et al. Novel insights into the genetically obese (Ob/Ob) and diabetic (Db/Db) mice: Two sides of the same coin. Microbiome (2021) 9(1):147. doi: 10.1186/s40168-021-01097-8
26. Wilkin TJ. The accelerator hypothesis: Weight gain as the missing link between type I and type II diabetes. Diabetologia (2001) 44(7):914–22. doi: 10.1007/s001250100548
27. Fourlanos S, Harrison LC, Colman PG. The accelerator hypothesis and increasing incidence of type 1 diabetes. Curr Opin Endocrinol Diabetes Obes (2008) 15(4):321–5. doi: 10.1097/MED.0b013e3283073a5a
28. Andersen MK. New insights into the genetics of latent autoimmune diabetes in adults. Curr Diabetes Rep (2020) 20(9):43. doi: 10.1007/s11892-020-01330-y
29. Bennabi M, Gaman A, Delorme R, Boukouaci W, Manier C, Scheid I, et al. HLA-class II haplotypes and autism spectrum disorders. Sci Rep (2018) 8(1):7639. doi: 10.1038/s41598-018-25974-9
30. Cousminer DL, Ahlqvist E, Mishra R, Andersen MK, Chesi A, Hawa MI, et al. First genome-wide association study of latent autoimmune diabetes in adults reveals novel insights linking immune and metabolic diabetes. Diabetes Care (2018) 41(11):2396–403. doi: 10.2337/dc18-1032
31. Pettersen E, Skorpen F, Kvaløy K, Midthjell K, Grill V. Genetic heterogeneity in latent autoimmune diabetes is linked to various degrees of autoimmune activity: Results from the nord-trøndelag health study. Diabetes (2010) 59(1):302–10. doi: 10.2337/db09-0923
32. Luo S, Lin J, Xie Z, Xiang Y, Zheng P, Huang G, et al. HLA genetic discrepancy between latent autoimmune diabetes in adults and type 1 diabetes: LADA China study no. 6. J Clin Endocrinol Metab (2016) 101(4):1693–700. doi: 10.1210/jc.2015-3771
33. Horton V, Stratton I, Bottazzo GF, Shattock M, Mackay I, Zimmet P, et al. Genetic heterogeneity of autoimmune diabetes: Age of presentation in adults is influenced by HLA DRB1 and DQB1 genotypes (UKPDS 43). UK prospective diabetes study (UKPDS) group. Diabetologia (1999) 42(5):608–16. doi: 10.1007/s001250051202
34. Andersen MK, Lundgren V, Turunen JA, Forsblom C, Isomaa B, Groop PH, et al. Latent autoimmune diabetes in adults differs genetically from classical type 1 diabetes diagnosed after the age of 35 years. Diabetes Care (2010) 33(9):2062–4. doi: 10.2337/dc09-2188
35. Takeda H, Kawasaki E, Shimizu I, Konoue E, Fujiyama M, Murao S, et al. Clinical, autoimmune, and genetic characteristics of adult-onset diabetic patients with GAD autoantibodies in Japan (Ehime study). Diabetes Care (2002) 25(6):995–1001. doi: 10.2337/diacare.25.6.995
36. Zhang N, Huang W, Dong F, Liu Y, Zhang B, Jing L, et al. Insulin gene VNTR polymorphisms -2221mspi and -23hphi are associated with type 1 diabetes and latent autoimmune diabetes in adults: A meta-analysis. Acta diabetologica (2015) 52(6):1143–55. doi: 10.1007/s00592-015-0805-1
37. Dong F, Yang G, Pan HW, Huang WH, Jing LP, Liang WK, et al. The association of PTPN22 Rs2476601 polymorphism and CTLA-4 Rs231775 polymorphism with LADA risks: A systematic review and meta-analysis. Acta diabetologica (2014) 51(5):691–703. doi: 10.1007/s00592-014-0613-z
38. Duran J, Obach M, Navarro-Sabate A, Manzano A, Gómez M, Rosa JL, et al. Pfkfb3 is transcriptionally upregulated in diabetic mouse liver through proliferative signals. FEBS J (2009) 276(16):4555–68. doi: 10.1111/j.1742-4658.2009.07161.x
39. Huo Y, Guo X, Li H, Wang H, Zhang W, Wang Y, et al. Disruption of inducible 6-Phosphofructo-2-Kinase ameliorates diet-induced adiposity but exacerbates systemic insulin resistance and adipose tissue inflammatory response. J Biol Chem (2010) 285(6):3713–21. doi: 10.1074/jbc.M109.058446
40. Huo Y, Guo X, Li H, Xu H, Halim V, Zhang W, et al. Targeted overexpression of inducible 6-Phosphofructo-2-Kinase in adipose tissue increases fat deposition but protects against diet-induced insulin resistance and inflammatory responses. J Biol Chem (2012) 287(25):21492–500. doi: 10.1074/jbc.M112.370379
41. Cai EP, Ishikawa Y, Zhang W, Leite NC, Li J, Hou S, et al. Genome-scale In vivo CRISPR screen identifies RNLS as a target for beta cell protection in type 1 diabetes. Nat Metab (2020) 2(9):934–45. doi: 10.1038/s42255-020-0254-1
42. Strom A, Menart B, Simon MC, Pham MN, Kolb H, Roden M, et al. Cellular interferon-γ and interleukin-13 immune reactivity in type 1, type 2 and latent autoimmune diabetes: Action LADA 6. Cytokine (2012) 58(2):148–51. doi: 10.1016/j.cyto.2012.01.002
43. Sachdeva N, Paul M, Badal D, Kumar R, Jacob N, Dayal D, et al. Preproinsulin specific CD8+ T cells in subjects with latent autoimmune diabetes show lower frequency and different pathophysiological characteristics than those with type 1 diabetes. Clin Immunol (2015) 157(1):78–90. doi: 10.1016/j.clim.2015.01.005
44. Mayer A, Fabien N, Gutowski MC, Dubois V, Gebuhrer L, Bienvenu J, et al. Contrasting cellular and humoral autoimmunity associated with latent autoimmune diabetes in adults. Eur J endocrinology (2007) 157(1):53–61. doi: 10.1530/EJE-07-0060
45. Lehuen A, Diana J, Zaccone P, Cooke A. Immune cell crosstalk in type 1 diabetes. Nat Rev Immunol (2010) 10(7):501–13. doi: 10.1038/nri2787
46. Jörns A, Wedekind D, Jähne J, Lenzen S. Pancreas pathology of latent autoimmune diabetes in adults (LADA) in patients and in a LADA rat model compared with type 1 diabetes. Diabetes (2020) 69(4):624–33. doi: 10.2337/db19-0865
47. Jansen A, Homo-Delarche F, Hooijkaas H, Leenen PJ, Dardenne M, Drexhage HA. Immunohistochemical characterization of monocytes-macrophages and dendritic cells involved in the initiation of the insulitis and beta-cell destruction in NOD mice. Diabetes (1994) 43(5):667–75. doi: 10.2337/diab.43.5.667
48. Walker R, Bone AJ, Cooke A, Baird JD. Distinct macrophage subpopulations in pancreas of prediabetic BB/E rats. possible role for macrophages in pathogenesis of IDDM. Diabetes (1988) 37(9):1301–4. doi: 10.2337/diab.37.9.1301
49. Lopez-Castejon G, Brough D. Understanding the mechanism of IL-1β secretion. Cytokine Growth Factor Rev (2011) 22(4):189–95. doi: 10.1016/j.cytogfr.2011.10.001
50. Wang Y, Yuan W, Guo H, Jiang Y. High frequency of activated NKp46(+) natural killer cells in patients with new diagnosed of latent autoimmune diabetes in adults. Autoimmunity (2015) 48(4):267–73. doi: 10.3109/08916934.2014.990629
51. Willcox A, Richardson SJ, Bone AJ, Foulis AK, Morgan NG. Analysis of islet inflammation in human type 1 diabetes. Clin Exp Immunol (2009) 155(2):173–81. doi: 10.1111/j.1365-2249.2008.03860.x
52. Akesson C, Uvebrant K, Oderup C, Lynch K, Harris RA, Lernmark A, et al. Altered natural killer (NK) cell frequency and phenotype in latent autoimmune diabetes in adults (LADA) prior to insulin deficiency. Clin Exp Immunol (2010) 161(1):48–56. doi: 10.1111/j.1365-2249.2010.04114.x
53. Rodacki M, Svoren B, Butty V, Besse W, Laffel L, Benoist C, et al. Altered natural killer cells in type 1 diabetic patients. Diabetes (2007) 56(1):177–85. doi: 10.2337/db06-0493
54. Ogasawara K, Hamerman JA, Hsin H, Chikuma S, Bour-Jordan H, Chen T, et al. Impairment of NK cell function by NKG2D modulation in NOD mice. Immunity (2003) 18(1):41–51. doi: 10.1016/S1074-7613(02)00505-8
55. Yoon JW, Jun HS. Autoimmune destruction of pancreatic beta cells. Am J Ther (2005) 12(6):580–91. doi: 10.1097/01.mjt.0000178767.67857.63
56. Singh K, Martinell M, Luo Z, Espes D, Stålhammar J, Sandler S, et al. Cellular immunological changes in patients with LADA are a mixture of those seen in patients with type 1 and type 2 diabetes. Clin Exp Immunol (2019) 197(1):64–73. doi: 10.1111/cei.13289
57. Huang J, Xiao Y, Zheng P, Zhou W, Wang Y, Huang G, et al. Distinct neutrophil counts and functions in newly diagnosed type 1 diabetes, latent autoimmune diabetes in adults, and type 2 diabetes. Diabetes/metabolism Res Rev (2019) 35(1):e3064. doi: 10.1002/dmrr.3064
58. Ilonen J, Lempainen J, Veijola R. The heterogeneous pathogenesis of type 1 diabetes mellitus. Nat Rev Endocrinology (2019) 15(11):635–50. doi: 10.1038/s41574-019-0254-y
59. Leete P, Willcox A, Krogvold L, Dahl-Jørgensen K, Foulis AK, Richardson SJ, et al. Differential insulitic profiles determine the extent of β-cell destruction and the age at onset of type 1 diabetes. Diabetes (2016) 65(5):1362–9. doi: 10.2337/db15-1615
60. Signore A, Capriotti G, Chianelli M, Bonanno E, Galli F, Catalano C, et al. Detection of insulitis by pancreatic scintigraphy with 99mtc-labeled IL-2 and MRI in patients with LADA (Action LADA 10). Diabetes Care (2015) 38(4):652–8. doi: 10.2337/dc14-0580
61. Shimada A, Imazu Y, Morinaga S, Funae O, Kasuga A, Atsumi Y, et al. T-Cell insulitis found in anti-GAD65+ diabetes with residual beta-cell function. A Case Rep Diabetes Care (1999) 22(4):615–7. doi: 10.2337/diacare.22.4.615
62. Rolandsson O, Palmer JP. Latent autoimmune diabetes in adults (LADA) is dead: Long live autoimmune diabetes! Diabetologia (2010) 53(7):1250–3. doi: 10.1007/s00125-010-1713-0
63. Liang H, Cheng Y, Tang W, Cui Q, Yuan J, Huang G, et al. Clinical manifestation and islet β-cell function of a subtype of latent autoimmune diabetes in adults (LADA): Positive for T cell responses in phenotypic type 2 diabetes. Acta diabetologica (2019) 56(11):1225–30. doi: 10.1007/s00592-019-01391-w
64. Brooks-Worrell B, Hampe CS, Hattery EG, Palomino B, Zangeneh SZ, Utzschneider K, et al. Islet autoimmunity is highly prevalent and associated with diminished β-cell function in patients with type 2 diabetes in the grade study. Diabetes (2022) 71:1261–71. doi: 10.2337/figshare.18621602.v1
65. Wang X, Yang L, Cheng Y, Liang H, Hu J, Zheng P, et al. Downregulation of T-cell transcription factors in adult latent autoimmune diabetes with high-titer glutamic acid decaroxylase antibody. Diabetes Ther (2019) 10(3):917–27. doi: 10.1007/s13300-019-0594-6
66. Li Y, Zhao M, Hou C, Liang G, Yang L, Tan Y, et al. Abnormal DNA methylation in CD4+ T cells from people with latent autoimmune diabetes in adults. Diabetes Res Clin practice (2011) 94(2):242–8. doi: 10.1016/j.diabres.2011.07.027
67. Hou C, Zhong Y, Wang Z, Ming Z, Huang G, Ouyang L, et al. STAT3-mediated epigenetic silencing of FOXP3 in LADA T cells is regulated through HDAC5 and DNMT1. Clin Immunol (2018) 191:116–25. doi: 10.1016/j.clim.2017.12.001
68. Deng C, Xiang Y, Tan T, Ren Z, Cao C, Huang G, et al. Altered peripheral b-lymphocyte subsets in type 1 diabetes and latent autoimmune diabetes in adults. Diabetes Care (2016) 39(3):434–40. doi: 10.2337/dc15-1765
69. El-Mokhtar MA, Elsherbiny NM, Sayed D, Raafat DM, Askar E, Hussein A, et al. Altered regulatory b cell subsets in children with type 1 diabetes mellitus. J Immunol Res (2020) 2020:8935694. doi: 10.1155/2020/8935694
70. Carlsson S, Midthjell K, Grill V. Influence of family history of diabetes on incidence and prevalence of latent autoimmune diabetes of the adult: Results from the nord-trøndelag health study. Diabetes Care (2007) 30(12):3040–5. doi: 10.2337/dc07-0718
71. Cernea S, Buzzetti R, Pozzilli P. Beta-cell protection and therapy for latent autoimmune diabetes in adults. Diabetes Care (2009) 32 Suppl 2Suppl 2:S246–52. doi: 10.2337/dc09-S317
72. van Deutekom AW, Heine RJ, Simsek S. The islet autoantibody titres: Their clinical relevance in latent autoimmune diabetes in adults (LADA) and the classification of diabetes mellitus. Diabetic Med (2008) 25(2):117–25. doi: 10.1111/j.1464-5491.2007.02316.x
73. Maioli M, Pes GM, Delitala G, Puddu L, Falorni A, Tolu F, et al. Number of autoantibodies and HLA genotype, more than high titers of glutamic acid decarboxylase autoantibodies, predict insulin dependence in latent autoimmune diabetes of adults. Eur J endocrinology (2010) 163(4):541–9. doi: 10.1530/EJE-10-0427
74. Zhu Y, Qian L, Liu Q, Zou J, Zhou Y, Yang T, et al. Glutamic acid decarboxylase autoantibody detection by electrochemiluminescence assay identifies latent autoimmune diabetes in adults with poor islet function. Diabetes Metab J (2020) 44(2):260–6. doi: 10.4093/dmj.2019.0007
75. Yu L. Islet autoantibody detection by electrochemiluminescence (ECL) assay. Methods Mol Biol (2016) 1433:85–91. doi: 10.1007/7651_2015_296
76. Bottazzo GF, Bosi E, Cull CA, Bonifacio E, Locatelli M, Zimmet P, et al. IA-2 antibody prevalence and risk assessment of early insulin requirement in subjects presenting with type 2 diabetes (UKPDS 71). Diabetologia (2005) 48(4):703–8. doi: 10.1007/s00125-005-1691-9
77. Tiberti C, Giordano C, Locatelli M, Bosi E, Bottazzo GF, Buzzetti R, et al. Identification of tyrosine phosphatase 2(256-760) construct as a new, sensitive marker for the detection of islet autoimmunity in type 2 diabetic patients: The non-insulin requiring autoimmune diabetes (NIRAD) study 2. Diabetes (2008) 57(5):1276–83. doi: 10.2337/db07-0874
78. Xu J, Wijesekara N, Regeenes R, Rijjal DA, Piro AL, Song Y, et al. Pancreatic β cell-selective zinc transporter 8 insufficiency accelerates diabetes associated with islet amyloidosis. JCI Insight (2021) 6(10):e143037. doi: 10.1172/jci.insight.143037
79. Shi X, Huang G, Wang Y, Liu Z, Deng C, Li X, et al. Tetraspanin 7 autoantibodies predict progressive decline of beta cell function in individuals with LADA. Diabetologia (2019) 62(3):399–407. doi: 10.1007/s00125-018-4799-4
80. Hjort R, Ahlqvist E, Andersson T, Alfredsson L, Carlsson PO, Grill V, et al. Physical activity, genetic susceptibility, and the risk of latent autoimmune diabetes in adults and type 2 diabetes. J Clin Endocrinol Metab (2020) 105(11):e4112–23. doi: 10.1210/clinem/dgaa549
81. Carlsson S. Environmental (Lifestyle) risk factors for LADA. Curr Diabetes Rev (2019) 15(3):178–87. doi: 10.2174/1573399814666180716150253
82. Lofvenborg JE, Andersson T, Carlsson PO, Dorkhan M, Groop L, Martinell M, et al. Coffee consumption and the risk of latent autoimmune diabetes in adults–results from a Swedish case-control study. Diabetic Med (2014) 31(7):799–805. doi: 10.1111/dme.12469
83. Fang Y, Zhang C, Shi H, Wei W, Shang J, Zheng R, et al. Characteristics of the gut microbiota and metabolism in patients with latent autoimmune diabetes in adults: A case-control study. Diabetes Care (2021) 44(12):2738–46. doi: 10.2337/dc20-2975
84. Rasouli B, Grill V, Midthjell K, Ahlbom A, Andersson T, Carlsson S. Smoking is associated with reduced risk of autoimmune diabetes in adults contrasting with increased risk in overweight men with type 2 diabetes: A 22-year follow-up of the HUNT study. Diabetes Care (2013) 36(3):604–10. doi: 10.2337/dc12-0913
85. Rasouli B, Andersson T, Carlsson PO, Grill V, Groop L, Martinell M, et al. Smoking and the risk of LADA: Results from a Swedish population-based case-control study. Diabetes Care (2016) 39(5):794–800. doi: 10.2337/dc15-2348
86. Wang C, Wang Y, Wu J, Liu S, Zhu Y, Lv S, et al. Current smoking dose-dependently associated with decreased β-cell function in Chinese men without diabetes. J Diabetes Res (2015) 2015:841768. doi: 10.1155/2015/841768
87. Ostgren CJ, Lindblad U, Ranstam J, Melander A, Råstam L. Associations between smoking and beta-cell function in a non-hypertensive and non-diabetic population. skaraborg hypertension and diabetes project. Diabetic Med (2000) 17(6):445–50. doi: 10.1046/j.1464-5491.2000.00294.x
88. Daniel M, Cargo MD. Association between smoking, insulin resistance and beta-cell function in a north-Western first nation. Diabetic Med (2004) 21(2):188–93. doi: 10.1046/j.1464-5491.2003.01064.x
89. Kim CH. Microbiota or short-chain fatty acids: Which regulates diabetes? Cell Mol Immunol (2018) 15(2):88–91. doi: 10.1038/cmi.2017.57
90. Canfora EE, Meex RCR, Venema K, Blaak EE. Gut microbial metabolites in obesity, NAFLD and T2DM. Nat Rev Endocrinology (2019) 15(5):261–73. doi: 10.1038/s41574-019-0156-z
91. Mariño E, Richards JL, McLeod KH, Stanley D, Yap YA, Knight J, et al. Gut microbial metabolites limit the frequency of autoimmune T cells and protect against type 1 diabetes. Nat Immunol (2017) 18(5):552–62. doi: 10.1038/ni.3713
92. Costa FR, Françozo MC, de Oliveira GG, Ignacio A, Castoldi A, Zamboni DS, et al. Gut microbiota translocation to the pancreatic lymph nodes triggers NOD2 activation and contributes to T1D onset. J Exp Med (2016) 213(7):1223–39. doi: 10.1084/jem.20150744
93. Thunander M, Thorgeirsson H, Törn C, Petersson C, Landin-Olsson M. β-cell function and metabolic control in latent autoimmune diabetes in adults with early insulin versus conventional treatment: A 3-year follow-up. Eur J endocrinology (2011) 164(2):239–45. doi: 10.1530/EJE-10-0901
94. Kobayashi T, Maruyama T, Shimada A, Kasuga A, Kanatsuka A, Takei I, et al. Insulin intervention to preserve beta cells in slowly progressive insulin-dependent (Type 1) diabetes mellitus. Ann New York Acad Sci (2002) 958:117–30. doi: 10.1111/j.1749-6632.2002.tb02954.x
95. Maruyama T, Tanaka S, Shimada A, Funae O, Kasuga A, Kanatsuka A, et al. Insulin intervention in slowly progressive insulin-dependent (Type 1) diabetes mellitus. J Clin Endocrinol Metab (2008) 93(6):2115–21. doi: 10.1210/jc.2007-2267
96. Weng J, Li Y, Xu W, Shi L, Zhang Q, Zhu D, et al. Effect of intensive insulin therapy on beta-cell function and glycaemic control in patients with newly diagnosed type 2 diabetes: A multicentre randomised parallel-group trial. Lancet (2008) 371(9626):1753–60. doi: 10.1016/S0140-6736(08)60762-X
97. RISE Consortium. Impact of insulin and metformin versus metformin alone on β-cell function in youth with impaired glucose tolerance or recently diagnosed type 2 diabetes. Diabetes Care (2018) 41(8):1717–25. doi: 10.2337/dc18-0787
98. Brooks-Worrell BM, Palmer JP. Attenuation of islet-specific T cell responses is associated with c-peptide improvement in autoimmune type 2 diabetes patients. Clin Exp Immunol (2013) 171(2):164–70. doi: 10.1111/cei.12012
99. Yang Z, Zhou Z, Li X, Huang G, Lin J. Rosiglitazone preserves islet beta-cell function of adult-onset latent autoimmune diabetes in 3 years follow-up study. Diabetes Res Clin practice (2009) 83(1):54–60. doi: 10.1016/j.diabres.2008.09.044
100. Zhou Z, Wang X, Yang L, Cheng Y. Altered T-cell subsets and transcription factors in latent autoimmune diabetes in adults taking sitagliptin, a dipeptidyl peptidase-4 inhibitor: A 1-year open-label randomized controlled trial. Acta diabetologica (2019) 10(2):375–82. doi: 10.1111/jdi.12873
101. Awata T, Shimada A, Maruyama T, Oikawa Y, Yasukawa N, Kurihara S, et al. Possible long-term efficacy of sitagliptin, a dipeptidyl peptidase-4 inhibitor, for slowly progressive type 1 diabetes (SPIDDM) in the stage of non-Insulin-Dependency: An open-label randomized controlled pilot trial (SPAN-s). Diabetes Ther (2017) 8(5):1123–34. doi: 10.1007/s13300-017-0299-7
102. Buzzetti R, Pozzilli P, Frederich R, Iqbal N, Hirshberg B. Saxagliptin improves glycaemic control and c-peptide secretion in latent autoimmune diabetes in adults (LADA). J Clin Endocrinol Metab (2016) 32(3):289–96. doi: 10.1002/dmrr.2717
103. Hals IK, Fiskvik Fleiner H, Reimers N, Astor MC, Filipsson K, Ma Z, et al. Investigating optimal β-Cell-Preserving treatment in latent autoimmune diabetes in adults: Results from a 21-month randomized trial. Diabetes Obes Metab (2019) 21(10):2219–27. doi: 10.1111/dom.13797
104. Zhang Z, Yan X, Wu C, Pei X, Li X, Wang X, et al. Adding vitamin D3 to the dipeptidyl peptidase-4 inhibitor saxagliptin has the potential to protect β-cell function in LADA patients: A 1-year pilot study. Diabetes/metabolism Res Rev (2020) 36(5):e3298. doi: 10.1002/dmrr.3298
105. Rapti E, Karras S, Grammatiki M, Mousiolis A, Tsekmekidou X, Potolidis E, et al. Combined treatment with sitagliptin and vitamin d in a patient with latent autoimmune diabetes in adults. Endocrinology Diabetes Metab Case Rep (2016) 2016:150136. doi: 10.1530/EDM-15-0136
106. Pieralice S, Pozzilli P. Latent autoimmune diabetes in adults: A review on clinical implications and management. Diabetes Metab J (2018) 42(6):451–64. doi: 10.4093/dmj.2018.0190
107. D'Alessio DA, Denney AM, Hermiller LM, Prigeon RL, Martin JM, Tharp WG, et al. Treatment with the dipeptidyl peptidase-4 inhibitor vildagliptin improves fasting islet-cell function in subjects with type 2 diabetes. J Clin Endocrinol Metab (2009) 94(1):81–8. doi: 10.1210/jc.2008-1135
108. Zhu LQ, Liu YH, Huang M, Wei H, Liu Z. [Study on improvement of islet beta cell function in patients with latent autoimmune diabetes mellitus in adults by integrative Chinese and Western medicine]. Zhongguo Zhong Xi Yi Jie He Za Zhi (2004) 24(7):581–4. doi: 10.3321/j.issn:1003-5370.2004.07.001
109. Baggio LL, Drucker DJ. Glucagon-like peptide-1 receptor Co-agonists for treating metabolic disease. Mol Metab (2021) 46:101090. doi: 10.1016/j.molmet.2020.101090
110. Pozzilli P, Leslie RD, Peters AL, Buzzetti R, Shankar SS, Milicevic Z, et al. Dulaglutide treatment results in effective glycaemic control in latent autoimmune diabetes in adults (LADA): A post-hoc analysis of the AWARD-2, -4 and -5 trials. Diabetes Obes Metab (2018) 20(6):1490–8. doi: 10.1111/dom.13237
111. von Herrath M, Bain SC, Bode B, Clausen JO, Coppieters K, Gaysina L, et al. Anti-Interleukin-21 antibody and liraglutide for the preservation of β-cell function in adults with recent-onset type 1 diabetes: A randomised, double-blind, placebo-controlled, phase 2 trial. Lancet Diabetes Endocrinol (2021) 9(4):212–24. doi: 10.1016/S2213-8587(21)00019-X
112. Pettus J, Hirsch I, Edelman S. GLP-1 agonists in type 1 diabetes. Clin Immunol (2013) 149(3):317–23. doi: 10.1016/j.clim.2013.04.006
113. Farilla L, Bulotta A, Hirshberg B, Li Calzi S, Khoury N, Noushmehr H, et al. Glucagon-like peptide 1 inhibits cell apoptosis and improves glucose responsiveness of freshly isolated human islets. Endocrinology (2003) 144(12):5149–58. doi: 10.1210/en.2003-0323
114. Zelniker TA, Wiviott SD, Raz I, Im K, Goodrich EL, Bonaca MP, et al. SGLT2 inhibitors for primary and secondary prevention of cardiovascular and renal outcomes in type 2 diabetes: A systematic review and meta-analysis of cardiovascular outcome trials. Lancet (2019) 393(10166):31–9. doi: 10.1016/S0140-6736(18)32590-X
115. Chen MB, Xu RJ, Zheng QH, Zheng XW, Wang H. Efficacy and safety of sotagliflozin adjuvant therapy for type 1 diabetes mellitus: A systematic review and meta-analysis. Medicine (2020) 99(33):e20875. doi: 10.1097/MD.0000000000020875
116. Mathieu C, Dandona P, Birkenfeld AL, Hansen TK, Iqbal N, Xu J, et al. Benefit/risk profile of dapagliflozin 5 mg in the DEPICT-1 and -2 trials in individuals with type 1 diabetes and body mass index ≥27 Kg/M(2). Diabetes Obes Metab (2020) 22(11):2151–60. doi: 10.1111/dom.14144
117. Koufakis T, Vas P, Kotsa K. Treating latent autoimmune diabetes in adults in the era of cardiovascular outcomes trials: Old dog should learn new tricks. Diabetic Med (2021) 38(3):e14496. doi: 10.1111/dme.14496
118. Tisch R, Yang XD, Singer SM, Liblau RS, Fugger L, McDevitt HO. Immune response to glutamic acid decarboxylase correlates with insulitis in non-obese diabetic mice. Nature (1993) 366(6450):72–5. doi: 10.1038/366072a0
119. Petersen JS, Karlsen AE, Markholst H, Worsaae A, Dyrberg T, Michelsen B. Neonatal tolerization with glutamic acid decarboxylase but not with bovine serum albumin delays the onset of diabetes in NOD mice. Diabetes (1994) 43(12):1478–84. doi: 10.2337/diab.43.12.1478
120. Pleau JM, Fernandez-Saravia F, Esling A, Homo-Delarche F, Dardenne M. Prevention of autoimmune diabetes in nonobese diabetic female mice by treatment with recombinant glutamic acid decarboxylase (GAD 65). Clin Immunol Immunopathol (1995) 76(1 Pt 1):90–5. doi: 10.1006/clin.1995.1092
121. Tian J, Atkinson MA, Clare-Salzler M, Herschenfeld A, Forsthuber T, Lehmann PV, et al. Nasal administration of glutamate decarboxylase (GAD65) peptides induces Th2 responses and prevents murine insulin-dependent diabetes. J Exp Med (1996) 183(4):1561–7. doi: 10.1084/jem.183.4.1561
122. Yoon JW, Yoon CS, Lim HW, Huang QQ, Kang Y, Pyun KH, et al. Control of autoimmune diabetes in NOD mice by GAD expression or suppression in beta cells. Science (1999) 284(5417):1183–7. doi: 10.1126/science.284.5417.1183
123. Tisch R, Wang B, Weaver DJ, Liu B, Bui T, Arthos J, et al. Antigen-specific mediated suppression of beta cell autoimmunity by plasmid DNA vaccination. J Immunol (2001) 166(3):2122–32. doi: 10.4049/jimmunol.166.3.2122
124. Jun HS, Chung YH, Han J, Kim A, Yoo SS, Sherwin RS, et al. Prevention of autoimmune diabetes by immunogene therapy using recombinant vaccinia virus expressing glutamic acid decarboxylase. Diabetologia (2002) 45(5):668–76. doi: 10.1007/s00125-002-0806-9
125. Agardh CD, Cilio CM, Lethagen A, Lynch K, Leslie RD, Palmér M, et al. Clinical evidence for the safety of GAD65 immunomodulation in adult-onset autoimmune diabetes. J Diabetes its complications (2005) 19(4):238–46. doi: 10.1016/j.jdiacomp.2004.12.003
126. Agardh CD, Lynch KF, Palmér M, Link K, Lernmark A. GAD65 vaccination: 5 years of follow-up in a randomised dose-escalating study in adult-onset autoimmune diabetes. Diabetologia (2009) 52(7):1363–8. doi: 10.1007/s00125-009-1371-2
127. Ma X, Xie Z, Qin J, Luo S, Zhou Z. Association of vitamin d pathway gene CYP27B1 and CYP2R1 polymorphisms with autoimmune endocrine disorders: A meta-analysis. J Clin Endocrinol Metab (2020) 105(11):3575–87. doi: 10.1210/clinem/dgaa525
128. Li X, Liao L, Yan X, Huang G, Lin J, Lei M, et al. Protective effects of 1-Alpha-Hydroxyvitamin D3 on residual beta-cell function in patients with adult-onset latent autoimmune diabetes (LADA). Diabetes/metabolism Res Rev (2009) 25(5):411–6. doi: 10.1002/dmrr.977
129. Pinheiro MM, Pinheiro FM, Torres MA. Four-year clinical remission of type 1 diabetes mellitus in two patients treated with sitagliptin and vitamin D3. Endocrinology Diabetes Metab Case Rep (2016) 2016:16-0099. doi: 10.1530/EDM-16-0099
130. Pinheiro MM, Pinheiro FMM, Trabachin ML. Dipeptidyl peptidase-4 inhibitors (DPP-4i) combined with vitamin D3: An exploration to treat new-onset type 1 diabetes mellitus and latent autoimmune diabetes in adults in the future. Int immunopharmacology (2018) 57:11–7. doi: 10.1016/j.intimp.2018.02.003
131. Pinheiro MM, Pinheiro FMM, Diniz SN, Fabbri A, Infante M. Combination of vitamin d and dipeptidyl peptidase-4 inhibitors (VIDPP-4i) as an immunomodulation therapy for autoimmune diabetes. Int immunopharmacology (2021) 95:107518. doi: 10.1016/j.intimp.2021.107518
132. Bluestone JA, Buckner JH, Herold KC. Immunotherapy: Building a bridge to a cure for type 1 diabetes. Science (2021) 373(6554):510–6. doi: 10.1126/science.abh1654
133. Ding L, Gysemans C, Mathieu C. β-cell differentiation and regeneration in type 1 diabetes. Diabetes Obes Metab (2013) 15 Suppl 3:98–104. doi: 10.1111/dom.12164
134. Du Y, Liang Z, Wang S, Sun D, Wang X, Liew SY, et al. Human pluripotent stem-Cell-Derived islets ameliorate diabetes in non-human primates. Nat Med (2022) 28(2):272–82. doi: 10.1038/s41591-021-01645-7
135. Chen C, Cohrs CM, Stertmann J, Bozsak R, Speier S. Human beta cell mass and function in diabetes: Recent advances in knowledge and technologies to understand disease pathogenesis. Mol Metab (2017) 6(9):943–57. doi: 10.1016/j.molmet.2017.06.019
Keywords: LADA (Latent Autoimmune Diabetes in Adults), autoimmune, β-cell function, type 1 diabetes, heterogeneous disease
Citation: Yin W, Luo S, Xiao Z, Zhang Z, Liu B and Zhou Z (2022) Latent autoimmune diabetes in adults: a focus on β-cell protection and therapy. Front. Endocrinol. 13:959011. doi: 10.3389/fendo.2022.959011
Received: 01 June 2022; Accepted: 14 July 2022;
Published: 05 August 2022.
Edited by:
Jinhua Yan, Third Affiliated Hospital of Sun Yat-sen University, ChinaReviewed by:
Lixin Guo, Peking University, ChinaRaffaella Buzzetti, Sapienza University of Rome, Italy
Alok Kumar, Indiab Foundation, India
Giovanni Mario Pes, University of Sassari, Italy
Marcelo Maia Pinheiro, Centro Universitário de Várzea Grande, Brazil
Copyright © 2022 Yin, Luo, Xiao, Zhang, Liu and Zhou. This is an open-access article distributed under the terms of the Creative Commons Attribution License (CC BY). The use, distribution or reproduction in other forums is permitted, provided the original author(s) and the copyright owner(s) are credited and that the original publication in this journal is cited, in accordance with accepted academic practice. No use, distribution or reproduction is permitted which does not comply with these terms.
*Correspondence: Shuoming Luo, c2h1b21pbmdsdW9AY3N1LmVkdS5jbg==