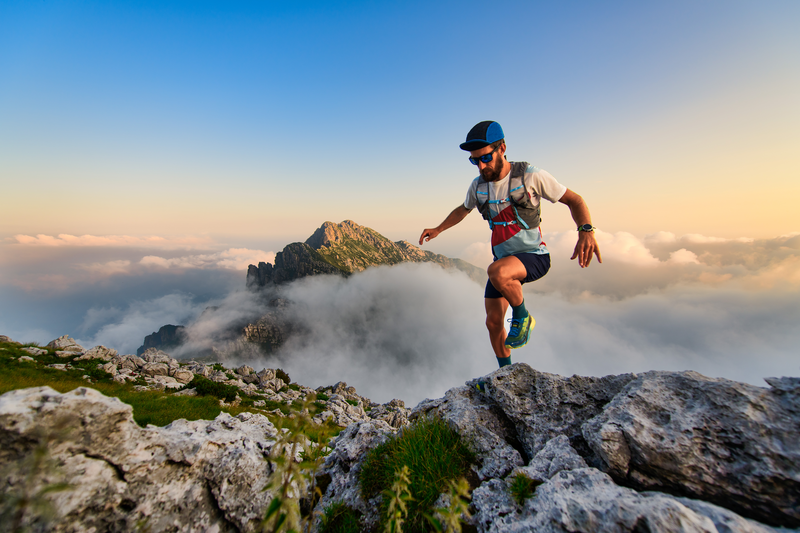
95% of researchers rate our articles as excellent or good
Learn more about the work of our research integrity team to safeguard the quality of each article we publish.
Find out more
REVIEW article
Front. Endocrinol. , 30 May 2022
Sec. Thyroid Endocrinology
Volume 13 - 2022 | https://doi.org/10.3389/fendo.2022.896287
This article is part of the Research Topic Targeted Therapy in Advanced Thyroid Cancer View all 9 articles
The treatment options that are currently available for management of metastatic, progressive radioactive iodine (RAI)-refractory differentiated thyroid cancers (DTCs), and medullary thyroid cancers (MTCs) are limited. While there are several systemic targeted therapies, such as tyrosine kinase inhibitors, that are being evaluated and implemented in the treatment of these cancers, such therapies are associated with serious, sometimes life-threatening, adverse events. Peptide receptor radionuclide therapy (PRRT) has the potential to be an effective and safe modality for treating patients with somatostatin receptor (SSTR)+ RAI-refractory DTCs and MTCs. MTCs and certain sub-types of RAI-refractory DTCs, such as Hürthle cell cancers which are less responsive to conventional modalities of treatment, have demonstrated a favorable response to treatment with PRRT. While the current literature offers hope for utilization of PRRT in thyroid cancer, several areas of this field remain to be investigated further, especially head-to-head comparisons with other systemic targeted therapies. In this review, we provide a comprehensive outlook on the current translational and clinical data on the use of various PRRTs, including diagnostic utility of somatostatin analogs, theranostic properties of PRRT, and the potential areas for future research.
Peptide receptor radionuclide therapy (PRRT) has emerged as one of the most pivotal modalities of treatment for neuroendocrine tumors (NETs). PRRT is a form of targeted therapy in which a radiolabeled peptide is used as a vector to deliver cytotoxic doses of radiation to those cancer cells which abundantly express receptors for that particular peptide (1). To date, several forms of radiolabeled peptides have been utilized for diagnostic purposes based on tumor receptor expression profiles, including somatostatin, cholecystokinin (CCK), neuropeptide-Y, gastrin, glucagon-like peptide-1, and others (2). However, the vast majority of PRRTs are radionuclides tagged to somatostatin analogs (SSAs), which target somatostatin receptors (SSTRs). While PRRT has been vastly utilized in the treatment of NETs, one of the actively investigated fields is the utility of PRRT in the treatment of thyroid cancer. In this review, we discuss the current standard-of-care for the management of various forms of thyroid cancer and then, discuss the utility of PRRT in oncology, along with the current evidence of SSA-based diagnostic imaging and PRRT in thyroid cancer. We also discuss the potential applicability of theranostics and future directions in the utility of PRRT as a crucial form of targeted therapy in thyroid cancer.
The yearly incidence of thyroid cancer has nearly tripled from 4.9 per 100,000 individuals in 1975 to 14.3 per 100,000 individuals in 2009 (3). Differentiated thyroid cancer (DTC), comprised of papillary thyroid cancer (PTC), and follicular thyroid cancer (FTC) along with the Hürthle cell subtype of thyroid cancer (HTC), constitutes over 90% of all thyroid cancers (3). Medullary thyroid cancer (MTC) and anaplastic thyroid cancer (ATC) are much rarer but carry a higher mortality risk compared to DTC (4, 5).
The conventional treatment for DTC is thyroidectomy (hemi- or total thyroidectomy depending on the extent of the disease) with or without central and/or lateral lymph node dissection. Following surgery, radioactive iodine (RAI) therapy with iodine-131 (131I) is generally considered for those DTCs that have an intermediate-to-high risk for structural disease recurrence (3). For local recurrence and for distant metastases, repeat surgery and/or RAI therapy remains the preferred modality of treatment, and most RAI-avid DTCs have a favorable prognosis despite the presence of metastatic disease (3). However, up to 22% of DTCs may become RAI-refractory due to their inability to concentrate iodine into the tumor cells, and certain DTC subtypes such as tall-cell and diffuse sclerosing variants of PTC, insular variant of FTC, HTC, and poorly differentiated thyroid cancers (PDTCs) have a propensity to be RAI-refractory (3). In these circumstances, RAI is not effective, and diagnostic imaging is performed using 18-fluorodeoxyglucose positron emission tomography computed tomography (18FDG-PET/CT). In general, the more differentiated forms of DTC tend to retain iodine-concentrating capacity, are metabolically less active, more RAI-avid, and less 18FDG-PET-avid, while the more dedifferentiated forms are hypermetabolic, less RAI-avid, and more 18FDG-PET-avid (6). Local treatment options for RAI-refractory DTCs include external beam radiation, radiofrequency ablation or cryoablation. Molecular characterization of DTCs (7), and the advent of widespread molecular testing has allowed for identification of several potential targets for therapy. The tyrosine kinase inhibitors (TKIs) lenvatinib and sorafenib have been approved by the Food and Drug Administration (FDA) in the United States for the treatment of progressive, RAI-refractory metastatic DTC (8, 9). Other systemic therapies such as proto-oncogene serine/threonine-protein kinase (BRAF) inhibitors (dabrafenib), mammalian target of rapamycin (mTOR) inhibitors (everolimus), neurotrophic receptor tyrosine kinase (NTRK) inhibitors and immune checkpoint inhibitors (ICIs) are being evaluated in several clinical trials (10, 11). Recently, re-differentiation and restoration of iodine-concentrating capacity within RAI-refractory tumors by using mitogen-activated protein kinase kinase (MEK) inhibitors (trametinib and selumetinib) is being actively investigated as another promising modality of therapy (12, 13). Selumetinib has been granted an orphan drug designation by the FDA as an agent re-inducing RAI uptake in advanced DTC. Certain forms of DTCs such as HTCs, and PDTCs are rare and data on the utility of targeted therapy in these thyroid cancer subtypes is limited, although there is recent data emerging on long-term remission noted in PDTCs with the use of combination of lenvatinib (TKI) and pembrolizumab (ICI) (14, 15).
MTC is considered as a type of NET and is derived from the parafollicular C-cells (4). The prevalence of MTC is 1% – 2% in the United States (4). MTC can be sporadic or can manifest as a part of multiple endocrine neoplasia 2A and 2B. As the C-cells do not have the ability to concentrate iodine, MTCs are RAI-refractory, and the major functional imaging of utility is the 18FDG-PET/CT (4). The conventional treatment for MTC is thyroidectomy and lymph node dissection (4). Other treatments include external beam radiation for recurrent disease in the neck, surgical resection, or chemoembolization of hepatic metastases, and systemic therapy for progressive, metastatic MTC (4). Initially, two TKIs, vandetanib and cabozantinib, demonstrated efficacy in improving progression-free survival (PFS) in MTC and are now approved by the FDA for the treatment of progressive metastatic MTC (16, 17). Recently, two selective rearranged during transfection (RET) inhibitors, selpercatinib and pralsetinib have been approved for the treatment of progressive metastatic MTC, and these agents harbor better adverse event (AE) profiles (18, 19). Several other targeted therapies, including ICIs and carcinoembryonic antigen (CEA) vaccine are also being investigated for the treatment of this condition (11).
ATC is the most aggressive form of thyroid cancer, with a prevalence of 1.7% in the United States and a median prevalence of 3.6% worldwide (5). The management of ATC is more complicated due to the rapid progression and poor prognosis of the disease. For potential surgical resection of the primary tumor, several factors should be considered, including patient’s age, functional status, extent of the disease, status of airway, use of neoadjuvant or adjuvant therapy, whether the surgery is curative, palliative, or for prevention of complications such as obstruction of trachea and/or esophagus, and the goals of care of the patients, with timely involvement of palliative care (5). Neoadjuvant or adjuvant chemoradiation should be implemented as soon as possible after careful consideration of risks and benefits (5). Some of the available chemotherapy regimens include paclitaxel/carboplatin and docetaxel/doxorubicin, and their use is recommended in patients treated with definitive-intention radiation (5). In patients who have undergone R0 (negative microscopic and gross tumor margin) or R1 (negative gross tumor margin) surgical resection, or in patients with R2 resection (positive gross tumor margin/debulking procedure) with good performance status, intensity-modulated radiation with systemic therapy is recommended (5). However, the overall survival (OS), and disease-free survival is not different among R0, R1, and R2 statuses (20). In BRAF V600E-mutated ATCs, a combination of BRAF and MEK inhibitors can be utilized. The BRAF/MEK inhibitor combination of dabrafenib and trametinib is approved by the FDA for the treatment of BRAF V600E-mutated ATCs based on the substantial response rate observed in a phase II, open label trial (5, 21). Anaplastic lymphoma kinase (ALK) inhibitors, NTRK inhibitors, mTOR inhibitors, ICIs and TKIs (particularly the combination of lenvatinib and pembrolizumab) are some of the other targeted therapies with potential utility in ATC (5, 15, 22, 23).
Despite the availability of several local and systemic treatment options for thyroid cancers, metastatic/inoperable forms of RAI-refractory DTCs, HTCs, PDTCs, and MTCs continue to pose a challenge for optimal management. This is either due to suboptimal and variable response to treatment, or due to severe or unacceptable AEs or toxicities associated with several of these systemic therapies. Some of the common AEs associated with TKIs used for thyroid cancer include nausea, diarrhea, anorexia, fatigue, mucositis, hypertension, cardiac failure, rash, palmar-plantar erythrodysesthesia, skin pigmentary changes, alopecia, and aerodigestive fistula formation (24–27). The AE profile of selective RET inhibitors is similar to that of TKIs, with common AEs observed being neutropenia, hepatic transaminase elevation, constipation, diarrhea, hypertension, and rash (28). Some of the BRAF and MEK inhibitor-associated AEs include hypertension, reduced cardiac ejection fraction, cardiomyopathy, fever, diarrhea, rash, and ocular AEs such as central serous retinopathy (29–31). Therefore, there is a need for a systemic therapy that is not only efficacious, but also has lower number of AEs. PRRT can potentially serve as a systemic targeted therapy in thyroid cancer due to its better safety profile and high treatment efficacy demonstrated in several other forms of tumors (32–35).
Somatostatin, also known as somatotropin release inhibiting factor (SRIF) is a cyclic peptide hormone that has several complex endocrine, paracrine, and modulatory effects across different organ systems, including inhibition of secretion of various hormones and enzymes and cell proliferation, and also functions as a neurotransmitter and as an immunomodulator (36–39). Somatostatin was initially isolated from ovine hypothalamus as a peptide capable of inhibiting the release of growth hormone (40). Further studies have identified the presence of somatostatin in almost every tissue of the body (36, 41, 42). The two active forms of somatostatin are SRIF-14, which contains 14 amino acids and SRIF-28, which has 24 amino acids (43). Somatostatin is known to inhibit the secretion of not only growth hormone, but also of prolactin, thyroid stimulating hormone, adrenocorticotropic hormone, insulin, glucagon, and gastrointestinal hormones such as CCK, gastrin, ghrelin, secretin, and vasoactive intestinal polypeptide (38, 41, 44). Somatostatin also inhibits gastrointestinal exocrine function, including production of gastric, pancreatic, and intestinal digestive fluids and enzymes (38). Therefore, it has been recommended for routine use in patients undergoing pancreatic surgeries (45, 46).
SSTRs are a family of seven-subunit transmembrane G protein-coupled receptors, with five distinct subtypes being identified in humans, namely SSTR 1, 2, 3, 4, and 5 (37). The SSTR genes for each subtype are located on different chromosomes, and SSTR2 has two splice variants (SSTR2A and SSTR2B) (38). These SSTRs are vastly and variably expressed in different normal tissues as well as in various benign and malignant tumors in humans, but several forms of tumors seem to predominantly express SSTR2 and sometimes SSTR1, 3 or 5, while SSTR4 expression is rare (38, 39, 47, 48).
SRIF-14 and SRIF-28 bind to all five subtypes of SSTRs with nanomolar affinity (38). Furthermore, based on structural homology, the SSTRs are classified into two sub-groups, the SRIF1, which includes SSTRs 2, 3, and 5, and SRIF2, which includes SSTRs 1 and 4 (38). The synthetic SSAs such as octreotide and seglitide demonstrate binding affinity to SRIF1 receptors, with high affinity to SSTR2 and intermediate affinity to SSTR 1 and 5, while no such binding affinity is demonstrated towards SRIF2 receptors (49). Activation of all SSTR subtypes decreases adenylyl cyclase activity, and promotes variable effects on the activities of phospholipase C, mitogen-activated protein kinase, calcium and potassium channels, and phosphotyrosine phosphatase (38).
Radiolabeled SSAs have emerged as some of the most widely used theranostic agents. Theranostics can be defined as the utilization of materials that can serve both as modalities of diagnostic imaging and as treatment (50). By combining diagnostics and therapeutics, theranostic agents harbor the advantage of sharing the same biodistribution and receptor selectivity that overcomes the potential disparity that may be encountered with using two different agents for imaging and treatment. The radionuclides tagged with the SSAs may serve a diagnostic or therapeutic purpose based on the type of the emitted radiation. Diagnostic imaging can be achieved by utilizing SSAs tagged with positron emitters such as gallium-68 (68Ga), copper-64 (64Cu) or fluorine-18 (18F) which can be imaged using positron (β+) emission tomography (PET), or with γ-emitters such as indium-111 (111In) or technetium-99 (99Tc) which can be imaged using single photon emission computed tomography (SPECT) (50). SSAs tagged with β-emitters such as lutetium-177 (177Lu) and yttrium-90 (90Y) or α-emitters such as lead-212 (212Pb), bismuth-213 (213Bi), and actinium-225 (225Ac) serve as therapeutic agents (PRRT) by destroying the targeted tumor cells (50). These radionuclides are chelated within various chelators such as DOTA (1,4,7,10-tetraazacyclododecane-1,4,7,20-tetra-acetic acid), DTPA (diethylenetriamine penta-acetic acid), or NODAGA (1,4,7-triazacyclononane,1-glutaric acid-4,7-acetic acid) and are ‘tagged’ to the SSAs. Earlier forms of PRRT comprised of conventional octreotide as the SSA. Later developments, such as modification of octreotide with insertion of tyrosine in the 3rd position (Tyr3-OC or TOC), or replacing the c-terminal amino alcohol, threoninol, with threonine (TATE) improves the SSA’s binding affinity to SSTR2 subtype (51). Some of the common chelator-SSA combinations include [DOTA0,Tyr3]octreotate (DOTATATE), [DOTA0,Tyr3]octreotide (DOTATOC), and [DOTA0-1-NaI3]octreotide (DOTA-NOC) (35). The individual components of a radiolabeled SSA analog have been delineated in Figure 1. So far, 177Lu-SSAs, especially 177Lu-DOTATATE has been the most commonly utilized form of PRRT. Certain radiolabeled SSAs such as 111In-DTPA-octreotide (111In-pentetreotide) may not be as effective for PRRT when compared to 177Lu- or 90Y-based SSAs as the latter two radionuclides are β-emitters which are long-range electron emitters with better cytolytic potential and irradiation of the environment neighboring the tumor, while 111In is an Auger electron emitter which has short-range low-energy electron emission (51).
Figure 1 Components of a radiolabeled somatostatin analog with individual examples of each component.
SSTRs are vastly overexpressed particularly in NETs; tumors that arise from neuroendocrine cells and/or neural crest cells (52). These cells are present in several organs, including the bronchi, pancreas, and the gastrointestinal tract. Based on the site of origin, NETs can be pancreatic NETs (PNETs), gastroenteropancreatic NETs (GEP-NETs), carcinoids, small cell lung cancer, or large cell neuroendocrine carcinoma (53). Other tumors of neuroendocrine origin include pheochromocytoma and paraganglioma (PPGL), MTC, neuroblastoma, meningioma, and Merkel cell carcinoma (53). In a landmark phase 3 clinical trial (NETTER-1), 177Lu-DOTATATE therapy in addition to long-acting repeatable (LAR) octreotide in patients with SSTR+ well-differentiated, metastatic midgut NETs resulted in substantially longer PFS compared to treatment with LAR octreotide alone (33). Based on the data from the NETTER-1 trial, the United States FDA approved 177Lu-DOTATATE in January 2018 for the treatment of SSTR+ GEP-NETs. The treatment was also approved in Europe based on the data from NETTER-1, as well as based on safety and efficacy data from the phase I/II ERASMUS study (34). In addition, PRRT has been effective in treating several other types of NETs, including PPGL (32, 54–60). The safety profile of PRRT is favorable and the treatment is generally well-tolerated. The most common AEs include nausea (65% all grades and 5% grades 3 and 4 in NETTER-1) and vomiting (53% all grades and 2% grades 3 and 4 in NETTER-1) which is mainly attributed to the renoprotective amino acid infusion that is concurrently administered with PRRT (61). This is managed with pre-infusion anti-emetic administration. Hematologic dysfunction, including transient myelosuppression, cytopenia (anemia: 81%, thrombocytopenia: 53%, neutropenia: 26% per NETTER-1 trial data, all grade 1 or 2), myelodysplastic syndrome (2.7% in NETTER-1, and 2% [median time to development: 28 months] in ERASMUS), and leukemia (0.5% [median time to development: 55 months] in ERASMUS) have been observed. Renal toxicity is of concern and <1% of patients in NETTER-1 trial developed renal failure, and this AE can be potentially prevented with amino acid infusion pre- and post-177Lu-DOTATATE administration. Other potential AEs include abdominal pain, constipation, diarrhea, hepatic dysfunction, hyperglycemia, electrolyte disturbances, and neurohormonal crises in cases of NETs producing biologically active products (33, 34). The principle of PRRT and the mechanism of action of radiolabeled SSAs has been illustrated in Figure 2.
Figure 2 An illustration of the principles of utility of radiolabeled somatostatin analogs in the management of thyroid cancer, along with the mechanisms of action and the theranostic properties of peptide receptor radionuclide therapy. Image created on Biorender.com.
In vitro studies from the 1990s have identified and characterized SSTR expression in normal thyroid tissue and in various thyroid cancer cell lines. One of the early studies showed that SSTRs 1, 3, and 5 were expressed in ATC cell lines while all SSTR subtypes were poorly expressed in PTC cell lines, and normal thyroid tissue predominantly expressed SSTR 3 and 5 (62). Administration of different types of SSAs to thyroid cancer cell lines, including those belonging to PTC, FTC, and ATC has demonstrated varying effects on cell growth depending on the cell line and the type of SSA (63). Similarly, MTC cell lines have also demonstrated avidity to somatostatin and to radiolabeled SSAs (64). SSTRs 1, 2, and 5 have been identified in tumor samples of patients with MTC, and differential effects on cell viability and on calcitonin and chromogranin A secretion has been demonstrated by the use of various receptor subtype agonists (65). Injection of 111In-pentetreotide and measurement of its activity in thyroid tumors and in peripheral blood in patients has revealed maximum tumor-to-blood ratio in MTCs, and while this ratio was lower in PTCs/HTCs compared to that of MTC, the ratio was still higher compared to normal thyroid and colloid goiters (66). Later studies have demonstrated SSTR2 and 5 expression in normal thyroid tissue and in DTCs (67–69). Klagge et al. identified expression of SSTRs 1, 2, 3, and 5 mRNA expression in normal thyroid and in various benign and malignant thyroid tumors, including PTC, FTC, and ATC (69). In this study, compared to normal thyroid tissue, expression of mRNA was significantly upregulated for SSTR2 in PTC, and for SSTR3 in PTC and ATC, while SSTR5 expression was non-significantly elevated in PTC and FTC. Our group also demonstrated a higher expression of SSTR2 in all types of thyroid cancers compared to normal thyroid based on immunohistochemical analysis of surgical tumor specimens obtained from patients with thyroid cancer (70). Furthermore, we identified in vivo uptake of 68Ga-DOTATATE in different thyroid cancers (70). While the maximum standardized uptake value (SUVmax) was variable in PTCs and MTCs, the highest SUVmax values were observed with HTCs.
Normal thyroid gland tissue expresses SSTR2 and a physiologic uptake in the thyroid gland can be expected to be witnessed on 68Ga-DOTA SSA scans, with normal thyroid activity appearing equal to or lower than salivary gland or liver uptake (71, 72). However, in a retrospective analysis, atypical uptake patterns in the thyroid gland (diffusely increased homogeneous/heterogenous uptake, or focal increased uptake with a homogeneous normal thyroid uptake) were noted on diagnostic 68Ga-DOTATATE PET/CT scans performed in patients with NETs (72). In this study, of the 237 patients, 26 patients had atypical thyroid uptake, among whom 14 patients had focal uptake and 12 had diffuse uptake. Thyroid nodules were found in 10 out of 14 patients with focal uptake, and three out of these 10 patients were diagnosed with PTC. On the other hand, patients with diffuse uptake tended to have underlying hypothyroidism, thyroiditis, or non-toxic multinodular goiter. Similarly, uptake in normal thyroid, Hashimoto’s thyroiditis, autonomous thyroid nodule, and goiters have been observed on 111In-DTPA-octreotide (111In-pentetreotide) and 68Ga-DOTATOC scintigraphy (48, 73).
In early studies, scintigraphic uptake of 111In-pentetreotide in the normal thyroid gland and in DTCs were identified, suggesting SSTR positivity in these tumors (74). In an early case series of 4 patients with metastatic thyroid cancers (2 RAI-refractory PTCs, and 2 ‘insular’ DTCs/PDTCs), 111In-pentetreotide scintigraphy revealed uptake in the tumors in both patients with insular variant, and in one PTC patient (75). Several other studies have evaluated 111In-labeled SSA scintigraphic uptake patterns in DTCs, including varying combinations of PTC, FTC, HTC, and PDTCs, with vast scintigraphy positivity rates ranging from 19% to 100% (51, 76–84). Binse et al. evaluated the diagnostic utility of 68Ga-DOTATOC in 15 consecutive patients with 18F-FDG-negative, RAI-refractory DTC with rising serum thyroglobulin (Tg) (85). 68Ga-DOTATOC uptake was demonstrated in 3/3 patients with PDTC, 1/1 patient with HTC, 1/5 patients with PTC, and 0/6 patients with FTC, and the serum Tg levels with 68Ga-DOTATOC-avid tumors showed a tendency to be higher. In another study by Kundu et al., 68Ga-DOTA-NOC-PET/CT was compared to 18F-FDG-PET/CT for diagnostic utility in 62 RAI-refractory DTC patients. Out of the 186 lesions identified on PET/CT, 68Ga-DOTA-NOC identified lesser number of lesions compared to 18F-FDG (65% vs. 90.3%; p <0.0001). However, 68Ga-DOTA-NOC influenced a change in management of disease in 34% patients as opposed to a change in management in 27% patients influenced by 18F-FDG. Using radio-isotopes that allow for more comprehensive detection of metastatic thyroid cancer is also of importance. In one study comparing intra-patient imaging differences between 111In-pentetreotide and 99Tc-ethylenediamine N,N′-diacetic acid hydrazinonicotinic acid-octreotide (99Tc-EDDA/HYNIC-TOC) in 11 thyroid cancer patients (8 PTC, 3 MTC), both modalities gave equivalent scintigraphic results, but 99Tc-EDDA/HYNIC-TOC managed to identify a solitary pulmonary metastatic lesion in 1 PTC patient, which was not visualized on the 111In-pentetreotide scan (86). Later studies have corroborated the diagnostic efficacy of 99Tc-EDDA/HYNIC-TOC in localizing metastatic disease in RAI-refractory DTCs, with particularly high sensitivities for detection of lymph node, bone, and lung metastases (87). There also lies a potential for utility of SSTR antagonists as pilot data has demonstrated superior tumor uptake noted on imaging on 111In-tagged SSTR antagonist when compared to 111In-pentetreotide (SSTR agonist) in NETs (88). Emerging data suggests that evaluation of tumor textural parameters rather than conventional parameters (such as SUV) on PET studies may be more predictive of survival outcomes in patients (89).
An early study utilizing 111In-octreotide scintigraphy demonstrated tumor uptake in 65% of patients with MTC, and scintigraphic uptake correlated with in vitro expression of SSTRs in these tumor samples (90). In a prospective study conducted on 30 patients with MTC, imaging with 68Ga-DOTATATE PET/CT demonstrated a superior sensitivity in identifying bone metastases compared to a conventional bone scan (91). Another study used 111In-pentetreotide and 123I/131I-metaiodobenzylguanidine (MIBG) for diagnostic imaging in 8 patients with metastatic MTC (92). Uptake was present in 5/8 patients with 111In-pentetreotide and in 4/8 patients with 123I/131I-MIBG imaging, and the number of metastatic lesions visualized on 111In-pentetreotide was higher than on 123I/131I-MIBG. In general, 111In-based SSA scintigraphy is positive in approximately 29% -77% of patients with MTC (92). In addition, CCK2 receptors are overexpressed in MTC, and scintigraphy DOTA-linked CCK agonists such as 111In-DOTA-CCK, 111In-DOTA-minigastrin 11, and 99Tc-demogastrin 2 for diagnostic imaging of MTC have yielded variable results (93). Other non-peptide-based radiolabeled compounds such as 18F-dihydroxyphenylalanine (DOPA) and 18F-dopamine have been evaluated for diagnostic imaging in MTC, and some results suggest a superior performance of 18F-DOPA in tumor detection compared to other functional imaging modalities, including those based on 68Ga-SSAs (94). However, head-to-head comparisons between 18F-DOPA and other radiolabeled SSAs (such as 111In-SSAs) or CCK-based imaging are yet to be assessed, and radiolabeled-SSAs are still of value in evaluating MTCs that demonstrate equivocal findings on 18F-DOPA or other functional imaging studies, and for selecting patients with SSTR+ tumors who can benefit from PRRT (94).
A concise description of studies evaluating PRRT in thyroid cancer is provided in Table 1. Early studies demonstrated the efficacy of 90Y-DOTATOC and 111In-pentetreotide in patients with DTC (97, 99). Similarly, another early study demonstrated the efficacy of 177Lu-DOTATATE therapy in DTC (104). In this study, administration of 22.4 – 30.1 GBq of 177Lu-DOTATATE in 5 patients with 111In-pentetreotide-scintigraphy positive thyroid cancer demonstrated variable efficacy: SD in 1 PTC and 1 HTC, minor remission (MR; 25% - 50% tumor shrinkage) in 1 HTC, partial remission (PR; tumor shrinkage >50%) in 1 HTC, and PD in 1 FTC and 1 HTC patients. The 2 HTC patients with MR and PR demonstrated the highest 177Lu-DOTATATE uptake to pre-treatment 111In-pentetreotide uptake ratios. Several studies (sample size for DTC patients ranging from a single case to 25) were conducted in late 1990s to early 2000s to evaluate the efficacy of several forms of PRRTs consisting of 111In, 90Y- and 177Lu-labeled SSAs on a total of 62 DTC patients (18 PTC, 12 FTC, 7 HTC, and 25 unspecified thyroid cancers) either as a dedicated study for thyroid cancer or as a part of a larger study evaluating several other types of tumors (79, 82, 83, 86, 97–100, 103, 104). A total of 8% of population (5 patients, including 2 HTCs) achieved an objective tumor response while SD was noted in 42%, and time-to-progression (TTP) was inconsistently evaluated (51). To compare, in the phase 3 lenvatinib trial for RAI-refractory DTC (n=261 patients), after a median follow-up of 17.1 months, complete response (CR) was seen in 1.5%, PR in 63.2%, SD in 23%, and PD in 6.9% of the patients (8), and in the phase 3 sorafenib trial, the median TTP was 11.1 months (9). Later studies have also demonstrated the efficacy of 90Y and 177Lu-based PRRT in larger cohorts of DTC patients (106, 107, 110, 115) (Table 1), and the treatment was also associated with favorable safety profile. The most recent study by Roll et al. utilized 177Lu-DOTATATE (2 – 4 cycles in a span of 3 months; mean dose of 7 GBq) for treating 5 RAI-refractory SSTR2+ DTC patients with rising serum Tg levels (110). Based on post-therapy PET/CT and serum Tg values, 1 patient achieved PR, 3 patients had PD, while 1 patient had SD on imaging but had increasing serum Tg levels. These results suggest that a pre-treatment SSTR+ status of DTCs may not translate to effective treatment with 177Lu-DOTATATE, however, larger studies and optimization of SUV thresholds associated with response to therapy are needed to confirm the findings. Other 177Lu-based peptide or protein-tagged radionuclide therapies such as 177Lu-prostate-specific membrane antigen (PSMA) have been anecdotally utilized (116), although larger studies are required to establish the safety and efficacy of this particular PRRT.
Based on the available evidence in 2020, an executive committee comprised of experts from nuclear medicine, thyroid oncology, and head and neck surgery published the appropriateness of use of nuclear medicine studies in DTC (117). The committee deemed that diagnostic imaging with 68Ga-DOTATATE PET/CT should be considered in patients with RAI-refractory DTC in whom the serum Tg levels are rising and there is no identified source on conventional imaging, although they acknowledged the lack of sufficient evidence to correlate Tg levels or the rate of increase in Tg levels with the likelihood of detecting DTC on 68Ga-DOTATATE imaging. The committee also agreed that 177Lu-labeled SSAs may be useful in the treatment of RAI-refractory SSTR+ DTCs based on preliminary evidence, suggested by the observed objective response of 27% - 60% tumor burden reduction as per Response Evaluation Criteria in Solid Tumors (RECIST) criteria (107). Both 68Ga- and 177Lu-labeled SSAs were deemed inappropriate for use during pregnancy and lactation.
As MTC is considered as a form of neural crest tumor/NET, experience with PRRT in MTC is more robust as compared to DTC or ATC. PRRT in MTC has been evaluated by utilizing 90Y, 177Lu, and 111In radioisotopes, either tagged to SSAs or to CCK2 receptor agonists (56–58, 101, 109, 118, 119). Studies related to PRRT in MTC have been summarized in Table 1. Initial studies mainly utilized 90Y-DOTATOC as the mode of PRRT in small cohorts of MTC patients (95–97). In a retrospective analysis performed on 21 patients with SSTR+ metastatic MTC treated with 90Y-DOTATOC (7.5-19.2 GBq; 2 – 8 cycles), 2 (10%) patients achieved structural CR, 12 (57%) patients achieved stable structural disease, while seven (33%) patients did not respond to treatment, with a 3 – 40 months treatment duration (102). Biochemical (calcitonin and carcinoembryonic antigen [CEA]) CR was seen in 1 (5%) patient, PR in 5 (24%) patients, SD in 3 (14%) patients, and PD in 12 (57%) patients. In a phase II clinical trial, treatment with 90Y-DOTATOC in 30 adult patients with SSTR+ metastatic MTC was associated with response to therapy in 29% of the patients (105). This response was associated with longer survival from the time of diagnosis, and from the first 90Y-DOTATOC dose. Interestingly, response and survival were not associated with the visual grade of scintigraphic uptake (a 4-point scale of uptake pattern i. no uptake, ii. less than liver uptake, iii. similar to liver uptake, or iv. more than liver uptake) in the tumors, suggesting a potential for tumor response independent of uptake patterns on pre-therapy diagnostic scintigraphy. This observation is in contrast with the studies evaluating the association between the efficacy of 177Lu-DOTATATE in NETs, where SUVmax exceeding 13 – 15 by 68Ga-DOTATAE PET/CT was associated with better PFS (120, 121).
Later studies started evaluating the efficacy of 177Lu-based PRRT in MTC. In the study by Budiawan et al., both 177Lu-DOTATATE and 90Y-DOTATOC were used in a patient population with MTC as well as DTC (106). However, there was no discrete data on which patient received 90Y- vs. 177Lu-based PRRT. Among the 8 MTC patients included in the study, response to treatment was assessed in 5 patients after the last PRRT. PR was seen in 1 patient (20%), SD in 3 patients (60%), and PD in 1 patient (20%). Other studies also demonstrated the efficacy of 177Lu-DOTATATE therapy in small cohorts of MTC patients (108, 111) (Table 1), with one study noting improved quality of life among responders at 6 – 12 months post-therapy (108), and a higher uptake on the pre-PRRT diagnostic 111In-pentetreotide scans among patients with SD being noted in the other study (111).
More recent studies have analyzed the efficacy of PRRT on larger cohorts of patients. Parghane et al. evaluated the efficacy and toxicity of 177Lu-DOTATATE (average of 5.5 GBq; average of 3 cycles; range: 1 – 6 cycles) in 43 patients with SSTR+ metastatic MTC (112). The PFS was 24 months (95% CI: 15.1 – 32.9 months), and OS was 26 months (95% CI: 16.6 – 35.3 months) since the first dose of 177Lu-DOTATATE. A calcitonin doubling time of >24 months was associated with longer PFS, and OS compared to a doubling time of <6 months. Regarding biochemical response, CR was noted in 11%, PR in 30%, SD in 10%, and PD in 49% of the patients. The treatment was well-tolerated with grade 1 nausea and hemotoxicity and no nephrotoxicity or grade 3 or 4 toxicities were observed. To compare, the median PFS was 11.2 months in the cabozantinib trial among the patients in the cabozantinib treatment group (n = 219), and the predicted median PFS was 30.5 months in the vandetanib trial among patients receiving vandetanib (n = 231). In the NETTER-1 trial, patients receiving 177Lu-DOTATATE (n = 116) had an estimated PFS of 65%. Therefore, the PFS with PRRT in MTC seems to be comparable to the PFS observed with TKI therapy, although randomized controlled trials with standard-of-care therapies as control group are needed to appropriately compare these outcomes. Hayes et al. performed a retrospective analysis of data obtained from 71 patients with 68Ga-DOTATATE-avid metastatic MTCs to evaluate efficacy of PRRT (114). Among the 21 patients who received PRRT, the median OS was 63 months and the median time to treatment failure was 14 months, and a calcitonin doubling time of ≤24 months, age ≥60 years, and strong 18FDG-PET avidity were associated with poorer survival outcomes.
Another emerging strategy for enhancing PRRT efficacy is a combination therapy with a radiosensitizer such as capecitabine, which has been efficacious in treating GEP-NETs (59). In a retrospective observational study, data from 8 patients with inoperable/metastatic MTC treated with 177Lu-DOTATATE (median cumulative dose: 20.9 GBq; 1 – 4 cycles) along with capecitabine (1.25 g/m on days 1 – 14 of each cycle) were analyzed for treatment response and AEs (113). SD was noted in 7/8 (86%) patients, and PD was noted in 1 patient, and reduction in serum calcitonin was observed in 3/5 (60%) patients. There were no observed toxicities except for a grade 2 anemia.
To date, the utility of PRRT and SSA-based theranostics have been poorly studied in ATC. Some of the likely reasons include: highly dedifferentiated nature of the tumor which makes it unlikely to express SSTRs, the extremely rare prevalence of the tumor, and the rapid progression, possibly exceeding the rate of the rather slower therapeutic mechanism of radiation-induced apoptosis, and high morbidity and mortality associated with the disease. In a study that evaluated the treatment response of 90Y-DOTATOC in 20 thyroid cancer patients, 1 patient had progressive ATC, and 90Y-DOTATOC therapy failed to achieve an objective tumor response and the disease progressed during PRRT (97). Currently, imaging modalities based on targeting of galectin-3, a protein overexpressed in thyroid cancers, using radiolabeled monoclonal antibodies such as zirconium 89 (89Zr)-deferoxamine B-monoclonal antibody are being studied for ATC (122).
Large-scale studies on PRRT in thyroid cancer are yet to commence. However, recently published systematic reviews and meta-analyses have allowed for evaluation of currently available data on the utility of PRRT in thyroid cancers. A meta-analysis by Lee et al. evaluated 11 published articles on DTC and/or MTC for efficacy and outcomes with various PRRTs (123). The objective response rate (ORR) in MTC was 8.5% (95% CI, 1.9% – 19.2%; i2 = 53.5%) and the disease control rate (DCR) was 60% (95% CI, 49.6%–69.8%; i2 = 28.7%), while for DTC, the ORR and DCR rates were 15.6% (95% CI, 7.80%–26.74%; i2 = 0%), and 53.9% (95% CI: 41.13%–66.39%; i2 = 0%), respectively. Proportion of serious/severe AEs (SAEs) was 2.8% (95% CI, 0.5%–8.3%) in MTC, and 2.82% (95% CI: 0.03%–17.61%) in DTC. In comparison, SAE frequency in other established systemic therapies for thyroid cancer have ranged from 0.4% to as high as 47% (Table 2), which further highlights the relatively better safety profile of PRRT. Also, 177Lu-based PRRT was associated with better ORR and DCR outcomes when compared to 90Y-based PRRT. In a systematic-review conducted by Maghsoomi et al. (124), on 41 studies related to PRRT in thyroid cancer (157 patients with RAI-refractory DTC and 220 patients with MTC), biochemical response was noted in 25.3% of DTC and 37.2% of MTC patients, and CR/PR were noted in 10.5% of DTC and 10.6% of MTC patients. In patients with advanced disease, 46 deaths out of 95 patients were noted in DTC and 63 deaths out of 144 patients were noted in MTC. While major AEs were noted with 90Y-based PRRT, the AEs with 177Lu- or 111In-based PRRTs were mild or transient. In comparison, in the NETTER-1 trial, the ORR was 18% (95% CI: 10% - 25%; 18/101 patients in the 177Lu-DOTATATE arm), while the frequency of SAEs was 9% in the 177Lu-DOTATATE arm (33). Among the TKIs, the ORRs have been between 12.2% - 64.8% for DTC, and 28% - 45% for MTC, and the SAE frequency is up to 30.3% in DTC and up to 15.9% in MTC (8, 9, 16, 17). With selective RET inhibitors, the ORR has been 60% - 73% for MTC and up to 79% - 89% in RET-fusion thyroid cancers, with SAEs up to 28% - 47% (18, 19). Although these are not head-to-head comparisons, PRRT for thyroid cancer seems to have comparable ORRs to PRRT in NETs, and while the ORRs are better with TKIs or with selective RET inhibitors, PRRT seems to have a better safety profile compared to these systemic therapies. A comparison of outcomes among the meta-analysis findings in PRRT and thyroid cancer, the various systemic therapies for thyroid cancer, and PRRT in NETs is provided in Table 2.
Table 2 A comparison of the outcomes of PRRT in thyroid cancer, other systemic therapies in thyroid cancer, and PRRT in NETs.
Targeted therapy for thyroid cancer with PRRT is a continuously and rapidly evolving field, with several radiolabeled SSAs being investigated for their potential theranostic utility in various clinical trials for NETs. Nevertheless, the field of theranostics and PRRT in thyroid cancer is relatively young and has several areas that need further research. One such area includes further optimization of diagnostic radiolabeled ligand-based imaging for better visual delineation of thyroid tumors. As most PRRTs utilize SSAs that bind to SSTR2, treatment of thyroid cancers that express other types of SSTRs would not be feasible with most of the currently available PRRTs. Particularly DTCs may demonstrate varied SSTR subtype expressions as opposed to NETs, which commonly express SSTR2 (51). While several studies have demonstrated SSTR2 positivity in thyroid cancers, other studies have demonstrated a relative abundance of other SSTR subtypes, such as SSTR5, in these tumors (62, 66, 109, 125). These findings highlight the importance of developing PRRTs based on SSAs that could target SSTRs apart from the SSTR2 subtype. For instance, 111In-DOTA-NOC has increased affinity towards SSTR3 and 5, along with a high affinity to SSTR2, thus potentially allowing for further optimization of SSA-based tumor imaging (51, 126). In this manner, development of the corresponding 177Lu-DOTA-NOC or α-emitter-DOTA-NOC PRRTs may potentially be more efficacious in treating thyroid cancers.
A dosimetry-based approach to the diagnostic evaluation of thyroid cancers using radiolabeled SSAs allows for better identification of tumors that are likely to respond to the PRRT. The SUVmax values on 68Ga-DOTATOC imaging have been shown to correlate with SSTR2 mRNA expression in human tissues (67), and on the contrary, SUVmax correlates negatively with higher Ki-67 index (suggestive of more aggressive, undifferentiated tumor) among GEP-NETs (127). More dosimetric studies are required to further characterize the potential of thyroid cancers that can be targeted by PRRT. Individualized dosimetry-based approach focused on predicting the tumoricidal effects of certain tumor-absorbed dose thresholds has been proven effective in other types of cancers such as hepatocellular carcinoma (128). While β emitters are currently widely used as components of PRRT for NETs and thyroid cancer, α emitter-based SSAs also hold potential to serve as alternative forms of PRRT. As opposed to β emitters, which have a longer penetration range and a potential for damaging normal surrounding tissue, α emitters have a shorter penetration range leading to lesser normal tissue damage and have a higher energy and high linear energy transfer, leading to higher cytotoxicity (129, 130). More data on clinical utility of' α emitter-based PRRT should be available in the upcoming years. Another interesting area for further research is exploring methods to upregulate the expression of SSTRs on the cell surface. Preliminary in vitro and in vivo animal model experiments using epigenetic modifier drugs have resulted in successful upregulation of SSTR2 at transcriptional and functional levels (131). Further studies in humans are necessary to evaluate the safety and efficacy of SSTR-upregulator molecules. Literature on the association between PRRT and the molecular signatures of thyroid cancer (for instance, sporadic thyroid cancers versus thyroid cancers with germline pathogenic variants) is currently lacking and this area holds potential as another interesting avenue for future studies.
Apart from trying alternate radionuclides and chelators, modifications of the SSA or the peptide component of a radiolabeled SSA can also allow for improvement in the pharmacokinetics, and in turn potentially improve the diagnostic and therapeutic performance. Recently, Evans Blue (EB) analog has been conjugated with DOTATATE to develop DOTA-EB-TATE, which reversibly binds to the circulating albumin and improves the half-life of the molecule (132). 90Y-DOTA-EB-TATE has demonstrated better tumor response and survival rates compared to DOTATATE in mouse models (132). Our group also demonstrated that 90Y-DOTA-EB-TATE exhibits the highest tumor uptake in mouse xenograft models, irrespective of the type of thyroid cancer cell line xenograft, when compared to 68Ga-DOTATATE and 68Ga-DOTA-JR11 (70). Moreover, we also demonstrated superior tumor volume reduction and disease-specific survival in the SSTR2+ thyroid cancer xenograft mouse models with the use of 177Lu-DOTA-EB-TATE when compared to 177Lu-DOTA-TATE and177Lu-DOTA-JR11 (70). Therefore, EB-TATE-based SSA imaging and PRRT holds potential to improve theranostics in thyroid cancer.
Currently, clinical trials on PRRT or SSA-based imaging in thyroid cancers are sparse. For instance, one trial (NCT04106843) had to be withdrawn due to lack of registration of participants. There is one study (NCT04927416) that is currently recruiting patients for further characterization of metastatic RAI-refractory DTCs, HTCs, and MTCs using 68Ga-DOTATATE PET/CT. Another randomized cross-over study utilizing 177Lu-PP-F11N, a CCK2 receptor analog with or without sacubitril for treatment of metastatic MTC (NCT03647657) has been completed, and the results are awaited, while another combined pilot and phase I clinical trial (NCT02088645) is currently recruiting advanced MTC patients for theranostics with 177Lu-PP-F11N.
In conclusion, PRRT is a rapidly evolving field, and holds great potential as a theranostic tool in the management of metastatic RAI-refractory thyroid cancers. Current literature has provided substantial evidence for the efficacy and the safety profile of PRRT. Certain forms of thyroid cancers, such as HTCs and MTCs could be potentially treated better with PRRT as opposed to other forms of systemic therapies. The efficacy of PRRT may also depend on the tumor characteristics observed on the diagnostic radiolabeled-SSA imaging, and the molecular landscape of the various thyroid cancers. Large-scale, randomized controlled trials are necessary to further delineate the functional and molecular characteristics of the tumors that do and do not respond to PRRT and to perform head-to-head comparisons with other commonly used systemic therapies for the management of RAI-refractory thyroid cancers.
SG prepared the manuscript. CK reviewed the manuscript and provided suggestions to improve the manuscript. JK-G conceptualized the manuscript, reviewed the manuscript, and provided suggestions to improve the manuscript. All authors were involved in the revision of the manuscript. All authors contributed to the article and approved the submitted version.
This work was funded by the intramural program of the National Institute of Diabetes and Digestive and Kidney Diseases of the National Institutes of Health.
The authors declare that the research was conducted in the absence of any commercial or financial relationships that could be construed as a potential conflict of interest.
All claims expressed in this article are solely those of the authors and do not necessarily represent those of their affiliated organizations, or those of the publisher, the editors and the reviewers. Any product that may be evaluated in this article, or claim that may be made by its manufacturer, is not guaranteed or endorsed by the publisher.
PRRT, peptide receptor radionuclide therapy; SSA, somatostatin analog; DTC, differentiated thyroid cancer; PTC, papillary thyroid cancer; FTC, follicular thyroid cancer; HTC, Hürthle cell thyroid cancer; MTC, medullary thyroid cancer; PDTC, poorly differentiated thyroid cancer; ATC, anaplastic thyroid cancer; NET, neuroendocrine tumor; RAI, radioactive iodine; ORR, objective response rate; DCR, disease control rate; OS, overall survival; PFS, progression-free survival; TTP,time-to-progression; TTF, time-to-treatment failure; DOR, duration of response; IQ, interquartile range; NA, not available; SAE, serious/severe adverse event; FDA, Food and Drug Administration; TKI, tyrosine kinase inhibitor; MEK, mitogen-activated protein kinase kinase; RET, rearranged during transfection; mTOR, mammalian target of rapamycin; NTRK, neurotrophic receptor tyrosine kinase; ICI, immune checkpoint inhibitor; ALK, Anaplastic lymphoma kinase; SRIF, somatotropin release inhibiting factor; LAR, long-acting repeatable; CT, computed tomography; MRI, magnetic resonance imaging; PET/CT, positron emission tomography computed tomography; SPECT, single photon emission computed tomography; FDG, fluorodeoxyglucose; DOPA, dihydroxyphenylalanine; MIBG, metaiodobenzylguanidine; DOTATOC, 1,4,7,10-tetraazacyclododecane-1,4,7,20-tetra-acetic acid-octreotide; DOTATATE, 1,4,7,10-tetraazacyclododecane-1,4,7,20-tetra-acetic acid-octreotate; DTPA, diethylenetriamine penta-acetic acid; DOTA-NOC, [DOTA0-1-NaI3]octreotide; NODAGA, 1,4,7-triazacyclononane,1-glutaric acid-4,7-acetic acid; (c[RGDyK])2, dimeric cyclic arginine-glycine-aspartic acid (RGD)-based peptide; EDDA/HYNIC-TOC, ethylenediamine N,N′-diacetic acid hydrazinonicotinic acid-octreotide; EB-TATE, Evans blue-DOTATATE; PSMA, Prostate-specific membrane antigen; CCK, cholecystokinin.
1. Dash A, Chakraborty S, Pillai MR, Knapp FF Jr. Peptide Receptor Radionuclide Therapy: An Overview. Cancer Biother Radiopharm (2015) 30(2):47–71. doi: 10.1089/cbr.2014.1741
2. Krenning EP, Kwekkeboom DJ, Valkema R, Pauwels S, Kvols LK, De Jong M. Peptide Receptor Radionuclide Therapy. Ann N Y Acad Sci (2004) 1014:234–45. doi: 10.1196/annals.1294.026
3. Haugen BR, Alexander EK, Bible KC, Doherty GM, Mandel SJ, Nikiforov YE, et al. 2015 American Thyroid Association Management Guidelines for Adult Patients With Thyroid Nodules and Differentiated Thyroid Cancer: The American Thyroid Association Guidelines Task Force on Thyroid Nodules and Differentiated Thyroid Cancer. Thyroid (2016) 26(1):1–133. doi: 10.1089/thy.2015.0020
4. Wells SA Jr., Asa SL, Dralle H, Elisei R, Evans DB, Gagel RF, et al. Revised American Thyroid Association Guidelines for the Management of Medullary Thyroid Carcinoma. Thyroid (2015) 25(6):567–610. doi: 10.1089/thy.2014.0335
5. Bible KC, Kebebew E, Brierley J, Brito JP, Cabanillas ME, Clark TJ Jr., et al. 2021 American Thyroid Association Guidelines for Management of Patients With Anaplastic Thyroid Cancer. Thyroid (2021) 31(3):337–86. doi: 10.1089/thy.2020.0944
6. Feine U, Lietzenmayer R, Hanke JP, Held J, Wöhrle H, Müller-Schauenburg W. Fluorine-18-FDG and Iodine-131-Iodide Uptake in Thyroid Cancer. J Nucl Med (1996) 37(9):1468–72.
7. Cancer Genome Atlas Research Network. Integrated Genomic Characterization of Papillary Thyroid Carcinoma. Cell (2014) 159(3):676–90. doi: 10.1016/j.cell.2014.09.050
8. Schlumberger M, Tahara M, Wirth LJ, Robinson B, Brose MS, Elisei R, et al. Lenvatinib Versus Placebo in Radioiodine-Refractory Thyroid Cancer. N Engl J Med (2015) 372(7):621–30. doi: 10.1056/NEJMoa1406470
9. Brose MS, Nutting CM, Jarzab B, Elisei R, Siena S, Bastholt L, et al. Sorafenib in Radioactive Iodine-Refractory, Locally Advanced or Metastatic Differentiated Thyroid Cancer: A Randomised, Double-Blind, Phase 3 Trial. Lancet (2014) 384(9940):319–28. doi: 10.1016/S0140-6736(14)60421-9
10. Al-Jundi M, Thakur S, Gubbi S, Klubo-Gwiezdzinska J. Novel Targeted Therapies for Metastatic Thyroid Cancer-A Comprehensive Review. Cancers (Basel) (2020) 12(8). doi: 10.3390/cancers12082104
11. Araque KA, Gubbi S, Klubo-Gwiezdzinska J. Updates on the Management of Thyroid Cancer. Horm Metab Res (2020) 52(8):562–77. doi: 10.1055/a-1089-7870
12. Ho AL, Grewal RK, Leboeuf R, Sherman EJ, Pfister DG, Deandreis D, et al. Selumetinib-Enhanced Radioiodine Uptake in Advanced Thyroid Cancer. N Engl J Med (2013) 368(7):623–32. doi: 10.1056/NEJMoa1209288
13. Iravani A, Solomon B, Pattison DA, Jackson P, Ravi Kumar A, Kong G, et al. Mitogen-Activated Protein Kinase Pathway Inhibition for Redifferentiation of Radioiodine Refractory Differentiated Thyroid Cancer: An Evolving Protocol. Thyroid (2019) 29(11):1634–45. doi: 10.1089/thy.2019.0143
14. Coca-Pelaz A, Rodrigo JP, Shah JP, Sanabria A, Al Ghuzlan A, Silver CE, et al. Hürthle Cell Carcinoma of the Thyroid Gland: Systematic Review and Meta-Analysis. Adv Ther (2021) 38(10):5144–64. doi: 10.1007/s12325-021-01876-7
15. Dierks C, Seufert J, Aumann K, Ruf J, Klein C, Kiefer S, et al. Combination of Lenvatinib and Pembrolizumab Is an Effective Treatment Option for Anaplastic and Poorly Differentiated Thyroid Carcinoma. Thyroid (2021) 31(7):1076–85. doi: 10.1089/thy.2020.0322
16. Wells SA Jr., Robinson BG, Gagel RF, Dralle H, Fagin JA, Santoro M, et al. Vandetanib in Patients With Locally Advanced or Metastatic Medullary Thyroid Cancer: A Randomized, Double-Blind Phase III Trial. J Clin Oncol (2012) 30(2):134–41. doi: 10.1200/JCO.2011.35.5040
17. Elisei R, Schlumberger MJ, Müller SP, Schöffski P, Brose MS, Shah MH, et al. Cabozantinib in Progressive Medullary Thyroid Cancer. J Clin Oncol (2013) 31(29):3639–46. doi: 10.1200/JCO.2012.48.4659
18. Wirth LJ, Sherman E, Robinson B, Solomon B, Kang H, Lorch J, et al. Efficacy of Selpercatinib in RET-Altered Thyroid Cancers. N Engl J Med (2020) 383(9):825–35. doi: 10.1056/NEJMoa2005651
19. Subbiah V, Hu MI, Wirth LJ, Schuler M, Mansfield AS, Curigliano G, et al. Pralsetinib for Patients With Advanced or Metastatic RET-Altered Thyroid Cancer (ARROW): A Multi-Cohort, Open-Label, Registrational, Phase 1/2 Study. Lancet Diabetes Endocrinol (2021) 9(8):491–501. doi: 10.1016/S2213-8587(21)00120-0
20. Hu S, Helman SN, Hanly E, Likhterov I. The Role of Surgery in Anaplastic Thyroid Cancer: A Systematic Review. Am J Otolaryngol (2017) 38(3):337–50. doi: 10.1016/j.amjoto.2017.02.005
21. Subbiah V, Kreitman RJ, Wainberg ZA, Cho JY, Schellens JHM, Soria JC, et al. Dabrafenib and Trametinib Treatment in Patients With Locally Advanced or Metastatic BRAF V600-Mutant Anaplastic Thyroid Cancer. J Clin Oncol (2018) 36(1):7–13. doi: 10.1200/JCO.2017.73.6785
22. Zwaenepoel K, Jacobs J, De Meulenaere A, Silence K, Smits E, Siozopoulou V, et al. CD70 and PD-L1 in Anaplastic Thyroid Cancer - Promising Targets for Immunotherapy. Histopathology (2017) 71(3):357–65. doi: 10.1111/his.13230
23. Wagle N, Grabiner BC, Van Allen EM, Amin-Mansour A, Taylor-Weiner A, Rosenberg M, et al. Response and Acquired Resistance to Everolimus in Anaplastic Thyroid Cancer. N Engl J Med (2014) 371(15):1426–33. doi: 10.1056/NEJMoa1403352
24. Cabanillas ME, Hu MI, Durand JB, Busaidy NL. Challenges Associated With Tyrosine Kinase Inhibitor Therapy for Metastatic Thyroid Cancer. J Thyroid Res (2011) 2011:985780. doi: 10.4061/2011/985780
25. Milling RV, Grimm D, Krüger M, Grosse J, Kopp S, Bauer J, et al. Pazopanib, Cabozantinib, and Vandetanib in the Treatment of Progressive Medullary Thyroid Cancer With a Special Focus on the Adverse Effects on Hypertension. Int J Mol Sci (2018) 19(10). doi: 10.3390/ijms19103258
26. Ancker OV, Krüger M, Wehland M, Infanger M, Grimm D. Multikinase Inhibitor Treatment in Thyroid Cancer. Int J Mol Sci (2019) 21(1). doi: 10.3390/ijms21010010
27. Blevins DP, Dadu R, Hu M, Baik C, Balachandran D, Ross W, et al. Aerodigestive Fistula Formation as a Rare Side Effect of Antiangiogenic Tyrosine Kinase Inhibitor Therapy for Thyroid Cancer. Thyroid (2014) 24(5):918–22. doi: 10.1089/thy.2012.0598
28. Subbiah V, Yang D, Velcheti V, Drilon A, Meric-Bernstam F. State-Of-the-Art Strategies for Targeting RET-Dependent Cancers. J Clin Oncol (2020) 38(11):1209–21. doi: 10.1200/JCO.19.02551
29. Méndez-Martínez S, Calvo P, Ruiz-Moreno O, Pardiñas Barón N, Leciñena Bueno J, Gil Ruiz MDR, et al. Ocular Adverse Events Associated With Mek Inhibitors. Retina (2019) 39(8):1435–50. doi: 10.1097/IAE.0000000000002451
30. Heinzerling L, Eigentler TK, Fluck M, Hassel JC, Heller-Schenck D, Leipe J, et al. Tolerability of BRAF/MEK Inhibitor Combinations: Adverse Event Evaluation and Management. ESMO Open (2019) 4(3):e000491. doi: 10.1136/esmoopen-2019-000491
31. Banks M, Crowell K, Proctor A, Jensen BC. Cardiovascular Effects of the MEK Inhibitor, Trametinib: A Case Report, Literature Review, and Consideration of Mechanism. Cardiovasc Toxicol (2017) 17(4):487–93. doi: 10.1007/s12012-017-9425-z
32. Zandee WT, Feelders RA, Smit Duijzentkunst DA, Hofland J, Metselaar RM, Oldenburg RA, et al. Treatment of Inoperable or Metastatic Paragangliomas and Pheochromocytomas With Peptide Receptor Radionuclide Therapy Using 177Lu-DOTATATE. Eur J Endocrinol (2019) 181(1):45–53. doi: 10.1530/EJE-18-0901
33. Strosberg J, El-Haddad G, Wolin E, Hendifar A, Yao J, Chasen B, et al. Phase 3 Trial of (177)Lu-Dotatate for Midgut Neuroendocrine Tumors. N Engl J Med (2017) 376(2):125–35. doi: 10.1056/NEJMoa1607427
34. Brabander T, van der Zwan WA, Teunissen JJM, Kam BLR, Feelders RA, de Herder WW, et al. Long-Term Efficacy, Survival, and Safety of [(177)Lu-DOTA(0),Tyr(3)]octreotate in Patients With Gastroenteropancreatic and Bronchial Neuroendocrine Tumors. Clin Cancer Res (2017) 23(16):4617–24. doi: 10.1158/1078-0432.CCR-16-2743
35. Bergsma H, van Vliet EI, Teunissen JJ, Kam BL, de Herder WW, Peeters RP, et al. Peptide Receptor Radionuclide Therapy (PRRT) for GEP-NETs. Best Pract Res Clin Gastroenterol (2012) 26(6):867–81. doi: 10.1016/j.bpg.2013.01.004
36. Patel YC. Somatostatin and its Receptor Family. Front Neuroendocrinol (1999) 20(3):157–98. doi: 10.1006/frne.1999.0183
37. Hoyer D, Bell GI, Berelowitz M, Epelbaum J, Feniuk W, Humphrey PP, et al. Classification and Nomenclature of Somatostatin Receptors. Trends Pharmacol Sci (1995) 16(3):86–8. doi: 10.1016/S0165-6147(00)88988-9
38. Weckbecker G, Lewis I, Albert R, Schmid HA, Hoyer D, Bruns C. Opportunities in Somatostatin Research: Biological, Chemical and Therapeutic Aspects. Nat Rev Drug Discovery (2003) 2(12):999–1017. doi: 10.1038/nrd1255
39. Ameri P, Ferone D. Diffuse Endocrine System, Neuroendocrine Tumors and Immunity: What’s New? Neuroendocrinology (2012) 95(4):267–76. doi: 10.1159/000334612
40. Burgus R, Ling N, Butcher M, Guillemin R. Primary Structure of Somatostatin, a Hypothalamic Peptide That Inhibits the Secretion of Pituitary Growth Hormone. Proc Natl Acad Sci U S A (1973) 70(3):684–8. doi: 10.1073/pnas.70.3.684
41. Modlin IM, Pavel M, Kidd M, Gustafsson BI. Review Article: Somatostatin Analogues in the Treatment of Gastroenteropancreatic Neuroendocrine (Carcinoid) Tumours. Aliment Pharmacol Ther (2010) 31(2):169–88. doi: 10.1111/j.1365-2036.2009.04174.x
42. Reichlin S. Secretion of Somatostatin and its Physiologic Function. J Lab Clin Med (1987) 109(3):320–6.
43. Andrzejewski S, Siegel PM, St-Pierre J. Metabolic Profiles Associated With Metformin Efficacy in Cancer. Front Endocrinol (2018) 9:372. doi: 10.3389/fendo.2018.00372
44. Vale W, Rivier C, Brazeau P, Guillemin R. Effects of Somatostatin on the Secretion of Thyrotropin and Prolactin. Endocrinology (1974) 95(4):968–77. doi: 10.1210/endo-95-4-968
45. Han X, Xu Z, Cao S, Zhao Y, Wu W. The Effect of Somatostatin Analogues on Postoperative Outcomes Following Pancreatic Surgery: A Meta-Analysis. PloS One (2017) 12(12):e0188928. doi: 10.1371/journal.pone.0188928
46. Gurusamy KS, Koti R, Fusai G, Davidson BR. Somatostatin Analogues for Pancreatic Surgery. Cochrane Database Syst Rev (2012) 6):Cd008370. doi: 10.1002/14651858.CD008370.pub2
47. Reubi JC, Waser B, Schaer JC, Laissue JA. Somatostatin Receptor Sst1-Sst5 Expression in Normal and Neoplastic Human Tissues Using Receptor Autoradiography With Subtype-Selective Ligands. Eur J Nucl Med (2001) 28(7):836–46. doi: 10.1007/s002590100541
48. Bhanat E, Koch CA, Parmar R, Garla V, Vijayakumar V. Somatostatin Receptor Expression in non-Classical Locations - Clinical Relevance? Rev Endocr Metab Disord (2018) 19(2):123–32. doi: 10.1007/s11154-018-9470-3
49. Hannon JP, Nunn C, Stolz B, Bruns C, Weckbecker G, Lewis I, et al. Drug Design at Peptide Receptors: Somatostatin Receptor Ligands. J Mol Neurosci (2002) 18(1-2):15–27. doi: 10.1385/JMN:18:1-2:15
50. Kelkar SS, Reineke TM. Theranostics: Combining Imaging and Therapy. Bioconjug Chem (2011) 22(10):1879–903. doi: 10.1021/bc200151q
51. Teunissen JJ, Kwekkeboom DJ, Krenning EP. Staging and Treatment of Differentiated Thyroid Carcinoma With Radiolabeled Somatostatin Analogs. Trends Endocrinol Metab (2006) 17(1):19–25. doi: 10.1016/j.tem.2005.11.005
52. Rosai J. The Origin of Neuroendocrine Tumors and the Neural Crest Saga. Modern Pathology (2011) 24(2):S53–S7. doi: 10.1038/modpathol.2010.166
53. Ahmadi Bidakhvidi N, Goffin K, Dekervel J, Baete K, Nackaerts K, Clement P, et al. Peptide Receptor Radionuclide Therapy Targeting the Somatostatin Receptor: Basic Principles, Clinical Applications and Optimization Strategies. Cancers (Basel) (2021) 14(1). doi: 10.3390/cancers14010129
54. Satapathy S, Mittal BR, Bhansali A. ‘Peptide Receptor Radionuclide Therapy in the Management of Advanced Pheochromocytoma and Paraganglioma: A Systematic Review and Meta-Analysis’. Clin Endocrinol (Oxf) (2019) 91(6):718–27. doi: 10.1111/cen.14106
55. Vyakaranam AR, Crona J, Norlén O, Granberg D, Garske-Román U, Sandström M, et al. Favorable Outcome in Patients With Pheochromocytoma and Paraganglioma Treated With (177)Lu-DOTATATE. Cancers (Basel) (2019) 11(7). doi: 10.3390/cancers11070909
56. Pasieka JL, McEwan AJ, Rorstad O. The Palliative Role of 131I-MIBG and 111In-Octreotide Therapy in Patients With Metastatic Progressive Neuroendocrine Neoplasms. Surgery (2004) 136(6):1218–26. doi: 10.1016/j.surg.2004.06.050
57. Caplin ME, Mielcarek W, Buscombe JR, Jones AL, Croasdale PL, Cooper MS, et al. Toxicity of High-Activity 111In-Octreotide Therapy in Patients With Disseminated Neuroendocrine Tumours. Nucl Med Commun (2000) 21(1):97–102. doi: 10.1097/00006231-200001000-00016
58. Buscombe JR, Caplin ME, Hilson AJ. Long-Term Efficacy of High-Activity 111in-Pentetreotide Therapy in Patients With Disseminated Neuroendocrine Tumors. J Nucl Med (2003) 44(1):1–6.
59. Ballal S, Yadav MP, Damle NA, Sahoo RK, Bal C. Concomitant 177lu-DOTATATE and Capecitabine Therapy in Patients With Advanced Neuroendocrine Tumors: A Long-Term-Outcome, Toxicity, Survival, and Quality-Of-Life Study. Clin Nucl Med (2017) 42(11):e457–e66. doi: 10.1097/RLU.0000000000001816
60. Koch CA, Petersenn S. Neuroendocrine Neoplasms - Think About it and Choose the Most Appropriate Diagnostic and Therapeutic Steps. Rev Endocr Metab Disord (2018) 19(2):107–9. doi: 10.1007/s11154-018-9472-1
61. Alonzo N, Seyer M, Kim E-J, Keshavarzi R, Yee K, Kunz PL. Evaluation of the Incidence of Acute Nausea and Vomiting After Administration of an Amino Acid Solution Containing Only Arginine and Lysine With Lutetium Lu-177 Dotatate. J Clin Oncol (2020) 38(15_suppl):12113–. doi: 10.1200/JCO.2020.38.15_suppl.12113
62. Ain KB, Taylor KD, Tofiq S, Venkataraman G. Somatostatin Receptor Subtype Expression in Human Thyroid and Thyroid Carcinoma Cell Lines. J Clin Endocrinol Metab (1997) 82(6):1857–62. doi: 10.1210/jc.82.6.1857
63. Ain KB, Taylor KD. Somatostatin Analogs Affect Proliferation of Human Thyroid Carcinoma Cell Lines In Vitro. J Clin Endocrinol Metab (1994) 78(5):1097–102. doi: 10.1210/jcem.78.5.7909817
64. Reubi JC, Chayvialle JA, Franc B, Cohen R, Calmettes C, Modigliani E. Somatostatin Receptors and Somatostatin Content in Medullary Thyroid Carcinomas. Lab Invest (1991) 64(4):567–73.
65. Zatelli MC, Piccin D, Tagliati F, Bottoni A, Luchin A, Vignali C, et al. Selective Activation of Somatostatin Receptor Subtypes Differentially Modulates Secretion and Viability in Human Medullary Thyroid Carcinoma Primary Cultures: Potential Clinical Perspectives. J Clin Endocrinol Metab (2006) 91(6):2218–24. doi: 10.1210/jc.2006-0334
66. Forssell-Aronsson EB, Nilsson O, Bejegård SA, Kölby L, Bernhardt P, Mölne J, et al. 111In-DTPA-D-Phe1-Octreotide Binding and Somatostatin Receptor Subtypes in Thyroid Tumors. J Nucl Med (2000) 41(4):636–42.
67. Boy C, Heusner TA, Poeppel TD, Redmann-Bischofs A, Unger N, Jentzen W, et al. 68Ga-DOTATOC PET/CT and Somatostatin Receptor (Sst1-Sst5) Expression in Normal Human Tissue: Correlation of Sst2 mRNA and SUVmax. Eur J Nucl Med Mol Imaging (2011) 38(7):1224–36. doi: 10.1007/s00259-011-1760-x
68. Unger N, Ueberberg B, Schulz S, Saeger W, Mann K, Petersenn S. Differential Expression of Somatostatin Receptor Subtype 1-5 Proteins in Numerous Human Normal Tissues. Exp Clin Endocrinol Diabetes (2012) 120(8):482–9. doi: 10.1055/s-0032-1314859
69. Klagge A, Krause K, Schierle K, Steinert F, Dralle H, Fuhrer D. Somatostatin Receptor Subtype Expression in Human Thyroid Tumours. Horm Metab Res (2010) 42(4):237–40. doi: 10.1055/s-0029-1243636
70. Thakur S, Daley B, Millo C, Cochran C, Jacobson O, Lu H, et al. (177)Lu-DOTA-EB-TATE, a Radiolabeled Analogue of Somatostatin Receptor Type 2, for the Imaging and Treatment of Thyroid Cancer. Clin Cancer Res (2021) 27(5):1399–409. doi: 10.1158/1078-0432.CCR-20-3453
71. Druckenthaner M, Schwarzer C, Ensinger C, Gabriel M, Prommegger R, Riccabona G, et al. Evidence for Somatostatin Receptor 2 in Thyroid Tissue. Regul Pept (2007) 138(1):32–9. doi: 10.1016/j.regpep.2006.08.005
72. Nockel P, Millo C, Keutgen X, Klubo-Gwiezdzinska J, Shell J, Patel D, et al. The Rate and Clinical Significance of Incidental Thyroid Uptake as Detected by Gallium-68 DOTATATE Positron Emission Tomography/Computed Tomography. Thyroid (2016) 26(6):831–5. doi: 10.1089/thy.2016.0174
73. Lincke T, Singer J, Kluge R, Sabri O, Paschke R. Relative Quantification of Indium-111 Pentetreotide and Gallium-68 DOTATOC Uptake in the Thyroid Gland and Association With Thyroid Pathologies. Thyroid (2009) 19(4):381–9. doi: 10.1089/thy.2008.0389
74. Krenning EP, Kwekkeboom DJ, Bakker WH, Breeman WA, Kooij PP, Oei HY, et al. Somatostatin Receptor Scintigraphy With [111In-DTPA-D-Phe1]- and [123I-Tyr3]-Octreotide: The Rotterdam Experience With More Than 1000 Patients. Eur J Nucl Med (1993) 20(8):716–31. doi: 10.1007/BF00181765
75. Tenenbaum F, Lumbroso J, Schlumberger M, Caillou B, Fragu P, Parmentier C. Radiolabeled Somatostatin Analog Scintigraphy in Differentiated Thyroid Carcinoma. J Nucl Med (1995) 36(5):807–10.
76. Baudin E, Schlumberger M, Lumbroso J, Travagli JP, Caillou B, Parmentier C. Octreotide Scintigraphy in Patients With Differentiated Thyroid Carcinoma: Contribution for Patients With Negative Radioiodine Scan. J Clin Endocrinol Metab (1996) 81(7):2541–4. doi: 10.1210/jcem.81.7.8675574
77. Postema PT, De Herder WW, Reubi JC, Oei HY, Kwekkeboom DJ, Bruining HJ, et al. Somatostatin Receptor Scintigraphy in non-Medullary Thyroid Cancer. Digestion (1996) 57 Suppl 1:36–7. doi: 10.1159/000201391
78. Valli N, Catargi B, Ronci N, Leccia F, Guyot M, Roger P, et al. Evaluation of Indium-111 Pentetreotide Somatostatin Receptor Scintigraphy to Detect Recurrent Thyroid Carcinoma in Patients With Negative Radioiodine Scintigraphy. Thyroid (1999) 9(6):583–9. doi: 10.1089/thy.1999.9.583
79. Görges R, Kahaly G, Müller-Brand J, Mäcke H, Roser HW, Bockisch A. Radionuclide-Labeled Somatostatin Analogues for Diagnostic and Therapeutic Purposes in Nonmedullary Thyroid Cancer. Thyroid (2001) 11(7):647–59. doi: 10.1089/105072501750362718
80. Garin E, Devillers A, Le Cloirec J, Bernard AM, Lescouarc’h J, Herry JY, et al. Use of Indium-111 Pentetreotide Somatostatin Receptor Scintigraphy to Detect Recurrent Thyroid Carcinoma in Patients Without Detectable Iodine Uptake. Eur J Nucl Med (1998) 25(7):687–94. doi: 10.1007/s002590050270
81. Haslinghuis LM, Krenning EP, De Herder WW, Reijs AE, Kwekkeboom DJ. Somatostatin Receptor Scintigraphy in the Follow-Up of Patients With Differentiated Thyroid Cancer. J Endocrinol Invest (2001) 24(6):415–22. doi: 10.1007/BF03351041
82. Christian JA, Cook GJ, Harmer C. Indium-111-Labelled Octreotide Scintigraphy in the Diagnosis and Management of non-Iodine Avid Metastatic Carcinoma of the Thyroid. Br J Cancer (2003) 89(2):258–61. doi: 10.1038/sj.bjc.6601072
83. Stokkel MP, Verkooijen RB, Smit JW. Indium-111 Octreotide Scintigraphy for the Detection of non-Functioning Metastases From Differentiated Thyroid Cancer: Diagnostic and Prognostic Value. Eur J Nucl Med Mol Imaging (2004) 31(7):950–7. doi: 10.1007/s00259-004-1478-0
84. Giammarile F, Houzard C, Bournaud C, Hafdi Z, Sassolas G, Borson-Chazot F. Diagnostic Management of Suspected Metastatic Thyroid Carcinoma: Clinical Value of Octreotide Scintigraphy in Patients With Negative High-Dose Radioiodine Scans. Eur J Endocrinol (2004) 150(3):277–83. doi: 10.1530/eje.0.1500277
85. Binse I, Poeppel TD, Ruhlmann M, Ezziddin S, Görges R, Sabet A, et al. 68Ga-DOTATOC PET/CT in Patients With Iodine- and 18F-FDG-Negative Differentiated Thyroid Carcinoma and Elevated Serum Thyroglobulin. J Nucl Med (2016) 57(10):1512–7. doi: 10.2967/jnumed.115.171942
86. Gabriel M, Decristoforo C, Donnemiller E, Ulmer H, Watfah Rychlinski C, Mather SJ, et al. An Intrapatient Comparison of 99mtc-EDDA/HYNIC-TOC With 111In-DTPA-Octreotide for Diagnosis of Somatostatin Receptor-Expressing Tumors. J Nucl Med (2003) 44(5):708–16.
87. Czepczyński R, Gryczyńska M, Ruchała M. 99mtc-EDDA/HYNIC-TOC in the Diagnosis of Differentiated Thyroid Carcinoma Refractory to Radioiodine Treatment. Nucl Med Rev Cent East Eur (2016) 19(2):67–73. doi: 10.5603/NMR.2016.0015
88. Wild D, Fani M, Behe M, Brink I, Rivier JE, Reubi JC, et al. First Clinical Evidence That Imaging With Somatostatin Receptor Antagonists is Feasible. J Nucl Med (2011) 52(9):1412–7. doi: 10.2967/jnumed.111.088922
89. Lapa C, Werner RA, Schmid JS, Papp L, Zsótér N, Biko J, et al. Prognostic Value of Positron Emission Tomography-Assessed Tumor Heterogeneity in Patients With Thyroid Cancer Undergoing Treatment With Radiopeptide Therapy. Nucl Med Biol (2015) 42(4):349–54. doi: 10.1016/j.nucmedbio.2014.12.006
90. Kwekkeboom DJ, Reubi JC, Lamberts SW, Bruining HA, Mulder AH, Oei HY, et al. In Vivo Somatostatin Receptor Imaging in Medullary Thyroid Carcinoma. J Clin Endocrinol Metab (1993) 76(6):1413–7. doi: 10.1210/jcem.76.6.8501144
91. Castroneves LA, Coura Filho G, de Freitas RMC, Salles R, Moyses RA, Lopez RVM, et al. Comparison of 68Ga PET/CT to Other Imaging Studies in Medullary Thyroid Cancer: Superiority in Detecting Bone Metastases. J Clin Endocrinol Metab (2018) 103(9):3250–9. doi: 10.1210/jc.2018-00193
92. Gao Z, Biersack HJ, Ezziddin S, Logvinski T, An R. The Role of Combined Imaging in Metastatic Medullary Thyroid Carcinoma: 111In-DTPA-Octreotide and 131I/123I-MIBG as Predictors for Radionuclide Therapy. J Cancer Res Clin Oncol (2004) 130(11):649–56. doi: 10.1007/s00432-004-0588-1
93. Fröberg AC, de Jong M, Nock BA, Breeman WA, Erion JL, Maina T, et al. Comparison of Three Radiolabelled Peptide Analogues for CCK-2 Receptor Scintigraphy in Medullary Thyroid Carcinoma. Eur J Nucl Med Mol Imaging (2009) 36(8):1265–72. doi: 10.1007/s00259-009-1098-9
94. Lee SW, Shim SR, Jeong SY, Kim SJ. Comparison of 5 Different PET Radiopharmaceuticals for the Detection of Recurrent Medullary Thyroid Carcinoma: A Network Meta-Analysis. Clin Nucl Med (2020) 45(5):341–8. doi: 10.1097/RLU.0000000000002940
95. Otte A, Herrmann R, Heppeler A, Behe M, Jermann E, Powell P, et al. Yttrium-90 DOTATOC: First Clinical Results. Eur J Nucl Med (1999) 26(11):1439–47. doi: 10.1007/s002590050476
96. Paganelli G, Zoboli S, Cremonesi M, Bodei L, Ferrari M, Grana C, et al. Receptor-Mediated Radiotherapy With 90Y-DOTA-D-Phe1-Tyr3-Octreotide. Eur J Nucl Med (2001) 28(4):426–34. doi: 10.1007/s002590100490
97. Waldherr C, Schumacher T, Pless M, Crazzolara A, Maecke HR, Nitzsche EU, et al. Radiopeptide Transmitted Internal Irradiation of non-Iodophil Thyroid Cancer and Conventionally Untreatable Medullary Thyroid Cancer Using [90Y]-DOTA-D-Phe1-Tyr3-Octreotide: A Pilot Study. Nucl Med Commun (2001) 22(6):673–8. doi: 10.1097/00006231-200106000-00011
98. Chinol M, Bodei L, Cremonesi M, Paganelli G. Receptor-Mediated Radiotherapy With Y-DOTA-DPhe-Tyr-Octreotide: The Experience of the European Institute of Oncology Group. Semin Nucl Med (2002) 32(2):141–7. doi: 10.1053/snuc.2002.31563
99. Valkema R, De Jong M, Bakker WH, Breeman WA, Kooij PP, Lugtenburg PJ, et al. Phase I Study of Peptide Receptor Radionuclide Therapy With [In-DTPA]octreotide: The Rotterdam Experience. Semin Nucl Med (2002) 32(2):110–22. doi: 10.1053/snuc/2002.31025
100. Virgolini I, Britton K, Buscombe J, Moncayo R, Paganelli G, Riva P. In- and Y-DOTA-Lanreotide: Results and Implications of the MAURITIUS Trial. Semin Nucl Med (2002) 32(2):148–55. doi: 10.1053/snuc.2002.31565
101. Bodei L, Cremonesi M, Zoboli S, Grana C, Bartolomei M, Rocca P, et al. Receptor-Mediated Radionuclide Therapy With 90Y-DOTATOC in Association With Amino Acid Infusion: A Phase I Study. Eur J Nucl Med Mol Imaging (2003) 30(2):207–16. doi: 10.1007/s00259-002-1023-y
102. Bodei L, Handkiewicz-Junak D, Grana C, Mazzetta C, Rocca P, Bartolomei M, et al. Receptor Radionuclide Therapy With 90Y-DOTATOC in Patients With Medullary Thyroid Carcinomas. Cancer Biother Radiopharm (2004) 19(1):65–71. doi: 10.1089/108497804773391694
103. Gabriel M, Froehlich F, Decristoforo C, Ensinger C, Donnemiller E, von Guggenberg E, et al. 99mtc-EDDA/HYNIC-TOC and (18)F-FDG in Thyroid Cancer Patients With Negative (131)I Whole-Body Scans. Eur J Nucl Med Mol Imaging (2004) 31(3):330–41. doi: 10.1007/s00259-003-1376-x
104. Teunissen JJ, Kwekkeboom DJ, Kooij PP, Bakker WH, Krenning EP. Peptide Receptor Radionuclide Therapy for non-Radioiodine-Avid Differentiated Thyroid Carcinoma. J Nucl Med (2005) 46 Suppl 1:107s–14s.
105. Iten F, M̈ller B, Schindler C, Rochlitz C, Oertli D, Mäcke HR, et al. Response to [90Yttrium-DOTA]-TOC Treatment is Associated With Long-Term Survival Benefit in Metastasized Medullary Thyroid Cancer: A Phase II Clinical Trial. Clin Cancer Res (2007) 13(22):6696–702. doi: 10.1158/1078-0432.CCR-07-0935
106. Budiawan H, Salavati A, Kulkarni HR, Baum RP. Peptide Receptor Radionuclide Therapy of Treatment-Refractory Metastatic Thyroid Cancer Using (90)Yttrium and (177)Lutetium Labeled Somatostatin Analogs: Toxicity, Response and Survival Analysis. Am J Nucl Med Mol Imaging (2013) 4(1):39–52.
107. Versari A, Sollini M, Frasoldati A, Fraternali A, Filice A, Froio A, et al. Differentiated Thyroid Cancer: A New Perspective With Radiolabeled Somatostatin Analogues for Imaging and Treatment of Patients. Thyroid (2014) 24(4):715–26. doi: 10.1089/thy.2013.0225
108. Vaisman F, Rosado de Castro PH, Lopes FP, Kendler DB, Pessoa CH, Bulzico DA, et al. Is There a Role for Peptide Receptor Radionuclide Therapy in Medullary Thyroid Cancer? Clin Nucl Med (2015) 40(2):123–7. doi: 10.1097/RLU.0000000000000628
109. Salavati A, Puranik A, Kulkarni HR, Budiawan H, Baum RP. Peptide Receptor Radionuclide Therapy (PRRT) of Medullary and Nonmedullary Thyroid Cancer Using Radiolabeled Somatostatin Analogues. Semin Nucl Med (2016) 46(3):215–24. doi: 10.1053/j.semnuclmed.2016.01.010
110. Roll W, Riemann B, Schäfers M, Stegger L, Vrachimis A. 177Lu-DOTATATE Therapy in Radioiodine-Refractory Differentiated Thyroid Cancer: A Single Center Experience. Clin Nucl Med (2018) 43(10):e346–e51. doi: 10.1097/RLU.0000000000002219
111. Beukhof CM, Brabander T, van Nederveen FH, van Velthuysen MF, de Rijke YB, Hofland LJ, et al. Peptide Receptor Radionuclide Therapy in Patients With Medullary Thyroid Carcinoma: Predictors and Pitfalls. BMC Cancer (2019) 19(1):325. doi: 10.1186/s12885-019-5540-5
112. Parghane RV, Naik C, Talole S, Desmukh A, Chaukar D, Banerjee S, et al. Clinical Utility of (177) Lu-DOTATATE PRRT in Somatostatin Receptor-Positive Metastatic Medullary Carcinoma of Thyroid Patients With Assessment of Efficacy, Survival Analysis, Prognostic Variables, and Toxicity. Head Neck (2020) 42(3):401–16. doi: 10.1002/hed.26024
113. Satapathy S, Mittal BR, Sood A, Verma R, Panda N. Efficacy and Safety of Concomitant 177Lu-DOTATATE and Low-Dose Capecitabine in Advanced Medullary Thyroid Carcinoma: A Single-Centre Experience. Nucl Med Commun (2020) 41(7):629–35. doi: 10.1097/MNM.0000000000001205
114. Hayes AR, Crawford A, Al Riyami K, Tang C, Bomanji J, Baldeweg SE, et al. Metastatic Medullary Thyroid Cancer: The Role of 68Gallium-DOTA-Somatostatin Analogue PET/CT and Peptide Receptor Radionuclide Therapy. J Clin Endocrinol Metab (2021) 106(12):e4903–e16. doi: 10.1210/clinem/dgab588
115. Czepczyński R, Matysiak-Grześ M, Gryczyńska M, Bączyk M, Wyszomirska A, Stajgis M, et al. Peptide Receptor Radionuclide Therapy of Differentiated Thyroid Cancer: Efficacy and Toxicity. Arch Immunol Ther Exp (Warsz) (2015) 63(2):147–54. doi: 10.1007/s00005-014-0318-6
116. Assadi M, Ahmadzadehfar H. (177)Lu-DOTATATE and (177)Lu-Prostate-Specific Membrane Antigen Therapy in a Patient With Advanced Metastatic Radioiodine-Refractory Differentiated Thyroid Cancer After Failure of Tyrosine Kinase Inhibitors Treatment. World J Nucl Med (2019) 18(4):406–8. doi: 10.4103/wjnm.WJNM_112_18
117. Donohoe KJ, Aloff J, Avram AM, Bennet KG, Giovanella L, Greenspan B, et al. Appropriate Use Criteria for Nuclear Medicine in the Evaluation and Treatment of Differentiated Thyroid Cancer. J Nucl Med (2020) 61(3):375–96. doi: 10.2967/jnumed.119.240945
118. Grossrubatscher E, Fanciulli G, Pes L, Sesti F, Dolci C, de Cicco F, et al. Advances in the Management of Medullary Thyroid Carcinoma: Focus on Peptide Receptor Radionuclide Therapy. J Clin Med (2020) 9(11). doi: 10.3390/jcm9113507
119. Makis W, McCann K, McEwan AJ. Medullary Thyroid Carcinoma (MTC) Treated With 177Lu-DOTATATE PRRT: A Report of Two Cases. Clin Nucl Med (2015) 40(5):408–12. doi: 10.1097/RLU.0000000000000706
120. Sharma R, Wang WM, Yusuf S, Evans J, Ramaswami R, Wernig F, et al. (68)Ga-DOTATATE PET/CT Parameters Predict Response to Peptide Receptor Radionuclide Therapy in Neuroendocrine Tumours. Radiother Oncol (2019) 141:108–15. doi: 10.1016/j.radonc.2019.09.003
121. Zhang J, Kulkarni HR, Singh A, Niepsch K, Müller D, Baum RP. Peptide Receptor Radionuclide Therapy in Grade 3 Neuroendocrine Neoplasms: Safety and Survival Analysis in 69 Patients. J Nucl Med (2019) 60(3):377–85. doi: 10.2967/jnumed.118.215848
122. Fu H, Sa R, Cheng L, Jin Y, Qiu X, Liu M, et al. Updated Review of Nuclear Molecular Imaging of Thyroid Cancers. Endocr Pract (2021) 27(5):494–502. doi: 10.1016/j.eprac.2020.10.001
123. Lee DY, Kim YI. Peptide Receptor Radionuclide Therapy in Patients With Differentiated Thyroid Cancer: A Meta-Analysis. Clin Nucl Med (2020) 45(8):604–10. doi: 10.1097/RLU.0000000000003110
124. Maghsoomi Z, Emami Z, Malboosbaf R, Malek M, Khamseh ME. Efficacy and Safety of Peptide Receptor Radionuclide Therapy in Advanced Radioiodine-Refractory Differentiated Thyroid Cancer and Metastatic Medullary Thyroid Cancer: A Systematic Review. BMC Cancer (2021) 21(1):579. doi: 10.1186/s12885-021-08257-x
125. Pisarek H, Pawlikowski M, Marchlewska M, Minias R, Winczyk K. An Immunohistochemical Investigation of the Expression of Somatostatin Receptor Subtypes - Should Therapeutic Trials be Performed to Determine the Efficacy of Somatostatin Analogs in Treating Advanced Thyroid Malignances? Exp Clin Endocrinol Diabetes (2015) 123(6):342–6. doi: 10.1055/s-0035-1548825
126. Wild D, Schmitt JS, Ginj M, Mäcke HR, Bernard BF, Krenning E, et al. DOTA-NOC, a High-Affinity Ligand of Somatostatin Receptor Subtypes 2, 3 and 5 for Labelling With Various Radiometals. Eur J Nucl Med Mol Imaging (2003) 30(10):1338–47. doi: 10.1007/s00259-003-1255-5
127. Komek H, Ansal Balci T, Can C. Efficacy of Galium-68 DOTATATE PET/CT in the Detection of Metastasis Rate of Well-Differentiated Gastroenteropancreatic Neuroendocrine Tumors. Asia Ocean J Nucl Med Biol (2019) 7(2):141–8. doi: 10.22038/AOJNMB.2019.13348
128. Garin E, Tselikas L, Guiu B, Chalaye J, Edeline J, de Baere T, et al. Personalised Versus Standard Dosimetry Approach of Selective Internal Radiation Therapy in Patients With Locally Advanced Hepatocellular Carcinoma (DOSISPHERE-01): A Randomised, Multicentre, Open-Label Phase 2 Trial. Lancet Gastroenterol Hepatol (2021) 6(1):17–29. doi: 10.1016/S2468-1253(20)30290-9
129. Kunikowska J, Królicki L. Targeted α-Emitter Therapy of Neuroendocrine Tumors. Semin Nucl Med (2020) 50(2):171–6. doi: 10.1053/j.semnuclmed.2019.11.003
130. Graf F, Fahrer J, Maus S, Morgenstern A, Bruchertseifer F, Venkatachalam S, et al. DNA Double Strand Breaks as Predictor of Efficacy of the Alpha-Particle Emitter Ac-225 and the Electron Emitter Lu-177 for Somatostatin Receptor Targeted Radiotherapy. PloS One (2014) 9(2):e88239. doi: 10.1371/journal.pone.0088239
131. Taelman VF, Radojewski P, Marincek N, Ben-Shlomo A, Grotzky A, Olariu CI, et al. Upregulation of Key Molecules for Targeted Imaging and Therapy. J Nucl Med (2016) 57(11):1805–10. doi: 10.2967/jnumed.115.165092
Keywords: thyroid cancer, PRRT, DOTATATE, medullary thyroid cancer (MTC), somatostatin receptor
Citation: Gubbi S, Koch CA and Klubo-Gwiezdzinska J (2022) Peptide Receptor Radionuclide Therapy in Thyroid Cancer. Front. Endocrinol. 13:896287. doi: 10.3389/fendo.2022.896287
Received: 14 March 2022; Accepted: 19 April 2022;
Published: 30 May 2022.
Edited by:
Laura Boucai, Memorial Sloan Kettering Cancer Center, United StatesReviewed by:
Yevgeniya Kushchayeva, University of South Florida, United StatesCopyright © 2022 Gubbi, Koch and Klubo-Gwiezdzinska. This is an open-access article distributed under the terms of the Creative Commons Attribution License (CC BY). The use, distribution or reproduction in other forums is permitted, provided the original author(s) and the copyright owner(s) are credited and that the original publication in this journal is cited, in accordance with accepted academic practice. No use, distribution or reproduction is permitted which does not comply with these terms.
*Correspondence: Joanna Klubo-Gwiezdzinska, joanna.klubo-gwiezdzinska@nih.gov
Disclaimer: All claims expressed in this article are solely those of the authors and do not necessarily represent those of their affiliated organizations, or those of the publisher, the editors and the reviewers. Any product that may be evaluated in this article or claim that may be made by its manufacturer is not guaranteed or endorsed by the publisher.
Research integrity at Frontiers
Learn more about the work of our research integrity team to safeguard the quality of each article we publish.