- 1Division of Nephrology, Longhua Hospital, Shanghai University of Traditional Chinese Medicine, Shanghai, China
- 2Department of Medicine, Division of Nephrology, Icahn School of Medicine at Mount Sinai, New York, NY, United States
The incidence and prevalence of chronic kidney disease (CKD) continue to increase worldwide remaining as a major public health burden. CKD eventually progresses to end-stage kidney failure and patients with CKD have high morbidity and mortality. Sirtuin 1 (SIRT1), a NAD+-dependent deacetylases, has significant renal protective effects through its regulation of fibrosis, apoptosis, and senescence, oxidative stress, inflammation and aging process. The renal protective effects of Sirt1 have been described in many kidney diseases such as diabetic kidney disease and HIV-related kidney disease. SIRT1 also has protective effects against vascular calcification and therefore could be developed as a therapy for both CKD and CKD complications. In this narrative review, we will give an overview of the recent progress on the role of SIRT1 and its downstream pathways in CKD. We will also discuss potential therapeutic approach by activating SIRT1-related pathway in patients with CKD. The purpose is to hope to provide some insights on the future direction of the research in the field of SIRT1 for CKD.
Introduction
About 10% of the human population have chronic kidney disease (CKD), which have high incidence of cardiovascular disease and mortality (1). CKD is characterized by a progressive loss of kidney function with glomerular, tubular and vascular injuries and cardiovascular disease is the most important complication of CKD which is characterized by vascular calcification (2–4). Therefore, new therapies are urgently needed to halt the progression of CKD and prevent complications of CKD. A number of studies have shown that SIRT1, a nicotinamide adenine dinucleotide-dependent histone deacetylase, has pivotal roles on renal protection through reduction of oxidative stress, inflammation, and fibrosis (5–8). In addition, Sirt1 also inhibits apoptosis and regulates metabolism (9). Therefore, the activation of SIRT1 may imply a therapeutic strategy to improve the clinical outcome of CKD. This review focuses on the protective effects of SIRT1 against CKD progression and complications through its regulation of fibrosis, apoptosis, oxidative stress, inflammation and aging process.
Expression of SIRT1 in the Kidney
SIRT1 is a highly conservative protein and contains 500 amino acid residues, which was first discovered in 1999 (10). SIRT1 is expressed in the kidneys and can protect and maintain normal kidney cell function by mediating in various physiological processes (10). SIRT1 is mainly resided in the nucleus where it facilitates nuclear cytoplasmic shuttling by regulating both nucleosome histone acetylation and the activity of several transcriptional factors (11–13). SIRT1 can also be found in the cytoplasm. A few studies showed SIRT1 inhibits TNFα-dependent transactivation of NF-κB in TNF-α induced cytokine production in fibroblast cells and the expression of several proinflammatory genes are inhibited (14). SIRT1 is a negative regulator of p53. SIRT1 can deacetylate p53 and result in reduced cellular senescence and apoptosis after DNA damage and oxidative stress (15, 16).
SIRT1 and Renal Fibrosis
Renal fibrosis is a major feature of progressive CKDs (3). Studies have focused on the role of SIRT1 for renal fibrosis. Deficiency of Sirt1 in endothelial cells increases peritubular capillary rarefaction (17) and aggravates nephrosclerosis, via downregulation of matrix metalloproteinase-14, which indicates a role of SIRT1 in renal fibrosis (18). SIRT1 also regulates kidney fibrosis by inducing deacetylation of Smad4 and inhibiting TGF-β-mediated matrix metalloproteinase-7 expression in kidney tubular epithelial cells (19). Activation of SIRT1 can lead to attenuated renal fibrotic processes. SIRT1 reverses Smad3 acetylation and thereby inhibiting the profibrotic response of TGF-β1 in vitro and in vivo such as in unilateral-ureteric obstruction mouse model of renal fibrosis (20, 21). In addition, a Chinese herbal formula, Shen Shuai IIRecipe (SSR), significantly attenuates renal injury and fibrosis in the remnant kidneys (22). SSR could contribute to renal protection by up-regulating SIRT1/Smad3 deacetylation pathway and attenuating renal fibrosis in 5/6 nephrectomy model of CKD (22). Furthermore, tubular cell-specific overexpression of SIRT1 attenuates the progression of AKI to CKD transition through Smad4 deacetylation (19). Therefore, activation of SIRT1 could be a potential approach to develop anti-fibrosis therapy for CKD.
SIRT1 in Different Kidney Diseases
SIRT1 plays a major role in diabetic kidney disease (DKD). In our previous studies, we also find SIRT1 can regulate NF-κB (p65) and STAT3 acetylation in DKD. Since SIRT1 expression is reduced in the diabetic kidney, the acetylation level of p65 and STAT3 is increased in diabetic kidneys (23). The loss of SIRT1 in the podocyte of diabetic db/db mice increases acetylation of p65 and STAT3 as a result of exacerbated proteinuria and kidney injury, likely through increased inflammatory response. Conversely, a bromodomain inhibitor, MS417, significantly attenuates proteinuria and kidney injury. MS417 is able to block acetylation-mediated association of p65 and STAT3 with bromodomain and extra-terminal domain (BET) proteins in the kidney cells from diabetic mice. These studies therefore indicate the critical role of SIRT1 against proteinuria and kidney injury by decreasing acetylation of p65 NF-κB and STAT3 in DKD (23). For further exploring the function of SIRT1, we generated inducible and reversible Sirt1-knockdown mice in a global, or podocyte-specific, or tubular-specific pattern. We find that knockout of SIRT1 either globally or specifically in podocytes induced more albuminuria and glomerulosclerosis in Adriamycin-induced nephropathy mouse model as compared to wild-type mice with Adriamycin-induced nephropathy. Knockdown of SIRT1 also induces more mitochondrial injury and cell senescence in the kidney cells in diabetic mice as compared with control diabetic mice (24). These results indicate a critical role of SIRT1 in the pathogenesis of DKD by regulating mitochondrial stress/injury and cell senescence of kidney cells.
Recently, we also demonstrate a role of SIRT1 in HIV-related kidney disease. We find that HIV infection suppresses SIRT1 expression in kidney cells, leading to increased acetylation and activation of NF-κB and STAT3, similar to those observed in DKD (25). Mechanistically, this is through HIV-induced miR-34a expression, which downregulates Sirt1 mRNA level. Sirt1 not only inhibits proinflammatory response but also HIV viral gene expression in podocytes and thereby attenuating HIV-induced podocyte injury as summarized in the Figure 1 (25). Therefore, SIRT1 is also a key protector against HIV-mediated CKD pathogenesis. In addition, HIV infection and diabetes have a synergistic or additive effect on the progression of CKD (25). We believe that SIRT1, which is suppressed by both diabetes and HIV infection, plays a key role in mediating the synergistic effects between diabetes and HIV and may explain why HIV infection aggravates DKD progression.
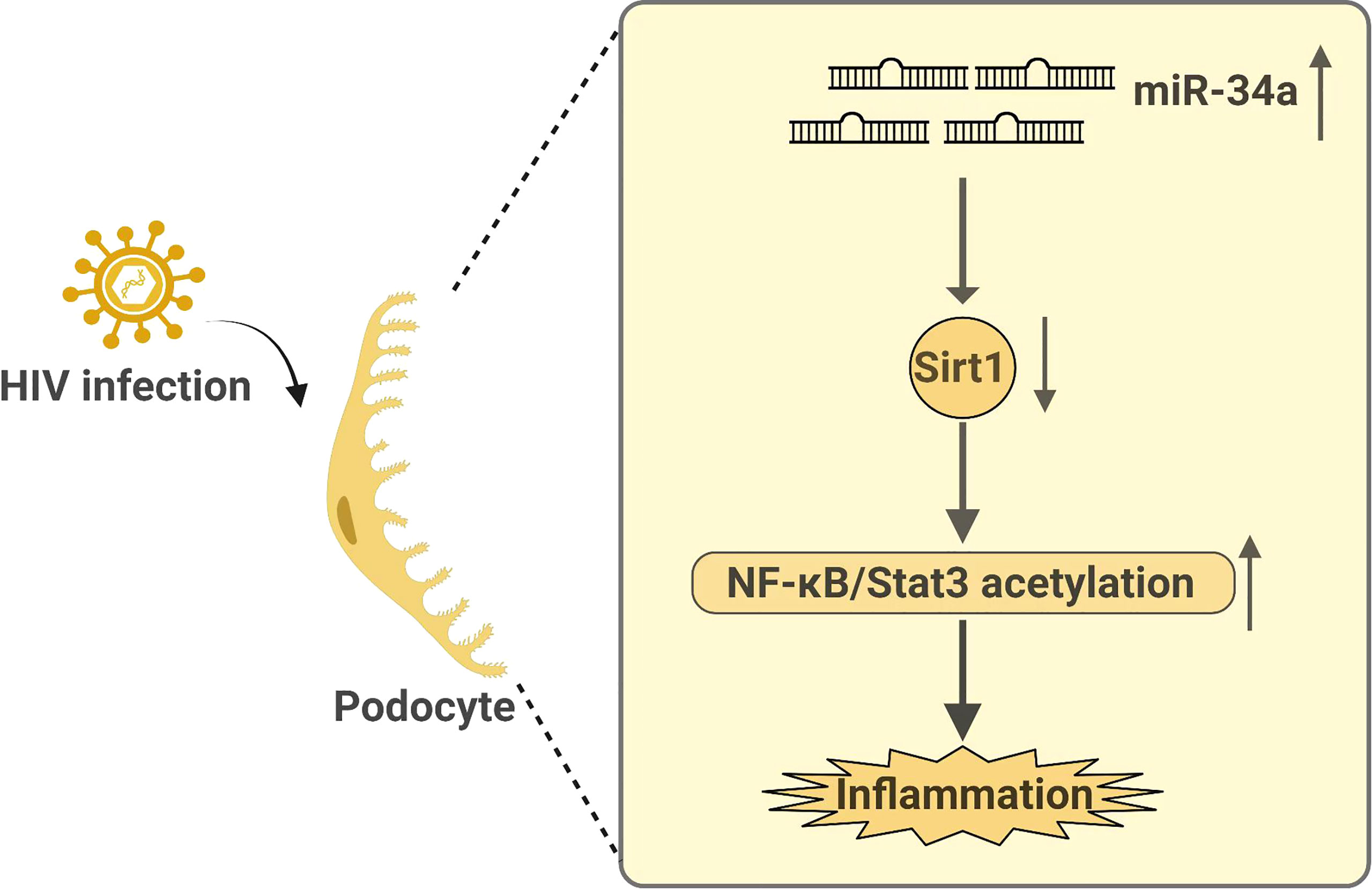
Figure 1 Summary on how HIV infection regulates Sirt1 expression and how Sirt1 mediates HIV infection-induced kidney disease.
The studies of Sirt1 in human CKD are limited. In our previous study, we find that both mRNA and protein levels of Sirt1 are reduced in glomeruli of patients with diabetic kidney disease (DKD) as assessed by qPCR and immunostaining (26). Consistent with this, we also show that acetylation of NF-kB and STAT3 is increased in human diabetic kidney (23). SIRT1 expression is also suppressed in the kidneys from HIV-infected patients (25). How Sirt1 expression and function are regulated in human CKD remains unclear. It has been shown that miRNA34a, an inhibitor of Sirt1 expression, is increased in human CKD (27) and, therefore we believe that miRNA34a could downregulate Sirt1 in human CKD.
SIRT1 and Aging
Experimental evidences show that SIRT1 plays critical roles in age-related pathological changes such as age-related kidney damage (28). Previous studies showed that SIRT1 activity is decreased in the kidneys of aged rodents (29). SIRT1 can protect cells from apoptosis and senescence induced by oxidative stress during aging process. SIRT1 regulates activation of multiple FOXO proteins such as FOXO1, FOXO3, and FOXO4 by inducing their deacetylation in the context of oxidative stress (30). It has been shown that PI3K-Akt, which is upstream signaling pathway for FOXO proteins, is downregulated in aging kidney (31). The aging process is highly associated with systemic hypoxia, which results in apoptosis, metabolism disorder, and abnormal cell cycle. Hypoxia decreases SIRT1 expression to promote FOXO3 acetylation and therefore it inhibits the expression of FOXO3 target genes such as p27Kip1 and Bnip3. In addition, hypoxia causes apoptosis and inhibits the autophagy of senescent cells, leading to the accumulation of senescent cells. In the kidney, SIRT1 also protects the apoptosis of kidney cells by inhibiting Smad7 acetylation, which is mediated by p300 (32). Together, these findings suggest a critical role of SIRT1 in age-related disease of CKD.
Role of SIRT1 in Vascular Calcification in Chronic Kidney Disease
Vascular calcification (VC) is an important pathological finding in patients with CKD, which mediates cardiovascular complication and accompanied by displaying the features of vascular aging (33–36). Vascular calcification is associated with vascular injury such as atherosclerosis, vascular stiffness and vascular aging (37). Vascular calcification can cause the abnormal calcium phosphate crystal deposition in the vessel wall. Increasing osteogenic transition will positively regulate vascular calcification in senescent vascular smooth muscle cells (5). SIRT1 can inhibit vascular calcification (38, 39). A decreased expression of SIRT1 was observed during the development of vascular calcification, and activation of SIRT1 can reduce vascular calcification, suggesting a protective role of SIRT1 in vascular calcification (38, 40, 41). Resveratrol, a pan-activator of SIRT1, ameliorates vascular calcification in animal models (42, 43). Resveratrol activates all Sirt1 isoforms and affects many downstream pathways such as COX and PGC1a (44, 45) and therefore these data do not suggest that this is Sirt1-specific. More specific Sirt1 agonists have been described and future studies are required to confirm whether more specific Sirt1 agonists could also improve vascular calcifications (46–48). Spermidine (Spd) can upregulate SIRT1 and inhibit ER stress, and thereby alleviating vascular calcification in CKD. When the expression of SIRT1 is decreased, the inhibitory effect of Spd on vascular smooth muscle cell calcification is also abolished, suggesting that SIRT mediates the protective effect of Spd on the progression of vascular calcification. In addition, a large body of evidence suggest that SIRT1 plays a critical role in inhibiting the senescence of vascular smooth muscle cells and endothelial cells (49, 50). Also, SIRT1 regulates RUNX2 deacetylation to affect its transcriptional regulation in hyperglycemic conditions (51). In endothelial cells, SIRT1 and eNOS/NO can crosstalk each other to increase their antioxidant and anti-inflammatory property. SIRT1 also inhibits p16 and p21 expression to reduce vascular smooth muscle cells (VSMCs) senescence. In addition, SIRT1 also inhibits osteogenic phenotypic transdifferentiation of VSMCs through deacetylation of RUNX2 and β-catenin (40). SIRT1 also can prevent adipocytokine release through the activation of AMPK pathway or normalization of adiponectin secretion in perivascular adipose tissues (PVAT). Because of these protective mechanisms of Sirt1 in endothelial cells, VSMCs, and PVAT, SIRT1 activators could be considered as potential drugs to inhibit vascular calcification in CKD patients (9, 52). The Figure 2 summarizes the potential mechanism of Sirt1 in protecting vascular calcification in CKD patients.
SIRT1 is a Potential Therapeutic Target of CKD
SIRT1 is known to inhibit renal cell apoptosis, inflammation, and fibrosis and therefore could be considered as a potential therapeutic target. A number of studies suggest that SIRT1 has a cytoprotective effect by reducing cell apoptosis, senescence, and by inhibiting inflammation (32, 53). SIRT1 has anti-fibrosis effect in CKD by interacting with TGF-β1 signaling (32). SIRT1 overexpression abolishes TGF-β1-induced kidney cell apoptosis and renal fibrosis, via suppression of CTGF expression (54). NF-κB can regulate senescence and cell cycle-specific gene expression (55). SIRT1 can interact directly with NF-kB p65, resulting in p65 deacetylation and NF-κB inactivation (56). Interestingly, the study indicates that SIRT1 and NF-kB regulates each other in a positive feedback loop (57). These studies suggest that SIRT1 activators should have multiple beneficial effects in kidney cells including anti-apoptosis, anti-inflammation, and anti-senescence. Therefore, Sirt1 activators could be developed as a new drug to treat patients with CKD. In addition, SIRT1 attenuates development of hyperphosphatemia-related vascular calcification via inhibiting cell senescence and osteogenic phenotype switching of VSMCs and therefore activation of SIRT1 or restauration of Sirt1 expression could be a reasonable approach to reduce hyperphosphatemia-induced medial calcification in CKD patients (38).
However, most of the studies are performed in Sirt1 deficient animals and these studies can only show the association of Sirt1 deficiency with kidney disease. In order to show whether increased Sirt1 expression after onset of CKD has any beneficial effects, we generated an inducible Sirt1 overexpression mouse mode. In these mice, we show that induction of Sirt1 expression after disease onset still has renal protective effects in mice with DKD and HIV kidney disease (25, 48). In addition, we show that BF175, a novel Sirt1 specific agonist, also reduces kidney injury in these animal models. Our studies suggest that targeting Sirt1 could be a potential new therapy for CKD (25, 48).
Clinical Perspectives
In summary, SIRT1 induces deacetylation of several key transcriptional factors in regulation of cell apoptosis, senescence, inflammation and fibrosis in the context of CKD. SIRT1 activators could be developed to inhibit the apoptosis and senescence of kidney cells, reduce renal inflammation and oxidative stress, improve mitochondrial function, and reduce renal fibrosis. Therefore, SIRT1 activators could be considered as a new therapy to prevent the development and progression of CKD. In addition, SIRT1 has protective effects against vascular calcification which is a major complication of CKD. Therefore, SIRT1 activators should have beneficial effects for both CKD and its complication.
Author Contributions
All authors listed have made a substantial, direct, and intellectual contribution to the work, and approved it for publication.
Funding
YZ is supported by National Natural Science Foundation of China (2019-81973772).
Conflict of Interest
The authors declare that the research was conducted in the absence of any commercial or financial relationships that could be construed as a potential conflict of interest.
Publisher’s Note
All claims expressed in this article are solely those of the authors and do not necessarily represent those of their affiliated organizations, or those of the publisher, the editors and the reviewers. Any product that may be evaluated in this article, or claim that may be made by its manufacturer, is not guaranteed or endorsed by the publisher.
References
1. National Kidney Foundation.. K/DOQI Clinical Practice Guidelines for Chronic Kidney Disease: Evaluation, Classification, and Stratification. Am J Kidney Dis (2002) 39:S1–266.
2. Yamaguchi J, Tanaka T, Nangaku M. Recent Advances in Understanding of Chronic Kidney Disease. F1000Research 4 (2015) 4:F1000 Faculty Rev-1212. doi: 10.12688/f1000research.6970.1
3. Liu Y. Cellular and Molecular Mechanisms of Renal Fibrosis. Nat Rev Nephrol (2011) 7:684–96. doi: 10.1038/nrneph.2011.149
4. Li J, Qu X, Ricardo SD, Bertram JF, Nikolic-Paterson DJ. Resveratrol Inhibits Renal Fibrosis in the Obstructed Kidney: Potential Role in Deacetylation of Smad3. Am J Pathol (2010) 177:1065–71. doi: 10.2353/ajpath.2010.090923
5. Bordone L, Guarente L. Calorie Restriction, SIRT1 and Metabolism: Understanding Longevity. Nat Rev Mol Cell Biol (2005) 6:298–305. doi: 10.1038/nrm1616
6. Buhrmann C, Busch F, Shayan P, Shakibaei M. Sirtuin-1 (SIRT1) Is Required for Promoting Chondrogenic Differentiation of Mesenchymal Stem Cells. J Biol Chem (2014) 289:22048–62. doi: 10.1074/jbc.M114.568790
7. Gorenne I, Kumar S, Gray K, Figg N, Yu H, Mercer J, et al. Vascular Smooth Muscle Cell Sirtuin 1 Protects Against DNA Damage and Inhibits Atherosclerosis. Circulation (2013) 127:386–96. doi: 10.1161/CIRCULATIONAHA.112.124404
8. Hsu YJ, Hsu SC, Hsu CP, Chen YH, Chang YL, Sadoshima J, et al. Sirtuin 1 Protects the Aging Heart From Contractile Dysfunction Mediated Through the Inhibition of Endoplasmic Reticulum Stress-Mediated Apoptosis in Cardiac-Specific Sirtuin 1 Knockout Mouse Model. Int J Cardiol (2017) 228:543–52. doi: 10.1016/j.ijcard.2016.11.247
9. Liu X, Chen A, Liang Q, Yang X, Dong Q, Fu M, et al. Spermidine Inhibits Vascular Calcification in Chronic Kidney Disease Through Modulation of SIRT1 Signaling Pathway. Aging Cell (2021) 20:e13377. doi: 10.1111/acel.13377
10. Dong YJ, Liu N, Xiao Z, Sun T, Wu SH, Sun WX, et al. Renal Protective Effect of Sirtuin 1. J Diabetes Res (2014) 2014:843786. doi: 10.1155/2014/843786
11. Vaquero A, Scher M, Lee D, Erdjument-Bromage H, Tempst P, Reinberg D. Human SirT1 Interacts With Histone H1 and Promotes Formation of Facultative Heterochromatin. Mol Cell (2004) 16:93–105. doi: 10.1016/j.molcel.2004.08.031
12. North BJ, Marshall BL, Borra MT, Denu JM, Verdin E. The Human Sir2 Ortholog, SIRT2, Is an NAD+-Dependent Tubulin Deacetylase. Mol Cell (2003) 11:437–44. doi: 10.1016/S1097-2765(03)00038-8
13. Lombard DB, Alt FW, Cheng HL, Bunkenborg J, Streeper RS, Mostoslavsky R, et al. Mammalian Sir2 Homolog SIRT3 Regulates Global Mitochondrial Lysine Acetylation. Mol Cell Biol (2007) 27:8807–14. doi: 10.1128/MCB.01636-07
14. Zhu X, Liu Q, Wang M, Liang M, Yang X, Xu X, et al. Activation of Sirt1 by Resveratrol Inhibits TNF-Alpha Induced Inflammation in Fibroblasts. PLoS One (2011) 6:e27081. doi: 10.1371/journal.pone.0027081
15. Langley E, Pearson M, Faretta M, Bauer UM, Frye RA, Minucci S, et al. Human SIR2 Deacetylates P53 and Antagonizes PML/p53-Induced Cellular Senescence. EMBO J (2002) 21:2383–96. doi: 10.1093/emboj/21.10.2383
16. Cheng HL, Mostoslavsky R, Saito S, Manis JP, Gu Y, Patel P, et al. Developmental Defects and P53 Hyperacetylation in Sir2 Homolog (SIRT1)-Deficient Mice. Proc Natl Acad Sci USA (2003) 100:10794–9. doi: 10.1073/pnas.1934713100
17. Kida Y, Zullo JA, Goligorsky MS. Endothelial Sirtuin 1 Inactivation Enhances Capillary Rarefaction and Fibrosis Following Kidney Injury Through Notch Activation. Biochem Biophys Res Commun (2016) 478:1074–9. doi: 10.1016/j.bbrc.2016.08.066
18. Vasko R, Xavier S, Chen J, Lin CH, Ratliff B, Rabadi M, et al. Endothelial Sirtuin 1 Deficiency Perpetrates Nephrosclerosis Through Downregulation of Matrix Metalloproteinase-14: Relevance to Fibrosis of Vascular Senescence. J Am Soc Nephrol JASN (2014) 25:276–91. doi: 10.1681/ASN.2013010069
19. Simic P, Williams EO, Bell EL, Gong JJ, Bonkowski M, Guarente L. SIRT1 Suppresses the Epithelial-to-Mesenchymal Transition in Cancer Metastasis and Organ Fibrosis. Cell Rep (2013) 3:1175–86. doi: 10.1016/j.celrep.2013.03.019
20. Liang J, Tian S, Han J, Xiong P. Resveratrol as a Therapeutic Agent for Renal Fibrosis Induced by Unilateral Ureteral Obstruction. Renal Fail (2014) 36:285–91. doi: 10.3109/0886022X.2013.844644
21. Huang XZ, Wen D, Zhang M, Xie Q, Ma L, Guan Y, et al. Sirt1 Activation Ameliorates Renal Fibrosis by Inhibiting the TGF-Beta/Smad3 Pathway. J Cell Biochem (2014) 115:996–1005. doi: 10.1002/jcb.24748
22. Wang M, Yang L, Yang J, Wang C. Shen Shuai IIRecipe Attenuates Renal Injury and Fibrosis in Chronic Kidney Disease by Regulating NLRP3 Inflammasome and Sirt1/Smad3 Deacetylation Pathway. BMC Complement Altern Med (2019) 19:107. doi: 10.1186/s12906-019-2524-6
23. Liu R, Zhong Y, Li X, Chen H, Jim B, Zhou MM, et al. Role of Transcription Factor Acetylation in Diabetic Kidney Disease. Diabetes (2014) 63:2440–53. doi: 10.2337/db13-1810
24. Chuang PY, Xu J, Dai Y, Jia F, Mallipattu SK, Yacoub R, et al. In Vivo RNA Interference Models of Inducible and Reversible Sirt1 Knockdown in Kidney Cells. Am J Pathol (2014) 184:1940–56. doi: 10.1016/j.ajpath.2014.03.016
25. Wang X, Liu R, Zhang W, Hyink DP, Das GC, Das B, et al. Role of SIRT1 in HIV-Associated Kidney Disease. Am J Physiol Renal Physiol (2020) 319:F335–44. doi: 10.1152/ajprenal.00140.2020
26. Chuang PY, Dai Y, Liu R, He H, Kretzler M, Jim B, et al. Alteration of Forkhead Box O (Foxo4) Acetylation Mediates Apoptosis of Podocytes in Diabetes Mellitus. PLoS One (2011) 6:e23566. doi: 10.1371/journal.pone.0023566
27. Liu Y, Bi X, Xiong J, Han W, Xiao T, Xu X, et al. MicroRNA-34a Promotes Renal Fibrosis by Downregulation of Klotho in Tubular Epithelial Cells. Mol Ther (2019) 27:1051–65. doi: 10.1016/j.ymthe.2019.02.009
28. Zeng L, Chen R, Liang F, Tsuchiya H, Murai H, Nakahashi T, et al. Silent Information Regulator, Sirtuin 1, and Age-Related Diseases. Geriatr Gerontol Int (2009) 9:7–15. doi: 10.1111/j.1447-0594.2008.00504.x
29. Braidy N, Guillemin GJ, Mansour H, Chan-Ling T, Poljak A, Grant R. Age Related Changes in NAD+ Metabolism Oxidative Stress and Sirt1 Activity in Wistar Rats. PLoS One (2011) 6:e19194. doi: 10.1371/journal.pone.0019194
30. Hori YS, Kuno A, Hosoda R, Horio Y. Regulation of FOXOs and P53 by SIRT1 Modulators Under Oxidative Stress. PLoS One (2013) 8:e73875. doi: 10.1371/journal.pone.0073875
31. Jin Q, Jhun BS, Lee SH, Lee J, Pi Y, Cho YH, et al. Differential Regulation of Phosphatidylinositol 3-Kinase/Akt, Mitogen-Activated Protein Kinase, and AMP-Activated Protein Kinase Pathways During Menadione-Induced Oxidative Stress in the Kidney of Young and Old Rats. Biochem Biophys Res Commun (2004) 315:555–61. doi: 10.1016/j.bbrc.2004.01.093
32. Kume S, Haneda M, Kanasaki K, Sugimoto T, Araki S, Isshiki K, et al. SIRT1 Inhibits Transforming Growth Factor Beta-Induced Apoptosis in Glomerular Mesangial Cells via Smad7 Deacetylation. J Biol Chem (2007) 282:151–8. doi: 10.1074/jbc.M605904200
33. Shanahan CM. Mechanisms of Vascular Calcification in CKD-Evidence for Premature Ageing? Nat Rev Nephrol (2013) 9:661–70. doi: 10.1038/nrneph.2013.176
34. Kang X, Yang W, Wang R, Xie T, Li H, Feng D, et al. Sirtuin-1 (SIRT1) Stimulates Growth-Plate Chondrogenesis by Attenuating the PERK-eIF-2alpha-CHOP Pathway in the Unfolded Protein Response. J Biol Chem (2018) 293:8614–25. doi: 10.1074/jbc.M117.809822
35. Lee GL, Yeh CC, Wu JY, Lin HC, Wang YF, Kuo YY, et al. TLR2 Promotes Vascular Smooth Muscle Cell Chondrogenic Differentiation and Consequent Calcification via the Concerted Actions of Osteoprotegerin Suppression and IL-6-Mediated RANKL Induction. Arterioscler Thromb Vasc Biol (2019) 39:432–45. doi: 10.1161/ATVBAHA.118.311874
36. Li Z, Wu J, Zhang X, Ou C, Zhong X, Chen Y, et al. CDC42 Promotes Vascular Calcification in Chronic Kidney Disease. J Pathol (2019) 249:461–71. doi: 10.1002/path.5334
37. Pescatore LA, Gamarra LF, Liberman M. Multifaceted Mechanisms of Vascular Calcification in Aging. Arterioscler Thromb Vasc Biol (2019) 39:1307–16. doi: 10.1161/ATVBAHA.118.311576
38. Takemura A, Iijima K, Ota H, Son BK, Ito Y, Ogawa S, et al. Sirtuin 1 Retards Hyperphosphatemia-Induced Calcification of Vascular Smooth Muscle Cells. Arterioscler Thromb Vasc Biol (2011) 31:2054–62. doi: 10.1161/ATVBAHA.110.216739
39. Akiyoshi T, Ota H, Iijima K, Son BK, Kahyo T, Setou M, et al. A Novel Organ Culture Model of Aorta for Vascular Calcification. Atherosclerosis (2016) 244:51–8. doi: 10.1016/j.atherosclerosis.2015.11.005
40. Bartoli-Leonard F, Wilkinson FL, Schiro A, Inglott FS, Alexander MY, Weston R. Suppression of SIRT1 in Diabetic Conditions Induces Osteogenic Differentiation of Human Vascular Smooth Muscle Cells via RUNX2 Signalling. Sci Rep (2019) 9:878. doi: 10.1038/s41598-018-37027-2
41. Badi I, Mancinelli L, Polizzotto A, Ferri D, Zeni F, Burba I, et al. miR-34a Promotes Vascular Smooth Muscle Cell Calcification by Downregulating SIRT1 (Sirtuin 1) and Axl (AXL Receptor Tyrosine Kinase). Arterioscler Thromb Vasc Biol (2018) 38:2079–90. doi: 10.1161/ATVBAHA.118.311298
42. Mattison JA, Wang M, Bernier M, Zhang J, Park SS, Maudsley S, et al. Resveratrol Prevents High Fat/Sucrose Diet-Induced Central Arterial Wall Inflammation and Stiffening in Nonhuman Primates. Cell Metab (2014) 20:183–90. doi: 10.1016/j.cmet.2014.04.018
43. Tomayko EJ, Cachia AJ, Chung HR, Wilund KR. Resveratrol Supplementation Reduces Aortic Atherosclerosis and Calcification and Attenuates Loss of Aerobic Capacity in a Mouse Model of Uremia. J Med Food (2014) 17:278–83. doi: 10.1089/jmf.2012.0219
44. Zykova TA, Zhu F, Zhai X, Ma WY, Ermakova SP, Lee KW, et al. Resveratrol Directly Targets COX-2 to Inhibit Carcinogenesis. Mol Carcinog (2008) 47:797–805. doi: 10.1002/mc.20437
45. Higashida K, Kim SH, Jung SR, Asaka M, Holloszy JO, Han DH. Effects of Resveratrol and SIRT1 on PGC-1alpha Activity and Mitochondrial Biogenesis: A Reevaluation. PLoS Biol (2013) 11:e1001603. doi: 10.1371/journal.pbio.1001603
46. Kortylewski M, Swiderski P, Herrmann A, Wang L, Kowolik C, Kujawski M, et al. In Vivo Delivery of siRNA to Immune Cells by Conjugation to a TLR9 Agonist Enhances Antitumor Immune Responses. Nat Biotechnol (2009) 27:925–32. doi: 10.1038/nbt.1564
47. Han Y, Sun W, Ren D, Zhang J, He Z, Fedorova J, et al. SIRT1 Agonism Modulates Cardiac NLRP3 Inflammasome Through Pyruvate Dehydrogenase During Ischemia and Reperfusion. Redox Biol (2020) 34:101538. doi: 10.1016/j.redox.2020.101538
48. Hong Q, Zhang L, Das B, Li Z, Liu B, Cai G, et al. Increased Podocyte Sirtuin-1 Function Attenuates Diabetic Kidney Injury. Kidney Int (2018) 93(6):1330–43. doi: 10.1016/j.kint.2017.12.008
49. Ota H, Akishita M, Eto M, Iijima K, Kaneki M, Ouchi Y. Sirt1 Modulates Premature Senescence-Like Phenotype in Human Endothelial Cells. J Mol Cell Cardiol (2007) 43:571–9. doi: 10.1016/j.yjmcc.2007.08.008
50. Wan YZ, Gao P, Zhou S, Zhang ZQ, Hao DL, Lian LS, et al. SIRT1-Mediated Epigenetic Downregulation of Plasminogen Activator Inhibitor-1 Prevents Vascular Endothelial Replicative Senescence. Aging Cell (2014) 13:890–9. doi: 10.1111/acel.12247
51. Lu CL, Liao MT, Hou YC, Fang YW, Zheng CM, Liu WC, et al. Sirtuin-1 and Its Relevance in Vascular Calcification. Int J Mol Sci (2020) 21(5):1593. doi: 10.3390/ijms21051593
52. Wang J, Li S, Wang J, Wu F, Chen Y, Zhang H, et al. Spermidine Alleviates Cardiac Aging by Improving Mitochondrial Biogenesis and Function. Aging (2020) 12:650–71. doi: 10.18632/aging.102647
53. Yang SR, Wright J, Bauter M, Seweryniak K, Kode A, Rahman I. Sirtuin Regulates Cigarette Smoke-Induced Proinflammatory Mediator Release via RelA/p65 NF-kappaB in Macrophages In Vitro and in Rat Lungs In Vivo: Implications for Chronic Inflammation and Aging. Am J Physiol Lung Cell Mol Physiol (2007) 292:L567–76. doi: 10.1152/ajplung.00308.2006
54. Ren Y, Du C, Yan L, Wei J, Wu H, Shi Y, et al. CTGF siRNA Ameliorates Tubular Cell Apoptosis and Tubulointerstitial Fibrosis in Obstructed Mouse Kidneys in a Sirt1-Independent Manner. Drug Design Dev Ther (2015) 9:4155–71. doi: 10.2147/DDDT.S86748
55. Adler AS, Sinha S, Kawahara TL, Zhang JY, Segal E, Chang HY. Motif Module Map Reveals Enforcement of Aging by Continual NF-kappaB Activity. Genes Dev (2007) 21:3244–57. doi: 10.1101/gad.1588507
56. Shimokawa I, Higami Y, Tsuchiya T, Otani H, Komatsu T, Chiba T, et al. Life Span Extension by Reduction of the Growth Hormone-Insulin-Like Growth Factor-1 Axis: Relation to Caloric Restriction. FASEB J (2003) 17:1108–9. doi: 10.1096/fj.02-0819fje
Keywords: kidney, SIRT1, vascular calcification, chronic kidney disease, HIV infection
Citation: Yan J, Wang J, He JC and Zhong Y (2022) Sirtuin 1 in Chronic Kidney Disease and Therapeutic Potential of Targeting Sirtuin 1. Front. Endocrinol. 13:917773. doi: 10.3389/fendo.2022.917773
Received: 11 April 2022; Accepted: 12 May 2022;
Published: 20 June 2022.
Edited by:
Yang Yang, Northwest University, ChinaReviewed by:
Sergey Dikalov, Vanderbilt University, United StatesValeria Conti, University of Salerno, Italy
Copyright © 2022 Yan, Wang, He and Zhong. This is an open-access article distributed under the terms of the Creative Commons Attribution License (CC BY). The use, distribution or reproduction in other forums is permitted, provided the original author(s) and the copyright owner(s) are credited and that the original publication in this journal is cited, in accordance with accepted academic practice. No use, distribution or reproduction is permitted which does not comply with these terms.
*Correspondence: Yifei Zhong, eWlmZWlsaWx5QDEyNi5jb20=; John Cijiang He, Q2lqaWFuZy5oZUBtc3NtLmVkdQ==