- 1Department of Endocrinology, Morbid Obesity and Preventive Medicine, Oslo University Hospital, Oslo, Norway
- 2The University of Oslo, Faculty of Medicine, Oslo, Norway
- 3Department of Biotechnology, University of Insubria, Varese, Italy
- 4Department of Medicine and Surgery, University of Milano-Bicocca, Monza, Italy
- 5Department of Breast and Endocrine Surgery, Oslo University Hospital, Oslo, Norway
- 6Department of Pediatric Medicine, Oslo University Hospital, Oslo, Norway
- 7Global Virus Network, University of Insubria, Varese, Italy
- 8The Specialist Center Pilestredet Park, Oslo, Norway
Introduction: Evidence points to viral infections as possible triggers of autoimmune thyroid disease (AITD), but little is known about the prevalence of common viruses in the thyroid gland. Using a novel approach based on virus enrichment in multiple cell lines followed by detection of the viral genome and visualization of viral proteins, we investigated the presence of multiple human viruses in thyroid tissue from AITD patients and controls.
Methods: Thyroid tissue was collected by core needle biopsy or during thyroid surgery from 35 patients with AITD (20 Graves’ disease and 15 Hashimoto’s thyroiditis). Eighteen thyroid tissue specimens from patients undergoing neck surgery for reasons other than thyroid autoimmunity served as controls. Specimens were tested for the presence of ten different viruses. Enteroviruses and human herpesvirus 6 were enriched in cell culture before detection by PCR and immunofluorescence, while the remaining viruses were detected by PCR of biopsied tissue.
Results: Forty of 53 cases (75%) carried an infectious virus. Notably, 43% of all cases had a single virus, whereas 32% were coinfected by two or more virus types. An enterovirus was found in 27/53 cases (51%), human herpesvirus 6 in 16/53 cases (30%) and parvovirus B19 in 12/53 cases (22%). Epstein-Barr virus and cytomegalovirus were found in a few cases only. Of five gastroenteric virus groups examined, only one was detected in a single specimen. Virus distribution was not statistically different between AITD cases and controls.
Conclusion: Common human viruses are highly prevalent in the thyroid gland. This is the first study in which multiple viral agents have been explored in thyroid. It remains to be established whether the detected viruses represent causal agents, possible cofactors or simple bystanders.
Introduction
Autoimmune thyroid diseases (AITD), mainly comprising Hashimoto’s thyroiditis (HT) and Graves’ disease (GD), are the most common autoimmune endocrine disorders and affect 2-6% of the population (1). Predisposing genetic factors include HLA genes and other immunoregulatory genes (2–6). However, environmental factors are believed to play an important part as well. Viral infections may be one such environmental factor, and there is mounting evidence that viruses play a role in thyroid autoimmunity (7, 8).
Enteroviruses (9–11), parvovirus B19 (12–14) and hepatitis C virus (HCV) (15, 16) have all been associated with AITD. Among herpesviruses, Epstein-Barr virus (EBV) has been associated with GD (17–19), while human herpes virus 6 (HHV-6) and cytomegalovirus (CMV) may be associated with both HT and GD (20–24). The aforementioned viruses may produce subclinical disease and persistent infection at any age (25–29).
Case reports and autopsy studies show further associations of AITD with viral infections: rubella virus in congenital illness (30), human T cell leukemia virus (31), Hantaan virus (32), hepatitis E virus (33), HIV (34) and – more recently – the Severe Acute Respiratory Syndrome Coronavirus-2 (35–39). In addition, various gastroenteric viruses have been detected in children with other autoimmune disorders such as celiac disease and type 1 diabetes (40, 41).
We set out to investigate the presence of highly circulating viruses in a well-characterized and large collection of thyroid tissue samples. Following indications of literature, the presence of the following viruses were examined: enteroviruses, parvovirus B19, HHV-6, EBV, CMV, HCV and five gastroenteric viruses (adenovirus, astrovirus, norovirus, rotavirus and sapovirus). The small amount of biopsied thyroid tissue and the need for a wide spectrum of viral detection techniques capable of revealing a broad range of RNA and DNA agents were particularly challenging (42, 43). Results indicate that several common human viruses are frequently present in thyroid tissue from ATID patients and controls alike.
Methods
Study Participants, Collection of Thyroid Tissue and Blood Donors
We investigated a previously described collection of thyroid tissue samples stored at the Oslo University Hospital (11, 44). Fifteen HT samples were collected by core needle biopsy, while 20 GD samples were collected either by core needle biopsy or from surgical samples. Thyroid tissue samples from 18 patients undergoing neck surgery for reasons other than AITD (i.e. thyroid tumors or parathyroid adenomas) served as controls. Controls with positive anti-thyroid antibodies were excluded. Specimens were snap frozen in liquid nitrogen and stored at - 80°C. The Regional Ethics Committee approved the study (REK no. 1.2006.1950) and written informed consent was obtained from all participants. A group of blood donors (Varese, Italy) was introduced as a technical control for evaluating the prevalence of the investigated viruses in blood leukocytes of healthy subjects. The virology study was approved by the Ethics Committee of Ospedale di Circolo and Fondazione Macchi (Varese, Italy; 2018/02357094) and performed in accordance with the Declaration of Helsinki and local regulatory laws.
Cultured Cell Lines Permissive for Enteroviruses and HHV-6
Five cell lines (AV3, RD, Ht-29, VC3 and HEK293) were obtained from The European Collection of Authenticated Cell Cultures and The American Type Culture Collection. These cell lines produce the main entry factors for enteroviruses (45, 46) and the two entry factors for HHV-6 (47, 48). Expression of virus receptors was evaluated by indirect immunofluorescence (IF) using the primary and secondary antibodies listed in Table S1.
Cell lines were cultured at 37°C in air with 5% CO2 using DMEM/F12 medium supplemented with L-glutamine, 7% heat-inactivated fetal bovine serum and penicillin/streptomycin. Cultures were checked monthly for mycoplasma contamination (MycoAlert ™PLUS Mycoplasma Detection Kit, Euroclone-Lonza, Pero, Italy).
PCR Detection of Enterovirus and HHV-6 in Thyroid Tissue Following Enrichment in Cell Culture
Following published protocols (43, 49), thyroid homogenates were made in medium containing PANTA (a mixture of antibacterials and antifungals) using a FastPrep-24™ blender with glass beads (MP Biomedicals, Eschewege, Germany). Homogenates were cultured with the five cell lines described above using T25 flasks. After 2-3 serial passages, to detect viral genomes, DNA and RNA were extracted from each flask (supernatant plus cells) using an automated m2000sp instrument (Abbott Molecular, Rome, Italy). RNA was reverse transcribed to cDNA using SuperScript™ III Reverse Transcriptase and VILO™ Master Mix (Thermo Fisher Scientific-Invitrogen, Monza, Italy). End-point PCR assays for enteroviruses and HHV-6 were run in duplicates on Veriti™ Dx thermal cyclers (Applied Biosystems, Thermo Fischer, Monza, Italy) in a final volume of 50 μl using 15 μl of template. Five different sets of enterovirus-specific primer pairs targeting the 5’untranslated region of enterovirus genomes were used (Table S2). For HHV-6, we used pan-HHV-6 primers targeting a conserved sequence that comprise the cytoplasmic envelopment protein 2 - tripartite terminase subunit 3 (Table S2). PCR tests were deemed positive when an amplicon of the expected size was observed in the electropherogram. The viral nature of amplicons was verified by Sanger sequencing. Public databases were employed for attributing the sequences to the appropriate virus species. A schematic presentation of the method is provided (Figure 1).
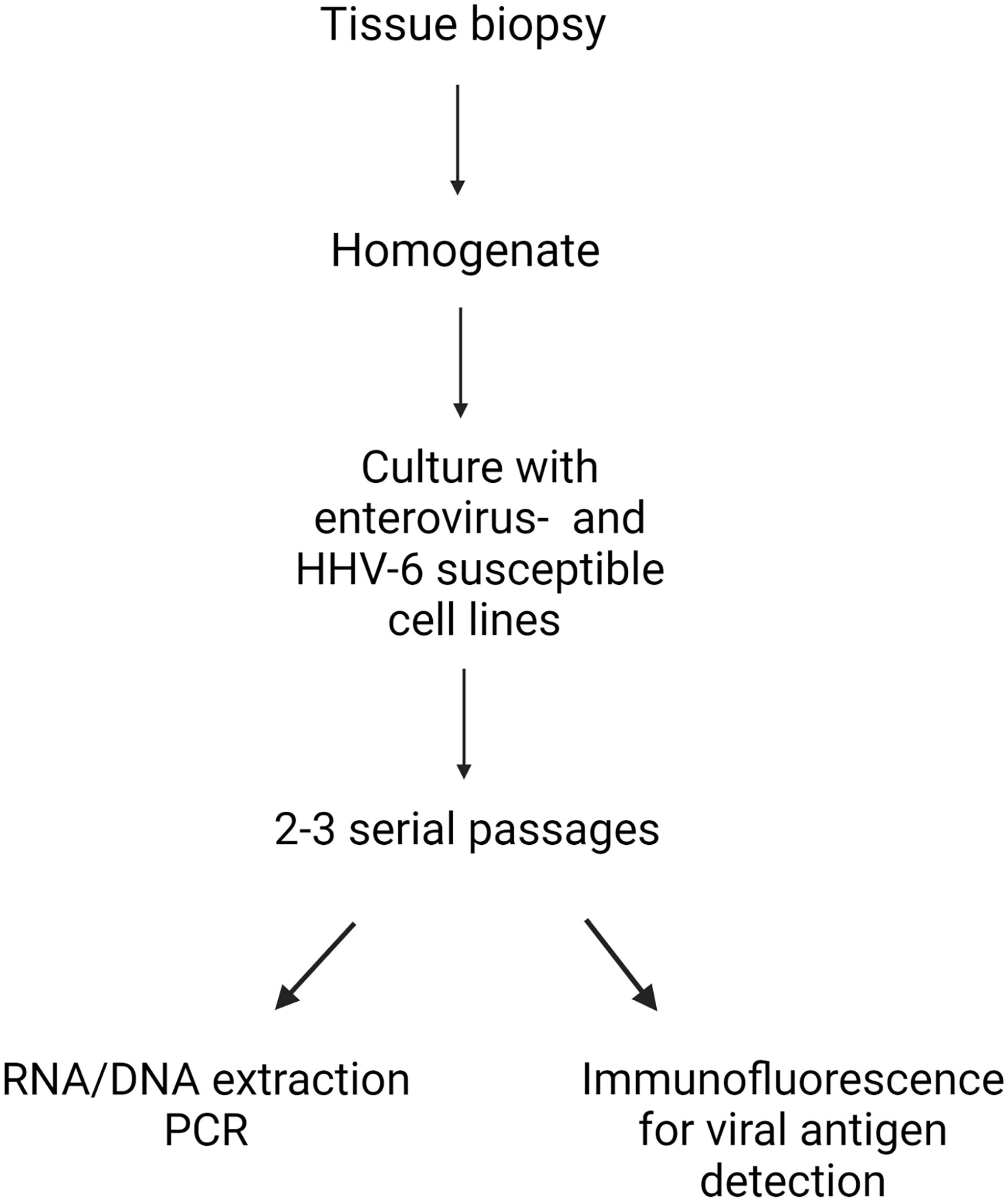
Figure 1 Model of the integrated procedure used for deriving virus strains from thyroid tissue.Thyroid biopsy tissues were made into homogenates, and then cultured with five cell lines with proven enterovirus- and human herpesvirus 6 (HHV-6) entry factors. After 2-3 serial passages, RNA (enterovirus) and DNA (HHV-6) were extracted, before viral genome detection by PCR. For each case, the expression of enterovirus and HHV-6 antigens in cultured cells were evaluated by immunofluorescence with a panel of enterovirus and HHV-6 antibodies. Created with BioRender.com.
Antigen Detection of Enteroviruses and HHV-6 Antigens in Infected Cell Cultures
Cell cultures from the previously described five cell lines in Millicell®EZ slide 4-well glass (Merck-Millipore, Vimodrone, Italy) were incubated with supernatant from T25 flasks containing the five cell line mix exposed to thyroid tissue homogenates. After 3-4 days, cell monolayers were fixed in PBS containing 4% paraformaldehyde. Expression of enterovirus protein antigens was evaluated by IF with anti-EV monoclonal antibodies (mAbs) (Table S1) targeting either the VP1 capsid protein (mAbs 9D5, 6-E9/2, 5-D8/1) or the viral 3D RNA polymerase (mAbs 3D-02 and 3D-05; our own laboratory) (50). Enteroviral VP1 staining was deemed positive when granular cytoplasmic fluorescence was detected (Figure 2). HHV-6 antigens were detected with a mouse mAb directed at the 140 kDA capsid polypeptide.
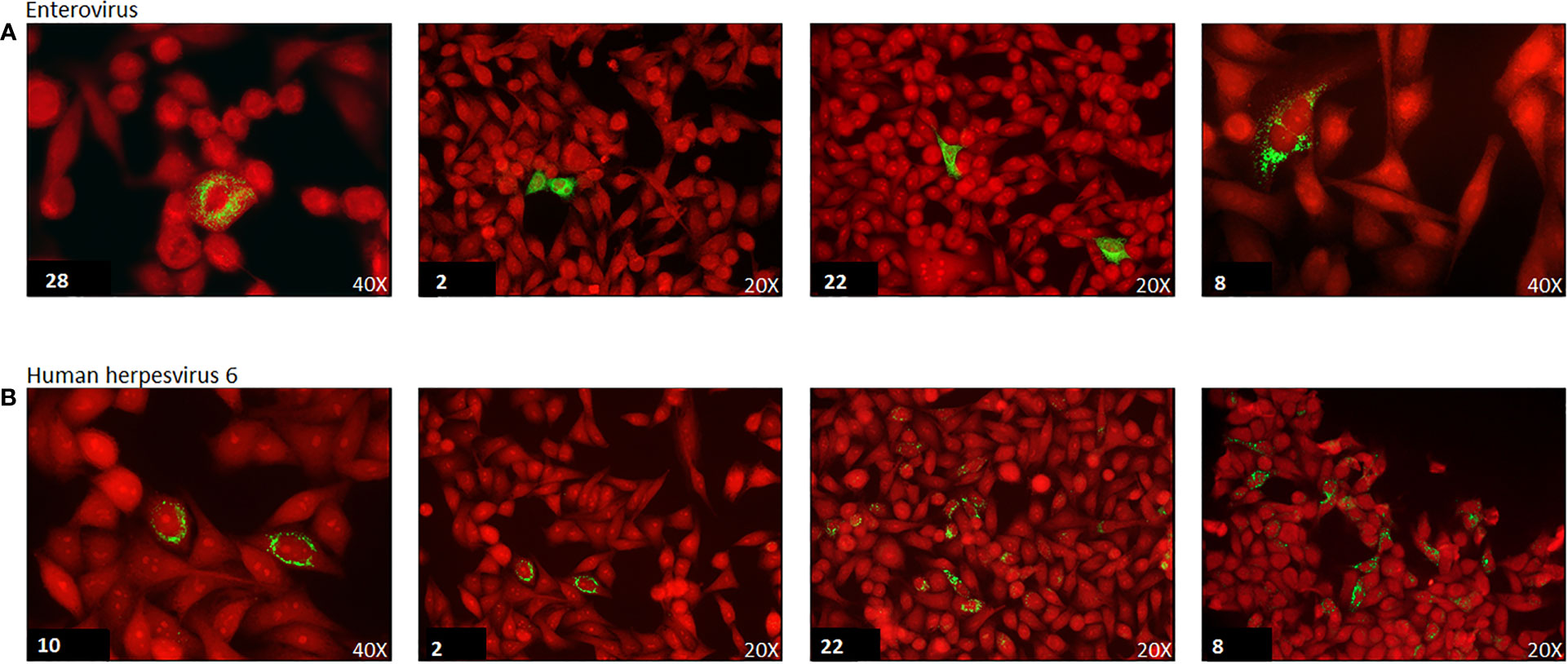
Figure 2 Immunofluorescence of enterovirus and human herpesvirus 6 (HHV-6) capsid protein in cell cultures of selected virus positive cases. Green immunofluorescence of cultured cell monolayers infected with enterovirus (panel A) or human herpesvirus-6 (panel B). Virus strains isolated from the thyroid of representative AITD cases. Case number is reported in the lower left corner. Red: Evans Blue counterstain. The original magnification is indicated (20x or 40x). Enterovirus staining of the VP1 capsid protein antigen by monoclonal antibody 6-E9/2. HHV-6 staining of the 140KD capsid polypeptide by monoclonal antibody F24H.
Whole Genome Amplification and qPCR for Viral Agents Other Than EVs and HHV-6
Since we searched for multiple viral agents, the small quantity of thyroid tissue posed a challenge. Thus, we resorted to whole genome amplification (WGA) technique for obtaining amounts of DNA templates sufficient for numerous PCR tests. The WGA2 method (Sigma-Aldrich, Milano Italy) was used according to the manufacturer’s protocol. Whereas DNA extracted from thyroid tissue was used directly in the WGA2 procedure, before WGA2 the RNA had to be converted into cDNA by reverse transcriptase (described above). qPCR assays were run in duplicate using 10 μl of WGA2-derived DNA template. A total volume of 25 μl was used for each PCR reaction run on ABI Prism 7500 thermal cyclers (Thermo Fischer-Applied Biosystems, Monza, Italy). Table S3 shows the qPCR kits that have been used to detect CMV, EBV, parvovirus B19, HCV, adenoviruses F40-F41, astrovirus species 1-8, norovirus genogroups I and II, rotaviruses, sapoviruses. The analytical sensitivity of qPCR tests was 10-100 genome equivalents per reaction.
Peripheral Blood Leukocytes From Blood Donors
Peripheral blood leukocytes from 19 healthy blood donors were used as technical controls. The investigated viruses were searched for in peripheral blood leukocytes of healthy persons since these cells represent a possible virus reservoir in persisting and latent infection. Leukocytes (including granulocytes) were obtained from a fresh aliquot of blood by centrifugation on discontinuous Ficoll-Hypaque gradients (density 1.077 and 1.119 g/ml). After washing 2x with medium, the leukocytes were cocultured with the aforementioned five cell line mix and processed as described above for detection of enterovirus and HHV-6. A leukocyte aliquot was preserved at -70°C for nucleic acids extraction, which was used for qPCR for viral agents other than enterovirus and HHV-6.
Statistics
Demographic and clinical data of the investigated groups are presented as means with standard deviations or medians with interquartile ranges. The statistical significance of differences in mean was calculated using the T-test for normally distributed values in independent groups, or by the Mann-Whitney U test to compare non-normally distributed outcomes in independent groups. Results of virus tests are presented as numbers with percentages. The Pearson’s chi-square test was used to determine the statistical significance of differences in proportions. Analyses were performed using IBM SPSSS Statistics version 25. Statistical significance was set at p<0.05.
Results
We investigated the presence of enteroviruses, parvovirus B19, HHV-6, EBV, CMV and HCV in addition to five gastroenteric viruses (adenovirus, astrovirus, norovirus, rotavirus and sapovirus) in thyroid tissue from AITD patients and controls. Patient characteristics are summarized in Table 1, and virus detection results are summarized in Table 2. Enteroviruses and HHV-6 were sought for using pre-enrichment of virus in cell culture before PCR and IF assays. As a technical control, the investigated viruses were searched for in peripheral blood leukocytes of 19 blood donors and were rarely detected: single, separated cases of EV, HHV-6 and EBV in three donors.
Regarding enteroviruses, genomes were detected by PCR in 19/53 (36%) thyroid specimens. However, IF for the VP1 capsid protein proved more sensitive with 25/53 (47%) specimens being positive. In cells persistently infected by EVs, staining for the 3D RNA polymerase produced dotted fluorescence in the nuclear area (Figure 2). Twentyseven samples in total were enterovirus positive, with concordant PCR and IF results in 17 of the samples (63%). Enteroviruses were more frequent in AITD compared to controls, albeit not significantly: 67% in the HT group, 50% in the GD group and 39% in thyroid controls. Enterovirus sequences (relative to the partially conserved 5’UTR or to the VP4-VP2 genome regions) matched those of members of the A and B enteroviral species or - in a few cases – of the rhinovirus C species (Table 3). Regarding HHV-6, the PCR and IF methods were always concordant and positive in 16/53 cases (30%). The HHV-6 capsid protein antibody produced granular cytoplasmic fluorescence, often perinuclear (Figure 2). Regarding HHV-6, genome sequences refer to a strongly conserved tract (CEP2-TRM3). The sensitive PCR method and the antibodies used for HHV-6 detection did not allow discerning the A from the B species of the virus. The HHV-6 distribution did not differ significantly among the three investigated groups.
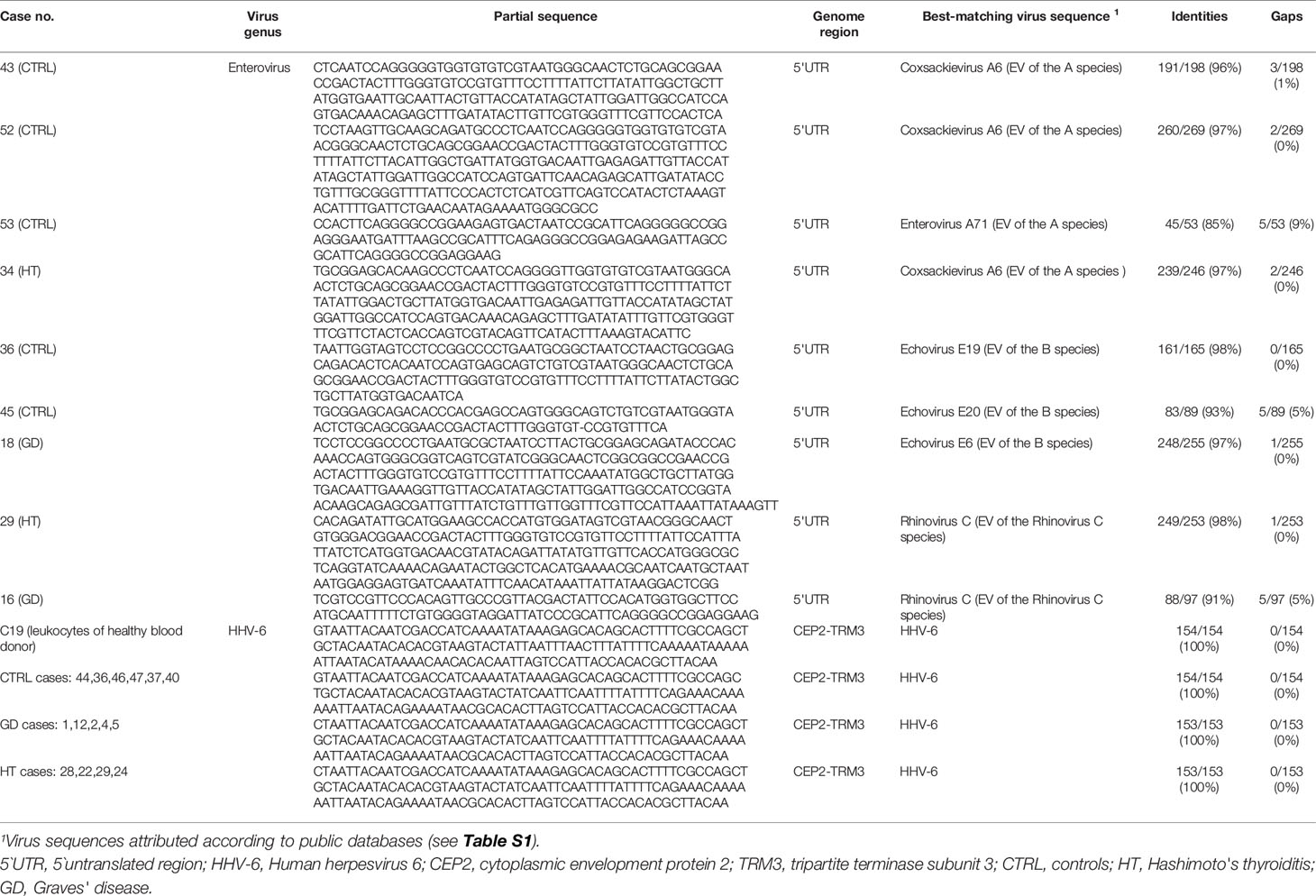
Table 3 RNA or DNA genome sequences representative of the enterovirus and HHV-6 strains obtained from the thyroid tissue of investigated cases.
Parvovirus B19 – a virus producing a variety of clinical manifestations (51), including thyroid disorders (7, 12), was investigated using PCR amplification alone. Twelve out of 53 cases (23%) were positive. The detection rate between the three groups did not differ significantly.
Among the five taxonomic groups of gastroenteric viruses studied, a single GD case carried levels of a rotavirus strain close to the detection limit, indicating that gastroenteric viruses rarely infect the thyroid gland. EBV and CMV - two members of the herpesvirus group known to cause life-long latent infections in humans -were detected only in a few cases. Although the association of HCV with AITD has been demonstrated (15, 16), HCV was not detected in any of the investigated thyroid specimens.
The thyroid specimens were rarely devoid of viruses. Seventy-five percent of the investigated cases carried at least one virus in the gland, suggesting that unapparent viral infection of the thyroid is commonplace (Table 4). Concomitant infections with enteroviruses, HHV-6 and parvovirus B19, the viruses most frequently detected, were identified in several samples (Table 5). A single virus was found in 23/53 cases (43%), while 17/53 cases (32%) harbored two or three different agents. The viral load in thyroid tissue was consistently low, but some samples contained more virus that others. The inter-sample differences, however, were insignificant and could not be related to clinical status.
Discussion
In this study, we investigated the presence of multiple viral agents in 53 thyroid tissue samples. To our knowledge, this is the first study to assess such a wide range of viruses in the thyroid. Results show that some human viruses are frequently present in the thyroid gland.
The Enterovirus genus contains more than 260 distinct virus types (52, 53). This makes the detection and distinction of the thyroid-infecting enterovirus types challenging. To increase the probability of virus detection, we increased the viral amount by enrichment in cell culture prior to searching for viral nucleic acids and antigens. Proof of live enteroviruses and HHV-6 in thyroid was obtained by: a) genome detection and sequencing as well as by b) visualization of viral proteins in cultured cells.
Enteroviruses were present in a high proportion of samples. The proportion of enterovirus-positive samples was slightly higher, albeit not significantly so, in the GD and HT group compared to controls. The majority of enterovirus-positive samples (63%) were positive by both PCR and IF assays. We previously published that the sensitivity of IF can be superior to PCR in the diagnosis of persistent infections (43), and analogous indications emerge from immunohistochemical studies (54). The huge variation of enterovirus genomes makes it impossible to match primer pairs with the enteroviral genomes of all genotypes. In contrast, the antigenic structure of the VP1 capsid protein, being more conserved, can be consistently detected by a small panel of pan-enterovirus antibodies.
By partial genome sequencing, the enterovirus strains found in thyroid appeared to belong to the A species (mainly Coxsackievirus A) or to the B species (likely echovirus types). However, the methods employed could not precisely identify the infecting virus genotype since this requires sequencing the VP1-VP2 enteroviral capsid region. This aim was not reached due to the minimal viral load present in the investigated thyroid samples (36, 43). Interestingly, surveillance and serology studies show that many different enterovirus types of the A and B species are circulating worldwide, often causing subclinical infection (55). More surprising is the finding of members of the rhinovirus C species within the thyroid. Notably, rhinoviruses are included in the Enterovirus genus (56, 57) and cause both the common cold and lower respiratory infections (58). A low-grade persistent enterovirus infection (not a latent one as in the case of herpesviruses) is linked to other autoimmune diseases, such as type 1 diabetes (40, 59–64). Experiments from our group showed that infection of cultured cells with enterovirus strains derived from the pancreas of type 1 diabetes cases enhance the expression of pro-inflammatory cytokines and chemokines associated with autoimmune disorders (65). In human thyroid, viral infection has also been shown to activate interferon signaling and the expression of interferon-stimulated genes (36, 49). Moreover, enhanced expression of HLA class I and of interferon-related STAT1 and PKR genes has been demonstrated in the thyroid tissue collection used in this study (9, 10).
HHV-6 comprises two species: HHV-6A which is more neurovirulent and associated with neuroinflammatory disorders (66) and HHV-6B that causes exanthema subitum (the sixth disease of infancy) (67). Unfortunately, the sensitive methods utilized in this study (PCR and IF) could not differentiate HHV-6A from HHV-6B. Thus, we may only conclude that an undetermined species of HHV-6 is frequently present in thyroid. Further research is needed in this regard since it has been suggested that HHV-6 of the A species is likely associated with HT and possibly with other autoimmune diseases (8, 21–23).
Among cases of coinfection, the association of enteroviruses with HHV-6 was the most frequent, followed by HHV-6 plus parvovirus B19, and enteroviruses plus parvovirus B19 (Table 4). It remains an open question whether carrying two or more viruses in the thyroid confers an increasing risk for AITD. It may be of interest to recall that HHV-6 transactivates EBV (68) and that the risk for multiple sclerosis increases when genetically predisposed persons become doubly infected with HHV-6A and EBV (69). Interestingly, a recent study shows that neonatal infection of mice with a roseolovirus related to HHV-6 may damage the thymus, thus disrupting central tolerance and causing autoimmunity later in life (70). Future studies may aim at evaluating whether similar dynamics may be operative in AITD.
Even though receptors for enterovirus, HCV and severe acute respiratory syndrome coronavirus 2, have been detected (9, 16, 71, 72), the entryway for viruses into the thyroid remains elusive. Since a wide range of viruses were detected, it seems reasonable to assume that there are multiple modes of entry to the thyroid. The majority of the investigated viruses (parvovirus B19, EBV, CMV, HHV-6 and enteroviruses) are transmitted via saliva and infect the mucosal epithelial cells of the oropharynx. The respiratory tract is in close proximity to the thyroid, thus one might speculate that respiratory viruses may enter the thyroid from the infected submucosal tissue. Moreover, the thyroid is highly vascularized, thus the blood stream could also be an entryway. Herpesviruses, like EBV, HHV-6 and CMV reside within host cells in a latent phase after primary infection (including granulocytes, lymphocytes and monocytes). EBV, HHV-6 and CMV are shed in the saliva, usually in the absence of symptoms, indicating that latency is a dynamic process and that reactivation may occur frequently, and usually in the absence of clinical disease or symptoms (73). Given the complexity of the herpesvirus life cycle, one explanation of the high prevalence of HHV-6 in thyroid tissue is fluctuating latent or low-grade infection in thyroid cells. The three viruses most frequently detected were enteroviruses of different species (51%) , HHV-6 (30%) and parvovirus B19 (22%). The seroprevalence of the two latter viruses in the adult population is high; approximately 70% for HHV-6 and 40-80% for parvovirus B19 (74, 75), thus, over their lifetime most people are being exposed to the above agents, in most cases without prolonged clinical signs. The high prevalence of infections by the latter agents may explain why the thyroid remains infected in some individuals.
Subacute thyroiditis (SAT) is a prevalent thyroid disease assumed to be of viral origin (76). Few authors have searched for virus within the thyroid gland in SAT, mainly due to the difficulty of justifying tissue biopsy or a needle aspirate in the absence of suspected neoplastic lesions. Evidence of virus from the few published studies is not conclusive, thus proving that viral etiology is hard to prove even though there is a strong clinical association of viral infection of the upper respiratory tract or influenza like malaise before the debut of SAT. Most people recover entirely from SAT, however, subsequent bouts of GD, persistent thyroid autoimmunity and a rise in thyroid autoantibodies occur (77–80).
Even though results show that the prevalence of the reported viral pathogens is not statistically different between AITD and thyroid controls, we do not believe that this upends the hypothesis that viruses may represent environmental triggers of thyroid autoimmunity. Actually, the findings may add evidence to this possibility proving that multiple viral agents are capable of producing unapparent infection of the gland. Moreover, genetic predisposition is a prerequisite for developing AITD. In genetically predisposed persons, susceptibility genes are expressed when the tissue undergoes microbial infection (81). The interplay of genetic predisposition and infectious insults could explain why common infections may give rise to autoimmunity in some but not in other individuals.
We recognize that criteria involving the detection of viral nucleic acids and proteins in diseased tissue cannot distinguish whether a virus is a causal agent, a causal cofactor or a simple bystander that homes to diseased tissue but does not contribute to pathology. However, the present study serves as a proof-of-concept and calls for further investigations of viral infections in the thyroid gland.
Data Availability Statement
The original contributions presented in the study are included in the article/Supplementary Material. Further inquiries can be directed to the corresponding author.
Ethics Statement
The studies involving human participants were reviewed and approved by The Regional Ethics Committee approved the study (REK no. 1.2006.1950) (Norway). The study was also approved by the Ethics Committee of Ospedale di Circolo and Fondazione Macchi (Varese, Italy; 2018/02357094). The patients/participants provided their written informed consent to participate in this study.
Author Contributions
TW, AG, FB, and AT performed experiments, analyzed data, and drafted the manuscript. TP contributed to thyroid surgery and core needle thyroid sampling, and writing of the manuscript. SH contributed to all parts of the study, study design, clinical coordination and patient recruitment, data collection, analysis, and interpretation, and drafting of the manuscript. KD-J, as the principal investigator of the study, had the initial idea of the study and contributed to the study design, regulatory issues, international collaboration, data collection, analysis, and interpretation, and writing of the manuscript. KD-J and AT obtained funding.
TW, SH, and AT are the guarantors of this work and, as such, had full access to all the data in the study and take responsibility for the integrity of the data and the accuracy of the data analysis. Furthermore, this study has been performed in full adherence to ethical and legal requirements in Norway and Italy.
Funding
South-Eastern Norway Regional Health Authority (HSØ grants to SH and KD-J) financed this work. For virus studies, funds have been obtained by the Juvenile Diabetes Research Foundation (JDRF grant 25-2012-770 to AT) and the Italian Ministry of Health (grant PE-2013-02357094 to AT). This research was performed with the support of the Network for Pancreatic Organ donors with Diabetes (nPOD; RRID: SCR_014641), a collaborative type 1 diabetes research project sponsored by JDRF (nPOD: 5-SRA-2018-557-Q-R). The content and views expressed are the responsibility of the authors and do not necessarily reflect the official view of nPOD. Organ Procurement Organizations partnering with nPOD to provide research resources are listed at http://www.jdrfnpod.org/for-partners/npod-partners.
Conflict of Interest
The authors declare that the research was conducted in the absence of any commercial or financial relationships that could be construed as a potential conflict of interest.
Publisher’s Note
All claims expressed in this article are solely those of the authors and do not necessarily represent those of their affiliated organizations, or those of the publisher, the editors and the reviewers. Any product that may be evaluated in this article, or claim that may be made by its manufacturer, is not guaranteed or endorsed by the publisher.
Acknowledgments
First, we are grateful to all study participants and patients without whom this work could not have been done. The study has been conducted in collaboration with The Global Virus Network (Baltimore, Maryland, USA) and the Centro Linceo Beniamino Segre, Accademia dei Lincei (Rome, Italy).
Supplementary Material
The Supplementary Material for this article can be found online at: https://www.frontiersin.org/articles/10.3389/fendo.2022.938633/full#supplementary-material
References
1. Bjoro T, Holmen J, Kruger O, Midthjell K, Hunstad K, Schreiner T, et al. Prevalence of Thyroid Disease, Thyroid Dysfunction and Thyroid Peroxidase Antibodies in a Large, Unselected Population. The Health Study of Nord-Trondelag (Hunt). Eur J Endocrinol (2000) 143(5):639–47.
2. Hwangbo Y, Park YJ. Genome-Wide Association Studies of Autoimmune Thyroid Diseases, Thyroid Function, and Thyroid Cancer. Endocrinol Metab (2018) 33(2):175–84.
3. Simmonds MJ. Gwas in Autoimmune Thyroid Disease: Redefining Our Understanding of Pathogenesis. Nat Rev Endocrinol (2013) 9(5):277–87. doi: 10.1038/nrendo.2013.56
4. Lee HJ, Li CW, Hammerstad SS, Stefan M, Tomer Y. Immunogenetics of Autoimmune Thyroid Diseases: A Comprehensive Review. J Autoimmun (2015) 64:82–90. doi: 10.1016/j.jaut.2015.07.009
5. Chu X, Pan C-M, Zhao S-X, Liang J, Gao G-Q, Zhang X-M, et al. A Genome-Wide Association Study Identifies Two New Risk Loci for Graves' Disease. Nat Genet (2011) 43(9):897–901. doi: 10.1038/ng.898
6. Davies TF, Andersen S, Latif R, Nagayama Y, Barbesino G, Brito M, et al. Graves’ Disease. Nat Rev Dis Primers (2020) 6(1):52. doi: 10.1038/s41572-020-0184-y
7. Wang L, Zhang WP, Yao L, Zhang W, Zhu J, Zhang WC, et al. Prdm1 Expression Via Human Parvovirus B19 Infection Plays a Role in the Pathogenesis of Hashimoto Thyroiditis. Hum Pathol (2015) 46(12):1913–21. doi: 10.1016/j.humpath.2015.08.009
8. Caselli E, D'Accolti M, Soffritti I, Zatelli MC, Rossi R, Degli Uberti E, et al. Hhv-6a in Vitro Infection of Thyrocytes and T Cells Alters the Expression of Mirna Associated to Autoimmune Thyroiditis. Virol J (2017) 14(1):3. doi: 10.1186/s12985-016-0672-6
9. Weider T, Richardson SJ, Morgan NG, Paulsen TH, Dahl-Jorgensen K, Hammerstad SS. Upregulation of Hla Class I and Antiviral Tissue Responses in Hashimoto's Thyroiditis. Thyroid (2020) 30(3):432–42. doi: 10.1089/thy.2019.0607
10. Weider T, Richardson SJ, Morgan NG, Paulsen TH, Dahl-Jorgensen K, Hammerstad SS. Hla Class I Upregulation and Antiviral Immune Responses in Graves Disease. J Clin Endocrinol Metab (2021) 106(4):e1763–e74. doi: 10.1210/clinem/dgaa958
11. Hammerstad SS, Tauriainen S, Hyoty H, Paulsen T, Norheim I, Dahl-Jorgensen K. Detection of Enterovirus in the Thyroid Tissue of Patients With Graves' Disease. J Med Virol (2013) 85(3):512–8. doi: 10.1002/jmv.23476
12. Ignatovich IV, Hobbs JA. Human Parvovirus B19 Infection Leads to Downregulation of Thyroid, Estrogen, and Retinoid Hormone Receptor Expression. Virology (2013) 446(1-2):173–9. doi: 10.1016/j.virol.2013.06.022
13. Page C, Duverlie G, Sevestre H, Desailloud R. Erythrovirus B19 and Autoimmune Thyroid Diseases. Review of the Literature and Pathophysiological Hypotheses. J Med Virol (2015) 87(1):162–9. doi: 10.1002/jmv.23963
14. Morohoshi K, Takahashi Y, Mori K. Viral Infection and Innate Pattern Recognition Receptors in Induction of Hashimoto's Thyroiditis. Discovery Med (2011) 12(67):505–11.
15. Hammerstad SS, Blackard JT, Lombardi A, Owen RP, Concepcion E, Yi Z, et al. Hepatitis C Virus Infection of Human Thyrocytes: Metabolic, Hormonal, and Immunological Implications. J Clin Endocrinol Metab (2019) 105(4):1157–68. doi: 10.1210/clinem/dgz241 %J The Journal of Clinical Endocrinology & Metabolism
16. Hammerstad SS, Stefan M, Blackard J, Owen RP, Lee HJ, Concepcion E, et al. Hepatitis C Virus E2 Protein Induces Upregulation of Il-8 Pathways and Production of Heat Shock Proteins in Human Thyroid Cells. J Clin Endocrinol Metab (2017) 102(2):689–97. doi: 10.1210/jc.2016-3403
17. Kumata K, Nagata K, Matsushita M, Kuwamoto S, Kato M, Murakami I, et al. Thyrotropin Receptor Antibody (Trab)-Igm Levels Are Markedly Higher Than Trab-Igg Levels in Graves' Disease Patients and Controls, and Trab-Igm Production Is Related to Epstein-Barr Virus Reactivation. Viral Immunol (2016) 29(8):459–63. doi: 10.1089/vim.2016.0043
18. Nagata K, Hara S, Nakayama Y, Higaki K, Sugihara H, Kuwamoto S, et al. Epstein-Barr Virus Lytic Reactivation Induces Igg4 Production by Host B Lymphocytes in Graves' Disease Patients and Controls: A Subset of Graves' Disease Is an Igg4-Related Disease-Like Condition. Viral Immunol (2018) 31(8):540–7. doi: 10.1089/vim.2018.0042
19. Pyzik A, Grywalska E, Matyjaszek-Matuszek B, Ludian J, Kiszczak-Bochyńska E, Smoleń A, et al. Does the Epstein-Barr Virus Play a Role in the Pathogenesis of Graves' Disease? Int J Mol Sci (2019) 20(13). doi: 10.3390/ijms20133145
20. Sultanova A, Cistjakovs M, Gravelsina S, Chapenko S, Roga S, Cunskis E, et al. Association of Active Human Herpesvirus-6 (Hhv-6) Infection With Autoimmune Thyroid Gland Diseases. Clin Microbiol Infect (2017) 23(1):50.e1–.e5. doi: 10.1016/j.cmi.2016.09.023
21. Caselli E, Zatelli MC, Rizzo R, Benedetti S, Martorelli D, Trasforini G, et al. Virologic and Immunologic Evidence Supporting an Association Between Hhv-6 and Hashimoto's Thyroiditis. PloS Pathog (2012) 8(10):e1002951. doi: 10.1371/journal.ppat.1002951
22. Rizzo R, Zatelli MC, Rotola A, Cassai E, Degli Uberti E, Di Luca D, et al. Increase in Peripheral Cd3-Cd56brightcd16- Natural Killer Cells in Hashimoto's Thyroiditis Associated With Hhv-6 Infection. Adv Exp Med Biol (2016) 897:113–20. doi: 10.1007/5584_2015_5010
23. Seyyedi N, Dehbidi GR, Karimi M, Asgari A, Esmaeili B, Zare F, et al. Human Herpesvirus 6a Active Infection in Patients With Autoimmune Hashimoto's Thyroiditis. Braz J Infect Dis (2019) 23(6):435–40. doi: 10.1016/j.bjid.2019.10.004
24. Thomas D, Liakos V, Michou V, Kapranos N, Kaltsas G, Tsilivakos V, et al. Detection of Herpes Virus DNA in Post-Operative Thyroid Tissue Specimens of Patients With Autoimmune Thyroid Disease. Exp Clin Endocrinol Diabetes (2008) 116(01):35–9. doi: 10.1055/s-2007-956171
25. Xue YC, Feuer R, Cashman N, Luo H. Enteroviral Infection: The Forgotten Link to Amyotrophic Lateral Sclerosis? Front Mol Neurosci (2018) 11:63. doi: 10.3389/fnmol.2018.00063
26. Page K, Melia MT, Veenhuis RT, Winter M, Rousseau KE, Massaccesi G, et al. Randomized Trial of a Vaccine Regimen to Prevent Chronic Hcv Infection. N Engl J Med (2021) 384(6):541–9. doi: 10.1056/NEJMoa2023345
27. Young LS. Epstein-Barr-Virus Infection and Persistence: A B-Cell Marriage in Sickness and in Health. Lancet (1999) 354(9185):1141–2. doi: 10.1016/s0140-6736(99)00237-8
28. Whyte ML, Smith KA, Buchberger A, Berg Luecke L, Tjan LH, Mori Y, et al. The Roseoloviruses Downregulate the Protein Tyrosine Phosphatase Ptprc (Cd45). J Virol (2021) 95(14):e0162820. doi: 10.1128/jvi.01628-20
29. Norja P, Eis-Hübinger AM, Söderlund-Venermo M, Hedman K, Simmonds P. Rapid Sequence Change and Geographical Spread of Human Parvovirus B19: Comparison of B19 Virus Evolution in Acute and Persistent Infections. J Virol (2008) 82(13):6427–33. doi: 10.1128/jvi.00471-08
30. Ziring PR, Gallo G, Finegold M, Buimovici-Klein E, Ogra P. Chronic Lymphocytic Thyroiditis: Identification of Rubella Virus Antigen in the Thyroid of a Child With Congenital Rubella. J Pediatr (1977) 90(3):419–20. doi: 10.1016/s0022-3476(77)80705-1
31. Kubonishi I, Kubota T, Sawada T, Tanaka Y, Machida H, Yoshida O, et al. An Htlv-I Carrier With Graves' Disease Followed by Uveitis: Isolation of Htlv-I From Thyroid Tissue. Int J Hematol (1997) 66(2):233–7. doi: 10.1016/s0925-5710(97)00595-1
32. Jin HY, Kang SM, Kim SY, Park JH, Baek HS, Park TS. A Case of Graves' Disease Combined With Hantaan Virus Infection. J Korean Med Sci (2009) 24(1):158–61. doi: 10.3346/jkms.2009.24.1.158
33. Dumoulin FL, Liese H. Acute Hepatitis E Virus Infection and Autoimmune Thyroiditis: Yet Another Trigger? BMJ Case Rep (2012) 2012. doi: 10.1136/bcr.12.2011.5441
34. Visser R, de Mast Q, Netea-Maier RT, van der Ven AJ. Hashimoto's Thyroiditis Presenting as Acute Painful Thyroiditis and as a Manifestation of an Immune Reconstitution Inflammatory Syndrome in a Human Immunodeficiency Virus-Seropositive Patient. Thyroid (2012) 22(8):853–5. doi: 10.1089/thy.2012.0055
35. Knack RS, Hanada T, Knack RS, Mayr K. Hashimoto's Thyroiditis Following Sars-Cov-2 Infection. BMJ Case Rep (2021) 14(8):e244909. doi: 10.1136/bcr-2021-244909
36. Poma AM, Basolo A, Bonuccelli D, Proietti A, Macerola E, Ugolini C, et al. Activation of Type I and Type Ii Interferon Signaling in Sars-Cov-2-Positive Thyroid Tissue of Patients Dying From Covid-19. Thyroid (2021) 31(12):1766–75. doi: 10.1089/thy.2021.0345
37. Mateu-Salat M, Urgell E, Chico A. Sars-Cov-2 as a Trigger for Autoimmune Disease: Report of Two Cases of Graves' Disease After Covid-19. J Endocrinol Invest (2020) 43(10):1527–8. doi: 10.1007/s40618-020-01366-7
38. Tee LY, Harjanto S, Rosario BH. Covid-19 Complicated by Hashimoto's Thyroiditis. Singapore Med J (2021) 62(5):265. doi: 10.11622/smedj.2020106
39. Anaya J-M, Monsalve DM, Rojas M, Rodríguez Y, Montoya-García N, Mancera-Navarro LM, et al. Latent Rheumatic, Thyroid and Phospholipid Autoimmunity in Hospitalized Patients With Covid-19. J Trans Autoimmun (2021) 4:100091.
40. Kahrs CR, Chuda K, Tapia G, Stene LC, Mårild K, Rasmussen T, et al. Enterovirus as Trigger of Coeliac Disease: Nested Case-Control Study Within Prospective Birth Cohort. BMJ (2019) 364:l231. doi: 10.1136/bmj.l231
41. Dian Z, Sun Y, Zhang G, Xu Y, Fan X, Yang X, et al. Rotavirus-Related Systemic Diseases: Clinical Manifestation, Evidence and Pathogenesis. Crit Rev Microbiol (2021) 47(5):580–95. doi: 10.1080/1040841x.2021.1907738
42. Wang D. 5 Challenges in Understanding the Role of the Virome in Health and Disease. PloS Pathog (2020) 16(3):e1008318. doi: 10.1371/journal.ppat.1008318
43. Genoni A, Canducci F, Rossi A, Broccolo F, Chumakov K, Bono G, et al. Revealing Enterovirus Infection in Chronic Human Disorders: An Integrated Diagnostic Approach. Sci Rep (2017) 7(1):5013. doi: 10.1038/s41598-017-04993-y
44. Hammerstad SS, Jahnsen FL, Tauriainen S, Hyoty H, Paulsen T, Norheim I, et al. Inflammation and Increased Myxovirus Resistance Protein a Expression in Thyroid Tissue in the Early Stages of Hashimoto's Thyroiditis. Thyroid (2013) 23(3):334–41. doi: 10.1089/thy.2012.0264
45. Wells AI, Coyne CB. Enteroviruses: A Gut-Wrenching Game of Entry, Detection, and Evasion. Viruses (2019) 11(5):460. doi: 10.3390/v11050460
46. Baggen J, Thibaut HJ, Strating J, van Kuppeveld FJM. The Life Cycle of Non-Polio Enteroviruses and How to Target It. Nat Rev Microbiol (2018) 16(6):368–81. doi: 10.1038/s41579-018-0005-4
47. Nishimura M, Novita BD, Kato T, Handayani Tjan L, Wang B, Wakata A, et al. Structural Basis for the Interaction of Human Herpesvirus 6b Tetrameric Glycoprotein Complex With the Cellular Receptor, Human Cd134. PloS Pathog (2020) 16(7):e1008648. doi: 10.1371/journal.ppat.1008648
48. Ma J, Jia J, Jiang X, Xu M, Guo J, Tang T, et al. Gp96 Is Critical for Both Human Herpesvirus 6a (Hhv-6a) and Hhv-6b Infections. J Virol (2020) 94(13):e00311-20. doi: 10.1128/jvi.00311-20
49. Poma AM, Hammerstad SS, Genoni A, Basolo A, Dahl-Jorgensen K, Toniolo A. Immune Transcriptome of Cells Infected With Enterovirus Strains Obtained From Cases of Autoimmune Thyroid Disease. Microorganisms (2021) 9(4):876. doi: 10.3390/microorganisms9040876
50. Maccari G, Genoni A, Sansonno S, Toniolo A. Properties of Two Enterovirus Antibodies That Are Utilized in Diabetes Research. Sci Rep (2016) 6:24757. doi: 10.1038/srep24757
51. Cnc Garcia Rd, Leon LAA. Human Parvovirus B19: A Review of Clinical and Epidemiological Aspects in Brazil. Future Microbiol (2021) 16(1):37–50. doi: 10.2217/fmb-2020-0123
52. Nikonov OS, Chernykh ES, Garber MB, Nikonova EY. Enteroviruses: Classification, Diseases They Cause, and Approaches to Development of Antiviral Drugs. Biochem Biokhimiia (2017) 82(13):1615–31. doi: 10.1134/S0006297917130041
53. Esneau C, Duff AC, Bartlett NW. Understanding Rhinovirus Circulation and Impact on Illness. Viruses (2022) 14(1). doi: 10.3390/v14010141
54. Richardson SJ, Morgan NG. Enteroviral Infections in the Pathogenesis of Type 1 Diabetes: New Insights for Therapeutic Intervention. Curr Opin Pharmacol (2018) 43:11–9. doi: 10.1016/j.coph.2018.07.006
55. Fischer TK, Simmonds P, Harvala H. The Importance of Enterovirus Surveillance in a Post-Polio World. Lancet Infect Dis (2021) 22(1):e35-e40. doi: 10.1016/s1473-3099(20)30852-5
56. Bochkov YA, Gern JE. Clinical and Molecular Features of Human Rhinovirus C. Microbes Infect (2012) 14(6):485–94. doi: 10.1016/j.micinf.2011.12.011
57. Simmonds P, Gorbalenya AE, Harvala H, Hovi T, Knowles NJ, Lindberg AM, et al. Recommendations for the Nomenclature of Enteroviruses and Rhinoviruses. Arch Virol (2020) 165(3):793–7. doi: 10.1007/s00705-019-04520-6
58. Cox DW, Khoo SK, Zhang G, Lindsay K, Keil AD, Knight G, et al. Rhinovirus Is the Most Common Virus and Rhinovirus-C Is the Most Common Species in Paediatric Intensive Care Respiratory Admissions. Eur Respir J (2018) 52(2):1800207. doi: 10.1183/13993003.00207-2018
59. Krogvold L, Edwin B, Buanes T, Frisk G, Skog O, Anagandula M, et al. Detection of a Low-Grade Enteroviral Infection in the Islets of Langerhans of Living Patients Newly Diagnosed With Type 1 Diabetes. Diabetes (2015) 64(5):1682–7. doi: 10.2337/db14-1370
60. Ylipaasto P, Klingel K, Lindberg AM, Otonkoski T, Kandolf R, Hovi T, et al. Enterovirus Infection in Human Pancreatic Islet Cells, Islet Tropism in Vivo and Receptor Involvement in Cultured Islet Beta Cells. Diabetologia (2004) 47(2):225–39. doi: 10.1007/s00125-003-1297-z
61. Busse N, Paroni F, Richardson SJ, Laiho JE, Oikarinen M, Frisk G, et al. Detection and Localization of Viral Infection in the Pancreas of Patients With Type 1 Diabetes Using Short Fluorescently-Labelled Oligonucleotide Probes. Oncotarget (2017) 8(8):12620–36. doi: 10.18632/oncotarget.14896
62. Dotta F, Censini S, van Halteren AG, Marselli L, Masini M, Dionisi S, et al. Coxsackie B4 Virus Infection of Beta Cells and Natural Killer Cell Insulitis in Recent-Onset Type 1 Diabetic Patients. Proc Natl Acad Sci U.S.A. (2007) 104(12):5115–20. doi: 10.1073/pnas.0700442104
63. Lundberg M, Krogvold L, Kuric E, Dahl-Jorgensen K, Skog O. Expression of Interferon-Stimulated Genes in Insulitic Pancreatic Islets of Patients Recently Diagnosed With Type 1 Diabetes. Diabetes (2016) 65(10):3104–10. doi: 10.2337/db16-0616
64. Richardson SJ, Leete P, Bone AJ, Foulis AK, Morgan NG. Expression of the Enteroviral Capsid Protein Vp1 in the Islet Cells of Patients With Type 1 Diabetes Is Associated With Induction of Protein Kinase R and Downregulation of Mcl-1. Diabetologia (2013) 56(1):185–93. doi: 10.1007/s00125-012-2745-4
65. Poma AM, Genoni A, Broccolo F, Denaro M, Pugliese A, Basolo F, et al. Immune Transcriptome of Cells Infected With Enterovirus Strains Obtained From Cases of Type 1 Diabetes. Microorganisms (2020) 8(7):1031. doi: 10.3390/microorganisms8071031
66. Komaroff AL, Pellett PE, Jacobson S. Human Herpesviruses 6a and 6b in Brain Diseases: Association Versus Causation. Clin Microbiol Rev (2020) 34(1):e00143-20. doi: 10.1128/cmr.00143-20
67. Agut H, Bonnafous P, Gautheret-Dejean A. Human Herpesviruses 6a, 6b, and 7. Microbiol Spectr (2016) 4(3). doi: 10.1128/microbiolspec.DMIH2-0007-2015
68. Flamand L, Menezes J. Cyclic Amp-Responsive Element-Dependent Activation of Epstein-Barr Virus Zebra Promoter by Human Herpesvirus 6. J Virol (1996) 70(3):1784–91. doi: 10.1128/jvi.70.3.1784-1791.1996
69. Meier UC, Cipian RC, Karimi A, Ramasamy R, Middeldorp JM. Cumulative Roles for Epstein-Barr Virus, Human Endogenous Retroviruses, and Human Herpes Virus-6 in Driving an Inflammatory Cascade Underlying Ms Pathogenesis. Front Immunol (2021) 12:757302. doi: 10.3389/fimmu.2021.757302
70. Bigley TM, Yang L, Kang L-I, Saenz JB, Victorino F, Yokoyama WM. Disruption of Thymic Central Tolerance by Infection With Murine Roseolovirus Induces Autoimmune Gastritis. J Exp Med (2022) 219(3):e20211403. doi: 10.1084/jem.20211403
71. Blackard JT, Kong L, Huber AK, Tomer Y. Hepatitis C Virus Infection of a Thyroid Cell Line: Implications for Pathogenesis of Hepatitis C Virus and Thyroiditis. Thyroid (2012) 23(7):863–70. doi: 10.1089/thy.2012.0507
72. Rotondi M, Coperchini F, Ricci G, Denegri M, Croce L, Ngnitejeu ST, et al. Detection of Sars-Cov-2 Receptor Ace-2 Mrna in Thyroid Cells: A Clue for Covid-19-Related Subacute Thyroiditis. J Endocrinol Invest (2021) 44(5):1085–90. doi: 10.1007/s40618-020-01436-w
74. Politou M, Koutras D, Kaparos G, Valsami S, Pittaras T, Logothetis E, et al. Seroprevalence of Hhv-6 and Hhv-8 Among Blood Donors in Greece. Virol J (2014) 11(1):153. doi: 10.1186/1743-422X-11-153
75. Qiu J, Söderlund-Venermo M, Young NS. Human Parvoviruses. Clinical Microbiology Reviews (2017) 30(1):43–113. doi: 10.1128/CMR.00040-16
76. Desailloud R, Hober D. Viruses and Thyroiditis: An Update. Virol J (2009) 6:5. doi: 10.1186/1743-422x-6-5
77. Wartofsky L, Schaaf M. Graves' Disease With Thyrotoxicosis Following Subacute Thyroiditis. Am J Med (1987) 83(4):761–4. doi: 10.1016/0002-9343(87)90910-7
78. Strakosch CR, Joyner D, Wall JR. Thyroid Stimulating Antibodies in Patients With Subacute Thyroiditis. J Clin Endocrinol Metab (1978) 46(2):345–8. doi: 10.1210/jcem-46-2-345
79. Weetman AP, Smallridge RC, Nutman TB, Burman KD. Persistent Thyroid Autoimmunity After Subacute Thyroiditis. J Clin Lab Immunol (1987) 23(1):1–6.
80. Volpé R, Row VV, Ezrin C. Circulating Viral and Thyroid Antibodies in Subacute Thyroiditis. J Clin Endocrinol Metab (1967) 27(9):1275–84. doi: 10.1210/jcem-27-9-1275
Keywords: autoimmune thyroid disease, Graves’ disease, Hashimoto’s thyroiditis, viral infection, enteroviruses, human herpesvirus 6, parvovirus B19
Citation: Weider T, Genoni A, Broccolo F, Paulsen TH, Dahl-Jørgensen K, Toniolo A and Hammerstad SS (2022) High Prevalence of Common Human Viruses in Thyroid Tissue. Front. Endocrinol. 13:938633. doi: 10.3389/fendo.2022.938633
Received: 07 May 2022; Accepted: 22 June 2022;
Published: 14 July 2022.
Edited by:
Alessandro Antonelli, University of Pisa, ItalyCopyright © 2022 Weider, Genoni, Broccolo, Paulsen, Dahl-Jørgensen, Toniolo and Hammerstad. This is an open-access article distributed under the terms of the Creative Commons Attribution License (CC BY). The use, distribution or reproduction in other forums is permitted, provided the original author(s) and the copyright owner(s) are credited and that the original publication in this journal is cited, in accordance with accepted academic practice. No use, distribution or reproduction is permitted which does not comply with these terms.
*Correspondence: Therese Weider, dGhlcmVzZXdlaWRlckBnbWFpbC5jb20=
†These authors share first authorship
‡These authors share senior authorship