- 1Institute of Reproductive Health, Tongji Medical College, Huazhong University of Science and Technology, Wuhan, China
- 2Department of Human Anatomy, Tongji Medical College, Huazhong University of Science and Technology, Wuhan, China
The discovery of kisspeptin as a critical central regulatory factor of GnRH release has given people a novel understanding of the neuroendocrine regulation in human reproduction. Kisspeptin activates the signaling pathway by binding to its receptor kisspeptin receptor (KISS1R) to promote GnRH secretion, thereby regulating the hypothalamic-pituitary-gonadal axis (HPG) axis. Recent studies have shown that kisspeptin neurons located in arcuate nucleus (ARC) co-express neurokinin B (NKB) and dynorphin (Dyn). Such neurons are called KNDy neurons. KNDy neurons participate in the positive and negative feedback of estrogen to GnRH secretion. In addition, kisspeptin is a key factor in the initiation of puberty, and also regulates the processes of female follicle development, oocyte maturation, and ovulation through the HPG axis. In male reproduction, kisspeptin also plays an important role, getting involved in the regulation of Leydig cells, spermatogenesis, sperm functions and reproductive behaviors. Mutations in the KISS1 gene or disorders of the kisspeptin/KISS1R system may lead to clinical symptoms such as idiopathic hypogonadotropic hypogonadism (iHH), central precocious puberty (CPP) and female infertility. Understanding the influence of kisspeptin on the reproductive axis and related mechanisms will help the future application of kisspeptin in disease diagnosis and treatment. In this review, we critically appraise the role of kisspeptin in the HPG axis, including its signaling pathways, negative and positive feedback mechanisms, and its control on female and male reproduction.
1 Introduction
Reproduction is essential to the survival of all species. The hypothalamic-pituitary-gonadal (HPG) axis which consists of anterior hypothalamus, pituitary, and the gonads plays an indispensable role in regulating reproduction in human and other animals (1). Female follicular development, egg maturation, ovulation and spermatogenesis in male are highly driven by HPG axis (2). As the promoter of HPG axis, the gonadotropin-releasing hormone (GnRH) neurons connect to their neuronal network and send projections to the median eminence (ME) after they travel to medial preoptic area (POA) (3, 4). They are final output cells of the neuronal network that controls secretion of gonadotropins by the pituitary gland in all mammals (5). However, it is well known that GnRH neurons lack estrogen receptor α and progesterone receptors, which indicates that the positive and negative feedback effect of estrogen and progesterone on the gonadal axis is not directly on GnRH neurons, but mediated by other neurons (6).
Kisspeptin is a neuropeptide which was first discovered by Lee et al. in 1996 (7). It was later found that it acts through the G-protein-coupled receptor, GPR54 (8). Although kisspeptins were first considered to be related to cancer metastasis, they were later found be closely related to reproduction, in which they modulate GnRH secretion, thus exerting a significant role in the regulation of the HPG axis (9, 10). Later, as the reproductive role for neurokinin B was discovered, kisspeptin was confirmed to be associated with positive and negative feedback of estrogen. In 2006, scientists found that proNKB was expressed in most prodynorphin-ir neurons in the rats arcuate nucleus (ARC), and almost all proNKB neurons are immunoreactive to prodynorphin, indicating that there is a close relationship between Dyn and NKB (11). The location of kisspeptin, neurokinin B(NKB) and dynorphin (Dyn) in the hypothalamus are overlapped and frequently colocalized in a very conserved region across species (11–13). By regulating the secretion of NKB and Dyn, ARC kisspeptin neurons modulate kisspeptin expression, thereby mediate the negative feedback of sex steroids (14). Besides, kisspeptin neurons which located in rostral periventricular region of the third ventricle (RP3V) (in rodents) and preoptic area (POA) (in human) regulate estradiol positive feedback by expressing ERα and cotransmitters such as tyrosine hydroxylase, GABA and glutamate, with the latter two have the effect of exciting GnRH neurons (15). These findings made the understanding of the HPG axis more complete and stimulated further interest in the field of the effect of kisspeptin in reproduction.
Since the relationship between kisspeptin and reproduction was reported, a large number of studies has been conducted about the regulatory role of kisspeptin in the HPG axis and its detailed mechanisms (10, 16–18).Besides, more and more studies have shown that kisspeptin, as an upstream regulator of HPG axis, plays an indispensable regulatory role in the beginning of puberty and participates in various processes of female reproduction whether in animals or human (9, 19). The function of kisspeptin in male reproduction have also been studied in a large number of amphibians, fish, rodents and primate, while it is still less clear (20–23). The increasing understanding of kisspeptin promotes the application of kisspeptin in clinical diagnosis and disease treatment. In this review, we summarize current understanding of the regulatory role of kisspeptin on HPG axis and discuss the effects of central and periphery kisspeptin on male and female reproduction.
2 Kisspeptin and Kisspeptin Receptor
2.1 Kisspeptin
In 1996, KISS1 gene were first found by Lee et al. when they were when studying different metastatic abilities of human melanoma cells (7). Interestingly, because it was discovered at the place where Hershey’s chocolate was produced, its name came from a kind of Hershey’s chocolate named kisses. In humans, KISS1 is located on the long (q) arm of chromosome 1 at q32 with four exons (7, 24). The KISS1 transcription start site (TSS) is located between -153 bp to -156 bp upstream of the ATG in human (25). The promoter of KISS1 contains numerous GC-rich elements, which are the binding sites of Sp1 family proteins. Sp1 and Sp3 are universal transcription factors which function by forming dimers. Sp1 activates the transcription while Sp3 represses it, thus the ratio of Sp1 and Sp3 is crucial for the differential expression of KISS1 in different tissues (26). The GC-rich motifs are also the targets of estrogen induced kisspeptin expression. Other regulators include Transcription Factor 1 (TTF1), ectoderm development (EED), Chromebox protein homolog 7 (CBX7) and so on (25). Among the four exons, the first two exons do not encode any peptides and the remaining two can be partially translated, encoding a precursor peptide containing 145 amino acids (24). The precursor, which has a 19 amino acid signal peptide, can further yield four short peptides, namely kisspeptin-54 (kp-54), kisspeptin-13 (kp-13), kisspeptin-10 (kp-10), distinguish by the number of amino acids, respectively (27, 28). These peptides are collectively referred to as kisspeptins, in which kp-54 is the main product. Kisspeptins belong to the RF amide peptide family, with special Arg – Phe – NH2 motifs in their C-terminal region, which contribute to their binding to kisspeptin receptor (KISS1R) (27).
The location of kisspeptin neurons are different between rodents and human. In rodents, kisspeptin neurons were discovered in ARC and the anteroventral periventricular nucleus (AVPV) which extends into the periventricular nucleus (PEN) (10, 29, 30). The latter two nucleus are also known collectively as RP3V (31). In humans, kisspeptin neurons were distributed predominantly in the infundibular nucleus and sparsely in POA (32). Interestingly, kisspeptin neurons within the rodent RP3V have obvious difference between male and female. The number of kisspeptin neurons in female rodents is prominently bigger than that in the male (33). In humans the situation is similar as studies have observed that there are much more kisspeptin fibers in the infundibular nucleus and rostral preoptic area in women than in men (34).
Apart from these places, kisspeptin can also be found in limbic and paralimbic brain regions in extra-hypothalamic areas and placenta, pancreas, ovary and liver in peripheral areas (33, 35–37). In rat and human ovaries, Kiss1 mRNA is primarily detected in granulosa cells, indicating that ovarian kisspeptins are possibly synthesized by granulosa cells (38, 39).
2.2 Kisspeptin Receptor
Kiss1R, first known as G-protein coupled receptor 54 (GPR54), AXOR12 or hOT7T175, was discovered in the rat brain in 1999 as an orphan receptor (17, 40). Being a G-protein-coupled receptor of seven transmembrane domains, KISS1R is a kind of Gq/11 protein-associated receptor. It was not until 2001 when GPR54 was identified as a putative receptor for KISS1-derived peptides (35, 36). In human, KISS1R is located on chromosome 19p13.3 and encodes a protein with 398 amino acids, which can be detected in the cerebral cortex, thalamus, pons-medulla and cerebellum in central areas (40, 41). In rodents, kisspeptin receptor is expressed in POA and ARC in hypothalamus and hippocampus outside of the hypothalamus (27, 35, 36, 40, 42). In fact, most GnRH neurons express Kiss1R mRNA in both young and adult mice (43). In the periphery, KISS1R mRNA exists in plenty of tissues in human, including pancreas, stomach, small intestine, thymus, spleen, lung, gonads and so on (35, 36). In human ovary, KISS1R is expressed in corpus luteum, parietal granulosa cells, granulosa cells, theca cells, oocytes, stromal cells and epithelial cells (44). Unlike Kiss1 mRNA is mainly expressed in granulosa cells, KISS1R expression is obviously higher in oocytes than that in granulosa cells (45). Also, KISS1R is expressed in human endometrial stromal cells and placental trophoblasts (46, 47).
2.3 Kisspeptin Signaling Pathway
After kisspeptin binds to KISS1R, the activated G protein-coupled receptor will be dissociated into Gαq/11-GTP and Gβγ (48). Gαq/11 then activate phospholipase C (PLC)-β, a key enzyme in the cytoplasm. Under the action of PLC-β, phosphatidylinositol 4, 5-bisphosphate (PIP2) is hydrolyzed to produce inositol- (1, 4, 5)-triphosphate (IP3) and diacylglycerol (DAG). IP3 then combines with the IP3-sensitive Ca2+ channel, inducing intracellular Ca2+ mobilization and release, which can promote various different functions depending on the cell context (27, 44, 49). An increase in Ca2+ could convey the ability of hormone secretion of kisspeptin and regulate its ability to inhibit cell proliferation (50). DAG activates protein kinase C, which subsequently causes phosphorylation signaling cascade and finally leads to the activation of mitogen-activated protein kinases (MAPKs), such as ERK1/2 and p38 (27) (Figure 1). However, the ERK1/2 and p38 activation caused by kisspeptin treatment varies between different cell types (50). In Chinese hamster ovary K1 cells treated with kisspeptin-10, ERK1/2 showed strong continuous phosphorylation, while p38 showed weak phosphorylation (27). Likewise, kisspeptin can stimulate ERK1/2 activation while P38 MAPK is not affected in cells from rat corpus luteum (51) and anaplastic thyroid cancer cells (52). In HT-1080 cell line, excessive expression with kisspeptin diminished synthesis of matrix metalloproteinase-9 (MMP-9) enzyme activity by inhibiting the translocation of nuclear factor κB (NF-κB), thereby decreasing promoter binding of NF-kB to the MMP-9 promoter (53). DAG also activates a nonselective cation channel (TRPC) and inhibits an inwardly rectifying potassium channel (Kir) at the same time, thus causing continuous depolarization of GnRH neurons and stimulates GnRH release (54). Additionally, activated KISS1R can recruit β-arrestin-1 and -2 to the membrane, which assist with KISS1R signaling (55, 56). β-arrestin-1 and β-arrestin-2 have contrast effects, while β-arrestin-1 promotes the phosphorylation of ERK1/2 while the other inhibits the process. Other studies have also confirmed that β-arrestin-1 and -2 play a role in modulating the ERK1/2 phosphorylation in different kinds of cells (57–59).
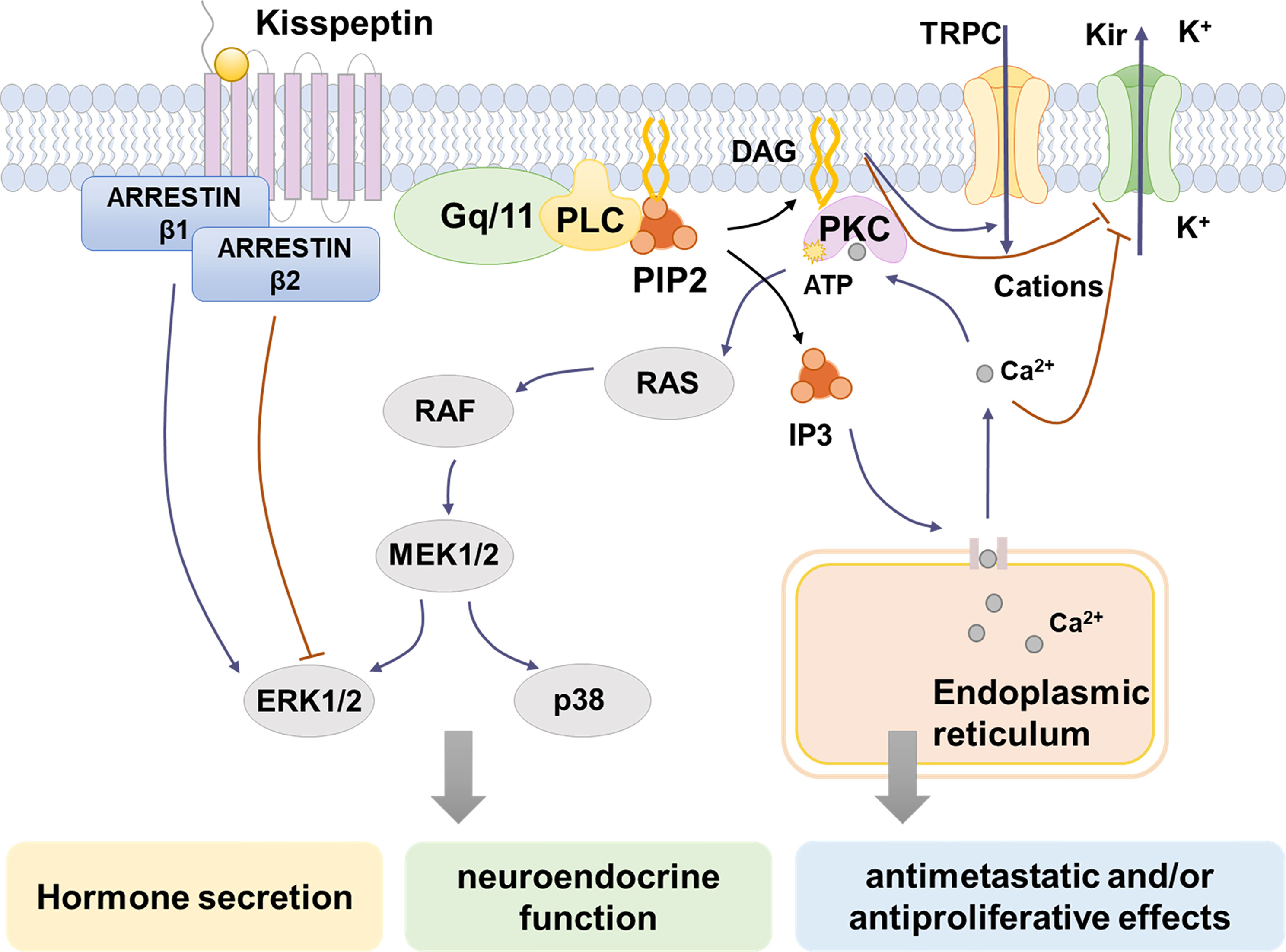
Figure 1 Kisspeptin/KISS1R signaling pathways. Kisspeptin binds to KISS1R, inducing the intracellular portion of KISS1R phosphorylates Gq/11. Then PLC is activated and it hydrolyzes PIP2 to IP3 and DAG. IP3 induces intracellular Ca2+ release from the endoplasmic reticulum, while DAG activates PKC, causing the phosphorylation of MAPK, such as ERK1/2 and p38. Moreover, the activation of KISS1R recruits arrestin-1 and -2, which decreases and increases ERK1/2 phosphorylation, respectively. The increase of intracellular Ca2+ changes ion channel permeability by block the inwardly rectifying potassium channel (Kir). The depolarization of GnRH neurons is caused by activation of a nonselective cation channel (TRPC) and suppression of Kir by DAG and increased Ca2+. Through the signaling pathways above, kisspeptins activate different MAPKs and cause the release of Ca2+, which contribute to hormone-releasing regulation, neuroendocrine function, antimetastatic and/or antiproliferative effects.
Thus, as mentioned above, kisspeptins stimulate KISS1R through a variety of signaling pathways. In different cell types, activation of KISS1R induces phosphorylation of different kinds of MAPK, which finally take part in hormone-releasing regulation, neuroendocrine function, antimetastatic and/or antiproliferative effects of kisspeptins (50).
3 The Function of Kisspeptin in HPG Axis
3.1 Kisspeptin Stimulates Gonadotrophin Release
In both animals and humans, kisspeptin is a powerful stimulator of the HPG axis (17, 60). In 2003, Seminara et al. first conducted studies to explore the relationship between kisspeptin and reproductive axis in mice (9). They found that injecting GnRH into kiss1r deficient mice improved their hypogonadotropic symptoms, suggesting that the effect of kisspeptin was mediated by stimulating GnRH release. In the following two years, several studies have reported the underlying promoting effect of kp-10 and kp-54 on LH secretion in rodents respectively (10, 61–63), providing preliminary evidence for the effects of kisspeptin in the process of GnRH release. More details have been found gradually since 2005. Neuroanatomical studies have confirmed that a large number of GnRH neurons were distributed in POA, and more than 90 percent of GnRH neurons express Kiss1R in mice (43). Projections from kisspeptin neurons to GnRH neurons are different between species (15). In mice, AVPV kisspeptin neurons fibers project directly to GnRH cell bodies, while ARC kisspeptin neurons are primarily apposed to GnRH processes which extend to the median eminence (15). In human, kisspeptin neurons in the hypothalamus project fibers into POA, stimulating GnRH secretion which later acts on the pituitary and leads to LH and FSH release (64, 65). Experiments have confirmed that central and peripheral addition of exogenous kisspeptin stimulates this reproductive cascade in both human and animals (63, 66). Additionally, the use of a GnRH antagonist blocks the promoting effect of kisspeptin (10), demonstrating that kisspeptin sits at the top of the HPG axis. In recent years, the application of modern methods confirmed the role of kisspeptin more directly. Using an optogenetic approach, scientists have found that the synchronous activation of ARC kisspeptin neurons in vivo resulted in pulsatile LH release in rodents in a sex steroid-dependent manner (67, 68). By transfecting Kiss1 to Kiss1 knockout rats, Nagae et al. demonstrated the ARC KNDy neurons were the generator of GnRH pulse and the presence of more than 20% of ARC KNDy neurons was able to rescue reproductive function (69).
Furthermore, it is important to notice that the production and secretion of FSH and LH are affected by different GnRH pulse frequencies and amplitudes, which is associated with various signaling pathways. Low frequency of GnRH pulses is conducive to FSH secretion, while high frequency of GnRH pulses tends to cause the release of LH. In detail, high GnRH pulse frequency can activate the PKC/MAPK and Calcium/Calmodulin-dependent kinase II (CaMK II) pathways and stimulate early growth response-1 protein (Egr1) expression largely, thereby increasing Lhb transcription and producing more LH. In contrast, low frequency of GnRH pulses helps recruit the histone acetyltransferase CREB-binding protein (CBP) to the promoter, which stimulates Fshb transcription (70). At present, LH is as an alternative marker for the effect of kisspeptins on GnRH secretion. However, few studies have researched the role of kisspeptin in regulating FSH secretion, which may be attributed to the difficulty in accurate continued measurement of serum FSH concentration. Taken together, the studies above indicate that kisspeptin acts as a gatekeeper in the HPG axis, controlling the onset of HPG axis by stimulating GnRH secretion, and is related to LH pulsatile secretion (70).
3.2 Kisspeptin Mediates Negative and Positive Sex Steroids Feedback
Apart from the stimulation of kisspeptin on GnRH, some studies also explored the expression of Kiss1 mRNA in ARC and RP3V respectively when exposed to sex steroids (71). The regulation in these regions were found to be different, as estradiol stimulates RP3V kisspeptin neurons while inhibits ARC kisspeptin neurons. In addition, scientists found that during LH surge, the level of KISS1 mRNA was raised in RP3V but was reduced in ARC (72). These studies initially revealed that negative feedback is mediated by ARC kisspeptin neurons, while positive feedback is modulated by kisspeptin neurons in RP3V. The detailed mechanism has been deeply studied in the last two decades. According to the model built by scientists, NKB acts on KNDy neurons to cause pulsatility and Dyn acts as the ‘brake’ halting pulses and kisspeptin is the final output to GnRH neurons (Figure 2). NKB acts in an autocrine/paracrine manner, inducing an inward current to increase the membrane potential of KNDy neurons, thus increasing the firing frequency of KNDy neurons and causing increased Ca2+ oscillations which finally promote the secretion of kisspeptin (73). The generation and termination of GnRH pulses are closely related to the change in the balance between stimulation (NKB) and inhibition (Dyn) tones, namely the ratio of NKB to Dyn (73). The model has been supported by mammal experiments and human studies. For example, ovariectomy increased NKB expression in ARC of monkeys, while estrogen treatment reduced it (74, 75). Post-menopausal women were found to have higher expression of Kiss1 mRNA (32). The hypertrophied neurons in their infundibular nuclei expressed both ESR1 (encoding ERα) and NKB mRNA, showing a similar distribution to that of kisspeptin neurons (76, 77). Also, prodynorphin mRNA expression in the ARC decreased, showing the mediating role of dynorphin in sex steroid negative feedback. In general, humans KNDy neurons may mediate negative sex steroid feedback in the infundibular nucleus by inhibiting the secretion of kisspeptin and NKB and stimulating the secretion of Dyn, causing reduced activity of the GnRH neuronal system, thus affects the secretion of GnRH (18). In recent years the application of optogenetics, GCaMP fiber photometry and mathematical models have enabled scientists to observe direct activities of neurons, perform long-term recordings and understand their complex dynamic behaviors (78–80). High-frequency photo stimulation of ARC kisspeptin neurons induced release of NKB and Dyn (80). In a latest research, Moore et al. observed KNDy neurons activity at a single cell level through in vivo calcium imaging and found the activation of KNDy neurons was in a temporal order (81). According to their study, some subsets of KNDy cells act as “leaders” and others serve as “followers” during each synchronized episode. These results offer more direct evidence on the model.
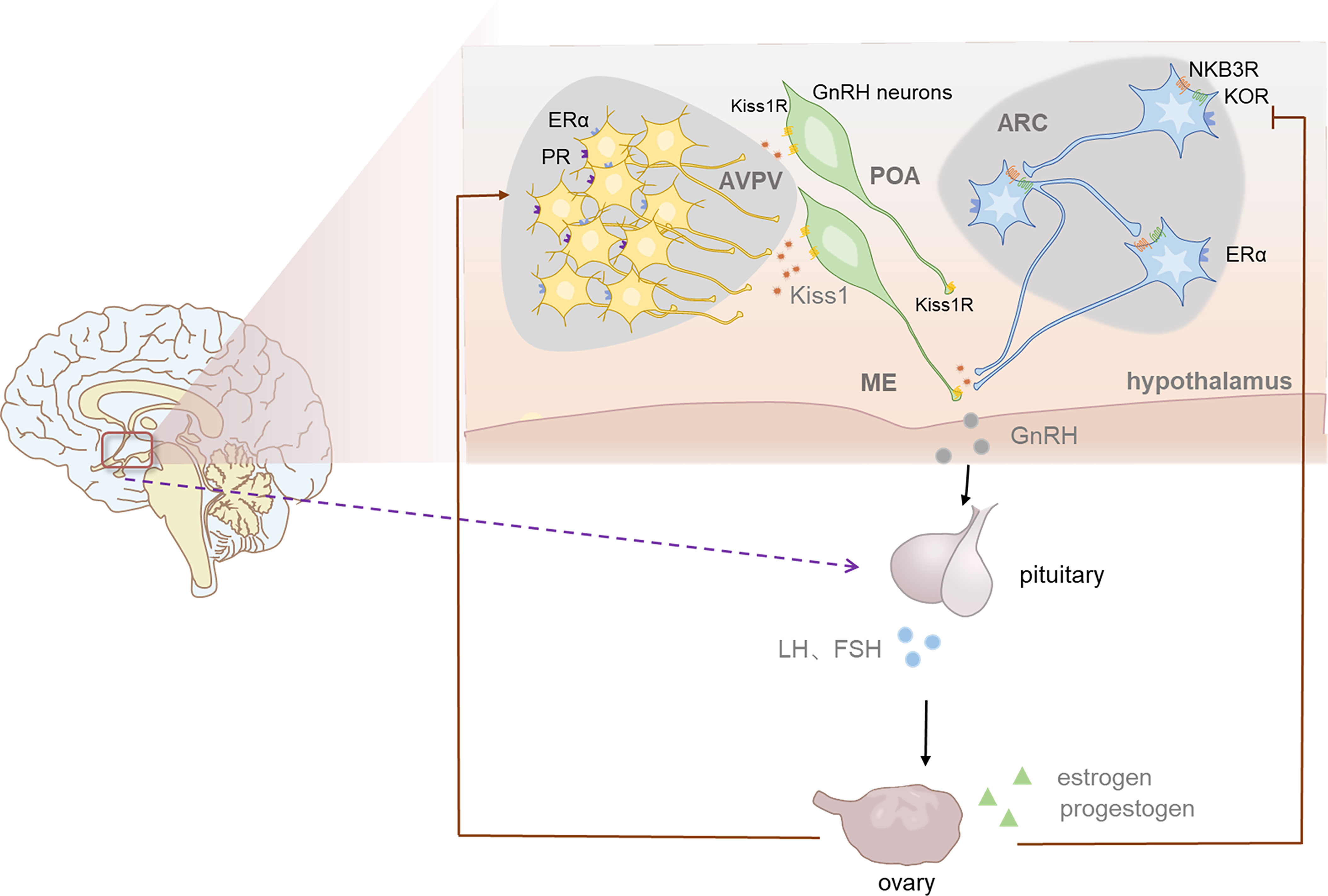
Figure 2 The role of kisspeptin in HPG axis and the positive and negative feedback of sex steoids in female. The population of kisspeptin neurons are located in anteroventral periventricular nucleus (AVPV) and the arcuate nucleus (ARC) in rodents. ARC kisspeptin neurons which coexpress NKB and Dyn are named KNDy neurons. Acting in an autocrine/paracrine manner, NKB stimulates kisspeptin secretion while Dyn inhibit it, thus regulate the release of GnRH indirectly. In this way KNDy neurons mediate negative feedback of estrogen and enables the generation and termination of GnRH pulse. AVPV NEURONS regulate the generation of GnRH sulge, thus induce LH surge, which is more significant in female ovulation. Progesterone also plays an indispensable role in the generation of GnRH surge.
Additionally, ARC kisspeptin neurons are important targets for progesterone feedback since progesterone receptor is expressed in almost all ARC kisspeptin neurons, while only a few is expressed in GnRH neurons (82, 83). As a neurosteriod, the level of progesterone dramatically increased after ovulation, which plays a role in inhibiting LH secretion. A study conducted in ewes has shown that the use of progesterone receptor antagonist in ARC kisspeptin neurons blocked the inhibiting effect of progesterone on LH pulse while the same effects did not occur when applying antagonist in POA (84). However, there are some differences in this model between different species. For example, the importance of NKB appears to be different between mice and human. NKB receptor-deficient mice show the same reproductive ability as wild type mice while human with NKB/NKB receptor mutations are reproductive incompetence (85–88). Moreover, although KNDy neurons express both estrogen and progesterone receptors, it seems that Dyn only mediates part of estrogen negative feedback in rodents, also (13, 89).
By contrast, AVPV kisspeptin neurons are more related to the positive feedback of estrogen. Estrogen feedback changes from negative to positive in the end of follicular phase to mediate the LH peak before ovulation. Emerging data suggest that AVPV kisspeptin neurons are more site and species specific and more sexually dimorphic (18). More than 70% AVPV kisspeptin neurons are ERα positive and express cFos, an immediate early gene whose expression represents increased neuronal activity during the LH surge (15, 33, 83, 90). A research found that in adult female mice more than one-third of AVPV kisspeptin neurons connect to GnRH cell bodies and nearly all of them express ERα (91). During the LH surge, AVPV kisspeptin neurons were observed higher level of cFos expression, which is an indication of increased activity of AVPV kisspeptin neurons (92). Experiments also confirmed that AVPV kisspeptin neurons are more active during estrogen positive feedback than negative feedback, as these neurons firing more action potentials and present stronger rapid action potential bursts on proestrus compared to other periods (93, 94). From these results collectively, preliminary conclusion could be drew that estrogen regulates AVPV kisspeptin neurons positively and increasing their activity, thus releasing GnRH stimulator kisspeptin and other cotransmitters, which induce the GnRH/LH surge. Besides, It is now established that progesterone receptors (PR), especially those expressed on kisspeptin neurons, are essential for positive feedback induced LH surge, since female mice lacking progesterone receptors exclusively in kisspeptin cells did not yield obvious LH surges when exposed to an estradiol-positive feedback paradigm (95–97). AVPV kisspeptin neurons coexpress PR, suggesting that they are targets for progesterone (96). A recent study found that selective reintroduction of PR into AVPV kisspeptin neurons, with other kisspeptin cells remaining PR absent, could rescue the LH surge in OVX (ovariectomized) + E2 females, indicating that PR in AVPV Kisspeptin neurons are enough for positive feedback induction of the LH surge (97).
Interestingly, despite ERα signaling in AVPV kisspeptin neurons is crucial for positive feedback, ERα signaling is not a necessary condition for negative feedback in ARC kisspeptin neurons, because mice with kisspeptin cells specifically lack ERα still retain negative feedback after estradiol therapy (98). This indicates that there is a redundant negative feedback path independent of kisspeptin signaling. In male, the number of AVPV kisspeptin neurons is very small (90). This sex-specific difference is determined by gonadal hormones during early neonatal development, in which neonatal testicular androgen leads to the decrease of AVPV kisspeptin expression (99). In male, ERα and androgen receptor (AR) are both expressed in most kisspeptin neurons of the two main kisspeptin neuronal populations (100). Compared with females, the detailed signaling mechanisms that mediate the testosterone feedback seem to have some differences between ARC and AVPV kisspeptin neurons. In AVPV, the effects of testosterone seem to be mediated by ERα or ERβ, since estradiol treatment is able to fully mimic the effect of testosterone (100, 101). In contrast to AVPV, ARC kisspeptin neurons do not show notable degree of sexual dimorphism (102). In conclusion, only the pulsatile LH release is evident in male mammals, and androgen negatively feedbacks to regulate the GnRH/LH pulse in order to maintain sustained spermatogenesis and steroidogenesis. The function of kisspeptin in the male AVPV is still unclear at present.
4 The Role of Kisspeptin in Reproduction
4.1 Kisspeptin Regulates Pubertal Onset
Puberty is described as the development of secondary sexual characteristics, the maturation of gonads, and the acquisition of reproductive competence (103). The process of puberty is complex and associated with thousands of genes (5). During the onset of puberty, the pulsatile release of GnRH from the hypothalamus plays a major role, which is the result of a couple of excitatory and inhibitory factors. For example, the excitatory factors include catecholamines and glutamate while the inhibitory factors include gamma-aminobutyric acid (GABA) and Opioid peptide (103). Among these excitatory factors, it is well known that the kisspeptin occupies an indispensable position. The KISS1 mRNA expression increases significantly when people transit from juvenile to adult in AVPV although the expression of KISS1R mRNA does not have a detectable change in mice (43). The underlying mechanism involves reduction of EED and Cbx7, dissociation of Polycomb Group (PcG) and recruitment of trithorax Group (TrxG) elements at the Kiss1 promoter, which finally increases Kiss1 transcription (104, 105). In females, the late development of the POA kisspeptin neuron projection to the GnRH neuron cell body in the pubertal period leads to the generation of the GnRH surge, thus resulting in the LH surge and the first ovulation (5).
In 2003, two independent studies reported patients with KISS1R gene inactivation or deletion mutation suffered familial or sporadic forms of idiopathic (or isolated) hypogonadotropic hypogonadism (iHH), which manifested as sexual immaturity and reproductive inability due to the defection of GnRH secretion (9, 106). The phenotype of human KISS1R mutation is also present in kiss1r knockout mice and rats (9, 107). By contrast, mutations which cause KISS1R hyperactive in humans result in central precocious puberty (CPP). In 2008, a mutation (Arg386Pro) in the kisspeptin receptor gene which results in extended activation of intracellular signaling pathways when responding to kisspeptin was identified in a girl with idiopathic CPP (108). Many studies have confirmed that girls with CPP had higher serum kisspeptin levels than normal controls (109–111). It suggested that the mutant may increase the promoting effects of kisspeptin on GnRH release, thereby speeding up the HPG axis maturation. Another mutation, p.P74S, discovered in a boy with CPP in 2010, has been confirmed to cause kisspeptin more resistant to degradation, suggesting an increased availability of bioactive kisspeptin as another mechanism of precocious puberty (112). In another study, the first compound with the ability to block kisspeptin actions in vivo and in vitro, namely p234, has been used in pubertal female mammals for experiments. Scientists has demonstrated that the application of p234 in rats for 7 days preceding puberty resulted in delayed puberty onset (113). In contrast, the addition of exogenous kisspeptin led to earlier puberty in rats and monkeys (114, 115). These results imply that kisspeptin plays an integral role in the coordination of pubertal onset.
In summary, kisspeptin is more likely to act as an amplifier of a series of GnRH secretion events rather than the trigger of puberty onset (116). In fact, other partners of kisspeptin neurons such as Substance P, NKA, RFRP-3 and α-MSH also take part in the regulation of puberty timing (70). To be exact, the precise control of puberty onset depends on the interaction and coordination of a large neuronal network.
4.2 Kisspeptin and Female Reproduction
Female reproduction is deeply regulated by the hypothalamic–pituitary–ovarian (HPO) axis. The ovary is closely related to follicular maturation, ovulation, corpus luteum formation and secretion of the steroid hormones. The distribution of kisspeptin in the ovary has obvious temporal and spatial specificity, showing its closed relationship with ovarian functions. Being a critical regulator, kisspeptin modulates female reproduction in several aspects, including gonadotropin secretion, follicular development, oocyte maturation and ovulation (17, 44, 117).
4.2.1 The Role in Follicular Development
In human, kisspeptin levels increase from early follicular to preovulatory phase (118). In the process of follicular development, Fernandois et al. showed that kisspeptin affects the primary and secondary follicle recruitment through reducing the FSH receptor (FSHR) expression (119). In both 6- and 10-month-old rats, local administration of kisspeptin into the ovary reduced the number of total antral follicles (including atretic follicles) and the use of kisspeptin receptor antagonist p234 played the opposite role. In an in vitro experiment, kisspeptin prevents the increase in FSHR expression produced by ISO (a β-adrenergic agonist), acting as a functional antagonist. Besides, kisspeptin can upregulate the level of serum anti-Müllerian hormone (AMH), which is a vital dimeric glycoprotein in the regulation of follicle development. Produced by preantral and small antral follicles, AMH exerts its regulatory role though attenuating primordial follicle recruitment and changing the sensitivity of follicles to FSH (120, 121). The study found that serum AMH level increased after local administration of kisspeptin and decreased after the use of p234 in 6- and 10-month-old rats. To sum up, kisspeptin may negatively affect the development of preantral follicles by upregulating AMH and downregulating the expression of FSHR in the ovary.
4.2.2 The Role in Oocyte Maturation
It is well known that the preovulatory LH surge triggers the resumption of meiosis and the progression to metaphase II (MII) during each reproductive cycle (122). Besides, the direct effect of kisspeptin on oocyte maturation has been studied in porcine cumulus-oocyte complexes (COCs). Adding kisspeptin to porcine COCs in vitro promotes oocyte maturation, suggesting kisspeptin acts on oocytes directly (123). The mechanisms may include upregulating the expression of C-MOS, growth differentiation factor 9 (GDF 9) and bone morphogenetic protein 15 (BMP 15) (124). C-MOS plays a stimulating role in various processes during oocyte maturation, including the meiosis process, normal spindle and chromosome formation, and reactivation of purified maturation promoting factor after first meiosis. Also, GDF 9 and BMP 15 take part in regulating follicle development, oocyte maturation, ovulation, luteinization and other physiological processes (124–127).
It has been found that cumulus granulosa cells (GCs) play a vital role in regulating oocyte maturation. Chakravarthi et al. has observed a remarkable expression of kisspeptin in gonadotropin treated GCs while KISSR in oocytes, suggesting that GC-derived Kisspeptin may have a direct function on oocytes KISS1R to modulate oocyte maturation via a MAPK signaling pathway (45, 128, 129). The kisspeptin expressed in GCs is estrogen receptor β (ERβ) dependent since the expression of kisspeptin in GCs is absent in ERβ knockout rat ovaries. Consistent with the findings above, the administration of kisspeptin can increase the maturity of oocytes without cumulus cells in both wildtype and ERβnull rats (45). Therefore, kisspeptin may have a persistent and direct effect on oocytes in an autocrine and paracrine manner.
4.2.3 The Role in Ovulation
Ovulation is a complicated process described as the follicle rupture and oocyte release, which is mediated by the LH surge and is regulated by a series of specific genes (130). At the end of the follicular phase, high levels of estrogen act on AVPV kisspeptin neurons, promoting the release of kisspeptin which then cause the cascade of GnRH surge, LH peak and ovulation (25). The functions of LH peak are achieved by upregulating of COX-2 and producing prostaglandin (131). It has been confirmed that peripheral kisspeptin administration induces ovulation in many species such as rats (61) and ewes (132). In fact, the effect of kisspeptin on ovulation is mainly achieved by increasing the levels of LH and FSH. Subcutaneous administration of kisspeptin markedly elevated plasma FSH and LH levels in 25-day-old female rats (61). In human, the LH pulses increased immediately after an administration of kisspeptin-10 (118). Kisspeptin-54 induced ovulation in mice by stimulating precisely timed endogenous LH release of consistent amplitude and duration (133). Both the expression of ovarian Kiss1 mRNA and the ovulation efficiency in rats could be reduced by the administration of COX-2 inhibitor or COX non-selective inhibitor, indicating that the upregulation of COX-2 may act on the expression levels of kisspeptin to induce LH peak (19).
The role of ovarian kisspeptin in ovulation may not be indispensable because in Kiss1r knockout mice, standard gonadotropin priming could induce ovulation (9), indicating that the ovarian kisspeptin signaling is not nexessary for ovulation. However, although the oocyte quality between neuron-specific Kiss1 and Kiss1R knockout mice and wild type mice shows little difference, the knockout mice presented obviously fewer ovulated oocytes and corpora lutea. This suggests the GnRH plus gonadotropin stimulation is not sufficient to reverse the loss of function due to Kiss1r knockout (134).
4.2.4 The Role in Reproductive Behaviors
Lordosis is an important posture adopt by non-primate female mammals in response to male mounting, characterized by ventral arching of the spine and elevation of the hips to facilitate penile penetration (135). A study has shown that female Kiss1-knockout mice were unable to exhibit normal lordosis behavior despite estrogen and progesterone replacement (136), while kisspeptin 10 injection in Kiss−/− females robustly stimulated lordosis behavior. Optogenetic activation of RP3V kisspeptin neurons caused strong firing and provoked the sexual behavior of lordosis, showing that RP3V kisspeptin neurons are essential for lordosis (136). The effect of kisspeptin is closely associated with NO, which is potentially a key transmitter downstream of kisspeptin neurons. Furthermore, this study also found that kisspeptin neurons induce mate preferences in female mice driven by olfaction via GnRH neurons. Together, these observations illustrate the crucial role of kisspeptin in controlling the sexual behaviors in female mice. However, ovariectomized Kiss1r-knockout mice showed normal female sexual behaviors such as lordosis after hormone replaced (with estrogen and progesterone) treatment (137). Consistent with this result, lordosis behavior is also unaffected in an additional transgenic mouse model which completely lacks GnRH immunoactivity in adulthood. These surprising results implied that the kisspeptin receptor is not necessary for normal lordosis behavior in female mice while sufficient sex steroid replacement is. In other words, kisspeptin-mediated lordosis is not dependent on GnRH signaling (136). One reasonable hypothesis is that kisspeptin-mediated-lordosis is facilitated via NPFF receptors as both NPFFR1 and NPFFR2 have been found in brain areas which are important in female sexual behavior (136, 138). However, the hypothesis has not been confirmed by any research at present.
4.3 Kisspeptin and Male Reproduction
Spermatogenesis is a complex process in testis in which the spermatogonia develop into male gametes through the adjustment of mitotic, meiotic and differentiation events (20). Each component of testis and the overall function are controlled by hypothalamic-pituitary-testicular axis. As the gatekeeper in HPG axis, GnRH induces the production of sex steroid by Leydig cells and the processes of spermatogenesis through LH and FSH released by the pituitary. Many studies have demonstrated the distribution of kisspeptin and its receptor proteins in the testes in multiple species including non-mammalian vertebrates such as frogs, fish and mammals such as rodents, goats and monkeys (21, 139–141). The major producer of kisspeptin is considered to be Leydig cell since scientists have detected the substantial expression of Kiss1 mRNA or protein in Leydig cells in various mammalian and non-mammalian species such as mice, horses, goats and frogs (141–144). Besides, kisspeptins were also expressed in epididymis. Mele et al. observed the differential kisspeptin expression in caput and cauda rat epididymis, while it is still not clear whether epidermal kisspeptins assist with maturation of sperm (140). In mammals, gene expression profiling showed that the initiation of kisspeptin/kiss1r expression in mouse testis and the formation of spermatozoa happened at the same time, indicating there is a connection between spermatogenesis and testicular kisspeptin/kiss1r system (145). In general, testicular kisspeptin system may act in the regulation of Leydig cell activity, spermatogenesis, and sperm function.
As for steroidogenesis, it has been reported that testosterone levels decreased after the administration of different doses of kp-10 (25). Other studies reported that kp-10 dramatically increased total testosterone plasma levels in the acute phase while chronic administration was associated with a decrease. In adult Wistar rats, chronic subcutaneous administration of kp-54 (50 nmol/d) in long term (13 days)decreased testicular weight, induced seminiferous tubules to degenerate (146). Continuous subcutaneous injection of kp-54 led to testicular degeneration after only 12 hours, at the time when LH and FSH were still considerably increased (147). Based on the results, the reduction of testosterone was attributed to central hyper-stimulation of the HPG axis, which needs to be further confirmed. However, some studies got different results. For example, a study reported that neither acute nor chronic injection of 50 nmol/kg kisspeptin-10 failed to induce degeneration of rat seminal tubules (148). According to the difference in results above, it is difficult to draw some exact conclusions at present.
In the process of spermatogenesis, in vivo kisspeptin administration accelerates spermatogenesis until sperm production in fish (149). During the late stage of spermatogenesis, the administration of kp-13 regulated sperm motility and caused momentary sperm hyperactivation, with a slow raise of sperm intracellular Ca2+concentration in human, suggesting the effects of kisspeptin to the sperm is achieved via the increase of Ca2+ levels (150). The use of p234 blocked the above effects of kp-13, indicating that the role of kisspeptin in human spermatogenesis is direct. Similar results were also obtained in rodents (22).
In terms of reproductive behaviors, male mice with Kiss1r knocked out are unable to show normal male sexual behaviors, such as mount, thrust and ejaculation (137). However, the failure can be compensated by testosterone, although the proportion of male ejaculation in testosterone substituted kiss1r knockout mice was lower than that in the wild type (137). Recent studies have concentrated on the positive role of the amygdala (MeA) kisspeptin system in modulating sexual behaviors (151, 152). It is reported that KISS1 mRNA expression in male MePD (posterodorsal medial amygdala) is higher than that in female (153). After exposing to female urine, MeA kisspeptin neuronal activity in male mice showed a doubled increase with an accompanied LH surge, indicating the important role of the amygdala kisspeptin system in olfactory-reproductive pathways (154). Moreover, the results triggered by intra-MePD and intra-cerebral injection of kp-10 are not exactly the same, the intra-MePD injection can induce penile erection in addition to inducing LH release, revealing that the enhancement of erectile function is achieved through the direct action of kisspeptin on MePD (152). However, in a recent study, DREADD (designer receptor exclusively activated by designer drugs)-mediated activation of MePD kisspeptin neurons in male mice caused notable levels of sexual behaviors such as mounts, intromissions, and ejaculations in the presence and absence of DREADDs-induced activation of these kisspeptin neurons, suggesting the role of MePD kisspeptin neurons is limited (151, 155). Also, in humans, male patients with KISS1R mutations successfully became fertile after treated with exogenous hormonal (156). These interesting findings showed that kisspeptin receptors may be important but not essential for male mammalian spermatogenesis and certain reproductive behaviors (157). Since there is not much research on it, it awaits further delineation.
5 Kisspeptin and Reproductive Diseases
As kisspeptin is an upstream regulator of GnRH neurons, it is related to some diseases associated with impaired HPG axis. It is widely known that the mutation of KISS1R gene lead to abnormal puberty onset, manifesting with CPP or iHH. A survey in Korea has shown that girls with CPP had higher serum kisspeptin levels compared with same aged prepubertal controls (111). The relevant contents have been mentioned in part 3.1.
PCOS is a common female reproductive endocrine disease whose typical clinical manifestations include infertility, ovulatory dysfunction, hyperandrogenism and so on (44). PCOS women show elevated LH/FSH, which indicates the impaired feedback of GnRH pulses (158). Due to the role of kisspeptin in LH release, the kisspeptin level in PCOS patients is speculated to be higher. This hypothesis has been supported by a large number of studies in human, most of which showed that the serum kisspeptin level of patients with PCOS is significantly higher than that of healthy controls (159, 160). Also, the role of ovarian kisspeptin has been confirmed in a previous study. The administration of kisspeptin in woman who accepted in vitro fertilization was found to change gene expression in granulosa lutein cells remarkably, triggering a higher expression of FSH and LH receptor, and higher expression of genes associated with ovarian steroid synthesis and action (161). In animal models, the expression kisspeptin only increased in PCOS models with increased LH and normal weight, while in androgen induced models kisspeptin expression were significantly suppressed (158, 162). In general, kisspeptin affects LH secretion and steroidogenesis through central and periphery pathways in PCOS women, while it needs more surveys and studies in both human and animals to illustrate and confirm its detailed role.
Hyperprolactinemia is another reproductive endocrine disease characterized by high level of serum prolactin (PRL) and hypogonadotropic anovulation which leads to infertility (163). It has been reported that kisspeptin neurons are the targets of PRL, as PRL receptors are expressed in most kisspeptin neurons but few GnRH neurons (164). Ovariectomized rats which accepted exogenous PRL were observed to have higher expression of phosphorylated signal transducer and activator of transcription 5 (pSTAT5) and reduced kisspeptin expression in ARC kisspeptin neurons, suggesting the direct effect of PRL on ARC kisspeptin neurons (163, 165). It seems that PRL acts on ARC kisspeptin neurons and results in reduction of GnRH levels, thereby leads to the decrease of LH levels and ovulation inhibition.
Currently kisspeptin is related to various reproductive diseases although the detailed role remains to be explored. Understanding the role of kisspeptin in the occurrence of these reproductive endocrine diseases will provide a theoretical basis for the treatment of these diseases in the future.
6 Potential Roles of Kisspeptin in Clinical Application
In recent years, many attempts have been made to explore the possibility of kisspeptin as a new diagnostic marker or therapeutic option. In humans, the plasma level of kisspeptin (kisspeptin-54) increases dramatically throughout pregnancy, making it possible to detect early pregnancy by measuring plasma kisspeptin concentrations (166). Also, because kisspeptin is produced by trophoblasts and trophoblast invasion is underway 5 days after blastocyst transplantation, plasma kisspeptin concentrations during the peri-implantation period may reflect the early developmental events associated with pregnancy outcome (167). In a comparative study, Sullivan-Pyke et al. measured serum kisspeptin in 20 women with 6-10 weeks of intrauterine pregnancy (IUP) and 20 women who suffered spontaneous abortion (SAB) at a similar time. They found the median serum kisspeptin levels were significantly higher in IUP women (1.50ng/ml) than in SAB women (0.20ng/ml), indicating that kisspeptin is detectable in serum in early pregnancy and can discriminate SAB from IUP (168). Also, as mentioned above, girls with CPP had higher serum kisspeptin levels compared with healthy girls (109–111). However, serum kisspeptin levels are not able to become a single diagnostic tool because the evident overlap limits its use, while it may still be useful as an adjunctive tool in the diagnosis of CPP.
As for therapeutic option, kisspeptin is found to have potential in stimulating oocyte maturation and inducing ovulation in infertile women. In 2014, a clinical study found that single administration of kisspeptin-54 induced female egg maturation in women who accepted in vitro fertilization (169), suggesting its potential application in treating women with infertility. Kisspeptin and its agonist are also be regard as potential therapeutic options of some reproductive diseases. In a clinical study, repeated administration of kp-54 successfully induced ovulation in two out of seven women with PCOS (170). Abbara et al. compared the therapeutic effect of nanopeptide KISS1R agonist MT-602 and kisspeptin 54 in PCOS women and found both MVT-602 and kp54 induced a LH peak with similar amplitude (171). Recent studies also reported the application of kisspeptin as a future therapeutic option in treatment of hyperprolactinemia. The administration of kisspeptin successfully caused LH pulses via stimulating GnRH in women with hyperprolactinemia (172). In another study, the use of kisspeptin induced recover of gonadotropin secretion and ovarian cyclicity (173). In addition, based on the fact that high dose of kisspeptin leads to desensitization of HPG axis, kisspeptin may be applied in the treatment of sex hormone-dependent malignancies. For example, prostate cancer is a kind of androgen dependent malignancy and the current primary treatment is androgen deprivation therapy (ADT) (174). Two animal studies have confirmed that chronic administration of the kisspeptin analog, TAK-448 caused stronger inhibiting effect of HPG axis than GnRH analog and obviously suppressed testosterone and LH release, indicating its great anti-tumor growth potential (175, 176).
7 Conclusion and Future Directions
The discovery of kisspeptin was a milestone in the field of reproductive biology. The last two decades have seen an explosion in kisspeptin literature, which elucidated of the pivotal role of kisspeptin in the control of HPG axis. Kisspeptin acts upstream of GnRH and thus regulates the secretion of LH and FSH, following paracrine promoting and inhibiting inputs from NKB and Dyn, signals directly to GnRH neurons to control pulsatile GnRH secretion, thus regulates the secretion of LH and FSH. Through the G-protein-coupled receptor, KISS1R, kisspeptin acts in the onset of puberty and maintenance of mammalian fertility. Although there is some difference between sexes in neuroanatomy and function, the functions of kisspeptin are apparent in both male and female. On the one hand, kisspeptin in hypothalamus acts on HPG axis, regulating reproduction processes through secretion of LH and FSH. On the other hand, periphery kisspeptin has a direct effect on the gonads in an autocrine-paracrine manner. In female, ovarian kisspeptin negatively regulate preantral follicular development and increase the maturity of oocyte. In male, kisspeptin are closely associated with spermatogenesis although the detailed role is not completely clear. Kisspeptin also regulates reproductive behaviors in both male and female including lordosis in female and mount, thrust or ejaculate in male (Figure 3).
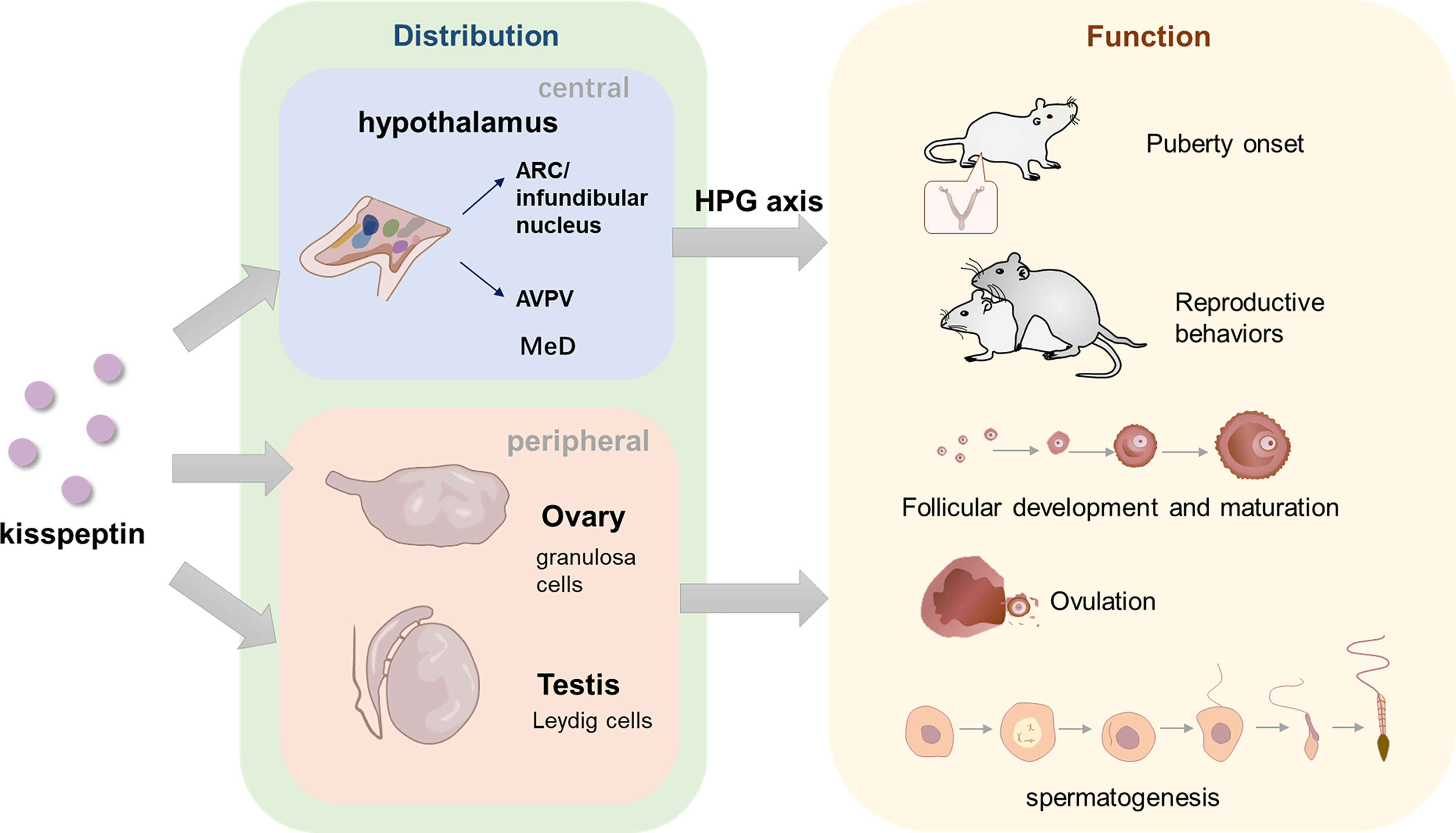
Figure 3 The distribution of kisspeptin in central and peripheral areas and its role in reproduction. In hypothalamus, kisspeptins are located in two main neuron populations, ARC/infundibular nucleus and AVPV. In the peripheral areas, kisspeptin are mainly discovered in ovary and testis. GCs are the main cell which product Ovarian-derived kisspeptin, while Leydig cells are considered to product testicular kisspeptin. Kisspeptins are also found in other organs such as pancreas, liver, placenta, etc. (not depicted in the figure). Kisspeptin in the hypothalamus functions through HPG axis, while gonads-derived kisspeptin might act directly in a autocrine-paracrine manner. In terms of the function, kisspeptin is likely to act as an amplifier of a series of GnRH secretion events, which are vital for normal puberty onset. Central and peripheral kisspeptins exert a role in various processes of female and male reproduction, maintaining the normal progress of follicular development, oocyte maturation, ovulation in female and spermatogenesis in male. In addition, kisspeptin is also essential in both male and female reproductive behaviors, regulating mount, ejaculation and thrust in male and lordosis in female.
The large number of experiments on mammals in recent years has significantly improved our understanding of the role of kisspeptins and related mechanisms. Modern approaches such as optogenetics, mathematical models and in vivo calcium imaging have made huge contribution to the solution of long-existing questions such as the mechanism of negative and positive feedback and the functions of kisspeptin/KISS1R system in female reproduction. However, there are still some questions which need further exploration. For instance, although many new advances have been made in male reproduction, the role of kisspeptin in steroidogenesis, sperm function and reproductive behaviors are not completely clear. The different results in experiments need further researches to explain. Recently the differential expression of kisspeptin receptor in epidermis was discovered, while its specific effect on sperm maturation is not confirmed yet. Besides, the potential effects of kisspeptin on testicular germ cells and somatic cells were studied by tissue and cell culture experiments, and its role in vivo is not fully understood (157). Also, most current studies were only conducted on animal models, thus more powerful evidence are needed to prove the relevance of these findings to human. In addition to the two main populations of kisspeptin neurons, a small number of kisspeptin neurons was also found in the limbic system of the brain (such as amygdala, the lateral septum, the dorsomedial and ventromedial hypothalamic nuclei, etc.). The function and precise lineage of these kisspeptin populations are also worthy of further exploration (70, 102). The development of new technologies will help bring new ideas about the unknown field.
Based on the essential effects of kisspeptin in reproductive axis summarized in this review, kisspeptin and its analogues may be used for diagnostic and therapeutic goals. This needs more data, more translational work and larger studies to expand our knowledge of kisspeptin. In conclusion, a complete understanding of the expression, function, and potential molecular mechanisms of kisspeptin/KISS1R in the HPG axis and its role in reproduction will bring new inspiration to the diagnosis, treatment and prevention of some reproductive diseases.
Author Contributions
QX, YK wrote the article and performed all of the necessary literature searches and data compilation. YX, CW, JL, CZ, CY performed the necessary literature searches and data compilation. HZ revised the article and give valuable suggestions. DH designed the review, reviewed it, and approved the submitted manuscript. All authors have read and approved the final manuscript.
Funding
This work was supported by the National Natural Science Foundation of China (NO. 81771575) and National Key Research & Developmental Program of China (2018YFC1003900).
Conflict of Interest
The authors declare that the research was conducted in the absence of any commercial or financial relationships that could be construed as a potential conflict of interest.
Publisher’s Note
All claims expressed in this article are solely those of the authors and do not necessarily represent those of their affiliated organizations, or those of the publisher, the editors and the reviewers. Any product that may be evaluated in this article, or claim that may be made by its manufacturer, is not guaranteed or endorsed by the publisher.
References
1. Maggi R, Cariboni AM, Marelli MM, Moretti RM, Andrè V, Marzagalli M, et al. GnRH and GnRH Receptors in the Pathophysiology of the Human Female Reproductive System. Hum Reprod Update. (2016) 22(3):358–81. doi: 10.1093/humupd/dmv059
2. Plant TM. The Hypothalamo-Pituitary-Gonadal Axis. J Endocrinol (2015) 226(2):T41–54. 60 Years Of Neuroendocrinology. doi: 10.1530/JOE-15-0113
3. Lopez-Rodriguez D, Franssen D, Bakker J, Lomniczi A, Parent AS. Cellular and Molecular Features of EDC Exposure: Consequences for the GnRH Network. Nat Rev Endocrinol (2021) 17(2):83–96. doi: 10.1038/s41574-020-00436-3
4. Kaprara A, Huhtaniemi IT. The Hypothalamus-Pituitary-Gonad Axis: Tales of Mice and Men. Metabolism: Clin Exp (2018) 86:3–17. doi: 10.1016/j.metabol.2017.11.018
5. Herbison AE. Control of Puberty Onset and Fertility by Gonadotropin-Releasing Hormone Neurons. Nat Rev Endocrinol (2016) 12(8):452–66. doi: 10.1038/nrendo.2016.70
6. Simonian SX, Spratt DP, Herbison AE. Identification and Characterization of Estrogen Receptor Alpha-Containing Neurons Projecting to the Vicinity of the Gonadotropin-Releasing Hormone Perikarya in the Rostral Preoptic Area of the Rat. J Comp Neurol (1999) 411(2):346–58. doi: 10.1002/(SICI)1096-9861(19990823)411:2<346::AID-CNE13>3.0.CO;2-S
7. Lee JH, Miele ME, Hicks DJ, Phillips KK, Trent JM, Weissman BE, et al. KiSS-1, a Novel Human Malignant Melanoma Metastasis-Suppressor Gene. J Natl Cancer Institute. (1996) 88(23):1731–7. doi: 10.1093/jnci/88.23.1731
8. Padda J, Khalid K, Moosa A, Syam M, Kakani V, Imdad U, et al. Role of Kisspeptin on Hypothalamic-Pituitary-Gonadal Pathology and Its Effect on Reproduction. Cureus (2021) 13(8):e17600. doi: 10.7759/cureus.17600
9. Seminara SB, Messager S, Chatzidaki EE, Thresher RR, Acierno JS Jr., Shagoury JK, et al. The GPR54 Gene as a Regulator of Puberty. New Engl J Med (2003) 349(17):1614–27. doi: 10.1056/NEJMoa035322
10. Gottsch ML, Cunningham MJ, Smith JT, Popa SM, Acohido BV, Crowley WF, et al. A Role for Kisspeptins in the Regulation of Gonadotropin Secretion in the Mouse. Endocrinology (2004) 145(9):4073–7. doi: 10.1210/en.2004-0431
11. Burke MC, Letts PA, Krajewski SJ, Rance NE. Coexpression of Dynorphin and Neurokinin B Immunoreactivity in the Rat Hypothalamus: Morphologic Evidence of Interrelated Function Within the Arcuate Nucleus. J Comp neurology. (2006) 498(5):712–26. doi: 10.1002/cne.21086
12. Goodman RL, Lehman MN, Smith JT, Coolen LM, de Oliveira CV, Jafarzadehshirazi MR, et al. Kisspeptin Neurons in the Arcuate Nucleus of the Ewe Express Both Dynorphin A and Neurokinin B. Endocrinology (2007) 148(12):5752–60. doi: 10.1210/en.2007-0961
13. Navarro VM, Gottsch ML, Chavkin C, Okamura H, Clifton DK, Steiner RA. Regulation of Gonadotropin-Releasing Hormone Secretion by Kisspeptin/Dynorphin/Neurokinin B Neurons in the Arcuate Nucleus of the Mouse. J Neurosci Off J Soc Neurosci (2009) 29(38):11859–66. doi: 10.1523/JNEUROSCI.1569-09.2009
14. Cheng G, Coolen LM, Padmanabhan V, Goodman RL, Lehman MN. The Kisspeptin/Neurokinin B/dynorphin (KNDy) Cell Population of the Arcuate Nucleus: Sex Differences and Effects of Prenatal Testosterone in Sheep. Endocrinology (2010) 151(1):301–11. doi: 10.1210/en.2009-0541
15. Wang L, Moenter SM. Differential Roles of Hypothalamic AVPV and Arcuate Kisspeptin Neurons in Estradiol Feedback Regulation of Female Reproduction. Neuroendocrinology (2020) 110(3-4):172–84. doi: 10.1159/000503006
16. Roseweir AK, Millar RP. The Role of Kisspeptin in the Control of Gonadotrophin Secretion. Hum Reprod Update (2009) 15(2):203–12. doi: 10.1093/humupd/dmn058
17. Pinilla L, Aguilar E, Dieguez C, Millar RP, Tena-Sempere M. Kisspeptins and Reproduction: Physiological Roles and Regulatory Mechanisms. Physiol Rev (2012) 92(3):1235–316. doi: 10.1152/physrev.00037.2010
18. Skorupskaite K, George JT, Anderson RA. The Kisspeptin-GnRH Pathway in Human Reproductive Health and Disease. Hum Reprod Update (2014) 20(4):485–500. doi: 10.1093/humupd/dmu009
19. Cao Y, Li Z, Jiang W, Ling Y, Kuang H. Reproductive Functions of Kisspeptin/KISS1R Systems in the Periphery. Reprod Biol Endocrinol RB&E. (2019) 17(1):65. doi: 10.1186/s12958-019-0511-x
20. Meccariello R, Fasano S, Pierantoni R. Kisspeptins, New Local Modulators of Male Reproduction: A Comparative Overview. Gen Comp Endocrinol. (2020) 299:113618. doi: 10.1016/j.ygcen.2020.113618
21. Tariq A, Shahab M, Clarke I, Pereira A, Smith J, S-u-H K, et al. Kiss1 and Kiss1 Receptor Expression in the Rhesus Monkey Testis: A Possible Local Regulator of Testicular Function. J Open Life Sci (2013) 8(10):968–74. doi: 10.2478/s11535-013-0219-4
22. Hsu MC, Wang JY, Lee YJ, Jong DS, Tsui KH, Chiu CH. Kisspeptin Modulates Fertilization Capacity of Mouse Spermatozoa. Reproduction (2014) 147(6):835–45. doi: 10.1530/REP-13-0368
23. Selvaraj S, Ohga H, Nyuji M, Kitano H, Nagano N, Yamaguchi A, et al. Subcutaneous Administration of Kiss1 Pentadecapeptide Accelerates Spermatogenesis in Prepubertal Male Chub Mackerel (Scomber Japonicus). Comp Biochem Physiol Part A Mol Integr Physiol (2013) 166(2):228–36. doi: 10.1016/j.cbpa.2013.06.007
24. West A, Vojta PJ, Welch DR, Weissman BE. Chromosome Localization and Genomic Structure of the KiSS-1 Metastasis Suppressor Gene (Kiss1). Genomics (1998) 54(1):145–8. doi: 10.1006/geno.1998.5566
25. Zeydabadi Nejad S, Ramezani Tehrani F, Zadeh-Vakili A. The Role of Kisspeptin in Female Reproduction. Int J Endocrinol Metab (2017) 15(3):e44337. doi: 10.5812/ijem.44337
26. Li D, Yu W, Liu M. Regulation of KiSS1 Gene Expression. Peptides (2009) 30(1):130–8. doi: 10.1016/j.peptides.2008.09.025
27. Kotani M, Detheux M, Vandenbogaerde A, Communi D, Vanderwinden JM, Le Poul E, et al. The Metastasis Suppressor Gene KiSS-1 Encodes Kisspeptins, the Natural Ligands of the Orphan G Protein-Coupled Receptor Gpr54. J Biol Chem (2001) 276(37):34631–6. doi: 10.1074/jbc.M104847200
28. Bilban M, Ghaffari-Tabrizi N, Hintermann E, Bauer S, Molzer S, Zoratti C, et al. Kisspeptin-10, a KiSS-1/Metastin-Derived Decapeptide, is a Physiological Invasion Inhibitor of Primary Human Trophoblasts. J Cell Sci (2004) 117(Pt 8):1319–28. doi: 10.1242/jcs.00971
29. Clarkson J, d'Anglemont de Tassigny X, Colledge WH, Caraty A, Herbison AE. Distribution of Kisspeptin Neurones in the Adult Female Mouse Brain. J Neuroendocrinol (2009) 21(8):673–82. doi: 10.1111/j.1365-2826.2009.01892.x
30. Mikkelsen JD, Simonneaux V. The Neuroanatomy of the Kisspeptin System in the Mammalian Brain. Peptides (2009) 30(1):26–33. doi: 10.1016/j.peptides.2008.09.004
31. de Croft S, Piet R, Mayer C, Mai O, Boehm U, Herbison AE. Spontaneous Kisspeptin Neuron Firing in the Adult Mouse Reveals Marked Sex and Brain Region Differences But No Support for a Direct Role in Negative Feedback. Endocrinology (2012) 153(11):5384–93. doi: 10.1210/en.2012-1616
32. Rometo AM, Krajewski SJ, Voytko ML, Rance NE. Hypertrophy and Increased Kisspeptin Gene Expression in the Hypothalamic Infundibular Nucleus of Postmenopausal Women and Ovariectomized Monkeys. J Clin Endocrinol Metab (2007) 92(7):2744–50. doi: 10.1210/jc.2007-0553
33. Clarkson J, Herbison AE. Postnatal Development of Kisspeptin Neurons in Mouse Hypothalamus; Sexual Dimorphism and Projections to Gonadotropin-Releasing Hormone Neurons. Endocrinology (2006) 147(12):5817–25. doi: 10.1210/en.2006-0787
34. Hrabovszky E, Ciofi P, Vida B, Horvath MC, Keller E, Caraty A, et al. The Kisspeptin System of the Human Hypothalamus: Sexual Dimorphism and Relationship With Gonadotropin-Releasing Hormone and Neurokinin B Neurons. Eur J Neurosci (2010) 31(11):1984–98. doi: 10.1111/j.1460-9568.2010.07239.x
35. Muir AI, Chamberlain L, Elshourbagy NA, Michalovich D, Moore DJ, Calamari A, et al. AXOR12, a Novel Human G Protein-Coupled Receptor, Activated by the Peptide KiSS-1. J Biol Chem (2001) 276(31):28969–75. doi: 10.1074/jbc.M102743200
36. Ohtaki T, Shintani Y, Honda S, Matsumoto H, Hori A, Kanehashi K, et al. Metastasis Suppressor Gene KiSS-1 Encodes Peptide Ligand of a G-Protein-Coupled Receptor. Nature (2001) 411(6837):613–7. doi: 10.1038/35079135
37. Gaytán F, Gaytán M, Castellano JM, Romero M, Roa J, Aparicio B, et al. KiSS-1 in the Mammalian Ovary: Distribution of Kisspeptin in Human and Marmoset and Alterations in KiSS-1 mRNA Levels in a Rat Model of Ovulatory Dysfunction. Am J Physiol Endocrinol Metab (2009) 296(3):E520–31. doi: 10.1152/ajpendo.90895.2008
38. Ricu MA, Ramirez VD, Paredes AH, Lara HE. Evidence for a Celiac Ganglion-Ovarian Kisspeptin Neural Network in the Rat: Intraovarian Anti-Kisspeptin Delays Vaginal Opening and Alters Estrous Cyclicity. Endocrinology (2012) 153(10):4966–77. doi: 10.1210/en.2012-1279
39. García-Ortega J, Pinto FM, Fernández-Sánchez M, Prados N, Cejudo-Román A, Almeida TA, et al. Expression of Neurokinin B/NK3 Receptor and Kisspeptin/KISS1 Receptor in Human Granulosa Cells. Hum Reprod (Oxford England) (2014) 29(12):2736–46. doi: 10.1093/humrep/deu247
40. Lee DK, Nguyen T, O'Neill GP, Cheng R, Liu Y, Howard AD, et al. Discovery of a Receptor Related to the Galanin Receptors. FEBS Lett (1999) 446(1):103–7. doi: 10.1016/S0014-5793(99)00009-5
41. Kirby HR, Maguire JJ, Colledge WH, Davenport AP. International Union of Basic and Clinical Pharmacology. LXXVII. Kisspeptin Receptor Nomenclature, Distribution, and Function. Pharmacol Rev (2010) 62(4):565–78. doi: 10.1124/pr.110.002774
42. Herbison AE, de Tassigny X, Doran J, Colledge WH. Distribution and Postnatal Development of Gpr54 Gene Expression in Mouse Brain and Gonadotropin-Releasing Hormone Neurons. Endocrinology (2010) 151(1):312–21. doi: 10.1210/en.2009-0552
43. Han SK, Gottsch ML, Lee KJ, Popa SM, Smith JT, Jakawich SK, et al. Activation of Gonadotropin-Releasing Hormone Neurons by Kisspeptin as a Neuroendocrine Switch for the Onset of Puberty. J Neurosci Off J Soc Neurosci (2005) 25(49):11349–56. doi: 10.1523/JNEUROSCI.3328-05.2005
44. Hu KL, Zhao H, Chang HM, Yu Y, Qiao J. Kisspeptin/Kisspeptin Receptor System in the Ovary. Front Endocrinol (2017) 8:365. doi: 10.3389/fendo.2017.00365
45. Chakravarthi VP, Ghosh S, Housami SM, Wang H, Roby KF, Wolfe MW, et al. Erβ Regulated Ovarian Kisspeptin Plays an Important Role in Oocyte Maturation. Mol Cell Endocrinol (2021) 527:111208. doi: 10.1016/j.mce.2021.111208
46. Wu S, Zhang H, Tian J, Liu L, Dong Y, Mao T. Expression of Kisspeptin/GPR54 and PIBF/PR in the First Trimester Trophoblast and Decidua of Women With Recurrent Spontaneous Abortion. Pathology Res Practice (2014) 210(1):47–54. doi: 10.1016/j.prp.2013.09.017
47. Baba T, Kang HS, Hosoe Y, Kharma B, Abiko K, Matsumura N, et al. Menstrual Cyclic Change of Metastin/GPR54 in Endometrium. Med Mol Morphol. (2015) 48(2):76–84. doi: 10.1007/s00795-014-0081-0
48. Babwah AV. The Wonderful and Masterful G Protein-Coupled Receptor (GPCR): A Focus on Signaling Mechanisms and the Neuroendocrine Control of Fertility. Mol Cell Endocrinol. (2020) 515:110886. doi: 10.1016/j.mce.2020.110886
49. d'Anglemont de Tassigny X, Colledge WH. The Role of Kisspeptin Signaling in Reproduction. Physiol (Bethesda Md) (2010) 25(4):207–17. doi: 10.1152/physiol.00009.2010
50. Castaño JP, Martínez-Fuentes AJ, Gutiérrez-Pascual E, Vaudry H, Tena-Sempere M, Malagón MM. Intracellular Signaling Pathways Activated by Kisspeptins Through GPR54: Do Multiple Signals Underlie Function Diversity? Peptides (2009) 30(1):10–5. doi: 10.1016/j.peptides.2008.07.025
51. Peng J, Tang M, Zhang BP, Zhang P, Zhong T, Zong T, et al. Kisspeptin Stimulates Progesterone Secretion via the Erk1/2 Mitogen-Activated Protein Kinase Signaling Pathway in Rat Luteal Cells. Fertility Steril (2013) 99(5):1436–43.e1. doi: 10.1016/j.fertnstert.2012.12.008
52. Ringel MD, Hardy E, Bernet VJ, Burch HB, Schuppert F, Burman KD, et al. Metastin Receptor Is Overexpressed in Papillary Thyroid Cancer and Activates MAP Kinase in Thyroid Cancer Cells. J Clin Endocrinol Metab (2002) 87(5):2399. doi: 10.1210/jcem.87.5.8626
53. Yan C, Wang H, Boyd DD. KiSS-1 Represses 92-kDa Type IV Collagenase Expression by Down-Regulating NF-Kappa B Binding to the Promoter as a Consequence of Ikappa Balpha -Induced Block of P65/P50 Nuclear Translocation. J Biol Chem (2001) 276(2):1164–72. doi: 10.1074/jbc.M008681200
54. Liu X, Lee K, Herbison AE. Kisspeptin Excites Gonadotropin-Releasing Hormone Neurons Through a Phospholipase C/calcium-Dependent Pathway Regulating Multiple Ion Channels. Endocrinology (2008) 149(9):4605–14. doi: 10.1210/en.2008-0321
55. Pampillo M, Camuso N, Taylor JE, Szereszewski JM, Ahow MR, Zajac M, et al. Regulation of GPR54 Signaling by GRK2 and {Beta}-Arrestin. Mol Endocrinol (Baltimore Md). (2009) 23(12):2060–74. doi: 10.1210/me.2009-0013
56. Szereszewski JM, Pampillo M, Ahow MR, Offermanns S, Bhattacharya M, Babwah AV. GPR54 Regulates ERK1/2 Activity and Hypothalamic Gene Expression in a Gα(Q/11) and β-Arrestin-Dependent Manner. PloS One (2010) 5(9):e12964. doi: 10.1371/journal.pone.0012964
57. Ahow M, Min L, Pampillo M, Nash C, Wen J, Soltis K, et al. KISS1R Signals Independently of Gαq/11 and Triggers LH Secretion via the β-Arrestin Pathway in the Male Mouse. Endocrinology (2014) 155(11):4433–46. doi: 10.1210/en.2014-1304
58. Paradis JS, Ly S, Blondel-Tepaz É, Galan JA, Beautrait A, Scott MG, et al. Receptor Sequestration in Response to β-Arrestin-2 Phosphorylation by ERK1/2 Governs Steady-State Levels of GPCR Cell-Surface Expression. Proc Natl Acad Sci USA (2015) 112(37):E5160–8. doi: 10.1073/pnas.1508836112
59. Cassier E, Gallay N, Bourquard T, Claeysen S, Bockaert J, Crépieux P, et al. Phosphorylation of β-Arrestin2 at Thr(383) by MEK Underlies β-Arrestin-Dependent Activation of Erk1/2 by GPCRs. Elife (2017) 6:e23777. doi: 10.7554/eLife.23777
60. Dhillo WS, Chaudhri OB, Thompson EL, Murphy KG, Patterson M, Ramachandran R, et al. Kisspeptin-54 Stimulates Gonadotropin Release Most Potently During the Preovulatory Phase of the Menstrual Cycle in Women. J Clin Endocrinol Metab (2007) 92(10):3958–66. doi: 10.1210/jc.2007-1116
61. Matsui H, Takatsu Y, Kumano S, Matsumoto H, Ohtaki T. Peripheral Administration of Metastin Induces Marked Gonadotropin Release and Ovulation in the Rat. Biochem Biophys Res Commun (2004) 320(2):383–8. doi: 10.1016/j.bbrc.2004.05.185
62. Navarro VM, Castellano JM, Fernández-Fernández R, Barreiro ML, Roa J, Sanchez-Criado JE, et al. Developmental and Hormonally Regulated Messenger Ribonucleic Acid Expression of KiSS-1 and its Putative Receptor, GPR54, in Rat Hypothalamus and Potent Luteinizing Hormone-Releasing Activity of KiSS-1 Peptide. Endocrinology (2004) 145(10):4565–74. doi: 10.1210/en.2004-0413
63. Thompson EL, Patterson M, Murphy KG, Smith KL, Dhillo WS, Todd JF, et al. Central and Peripheral Administration of Kisspeptin-10 Stimulates the Hypothalamic-Pituitary-Gonadal Axis. J Neuroendocrinol (2004) 16(10):850–8. doi: 10.1111/j.1365-2826.2004.01240.x
64. Messager S, Chatzidaki EE, Ma D, Hendrick AG, Zahn D, Dixon J, et al. Kisspeptin Directly Stimulates Gonadotropin-Releasing Hormone Release via G Protein-Coupled Receptor 54. Proc Natl Acad Sci USA (2005) 102(5):1761–6. doi: 10.1073/pnas.0409330102
65. Hrabovszky E. Neuroanatomy of the Human Hypothalamic Kisspeptin System. Neuroendocrinology (2014) 99(1):33–48. doi: 10.1159/000356903
66. Dhillo WS, Chaudhri OB, Patterson M, Thompson EL, Murphy KG, Badman MK, et al. Kisspeptin-54 Stimulates the Hypothalamic-Pituitary Gonadal Axis in Human Males. J Clin Endocrinol Metab (2005) 90(12):6609–15. doi: 10.1210/jc.2005-1468
67. Han SY, McLennan T, Czieselsky K, Herbison AE. Selective Optogenetic Activation of Arcuate Kisspeptin Neurons Generates Pulsatile Luteinizing Hormone Secretion. Proc Natl Acad Sci USA (2015) 112(42):13109–14. doi: 10.1073/pnas.1512243112
68. Lin XH, Lass G, Kong LS, Wang H, Li XF, Huang HF, et al. Optogenetic Activation of Arcuate Kisspeptin Neurons Generates a Luteinizing Hormone Surge-Like Secretion in an Estradiol-Dependent Manner. Front Endocrinol (2021) 12:775233. doi: 10.3389/fendo.2021.775233
69. Nagae M, Uenoyama Y, Okamoto S, Tsuchida H, Ikegami K, Goto T, et al. Direct Evidence That KNDy Neurons Maintain Gonadotropin Pulses and Folliculogenesis as the GnRH Pulse Generator. Proc Natl Acad Sci USA (2021) 118(5):e2009156118. doi: 10.1073/pnas.2009156118
70. Sobrino V, Avendaño MS, Perdices-López C, Jimenez-Puyer M, Tena-Sempere M. Kisspeptins and the Neuroendocrine Control of Reproduction: Recent Progress and New Frontiers in Kisspeptin Research. Front Neuroendocrinol (2022) 65:100977. doi: 10.1016/j.yfrne.2021.100977
71. Smith JT, Cunningham MJ, Rissman EF, Clifton DK, Steiner RA. Regulation of Kiss1 Gene Expression in the Brain of the Female Mouse. Endocrinology (2005) 146(9):3686–92. doi: 10.1210/en.2005-0488
72. Smith JT, Popa SM, Clifton DK, Hoffman GE, Steiner RA. Kiss1 Neurons in the Forebrain as Central Processors for Generating the Preovulatory Luteinizing Hormone Surge. J Neurosci Off J Soc Neurosci (2006) 26(25):6687–94. doi: 10.1523/JNEUROSCI.1618-06.2006
73. Ikegami K, Watanabe Y, Nakamura S, Goto T, Inoue N, Uenoyama Y, et al. Cellular and Molecular Mechanisms Regulating the KNDy Neuronal Activities to Generate and Modulate GnRH Pulse in Mammals. Front Neuroendocrinol (2022) 64:100968. doi: 10.1016/j.yfrne.2021.100968
74. Abel TW, Voytko ML, Rance NE. The Effects of Hormone Replacement Therapy on Hypothalamic Neuropeptide Gene Expression in a Primate Model of Menopause. J Clin Endocrinol Metab (1999) 84(6):2111–8. doi: 10.1210/jc.84.6.2111
75. Sandoval-Guzmán T, Stalcup ST, Krajewski SJ, Voytko ML, Rance NE. Effects of Ovariectomy on the Neuroendocrine Axes Regulating Reproduction and Energy Balance in Young Cynomolgus Macaques. J Neuroendocrinol (2004) 16(2):146–53. doi: 10.1111/j.0953-8194.2004.01143.x
76. Rance NE, McMullen NT, Smialek JE, Price DL, Young WS 3rd. Postmenopausal Hypertrophy of Neurons Expressing the Estrogen Receptor Gene in the Human Hypothalamus. J Clin Endocrinol Metab (1990) 71(1):79–85. doi: 10.1210/jcem-71-1-79
77. Rance NE, Young WS 3rd. Hypertrophy and Increased Gene Expression of Neurons Containing Neurokinin-B and Substance-P Messenger Ribonucleic Acids in the Hypothalami of Postmenopausal Women. Endocrinology (1991) 128(5):2239–47. doi: 10.1210/endo-128-5-2239
78. Han SY, Kane G, Cheong I, Herbison AE. Characterization of GnRH Pulse Generator Activity in Male Mice Using GCaMP Fiber Photometry. Endocrinology (2019) 160(3):557–67. doi: 10.1210/en.2018-01047
79. Voliotis M, Plain Z, Li XF, McArdle CA, O'Byrne KT, Tsaneva-Atanasova K. Mathematical Models in GnRH Research. J Neuroendocrinol (2022) 34(5):e13085. doi: 10.1111/jne.13085
80. Qiu J, Nestor CC, Zhang C, Padilla SL, Palmiter RD, Kelly MJ, et al. High-Frequency Stimulation-Induced Peptide Release Synchronizes Arcuate Kisspeptin Neurons and Excites GnRH Neurons. Elife (2016) 5:1–24. doi: 10.7554/eLife.16246
81. Moore AM, Coolen LM, Lehman MN. In Vivo Imaging of the GnRH Pulse Generator Reveals a Temporal Order of Neuronal Activation and Synchronization During Each Pulse. Proc Natl Acad Sci USA (2022) 119(6):e2117767119. doi: 10.1073/pnas.2117767119
82. He W, Li X, Adekunbi D, Liu Y, Long H, Wang L, et al. Hypothalamic Effects of Progesterone on Regulation of the Pulsatile and Surge Release of Luteinising Hormone in Female Rats. Sci Rep (2017) 7(1):8096. doi: 10.1038/s41598-017-08805-1
83. Clarkson J, d'Anglemont de Tassigny X, Moreno AS, Colledge WH, Herbison AE. Kisspeptin-GPR54 Signaling is Essential for Preovulatory Gonadotropin-Releasing Hormone Neuron Activation and the Luteinizing Hormone Surge. J Neurosci Off J Soc Neurosci (2008) 28(35):8691–7. doi: 10.1523/JNEUROSCI.1775-08.2008
84. Goodman RL, Holaskova I, Nestor CC, Connors JM, Billings HJ, Valent M, et al. Evidence That the Arcuate Nucleus is an Important Site of Progesterone Negative Feedback in the Ewe. Endocrinology (2011) 152(9):3451–60. doi: 10.1210/en.2011-0195
85. Siuciak JA, McCarthy SA, Martin AN, Chapin DS, Stock J, Nadeau DM, et al. Disruption of the Neurokinin-3 Receptor (NK3) in Mice Leads to Cognitive Deficits. Psychopharmacology (2007) 194(2):185–95. doi: 10.1007/s00213-007-0828-6
86. Gianetti E, Tusset C, Noel SD, Au MG, Dwyer AA, Hughes VA, et al. TAC3/TACR3 Mutations Reveal Preferential Activation of Gonadotropin-Releasing Hormone Release by Neurokinin B in Neonatal Life Followed by Reversal in Adulthood. J Clin Endocrinol Metab (2010) 95(6):2857–67. doi: 10.1210/jc.2009-2320
87. Guran T, Tolhurst G, Bereket A, Rocha N, Porter K, Turan S, et al. Hypogonadotropic Hypogonadism Due to a Novel Missense Mutation in the First Extracellular Loop of the Neurokinin B Receptor. J Clin Endocrinol Metab (2009) 94(10):3633–9. doi: 10.1210/jc.2009-0551
88. True C, Nasrin Alam S, Cox K, Chan YM, Seminara SB. Neurokinin B is Critical for Normal Timing of Sexual Maturation But Dispensable for Adult Reproductive Function in Female Mice. Endocrinology (2015) 156(4):1386–97. doi: 10.1210/en.2014-1862
89. Uenoyama Y, Inoue N, Nakamura S, Tsukamura H. Kisspeptin Neurons and Estrogen-Estrogen Receptor α Signaling: Unraveling the Mystery of Steroid Feedback System Regulating Mammalian Reproduction. Int J Mol Sci (2021) 22(17):9229. doi: 10.3390/ijms22179229
90. Kauffman AS, Gottsch ML, Roa J, Byquist AC, Crown A, Clifton DK, et al. Sexual Differentiation of Kiss1 Gene Expression in the Brain of the Rat. Endocrinology (2007) 148(4):1774–83. doi: 10.1210/en.2006-1540
91. Kumar D, Candlish M, Periasamy V, Avcu N, Mayer C, Boehm U. Specialized Subpopulations of Kisspeptin Neurons Communicate With GnRH Neurons in Female Mice. Endocrinology (2015) 156(1):32–8. doi: 10.1210/en.2014-1671
92. Le WW, Berghorn KA, Rassnick S, Hoffman GE. Periventricular Preoptic Area Neurons Coactivated With Luteinizing Hormone (LH)-Releasing Hormone (LHRH) Neurons at the Time of the LH Surge are LHRH Afferents. Endocrinology (1999) 140(1):510–9. doi: 10.1210/endo.140.1.6403
93. Wang L, DeFazio RA, Moenter SM. Excitability and Burst Generation of AVPV Kisspeptin Neurons Are Regulated by the Estrous Cycle Via Multiple Conductances Modulated by Estradiol Action. eNeuro (2016) 3(3):ENEURO.0094-16.2016. doi: 10.1523/ENEURO.0094-16.2016
94. Piet R, Boehm U, Herbison AE. Estrous Cycle Plasticity in the Hyperpolarization-Activated Current Ih is Mediated by Circulating 17β-Estradiol in Preoptic Area Kisspeptin Neurons. J Neurosci Off J Soc Neurosci (2013) 33(26):10828–39. doi: 10.1523/JNEUROSCI.1021-13.2013
95. Mittelman-Smith MA, Rudolph LM, Mohr MA, Micevych PE. Rodent Models of Non-Classical Progesterone Action Regulating Ovulation. Front Endocrinol (2017) 8:165. doi: 10.3389/fendo.2017.00165
96. Stephens SB, Tolson KP, Rouse ML Jr., Poling MC, Hashimoto-Partyka MK, Mellon PL, et al. Absent Progesterone Signaling in Kisspeptin Neurons Disrupts the LH Surge and Impairs Fertility in Female Mice. Endocrinology (2015) 156(9):3091–7. doi: 10.1210/en.2015-1300
97. Mohr MA, Esparza LA, Steffen P, Micevych PE, Kauffman AS. Progesterone Receptors in AVPV Kisspeptin Neurons Are Sufficient for Positive Feedback Induction of the LH Surge. Endocrinology (2021) 162(11):bqab161. doi: 10.1210/endocr/bqab161
98. Dubois SL, Acosta-Martínez M, DeJoseph MR, Wolfe A, Radovick S, Boehm U, et al. Positive, But Not Negative Feedback Actions of Estradiol in Adult Female Mice Require Estrogen Receptor α in Kisspeptin Neurons. Endocrinology (2015) 156(3):1111–20. doi: 10.1210/en.2014-1851
99. Tsukamura H, Homma T, Tomikawa J, Uenoyama Y, Maeda K. Sexual Differentiation of Kisspeptin Neurons Responsible for Sex Difference in Gonadotropin Release in Rats. Ann New York Acad Sci (2010) 1200:95–103. doi: 10.1111/j.1749-6632.2010.05645.x
100. Smith JT, Dungan HM, Stoll EA, Gottsch ML, Braun RE, Eacker SM, et al. Differential Regulation of KiSS-1 mRNA Expression by Sex Steroids in the Brain of the Male Mouse. Endocrinology (2005) 146(7):2976–84. doi: 10.1210/en.2005-0323
101. Smith JT. Sex Steroid Regulation of Kisspeptin Circuits. Adv Exp Med Biol (2013) 784:275–95. doi: 10.1007/978-1-4614-6199-9_13
102. Yeo SH, Kyle V, Morris PG, Jackman S, Sinnett-Smith LC, Schacker M, et al. Visualisation of Kiss1 Neurone Distribution Using a Kiss1-CRE Transgenic Mouse. J Neuroendocrinol (2016) 28(11):10.1111/jne.12435. doi: 10.1111/jne.12435
103. Abreu AP, Kaiser UB. Pubertal Development and Regulation. Lancet Diabetes Endocrinol (2016) 4(3):254–64. doi: 10.1016/S2213-8587(15)00418-0
104. Semaan SJ, Kauffman AS. Emerging Concepts on the Epigenetic and Transcriptional Regulation of the Kiss1 Gene. Int J Dev Neurosci Off J Int Soc Dev Neurosci (2013) 31(6):452–62. doi: 10.1016/j.ijdevneu.2013.03.006
105. Sánchez-Garrido MA, García-Galiano D, Tena-Sempere M. Early Programming of Reproductive Health and Fertility: Novel Neuroendocrine Mechanisms and Implications in Reproductive Medicine. Hum Reprod Update (2022) 28(3):346–75. doi: 10.1093/humupd/dmac005
106. de Roux N, Genin E, Carel JC, Matsuda F, Chaussain JL, Milgrom E. Hypogonadotropic Hypogonadism Due to Loss of Function of the KiSS1-Derived Peptide Receptor Gpr54. Proc Natl Acad Sci USA (2003) 100(19):10972–6. doi: 10.1073/pnas.1834399100
107. Uenoyama Y, Nakamura S, Hayakawa Y, Ikegami K, Watanabe Y, Deura C, et al. Lack of Pulse and Surge Modes and Glutamatergic Stimulation of Luteinising Hormone Release in Kiss1 Knockout Rats. J Neuroendocrinol (2015) 27(3):187–97. doi: 10.1111/jne.12257
108. Teles MG, Bianco SD, Brito VN, Trarbach EB, Kuohung W, Xu S, et al. A GPR54-Activating Mutation in a Patient With Central Precocious Puberty. New Engl J Med (2008) 358(7):709–15. doi: 10.1056/NEJMoa073443
109. de Vries L, Shtaif B, Phillip M, Gat-Yablonski G. Kisspeptin Serum Levels in Girls With Central Precocious Puberty. Clin Endocrinol (2009) 71(4):524–8. doi: 10.1111/j.1365-2265.2009.03575.x
110. Li M, Chen Y, Liao B, Tang J, Zhong J, Lan D. The Role of Kisspeptin and MKRN3 in the Diagnosis of Central Precocious Puberty in Girls. Endocrine connections. (2021) 10(9):1147–54. doi: 10.1530/EC-21-0182
111. Rhie YJ, Lee KH, Eun SH, Choi BM, Chae HW, Kwon AR, et al. Serum Kisspeptin Levels in Korean Girls With Central Precocious Puberty. J Korean Med Sci (2011) 26(7):927–31. doi: 10.3346/jkms.2011.26.7.927
112. Silveira LG, Noel SD, Silveira-Neto AP, Abreu AP, Brito VN, Santos MG, et al. Mutations of the KISS1 Gene in Disorders of Puberty. J Clin Endocrinol Metab (2010) 95(5):2276–80. doi: 10.1210/jc.2009-2421
113. Pineda R, Garcia-Galiano D, Roseweir A, Romero M, Sanchez-Garrido MA, Ruiz-Pino F, et al. Critical Roles of Kisspeptins in Female Puberty and Preovulatory Gonadotropin Surges as Revealed by a Novel Antagonist. Endocrinology (2010) 151(2):722–30. doi: 10.1210/en.2009-0803
114. Navarro VM, Fernández-Fernández R, Castellano JM, Roa J, Mayen A, Barreiro ML, et al. Advanced Vaginal Opening and Precocious Activation of the Reproductive Axis by KiSS-1 Peptide, the Endogenous Ligand of GPR54. J Physiol (2004) 561(Pt 2):379–86. doi: 10.1113/jphysiol.2004.072298
115. Plant TM, Ramaswamy S, Dipietro MJ. Repetitive Activation of Hypothalamic G Protein-Coupled Receptor 54 With Intravenous Pulses of Kisspeptin in the Juvenile Monkey (Macaca Mulatta) Elicits a Sustained Train of Gonadotropin-Releasing Hormone Discharges. Endocrinology (2006) 147(2):1007–13. doi: 10.1210/en.2005-1261
116. Clarkson J, Boon WC, Simpson ER, Herbison AE. Postnatal Development of an Estradiol-Kisspeptin Positive Feedback Mechanism Implicated in Puberty Onset. Endocrinology (2009) 150(7):3214–20. doi: 10.1210/en.2008-1733
117. Roa J, Aguilar E, Dieguez C, Pinilla L, Tena-Sempere M. New Frontiers in Kisspeptin/GPR54 Physiology as Fundamental Gatekeepers of Reproductive Function. Front Neuroendocrinol (2008) 29(1):48–69. doi: 10.1016/j.yfrne.2007.07.002
118. Trevisan CM, Montagna E, de Oliveira R, Christofolini DM, Barbosa CP, Crandall KA, et al. Kisspeptin/GPR54 System: What Do We Know About Its Role in Human Reproduction? Cell Physiol Biochem Int J Exp Cell physiology Biochem Pharmacol (2018) 49(4):1259–76. doi: 10.1159/000493406
119. Fernandois D, Na E, Cuevas F, Cruz G, Lara HE, Paredes AH. Kisspeptin Is Involved in Ovarian Follicular Development During Aging in Rats. J Endocrinol (2016) 228(3):161–70. doi: 10.1530/JOE-15-0429
120. Fleming R. Anti-Müllerian Hormone: A Personal View of the Empowering Analyte. J Hum Reprod Sci (2020) 13(4):257–60. doi: 10.4103/jhrs.JHRS_231_20
121. Dewailly D, Andersen CY, Balen A, Broekmans F, Dilaver N, Fanchin R, et al. The Physiology and Clinical Utility of Anti-Mullerian Hormone in Women. Hum Reprod Update (2014) 20(3):370–85. doi: 10.1093/humupd/dmt062
122. Sen A, Caiazza F. Oocyte Maturation: A Story of Arrest and Release. Front Bioscience (Scholar edition) (2013) 5(2):451–77. doi: 10.2741/S383
123. Saadeldin IM, Koo OJ, Kang JT, Kwon DK, Park SJ, Kim SJ, et al. Paradoxical Effects of Kisspeptin: It Enhances Oocyte In Vitro Maturation But has an Adverse Impact on Hatched Blastocysts During In Vitro Culture. Reproduction Fertility Dev (2012) 24(5):656–68. doi: 10.1071/RD11118
124. Persani L, Rossetti R, Di Pasquale E, Cacciatore C, Fabre S. The Fundamental Role of Bone Morphogenetic Protein 15 in Ovarian Function and Its Involvement in Female Fertility Disorders. Hum Reprod Update (2014) 20(6):869–83. doi: 10.1093/humupd/dmu036
125. Sagata N. What Does Mos do in Oocytes and Somatic Cells? BioEssays News Rev Mol Cell Dev Biol (1997) 19(1):13–21. doi: 10.1002/bies.950190105
126. Gebauer F, Richter JD. Synthesis and Function of Mos: The Control Switch of Vertebrate Oocyte Meiosis. BioEssays News Rev Mol Cell Dev Biol (1997) 19(1):23–8. doi: 10.1002/bies.950190106
127. McNatty KP, Juengel JL, Reader KL, Lun S, Myllymaa S, Lawrence SB, et al. Bone Morphogenetic Protein 15 and Growth Differentiation Factor 9 Co-Operate to Regulate Granulosa Cell Function. Reprod (Cambridge England). (2005) 129(4):473–80. doi: 10.1530/rep.1.0511
128. Reader KL, Heath DA, Lun S, McIntosh CJ, Western AH, Littlejohn RP, et al. Signalling Pathways Involved in the Cooperative Effects of Ovine and Murine GDF9+BMP15-Stimulated Thymidine Uptake by Rat Granulosa Cells. Reproduction (2011) 142(1):123–31. doi: 10.1530/REP-10-0490
129. Mottershead DG, Ritter LJ, Gilchrist RB. Signalling Pathways Mediating Specific Synergistic Interactions Between GDF9 and BMP15. Mol Hum Reprod. (2012) 18(3):121–8. doi: 10.1093/molehr/gar056
130. Richards JS, Russell DL, Ochsner S, Espey LL. Ovulation: New Dimensions and New Regulators of the Inflammatory-Like Response. Annu Rev Physiol (2002) 64:69–92. doi: 10.1146/annurev.physiol.64.081501.131029
131. Sirois J, Sayasith K, Brown KA, Stock AE, Bouchard N, Doré M. Cyclooxygenase-2 and its Role in Ovulation: A 2004 Account. Hum Reprod Update. (2004) 10(5):373–85. doi: 10.1093/humupd/dmh032
132. Caraty A, Smith JT, Lomet D, Ben Saïd S, Morrissey A, Cognie J, et al. Kisspeptin Synchronizes Preovulatory Surges in Cyclical Ewes and Causes Ovulation in Seasonally Acyclic Ewes. Endocrinology (2007) 148(11):5258–67. doi: 10.1210/en.2007-0554
133. Owen CM, Zhou X, Bernard DJ, Jaffe LA. Kisspeptin-54 Injection Induces a Physiological Luteinizing Hormone Surge and Ovulation in Mice. Biol Reproduction (2021) 104(6):1181–3. doi: 10.1093/biolre/ioab067
134. Gaytan F, Garcia-Galiano D, Dorfman MD, Manfredi-Lozano M, Castellano JM, Dissen GA, et al. Kisspeptin Receptor Haplo-Insufficiency Causes Premature Ovarian Failure Despite Preserved Gonadotropin Secretion. Endocrinology (2014) 155(8):3088–97. doi: 10.1210/en.2014-1110
135. Kow LM, Florea C, Schwanzel-Fukuda M, Devidze N, Kami Kia H, Lee A, et al. Development of a Sexually Differentiated Behavior and its Underlying CNS Arousal Functions. Curr Topics Dev Biol (2007) 79:37–59. doi: 10.1016/S0070-2153(06)79002-0
136. Hellier V, Brock O, Candlish M, Desroziers E, Aoki M, Mayer C, et al. Female Sexual Behavior in Mice is Controlled by Kisspeptin Neurons. Nat Commun (2018) 9(1):400. doi: 10.1038/s41467-017-02797-2
137. Kauffman AS, Park JH, McPhie-Lalmansingh AA, Gottsch ML, Bodo C, Hohmann JG, et al. The Kisspeptin Receptor GPR54 is Required for Sexual Differentiation of the Brain and Behavior. J Neurosci Off J Soc Neurosci (2007) 27(33):8826–35. doi: 10.1523/JNEUROSCI.2099-07.2007
138. Hellier V, Brock O, Bakker J. The Role of Kisspeptin in Sexual Behavior. Semin Reprod Med (2019) 37(2):84–92. doi: 10.1055/s-0039-3400992
139. Chiang CM, Chiu HY, Jong DS, Wu LS, Lee YJ, Chiu CH. Role of the Kisspeptin/KISS1 Receptor System in the Testicular Development of Mice. J Chin Med Assoc JCMA (2021) 84(2):203–11. doi: 10.1097/JCMA.0000000000000443f
140. Mele E, D'Auria R, Scafuro M, Marino M, Fasano S, Viggiano A, et al. Differential Expression of Kisspeptin System and Kisspeptin Receptor Trafficking During Spermatozoa Transit in the Epididymis. Genes (2022) 13(2):295. doi: 10.3390/genes13020295
141. Han Y, Peng X, Si W, Liu G, Han Y, Jiang X, et al. Local Expressions and Function of Kiss1/GPR54 in Goats' Testes. Gene (2020) 738:144488. doi: 10.1016/j.gene.2020.144488
142. Salehi S, Adeshina I, Chen H, Zirkin BR, Hussain MA, Wondisford F, et al. Developmental and Endocrine Regulation of Kisspeptin Expression in Mouse Leydig Cells. Endocrinology (2015) 156(4):1514–22. doi: 10.1210/en.2014-1606
143. Petrucci L, Maranesi M, Verini Supplizi A, Dall'Aglio C, Mandara MT, Quassinti L, et al. Kisspeptin/GnRH1 System in Leydig Cells of Horse (Equus Caballus): Presence and Function. Theriogenology (2020) 152:1–7. doi: 10.1016/j.theriogenology.2020.04.006
144. Chianese R, Ciaramella V, Fasano S, Pierantoni R, Meccariello R. Kisspeptin Regulates Steroidogenesis and Spermiation in Anuran Amphibian. Reproduction (2017) 154(4):403–14. doi: 10.1530/REP-17-0030
145. Mei H, Doran J, Kyle V, Yeo SH, Colledge WH. Does Kisspeptin Signaling Have a Role in the Testes? Front Endocrinol (2013) 4:198. doi: 10.3389/fendo.2013.00198
146. Thompson EL, Murphy KG, Patterson M, Bewick GA, Stamp GW, Curtis AE, et al. Chronic Subcutaneous Administration of Kisspeptin-54 Causes Testicular Degeneration in Adult Male Rats. Am J Physiol Endocrinol Metab (2006) 291(5):E1074–82. doi: 10.1152/ajpendo.00040.2006
147. Thompson EL, Amber V, Stamp GW, Patterson M, Curtis AE, Cooke JH, et al. Kisspeptin-54 at High Doses Acutely Induces Testicular Degeneration in Adult Male Rats via Central Mechanisms. Br J Pharmacol (2009) 156(4):609–25. doi: 10.1111/j.1476-5381.2008.00061.x
148. Aytürk N, Firat T, Kükner A, Özoğul C, Töre F, Kandirali İE, et al. The Effect of Kisspeptin on Spermatogenesis and Apoptosis in Rats. Turkish J Med Sci (2017) 47(1):334–42. doi: 10.3906/sag-1505-69
149. Ohga H, Selvaraj S, Matsuyama M. The Roles of Kisspeptin System in the Reproductive Physiology of Fish With Special Reference to Chub Mackerel Studies as Main Axis. Front Endocrinol (2018) 9:147. doi: 10.3389/fendo.2018.00147
150. Pinto FM, Cejudo-Román A, Ravina CG, Fernández-Sánchez M, Martín-Lozano D, Illanes M, et al. Characterization of the Kisspeptin System in Human Spermatozoa. Int J Andrology (2012) 35(1):63–73. doi: 10.1111/j.1365-2605.2011.01177.x
151. Adekunbi DA, Li XF, Lass G, Shetty K, Adegoke OA, Yeo SH, et al. Kisspeptin Neurones in the Posterodorsal Medial Amygdala Modulate Sexual Partner Preference and Anxiety in Male Mice. J Neuroendocrinol (2018) 30(3):e12572. doi: 10.1111/jne.12572
152. Nakamura S, Watanabe Y, Goto T, Ikegami K, Inoue N, Uenoyama Y, et al. Kisspeptin Neurons as a Key Player Bridging the Endocrine System and Sexual Behavior in Mammals. Front Neuroendocrinol (2022) 64:100952. doi: 10.1016/j.yfrne.2021.100952
153. Kim J, Semaan SJ, Clifton DK, Steiner RA, Dhamija S, Kauffman AS. Regulation of Kiss1 Expression by Sex Steroids in the Amygdala of the Rat and Mouse. Endocrinology (2011) 152(5):2020–30. doi: 10.1210/en.2010-1498
154. Mills EGA, O'Byrne KT, Comninos AN. The Roles of the Amygdala Kisspeptin System. Semin Reprod Med (2019) 37(2):64–70. doi: 10.1055/s-0039-3400462
155. Mills EGA, O'Byrne KT, Comninos AN. Kisspeptin as a Behavioral Hormone. Semin Reprod Med (2019) 37(2):56–63. doi: 10.1055/s-0039-3400239
156. Wahab F, Quinton R, Seminara SB. The Kisspeptin Signaling Pathway and its Role in Human Isolated GnRH Deficiency. Mol Cell Endocrinol (2011) 346(1-2):29–36. doi: 10.1016/j.mce.2011.05.043
157. Sharma A, Thaventhiran T, Minhas S, Dhillo WS, Jayasena CN. Kisspeptin and Testicular Function-Is it Necessary? Int J Mol Sci (2020) 21(8):2958. doi: 10.3390/ijms21082958
158. Tang R, Ding X, Zhu J. Kisspeptin and Polycystic Ovary Syndrome. Front endocrinology. (2019) 10:298. doi: 10.3389/fendo.2019.00298
159. Ibrahim RO, Omer SH, Fattah CN. The Correlation Between Hormonal Disturbance in PCOS Women and Serum Level of Kisspeptin. Int J Endocrinol. (2020) 2020:6237141. doi: 10.1155/2020/6237141
160. Liu J, Qu T, Li Z, Yu L, Zhang S, Yuan D, et al. Serum Kisspeptin Levels in Polycystic Ovary Syndrome: A Meta-Analysis. J Obstet Gynaecol Res (2021) 47(6):2157–65. doi: 10.1111/jog.14767
161. Owens LA, Abbara A, Lerner A, O'Floinn S, Christopoulos G, Khanjani S, et al. The Direct and Indirect Effects of Kisspeptin-54 on Granulosa Lutein Cell Function. Hum Reprod (Oxford England) (2018) 33(2):292–302. doi: 10.1093/humrep/dex357
162. Iwata K, Kunimura Y, Matsumoto K, Ozawa H. Effect of Androgen on Kiss1 Expression and Luteinizing Hormone Release in Female Rats. J Endocrinology (2017) 233(3):281–92. doi: 10.1530/JOE-16-0568
163. Araujo-Lopes R, Crampton JR, Aquino NS, Miranda RM, Kokay IC, Reis AM, et al. Prolactin Regulates Kisspeptin Neurons in the Arcuate Nucleus to Suppress LH Secretion in Female Rats. Endocrinology (2014) 155(3):1010–20. doi: 10.1210/en.2013-1889
164. Grattan DR, Jasoni CL, Liu X, Anderson GM, Herbison AE. Prolactin Regulation of Gonadotropin-Releasing Hormone Neurons to Suppress Luteinizing Hormone Secretion in Mice. Endocrinology (2007) 148(9):4344–51. doi: 10.1210/en.2007-0403
165. Brown RS, Herbison AE, Grattan DR. Prolactin Regulation of Kisspeptin Neurones in the Mouse Brain and its Role in the Lactation-Induced Suppression of Kisspeptin Expression. J Neuroendocrinol (2014) 26(12):898–908. doi: 10.1111/jne.12223
166. Hu KL, Zhao H, Yu Y, Li R. Kisspeptin as a Potential Biomarker Throughout Pregnancy. Eur J Obstet Gynecol Reprod Biol (2019) 240:261–6. doi: 10.1016/j.ejogrb.2019.07.016
167. Hu KL, Chang HM, Zhao HC, Yu Y, Li R, Qiao J. Potential Roles for the Kisspeptin/Kisspeptin Receptor System in Implantation and Placentation. Hum Reprod Update (2019) 25(3):326–43. doi: 10.1093/humupd/dmy046
168. Sullivan-Pyke C, Haisenleder DJ, Senapati S, Nicolais O, Eisenberg E, Sammel MD, et al. Kisspeptin as a New Serum Biomarker to Discriminate Miscarriage From Viable Intrauterine Pregnancy. Fertility sterility. (2018) 109(1):137–41.e2. doi: 10.1016/j.fertnstert.2017.09.029
169. Jayasena CN, Abbara A, Comninos AN, Nijher GM, Christopoulos G, Narayanaswamy S, et al. Kisspeptin-54 Triggers Egg Maturation in Women Undergoing In Vitro Fertilization. J Clin Invest (2014) 124(8):3667–77. doi: 10.1172/JCI75730
170. Romero-Ruiz A, Skorupskaite K, Gaytan F, Torres E, Perdices-Lopez C, Mannaerts BM, et al. Kisspeptin Treatment Induces Gonadotropic Responses and Rescues Ovulation in a Subset of Preclinical Models and Women With Polycystic Ovary Syndrome. Hum Reprod (Oxford England) (2019) 34(12):2495–512. doi: 10.1093/humrep/dez205
171. Abbara A, Eng PC, Phylactou M, Clarke SA, Richardson R, Sykes CM, et al. Kisspeptin Receptor Agonist has Therapeutic Potential for Female Reproductive Disorders. J Clin Invest (2020) 130(12):6739–53. doi: 10.1172/JCI139681
172. Hoskova K, Bryant NK, Chen ME, Nachtigall LB, Lippincott M, Balasubramanian R, et al. Kisspeptin Overcomes GnRH Neuronal Suppression Secondary to Hyperprolactinemia in the Human. J Clin Endocrinol Metab (2022) dgac166. doi: 10.1210/clinem/dgac166
173. Sonigo C, Bouilly J, Carré N, Tolle V, Caraty A, Tello J, et al. Hyperprolactinemia-Induced Ovarian Acyclicity is Reversed by Kisspeptin Administration. J Clin Invest. (2012) 122(10):3791–5. doi: 10.1172/JCI63937
174. Penning TM, Detlefsen AJ. Intracrinology-Revisited and Prostate Cancer. J Steroid Biochem Mol Biol (2020) 196:105499. doi: 10.1016/j.jsbmb.2019.105499
175. Ishikawa K, Tanaka A, Kogame A, Watanabe T, Tagawa Y, Matsui H. Usefulness of Pharmacokinetic/Efficacy Analysis of an Investigational Kisspeptin Analog, TAK-448, in Quantitatively Evaluating Anti-Tumor Growth Effect in the Rat VCaP Androgen-Sensitive Prostate Cancer Model. Eur J Pharmacol (2018) 828:126–34. doi: 10.1016/j.ejphar.2018.03.032
176. Matsui H, Tanaka A, Yokoyama K, Takatsu Y, Ishikawa K, Asami T, et al. Chronic Administration of the Metastin/Kisspeptin Analog KISS1-305 or the Investigational Agent TAK-448 Suppresses Hypothalamic Pituitary Gonadal Function and Depletes Plasma Testosterone in Adult Male Rats. Endocrinology (2012) 153(11):5297–308. doi: 10.1210/en.2012-1388
Keywords: kisspeptin, KISS1R, HPG axis, KNDy neurons, reproduction
Citation: Xie Q, Kang Y, Zhang C, Xie Y, Wang C, Liu J, Yu C, Zhao H and Huang D (2022) The Role of Kisspeptin in the Control of the Hypothalamic-Pituitary-Gonadal Axis and Reproduction. Front. Endocrinol. 13:925206. doi: 10.3389/fendo.2022.925206
Received: 21 April 2022; Accepted: 30 May 2022;
Published: 28 June 2022.
Edited by:
Alfredo Ulloa-Aguirre, National Autonomous University of Mexico, MexicoReviewed by:
Tatiana Fiordelisio, National Autonomous University of Mexico, MexicoFernando Larrea, Instituto Nacional de Ciencias Médicas y Nutrición Salvador Zubirán (INCMNSZ), Mexico
Copyright © 2022 Xie, Kang, Zhang, Xie, Wang, Liu, Yu, Zhao and Huang. This is an open-access article distributed under the terms of the Creative Commons Attribution License (CC BY). The use, distribution or reproduction in other forums is permitted, provided the original author(s) and the copyright owner(s) are credited and that the original publication in this journal is cited, in accordance with accepted academic practice. No use, distribution or reproduction is permitted which does not comply with these terms.
*Correspondence: Donghui Huang, jhsyyjs@126.com; Hu Zhao, zhaohu@hust.edu.cn
†These authors have contributed equally to this work