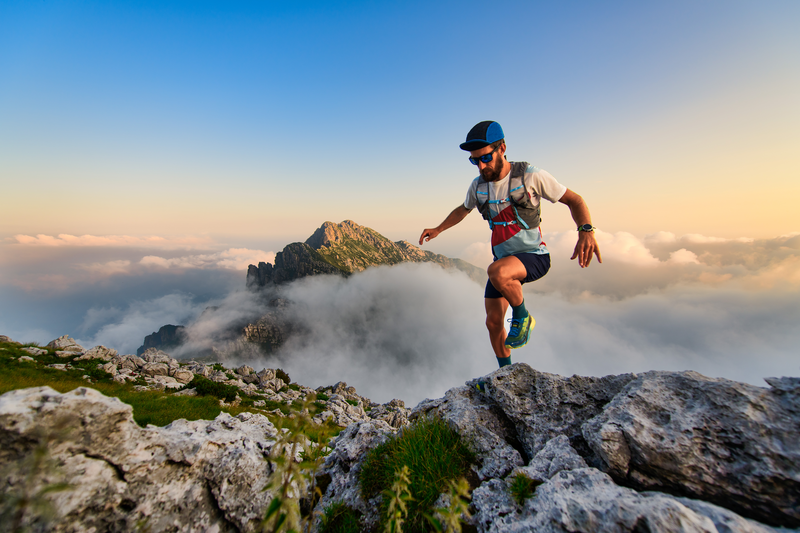
95% of researchers rate our articles as excellent or good
Learn more about the work of our research integrity team to safeguard the quality of each article we publish.
Find out more
PERSPECTIVE article
Front. Endocrinol. , 13 September 2022
Sec. Pituitary Endocrinology
Volume 13 - 2022 | https://doi.org/10.3389/fendo.2022.933039
This article is part of the Research Topic Personalized Management of Acromegaly View all 7 articles
Growth hormone (GH) and insulin-like growth factor 1 (IGF-1) are essential to normal growth, metabolism, and body composition, but in acromegaly, excesses of these hormones strikingly alter them. In recent years, the use of modern methodologies to assess body composition in patients with acromegaly has revealed novel aspects of the acromegaly phenotype. In particular, acromegaly presents a unique pattern of body composition changes in the setting of insulin resistance that we propose herein to be considered an acromegaly-specific lipodystrophy. The lipodystrophy, initiated by a distinctive GH-driven adipose tissue dysregulation, features insulin resistance in the setting of reduced visceral adipose tissue (VAT) mass and intra-hepatic lipid (IHL) but with lipid redistribution, resulting in ectopic lipid deposition in muscle. With recovery of the lipodystrophy, adipose tissue mass, especially that of VAT and IHL, rises, but insulin resistance is lessened. Abnormalities of adipose tissue adipokines may play a role in the disordered adipose tissue metabolism and insulin resistance of the lipodystrophy. The orexigenic hormone ghrelin and peptide Agouti-related peptide may also be affected by active acromegaly as well as variably by acromegaly therapies, which may contribute to the lipodystrophy. Understanding the pathophysiology of the lipodystrophy and how acromegaly therapies differentially reverse its features may be important to optimizing the long-term outcome for patients with this disease. This perspective describes evidence in support of this acromegaly lipodystrophy model and its relevance to acromegaly pathophysiology and the treatment of patients with acromegaly.
GH and IGF-1 are vital to normal growth, metabolism, and body composition (1). In acromegaly, however, excesses of GH and IGF-1 markedly alter these processes. Changes in body composition and metabolic abnormalities are prominent features of the acromegaly phenotype and reflect, predominantly, direct actions of GH on peripheral tissues (2, 3). In particular, disordered glucose metabolism and insulin resistance (IR) are common at acromegaly diagnosis and often persist despite effective acromegaly treatment and reduce survival (4–11). In the general population, body fat quantity and distribution are important determinants of metabolic and cardiovascular risk (12–16). For example, increased visceral adipose tissue (VAT) mass associates with IR and type 2 diabetes (13, 17–19). However, this paradigm linking body composition pattern to metabolic abnormalities in the general population does not apply to acromegaly. Rather, acromegaly presents a unique constellation of these features that we propose to be considered an acromegaly-specific lipodystrophy (Figure 1). The lipodystrophy, initiated by a distinctive GH-driven AT dysregulation, features IR in the setting of reduced VAT and intra-hepatic lipid (IHL) but with lipid redistribution, resulting in ectopic lipid deposition in muscle (20, 21). How acromegaly therapies may differentially reverse the lipodystrophy’s features should be considered. This perspective describes evidence in support of this acromegaly lipodystrophy model and its relevance to acromegaly pathophysiology and treatment.
Figure 1 (A) Model of the acromegaly lipodystrophy that is present when the disease is active (i.e., elevated levels of GH and IGF-1). The lipodystrophy is initiated by a GH-induced accelerated lipolysis leading to insulin resistance, adipose tissue inflammation, and reduced adipose tissue mass, especially that of the VAT depot. Hepatic lipid is reduced, but hepatic insulin resistance occurs. Lipid is redistributed from VAT and SAT depots to ectopic deposition in muscle and may contribute to muscle insulin resistance. (B) Model of recovery of the lipodystrophy with biochemical remission after acromegaly treatment. After normalization of GH by surgery or medical therapy, adipose tissue lipolysis is reduced, permitting a re-accumulation of VAT and SAT lipid stores, a rise in intra-hepatic lipid and reduction in insulin resistance. Muscle lipid may not decrease due to the rise in total adipose tissue mass with acromegaly therapy despite improvement in insulin resistance.
The acromegaly lipodystrophy originates in AT under the influence of GH excess. GH modulates AT metabolism and is lipolytic (1, 22–26). Mechanisms for this predominate in VAT (19, 27–31). GH increases HSL, reduces LPL activity, activates the β-adrenergic 3 receptor and other lipolytic pathways, and inhibits lipogenesis (29, 32–44). GH may also impair adipocyte differentiation and adipogenesis (45–47). These effects act in concert to reduce AT mass in acromegaly (20, 48–50). Initial studies utilized a four-compartment body composition model that could not define specific depot changes (49, 51, 52), and although DXA finds reduced trunk fat (53, 54) and suggests VAT changes (55), techniques for VAT estimation by dual-energy X-ray absorptiometry (DXA) require validation in acromegaly. When directly quantified by whole-body MRI, both VAT and subcutaneous adipose tissue (SAT) mass were lower than predicted in active acromegaly (20). With surgical treatment, specific AT depot changes include increases in VAT and SAT, as quantified by CT (50) or whole-body MRI (20, 21) (Table 1). Pegvisomant therapy also increases intra-abdominal fat with a short-term treatment (56) and VAT and SAT with a long-term treatment (57) (Table 1). Limited data suggest increases in fat mass and decreases in lean mass (by DXA) with short-acting octreotide or Somatostatin receptor ligands (SRL) therapy (58, 59). Body composition changes in acromegaly relate to disease activity, including IGF-1 levels (54, 59) and rise in VAT mass after surgery correlates with the decrease in GH (21). Interestingly, AT mass changes, especially those of VAT, are greater in men than women with acromegaly (20, 53, 60). Mechanisms for this are incompletely understood, but known gender differences in body composition (61) and greater sensitivity of VAT to GH in men (62) may contribute to these differences. VAT may rise to above expected in men (21), but in one study, VAT lowering followed an early post-operative rise (60) and another suggested that gender differences attenuate in the long-term (59). Further investigation into the time course, patterns, and long-term outcome of AT mass changes after acromegaly therapy in men vs. women is warranted. Given the reduced AT mass in acromegaly, dysfunctional AT appears to be a greater determinant than absolute AT mass to the metabolic abnormalities. The contributions of the former and its changes with acromegaly treatment are incompletely understood yet highly relevant to optimizing therapy.
Table 1 Body composition changes in patients with acromegaly treated with surgery (top) or pegvisomant therapy (bottom).
GH has important effects on protein metabolism that favor anabolism in muscle (63, 64), and some evidence for this in acromegaly exists (65, 66). Recent advances in body composition testing methodologies have also enabled the assessment of skeletal muscle (SM) mass in acromegaly. In patients with active acromegaly, SM mass did not differ from predicted (by whole-body MRI) (21, 67) but was higher [by Bioelectrical Impedance Analysis (BIA)] in pre-operative patients (60). Whereas earlier studies reported no change in body cell mass with acromegaly treatment (68), subsequent studies found decreases in SM volume (by CT) (50) and in SM mass by BIA (60) and by MRI (in men) (20) after surgery (Table 1). SM mass did not change with the long-term pegvisomant therapy (57) (Table 1). In cross-sectional studies, lean tissue mass (by DXA) was increased in active acromegaly compared with that in remission acromegaly (53, 54, 69). Importantly, however, DXA lean tissue estimates are not a surrogate for SM in acromegaly, as they include measures of soft tissues (51, 70) that are influenced by the increased tissue hydration of acromegaly (48, 49). In fact, changes in DXA lean tissue with acromegaly treatment are accounted for by those of the non-SM components that make up lean tissue (57, 67). Genders differ in the interaction of acromegaly disease activity with lean or SM changes (54), which are greater in men (53). SM mass is likely influenced by gonadal steroid changes in men with acromegaly (66, 71). Overall, reductions in SM mass with acromegaly therapy are small, and reports on the effect of acromegaly and its treatment on SM function vary (72–75), but the impact on SM metabolism and other outcomes requires further study.
MRI and proton magnetic resonance spectroscopy (1HMRS) imaging use has also enabled visualization of muscle lipid content in acromegaly. MRI revealed that inter-muscular AT (IMAT), AT located between muscle groups and beneath the fascia (76–78), is increased in active acromegaly. This is an important feature of the lipodystrophy (20). GH-induced AT lipolysis may lead to lipid movement from VAT and SAT depots to muscle where it is deposited ectopically. Free fatty acid (FFA) flux and uptake in muscle are increased in acromegaly and with GH use (32, 79–82), and supraphysiologic GH increased intra-myocellular lipid (IMCL) on SM biopsy (83). In other settings, FFA rise is associated with an increase in IMCL (84, 85) and IMAT (86–89) and with IR (90, 91). Anti-lipolysis along with GH administration reduces its effect on muscle IR (32, 92), supporting a role for muscle lipid in IR in acromegaly. IMAT may relate to IR in acromegaly (20), but IMAT was lowered only in women after surgery and not lowered with pegvisomant therapy despite improved IR (21, 57). Increases in total AT, a major determinant of IMAT, may obscure IMAT changes with acromegaly therapy. IMCL did not change with surgery (21, 93) or in pegvisomant-treated acromegaly patients vs. controls (94) but did decrease with the addition of pegvisomant to SRL therapy (95) and correlated inversely with insulin sensitivity in a combined acromegaly and control cohort (94). Further study is needed to understand the effects of acromegaly and its therapy on muscle lipid and its relationship to IR in acromegaly.
Another key component of the acromegaly lipodystrophy revealed by 1HMRS is that IHL is low in active acromegaly in the setting of IR (21, 93). This appears to contrast with the positive correlation between IHL and IR in other populations (96). Other data support an influence of GH on liver fat: mice with impaired GH signaling or GH receptor deletion have increased liver fat and steatosis (97–99), and, in case reports, liver fat was increased in patients with growth hormone deficiency (GHD) (100–102). In small cross-sectional studies, IHL was up to three-fold higher in treated acromegaly vs. controls (94, 103). IHL rises with surgical therapy (21, 93, 104), when pegvisomant is added to SRL therapy (95) and with long-term pegvisomant monotherapy (57) (Table 1). On pegvisomant, IHL was similar to controls, suggesting that it returns to the expected levels on therapy (57). It seems that hepatic lipid accumulation cannot be implicated in hepatic IR in acromegaly (96), but whether other characteristics of IHL can, for example, altered proportions of certain lipid species (105) requires further study.
Amount and distribution of AT are important determinants of IR in acromegaly (54, 106). In other lipodystrophies, inability to store lipid in SAT is thought to promote ectopic lipid deposition and IR, and, conversely, lipid storage in SAT may be protective for ectopic lipid deposition, IR and diabetes (12, 14, 107, 108). Potentially, in the acromegaly lipodystrophy, inability to store lipid in SAT contributes to IR, and SAT re-expansion with its recovery is important to its resolution. We found that lowering of homeostatic model assessment (HOMA) score correlated with SAT and VAT increases after surgical treatment (21), but others found less rise in trunk fat after surgery to be associated with greater improvement in HOMA score (55). These relationships require further study.
IR, a central feature of acromegaly’s metabolic abnormalities, is thought to be due primarily to GH’s insulin-antagonistic and lipolytic effects (63, 79, 109–112). IGF-1’s insulin agonism may partially offset those of GH, but circulating IGF-1 has a little role in regulating glucose homeostasis in acromegaly (10). Both hepatic and peripheral IR occur in acromegaly (79, 109, 110, 112, 113). Mechanisms for this are incompletely understood but may include impaired insulin signaling and substrate competition (10, 114–117). In other settings, IR relates strongly to increased FFAs from lipolysis by a number of proposed mechanisms (41, 63, 90, 116–134). GH increases FFA flux into muscle, which may increase muscle lipid and IR as described above (32, 79–81, 83–85, 92). Interestingly, although GH administration acutely increases FFA levels (43) and leads to increased muscle IR (135, 136), circulating FFA levels are not consistently elevated in acromegaly (110, 137, 138) but acromegaly treatment lowers them along with IR (139).
The importance of IR in AT has recently been emphasized (106, 140) and is a central feature of the acromegaly lipodystrophy. Dysfunctional AT is a major contributor to systemic IR in other populations (141). GH has complex effects that impair insulin action in AT and decrease its uptake and utilization of glucose (44, 114, 142–144). In acromegaly, accelerated lipolysis is the likely inciting precipitant of AT IR (140). In other models, disordered lipid metabolism in AT, particularly lipolysis and FFA release, may signal to induce inflammation in AT, which, in turn, promotes IR (145–154). In mice, GH excess is associated with immune and inflammatory changes in AT (155). In vitro, GH induced pro-inflammatory cytokines in pre-adipocytes yet suppressed them in AT macrophages (ATMs) (156). Because ATMs buffer lipid increases (145, 146, 148, 149) and the lack of functional GHRs in macrophages is associated with inflammatory ATM migration and IR in AT (157), acromegaly’s effects on immune components of AT could contribute to AT IR.
GH-induced changes in adipokines may also contribute to the development of IR in acromegaly. GH reduces leptin gene expression in VAT (158), and in acromegaly, circulating leptin levels are low (159–162) and rise with surgical (159) or pegvisomant (57, 163) therapy. Whether leptin changes are explained by or independent of those in fat mass is unclear (57, 164–167). Leptin deficiency contributes to IR and abnormal metabolism in other settings (168–170): leptin therapy corrects hyperglycemia in diabetic mice (171, 172) and lipodystrophy patients (170, 173, 174). However, changes in leptin and IR with acromegaly treatment do not consistently correlate (21), and whether low leptin contributes to IR in acromegaly and its rise to IR improvement is unknown. Circulating levels of visfatin (175) were increased in acromegaly in some (106, 160, 176) but not other studies (177) and correlated with those of IGF-1 (176), variably with IR (176, 178) and inversely with percentage body fat (160). GH increases visfatin expression in mature human adipocytes, supporting a pathogenic role in AT inflammation in acromegaly (160). Data conflict with regard to adiponectin levels in acromegaly, these were reduced in some (106, 177, 179) but not other (160, 161) studies. Some in vitro data suggest that GH may reduce adiponectin gene transcription (180). GH modulates 11B-HSD1 in AT and in acromegaly (34, 181–183), which could also play a role the lipodystrophy and its recovery.
The effect of acromegaly on ghrelin is also relevant to the lipodystrophy. Ghrelin is an orexigenic hormone that is importantly linked to appetite and body composition (184–186) and to the GH–IGF-1 axis as a stimulator of pituitary GH secretion (187). Evidence supports that GH excess suppresses ghrelin: Ghrelin levels are lowered in active acromegaly (159, 188, 189) and rise with surgical treatment (159, 188). Most rodent data (190–192) and the finding that high-dose GH suppresses ghrelin (193) are consistent with this. In acromegaly, ghrelin levels correlated inversely with insulin levels and HOMA score in most studies (159, 188, 189), and ghrelin rise was inversely related to the decrease in these with surgery (159, 188). Other data support that hyperinsulinemia suppresses ghrelin (194–197). Interestingly, in one study, rise in ghrelin correlated with increase in body fat with surgical remission (159). These may be related. In rodents, ghrelin induces lipogenesis, reduces fat utilization, and promotes weight gain (198–200), and in humans, ghrelin increased food intake (201) and promoted weight gain. Ghrelin and body fat also relate inversely during GH therapy (202). Transition from the state of increased lipolysis and energy expenditure (EE) before to that favoring lipogenesis and decreased EE after surgery (48, 110) could, in part, reflect ghrelin effects. A GH-overexpressing rodent model featured increased EE and resisted diet-induced obesity (203). Although a rise in ghrelin with increasing fat mass may seem paradoxical, ghrelin is dysregulated in active acromegaly, but after surgical remission, this may be reset to the expected negative correlations between ghrelin levels and BMI. By contrast, somatostatin analogs (SRLs) suppress ghrelin levels (188, 204, 205). Potentially, ghrelin suppression protects from weight gain similarly to the resistance to diet-induced obesity and increased EE of mice lacking ghrelin receptors (206). However, it is unknown whether SRL therapy is associated with less gain in central adiposity than other acromegaly therapies, but if shown, this would be important to consider in choosing an acromegaly therapy. SRL suppression of other gut and pancreatic hormones, which may impair glucose tolerance despite biochemical control, may also contribute to their effect on the acromegaly lipodystrophy and its metabolic consequences (207).
GH has well-known direct effects on metabolism in peripheral tissues (208), but recent data show that GH also acts in brain to control energy metabolism (209, 210). Systemic GH administration in mice was shown to activate Agouti-related peptide (AgRP) neurons to produce orexigenic responses, and GH receptor antagonism with pegvisomant attenuated them (209). Transgenic central nervous system (CNS) GH overexpression increased hypothalamic AgRP and Neuropeptide Y(NPY) expression and food intake (211). These data suggest that GH stimulation of AgRP may be a mechanism by which GH restores energy homeostasis during nutrient deprivation (209). Because hypothalamic AgRP promotes food intake and weight gain (212) and plasma AgRP levels rise with caloric restriction in humans (213), AgRP may mediate the metabolic effects of GH’s rise in physiologic settings that need nutritional intake (214). AgRP-Growth Hormone Releasing Hormone (GHRH) neuron interaction may also couple nutritional status with growth (82). In humans, plasma AgRP levels increase after acute and chronic caloric restriction and relate to nutritional state in the pattern expected for hypothalamic AgRP (213, 215–217). We recently found that the plasma AgRP levels are higher in active acromegaly than in matched healthy subjects and are lower after surgery that reduced GH/IGF-1 or pegvisomant that lowered IGF-1 levels (218), suggesting that GH/IGF-1 and AgRP are positively related. AgRP and proopiomelanocortin (POMC) neurons also integrate CNS pathways that modulate glucose utilization and production (219–224). In healthy humans, the plasma AgRP levels relate to insulin levels and HOMA score (213, 215, 216), and, in mice, evidence supports an effect of AgRP neuron activation to impair glucose metabolism (220, 225–231). These data suggest a possible role for GH excess, acting centrally on AgRP, in the metabolic abnormalities of acromegaly. Although, in acromegaly, the peripheral actions of chronic GH excess to reduce AT stores predominate clinically over AgRP’s central mechanisms that, conversely, promote adiposity and fatty liver (230, 231), the rise in AgRP could prevent even more fat loss in this setting. A potential role for the GH-AgRP axis in the acromegaly lipodystrophy warrants further study.
The acromegaly-specific lipodystrophy features IR in the setting of a unique body composition pattern of reduced VAT and IHL and of impaired lipid storage in SAT, resulting in ectopic lipid deposition in muscle (20). The lipodystrophy results from a complex interplay of direct effects of GH on AT, primarily driven by accelerated lipolysis and the resultant promotion of IR. The effects on adipokines, ghrelin, and AgRP may also be important to producing IR in the face of low AT storage, as well as promoting AT mass regain along with reductions in IR during the lipodystrophy recovery. Increases in body fat with recovery of the lipodystrophy are nearly balanced by reduction in non-SM lean tissues such that only small increases in body weight occur (21, 159). Acromegaly therapies act by different mechanisms and at different targets along the GH–IGF-1 axis and may not impact all aspects of the lipodystrophy similarly. Gender differences in the lipodystrophy and its recovery require further study. Further investigation on the mechanisms of IR in AT, how AT distribution changes relate to IR, and the role of muscle lipid in IR in active acromegaly and during its treatment is warranted. Pegvisomant seems to reverse the acromegaly lipodystrophy pattern similarly to surgical therapy, but modern body composition methods have not yet been used to assess how it changes with SLR therapy. Interestingly, biochemical control of acromegaly reduces cardiovascular (CV) disease despite the post-treatment rise in VAT mass that, in the general population, is linked to CV risk. Whether VAT/IHL rise with acromegaly treatment persists and whether overtreatment could lead them to become above normal and potentially effect cardiovascular or diabetes risk require further study. Increases in fat mass may impact negatively on patients’ body image (232) and worsen quality of life (233) despite effective acromegaly treatment, and the development of mechanistic-directed therapies aimed at mitigating this during acromegaly therapy should be considered. Mechanisms for the acromegaly lipodystrophy are not fully elucidated, and understanding its pathophysiology and how therapies differentially impact its recovery is important to optimizing the long-term outcome for patients with this disease.
The original contributions presented in the study are included in the article/supplementary material. Further inquiries can be directed to the corresponding author.
The studies involving human participants were reviewed and approved by Institutional Review Board, Columbia University Irving Medical Center. The patients/participants provided their written informed consent to participate in this study.
The author confirms being the sole contributor of this work and has approved it for publication.
This work was funded by NIH grant DK110771.
The author declares that the research was conducted in the absence of any commercial or financial relationships that could be construed as a potential conflict of interest.
All claims expressed in this article are solely those of the authors and do not necessarily represent those of their affiliated organizations, or those of the publisher, the editors and the reviewers. Any product that may be evaluated in this article, or claim that may be made by its manufacturer, is not guaranteed or endorsed by the publisher.
1. Ho KK, O’Sullivan AJ, Hoffman DM. Metabolic actions of growth hormone in man. Endocr J (1996) 43 Suppl:S57–63. doi: 10.1507/endocrj.43.suppl_s57
2. Mauras N, O’Brien KO, Welch S, Rini A, Helgeson K, Vieira NE, et al. Insulin-like growth factor I and growth hormone (GH) treatment in GH-deficient humans: differential effects on protein, glucose, lipid, and calcium metabolism. J Clin Endocrinol Metab (2000) 85(4):1686–94. doi: 10.1210/jcem.85.4.6541
3. Borland CA, Barber MC, Travers MT, Vernon RG. Growth hormone inhibition of lipogenesis in sheep adipose tissue: requirement for gene transcription and polyamines. J Endocrinol (1994) 142(2):235–43. doi: 10.1677/joe.0.1420235
4. Alexopoulou O, Bex M, Kamenicky P, Mvoula AB, Chanson P, Maiter D. Prevalence and risk factors of impaired glucose tolerance and diabetes mellitus at diagnosis of acromegaly: a study in 148 patients. Pituitary (2014) 17(1):81–9. doi: 10.1007/s11102-013-0471-7
5. Gonzalez B, Vargas G, de Los Monteros ALE, Mendoza V, Mercado M. Persistence of diabetes and hypertension after multimodal treatment of acromegaly. J Clin Endocrinol Metab (2018) 103(6):2369–75. doi: 10.1210/jc.2018-00325
6. Petrossians P, Daly AF, Natchev E, Maione L, Blijdorp K, Sahnoun-Fathallah M, et al. Acromegaly at diagnosis in 3173 patients from the liege acromegaly survey (LAS) database. Endocr Relat Cancer (2017) 24(10):505–18. doi: 10.1530/ERC-17-0253
7. Espinosa-de-los-Monteros AL, Gonzalez B, Vargas G, Sosa E, Mercado M. Clinical and biochemical characteristics of acromegalic patients with different abnormalities in glucose metabolism. Pituitary (2011) 14(3):231–5. doi: 10.1007/s11102-010-0284-x
8. Roelfsema F, Frolich M. Glucose tolerance and plasma immunoreactive insulin levels in acromegalics before and after selective transsphenoidal surgery. Clin Endocrinol (Oxf) (1985) 22(4):531–7. doi: 10.1111/j.1365-2265.1985.tb00153.x
9. Kinoshita Y, Fujii H, Takeshita A, Taguchi M, Miyakawa M, Oyama K, et al. Impaired glucose metabolism in Japanese patients with acromegaly is restored after successful pituitary surgery if pancreatic {beta}-cell function is preserved. Eur J Endocrinol (2011) 164(4):467–73. doi: 10.1530/EJE-10-1096
10. Vila G, Jorgensen JOL, Luger A, Stalla GK. Insulin resistance in patients with acromegaly. Front Endocrinol (Lausanne) (2019) 10:509. doi: 10.3389/fendo.2019.00509
11. Rajasoorya C, Holdaway IM, Wrightson P, Scott DJ, Ibbertson HK. Determinants of clinical outcome and survival in acromegaly. Clin Endocrinol (Oxf) (1994) 41(1):95–102. doi: 10.1111/j.1365-2265.1994.tb03789.x
12. Hocking S, Samocha-Bonet D, Milner KL, Greenfield JR, Chisholm DJ. Adiposity and insulin resistance in humans: the role of the different tissue and cellular lipid depots. Endocr Rev (2013) 34(4):463–500. doi: 10.1210/er.2012-1041
13. Tchernof A, Despres JP. Pathophysiology of human visceral obesity: an update. Physiol Rev (2013) 93(1):359–404. doi: 10.1152/physrev.00033.2011
14. Tchkonia T, Thomou T, Zhu Y, Karagiannides I, Pothoulakis C, Jensen MD, et al. Mechanisms and metabolic implications of regional differences among fat depots. Cell Metab (2013) 17(5):644–56. doi: 10.1016/j.cmet.2013.03.008
15. Nielsen TS, Jessen N, Jorgensen JO, Moller N, Lund S. Dissecting adipose tissue lipolysis: molecular regulation and implications for metabolic disease. J Mol Endocrinol (2014) 52(3):R199–222. doi: 10.1530/JME-13-0277
16. Vega GL, Adams-Huet B, Peshock R, Willett D, Shah B, Grundy SM. Influence of body fat content and distribution on variation in metabolic risk. J Clin Endocrinol Metab (2006) 91(11):4459–66. doi: 10.1210/jc.2006-0814
17. Despres JP. Abdominal obesity as important component of insulin-resistance syndrome. Nutrition (1993) 9(5):452–9.
18. Bjorntorp P. The associations between obesity, adipose tissue distribution and disease. Acta Med Scand Suppl (1988) 723:121–34. doi: 10.1111/j.0954-6820.1987.tb05935.x
19. Lafontan M, Berlan M. Do regional differences in adipocyte biology provide new pathophysiological insights? Trends Pharmacol Sci (2003) 24(6):276–83. doi: 10.1016/S0165-6147(03)00132-9
20. Freda PU, Shen W, Heymsfield SB, Reyes-Vidal CM, Geer EB, Bruce JN, et al. Lower visceral and subcutaneous but higher intermuscular adipose tissue depots in patients with growth hormone and insulin-like growth factor I excess due to acromegaly. J Clin Endocrinol Metab (2008) 93(6):2334–43. doi: 10.1210/jc.2007-2780
21. Reyes-Vidal CM, Mojahed H, Shen W, Jin Z, Arias-Mendoza F, Fernandez JC, et al. Adipose tissue redistribution and ectopic lipid deposition in active acromegaly and effects of surgical treatment. J Clin Endocrinol Metab (2015) 100(8):2496–55. doi: 10.1210/jc.2015-1917
22. Fisker S, Hansen B, Fuglsang J, Kristensen K, Ovesen P, Orskov H, et al. Gene expression of the GH receptor in subcutaneous and intraabdominal fat in healthy females: relationship to GH-binding protein. Eur J Endocrinol (2004) 150(6):773–7. doi: 10.1530/eje.0.1500773
23. Vendelbo MH, Christensen B, Gronbaek SB, Hogild M, Madsen M, Pedersen SB, et al. GH signaling in human adipose and muscle tissue during ‘feast and famine’: amplification of exercise stimulation following fasting compared to glucose administration. Eur J Endocrinol (2015) 173(3):283–90. doi: 10.1530/EJE-14-1157
24. Richard AJ, Stephens JM. Emerging roles of JAK-STAT signaling pathways in adipocytes. Trends Endocrinol Metab (2011) 22(8):325–32. doi: 10.1016/j.tem.2011.03.007
25. Nielsen C, Gormsen LC, Jessen N, Pedersen SB, Moller N, Lund S, et al. Growth hormone signaling in vivo in human muscle and adipose tissue: impact of insulin, substrate background, and growth hormone receptor blockade. J Clin Endocrinol Metab (2008) 93(7):2842–50. doi: 10.1210/jc.2007-2414
26. Russell-Jones DL, Weissberger AJ. The role of growth hormone in the regulation of body composition in the adult. Growth Regul (1996) 6(4):247–52.
27. Engelson ES, Glesby MJ, Mendez D, Albu JB, Wang J, Heymsfield SB, et al. Effect of recombinant human growth hormone in the treatment of visceral fat accumulation in HIV infection. J Acquir Immune Defic Syndr (2002) 30(4):379–91. doi: 10.1097/00042560-200208010-00002
28. Johannsson G, Marin P, Lonn L, Ottosson M, Stenlof K, Bjorntorp P, et al. Growth hormone treatment of abdominally obese men reduces abdominal fat mass, improves glucose and lipoprotein metabolism, and reduces diastolic blood pressure. J Clin Endocrinol Metab (1997) 82(3):727–34. doi: 10.1210/jcem.82.3.3809
29. Richelsen B. Action of growth hormone in adipose tissue. Horm Res (1997) 48 Suppl 5:105–10. doi: 10.1159/000191338
30. Hoffstedt J, Arner P, Hellers G, Lonnqvist F. Variation in adrenergic regulation of lipolysis between omental and subcutaneous adipocytes from obese and non-obese men. J Lipid Res (1997) 38(4):795–804. doi: 10.1016/S0022-2275(20)37246-1
31. Richelsen B, Pedersen SB, Moller-Pedersen T, Bak JF. Regional differences in triglyceride breakdown in human adipose tissue: effects of catecholamines, insulin, and prostaglandin E2. Metabolism (1991) 40(9):990–6. doi: 10.1016/0026-0495(91)90078-B
32. Nielsen S, Moller N, Christiansen JS, Jorgensen JO. Pharmacological antilipolysis restores insulin sensitivity during growth hormone exposure. Diabetes (2001) 50(10):2301–8. doi: 10.2337/diabetes.50.10.2301
33. Richelsen B, Pedersen SB, Kristensen K, Borglum JD, Norrelund H, Christiansen JS, et al. Regulation of lipoprotein lipase and hormone-sensitive lipase activity and gene expression in adipose and muscle tissue by growth hormone treatment during weight loss in obese patients. Metabolism (2000) 49(7):906–11. doi: 10.1053/meta.2000.6738
34. Zhao JT, Cowley MJ, Lee P, Birzniece V, Kaplan W, Ho KK. Identification of novel GH-regulated pathway of lipid metabolism in adipose tissue: a gene expression study in hypopituitary men. J Clin Endocrinol Metab (2011) 96(7):E1188–96. doi: 10.1210/jc.2010-2679
35. Johansen T, Richelsen B, Hansen HS, Din N, Malmlof K. Growth hormone-mediated breakdown of body fat: effects of GH on lipases in adipose tissue and skeletal muscle of old rats fed different diets. Horm Metab Res (2003) 35(4):243–50. doi: 10.1055/s-2003-39481
36. Simsolo RB, Ezzat S, Ong JM, Saghizadeh M, Kern PA. Effects of acromegaly treatment and growth hormone on adipose tissue lipoprotein lipase. J Clin Endocrinol Metab (1995) 80(11):3233–8. doi: 10.1210/jcem.80.11.7593431
37. Oscarsson J, Ottosson M, Eden S. Effects of growth hormone on lipoprotein lipase and hepatic lipase. J Endocrinol Invest (1999) 22(5 Suppl):2–9.
38. Baik M, Yu JH, Hennighausen L. Growth hormone-STAT5 regulation of growth, hepatocellular carcinoma, and liver metabolism. Ann N Y Acad Sci (2011) 1229:29–37. doi: 10.1111/j.1749-6632.2011.06100.x
39. Hogan JC, Stephens JM. The regulation of fatty acid synthase by STAT5A. Diabetes (2005) 54(7):1968–75. doi: 10.2337/diabetes.54.7.1968
40. Sharma R, Luong Q, Sharma VM, Harberson M, Harper B, Colborn A, et al. Growth hormone controls lipolysis by regulation of FSP27 expression. J Endocrinol (2018) 239(3):289–301. doi: 10.1530/JOE-18-0282
41. Sharma VM, Vestergaard ET, Jessen N, Kolind-Thomsen P, Nellemann B, Nielsen TS, et al. Growth hormone acts along the PPARgamma-FSP27 axis to stimulate lipolysis in human adipocytes. Am J Physiol Endocrinol Metab (2019) 316(1):E34–42. doi: 10.1152/ajpendo.00129.2018
42. Ottosson M, Vikman-Adolfsson K, Enerback S, Elander A, Bjorntorp P, Eden S. Growth hormone inhibits lipoprotein lipase activity in human adipose tissue. J Clin Endocrinol Metab (1995) 80(3):936–41. doi: 10.1210/jcem.80.3.7883853
43. Clasen BF, Krusenstjerna-Hafstrom T, Vendelbo MH, Thorsen K, Escande C, Moller N, et al. Gene expression in skeletal muscle after an acute intravenous GH bolus in human subjects: identification of a mechanism regulating ANGPTL4. J Lipid Res (2013) 54(7):1988–97. doi: 10.1194/jlr.P034520
44. Hochberg I, Tran QT, Barkan AL, Saltiel AR, Chandler WF, Bridges D. Gene expression signature in adipose tissue of acromegaly patients. PloS One (2015) 10(6):e0129359. doi: 10.1371/journal.pone.0129359
45. Olarescu NC, Berryman DE, Householder LA, Lubbers ER, List EO, Benencia F, et al. GH action influences adipogenesis of mouse adipose tissue-derived mesenchymal stem cells. J Endocrinol (2015) 226(1):13–23. doi: 10.1530/JOE-15-0012
46. Bluher S, Kratzsch J, Kiess W. Insulin-like growth factor I, growth hormone and insulin in white adipose tissue. Best Pract Res Clin Endocrinol Metab (2005) 19(4):577–87. doi: 10.1016/j.beem.2005.07.011
47. Wabitsch M, Heinze E, Hauner H, Shymko RM, Teller WM, De Meyts P, et al. Biological effects of human growth hormone in rat adipocyte precursor cells and newly differentiated adipocytes in primary culture. Metabolism (1996) 45(1):34–42. doi: 10.1016/s0026-0495(96)90197-3
48. O’Sullivan AJ, Kelly JJ, Hoffman DM, Freund J, Ho KK. Body composition and energy expenditure in acromegaly. J Clin Endocrinol Metab (1994) 78(2):381–6. doi: 10.1210/jcem.78.2.8106626
49. Bengtsson BA, Brummer RJ, Eden S, Bosaeus I. Body composition in acromegaly. Clin Endocrinol (Oxf) (1989) 30(2):121–30. doi: 10.1111/j.1365-2265.1989.tb03733.x
50. Brummer RJ, Lonn L, Kvist H, Grangard U, Bengtsson BA, Sjostrom L. Adipose tissue and muscle volume determination by computed tomography in acromegaly, before and 1 year after adenomectomy. Eur J Clin Invest (1993) 23(4):199–205. doi: 10.1111/j.1365-2362.1993.tb00762.x
51. Heymsfield SB, Wang Z, Baumgartner RN, Ross R. Human body composition: advances in models and methods. Annu Rev Nutr (1997) 17:527–58. doi: 10.1146/annurev.nutr.17.1.527
52. McLellan AR, Connell JM, Beastall GH, Teasdale G, Davies DL. Growth hormone, body composition and somatomedin c after treatment of acromegaly. Q J Med (1988) 69(260):997–1008.
53. Sucunza N, Barahona MJ, Resmini E, Fernandez-Real JM, Farrerons J, Lluch P, et al. Gender dimorphism in body composition abnormalities in acromegaly: males are more affected than females. Eur J Endocrinol (2008) 159(6):773–9. doi: 10.1530/EJE-08-0449
54. Reid TJ, Jin Z, Shen W, Reyes-Vidal CM, Fernandez JC, Bruce JN, et al. IGF-1 levels across the spectrum of normal to elevated in acromegaly: relationship to insulin sensitivity, markers of cardiovascular risk and body composition. Pituitary (2015) 18(6):808–19. doi: 10.1007/s11102-015-0657-2
55. Olarescu NC, Heck A, Godang K, Ueland T, Bollerslev J. The metabolic risk in patients newly diagnosed with acromegaly is related to fat distribution and circulating adipokines and improves after treatment. Neuroendocrinology (2016) 103(3-4):197–206. doi: 10.1159/000371818
56. Plockinger U, Reuter T. Pegvisomant increases intra-abdominal fat in patients with acromegaly: a pilot study. Eur J Endocrinol (2008) 158(4):467–71. doi: 10.1530/EJE-07-0637
57. Kuker AP, Shen W, Jin Z, Singh S, Chen J, Bruce JN, et al. Body composition changes with long-term pegvisomant therapy of acromegaly. J Endocr Soc (2021) 5(3):bvab004. doi: 10.1210/jendso/bvab004
58. Hansen TB, Gram J, Bjerre P, Hagen C, Bollerslev J. Body composition in active acromegaly during treatment with octreotide: a double-blind, placebo-controlled cross-over study. Clin Endocrinol (Oxf) (1994) 41(3):323–9. doi: 10.1111/j.1365-2265.1994.tb02552.x
59. Wolf P, Salenave S, Durand E, Young J, Kamenicky P, Chanson P, et al. Treatment of acromegaly has substantial effects on body composition: a long-term follow-up study. Eur J Endocrinol (2021) 186(2):173–81. doi: 10.1530/EJE-21-0900
60. Guo X, Gao L, Shi X, Li H, Wang Q, Wang Z, et al. Pre- and postoperative body composition and metabolic characteristics in patients with acromegaly: A prospective study. Int J Endocrinol (2018) 2018:4125013. doi: 10.1155/2018/4125013
61. Geer EB, Shen W. Gender differences in insulin resistance, body composition, and energy balance. Gend Med (2009) 6 Suppl 1:60–75. doi: 10.1016/j.genm.2009.02.002
62. Ho KKY, Gibney J, Johannsson G, Wolthers T. Regulating of growth hormone sensitivity by sex steroids: implications for therapy. Front Horm Res (2006) 35:115–28. doi: 10.1159/000094314
63. Moller N, Jorgensen JO. Effects of growth hormone on glucose, lipid, and protein metabolism in human subjects. Endocr Rev (2009) 30(2):152–77. doi: 10.1210/er.2008-0027
64. Liu W, Thomas SG, Asa SL, Gonzalez-Cadavid N, Bhasin S, Ezzat S. Myostatin is a skeletal muscle target of growth hormone anabolic action. J Clin Endocrinol Metab (2003) 88(11):5490–6. doi: 10.1210/jc.2003-030497
65. Battezzati A, Benedini S, Fattorini A, Losa M, Mortini P, Bertoli S, et al. Insulin action on protein metabolism in acromegalic patients. Am J Physiol Endocrinol Metab (2003) 284(4):E823–9. doi: 10.1152/ajpendo.00020.2002
66. Gibney J, Wolthers T, Johannsson G, Umpleby AM, Ho KK. Growth hormone and testosterone interact positively to enhance protein and energy metabolism in hypopituitary men. Am J Physiol Endocrinol Metab (2005) 289(2):E266–71. doi: 10.1152/ajpendo.00483.2004
67. Freda PU, Shen W, Reyes-Vidal CM, Geer EB, Arias-Mendoza F, Gallagher D, et al. Skeletal muscle mass in acromegaly assessed by magnetic resonance imaging and dual-photon x-ray absorptiometry. J Clin Endocrinol Metab (2009) 94(8):2880–6. doi: 10.1210/jc.2009-0026
68. Bengtsson BA, Brummer RJ, Eden S, Bosaeus I, Lindstedt G. Body composition in acromegaly: the effect of treatment. Clin Endocrinol (Oxf) (1989) 31(4):481–90. doi: 10.1111/j.1365-2265.1989.tb01272.x
69. Madeira M, Neto LV, de Lima GA, Moreira RO, de Mendonca LM, Gadelha MR, et al. Effects of GH-IGF-I excess and gonadal status on bone mineral density and body composition in patients with acromegaly. Osteoporos Int (2010) 21(12):2019–25. doi: 10.1007/s00198-009-1165-x
70. Pietrobelli A, Wang Z, Heymsfield SB. Techniques used in measuring human body composition. Curr Opin Clin Nutr Metab Care (1998) 1(5):439–48. doi: 10.1097/00075197-199809000-00013
71. Katznelson L, Kleinberg D, Vance ML, Stavrou S, Pulaski KJ, Schoenfeld DA, et al. Hypogonadism in patients with acromegaly: data from the multi-centre acromegaly registry pilot study. Clin Endocrinol (Oxf) (2001) 54(2):183–8. doi: 10.1046/j.1365-2265.2001.01214.x
72. Mastaglia FL, Barwich DD, Hall R. Myopathy in acromegaly. Lancet (1970) 2(7679):907–9. doi: 10.1016/s0140-6736(70)92072-6
73. Lopes AJ, Ferreira AS, Walchan EM, Soares MS, Bunn PS, Guimaraes FS. Explanatory models of muscle performance in acromegaly patients evaluated by knee isokinetic dynamometry: Implications for rehabilitation. Hum Mov Sci (2016) 49:160–9. doi: 10.1016/j.humov.2016.07.005
74. Homem TS, Guimaraes FS, Soares MS, Kasuki L, Gadelha MR, Lopes AJ. Balance control and peripheral muscle function in aging: A comparison between individuals with acromegaly and healthy subjects. J Aging Phys Act (2017) 25(2):218–27. doi: 10.1123/japa.2016-0100
75. Fuchtbauer L, Olsson DS, Bengtsson BA, Norrman LL, Sunnerhagen KS, Johannsson G. Muscle strength in patients with acromegaly at diagnosis and during long-term follow-up. Eur J Endocrinol (2017) 177(2):217–26. doi: 10.1530/EJE-17-0120
76. Song MY, Ruts E, Kim J, Janumala I, Heymsfield S, Gallagher D. Sarcopenia and increased adipose tissue infiltration of muscle in elderly African American women. Am J Clin Nutr (2004) 79(5):874–80. doi: 10.1093/ajcn/79.5.874
77. Gallagher D, Kuznia P, Heshka S, Albu J, Heymsfield SB, Goodpaster B, et al. Adipose tissue in muscle: a novel depot similar in size to visceral adipose tissue. Am J Clin Nutr (2005) 81(4):903–10. doi: 10.1093/ajcn/81.4.903
78. Kim J, Heshka S, Gallagher D, Kotler DP, Mayer L, Albu J, et al. Intermuscular adipose tissue-free skeletal muscle mass: estimation by dual-energy X-ray absorptiometry in adults. J Appl Physiol (2004) 97(2):655–60. doi: 10.1152/japplphysiol.00260.2004
79. Rabinowitz D, Klassen GA, Zierler KL. Effect of human growth hormone on muscle and adipose tissue metabolism in the forearm of man. J Clin Invest (1965) 44:51–61. doi: 10.1172/JCI105126
80. Moller N, Jorgensen JO, Alberti KG, Flyvbjerg A, Schmitz O. Short-term effects of growth hormone on fuel oxidation and regional substrate metabolism in normal man. J Clin Endocrinol Metab (1990) 70(4):1179–86. doi: 10.1210/jcem-70-4-1179
81. Moller N, Butler PC, Antsiferov MA, Alberti KG. Effects of growth hormone on insulin sensitivity and forearm metabolism in normal man. Diabetologia (1989) 32(2):105–10. doi: 10.1007/BF00505182
82. Lee B, Kim J, An T, Kim S, Patel EM, Raber J, et al. Dlx1/2 and otp coordinate the production of hypothalamic GHRH- and AgRP-neurons. Nat Commun (2018) 9(1):2026. doi: 10.1038/s41467-018-04377-4
83. Krag MB, Gormsen LC, Guo Z, Christiansen JS, Jensen MD, Nielsen S, et al. Growth hormone-induced insulin resistance is associated with increased intramyocellular triglyceride content but unaltered VLDL-triglyceride kinetics. Am J Physiol Endocrinol Metab (2007) 292(3):E920–7. doi: 10.1152/ajpendo.00374.2006
84. Krssak M, Falk Petersen K, Dresner A, DiPietro L, Vogel SM, Rothman DL, et al. Intramyocellular lipid concentrations are correlated with insulin sensitivity in humans: a 1H NMR spectroscopy study. Diabetologia (1999) 42(1):113–6. doi: 10.1007/s001250051123
85. Boden G, Lebed B, Schatz M, Homko C, Lemieux S. Effects of acute changes of plasma free fatty acids on intramyocellular fat content and insulin resistance in healthy subjects. Diabetes (2001) 50(7):1612–7. doi: 10.2337/diabetes.50.7.1612
86. Goodpaster BH, Krishnaswami S, Resnick H, Kelley DE, Haggerty C, Harris TB, et al. Association between regional adipose tissue distribution and both type 2 diabetes and impaired glucose tolerance in elderly men and women. Diabetes Care (2003) 26(2):372–9. doi: 10.2337/diacare.26.2.372
87. Albu JB, Kovera AJ, Allen L, Wainwright M, Berk E, Raja-Khan N, et al. Independent association of insulin resistance with larger amounts of intermuscular adipose tissue and a greater acute insulin response to glucose in African American than in white nondiabetic women. Am J Clin Nutr (2005) 82(6):1210–7. doi: 10.1093/ajcn/82.6.1210
88. Albu JB, Kenya S, He Q, Wainwright M, Berk ES, Heshka S, et al. Independent associations of insulin resistance with high whole-body intermuscular and low leg subcutaneous adipose tissue distribution in obese HIV-infected women. Am J Clin Nutr (2007) 86(1):100–6. doi: 10.1093/ajcn/86.1.100
89. Joy T, Grinspoon SK. Adipose compartmentalization and insulin resistance among obese HIV-infected women: the role of intermuscular adipose tissue. Am J Clin Nutr (2007) 86(1):5–6. doi: 10.1093/ajcn/86.1.5
90. Samuel VT, Petersen KF, Shulman GI. Lipid-induced insulin resistance: unravelling the mechanism. Lancet (2010) 375(9733):2267–77. doi: 10.1016/S0140-6736(10)60408-4
91. Samuel VT, Shulman GI. The pathogenesis of insulin resistance: integrating signaling pathways and substrate flux. J Clin Invest (2016) 126(1):12–22. doi: 10.1172/JCI77812
92. Moller N, Gjedsted J, Gormsen L, Fuglsang J, Djurhuus C. Effects of growth hormone on lipid metabolism in humans. Growth Horm IGF Res (2003) 13 Suppl A:S18–21. doi: 10.1016/S1096-6374(03)00048-0
93. Bredella MA, Schorr M, Dichtel LE, Gerweck AV, Young BJ, Woodmansee WW, et al. Body composition and ectopic lipid changes with biochemical control of acromegaly. J Clin Endocrinol Metab (2017) 102(11):4218–25. doi: 10.1210/jc.2017-01210
94. Szendroedi J, Zwettler E, Schmid AI, Chmelik M, Pacini G, Kacerovsky G, et al. Reduced basal ATP synthetic flux of skeletal muscle in patients with previous acromegaly. PloS One (2008) 3(12):e3958. doi: 10.1371/journal.pone.0003958
95. Madsen M, Krusenstjerna-Hafstrom T, Moller L, Christensen B, Vendelbo MH, Pedersen SB, et al. Fat content in liver and skeletal muscle changes in a reciprocal manner in patients with acromegaly during combination therapy with a somatostatin analog and a GH receptor antagonist: a randomized clinical trial. J Clin Endocrinol Metab (2012) 97(4):1227–35. doi: 10.1210/jc.2011-2681
96. Yki-Jarvinen H. Fat in the liver and insulin resistance. Ann Med (2005) 37(5):347–56. doi: 10.1080/07853890510037383
97. Fan Y, Menon RK, Cohen P, Hwang D, Clemens T, DiGirolamo DJ, et al. Liver-specific deletion of the growth hormone receptor reveals essential role of growth hormone signaling in hepatic lipid metabolism. J Biol Chem (2009) 284(30):19937–44. doi: 10.1074/jbc.M109.014308
98. Barclay JL, Nelson CN, Ishikawa M, Murray LA, Kerr LM, McPhee TR, et al. GH-dependent STAT5 signaling plays an important role in hepatic lipid metabolism. Endocrinology (2011) 152(1):181–92. doi: 10.1210/en.2010-0537
99. Cordoba-Chacon J, Majumdar N, List EO, Diaz-Ruiz A, Frank SJ, Manzano A, et al. Growth hormone inhibits hepatic De novo lipogenesis in adult mice. Diabetes (2015) 64(9):3093–103. doi: 10.2337/db15-0370
100. Ichikawa T, Nakao K, Hamasaki K, Furukawa R, Tsuruta S, Ueda Y, et al. Role of growth hormone, insulin-like growth factor 1 and insulin-like growth factor-binding protein 3 in development of non-alcoholic fatty liver disease. Hepatol Int (2007) 1(2):287–94. doi: 10.1007/s12072-007-9007-4
101. Takahashi Y, Iida K, Takahashi K, Yoshioka S, Fukuoka H, Takeno R, et al. Growth hormone reverses nonalcoholic steatohepatitis in a patient with adult growth hormone deficiency. Gastroenterology (2007) 132(3):938–43. doi: 10.1053/j.gastro.2006.12.024
102. Takano S, Kanzaki S, Sato M, Kubo T, Seino Y. Effect of growth hormone on fatty liver in panhypopituitarism. Arch Dis Child (1997) 76(6):537–8. doi: 10.1136/adc.76.6.537
103. Winhofer Y, Wolf P, Krssak M, Wolfsberger S, Tura A, Pacini G, et al. No evidence of ectopic lipid accumulation in the pathophysiology of the acromegalic cardiomyopathy. J Clin Endocrinol Metab (2014) 99(11):4299–306. doi: 10.1210/jc.2014-2242
104. Xie T, Ding H, Xia M, Zhang X, Sun W, Liu T, et al. Dynamic changes in the distribution of facial and abdominal adipose tissue correlated with surgical treatment in acromegaly. Endocrine (2018) 62(3):552–9. doi: 10.1007/s12020-018-1742-x
105. Fellinger P, Wolf P, Pfleger L, Krumpolec P, Krssak M, Klavins K, et al. Increased ATP synthesis might counteract hepatic lipid accumulation in acromegaly. JCI Insight (2020) 5(5):e134638. doi: 10.1172/jci.insight.134638
106. Olarescu NC, Bollerslev J. The impact of adipose tissue on insulin resistance in acromegaly. Trends Endocrinol Metab (2016) 27(4):226–37. doi: 10.1016/j.tem.2016.02.005
107. Rosen ED, Spiegelman BM. What we talk about when we talk about fat. Cell (2014) 156(1-2):20–44. doi: 10.1016/j.cell.2013.12.012
108. Bays HE. Adiposopathy is “sick fat” a cardiovascular disease? J Am Coll Cardiol (2011) 57(25):2461–73. doi: 10.1016/j.jacc.2011.02.038
109. Hansen I, Tsalikian E, Beaufrere B, Gerich J, Haymond M, Rizza R. Insulin resistance in acromegaly: defects in both hepatic and extrahepatic insulin action. Am J Physiol (1986) 250(3 Pt 1):E269–73. doi: 10.1152/ajpendo.1986.250.3.E269
110. Moller N, Schmitz O, Joorgensen JO, Astrup J, Bak JF, Christensen SE, et al. Basal- and insulin-stimulated substrate metabolism in patients with active acromegaly before and after adenomectomy. J Clin Endocrinol Metab (1992) 74(5):1012–9. doi: 10.1210/jcem.74.5.1569148
111. Forrest L, Sedmak C, Sikder S, Grewal S, Harman SM, Blackman MR, et al. Effects of growth hormone on hepatic insulin sensitivity and glucose effectiveness in healthy older adults. Endocrine (2019) 63(3):497–506. doi: 10.1007/s12020-018-01834-4
112. Fineberg SE, Merimee TJ, Rabinowitz D, Edgar PJ. Insulin secretion in acromegaly. J Clin Endocrinol Metab (1970) 30(3):288–92. doi: 10.1210/jcem-30-3-288
113. Karlander S, Vranic M, Efendic S. Increased glucose turnover and glucose cycling in acromegalic patients with normal glucose tolerance. Diabetologia (1986) 29(11):778–83. doi: 10.1007/BF00873216
114. del Rincon JP, Iida K, Gaylinn BD, McCurdy CE, Leitner JW, Barbour LA, et al. Growth hormone regulation of p85alpha expression and phosphoinositide 3-kinase activity in adipose tissue: mechanism for growth hormone-mediated insulin resistance. Diabetes (2007) 56(6):1638–46. doi: 10.2337/db06-0299
115. Barbour LA, Mizanoor Rahman S, Gurevich I, Leitner JW, Fischer SJ, Roper MD, et al. Increased P85alpha is a potent negative regulator of skeletal muscle insulin signaling and induces in vivo insulin resistance associated with growth hormone excess. J Biol Chem (2005) 280(45):37489–94. doi: 10.1074/jbc.M506967200
116. Randle PJ. Regulatory interactions between lipids and carbohydrates: the glucose fatty acid cycle after 35 years. Diabetes Metab Rev (1998) 14(4):263–83. doi: 10.1002/(SICI)1099-0895(199812)14:4<263::AID-DMR233>3.0.CO;2-C
117. Nellemann B, Vendelbo MH, Nielsen TS, Bak AM, Hogild M, Pedersen SB, et al. Growth hormone-induced insulin resistance in human subjects involves reduced pyruvate dehydrogenase activity. Acta Physiol (Oxf) (2014):392–402. doi: 10.1111/apha.12183
118. Yeop Han C, Kargi AY, Omer M, Chan CK, Wabitsch M, O’Brien KD, et al. Differential effect of saturated and unsaturated free fatty acids on the generation of monocyte adhesion and chemotactic factors by adipocytes: dissociation of adipocyte hypertrophy from inflammation. Diabetes (2010) 59(2):386–96. doi: 10.2337/db09-0925
119. Rabinowitz D, Zierler KL. Differentiation of active from inactive acromegaly by studies of forearm metabolism and response to intra-arterial insulin. Bull Johns Hopkins Hosp (1963) 113:211–24.
120. Rizza RA, Mandarino LJ, Gerich JE. Effects of growth hormone on insulin action in man. mechanisms of insulin resistance, impaired suppression of glucose production, and impaired stimulation of glucose utilization. Diabetes (1982) 31(8 Pt 1):663–9. doi: 10.2337/diab.31.8.663
121. Yu C, Chen Y, Cline GW, Zhang D, Zong H, Wang Y, et al. Mechanism by which fatty acids inhibit insulin activation of insulin receptor substrate-1 (IRS-1)-associated phosphatidylinositol 3-kinase activity in muscle. J Biol Chem (2002) 277(52):50230–6. doi: 10.1074/jbc.M200958200
122. Petersen KF, Shulman GI. Etiology of insulin resistance. Am J Med (2006) 119(5 Suppl 1):S10–6. doi: 10.1016/j.amjmed.2006.01.009
123. Dresner A, Laurent D, Marcucci M, Griffin ME, Dufour S, Cline GW, et al. Effects of free fatty acids on glucose transport and IRS-1-associated phosphatidylinositol 3-kinase activity. J Clin Invest (1999) 103(2):253–9. doi: 10.1172/JCI5001
124. Perseghin G, Petersen K, Shulman GI. Cellular mechanism of insulin resistance: potential links with inflammation. Int J Obes Relat Metab Disord (2003) 27 Suppl 3:S6–11. doi: 10.1038/sj.ijo.0802491
125. Krebs M, Roden M. Molecular mechanisms of lipid-induced insulin resistance in muscle, liver and vasculature. Diabetes Obes Metab (2005) 7(6):621–32. doi: 10.1111/j.1463-1326.2004.00439.x
126. Griffin ME, Marcucci MJ, Cline GW, Bell K, Barucci N, Lee D, et al. Free fatty acid-induced insulin resistance is associated with activation of protein kinase c theta and alterations in the insulin signaling cascade. Diabetes (1999) 48(6):1270–4. doi: 10.1172/JCI5001
127. Boden G, Chen X. Effects of fat on glucose uptake and utilization in patients with non-insulin-dependent diabetes. J Clin Invest (1995) 96(3):1261–8. doi: 10.1172/JCI118160
128. Boden G. Fatty acid-induced inflammation and insulin resistance in skeletal muscle and liver. Curr Diabetes Rep (2006) 6(3):177–81. doi: 10.1007/s11892-006-0031-x
129. Roden M, Price TB, Perseghin G, Petersen KF, Rothman DL, Cline GW, et al. Mechanism of free fatty acid-induced insulin resistance in humans. J Clin Invest (1996) 97(12):2859–65. doi: 10.1172/JCI118742
130. Allister CA, Liu LF, Lamendola CA, Craig CM, Cushman SW, Hellerstein MK, et al. In vivo 2H2O administration reveals impaired triglyceride storage in adipose tissue of insulin-resistant humans. J Lipid Res (2015) 56(2):435–9. doi: 10.1194/jlr.M052860
131. Belfort R, Mandarino L, Kashyap S, Wirfel K, Pratipanawatr T, Berria R, et al. Dose-response effect of elevated plasma free fatty acid on insulin signaling. Diabetes (2005) 54(6):1640–8. doi: 10.2337/diabetes.54.6.1640
132. Kashyap S, Belfort R, Gastaldelli A, Pratipanawatr T, Berria R, Pratipanawatr W, et al. A sustained increase in plasma free fatty acids impairs insulin secretion in nondiabetic subjects genetically predisposed to develop type 2 diabetes. Diabetes (2003) 52(10):2461–74. doi: 10.2337/diabetes.52.10.2461
133. Ferrannini E, Barrett EJ, Bevilacqua S, DeFronzo RA. Effect of fatty acids on glucose production and utilization in man. J Clin Invest (1983) 72(5):1737–47. doi: 10.1172/JCI111133
134. Roden M, Stingl H, Chandramouli V, Schumann WC, Hofer A, Landau BR, et al. Effects of free fatty acid elevation on postabsorptive endogenous glucose production and gluconeogenesis in humans. Diabetes (2000) 49(5):701–7. doi: 10.2337/diabetes.49.5.701
135. Dominici FP, Turyn D. Growth hormone-induced alterations in the insulin-signaling system. Exp Biol Med (Maywood) (2002) 227(3):149–57. doi: 10.1177/153537020222700301
136. Krusenstjerna-Hafstrom T, Madsen M, Vendelbo MH, Pedersen SB, Christiansen JS, Moller N, et al. Insulin and GH signaling in human skeletal muscle in vivo following exogenous GH exposure: impact of an oral glucose load. PloS One (2011) 6(5):e19392. doi: 10.1371/journal.pone.0019392
137. Boero L, Manavela M, Merono T, Maidana P, Gomez Rosso L, Brites F. GH levels and insulin sensitivity are differently associated with biomarkers of cardiovascular disease in active acromegaly. Clin Endocrinol (Oxf) (2012) 77(4):579–85. doi: 10.1111/j.1365-2265.2012.04414.x
138. Foss MC, Saad MJ, Paccola GM, Paula FJ, Piccinato CE, Moreira AC. Peripheral glucose metabolism in acromegaly. J Clin Endocrinol Metab (1991) 72(5):1048–53. doi: 10.1210/jcem-72-5-1048
139. Higham CE, Rowles S, Russell-Jones D, Umpleby AM, Trainer PJ. Pegvisomant improves insulin sensitivity and reduces overnight free fatty acid concentrations in patients with acromegaly. J Clin Endocrinol Metab (2009) 94(7):2459–63. doi: 10.1210/jc.2008-2086
140. Arlien-Soborg MC, Dal J, Madsen MA, Hogild ML, Hjelholt AJ, Pedersen SB, et al. Reversible insulin resistance in muscle and fat unrelated to the metabolic syndrome in patients with acromegaly. EBioMedicine (2022) 75:103763. doi: 10.1016/j.ebiom.2021.103763
141. Olefsky JM, Glass CK. Macrophages, inflammation, and insulin resistance. Annu Rev Physiol (2010) 72:219–46. doi: 10.1146/annurev-physiol-021909-135846
142. Troike KM, Henry BE, Jensen EA, Young JA, List EO, Kopchick JJ, et al. Impact of growth hormone on regulation of adipose tissue. Compr Physiol (2017) 7(3):819–40. doi: 10.1002/cphy.c160027
143. Kim SH, Park MJ. Effects of growth hormone on glucose metabolism and insulin resistance in human. Ann Pediatr Endocrinol Metab (2017) 22(3):145–52. doi: 10.6065/apem.2017.22.3.145
144. Bolinder J, Ostman J, Werner S, Arner P. Insulin action in human adipose tissue in acromegaly. J Clin Invest (1986) 77(4):1201–6. doi: 10.1172/JCI112422
145. Ferrante AW Jr. Macrophages, fat, and the emergence of immunometabolism. J Clin Invest (2013) 123(12):4992–3. doi: 10.1172/JCI73658
146. Xu X, Grijalva A, Skowronski A, van Eijk M, Serlie MJ, Ferrante AW Jr. Obesity activates a program of lysosomal-dependent lipid metabolism in adipose tissue macrophages independently of classic activation. Cell Metab (2013) 18(6):816–30. doi: 10.1016/j.cmet.2013.11.001
147. Olarescu NC, Ueland T, Godang K, Lindberg-Larsen R, Jorgensen JO, Bollerslev J. Inflammatory adipokines contribute to insulin resistance in active acromegaly and respond differently to different treatment modalities. Eur J Endocrinol (2014) 170(1):39–48. doi: 10.1530/EJE-13-0523
148. Ferrante AW Jr. The immune cells in adipose tissue. Diabetes Obes Metab (2013) 15 Suppl 3:34–8. doi: 10.1111/dom.12154
149. Kosteli A, Sugaru E, Haemmerle G, Martin JF, Lei J, Zechner R, et al. Weight loss and lipolysis promote a dynamic immune response in murine adipose tissue. J Clin Invest (2010) 120(10):3466–79. doi: 10.1172/JCI42845
150. Capel F, Klimcakova E, Viguerie N, Roussel B, Vitkova M, Kovacikova M, et al. Macrophages and adipocytes in human obesity: adipose tissue gene expression and insulin sensitivity during calorie restriction and weight stabilization. Diabetes (2009) 58(7):1558–67. doi: 10.2337/db09-0033
151. Shi H, Kokoeva MV, Inouye K, Tzameli I, Yin H, Flier JS. TLR4 links innate immunity and fatty acid-induced insulin resistance. J Clin Invest (2006) 116(11):3015–25. doi: 10.1172/JCI28898
152. Suganami T, Tanimoto-Koyama K, Nishida J, Itoh M, Yuan X, Mizuarai S, et al. Role of the toll-like receptor 4/NF-kappaB pathway in saturated fatty acid-induced inflammatory changes in the interaction between adipocytes and macrophages. Arterioscler Thromb Vasc Biol (2007) 27(1):84–91. doi: 10.1161/01.ATV.0000251608.09329.9a
153. Osborn O, Olefsky JM. The cellular and signaling networks linking the immune system and metabolism in disease. Nat Med (2012) 18(3):363–74. doi: 10.1038/nm.2627
154. Cullberg KB, Larsen JO, Pedersen SB, Richelsen B. Effects of LPS and dietary free fatty acids on MCP-1 in 3T3-L1 adipocytes and macrophages in vitro. Nutr Diabetes (2014) 4:e113. doi: 10.1038/nutd.2014.10
155. Benencia F, Harshman S, Duran-Ortiz S, Lubbers ER, List EO, Householder L, et al. Male Bovine GH transgenic mice have decreased adiposity with an adipose depot-specific increase in immune cell populations. Endocrinology (2015) 156(5):1794–803. doi: 10.1210/en.2014-1794
156. Kumar PA, Chitra PS, Lu C, Sobhanaditya J, Menon R. Growth hormone (GH) differentially regulates NF-kB activity in preadipocytes and macrophages: implications for GH’s role in adipose tissue homeostasis in obesity. J Physiol Biochem (2014) 70(2):433–40. doi: 10.1007/s13105-014-0321-8
157. Lu C, Kumar PA, Sun J, Aggarwal A, Fan Y, Sperling MA, et al. Targeted deletion of growth hormone (GH) receptor in macrophage reveals novel osteopontin-mediated effects of GH on glucose homeostasis and insulin sensitivity in diet-induced obesity. J Biol Chem (2013) 288(22):15725–35. doi: 10.1074/jbc.M113.460212
158. Isozaki O, Tsushima T, Miyakawa M, Nozoe Y, Demura H, Seki H. Growth hormone directly inhibits leptin gene expression in visceral fat tissue in fatty zucker rats. J Endocrinol (1999) 161(3):511–6. doi: 10.1677/joe.0.1610511
159. Reyes-Vidal C, Fernandez JC, Bruce JN, Crisman C, Conwell IM, Kostadinov J, et al. Prospective study of surgical treatment of acromegaly: Effects on ghrelin, weight, adiposity, and markers of CV risk. J Clin Endocrinol Metab (2014) 99:2124–32 doi: 10.1210/jc.2014-2259
160. Olarescu NC, Ueland T, Lekva T, Dahl TB, Halvorsen B, Aukrust P, et al. Adipocytes as a source of increased circulating levels of nicotinamide phosphoribosyltransferase/visfatin in active acromegaly. J Clin Endocrinol Metab (2012) 97(4):1355–62. doi: 10.1210/jc.2011-2417
161. Ueland T, Fougner SL, Godang K, Lekva T, Schurgers LJ, Scholz H, et al. Associations between body composition, circulating interleukin-1 receptor antagonist, osteocalcin, and insulin metabolism in active acromegaly. J Clin Endocrinol Metab (2010) 95(1):361–8. doi: 10.1210/jc.2009-0422
162. Ronchi CL, Corbetta S, Cappiello V, Morpurgo PS, Giavoli C, Beck-Peccoz P, et al. Circulating adiponectin levels and cardiovascular risk factors in acromegalic patients. Eur J Endocrinol (2004) 150(5):663–9. doi: 10.1530/eje.0.1500663
163. Parkinson C, Whatmore AJ, Yates AP, Drake WM, Brabant G, Clayton PE, et al. The effect of pegvisomant-induced serum IGF-I normalization on serum leptin levels in patients with acromegaly. Clin Endocrinol (Oxf) (2003) 59(2):168–74. doi: 10.1046/j.1365-2265.2003.01795.x
164. Damjanovic SS, Petakov MS, Raicevic S, Micic D, Marinkovic J, Dieguez C, et al. Serum leptin levels in patients with acromegaly before and after correction of hypersomatotropism by trans-sphenoidal surgery. J Clin Endocrinol Metab (2000) 85(1):147–54. doi: 10.1210/jcem.85.1.6296
165. Miyakawa M, Tsushima T, Murakami H, Isozaki O, Demura H, Tanaka T. Effect of growth hormone (GH) on serum concentrations of leptin: study in patients with acromegaly and GH deficiency. J Clin Endocrinol Metab (1998) 83(10):3476–9. doi: 10.1210/jc.83.10.3476
166. Roemmler J, Otto B, Steffin B, Bidlingmaier M, Schopohl J. Serum leptin and ghrelin levels in active and inactive acromegalic patients during an oral glucose tolerance test. Exp Clin Endocrinol Diabetes (2009) 117(3):135–41. doi: 10.1055/s-2008-1078739
167. Bolanowski M, Milewicz A, Bidzinska B, Jedrzejuk D, Daroszewski J, Mikulski E. Serum leptin levels in acromegaly–a significant role for adipose tissue and fasting insulin/glucose ratio. Med Sci Monit (2002) 8(10):CR685–9.
168. Zhang Y, Chua S Jr. Leptin function and regulation. Compr Physiol (2017) 8(1):351–69. doi: 10.1002/cphy.c160041
169. Triantafyllou GA, Paschou SA, Mantzoros CS. Leptin and hormones: Energy homeostasis. Endocrinol Metab Clin North Am (2016) 45(3):633–45. doi: 10.1016/j.ecl.2016.04.012
170. D’Souza AM, Neumann UH, Glavas MM, Kieffer TJ. The glucoregulatory actions of leptin. Mol Metab (2017) 6(9):1052–65. doi: 10.1016/j.molmet.2017.04.011
171. Fujikawa T, Chuang JC, Sakata I, Ramadori G, Coppari R. Leptin therapy improves insulin-deficient type 1 diabetes by CNS-dependent mechanisms in mice. Proc Natl Acad Sci U S A (2010) 107(40):17391–6. doi: 10.1073/pnas.1008025107
172. Goncalves GH, Li W, Garcia AV, Figueiredo MS, Bjorbaek C. Hypothalamic agouti-related peptide neurons and the central melanocortin system are crucial mediators of leptin’s antidiabetic actions. Cell Rep (2014) 7(4):1093–103. doi: 10.1016/j.celrep.2014.04.010
173. Oral EA, Simha V, Ruiz E, Andewelt A, Premkumar A, Snell P, et al. Leptin-replacement therapy for lipodystrophy. N Engl J Med (2002) 346(8):570–8. doi: 10.1056/NEJMoa012437
174. Shimomura I, Hammer RE, Ikemoto S, Brown MS, Goldstein JL. Leptin reverses insulin resistance and diabetes mellitus in mice with congenital lipodystrophy. Nature (1999) 401(6748):73–6. doi: 10.1038/43448
175. Kumari B, Yadav UCS. Adipokine visfatin’s role in pathogenesis of diabesity and related metabolic derangements. Curr Mol Med (2018) 18(2):116–25. doi: 10.2174/1566524018666180705114131
176. Ciresi A, Amato MC, Pizzolanti G, Giordano C. Serum visfatin levels in acromegaly: Correlation with disease activity and metabolic alterations. Growth Horm IGF Res (2015) 25(5):240–6. doi: 10.1016/j.ghir.2015.07.002
177. Sucunza N, Barahona MJ, Resmini E, Fernandez-Real JM, Ricart W, Farrerons J, et al. A link between bone mineral density and serum adiponectin and visfatin levels in acromegaly. J Clin Endocrinol Metab (2009) 94(10):3889–96. doi: 10.1210/jc.2009-0474
178. Silha JV, Krsek M, Hana V, Marek J, Jezkova J, Weiss V, et al. Perturbations in adiponectin, leptin and resistin levels in acromegaly: lack of correlation with insulin resistance. Clin Endocrinol (Oxf) (2003) 58(6):736–42. doi: 10.1046/j.1365-2265.2003.01789.x
179. Lam KS, Xu A, Tan KC, Wong LC, Tiu SC, Tam S. Serum adiponectin is reduced in acromegaly and normalized after correction of growth hormone excess. J Clin Endocrinol Metab (2004) 89(11):5448–53. doi: 10.1210/jc.2003-032023
180. White UA, Maier J, Zhao P, Richard AJ, Stephens JM. The modulation of adiponectin by STAT5-activating hormones. Am J Physiol Endocrinol Metab (2016) 310(2):E129–36. doi: 10.1152/ajpendo.00068.2015
181. Frajese GV, Taylor NF, Jenkins PJ, Besser GM, Monson JP. Modulation of cortisol metabolism during treatment of acromegaly is independent of body composition and insulin sensitivity. Horm Res (2004) 61(5):246–51. doi: 10.1159/000077135
182. Stewart PM, Toogood AA, Tomlinson JW. Growth hormone, insulin-like growth factor-I and the cortisol-cortisone shuttle. Horm Res (2001) 56 Suppl 1:1–6. doi: 10.1159/000048126
183. Trainer PJ, Drake WM, Perry LA, Taylor NF, Besser GM, Monson JP. Modulation of cortisol metabolism by the growth hormone receptor antagonist pegvisomant in patients with acromegaly. J Clin Endocrinol Metab (2001) 86(7):2989–92. doi: 10.1210/jc.86.7.2989
184. Kojima M, Hosoda H, Date Y, Nakazato M, Matsuo H, Kangawa K. Ghrelin is a growth-hormone-releasing acylated peptide from stomach. Nature (1999) 402(6762):656–60. doi: 10.1038/45230
185. Cummings DE, Weigle DS, Frayo RS, Breen PA, Ma MK, Dellinger EP, et al. Plasma ghrelin levels after diet-induced weight loss or gastric bypass surgery. N Engl J Med (2002) 346(21):1623–30. doi: 10.1056/NEJMoa012908
186. Hansen TK, Dall R, Hosoda H, Kojima M, Kangawa K, Christiansen JS, et al. Weight loss increases circulating levels of ghrelin in human obesity. Clin Endocrinol (Oxf) (2002) 56(2):203–6. doi: 10.1046/j.0300-0664.2001.01456.x
187. Arvat E, Di Vito L, Broglio F, Papotti M, Muccioli G, Dieguez C, et al. Preliminary evidence that ghrelin, the natural GH secretagogue (GHS)-receptor ligand, strongly stimulates GH secretion in humans. J Endocrinol Invest (2000) 23(8):493–5. doi: 10.1007/BF03343763
188. Freda PU, Reyes CM, Conwell IM, Sundeen RE, Wardlaw SL. Serum ghrelin levels in acromegaly: effects of surgical and long-acting octreotide therapy. J Clin Endocrinol Metab (2003) 88(5):2037–44. doi: 10.1210/jc.2002-021683
189. Cappiello V, Ronchi C, Morpurgo PS, Epaminonda P, Arosio M, Beck-Peccoz P, et al. Circulating ghrelin levels in basal conditions and during glucose tolerance test in acromegalic patients. Eur J Endocrinol (2002) 147(2):189–94. doi: 10.1530/eje.0.1470189
190. Lee HM, Wang G, Englander EW, Kojima M, Greeley GH Jr. Ghrelin, a new gastrointestinal endocrine peptide that stimulates insulin secretion: enteric distribution, ontogeny, influence of endocrine, and dietary manipulations. Endocrinology (2002) 143(1):185–90. doi: 10.1210/endo.143.1.8602
191. Qi X, Reed J, Englander EW, Chandrashekar V, Bartke A, Greeley GH Jr. Evidence that growth hormone exerts a feedback effect on stomach ghrelin production and secretion. Exp Biol Med (Maywood) (2003) 228(9):1028–32. doi: 10.1177/153537020322800907
192. Nass R, Liu J, Hellmann P, Coschigano KT, Gaylinn B, Berryman DE, et al. Chronic changes in peripheral growth hormone levels do not affect ghrelin stomach mRNA expression and serum ghrelin levels in three transgenic mouse models. J Neuroendocrinol (2004) 16(8):669–75. doi: 10.1111/j.1365-2826.2004.01220.x
193. Vestergaard ET, Dall R, Lange KH, Kjaer M, Christiansen JS, Jorgensen JO. The ghrelin response to exercise before and after growth hormone administration. J Clin Endocrinol Metab (2007) 92(1):297–303. doi: 10.1210/jc.2006-1435
194. Tschop M, Weyer C, Tataranni PA, Devanarayan V, Ravussin E, Heiman ML. Circulating ghrelin levels are decreased in human obesity. Diabetes (2001) 50(4):707–9. doi: 10.2337/diabetes.50.4.707
195. Flanagan DE, Evans ML, Monsod TP, Rife F, Heptulla RA, Tamborlane WV, et al. The influence of insulin on circulating ghrelin. Am J Physiol Endocrinol Metab (2003) 284(2):E313–6. doi: 10.1152/ajpendo.00569.2001
196. Mohlig M, Spranger J, Otto B, Ristow M, Tschop M, Pfeiffer AF. Euglycemic hyperinsulinemia, but not lipid infusion, decreases circulating ghrelin levels in humans. J Endocrinol Invest (2002) 25(11):RC36–8. doi: 10.1007/BF03344062
197. Saad MF, Bernaba B, Hwu CM, Jinagouda S, Fahmi S, Kogosov E, et al. Insulin regulates plasma ghrelin concentration. J Clin Endocrinol Metab (2002) 87(8):3997–4000. doi: 10.1210/jcem.87.8.8879
198. Thompson NM, Gill DA, Davies R, Loveridge N, Houston PA, Robinson IC, et al. Ghrelin and des-octanoyl ghrelin promote adipogenesis directly in vivo by a mechanism independent of the type 1a growth hormone secretagogue receptor. Endocrinology (2004) 145(1):234–42. doi: 10.1210/en.2003-0899
199. Tschop M, Smiley DL, Heiman ML. Ghrelin induces adiposity in rodents. Nature (2000) 407(6806):908–13. doi: 10.1038/35038090
200. Wren AM, Small CJ, Abbott CR, Dhillo WS, Seal LJ, Cohen MA, et al. Ghrelin causes hyperphagia and obesity in rats. Diabetes (2001) 50(11):2540–7. doi: 10.2337/diabetes.50.11.2540
201. Wren AM, Seal LJ, Cohen MA, Brynes AE, Frost GS, Murphy KG, et al. Ghrelin enhances appetite and increases food intake in humans. J Clin Endocrinol Metab (2001) 86(12):5992. doi: 10.1210/jcem.86.12.8111
202. Eden Engstrom B, Burman P, Holdstock C, Karlsson FA. Effects of growth hormone (GH) on ghrelin, leptin, and adiponectin in GH-deficient patients. J Clin Endocrinol Metab (2003) 88(11):5193–8. doi: 10.1210/jc.2003-030713
203. Olsson B, Bohlooly YM, Fitzgerald SM, Frick F, Ljungberg A, Ahren B, et al. Bovine growth hormone transgenic mice are resistant to diet-induced obesity but develop hyperphagia, dyslipidemia, and diabetes on a high-fat diet. Endocrinology (2005) 146(2):920–30. doi: 10.1210/en.2004-1232
204. Broglio F, Koetsveld Pv P, Benso A, Gottero C, Prodam F, Papotti M, et al. Ghrelin secretion is inhibited by either somatostatin or cortistatin in humans. J Clin Endocrinol Metab (2002) 87(10):4829–32. doi: 10.1210/jc.2002-020956
205. Norrelund H, Hansen TK, Orskov H, Hosoda H, Kojima M, Kangawa K, et al. Ghrelin immunoreactivity in human plasma is suppressed by somatostatin. Clin Endocrinol (Oxf) (2002) 57(4):539–46. doi: 10.1046/j.1365-2265.2002.01649.x
206. Zigman JM, Nakano Y, Coppari R, Balthasar N, Marcus JN, Lee CE, et al. Mice lacking ghrelin receptors resist the development of diet-induced obesity. J Clin Invest (2005) 115(12):3564–72. doi: 10.1172/JCI26002
207. Henry RR, Ciaraldi TP, Armstrong D, Burke P, Ligueros-Saylan M, Mudaliar S. Hyperglycemia associated with pasireotide: results from a mechanistic study in healthy volunteers. J Clin Endocrinol Metab (2013) 98(8):3446–53. doi: 10.1210/jc.2013-1771
208. Jorgensen JO, Moller L, Krag M, Billestrup N, Christiansen JS. Effects of growth hormone on glucose and fat metabolism in human subjects. Endocrinol Metab Clin North Am (2007) 36(1):75–87. doi: 10.1016/j.ecl.2006.11.005
209. Furigo IC, Teixeira PDS, de Souza GO, Couto GCL, Romero GG, Perello M, et al. Growth hormone regulates neuroendocrine responses to weight loss via AgRP neurons. Nat Commun (2019) 10(1):662. doi: 10.1038/s41467-019-08607-1
210. Aramburo C, Alba-Betancourt C, Luna M, Harvey S. Expression and function of growth hormone in the nervous system: a brief review. Gen Comp Endocrinol (2014) 203:35–42. doi: 10.1016/j.ygcen.2014.04.035
211. Bohlooly YM, Olsson B, Bruder CE, Linden D, Sjogren K, Bjursell M, et al. Growth hormone overexpression in the central nervous system results in hyperphagia-induced obesity associated with insulin resistance and dyslipidemia. Diabetes (2005) 54(1):51–62. doi: 10.2337/diabetes.54.1.51
212. Krashes MJ, Koda S, Ye C, Rogan SC, Adams AC, Cusher DS, et al. Rapid, reversible activation of AgRP neurons drives feeding behavior in mice. J Clin Invest (2011) 121(4):1424–8. doi: 10.1172/JCI46229
213. Page-Wilson G, Nguyen KT, Atalayer D, Meece K, Bainbridge HA, Korner J, et al. Evaluation of CSF and plasma biomarkers of brain melanocortin activity in response to caloric restriction in humans. Am J Physiol Endocrinol Metab (2017) 312(1):E19–26. doi: 10.1152/ajpendo.00330.2016
214. Merle JV, Haas V, Burghardt R, Dohler N, Schneider N, Lehmkuhl U, et al. Agouti-related protein in patients with acute and weight-restored anorexia nervosa. Psychol Med (2011) 41(10):2183–92. doi: 10.1017/S0033291711000365
215. Page-Wilson G, Meece K, White A, Rosenbaum M, Leibel RL, Smiley R, et al. Proopiomelanocortin, agouti-related protein, and leptin in human cerebrospinal fluid: correlations with body weight and adiposity. Am J Physiol Endocrinol Metab (2015) 309(5):E458–65. doi: 10.1152/ajpendo.00206.2015
216. Page-Wilson G, Peters JB, Panigrahi SK, Jacobs TP, Korner J, Otten M, et al. Plasma agouti-related protein and cortisol levels in cushing disease: Evidence for the regulation of agouti-related protein by glucocorticoids in humans. J Clin Endocrinol Metab (2019) 104(3):961–9. doi: 10.1210/jc.2018-01909
217. Zagmutt S, Mera P, Soler-Vazquez MC, Herrero L, Serra D. Targeting AgRP neurons to maintain energy balance: Lessons from animal models. Biochem Pharmacol (2018) 155:224–32. doi: 10.1016/j.bcp.2018.07.008
218. Freda PU, Reyes-Vidal C, Jin Z, Pugh M, Panigrahi SK, Bruce JN, et al. Plasma agouti-related protein levels in acromegaly and effects of surgical or pegvisomant therapy. J Clin Endocrinol Metab (2019) 104(11):5453–61. doi: 10.1210/jc.2019-01079
219. Obici S, Feng Z, Tan J, Liu L, Karkanias G, Rossetti L. Central melanocortin receptors regulate insulin action. J Clin Invest (2001) 108(7):1079–85. doi: 10.1172/JCI12954
220. Uner AG, Kecik O, Quaresma PGF, De Araujo TM, Lee H, Li W, et al. Role of POMC and AgRP neuronal activities on glycaemia in mice. Sci Rep (2019) 9(1):13068. doi: 10.1038/s41598-019-49295-7
221. Ruud J, Steculorum SM, Bruning JC. Neuronal control of peripheral insulin sensitivity and glucose metabolism. Nat Commun (2017) 8:15259. doi: 10.1038/ncomms15259
222. Bruning JC, Gautam D, Burks DJ, Gillette J, Schubert M, Orban PC, et al. Role of brain insulin receptor in control of body weight and reproduction. Science (2000) 289(5487):2122–5. doi: 10.1126/science.289.5487.2122
223. Ren H, Cook JR, Kon N, Accili D. Gpr17 in AgRP neurons regulates feeding and sensitivity to insulin and leptin. Diabetes (2015) 64(11):3670–9. doi: 10.2337/db15-0390
224. Bell BB, Harlan SM, Morgan DA, Guo DF, Cui H, Rahmouni K. Differential contribution of POMC and AgRP neurons to the regulation of regional autonomic nerve activity by leptin. Mol Metab (2018) 8:1–12. doi: 10.1016/j.molmet.2017.12.006
225. Konner AC, Janoschek R, Plum L, Jordan SD, Rother E, Ma X, et al. Insulin action in AgRP-expressing neurons is required for suppression of hepatic glucose production. Cell Metab (2007) 5(6):438–49. doi: 10.1016/j.cmet.2007.05.004
226. Maier MT, Vilhelmsson A, Louie SM, Vagena E, Nomura DK, Koliwad SK, et al. Regulation of hepatic lipid accumulation and distribution by agouti-related protein in Male mice. Endocrinology (2018) 159(6):2408–20. doi: 10.1210/en.2018-00040
227. Nogueiras R, Wiedmer P, Perez-Tilve D, Veyrat-Durebex C, Keogh JM, Sutton GM, et al. The central melanocortin system directly controls peripheral lipid metabolism. J Clin Invest (2007) 117(11):3475–88. doi: 10.1172/JCI31743
228. Steculorum SM, Ruud J, Karakasilioti I, Backes H, Engstrom Ruud L, Timper K, et al. AgRP neurons control systemic insulin sensitivity via myostatin expression in brown adipose tissue. Cell (2016) 165(1):125–38. doi: 10.1016/j.cell.2016.02.044
229. Ruud L, Mafalda MA, Pereira MMA, de Solis AJ, Henning F,H, Bruning JC. NPY mediates the rapid feeding and glucose metabolism regulatory functions of AgRP neurons. Nat Commun (2020) 11(1):442. doi: 10.1038/s41467-020-14291-3
230. Korner J, Wissig S, Kim A, Conwell IM, Wardlaw SL. Effects of agouti-related protein on metabolism and hypothalamic neuropeptide gene expression. J Neuroendocrinol (2003) 15(12):1116–21. doi: 10.1111/j.1365-2826.2003.01113.x
231. Graham M, Shutter JR, Sarmiento U, Sarosi I, Stark KL. Overexpression of agrt leads to obesity in transgenic mice. Nat Genet (1997) 17(3):273–4. doi: 10.1038/ng1197-273
232. Conaglen HM, de Jong D, Crawford V, Elston MS, Conaglen JV. Body image disturbance in acromegaly patients compared to nonfunctioning pituitary adenoma patients and controls. Int J Endocrinol (2015) 2015:624872. doi: 10.1155/2015/624872
Keywords: acromegaly, growth hormone, lipodystrophy, adipose tissue, body composition, insulin resistance, ghrelin, AgRP
Citation: Freda PU (2022) The acromegaly lipodystrophy. Front. Endocrinol. 13:933039. doi: 10.3389/fendo.2022.933039
Received: 30 April 2022; Accepted: 15 August 2022;
Published: 13 September 2022.
Edited by:
Adriana G. Ioachimescu, School of Medicine, Emory University, United StatesReviewed by:
Zhu Huijuan, Peking Union Medical College Hospital (CAMS), ChinaCopyright © 2022 Freda. This is an open-access article distributed under the terms of the Creative Commons Attribution License (CC BY). The use, distribution or reproduction in other forums is permitted, provided the original author(s) and the copyright owner(s) are credited and that the original publication in this journal is cited, in accordance with accepted academic practice. No use, distribution or reproduction is permitted which does not comply with these terms.
*Correspondence: Pamela U. Freda, cHVmMUBjdW1jLmNvbHVtYmlhLmVkdQ==
Disclaimer: All claims expressed in this article are solely those of the authors and do not necessarily represent those of their affiliated organizations, or those of the publisher, the editors and the reviewers. Any product that may be evaluated in this article or claim that may be made by its manufacturer is not guaranteed or endorsed by the publisher.
Research integrity at Frontiers
Learn more about the work of our research integrity team to safeguard the quality of each article we publish.