- 1Department of Endocrinology, Nanjing First Hospital, Nanjing Medical University, Nanjing, China
- 2Department of Endocrinology, Wuxi People’s Hospital Affiliated to Nanjing Medical University, Wuxi, China
- 3Department of Endocrinology, Chunjiang People’s Hospital, Changzhou, China
Objective: Our previous study has found that short-term intensive insulin therapy in patients with newly diagnosed type 2 diabetes mellitus (T2DM) increased serum testosterone levels, but the underlying mechanisms remain unclear.
Design and methods: In this self-controlled study, 43 men with newly diagnosed drug naïve T2DM, aged 18-60 years, with HbA1c >9.0% were treated with continuous subcutaneous insulin infusion (CSII) to normalize blood glucose within one week. Venous blood specimens were collected for measuring of serum total testosterone, dehydroepiandrosterone sulfate (DHEA-S), 3β- and 17β-hydroxysteroid dehydrogenase (3β- and 17β-HSD) concentrations before and after insulin therapy.
Results: Testosterone increased from 13.0 (11.3, 14.6) nmol/L to 15.7 (13.9, 17.5) nmol/L after intensive insulin therapy (p<0.001), while the levels of DHEA-S decreased significantly after treatment (from 6.5 (5.7, 7.3) μmol/L to 6.0 (5.3, 6.7) μmol/L, p=0.001). The ratio of testosterone/DHEA-S increased significantly (2.4 (2.0, 2.8) vs. 3.1 (2.6, 3.7) nmol/μmol, p<0.001). After blood glucose normalization with the short-term CSII therapy, 3β-HSD increased from 11.0 (9.5, 12.5) pg/mL to 14.6 (13.5, 15.7) pg/mL, p=0.001, and 17β-HSD increased from 20.7 (16.3, 25.2) pg/mL to 28.2 (23.8, 32.5) pg/mL, p=0.009.
Conclusions: Blood glucose normalization via short-term intensive insulin therapy increases plasma total testosterone levels in men with newly diagnosed type 2 diabetes, associated with a decreased level of DHEA-S, probably because of the enhanced conversion from DHEA to testosterone catalyzed by 3β-HSD and 17β-HSD.
Introduction
Increasing evidence has indicated that testosterone level was significantly lower in men with type 2 diabetes mellitus (T2DM) (1). Reduced testosterone was recognized as an accompanying phenomena of reduced insulin sensitivity (2), poor glycemic control (3), and obesity (4). In our previous study, a short-term intensive insulin therapy of 3-5 days upregulated serum testosterone levels significantly (5), while the underlying mechanisms remain unclear.
In testes, 3β-hydroxysteroid dehydrogenase (3β-HSD) and 17β-hydroxysteroid dehydrogenase (17β-HSD) are the key enzymes in changing the dehydroepiandrosterone (DHEA) into testosterone (6). It has been reported that insulin administration increased testosterone synthesis by stimulating testicular β-HSD activity in streptozotocin-induced diabetic rats (7). In vitro studies, insulin stimulated the HSD activity of human placental cytotrophoblasts (8) and ovarian thecal-like tumor cells (9). Therefore, we hypothesized that short-term insulin therapy might increase transformation from DHEA to testosterone via regulating β-HSD activity. However, the relationship between insulin therapy and DHEA and β-HSD levels in patients with diabetes has not been reported.
Therefore, we further analyzed the changes of dehydroepiandrosterone sulfate [DHEA-S, the major circulating form of DHEA (10)] and testosterone levels, as well as the 3 and 17 β-HSD activity after intensive insulin therapy in newly diagnosed males with T2DM.
Materials and methods
Study design and participants
The present study is a secondary analysis of our previous study (clinicaltrials.gov, NCT03982238) (5). This study recruited men with newly diagnosed T2DM from June 2019 to November 2019. The inclusion criteria were as follows: (1) aged 18-60 years, met the WHO 1999 diagnostic criteria of diabetes, and had not been treated with any hypoglycemic drugs; (2) who had an HbA1c 9.0% (75 mmol/mol) or higher which was recommended to take intense insulin therapy according to the Chinese Guidelines for the prevention and management of T2DM (2017 edition); (3) willingness to follow dietary and exercise advice. Exclusion criteria were as follows: (1) already on treatment with lipid-lowering or anti-hypertensive medications; (2) with serum alanine aminotransferase (ALT) levels more than 2.5 times the upper normal range (100 U/L) or creatinine levels more than 1.3 upper normal range (105 μmol/L); (3) a history of systemic corticosteroids use in the past 3 months; (4) any infection or acute diabetic complication such as ketoacidosis or hyperosmolar state (coma); (5) patients who developed insulin allergy.
Procedures
After admission, baseline parameters of height, weight, age, and medical history were collected. Body mass index (BMI) was calculated as weight divided by the square of height (kg/m2). Blood samples were collected after an overnight fast for assessment of blood glucose, beta-cell function (C-peptide and insulin levels), HbA1c, testosterone, DHEA-S and 3/17 β-HSD. The patients were then started on intensive treatment with continuous subcutaneous insulin infusion (CSII). The patients were tested for capillary blood glucose at least 7 times a day and insulin doses were titrated daily according to blood glucose levels by specialist physicians. In our hospital, this almost always restores the glucose values to the normal range within 3 to 5 days, and no more than a week.
After achieving the required standard of satisfactory blood glucose levels (>80% of values within the range of pre-meal blood glucose 3.9~7.0 mmol/L, postprandial blood glucose 3.9~10.0 mmol/L), measurements of fasting blood glucose, beta-cell function, testosterone, DHEA-S and 3/17 β-HSD were performed again.
Laboratory tests
Fasting blood glucose was measured by an auto-analyzer (Modular E170; Roche, Mannheim, Germany); HbA1c was measured using high-performance liquid chromatography assay (Bio-Rad Laboratories, USA); C-peptide, insulin, and sexual hormones including total testosterone, DHEA-S, estradiol, luteinizing hormone (LH), follicle-stimulating hormone (FSH), and sex hormone binding globulin (SHBG) were measured using chemiluminescent microparticle immunoassay (Architect system, USA). 3β-HSD and 17β-HSD were measured by enzyme-linked immunosorbent assay (Jiangsu Meimian Industrial, China). Both the intra- and inter-assay coefficients of variation were less than 10%.
Statistical analysis
All statistical analyses were performed using SPSS version 22.0 software (IBM Corp., USA). All variables were tested for normal distribution. Data are presented as mean (95%CI) or percentage. Changes from baseline to the endpoint of the study were assessed by a paired t-test for parametric data or a Wilcoxon for nonparametric data, respectively. Spearman analysis was used to find the factors which may be correlated with the testosterone/DHEA-S transform. The categorical data were examined with chi-square test. A p value < 0.05 was considered statistically significant.
We tested the 3/17 β-HSD before and after intensive insulin therapy in six subjects in a pre-study. The 3β-HSD increased from 11.8 to 15.0 pg/ml, and the standard deviation was 3.2 pg/ml. The 17β-HSD increased from 15.0 to 26.7 pg/ml, and the standard deviation was 11.7 pg/ml. Therefore, we need at least 13 subjects with 80% power and an α of 0.05. The sample size was calculated using PASS software.
Results
1. Clinical and biochemical characteristics at baseline
A total of 43 males who had data of testosterone and DHEA-S levels were enrolled in the study. The mean age of these patients was 45.7 (42.6, 48.9) years, BMI was 25.5 (24.5, 26.6) kg/m2, and the HbA1c was 10.8 (10.3, 11.2) %.
2. Changes of serum testosterone and DHEA-S levels
We measured testosterone and DHEA-S levels before and after to explore the change of 3/17 β-HSD activity. After the intensive insulin therapy, testosterone level increased significantly (from 13.0 (11.3, 14.6) nmol/L to 15.7 (13.9, 17.5) nmol/L, p<0.001, Figure 1A), while DHEA-S level decreased (from 6.5 (5.7, 7.3) μmol/L to 6.0 (5.3, 6.7) μmol/L, p= 0.001, Figure 1B). Therefore, the ratio of testosterone/DHEA-S increased significantly (2.4 (2.0, 2.8) nmol/μmol vs. 3.1 (2.6, 3.7) nmol/μmol, p<0.001, Figure 1C). Free testosterone (FT), bioavailable testosterone (Bio-T) were also calculated as reported previously (11). FT increased from 0.21 (0.18, 0.24) nmol/L to 0.26 (0.22, 0.29) nmol/L (p<0.001), and Bio-T increased from 4.9 (4.3, 5.4) nmol/L to 5.6 (5.0, 6.1) nmol/L (p = 0.001).
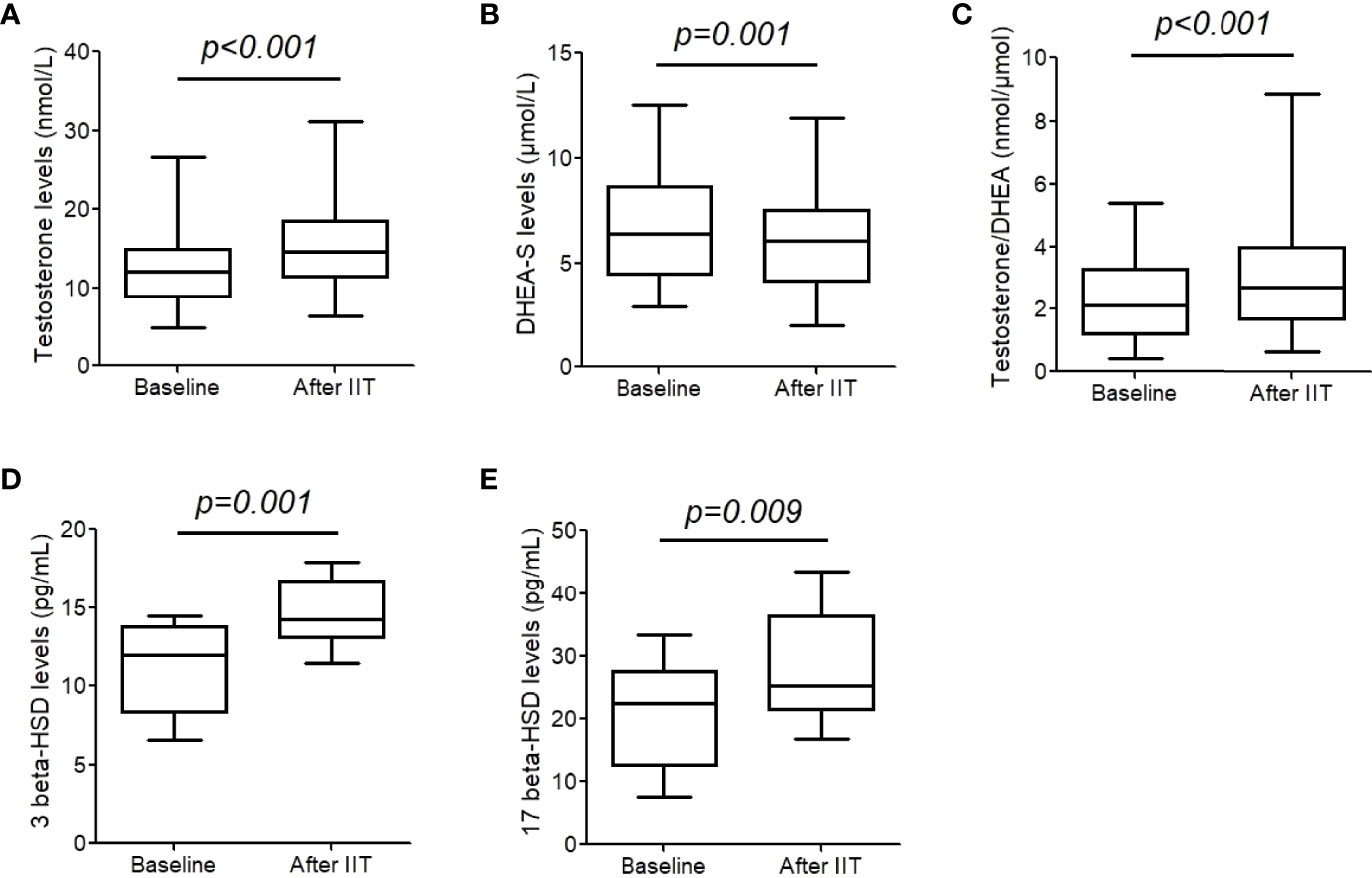
Figure 1 Testosterone, dehydroepiandrosterone sulfate (DHEA-S), and 3/17 β-hydroxysteroid dehydrogenase (HSD) levels before and after intensive insulin therapy (IIT). (A-C) testosterone and DHEA-S levels were measure in 43 patients with T2DM. (D, E) 3/17 β- HSD levels were measured in 17 patients. The differences between two groups were analyzed were assessed by a paired t-test. The whiskers of the box were showed as 5–95 percentile.
3. Changes of serum 3/17β-HSD concentrations
We further performed serum 3 and 17 β-HSD concentrations in 17 males. After blood glucose normalization with the short-term CSII therapy, 3β-HSD increased from 11.0 (9.5, 12.5) pg/mL to 14.6 (13.5, 15.7) pg/mL, p= 0.001, and 17β-HSD increased from 20.7 (16.3, 25.2) pg/mL to 28.2 (23.8, 32.5) pg/mL, p= 0.009 (Figures 1D, E).
4. Changes of other sexual hormones
As shown in Figure 2, estrogen and LH did not change significantly after intensive insulin therapy (both p >0.05). However, SHBG significantly increased (22.9 (19.2, 26.7) nmol/L vs. 28.8 (24.5, 33.0) nmol/L, p< 0.001), and FSH decreased from 5.3 (4.2, 6.4) IU/L to 5.0 (4.0,6.1) IU/L, p = 0.003.
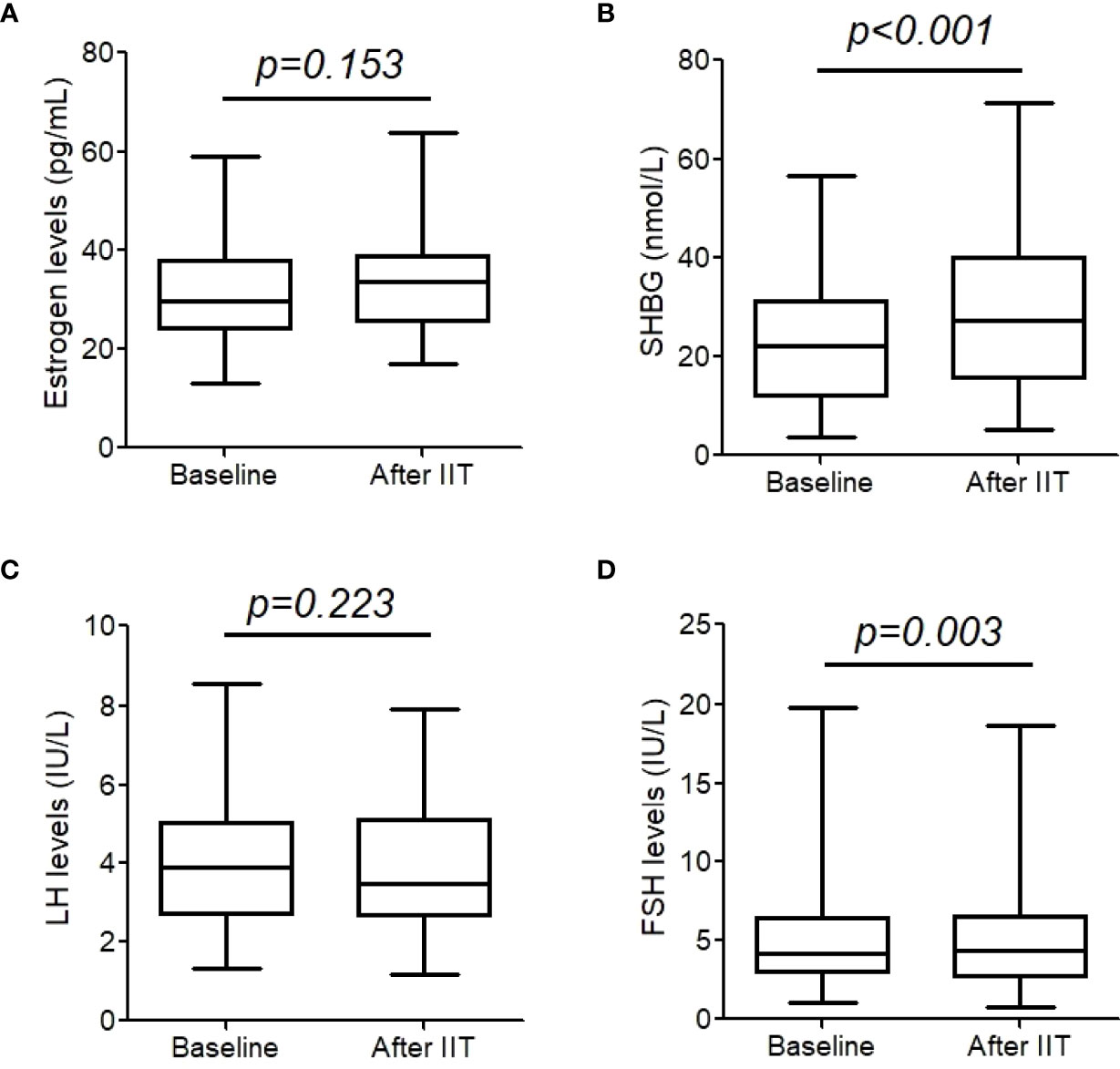
Figure 2 Estrogen (A), sex hormone binding protein (SHBG) (B), luteinizing hormone (LH) (C), and follicle-stimulating hormone (FSH) (D) before and after intensive insulin therapy (IIT). These hormones were measure in 43 patients with T2DM. The whiskers of the box were showed as 5–95 percentile.
5. Changes of blood glucose and insulin levels
The average insulin dose used in the insulin pump was 0.6 (0.5,0.7) IU/kg per day when the blood glucose normalized. Fasting blood glucose decreased from 9.6 (8.7, 10.5) mmol/L to 6.1 (5.5, 6.6) mmol/L, p<0.001. Fasting insulin levels increased from 9.5 (7.8, 11.1) IU/L to 15.0 (12.1, 18.0) IU/L (p< 0.001), and C-peptide decreased from 1.5 (1.2, 1.7) ng/mL to 0.6 (0.5, 0.7) ng/mL (p= 0.007).
6. Factors that may influence the ratio of testosterone/DHEA-S
Spearman analysis showed that the change of testosterone/DHEA-S (the ratio of testosterone/DHEA-S after intensive insulin therapy minus that at baseline, Δtestosterone/DHEA-S) was correlated with Δ17β-HSD (r = 0.571, p = 0.013). The correlation between Δtestosterone/DHEA-S and Δ3β-HSD was not statistically significant (r = 0.401, p = 0.099), and there were no significant differences between Δtestosterone/DHEA-S and insulin dose at the time point of blood glucose normalization (r = -0.140, p = 0.578), the change of serum insulin levels (r = -0.051, p = 0.830), and the change of fasting blood glucose (r = 0.006, p = 0.972).
Discussion
The present study demonstrates for the first time that in men with newly diagnosed T2DM, short-term insulin therapy upregulated testosterone level, accompanied by a decreased DHEA-S level. Insulin administration enhanced the activities of 3β-HSD and 17β-HSD, which may at least partly explain the mechanisms of insulin therapy on testosterone elevation.
A number of studies have confirmed that poor glycemic control and insulin resistance lead to low testosterone levels, testosterone deficiency, and male hypogonadism (12, 13). Intensive insulin therapy can improve glycemic control effectively and rapidly in patients with T2DM (14). In the present study, we found that in male patients with newly diagnosed T2DM, intensive insulin therapy within a week increased their circulating testosterone levels, including total testosterone, FT and Bio-T levels. The results confirmed our previous study (5). Gagliano et al. found that in non-diabetic subjects, ingestion of either a glucose load or a mixed meal resulted in a significantly increased blood glucose as well as a decrease in serum total testosterone levels, suggesting that changes in blood glucose can have a rapid and significant effect on testosterone levels (15). While in males with obesity and T2DM, researchers believed that weight loss more than glycemic control may improve testosterone levels (16). They found that circulating free testosterone increased after bariatric surgery (17) as well as exenatide therapy (18, 19). However, in our study, CSII therapy for a week increased serum testosterone without significant weight change, suggesting that, in those subjects, the elevated testosterone levels are mainly due to the improved glycemic control or direct action of insulin on Leydig cells.
DHEA, a steroid hormone produced mainly by the adrenal cortex, works as the most important precursor for testosterone production. Therefore, DHEA has been aggressively sold as a dietary supplement to boost testosterone levels (20). In Leydig cells, DHEA-S is hydrolyzed back to DHEA by steroid sulfatase for further conversion. Firstly, DHEA is oxidized by 3β-HSD on the 3β-hydroxy group to generate androstenedione, later then catalyzed by 17β-HSD and results in a reduction of its C17 keto group to a β-hydroxyl group, and finally generates testosterone (10). Interestingly, it has been reported that insulin reduces serum DHEA and DHEA-S in men either by inhibiting their production or by increasing the metabolic clearance rate of DHEA (21). In addition, patients with T2DM, whose serum insulin levels were high, had significantly lower DHEA and DHEA-S serum levels than normal subjects and patients with T2DM whose serum insulin levels were normal (22). In PCOS rat models induced by DHEA administration, serum testosterone concentrations significantly increased with DHEA administration, but the increase was inhibited by oral administration of insulin-lowering agents (23). In the present study, we also found a reduction of serum DHEA-S levels in those newly diagnosed males with T2DM after insulin administration, which means that DHEA has a contradictory tendency to testosterone under insulin therapy, indicating that insulin may promote the conversion from DHEA to testosterone.
Previous studies showed that STZ induced diabetic rats have a marked reduction in serum testosterone as well as the activities of Leydig cellular 3β-and 17β-HSD, while insulin treatment reversed these changes (24). Our present study directly confirmed the activation of 3β-and 17β-HSD by short-term insulin therapy in humans in vivo for the first time. Certain commercially available drugs, such as gentamicin, can decrease the basal plasma testosterone concentration through inhibiting 3 and 17β HSD activity (25), while Ginkgo biloba extract can induce an ascending tendency of the expression of 17β-HSD3 and 3β-HSD1 and significantly increased the concentrations of serum testosterone levels in patients with T2DM (26). Thus, it is quite possible that the upregulating effect of insulin on serum testosterone levels as well as the downregulating effect on DHEA, are mainly dependent on the enhanced activities of 3β-HSD and 17β-HSD, especially 17β-HSD according to our correlation analysis. In future, further research is needed to understand the effect of insulin on testosterone.
The effects of DHEA and DHEA-S other than sex hormones have attracted increasing attentions recently. A comment in Nature reported a study that the combination of growth hormone, metformin, and DHEA treatment can reverse biological age (27, 28). In male patients with T2DM, low serum DHEA-S concentration has reported as a predictor for deterioration of urinary albumin excretion (29). Previous study also showed that serum DHEA-S concentration was negatively associated with carotid atherosclerosis in men with type 2 diabetes (30). DHEA and DHEA-S present protective actions on the cardiovascular system (31). Moreover, DHEA may have acute effects to protect against hypoglycemia-associated neuroendocrine and autonomic failure in healthy humans (32). Thus, for patients who are receiving insulin therapy, whether DHEA replacement, rather than testosterone may have long-term benefit deserves further prospective study.
Consistent with previous studies, insulin therapy increased serum SHBG levels (33). Studies in vivo have shown that metabolic disturbances can decrease SHBG production through inhibiting HepG2 expression (34). Therefore, the upregulated SHBG level after insulin therapy may be due to the improvement of glycemic control. In the present study, FSH were decreased after insulin therapy. FSH mainly stimulates Sertoli cells to secrete androgen-binding protein (35), and also increases testosterone levels by amplifying the LH response through the 17β HSD (36). Therefore, we speculated that the elevation of 17β HSD and SHBG may inhibit FSH levels through a feedback regulatory mechanism. However, the reason of FSH reduction after insulin therapy needs further study. It is a pity that we failed to find any correlation between the blood glucose or insulin dose with the change of testosterone/DHEA, the possible reason may be due to the limited sample size. In addition, another group of subjects, which achieves the standard glycemic range through dietary control only, should be set up to further clarify the regulation mechanism of insulin on testosterone, either due to the direct effect of insulin itself or secondary to the decreased glycemic level.
In conclusion, short-term insulin intensive therapy in patients with T2DM can increase 3 and 17 β-HSD levels, which leads to elevation of testosterone and reduction of DHEA levels. Given the importance of DHEA for health, further studies are necessary to verify the present results by replicating them in a longer-term randomized controlled study.
Data availability statement
The original contributions presented in the study are included in the article/supplementary material. Further inquiries can be directed to the corresponding authors.
Ethics statement
The studies involving human participants were reviewed and approved by Institutional Ethics Committee of Nanjing First Hospital, Nanjing Medical University. The patients/participants provided their written informed consent to participate in this study.
Author contribution
Conceptualization: BD, J-HM. Data curation: YH, YW, T-TC, LL, D-ML. Formal analysis: YW, YH. Funding acquisition: J-HM. Investigation: D-ML. Methodology: YH, BD. Project administration: J-HM, BD. Resources: J-HM. Software: YH. Supervision: J-HM. Validation: J-HM. Visualization: YH. Writing – original draft: YW. Writing – review and editing: YH. All authors contributed to the article and approved the submitted version.
Funding
This study was supported by the National Natural Science Foundation of China (No. 81870563).
Conflict of interest
The authors declare that the research was conducted in the absence of any commercial or financial relationships that could be construed as a potential conflict of interest.
Publisher’s note
All claims expressed in this article are solely those of the authors and do not necessarily represent those of their affiliated organizations, or those of the publisher, the editors and the reviewers. Any product that may be evaluated in this article, or claim that may be made by its manufacturer, is not guaranteed or endorsed by the publisher.
Acknowledgments
We thank the participants for their cooperation, and members of Endocrinology department of Nanjing First Hospital for their support. The guarantor is of this manuscript is J-HM.
References
1. Ding EL, Song Y, Malik VS, Liu S. Sex differences of endogenous sex hormones and risk of type 2 diabetes: a systematic review and meta-analysis. Jama (2006) 295(11):1288–99. doi: 10.1001/jama.295.11.1288
2. Souteiro P, Belo S, Oliveira SC, Neves JS, Magalhaes D, Pedro J, et al. Insulin resistance and sex hormone-binding globulin are independently correlated with low free testosterone levels in obese males. Andrologia (2018) 50(7):e13035. doi: 10.1111/and.13035
3. Sepu N, Adeleye JO, Kuti MO. Serum testosterone in Nigerian men with type 2 diabetes mellitus and its relationship with insulin sensitivity and glycemic control. J Natl Med Assoc (2021) 113(3):285–93. doi: 10.1016/j.jnma.2020.11.014
4. Kapoor D, Aldred H, Clark S, Channer KS, Jones TH. Clinical and biochemical assessment of hypogonadism in men with type 2 diabetes: correlations with bioavailable testosterone and visceral adiposity. Diabetes Care (2007) 30(4):911–7. doi: 10.2337/dc06-1426
5. Hu Y, Ding B, Shen Y, Yan RN, Li FF, Sun R, et al. Rapid changes in serum testosterone in men with newly diagnosed type 2 diabetes with intensive insulin and metformin. Diabetes Care (2021) 44(4):1059–61. doi: 10.2337/dc20-1558
6. Payne AH, Hales DB. Overview of steroidogenic enzymes in the pathway from cholesterol to active steroid hormones. Endocrine Rev (2004) 25(6):947–70. doi: 10.1210/er.2003-0030
7. Blanco FL, Fanjul LF, Ruiz de Galarreta CM. The effect of insulin and luteinizing hormone treatment on serum concentrations of testosterone and dihydrotestosterone and testicular 3 beta-hydroxysteroid dehydrogenase activity in intact and hypophysectomized diabetic rats. Endocrinology (1981) 109(4):1248–53. doi: 10.1210/endo-109-4-1248
8. Nestler JE. Insulin and insulin-like growth factor-I stimulate the 3 beta-hydroxysteroid dehydrogenase activity of human placental cytotrophoblasts. Endocrinology (1989) 125(4):2127–33. doi: 10.1210/endo-125-4-2127
9. McGee EA, Sawetawan C, Bird I, Rainey WE, Carr BR. The effect of insulin and insulin-like growth factors on the expression of steroidogenic enzymes in a human ovarian thecal-like tumor cell model. Fertil Steril (1996) 65(1):87–93. doi: 10.1016/s0015-0282(16)58032-7
10. Klinge CM, Clark BJ, Prough RA. Dehydroepiandrosterone research: Past, current, and future. Vitam Hormones (2018) 108:1–28. doi: 10.1016/bs.vh.2018.02.002
11. de Ronde W, van der Schouw YT, Pols HA, Gooren LJ, Muller M, Grobbee DE, et al. Calculation of bioavailable and free testosterone in men: a comparison of 5 published algorithms. Clin Chem (2006) 52(9):1777–84. doi: 10.1373/clinchem.2005.063354
12. Defeudis G, Mazzilli R, Gianfrilli D, Lenzi A, Isidori AM. The CATCH checklist to investigate adult-onset hypogonadism. Andrology (2018) 6(5):665–79. doi: 10.1111/andr.12506
13. El-Sakka AI, Sayed HM, Tayeb KA. Type 2 diabetes-associated androgen alteration in patients with erectile dysfunction. Int J Androl (2008) 31(6):602–8. doi: 10.1111/j.1365-2605.2007.00815.x
14. Corbett EC. Intensive insulin therapy improved glycemic control more than oral hypoglycemic agents in newly diagnosed type 2 diabetes. ACP J Club (2008) 149(3):5. doi: 10.7326/0003-4819-149-6-200809160-02005
15. Gagliano-Juca T, Li Z, Pencina KM, Beleva YM, Carlson OD, Egan JM, et al. Oral glucose load and mixed meal feeding lowers testosterone levels in healthy eugonadal men. Endocrine (2019) 63(1):149–56. doi: 10.1007/s12020-018-1741-y
16. Giagulli VA, Castellana M, Carbone MD, Pelusi C, Ramunni MI, De Pergola G, et al. Weight loss more than glycemic control may improve testosterone in obese type 2 diabetes mellitus men with hypogonadism. Andrology (2020) 8(3):654–62. doi: 10.1111/andr.12754
17. Botella-Carretero JI, Balsa JA, Gomez-Martin JM, Peromingo R, Huerta L, Carrasco M, et al. Circulating free testosterone in obese men after bariatric surgery increases in parallel with insulin sensitivity. J Endocrinol Invest (2013) 36(4):227–32. doi: 10.3275/8469
18. Shao N, Yu XY, Yu YM, Li BW, Pan J, Wu WH, et al. Short-term combined treatment with exenatide and metformin is superior to glimepiride combined metformin in improvement of serum testosterone levels in type 2 diabetic patients with obesity. Andrologia (2018) 50(7):e13039. doi: 10.1111/and.13039
19. Kapoor D, Channer KS, Jones TH. Rosiglitazone increases bioactive testosterone and reduces waist circumference in hypogonadal men with type 2 diabetes. Diabetes Vasc Dis Res (2008) 5(2):135–7. doi: 10.3132/dvdr.2008.022
20. Li Y, Ren J, Li N, Liu J, Tan SC, Low TY, et al. A dose-response and meta-analysis of dehydroepiandrosterone (DHEA) supplementation on testosterone levels: perinatal prediction of randomized clinical trials. Exp Gerontol (2020) 141:111110. doi: 10.1016/j.exger.2020.111110
21. Nestler JE. Regulation of human dehydroepiandrosterone metabolism by insulin. Ann New York Acad Sci (1995) 774:73–81. doi: 10.1111/j.1749-6632.1995.tb17373.x
22. Yamaguchi Y, Tanaka S, Yamakawa T, Kimura M, Ukawa K, Yamada Y, et al. Reduced serum dehydroepiandrosterone levels in diabetic patients with hyperinsulinaemia. Clin Endocrinol (1998) 49(3):377–83. doi: 10.1046/j.1365-2265.1998.00533.x
23. Misugi T, Ozaki K, El Beltagy K, Tokuyama O, Honda K, Ishiko O. Insulin-lowering agents inhibit synthesis of testosterone in ovaries of DHEA-induced PCOS rats. Gynecol Obstetr Invest (2006) 61(4):208–15. doi: 10.1159/000091496
24. Sudha S, Valli G, Julie PM, Arunakaran J, Govindarajulu P, Balasubramanian K. Influence of streptozotocin-induced diabetes and insulin treatment on the pituitary-testicular axis during sexual maturation in rats. Exp Clin Endocrinol Diabetes (2000) 108(1):14–20. doi: 10.1055/s-0032-1329210
25. Poirier D. Inhibitors of 17 beta-hydroxysteroid dehydrogenases. Curr Med Chem (2003) 10(6):453–77. doi: 10.2174/0929867033368222
26. Wu XY, Wang WY, Wang RR, Xie L, Fang ZX, Chen GR. [Ginkgo biloba extract enhances testosterone synthesis of leydig cells in type 2 diabetic rats]. Zhonghua nan ke xue = Natl J Androl (2008) 14(4):371–6. doi: 10.3969/j.issn.1009-3591.2008.04.021
27. Abbott A. First hint that body’s ‘biological age’ can be reversed. Nature (2019) 573(7773):173. doi: 10.1038/d41586-019-02638-w
28. Fahy GM, Brooke RT, Watson JP, Good Z, Vasanawala SS, Maecker H, et al. Reversal of epigenetic aging and immunosenescent trends in humans. Aging Cell (2019) 18(6):e13028. doi: 10.1111/acel.13028
29. Fukui M, Kitagawa Y, Kamiuchi K, Hasegawa G, Yoshikawa T, Nakamura N. Low serum dehydroepiandrosterone sulfate concentration is a predictor for deterioration of urinary albumin excretion in male patients with type 2 diabetes. Diabetes Res Clin Pract (2006) 73(1):47–50. doi: 10.1016/j.diabres.2005.11.006
30. Fukui M, Kitagawa Y, Nakamura N, Kadono M, Yoshida M, Hirata C, et al. Serum dehydroepiandrosterone sulfate concentration and carotid atherosclerosis in men with type 2 diabetes. Atherosclerosis (2005) 181(2):339–44. doi: 10.1016/j.atherosclerosis.2005.01.014
31. Mannella P, Simoncini T, Caretto M, Genazzani AR. Dehydroepiandrosterone and cardiovascular disease. Vitam Horm (2018) 108:333–53. doi: 10.1016/bs.vh.2018.05.001
32. Mikeladze M, Hedrington MS, Joy N, Tate DB, Younk LM, Davis I, et al. Acute effects of oral dehydroepiandrosterone on counterregulatory responses during repeated hypoglycemia in healthy humans. Diabetes (2016) 65(10):3161–70. doi: 10.2337/db16-0406
33. Tong G, Hua X, Zhong Y, Zhang K, Gu G, Feng W, et al. Intensive insulin therapy increases sex hormone-binding globulin in newly diagnosed type 2 diabetic patients. Eur J Endocrinol (2014) 170(2):237–45. doi: 10.1530/EJE-13-0557
34. Selva DM, Hogeveen KN, Innis SM, Hammond GL. Monosaccharide-induced lipogenesis regulates the human hepatic sex hormone-binding globulin gene. J Clin Invest (2007) 117(12):3979–87. doi: 10.1172/JCI32249
35. Santiemma V, Rosati P, Guerzoni C, Mariani S, Beligotti F, Magnanti M, et al. Human sertoli cells in vitro: morphological features and androgen-binding protein secretion. J Steroid Biochem Mol Biol (1992) 43(5):423–9. doi: 10.1016/0960-0760(92)90080-3
36. Levalle O, Zylbersztein C, Aszpis S, Aquilano D, Terradas C, Colombani M, et al. Recombinant human follicle-stimulating hormone administration increases testosterone production in men, possibly by a sertoli cell-secreted nonsteroid factor. J Clin Endocrinol Metab (1998) 83(11):3973–6. doi: 10.1210/jcem.83.11.5177
Keywords: beta-hydroxysteroid dehydrogenase, diabetes mellitus, dehydroepiandrosterone sulfate, testosterone, insulin
Citation: Hu Y, Wang Y, Cai T-t, Liu L, Li D-m, Ma J-h and Ding B (2022) Short-time intensive insulin therapy upregulates 3 beta- and 17 beta-hydroxysteroid dehydrogenase levels in men with newly diagnosed T2DM. Front. Endocrinol. 13:894743. doi: 10.3389/fendo.2022.894743
Received: 12 March 2022; Accepted: 29 June 2022;
Published: 19 July 2022.
Edited by:
Stefan Zoltán Lutz, Bad Sebastiansweiler, GermanyReviewed by:
Mohd Ashraf Ganie, Sher-I-Kashmir Institute of Medical Sciences, IndiaHong Sun, The First Affiliated Hospital of Soochow University, China
Bingyin Shi, The First Affiliated Hospital of Xi’an Jiaotong University, China
Liang Cheng, Huai’an Second People’s Hospital, China
Copyright © 2022 Hu, Wang, Cai, Liu, Li, Ma and Ding. This is an open-access article distributed under the terms of the Creative Commons Attribution License (CC BY). The use, distribution or reproduction in other forums is permitted, provided the original author(s) and the copyright owner(s) are credited and that the original publication in this journal is cited, in accordance with accepted academic practice. No use, distribution or reproduction is permitted which does not comply with these terms.
*Correspondence: Jian-hua Ma, majianhua196503@126.com; Bo Ding, dingbonanjing@sina.com
†These authors have contributed equally to this work