- 1National Clinical Research Center for Metabolic Diseases, Metabolic Syndrome Research Center, Key Laboratory of Diabetes Immunology, Ministry of Education, and Department of Metabolism and Endocrinology, The Second Xiangya Hospital of Central South University, Changsha, China
- 2Department of Metabolism and Endocrinology, The Affiliated Zhuzhou Hospital Xiangya Medical College CSU, Zhuzhou, China
Aims: Coronavirus disease 2019 (COVID-19) is caused by infection with severe acute respiratory syndrome coronavirus 2 (SARS-CoV-2), and within a few months of the first outbreak, it was declared a global pandemic by the WHO. The lethal virus SARS-CoV-2 is transmitted through respiratory droplets and enters host cells through angiotensin-converting enzyme 2 (ACE-2) receptors. ACE-2 receptors are highly expressed in many tissues, including testes. Therefore, the objective of this study was to summarize the available literature regarding the correlation between sex hormone levels and COVID-19.
Methods: The PubMed, Web of Science, Embase, and Cochrane Library databases were reviewed systematically through August 2022 for studies comparing sex hormone levels between different patient groups: COVID-19 versus no COVID-19, more severe versus less severe COVID-19, and non-survivors versus survivors. Various types of clinical research reporting sex hormone levels, including free testosterone (FT), luteinizing hormone (LH), follicle-stimulating hormone (FSH), 17β-oestradiol (E2), the oestradiol-to-testosterone ratio (E2/T), prolactin (PRL), and sex hormone-binding globulin (SHBG), were included. Random- or fixed-effects models were used to calculate weighted mean differences (WMDs) and 95% confidence intervals (CIs). Heterogeneity among the studies was assessed by the I2 index, and data analyses were performed using meta-analysis with Stata version 12.0.
Results: Twenty-two articles that included 3369 patients were ultimately included in the meta-analysis. According to analysis of the included studies, patients with COVID-19 had significantly low T/LH, FSH/LH, and SHBG levels and high levels of LH, and E2/T, but their levels of FT, FSH, PRL, E2, and progesterone were not affected. Publication bias was not found according to funnel plots and Egger’s regression and Begg’s rank correlation tests.
Conclusion: Low T/LH, FSH/LH, and SHBG serum levels and high LH, and E2/T levels may increase the risk of COVID-19. Additionally, the greater is the clinical severity of COVID-19, the higher is the probability of increases in LH, and E2/T serum levels and decreases in T/LH, FSH/LH, and SHBG levels. COVID-19 may have unfavourable effects on gonadal functions, which should be taken seriously by clinicians. Routine monitoring of sex hormone levels might help clinicians to evaluate disease severity in patients with COVID-19.
Introduction
In early December 2019, the outbreak of coronavirus disease 2019 (COVID-19) quickly progressed to a pandemic, bringing severe challenges to global health; this disease can be transmitted through respiratory droplets or by direct contact (1). As of September 8th, 2021, there were more than 4,582,338 deaths (2). In addition, vulnerable individuals may experience a variety of serious and life-threatening complications, including acute respiratory distress syndrome (ARDS), sepsis, coagulopathy, disseminated intravascular coagulation (DIC), acute kidney injury (AKI), and multiorgan dysfunction (3–5). Thus, a better understanding of clinical risk factors that can distinguish between severe and non-severe cases or between a high and low risk of death is vital for improving therapeutic interventions.
Currently, studies have indicated that sex differences are present in the clinical outcomes of COVID-19 patients (6). Sex disaggregated data indeed showed that men had higher rates of mortality than women (7). In particular, the mortality rates of men are 2.4 times higher than those of women, thus indicating sex-specific differences in severe acute respiratory syndrome coronavirus 2 (SARS-CoV-2)-associated sequelae (8). Angiotensin-converting enzyme 2 (ACE2) and TMPRSS2 are critical factors for virus transmission. SARS-CoV-2 is mainly transmitted via respiratory droplets and enters human cells through ACE-2 receptors (9). In addition, expression pattern analysis of ACE2 in adult human testes indicated that ACE2 is mainly distributed in spermatogonia and Sertoli and Leydig cells, suggesting that the human testis is susceptible to SARS-CoV-2 (10). TMPRSS2 is predominantly expressed in spermatogonia and spermatids. In a retrospective study, a large proportion of the total patients with COVID-19 were male (11). Consequently, concern has been raised about whether SARS-CoV-2 may affect the male reproductive system. It is assumed that SARS-CoV-2 may have a negative impact on the male reproductive tract; however, results are inconsistent.
Method
Search strategy
To find all studies that evaluated the association between sex hormone levels and the risk of COVID-19, two of the authors (ZC and JZ) independently searched the PubMed, Embase, Web of Science, and Cochrane Library databases through August 2022. The search terms included the following key words: (a) COVID-19; (b) testosterone (FT), luteinizing hormone (LH), follicle-stimulating hormone (FSH), 17β-oestradiol (E2), the oestradiol-to-testosterone ratio (E2/T), prolactin (PRL), progesterone and sex hormone-binding globulin (SHBG). The reference lists of relevant reviews or included studies were also manually searched to identify relevant articles.
Study selection
Using the PECO/PICO (population, exposure/intervention, comparison/control, and outcome) strategy, we included the studies that met the following criteria in the study.
● Populations: subjects participating in studies that assessed the impact of sex hormone levels on COVID-19.
● Exposure/Intervention: presence or absence of COVID-19
● Comparison: sex hormone levels
● The outcome of the study: sex hormone levels
● Exclusion criteria
● Studies without full text
● In vitro and animal studies
● Data of interest were not presented
● Abstracts, commentary articles, reviews, meta-analyses, editorials and conference presentations
Data extraction and quality assessment
Two of us (ZC, JZ) independently extracted the data using a standardized data extraction form. Extracted information included the following: (1) the characteristics of the study, including the first author, year of publication, study design, and country; (2) basic characteristics of the population, including the sample size, mean age, and sex ratio; and (3) sex hormone indicators, including FT, LH, FSH, E2, E2/T, PRL, progesterone and SHBG. The weighted mean difference (WMD) and corresponding 95% confidence intervals (CIs) as well as I2 were extracted. For studies reporting only the median ± interquartile range (IQR), we converted these values into the mean and standard deviation (SD) (12). Any dissenting opinions were resolved through discussion and consensus. Quality assessment of the nonrandomized comparative studies was performed with the Newcastle–Ottawa scale (NOS) (13).
Statistical analysis
Statistical analyses were carried out by STATA (Version 12.0; STATA Corporation, College Station, TX, USA) software. Fixed-effects or random-effects models were adopted according to the heterogeneity of the studies (I2 < 50%, fixed-effects models; I2 > 50%, random-effects models). The WMD with the random-effects model (DerSimonian–Laird method) and 95% CI were applied for continuous data. Sensitivity analysis was conducted by excluding one study each time through influence analysis to assess the stability of the results. Heterogeneity among the included studies was assessed with the I2 statistic. I2 values above 70% were considered to indicate the presence of extreme heterogeneity. The potential evidence of publication bias was assessed using a funnel plot, Egger’s regression and Begg’s rank correlation tests. If publication bias was confirmed, it was corrected using Duval’s trim-and-fill method used the properties of the funnel plot. Subgroup analysis was conducted based on the severity of the disease or age and country. Statistical significance was determined with a two-tailed p < 0.05.
Results
Study selection
Following the Preferred Reporting Items for Systematic Reviews and Meta-Analysis (PRISMA) guidelines, we included 22 studies that involved 3369 patients and satisfied our inclusion/exclusion criteria for meta-analysis. The preliminary literature search resulted in 5218 articles, and 756 studies remained after exclusion due to duplication. After scanning the titles and abstracts, we obtained 52 studies by excluding an additional 346 studies. After reading the full texts and review articles, we further excluded 30 studies that did not report sex hormone levels. Finally, 22 studies (14–30) (19, 31–33) were included in our analysis. The process of study identification and selection is shown in Figure 1.
Description of included studies
In total, there were 3369 patients: 8 studies from Turkey (930 patients), 7 from Italy (975 patients), 5 from China (773 patients), and 1 each from France (118 patients), and the USA (152 patients). The patient ages ranged from 18 to 73 years old. Overall, there were nine cohort studies, six case–control studies, and seven cross-sectional studies. Virtually all respondents were male, with only four studies including female respondents. The articles were published during the period from 2020 to 2021. According to the NOS scores, all studies were deemed to be of high quality. The characteristics of the 22 eligible studies and their NOS scores are summarized in Table 1.
Overall analysis
Results of the meta-analysis of free testosterone levels in the COVID-19 group and controls
Overall, we found a milieu of clinical androgenicity (sexual function, bone density and haematopoiesis) resulting from the interaction between polyQ polymorphism in the androgen receptor (AR) and serum testosterone levels. In particular, the calculated FT correlated better than total testosterone (TT) with all relevant clinical parameters of androgenicity, and androgenicity was reduced when the AR showed a large number of CAG repeats. We identified 3 studies published from 2020 to 2022 (three cohort studies) that presented results on FT levels and COVID-19, including a total of 194 subjects. Subgroup analyses were stratified by disease severity (patients with COVID-19 vs. controls; patients with severe COVID-19 vs. those without severe COVID-19. In 1 study that measured levels of FT in patients with COVID-19 or controls, the aggregated WMD was 0.03, with a 95% CI of 0.01 and 0.05. Based on 3 studies that compared patients with severe COVID-19 vs. those without severe COVID-19, the pooled WMD was -0.08 (95% CI: -0.17 to 0.02) (Table 2 and Figure 2). Egger’s test showed that no publication bias existed among the included studies (p > 0.05). The publication bias analysis showed that the funnel plot was nearly symmetrical (Figure 7A). The results of the sensitivity analyses indicated that the conclusions were robust (Figure 8A). The above results revealed that patients with COVID-19 have no significance FT levels than subjects without COVID-19.
Results of the meta-analysis of FSH levels in the COVID-19 group and controls
We identified 12 studies published from 2020 to 2021 (four case–control studies, five cohort studies, and three cross-sectional studies) that presented results on FSH levels and COVID-19. The 12 studies included a total of 3257 subjects. The effect size from the random-effects model showed no significant changes in FSH levels (pooled WMD: 0.60, CI: -0.14 and 1.35) (Figure 3). The heterogeneity test results found obvious heterogeneity (I2 = 89.1%, p = 0.000). Sensitivity analysis confirmed that no individual study influenced the overall results.
Subgroup analyses were stratified by sex (male, female), study design (cohort, case–control and cross-sectional) and country (Italy, Turkey and China). In 1 study with females that assessed levels of FSH in patients, the aggregated WMD was 19.65, with a 95% CI of -1.12 and 40.42. From 11 studies with males, the pooled WMD was found to be 0.58 (95% CI: -0.16 to 1.32). In 4 studies with a cohort design that assessed the levels of FSH in patients, the aggregated WMD was 2.05 with a 95% CI of 1.03 and 3.07. From 3 studies using a case–control design, the pooled SDM was found to be 0.02 (95% CI: -0.30 to 0.34). From 3 studies with a cross-sectional design, the pooled WMD was found to be 0.22 (95% CI: -0.93 to 1.38). Subgroup analysis was performed according to country, and decreased FSH levels were found in Turkey but not in Italy or China (pooled WMD: 1.21, CI: 0.24 and 2.19 for Turkey; pooled WMD: -1.13, CI: -3.15 and 0.88 for Italy; pooled WMD: -0.08, CI: -0.85 and 0.69 for China) (Table 2). Egger’s test showed that no publication bias was present among the included studies (p > 0.05). The publication bias analysis showed that the funnel plot was nearly symmetrical (Figure 7B). The results of the sensitivity analyses indicated that the conclusions were robust (Figure 8B). The above results revealed that patients with COVID-19 have higher FSH levels than individuals without COVID-19.
Results of the meta-analysis of LH levels in the COVID-19 group and controls
We identified 13 studies published from 2020 to 2021 (four case–control studies, five cohort studies, and four cross-sectional studies) that presented results on LH levels and COVID-19. The 13 studies included a total of 3288 subjects. The effect size from the random-effects model showed a significant increase in LH levels (pooled WMD: 0.92, CI: 0.12 and 1.72) (Figure 4). The heterogeneity test results found obvious heterogeneity (I2 = 93.4%, p = 0.000). Sensitivity analysis confirmed that no individual study influenced the overall results.
Subgroup analyses were stratified by sex (male, female), disease severity (patients with COVID-19 vs. controls; patients with severe COVID-19 vs. those without severe COVID-19), age (younger than 50 years old vs. older than 50 years old), and study design (cohort, case–control and cross-sectional). In 1 study that measured levels of LH in female patients with COVID-19 or controls, the aggregated WMD was 11.30, with a CI of -7.88 and 30.48. From 12 studies that compared male patients with COVID-19 or those without COVID-19, the pooled WMD was 0.90 (95% CI: 0.10 to 1.71). In 7 studies that measured the levels of LH in patients with COVID-19 or controls, the aggregated WMD was 1.42 with a CI of 0.21 and 2.63. From 6 studies that compared patients with severe COVID-19 with those without severe COVID-19, the pooled WMD was found to be -0.48 (95% CI: -1.59 to 0.63). In 5 studies with a cohort design that assessed the levels of LH in patients, the aggregated WMD was 1.11 with a CI of 0.06 and 2.16. From 4 studies with a case–control design, the pooled WMD was found to be 1.57 (95% CI: 0.33 to 2.82). From 4 studies that used a cross-sectional design, the pooled SDM was found to be -0.17 (95% CI: -0.93 to 1.38). In a subgroup analysis according to age, LH levels were found to be significantly higher only in patients younger than 50 years old but not in those older than 50 years old (pooled WMD: 1.28, CI: 0.06 and 2.5 for younger than 50 years old; pooled WMD: 0.66, CI: -0.43 and 1.75 for older than 50 years old) (Table 2). Egger’s test showed that no publication bias was present among the included studies (p > 0.05). The publication bias analysis showed that the funnel plot was nearly symmetrical (Figure 7C). The results of the sensitivity analyses indicated that the conclusions were robust (Figure 8C). The above results revealed that patients with COVID-19 present higher LH levels than subjects without COVID-19.
Results of the meta-analysis of PRL levels in COVID-19 and control groups
We identified 8 studies published from 2020 to 2021 (three case–control studies, two cohort studies, and three cross-sectional studies) that presented results on PRL levels and COVID-19. The 8 studies included a total of 810 subjects. The effect size from the fixed-effects model showed a significant increase in PRL levels (pooled WMD: 0.65, CI: -0.03 and 1.33) (Figure 5).
Subgroup analyses were stratified by sex (male, female), study design (cohort, case–control and cross-sectional). In 1 study with females that detected levels of PRL in patients, the aggregated WMD was -0.77, with a CI of -5.28 and 3.74. From 6 studies involving males, the pooled WMD was 0.68 (95% CI: -0.01 to 1.37). In 2 studies with a cohort design that detected the levels of PRL in patients, the aggregated WMD was 1.13 with a CI of 0.16 and 2.10. From 3 studies with a case–control design, the pooled WMD was found to be 2.26 (95% CI: -13.60 to 18.13). From 3 studies that used a cross-sectional design, the pooled WMD was found to be 0.12 (95% CI: -0.91 to 1.15). Egger’s test showed that no publication bias existed among the included studies (p > 0.05). The publication bias analysis showed that the funnel plot was nearly symmetrical (Figure 7D). The results of the sensitivity analyses indicated that the conclusions were robust (Figure 8D). The above results revealed that patients with COVID-19 present no significant changes in PRL levels compared to subjects without COVID-19.
Results of the meta-analysis of E2 levels in the COVID-19 group and controls
We identified 10 studies published from 2020 to 2021 (three case–control studies, four cohort studies, and two cross-sectional studies) that presented results on E2 levels and COVID-19. The 10 studies included a total of 1584 subjects. The effect size from the random-effects model showed no significant difference in PRL levels (pooled WMD: 0.88, CI: -2.19 and 3.95) (Figure 6). The heterogeneity test results found obvious heterogeneity (I2 = 77.6%, p = 0.000).
Subgroup analyses were stratified by sex (male, female), disease severity (patients with COVID-19 vs. controls; patients with severe COVID-19 vs. those without severe COVID-19; non-survivors vs. survivors). In 2 studies that measured levels of E2 in female patients with COVID-19 or controls, the aggregated WMD was -5.36, with a CI of -32.44 and 21.71. Based on 7 studies comparing male patients with and without COVID-19, the pooled WMD was found to be 0.89 (95% CI: -2.17 to 3.95). In 4 studies that measured the levels of E2 in patients with COVID-19 or controls, the aggregated WMD was 6.49 with a CI of 0.27 and 12.7. From 5 studies that compared patients with severe COVID-19 with those without severe COVID-19, the pooled WMD was found to be 0.51 (95% CI: -3.22 to 4.25). Non-survivors vs. survivors were assessed in only 2 studies, with a WMD value of 7.36 (95% CI: -7.98 to 22.69). Egger’s test showed that no publication bias existed among the included studies (p > 0.05). The publication bias analysis showed that the funnel plot was nearly symmetrical (Figure 7E). The results of the sensitivity analyses indicated that the conclusions were robust (Figure 8E). The above results revealed that patients with COVID-19 present lower E2 levels than subjects without COVID-19.
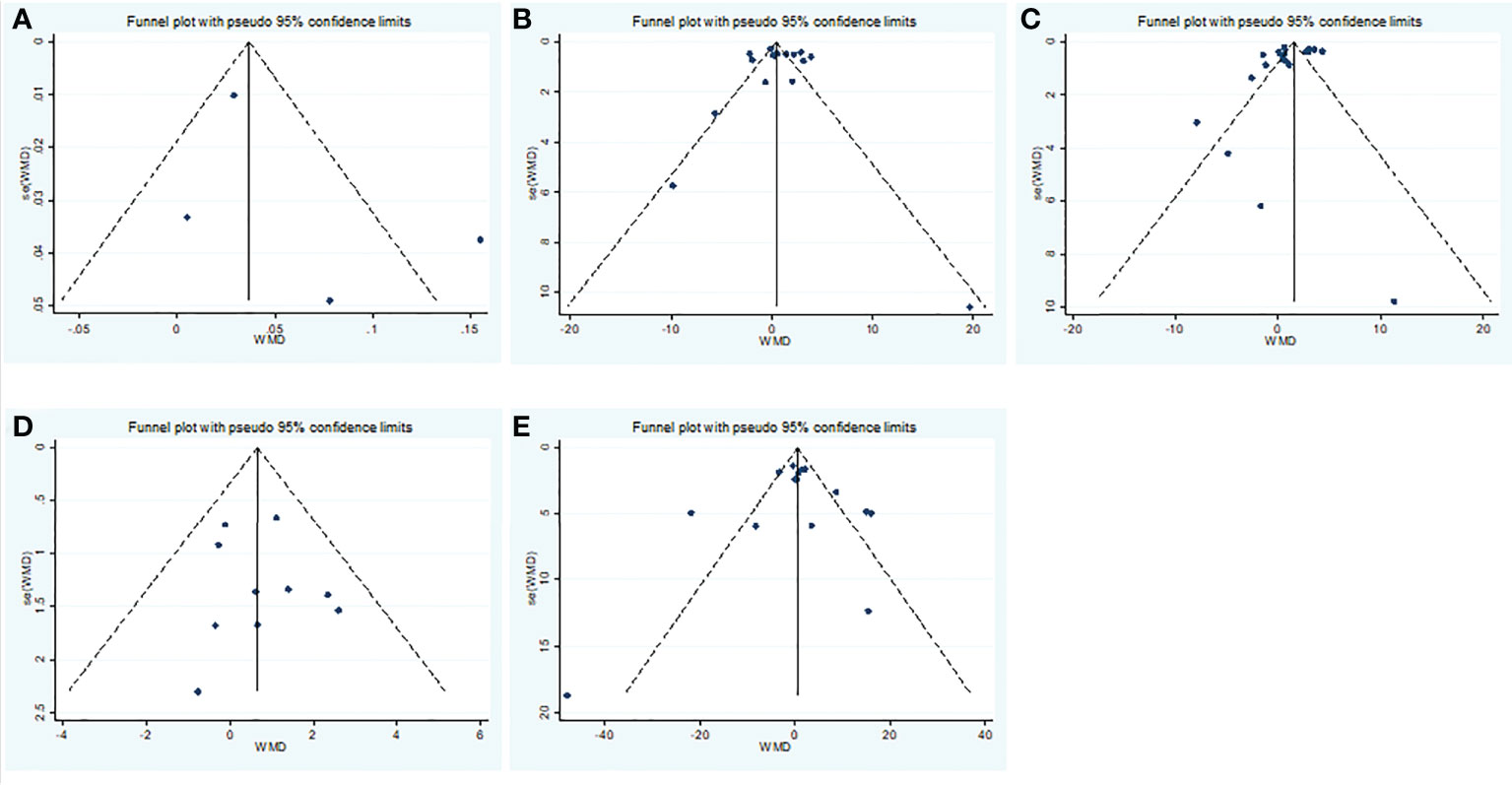
Figure 7 Publication bias funnel plots of the WMD for (A) TT, (B) FSH, (C) LH, (D) PRL, (E) E2 and COVID-19.
Results of the meta-analysis of progesterone, SHBG, T/LH, FSH/LH, and E2/T levels in the COVID-19 group and controls
We identified 3 studies published from 2020 to 2021 that presented results on progesterone levels and COVID-19. The 3 studies included a total of 196 subjects and 181 COVID-19 patients. The effect size from the random-effects model showed a significant increase in progesterone levels (pooled WMD: 0.01, CI: -0.05 and 0.07) (Table 2). The heterogeneity test results found obvious heterogeneity (I2 = 73.4%, p = 0.000).
We identified 3 studies published from 2020 to 2021 that presented results on SHBG levels and COVID-19. The 3 studies included a total of 120 subjects and 61 COVID-19 patients. The effect size from the random-effects model showed a significant increase in SHBG levels (pooled WMD: -8.69, CI: -17.20 and -0.18) (Table 2). The heterogeneity test results found obvious heterogeneity (I2 = 0%, p = 0.000).
We identified 4 studies published from 2020 to 2021 that presented results on T/LH levels and COVID-19. The 4 studies included a total of 874 subjects and 1088 COVID-19 patients. The effect size from the random-effects model showed a significant increase in T/LH values (pooled WMD: -0.95, CI: -1.36 and -0.55) (data not shown). The heterogeneity test results found obvious heterogeneity (I2 = 93.4%, p = 0.000). Sensitivity analyses were performed and showed that the study type was the main factor impacting the results (pooled WMD: -1.10, CI: -1.42 and -0.79) (Table 2).
We identified 3 studies published from 2020 to 2021 that presented results on FSH/LH levels and COVID-19. The 3 studies included a total of 434 subjects and 217 COVID-19 patients. The effect size from the random-effects model showed a significant increase in FSH/LH values (pooled WMD: -0.66, CI: -0.74 and -0.57) (Table 2). The heterogeneity test results found no heterogeneity (I2 = 0%, p = 0.000).
We identified 3 studies published from 2020 to 2021 that presented results on E2/T levels and COVID-19. The 3 studies included a total of 144 subjects and 186 COVID-19 patients. The effect size from the random-effects model showed a significant increase in E2/T values (pooled WMD: 0.40, CI: 0.18 and 0.63) (Table 2). The heterogeneity test results found no heterogeneity (I2 = 26.4%, p = 0.000).
Discussion
Association between COVID-19 and sex hormone levels
To the best of our knowledge, our meta-analysis is the first to systematically assess the potential correlation between COVID-19 and sex hormone levels. In total, we included 22 studies for estimation of the effect size for sex hormone levels. Overall, patients with COVID-19 exhibited a significant change in the levels of sex hormones. Specifically, COVID-19 patients showed a significant decrease in the levels of T/LH, FSH/LH, and SHBG hormones. In contrast, COVID-19 patients displayed a significant increase in the levels of LH, and E2/T. There were no significant changes in levels of FT, FSH, PRL, E2, or progesterone. Sensitivity analysis and subgroup analyses both identified an obvious association between sex hormone levels and the risk of COVID-19.
Low SHBG levels seem to correlate with worse COVID-19 prognosis (Table 2). SHBG levels are known to be inversely related to obesity and insulin-resistance (34). Increasing evidence demonstrates that obesity is reversely associated with the development of COVID-19 (35). Thus, low plasma SHBG level predicts the severity of COVID-19.
Notably, this is closely linked to the infection-related failure of homeostatic HPG axis feedback to compensate for weakened AR signalling (36). Production of testosterone by testicular Leydig cells is tightly regulated by the hypothalamic-pituitary-gonadal (HPG) axis, forming a homeostatic negative feedback loop (37). Secondary hypogonadism involves pathology of the pituitary or hypothalamus, leading to disturbance in the HPG axis and subsequently in reduced testosterone (38). In addition, the overall milieu of clinical androgenicity (sexual function, bone density and haematopoiesis) results from interaction between polyQ polymorphism in the androgen receptor (AR) and serum testosterone (T) levels. The AR gene, located on the X chromosome, encodes a member of the receptor group that binds to and mediates the actions of androgens (39). AR gene polymorphisms and testosterone level may enhance the behaviours involved in obtaining and maintaining high social status and reproductive success in men (40). Therefore, HPG axis insufficiency and androgen receptor polymorphisms are jointly involved in testosterone function. According to the present meta-analysis, higher gonadotropin concentrations (LH) may increase COVID-19 risk and severity, whereas FT was relatively lower in these patients. These results suggest a decrease in peripheral organ function and a compensatory increase in central function. While, a compensatory increase in central function” which may be nonetheless insufficient due to the “infection-related failure of homeostatic HPG axis feedback.
Many risk factors associated with the progression of COVID-19 to a severe and critical stage have been identified, including old age, male sex, underlying comorbidities such as hypertension, diabetes, obesity, chronic lung disease, heart, liver and kidney diseases, and tumours, clinically apparent immunodeficiencies, local immunodeficiencies such as early type I interferon secretion capacity, and pregnancy. Sex hormones such as oestrogen and testosterone as well as sex chromosome complement likely contribute to sex differences in blood pressure (BP) and cardiovascular disease (CVD). At the cellular level, differences in cell senescence pathways may contribute to increased longevity in women and may also the limit organ damage caused by hypertension. In addition, many lifestyle and environmental factors - such as smoking, alcohol consumption and diet - may influence BP and CVD in a sex-specific manner. Evidence suggests that cardioprotection in women is lost under conditions of obesity and type 2 diabetes mellitus. Treatment strategies for hypertension and CVD that are tailored according to sex may lead to improved outcomes for affected patients. We also found that FSH and LH levels to be significantly increased in female COVID-19 patients. However, in male patients, only LH levels were significantly increased, and there was no significant difference in FSH expression. Treatment strategies for COVID-19 that are tailored according to sex may lead to improved outcomes for patients. This also reflects the different manifestations of sex in disease.
Underlying mechanisms of COVID-19 effects on sex hormone levels
In COVID-19, SARS-CoV-2 may directly act on ACE2-positive spermatogonia, Sertoli cells and Leydig cells, resulting in disruption of spermatogenesis and male gonadal function (41).
Furthermore, in addition to the direct damage to the testes by viruses, other factors, such as fever, inflammation, and dysregulation of the hypothalamic-pituitary-gonadal axis (HPG axis), may also play a role in testosterone secretion or sperm production (42).
During viral infection, virus-induced inflammation leads to systemic or local production of cytokines, such as interleukin-6 (IL-6), tumor necrosis factor-α (TNF-α), interferon (IFN) and monocyte chemoattractant protein 1 (MCP-1). These cytokines are harmful to testicular cells. For example, IL-6 inhibits the differentiation of Leydig cells. In addition, IFN-γ has been shown to suppress the expression of the rate-limiting enzyme steroidogenic acute regulatory protein (StAR) to inhibit testosterone production (43–45). In addition, COVID-19 has also been reported within the central nervous system, including increased antidiuretic hormone secretion (46). The emotional, physical, or psychological stresses and pain associated with infections can affect the hypothalamohypophyseal axis (47). Thus, abnormalities in the hypothalamic-pituitary axis and abnormalities in LH secretion rhythm may also be a possible cause.
Aromatase is the enzyme responsible for the conversion of testosterone and androstenedione into E2 and oestrone, respectively. In men, aromatase is expressed in the testes (mainly in Leydig cells) as well as in a number of extragonadal sites, including adipose tissue, bone, the breasts and the brain (48). The upregulation of aromatase enzyme production in adipose tissue during critical illness (as a possible consequence of the excessive production of proinflammatory cytokines) may promote the conversion of testosterone to oestradiol (49–52).
Inflammatory mediators contribute to the suppression of the gonadal axis, and an increased metabolic clearance rate of testosterone may be the underlying cause of lower testosterone levels (53, 54).
There was no significant difference in the values of oestradiol in patients with COVID-19. The probable cause is that a potential upregulation of aromatase enzyme production in adipose tissue during COVID-19, possibly due to inflammatory cytokines, is likely to increase the conversion of testosterone to oestradiol.
Strengths and limitations
The advantages of this study lie in the study design, which included cohort studies, cross-sectional studies, and case-control studies. To the best of our knowledge, our meta-analysis comprising 22 studies is the largest and first meta-analysis to evaluate the relationship between sex hormone levels and COVID-19. There are, however, also a number of disadvantages. First, the present meta-analysis had substantial heterogeneity across studies, which might be due to differences in study design and inconsistencies in baseline characteristics. Second, none of the studies were RCTs, so any causal pathway conclusions should be treated with appropriate caution. Third, many factors may have an impact on sex hormone levels, including genetic polymorphisms, age, other health conditions, sun exposure behaviour, and season. We thus cannot rule out that potential risk factors might have influenced our results. Finally, there is no specific cut-off point for sex hormone levels, which is important for clinical relevance. In the future, we will consider additional confounding factors to obtain more reliable and repeatable results.
Conclusion
Overall, this study demonstrated that changes in sex hormone levels, such as decreased T/LH, FSH/LH, and SHBG levels and elevated LH, and E2/T levels, were strongly correlated with the severity and prognosis of COVID-19. We suggest that clinicians should be aware of the changes in sex hormone parameters of COVID-19 patients and seek guidance for treatment.
Data availability statement
The original contributions presented in the study are included in the article/supplementary material. Further inquiries can be directed to the corresponding author.
Author contributions
JinZ coordinated the study. ZC had the idea for the study, along with JiaZ, YJ and JinZ, contributed to the study design, literature search, figures, statistical analysis, data synthesis of outcomes and drafted and edited the final paper. All authors critically revised the report. All members have confirmed and agreed to submit the manuscript.
Funding
This work was supported by grants from the National Natural Science Foundation of China (82070807, 91749118, 81770775, 81730022), the Planned Science and Technology Project of Hunan Province (2017RS3015) and National key research and development program (2019YFA0801903, 2018YFA2000100).
Conflict of interest
The authors declare that the research was conducted in the absence of any commercial or financial relationships that could be construed as a potential conflict of interest.
Publisher’s note
All claims expressed in this article are solely those of the authors and do not necessarily represent those of their affiliated organizations, or those of the publisher, the editors and the reviewers. Any product that may be evaluated in this article, or claim that may be made by its manufacturer, is not guaranteed or endorsed by the publisher.
References
1. Wang J, Du G. COVID-19 may transmit through aerosol. Ir J Med Sci (2020) 189(4):1143–4. doi: 10.1007/s11845-020-02218-2
2. Matrajt L, Eaton J, Leung T, Dimitrov D, Schiffer JT, Swan DA, et al. Optimizing vaccine allocation for COVID-19 vaccines: Potential role of single-dose vaccination. medRxiv (2021) 12(1):3449. doi: 10.1101/2020.12.31.20249099
3. Mafham MM, Spata E, Goldacre R, Gair D, Curnow P, Bray M, et al. COVID-19 pandemic and admission rates for and management of acute coronary syndromes in England. Lancet (2020) 396(10248):381–9. doi: 10.1016/S0140-6736(20)31356-8
4. Lim MA, Pranata R, Huang I, Yonas E, Soeroto AY, Supriyadi R. Multiorgan failure with emphasis on acute kidney injury and severity of COVID-19: Systematic review and meta-analysis. Can J Kidney Health Dis (2020) 7:2054358120938573. doi: 10.1177/2054358120938573
5. Henry BM, de Oliveira MHS, Benoit S, Plebani M, Lippi G. Hematologic, biochemical and immune biomarker abnormalities associated with severe illness and mortality in coronavirus disease 2019 (COVID-19): A meta-analysis. Clin Chem Lab Med (2020) 58(7):1021–8. doi: 10.1515/cclm-2020-0369
6. Pivonello R, Auriemma RS, Pivonello C, Isidori AM, Corona G, Colao A, et al. Sex disparities in COVID-19 severity and outcome: Are men weaker or women stronger? Neuroendocrinology (2021) 111(11):1066–85. doi: 10.1159/000513346
7. Perrotta F, Corbi G, Mazzeo G, Boccia M, Aronne L, D'Agnano V, et al. COVID-19 and the elderly: Insights into pathogenesis and clinical decision-making. Aging Clin Exp Res (2020) 32(8):1599–608. doi: 10.1007/s40520-020-01631-y
8. Jin JM, Bai P, He W, Wu F, Liu XF, Han DM, et al. Gender differences in patients with COVID-19: Focus on severity and mortality. Front Public Health (2020) 8:152. doi: 10.3389/fpubh.2020.00152
9. South AM, Diz DI, Chappell MC. COVID-19, ACE2, and the cardiovascular consequences. Am J Physiol Heart Circ Physiol (2020) 318(5):H1084–90. doi: 10.1152/ajpheart.00217.2020
10. Wang Z, Xu X. scRNA-seq profiling of human testes reveals the presence of the ACE2 receptor, a target for SARS-CoV-2 infection in spermatogonia, leydig and sertoli cells. Cells (2020) 9(4):920. doi: 10.3390/cells9040920
11. Gutmann C, Takov K, Burnap SA, Singh B, Ali H, Theofilatos K, et al. SARS-CoV-2 RNAemia and proteomic trajectories inform prognostication in COVID-19 patients admitted to intensive care. Nat Commun (2021) 12(1):3406. doi: 10.1038/s41467-021-23494-1
12. Hozo SP, Djulbegovic B, Hozo I. Estimating the mean and variance from the median, range, and the size of a sample. BMC Med Res Methodol (2005) 5:13. doi: 10.1186/1471-2288-5-13
13. Stang A. Critical evaluation of the Newcastle-Ottawa scale for the assessment of the quality of nonrandomized studies in meta-analyses. Eur J Epidemiol (2010) 25(9):603–5. doi: 10.1007/s10654-010-9491-z
14. Infante M, Pieri M, Lupisella S, D'Amore L, Bernardini S, Fabbri A, et al. Low testosterone levels and high estradiol to testosterone ratio are associated with hyperinflammatory state and mortality in hospitalized men with COVID-19. Eur Rev Med Pharmacol Sci (2021) 25(19):5889–903. doi: 10.26355/eurrev_202110_26865
15. Gul A, Zengin S, Dundar G, Ozturk M. Do SARS-CoV-2 infection (COVID-19) and the medications administered for its treatment impair testicular functions? Urol Int (2021) 105(11-12):944–8. doi: 10.1159/000517925
16. Koç E, Keseroğlu BB. Does COVID-19 worsen the semen parameters? Early Results Tertiary Healthcare Center. Urol Int (2021) 105(9-10):743–8. doi: 10.1159/000517276
17. Ma L, Xie W, Li D, Shi L, Ye G, Mao Y, et al. Evaluation of sex-related hormones and semen characteristics in reproductive-aged male COVID-19 patients. J Med Virol (2021) 93(1):456–62. doi: 10.1002/jmv.26259
18. Temiz MZ, Dincer MM, Hacibey I, Yazar RO, Celik C, Kucuk SH, et al. Investigation of SARS-CoV-2 in semen samples and the effects of COVID-19 on male sexual health by using semen analysis and serum male hormone profile: A cross-sectional, pilot study. Andrologia (2021) 53(2):e13912. doi: 10.1111/and.13912
19. Xu H, Wang Z, Feng C, Yu W, Chen Y, Zeng X, et al. Effects of SARS-CoV-2 infection on male sex-related hormones in recovering patients. Andrology (2021) 9(1):107–14. doi: 10.1111/andr.12942
20. Camici M, Zuppi P, Lorenzini P, Scarnecchia L, Pinnetti C, Cicalini S, et al. Role of testosterone in SARS-CoV-2 infection: A key pathogenic factor and a biomarker for severe pneumonia. Int J Infect Dis (2021) 108:244–51. doi: 10.1016/j.ijid.2021.05.042
21. Salonia A, Pontillo M, Capogrosso P, Gregori S, Tassara M, Boeri L, et al. Severely low testosterone in males with COVID-19: A case-control study. Andrology (2021) 9(4):1043–52. doi: 10.1111/andr.12993
23. Salonia A, Pontillo M, Capogrosso P, Gregori S, Carenzi C, Ferrara AM, et al. Testosterone in males with COVID-19: A 7-month cohort study. Andrology (2022) 10(1):34–41. doi: 10.1111/andr.13097
24. Rastrelli G, Di Stasi V, Inglese F, Beccaria M, Garuti M, Di Costanzo D, et al. Low testosterone levels predict clinical adverse outcomes in SARS-CoV-2 pneumonia patients. Andrology (2021) 9(1):88–98. doi: 10.1111/andr.12821
25. Çayan S, Uğuz M, Saylam B, Akbay E. Effect of serum total testosterone and its relationship with other laboratory parameters on the prognosis of coronavirus disease 2019 (COVID-19) in SARS-CoV-2 infected male patients: A cohort study. Aging Male (2020) 23(5):1493–503. doi: 10.1080/13685538.2020.1807930;
26. Dhindsa S, Zhang N, McPhaul MJ, Wu Z, Ghoshal AK, Erlich EC, et al. Association of circulating sex hormones with inflammation and disease severity in patients with COVID-19. JAMA Netw Open (2021) 4(5):e2111398. doi: 10.1001/jamanetworkopen.2021.11398
27. Beltrame A, Salguero P, Rossi E, Conesa A, Moro L, Bettini LR, et al. Association between sex hormone levels and clinical outcomes in patients with COVID-19 admitted to hospital: An observational, retrospective, cohort study. Front Immunol (2022) 13:834851. doi: 10.3389/fimmu.2022.834851
28. Ding T, Zhang J, Wang T, Cui P, Chen Z, Jiang J, et al. Potential influence of menstrual status and sex hormones on female severe acute respiratory syndrome coronavirus 2 infection: A cross-sectional multicenter study in Wuhan, China. Clin Infect Dis (2021) 72(9):e240–8. doi: 10.1093/cid/ciaa1022
29. Cinislioglu AE, Cinislioglu N, Demirdogen SO, Sam E, Akkas F, Altay MS, et al. The relationship of serum testosterone levels with the clinical course and prognosis of COVID-19 disease in male patients: A prospective study. Andrology (2022) 10(1):24–33. doi: 10.1111/andr.13081
30. Zheng S, Zou Q, Zhang D, Yu F, Bao J, Lou B, et al. Serum level of testosterone predicts disease severity of male COVID-19 patients and is related to T-cell immune modulation by transcriptome analysis. Clin Chim Acta (2022) 524:132–8. doi: 10.1016/j.cca.2021.11.006
31. Salciccia S, Del Giudice F, Gentile V, Mastroianni CM, Pasculli P, Di Lascio G, et al. Interplay between male testosterone levels and the risk for subsequent invasive respiratory assistance among COVID-19 patients at hospital admission. Endocrine (2020) 70(2):206–10. doi: 10.1007/s12020-020-02515-x
32. Apaydin T, Sahin B, Dashdamirova S, Dincer Yazan C, Elbasan O, Ilgin C, et al. The association of free testosterone levels with coronavirus disease 2019. Andrology (2022) 10(6):1038–46. doi: 10.1111/andr.13152
33. Kolanska K, Hours A, Jonquière L, Mathieu d'Argent E, Dabi Y, Dupont C, et al. Mild COVID-19 infection does not alter the ovarian reserve in women treated with ART. Reprod BioMed Online (2021) 43(6):1117–21. doi: 10.1016/j.rbmo.2021.09.001
34. Casimiro I, Sam S, Brady MJ. Endocrine implications of bariatric surgery: a review on the intersection between incretins, bone, and sex hormones. Physiol Rep (2019) 7(10):e14111. doi: 10.14814/phy2.14111
35. Cai Z, Yang Y, Zhang J. Obesity is associated with severe disease and mortality in patients with coronavirus disease 2019 (COVID-19): A meta-analysis. BMC Public Health (2021) 21(1):1505. doi: 10.1186/s12889-021-11546-6
36. Schneider JE. Energy balance and reproduction. Physiol Behav (2004) 81(2):289–317. doi: 10.1016/j.physbeh.2004.02.007
37. Traish AM. Negative impact of testosterone deficiency and 5α-reductase inhibitors therapy on metabolic and sexual function in men. Adv Exp Med Biol (2017) 1043:473–526. doi: 10.1007/978-3-319-70178-3_22
38. Heinlein CA, Chang C. Androgen receptor (AR) coregulators: an overview. Endocr Rev (2002) 23(2):175–200. doi: 10.1210/edrv.23.2.0460
39. Butovskaya ML, Lazebny OE, Vasilyev VA, Dronova DA, Karelin DV, Mabulla AZ, et al. Androgen receptor gene polymorphism, aggression, and reproduction in Tanzanian foragers and pastoralists. PLoS One (2015) 10(8):e0136208. doi: 10.1371/journal.pone.0136208
40. Barbagallo F, Calogero AE, Cannarella R, Condorelli RA, Mongioì LM, Aversa A, et al. The testis in patients with COVID-19: Virus reservoir or immunization resource? Transl Androl Urol (2020) 9(5):1897–900. doi: 10.21037/tau-20-900
41. Carlsen E, Andersson AM, Petersen JH, Skakkebaek NE. History of febrile illness and variation in semen quality. Hum Reprod (2003) 18(10):2089–92. doi: 10.1093/humrep/deg412
42. Hu B, Huang S, Yin L. The cytokine storm and COVID-19. J Med Virol (2021) 93(1):250–6. doi: 10.1002/jmv.26232
43. Wang Y, Xie L, Li L, Li X, Li H, Liu J, et al. Interleukin 6 inhibits the differentiation of rat stem leydig cells. Mol Cell Endocrinol (2018) 472:26–39. doi: 10.1016/j.mce.2017.11.016
44. Lin T, Hu J, Wang D, Stocco DM. Interferon-gamma inhibits the steroidogenic acute regulatory protein messenger ribonucleic acid expression and protein levels in primary cultures of rat leydig cells. Endocrinology (1998) 139(5):2217–22. doi: 10.1210/endo.139.5.6006
45. Yousaf Z, Al-Shokri SD, Al-Soub H, Mohamed MFH. COVID-19-associated SIADH: a clue in the times of pandemic! Am J Physiol Endocrinol Metab (2020) 318(6):E882–5. doi: 10.1152/ajpendo.00178.2020
46. Leonard BE. The HPA and immune axes in stress: the involvement of the serotonergic system. Eur Psychiatry (2005) 20 Suppl 3:S302–6. doi: 10.1016/S0924-9338(05)80180-4
47. Stocco C. Tissue physiology and pathology of aromatase. Steroids (2012) 77(1-2):27–35. doi: 10.1016/j.steroids.2011.10.013
48. Carreau S, Lambard S, Delalande C, Denis-Galeraud I, Bilinska B, Bourguiba S, et al. Aromatase expression and role of estrogens in male gonad : A review. Reprod Biol Endocrinol (2003) 1:35. doi: 10.1186/1477-7827-1-35
49. Spratt DI, Morton JR, Kramer RS, Mayo SW, Longcope C, Vary CPH, et al. Increases in serum estrogen levels during major illness are caused by increased peripheral aromatization. Am J Physiol Endocrinol Metab (2006) 291(3):E631–8. doi: 10.1152/ajpendo.00467.2005
50. van den Berghe G, Weekers F, Baxter RC, Wouters P, Iranmanesh A, Bouillon R, et al. Five-day pulsatile gonadotropin-releasing hormone administration unveils combined hypothalamic-pituitary-gonadal defects underlying profound hypoandrogenism in men with prolonged critical illness. J Clin Endocrinol Metab (2001) 86(7):3217–26. doi: 10.1210/jcem.86.7.7680
51. Watanobe H, Hayakawa Y. Hypothalamic interleukin-1 beta and tumor necrosis factor-alpha, but not interleukin-6, mediate the endotoxin-induced suppression of the reproductive axis in rats. Endocrinology (2003) 144(11):4868–75. doi: 10.1210/en.2003-0644
52. Russell SH, Small CJ, Stanley SA, Franks S, Ghatei MA, Bloom SR, et al. The in vitro role of tumour necrosis factor-alpha and interleukin-6 in the hypothalamic-pituitary gonadal axis. J Neuroendocrinol (2001) 13(3):296–301. doi: 10.1046/j.1365-2826.2001.00632.x
53. Spratt DI, Bigos ST, Beitins I, Cox P, Longcope C, Orav J, et al. Both hyper- and hypogonadotropic hypogonadism occur transiently in acute illness: bio- and immunoactive gonadotropins. J Clin Endocrinol Metab (1992) 75(6):1562–70. doi: 10.1210/jcem.75.6.1464665
Keywords: COVID-19, sex hormones, meta-analysis, predict, WMD
Citation: Cai Z, Zhong J, Jiang Y and Zhang J (2022) Associations between COVID-19 infection and sex steroid hormones. Front. Endocrinol. 13:940675. doi: 10.3389/fendo.2022.940675
Received: 10 May 2022; Accepted: 14 September 2022;
Published: 11 October 2022.
Edited by:
Jeff M. P. Holly, University of Bristol, United KingdomReviewed by:
Luca Giovanelli, University of Milan, ItalyNazareth Novaes Rocha, Fluminense Federal University, Brazil
Copyright © 2022 Cai, Zhong, Jiang and Zhang. This is an open-access article distributed under the terms of the Creative Commons Attribution License (CC BY). The use, distribution or reproduction in other forums is permitted, provided the original author(s) and the copyright owner(s) are credited and that the original publication in this journal is cited, in accordance with accepted academic practice. No use, distribution or reproduction is permitted which does not comply with these terms.
*Correspondence: Jingjing Zhang, RG9jdG9yemhhbmdqakBjc3UuZWR1LmNu
†These authors have contributed equally to this work