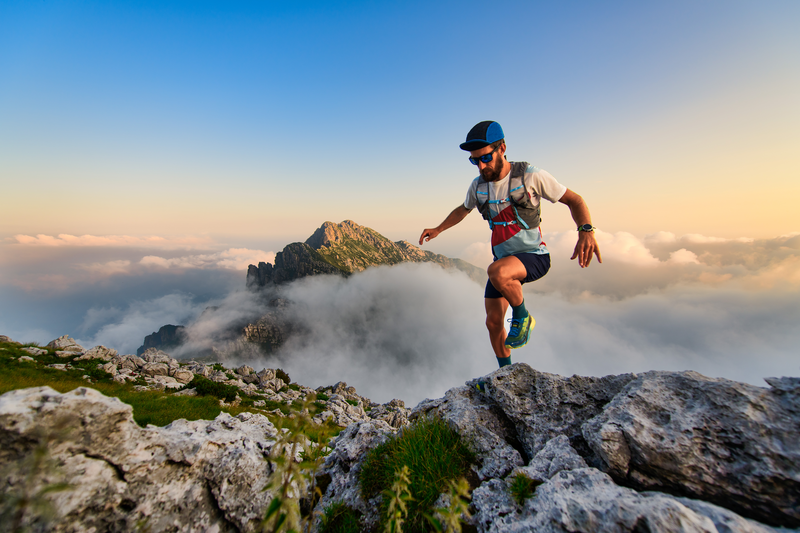
94% of researchers rate our articles as excellent or good
Learn more about the work of our research integrity team to safeguard the quality of each article we publish.
Find out more
ORIGINAL RESEARCH article
Front. Endocrinol. , 17 October 2022
Sec. Bone Research
Volume 13 - 2022 | https://doi.org/10.3389/fendo.2022.948176
This article is part of the Research Topic Bone and Muscle Interactions in Bone Pathologies View all 8 articles
This study aims to clarify the potential role of growth differentiation factor-15 (GDF-15) as a myokine in bone metabolism and muscle function in females with osteoporosis. In total, 45 female participants (71.0 ± 8.5 years) with distal radius fractures were recruited. Participants were classified as healthy/osteopenic (n = 28) (CON) or osteoporotic (n = 17) (OP) according to their T-score from the areal bone mineral density (aBMD) of the femoral neck. Body mass index, upper arm and calf circumferences, and handgrip strength were assessed. Total hip, femoral neck, and lumbar spine aBMD was measured via dual-energy x-ray absorptiometry. The focal bone quality of the distal radius was evaluated via 3D reconstructed computed tomographic images. Serum levels of GDF-15, insulin-like growth factor-1, and inflammatory markers such as tumor necrosis factor-α (TNF-α), interleukin-6, and interleukin-1β (IL-1β), as well as the corresponding mRNA levels in the pronator quadratus muscle were determined. Participants in the OP group had higher serum GDF-15 levels than those in the CON group. The mRNA levels of GDF-15, IL-1β, and TNF-α in the pronator quadratus muscle were significantly higher in the OP group than in the CON one. Levels of both serum GDF-15 and GDF-15 mRNA in muscle were positively correlated with age and negatively associated with the aBMD of the total hip and focal bone quality of the distal radius. Handgrip power was not correlated with circulating GDF-15 levels but was correlated with circumferences of the upper arm and calf, and levels of GDF-15 mRNA in muscle specimens. The mRNA levels of GDF-15 were correlated with those of inflammatory cytokines such as TNF-α and IL-1β. The mRNA levels of TNF-α were associated with circumferences of the upper arm and calf and with the aBMD of the total hip. The mRNA levels of GDF-15 in muscle were correlated with serum levels of GDF-15 and TNF-α. GDF-15 may have associations with bone metabolism in humans via paracrinological and endocrinological mechanisms. Maintenance of muscle mass and function would be influenced more by GDF-15 in muscle than by circulating GDF-15. The role of GDF-15 in bone metabolism and muscle homeostasis could be related to inflammatory responses.
Aging is accompanied by changes in neuromuscular, immunological, and endocrinological functions that may result in decreased muscle and bone mass and frailty in elderly people (1). The concept of “sarco-osteopenia” was proposed to diagnose the coexistence of osteoporosis and sarcopenia (2). Skeletal muscle and bone are the two major components of the musculoskeletal system, and they are closely related mechanically through the lever and pulley system and also have bidirectional chemical interactions involving endocrinological and paracrinological mechanisms (3). Myokines are soluble molecules that are expressed and released by muscle fibers and regulate the biological and pathological activities of cells and organs (4). The various types of myokines that maintain homeostasis between muscle and bone and influence the bone remodeling process have been identified (5). The recent trends in therapeutic approaches to prevent osteoporosis and sarcopenia have focused on this muscle-bone interaction and myokines.
Growth differentiation factor-15 (GDF-15), a member of the transforming growth factor β (TGF-β) superfamily, has been implicated as a biomarker of aging, sarcopenia, muscle wasting, cachexia, mitochondrial disease, decreased physical activity, and energy imbalance (6–15). As a circulating myokine, the serum level of GDF-15 is increased as a result of a metabolic response to muscle-specific mitochondrial stress and muscle dysfunction in humans (9, 16, 17). Increased levels of GDF-15 in skeletal muscle affect metabolic homeostasis, improve insulin resistance, and protect against obesity by inducing lipolysis and oxidative metabolism (16–18). Hence, the importance of GDF-15 activity in maintaining the proper function and metabolic balance of skeletal muscles could be assumed.
Several previous studies revealed links between GDF-15 and the bone remodeling process, but the findings were contradictory. One study showed that GDF-15 was a positive secretory regulator of osteoclastic differentiation in osteocytes under conditions of hypoxia during bone remodeling (19). Another study demonstrated that GDF-15 plays a role in the functional modulation of osteoclast progenitors in response to compressive force (20). Yet another study showed that prostate adenocarcinoma Cell line treated with GDF-15 suppressed the formation of mature osteoclast differentiation in macrophages and bone marrow precursors (21). Furthermore, it was hypothesized that GDF-15 could control osteoclast activity and bone metastasis from osteosarcoma, lung cancer, and prostate cancer in animal models (22–24) and patients with multiple myeloma (25), but those reports also showed conflicting results. Even though the exact mechanisms between GDF-15 and bone remodeling have not been elucidated so far, GDF-15 does play a role in the bone remodeling process.
Previous studies have described the links among GDF-15, osteoclastic activity, and muscle metabolism. The precise role of GDF-15 in skeletal muscle in maintaining bone homeostasis and metabolism, however, remains unclear. To the best of our knowledge, few attempts have been made to reveal the exact role of GDF-15 as a myokine in skeletal muscles in bone-muscle interactions. Therefore, the aim of the current study was to clarify the potential role of GDF-15 as a myokine in bone metabolism and muscle function in patients with osteoporosis.
In total, 45 Korean female participants (mean age 71.0 ± 8.5 years, age range 55–93 years) with distal radius fractures that required surgical treatment were recruited from a tertiary care hospital. All participants were post-menopausal. The participants were subsequently divided into healthy/osteopenic participants (n = 28) (CON) and osteoporotic participants (n = 17) (OP) based on their T-score from the bone mineral density in the femoral neck. An orthopedic surgeon treated all fractures with open reduction and internal fixation using a volar plate fixation system. A trained nurse measured the body mass index (BMI) and circumferences of the upper arm and calf. Participants with a history of taking bone active medications, including bisphosphonates, denosumab, and hormone modulating agents, as well as those with neurodegenerative diseases, rheumatoid arthritis, uncontrolled diabetes mellitus, heart failure, kidney failure, cancer, and thyroid disorder were excluded, as were those who were uncommunicative.
The research protocol was approved by the Institutional Review Board of the University Hospital (KBSMC-2020-07-016). Informed consent was obtained from all participants.
Handgrip strength was measured by a hand dynamometer (Jamar® 5030J1 hydraulic hand dynamometer, Sammons Preston Rolyan, Bolingbrook, IL, USA) with the non-injured contralateral hand by an orthopedic surgeon. The measurement was obtained while the patient sat with the non-injured elbow flexed to 90˚ and the forearm in a neutral position (26). All participants were instructed to perform a handgrip strength test with their maximum grip strength. Handgrip strength was measured three times for each participant, and the average value was subsequently calculated. If the dominant hand was on the injured side, the 10% rule was applied to estimate handgrip strength (27, 28).
The areal bone mineral densities (aBMDs, in grams per square centimeter) of the total hip, femoral neck, and lumbar spine (L1-L4) were assessed with a dual-energy x-ray absorptiometry (DEXA) with a Hologic device (Horizon-W; Hologic Inc., Bedford, MA, USA). The T-scores of the aBMDs of the total hip, femoral neck, and lumbar spine were evaluated based on the value of the aBMDs.
Peripheral venous blood samples from each participant were collected between 8:00 and 11:00 a.m. after fasting overnight to minimize circadian rhythm variabilities. The serum levels of the C-telopeptide of type I collagen (CTx), osteocalcin, insulin-like growth factor-1 (IGF-1), and 25-hydroxyvitamin D were analyzed. Electrochemiluminescence immunoassays were used to measure the levels of CTx (Roche Diagnostics, Basel, Switzerland) and IGF-1 (Siemens Healthcare Diagnostics, NY, USA). A chemiluminescence immunoassay (Roche Diagnostics, Basel, Switzerland) was used to determine the concentration of osteocalcin. The concentration of serum 25-hydroxyvitamin D was assessed with an electrochemiluminescence binding assay (Roche Diagnostics, Basel, Switzerland).
To determine the serum levels of GDF-15, tumor necrosis factor-α (TNF-α), interleukin-6 (IL-6), and interleukin-1β (IL-1β), we used Quantikine ELISA kits (R&D Systems, Minneapolis, MN, USA). All assays were performed in triplicate, and the data were averaged. The intra- and inter-assay coefficients of variation of the GDF-15 assay were 1.8% and 4.7%, respectively, and its sensitivity was 4.39 pg/ml. Those for TNF-α were 2.2% and 7.3%, and its sensitivity was 6.23 pg/ml; those for IL-6 were 1.6% and 3.3%, and its sensitivity was 0.7 pg/ml, and; those for IL-1 β were 2.8% and 4.1%, and its sensitivity was 1.0 pg/ml.
An orthopedic surgeon harvested all muscles during the open reduction and internal fixation procedures of the distal radius fracture. While the patients were under general anesthesia, a 4 cm longitudinal incision was made along the radial border of the flexor carpi radialis (FCR) tendon. The FCR tendon was retracted ulnarly, and the dorsal aspect of the FCR sheath was incised to expose the flexor pollicis longus tendon. The flexor pollicis longus (FPL) tendon was also retracted ulnarly, and the pronator quadratus muscle was exposed. A small part of the pronator quadratus muscle (1 mm x 1 mm x 2 mm) was harvested from all participants during the elevation of the muscle from the distal radius bone. Immediately after harvesting, the muscle specimens were placed in a cryotube, frozen with liquid nitrogen, and stored at -80°C until analysis began.
Total RNA was extracted from the pronator quadratus muscle specimens using QIAZOL Lysis Reagent (QIAGEN, Hilden, Germany) with a homogenizer. Genomic DNA contamination was removed using RNase-free DNase I (QIAGEN, Hilden, Germany). The amount of total RNA was measured using NanoDrop 2000 (Thermo Fisher Scientific Invitrogen Inc., MA, USA). Real-time qPCR reactions were performed with an ABI 7500 (Applied Biosystem, Foster City, CA, USA) using an SYBR Green PCR kit (Takara Bio, Shiga, Japan), and cDNA was synthesized using 1000 μɡ of total RNA (Thermo Fisher Scientific Invitrogen Inc., MA, USA). Each sample was assessed in triplicate and normalized against the expression level of the housekeeping gene GAPDH. All primer sequences including those of GAPDH, GDF-15, IGF-1, and the inflammatory markers TNF-α, IL-6, and IL-1β are reported in Table 1.
Wrist CT images were obtained immediately after the closed reduction of the fracture at the emergency department and before open reduction surgery using a 256-slice multi-detector CT scanner (Brilliance iCT 256, Philips Medical Systems, Amsterdam, Netherlands). The scanning protocol described below was used: 120 kVp tube potential; 149 mAs tube current-time product; 128 mm × 0.625 mm section collimation; 0.5 ms rotation time; 0.4 pitch; 180 mm display field of view; pixel size 0.3 mm × 0.3 mm; and 1 mm section thickness. Corrected coronal, sagittal, and axial images of the wrist were saved as Digital Imaging and Communications in Medicine (DICOM) files. DICOM format files were imported into a 3D reconstruction modeling software (Mimics 22.0, Materialise, Antwerp, Belgium). A 2 cm long cylindrical bone model was constructed 3 cm proximal to the lunate fossa of the radius. A global threshold of 200 Hounsfield units (HU) was applied to the CT scans to obtain a representation of the 3D wrist bone model, and 850 HU was used to reconstruct the cortical bone structures. The average cortical and trabecular HU, total HU, and cortical thickness of the wrist bone models were calculated automatically using the Mimics 22.0® software and 3-matic 14.0 ® software (Materialise, Antwerp, Belgium) (29–31) (Figure 1). To assess inter-examiner reliability, one orthopedic surgeon and one orofacial pain specialist assessed 30 randomly selected CT data, and the analyses from each examiner were compared (inter-examiner) using intraclass correlation coefficient (ICC) to assess the reliability. The ICCs for average HU and cortical thickness were 0.786 and 0.763 with statistical significance, respectively. One examiner repeated the process on 10 randomly selected CT images after 2 weeks (intra-examiner), and the data were compared using ICC, and an acceptable agreement was found. The ICC was 0.816 with statistical significance.
Figure 1 3D reconstructed distal radius bone model: (A) coronal section of the original CT images; dark blue indicated cortical bone and light blue indicated trabecular bone, (B) axial section of the original CT image; dark blue indicated cortical bone and light blue indicated trabecular bone, (C) reconstructed bone model.
The Shapiro-Wilk normality test revealed that the data from the present study were normally distributed, and parametric tests were applied. In comparisons of the parameters of the CON and OP groups, the independent t-test and Chi-square test were used for continuous and categorical variables, respectively. To determine the relationships between the variables, Pearson’s correlation coefficient was applied. All values were considered significant when P < 0.05.
The age (P = 0.144) between the two groups was not statistically different. On the other hand, BMI (P = 0.044), handgrip strength (P = 0.004), and the circumferences of the upper arm and calf (P = 0.047) showed significant differences between groups. The aBMD and T-scores of the total hip (P < 0.001), femoral neck (P < 0.001), and lumbar spine (P < 0.001) also demonstrated significant differences between groups, respectively. Significant differences were also found in the total HU (P < 0.001), cortical HU (P < 0.001), cortical thickness (P = 0.005), and trabecular HU (P < 0.001) of 3D reconstructed bone models of the distal radius. Participants in OP had significantly lower grip power, aBMD of the total hip, femoral neck, lumbar spine, and focal bone quality of the distal radius (Table 2).
Table 2 Differences of demographic features, BMD, and local radial forearm bone quality between two groups.
The serum levels of CTx (P = 0.904), osteocalcin (P = 0.112), IGF-1 (P = 0.799), 25-hydroxyvitamin D (P = 0.788), TNF-α (P = 0.115), IL-6 (P = 0.203), and IL-1β (P = 0.459) did not show significant differences between CON and OP. Otherwise, the differences in the serum concentrations of GDF-15 were statistically significant (P = 0.019), and participants in OP had significantly higher levels of serum GDF-15 than those in CON (Table 3).
Table 3 Differences of serum levels of bone turnover markers, IGF-1, vitamin D, GDF-15, and inflammatory cytokines between CON and OP.
The significantly different levels of mRNA expression in the pronator quadratus muscle in GDF-15 (P = 0.003), IL-1β (P = 0.013), and TNF-α (P = 0.034) between the two groups were shown with those in the OP were significantly higher than those in the CON. Otherwise, there were no statistically significant differences expressed in the mRNA levels of IL-6 (P = 0.580) and IGF-1 (P = 0.955) between the groups (Figure 2).
Figure 2 The results of real-time PCR. Normalized fold expressions of mRNA are shown. The error bar represents the mean ± standard error. To compare gene expression levels between groups, independent t-test was applied (*P < 0.05).
The extents of muscle mass and power showed significant associations with skeletal BMD, and age was shown to have significantly negative impacts on skeletal aBMD and handgrip strength. Handgrip strength showed a significantly negative correlation with age (r2 = -0.579, P < 0.001) and a positive correlation with the circumferences of the upper arm and calf (r2 = 0.388, P = 0.009), aBMD of the total hip (r2 = 0.452, P < 0.001) and femoral neck (r2 = 0.459, P < 0.001), cortical HU (r2 = 0.348, P = 0.019), and cortical thickness (r2 = 0.315, P = 0.035) of the bone model of the distal radius. The focal bone density of the distal radius showed significant relationships with aBMD of the total hip, femoral neck, and lumbar spine. The circumferences of the upper arm and calf showed significantly positive associations with aBMD of the total hip (r2 = 0.413, P < 0.001), femoral neck (r2 = 0.329, P = 0.027), and trabecular HU of the distal radius bone (r2 = 0.347, P = 0.020), respectively (Table 4).
Table 4 Correlation coefficient among age, handgrip strength, BMI, circumferences of upper arm and calf, aBMD of total hip, femoral neck, and lumbar spine, and local bone quality of radial forearm.
The serum levels of GDF-15 showed significantly positive correlations with age (r2 = 0.374, P = 0.011). It had significantly negative associations with the aBMD of the total hip (r2 = -0.366, P = 0.013) and focal bone quality of the distal radius. Otherwise, the serum concentrations of GDF-15 did not show significant correlations with BMI (r2 = -0.234, P = 0.122), handgrip power (r2 = -0.227, P = 0.134), the circumferences of the upper arm (r2 = -0.213, P = 0.161) and calf (r2 = -0.219, P = 0.149), and the serum levels of IGF-1 (r2 = -0.125, P = 0.412) and inflammatory cytokines such as TNF-α (r2 = 0.181, P = 0.235), IL-6 (r2 = -0.073, P = 0.631), and IL-1β (r2 = 0.161, P = 0.289). The serum concentration of TNF-α showed negative correlations with handgrip strength (r2 = -0.536, P < 0.001) and the circumferences of the upper arm and calf (r2 = -0.438, P < 0.001). Circulating levels of GDF-15, TNF-α, IL-6, and IL-1β showed significant relationships with focal density for the distal radius (Table 5). When correlations were analyzed in the CON and OP groups separately, GDF-15 showed a significant association with the aBMD of the total hip, and cortical and trabecular bone density of the distal radius bone were only significant in OP not in CON (Supplementary Figure).
Table 5 Relationships among age, handgrip strength, BMI, circumferences of upper arm and calf, serum GDF-15 and cytokine levels, handgrip strength, aBMD of total hip, femoral neck, and lumbar spine, and local bone quality of radial forearm.
The mRNA expression levels of GDF-15 in muscle specimens were significantly associated with age (r2 = 0.404, P < 0.001). Muscle specimens had significantly negative relationships with handgrip strength (r2 = -0.515, P < 0.001), circumferences of the upper arm (r2 = -0.378, P = 0.011) and calf (r2 = -0.443, P < 0.001), aBMD of the total hip (r2 = -0.392, P = 0.008), and cortical HU (r2 = -0.480, P < 0.001) and trabecular HU (r2 = -0.374, P = 0.011) of the distal radius. Furthermore, it correlated significantly with expression levels of inflammatory cytokines such as TNF-α (r2 = 0.643, P < 0.001) and IL-1β (r2 = 0.909, P < 0.001). The mRNA expression levels of TNF-α significantly negatively associated with circumferences of upper arm (r2 = -0.309, P = 0.044) and calf (r2 = -0.314, P = 0.035), aBMD of the total hip (r2 = -0.426, P < 0.001), and trabecular HU (r2 = -0.351, P = 0.018) of the distal radius. The expression levels of GDF-15 correlated significantly with serum GDF-15 (r2 = 0.564, P < 0.001) and TNF-α levels (r2 = 0.694, P < 0.001) (Table 6). When the correlation analysis was conducted separately in CON and OP, only in OP did the mRNA levels of GDF-15 in muscle show significant relationships with age, grip strength, circumferences of upper arm and calf, and cortical and trabecular bone density of the distal radius bone. The relationship between mRNA levels of GDF-15 and those of TNF-α showed significant correlations in both CON and OP (Supplementary Figure).
Table 6 Correlation among age, handgrip strength, BMI, circumferences of upper arm and calf, mRNA expression levels of GDF-15 and cytokine in muscles, aBMD of total hip, femoral neck, and lumbar spine, and local bone quality of radial forearm.
GDF-15, a member of TGF-β superfamily, has been identified as a marker of aging, muscle weakness, and frailty (6–17). Several studies have demonstrated the link between GDF-15 and bone metabolism (19–21). However, the effect of GDF-15 as a myokine on bone metabolism and sarco-osteoporosis in humans is not clear. Hence, the aim of this study was to investigate the potential role of GDF-15 as a myokine in bone metabolism and muscle function in patients with osteoporosis.
The novel finding of the study was that GDF-15 may play a role in the inhibition of maintaining BMD and bone mass via endocrinological and paracrinological mechanisms. That role seemed to be more prominent in females with osteoporosis compared to healthy females. Both circulating and expression levels of GDF-15 in the pronator quadratus muscle were higher in osteoporotic patients than in controls. Furthermore, both serum concentration and mRNA expression levels of GDF-15 in muscle were negatively correlated with focal bone density, especially the cortical bone density of the distal radius, the attachment site of the pronator quadratus muscle as well as aBMD of the total hip, particularly in females with osteoporosis. According to several studies, the circulating level of GDF-15 is a negative predictor of BMD in post-menopausal females (32, 33) and other in vitro studies have demonstrated the influences of GDF-15 on osteoclastic activity (20, 21). This study supports the findings that circulating GDF-15 has a negative modulatory effect on maintaining BMD in the distal radius and total hip via endocrinological pathways. Moreover, the expression levels of GDF-15 in the pronator quadratus muscle that is attached to the distal radius appeared to be negatively related to maintaining BMD of the distal radius. Therefore, because levels of both circulating GDF-15 and expression GDF-15 in muscle were negatively related to the focal bone quality of the distal radius bone as well as aBMD of the total hip, we cautiously suggest that GDF-15 may have negative effects on bone mass maintenance via both endocrinological and paracrinological mechanisms, as a myokine that is released from the muscle.
The association between circulating GDF-15 levels and muscle mass and strength has been studied (6, 8). The findings from this study revealed patterns that differ from those found in previous reports. The circulating GDF-15 concentration did not correlate significantly with grip strength or circumferences of the upper arm and calf. Otherwise the mRNA expression levels of GDF-15 in the pronator quadratus muscle were significantly related to those variables. In one study, no significant correlations between circulating GDF-15 and muscle mass or strength were found in the elderly (34); another study that suggested plasma GDF-15 predicted sarcopenia-associated outcomes found no significant correlations between plasma GDF-15 levels and muscle mass or strength (12). The other study demonstrated that the expression levels of GDF-15 in muscle were negatively correlated with muscle weight and endurance capacity (10). We postulate that GDF-15 in muscle could be related to muscle mass and strength more significantly than is circulating GDF-15. We further postulate that this interaction might be more prominent in females with low bone mass.
TNF-α is a pro-inflammatory cytokine that is related to inflammation, mitochondrial dysfunction, and aging (35). TNF-α is involved in the pathogenesis of sarcopenia (36) and promotes pathological osteoclastogenesis and bone resorption in collaboration with receptor activator of nuclear factor kappa-B ligand (RANKL) (37). Our findings of negative correlations between circulating TNF-α levels and bone quality of the distal radius, circumference of calf, or grip strength are consistent with this phenomenon. Several studies have proposed that GDF-15 plays a role in the development of inflammation-driven states and in aging (13, 38), and one study suggested that GDF-15 may contribute to inflammatory damage through triglyceride metabolism (39). The finding that both circulating and mRNA expression levels of TNF-α in muscle were significantly correlated positively with those of GDF-15 could be understood in the same manner. The negative role of GDF-15 on maintaining bone mass could be related to the osteoclastic activity of TNF-α and the inflammation process that have negative effects on bone remodeling and muscle function.
This study primarily attempted to reveal the role of GDF-15 in bone and muscle metabolism in patients with osteoporosis. However, this study had several limitations. First, due to the small sample size, the statistical significance of the results is inevitably compromised. Second, because this study included only female participants, the information provided about the role of GDF-15 on bone metabolism and muscle function is limited. Third, because this study did not include data about skeletal muscle mass, degree of muscle fibrosis, and adipogenesis, the precise endocrinological mechanisms of GDF-15 could not be derived. Furthermore, the effects of aging on the circulation and expression levels of GDF-15 could not be ruled out because correlation analysis was not adjusted for age and other potential confounding factors due to multicollinearity among the variables. Finally, we measured only mRNA expression levels of the GDF-15 and inflammatory cytokines in muscle specimens, not protein levels, therefore the actual relationships between myokines and bone and muscle metabolism could not be assumed.
In conclusion, GDF-15 may have associations with bone metabolism in humans via both paracrinological and endocrinological pathways. Muscle mass and function would be influenced more prominent by GDF-15 mRNA expressed in muscle than by circulating GDF-15. The role of GDF-15 in bone metabolism and muscle homeostasis may be related to inflammatory responses. The findings of this study indicate that GDF-15 may be a target for future therapeutics for sarco-osteopenia and, eventually, for recovery and longevity. Future research is required.
The original contributions presented in the study are included in the article/Supplementary Material. Further inquiries can be directed to the corresponding author.
The studies involving human participants were reviewed and approved by Kangbuk Samsung Hospital. The patients/participants provided their written informed consent to participate in this study.
All authors listed have made a substantial, direct, and intellectual contribution to the work and approved it for publication.
This research was supported by the National Research Foundation of Korea (NRF) grant funded by the Korea government (No. 2020R1I1A1A01071537).
The authors declare that the research was conducted in the absence of any commercial or financial relationships that could be construed as a potential conflict of interest.
All claims expressed in this article are solely those of the authors and do not necessarily represent those of their affiliated organizations, or those of the publisher, the editors and the reviewers. Any product that may be evaluated in this article, or claim that may be made by its manufacturer, is not guaranteed or endorsed by the publisher.
The Supplementary Material for this article can be found online at: https://www.frontiersin.org/articles/10.3389/fendo.2022.948176/full#supplementary-material
Supplementary Figure | Correlations between circulating levels of GDF-15 and (A) age, (B) aBMD of the total hip, (C) cortical bone density of the distal radius bone, (D) trabecular bone density of the distal radius bone. Correlations between extents of mRNA expression of GDF-15 and (E) age, (F) handgrip strength, (G) circumferences of upper arm, (H) circumferences of calf, (I) cortical bone density of the distal radius bone, (J) trabecular bone density of the distal radius bone, (K) serum concentration of the TNF-α, (L) mRNA expression levels of TNF-α.
1. Fried LP, Ferrucci L, Darer J, Williamson JD, Anderson G. Untangling the concepts of disability, frailty, and comorbidity: Implications for improved targeting and care. J Gerontol A Biol Sci Med Sci (2004) 59:255–63. doi: 10.1093/gerona/59.3.m255
2. Binkley N, Buehring B. Beyond FRAX: It’s time to consider “sarco-osteopenia”. J Clin Densitom (2009) 12:.413–416. doi: 10.1016/j.jocd.2009.06.004
3. Avin KG, Bloomfield SA, Gross TS, Warden SJ. Biomechanical aspects of the muscle-bone interaction. Curr Osteoporos Rep (2015) 13:1–8. doi: 10.1007/s11914-014-0244-x
5. Li G, Zhang L, Wang D, AI L, Jiang JX, Xu H, et al. Muscle-bone crosstalk and potential therapies for sarco-osteoporosis. J Cell Biochem (2019) 120:14262–73. doi: 10.1002/jcb.28946
6. Alcazar J, Frandsen U, Prokhorova T, Kamper RS, Haddock B, Aagaard P, et al. Changes in systemic GDF15 across the adult lifespan and their impact on maximal muscle power: The Copenhagen sarcopenia study. J Cachexia Sarcopenia Muscle (2021) 12:1418–27. doi: 10.1002/jcsm.12823
7. Bloch SA, Lee JY, Syburra T, Rosendahl U, Griffiths MJ, Kemp PR, et al. Increased expression of GDF-15 may mediate ICU-acquired weakness by down-regulating muscle microRNAs. Thorax (2015) 70:219–28. doi: 10.1136/thoraxjnl-2014-206225
8. Conte M, Martucci M, Mosconi G, Chiariello A, Cappuccilli M, Totti V, et al. GDF15 plasma level is inversely associated with level of physical activity and correlates with markers of inflammation and muscle weakness. Front Immunol (2020) 11:915. doi: 10.3389/fimmu.2020.00915
9. Fujita Y, Taniguchi Y, Shinkai S, Tanaka M, Ito M. Secreted growth differentiation factor 15 as a potential biomarker for mitochondrial dysfunctions in aging and age-related disorders. Geriatr Gerontol Int (2016) 16 Suppl 1:17–29. doi: 10.1111/ggi.12724
10. Kim H, Kim KM, Kang MJ, Lim S. Growth differentiation factor-15 as a biomarker for sarcopenia in aging humans and mice. Exp Gerontol (2020) 142:111115. doi: 10.1016/j.exger.2020.111115
11. Oba K, Ishikawa J, Tamura Y, Fujita Y, Ito M, Iizuka A, et al. Serum growth differentiation factor 15 level is associated with muscle strength and lower extremity function in older patients with cardiometabolic disease. Geriatr Gerontol Int (2020) 20:980–7. doi: 10.1111/ggi.14021
12. Semba RD, Gonzalez-Freire M, Tanaka T, Biancotto A, Zhang P, Shardell M, et al. Elevated plasma growth and differentiation factor 15 is associated with slower gait speed and lower physical performance in healthy community-dwelling adults. J Gerontol A Biol Sci Med Sci (2020) 75:175–80. doi: 10.1093/gerona/glz071
13. Tavenier J, Rasmussen LJH, Andersen AL, Houlind MB, Langkilde A, Andersen O, et al. Association of GDF15 with inflammation and physical function during aging and recovery after acute hospitalization: A longitudinal study of older patients and age-matched controls. J Gerontol A Biol Sci Med Sci (2021) 76:964–74. doi: 10.1093/gerona/glab011
14. Ortola R, Garcia-Esquinas E, Buno-Soto A, Cabanas-Sanchez V, Martinez-Gomez D, Sotos-Prieto M, et al. Associations of device-measured sleep, sedentariness and physical activity with growth differentiation factor 15 in older adults. J Cachexia Sarcopenia Muscle (2022) 13:1003–12. doi: 10.1002/jcsm.12924
15. Kim M, Walston JD, Won CW. Associations between elevated growth differentiation factor-15 and sarcopenia among community-dwelling older adults. J Gerontol A Biol Sci Med Sci (2021) 77:770–80. doi: 10.1093/gerona/glab201
16. Chung HK, Ryu D, Kim KS, Chang JY, Kim YK, Yi HS, et al. Growth differentiation factor 15 is a myomitokine governing systemic energy homeostasis. J Cell Biol (2017) 216:149–65. doi: 10.1083/jcb.201607110
17. Ost M, Igual Gil C, Coleman V, Keipert S, Efstathiou S, Vidic V, et al. Muscle-derived GDF15 drives diurnal anorexia and systemic metabolic remodeling during mitochondrial stress. EMBO Rep (2020) 21:e48804. doi: 10.15252/embr.201948804
18. Ito T, Nakanishi Y, Yamaji N, Murakami S, Schaffer SW. Induction of growth differentiation factor 15 in skeletal muscle of old taurine transporter knockout mouse. Biol Pharm Bull (2018) 41:435–9. doi: 10.1248/bpb.b17-00969
19. Hinoi E, Ochi H, Takarada T, Nakatani E, Iezaki T, Nakajima H, et al. Positive regulation of osteoclastic differentiation by growth differentiation factor 15 upregulated in osteocytic cells under hypoxia. J Bone Miner Res (2012) 27:938–49. doi: 10.1002/jbmr.1538
20. Li S, Li Q, Zhu Y, Hu W. GDF15 induced by compressive force contributes to osteoclast differentiation in human periodontal ligament cells. Exp Cell Res (2020) 387:111745. doi: 10.1016/j.yexcr.2019.111745
21. Vanhara P, Lincova E, Kozubik A, Jurdic P, Soucek K. Smarda J growth/differentiation factor-15 inhibits differentiation into osteoclasts–a novel factor involved in control of osteoclast differentiation. Differentiation (2009) 78:213–22. doi: 10.1016/j.diff.2009.07.008
22. Duan L, Pang HL, Chen WJ, Shen WW, Cao PP, Wang SM, et al. The role of GDF15 in bone metastasis of lung adenocarcinoma cells. Oncol Rep (2019) 41:2379–88. doi: 10.3892/or.2019.7024
23. Wakchoure S, Swain TM, Hentunen TA, Bauskin AR, Brown DA, Breit SN, et al. Selander KS expression of macrophage inhibitory cytokine-1 in prostate cancer bone metastases induces osteoclast activation and weight loss. Prostate (2009) 69:652–61. doi: 10.1002/pros.20913
24. Chen G, Wang M, Liu X. GDF15 promotes osteosarcoma cell migration and invasion by regulating the TGFbeta signaling pathway. Mol Med Rep (2019) 20:4262–70. doi: 10.3892/mmr.2019.10664
25. Westhrin M, Moen SH, Holien T, Mylin AK, Heickendorff L, Olsen OE, et al. Growth differentiation factor 15 (GDF15) promotes osteoclast differentiation and inhibits osteoblast differentiation and high serum GDF15 levels are associated with multiple myeloma bone disease. Haematologica (2015) 100:e511–4. doi: 10.3324/haematol.2015.124511
26. Sousa-Santos AR, Amaral TF. Differences in handgrip strength protocols to identify sarcopenia and frailty - a systematic review. BMC Geriatr (2017) 17:238. doi: 10.1186/s12877-017-0625-y
27. Bechtol CO. Grip test; the use of a dynamometer with adjustable handle spacings. J Bone Joint Surg Am (1954) 36-A:820–4.
28. Petersen P, Petrick M, Connor H, Conklin D. Grip strength and hand dominance: challenging the 10% rule. Am J Occup Ther (1989) 43:444–7. doi: 10.5014/ajot.43.7.444
29. Hong SW, Kang JH. Decreased mandibular cortical bone quality after botulinum toxin injections in masticatory muscles in female adults. Sci Rep (2020) 10:3623. doi: 10.1038/s41598-020-60554-w
30. Hong SW, Kang JH. Bone mineral density, bone microstructure, and bone turnover markers in females with temporomandibular joint osteoarthritis. Clin Oral Investig (2021) 25:6435–48. doi: 10.1007/s00784-021-03946-0
31. Hong SW, Kang JH, Kim JS, Gong HS. Association between forearm cortical bone properties and handgrip strength in women with distal radius fractures: A cross-sectional study. PloS One (2020) 15:e0243294. doi: 10.1371/journal.pone.0243294
32. Li W, Li C, Zhou X, Jiang T, Guo L, Liu H. Relationship between GDF15 level and bone metabolism in postmenopausal Chinese women. Gynecol Endocrinol (2020) 36:714–7. doi: 10.1080/09513590.2020.1764929
33. Lee SH, Lee JY, Lim KH, Lee YS, Koh JM. Associations between plasma growth and differentiation factor-15 with aging phenotypes in muscle, adipose tissue, and bone. Calcif Tissue Int (2022) 110:236–43. doi: 10.1007/s00223-021-00912-6
34. Nga HT, Jang IY, Kim DA, Park SJ, Lee JY, Lee S, et al. Serum GDF15 level is independent of sarcopenia in older Asian adults. Gerontology (2021) 67:525–31. doi: 10.1159/000513600
35. Akash MSH, Rehman K, Liaqat A. Tumor necrosis factor-alpha: Role in development of insulin resistance and pathogenesis of type 2 diabetes mellitus. J Cell Biochem (2018) 119:105–10. doi: 10.1002/jcb.26174
36. Picca A, Coelho-Junior HJ, Calvani R, Marzetti E, Vetrano DL. Biomarkers shared by frailty and sarcopenia in older adults: A systematic review and meta-analysis. Ageing Res Rev (2022) 73:101530. doi: 10.1016/j.arr.2021.101530
37. Lam J, Takeshita S, Barker JE, Kanagawa O, Ross FP, Teitelbaum SL. TNF-alpha induces osteoclastogenesis by direct stimulation of macrophages exposed to permissive levels of RANK ligand. J Clin Invest (2000) 106:1481–8. doi: 10.1172/JCI11176
38. Abulizi P, Loganathan N, Zhao D, Mele T, Zhang Y, Zwiep T, et al. Growth differentiation factor-15 deficiency augments inflammatory response and exacerbates septic heart and renal injury induced by lipopolysaccharide. Sci Rep (2017) 7:1037. doi: 10.1038/s41598-017-00902-5
Keywords: GDF-15, osteoporosis, sarcopenia, grip power, TNF-α, inflammation
Citation: Hong SW and Kang J-H (2022) Growth differentiation factor-15 as a modulator of bone and muscle metabolism. Front. Endocrinol. 13:948176. doi: 10.3389/fendo.2022.948176
Received: 19 May 2022; Accepted: 20 September 2022;
Published: 17 October 2022.
Edited by:
Trupti Trivedi, University of Texas MD Anderson Cancer Center, United StatesReviewed by:
Gemma Carreras-Badosa, Institute of Biomedical Research of Girona, SpainCopyright © 2022 Hong and Kang. This is an open-access article distributed under the terms of the Creative Commons Attribution License (CC BY). The use, distribution or reproduction in other forums is permitted, provided the original author(s) and the copyright owner(s) are credited and that the original publication in this journal is cited, in accordance with accepted academic practice. No use, distribution or reproduction is permitted which does not comply with these terms.
*Correspondence: Jeong-Hyun Kang, aXJlbmU4NUBzbnUuYWMua3I=
†ORCID: IDs: Seok Woo Hong, orcid.org/0000-0003-4059-1557
Jeong-Hyun Kang, orcid.org/0000-0001-7124-8693
Disclaimer: All claims expressed in this article are solely those of the authors and do not necessarily represent those of their affiliated organizations, or those of the publisher, the editors and the reviewers. Any product that may be evaluated in this article or claim that may be made by its manufacturer is not guaranteed or endorsed by the publisher.
Research integrity at Frontiers
Learn more about the work of our research integrity team to safeguard the quality of each article we publish.