- 1Department of Clinical Sciences and Community Health, University of Milan, Milan, Italy
- 2University Sapienza of Rome, Rome, Italy
- 3Institute of Metabolism and Systems Research, University of Birmingham, Birmingham, United Kingdom
- 4Centre of Membrane Proteins and Receptors, Universities of Birmingham and Nottingham, Birmingham, United Kingdom
- 5Endocrinology Unit, Fondazione Istituto di Ricovero e Cura a Carattere Scientifico (IRCCS) Ca’ Granda Ospedale Maggiore Policlinico, Milan, Italy
The process of GPCR dimerization can have profound effects on GPCR activation, signaling, and intracellular trafficking. Somatostatin receptors (SSTs) are class A GPCRs abundantly expressed in pituitary tumors where they represent the main pharmacological targets of somatostatin analogs (SSAs), thanks to their antisecretory and antiproliferative actions. The cytoskeletal protein filamin A (FLNA) directly interacts with both somatostatin receptor type 2 (SST2) and 5 (SST5) and regulates their expression and signaling in pituitary tumoral cells. So far, the existence and physiological relevance of SSTs homo- and hetero-dimerization in the pituitary have not been explored. Moreover, whether octreotide or pasireotide may play modulatory effects and whether FLNA may participate to this level of receptor organization have remained elusive. Here, we used a proximity ligation assay (PLA)–based approach for the in situ visualization and quantification of SST2/SST5 dimerization in rat GH3 as well as in human melanoma cells either expressing (A7) or lacking (M2) FLNA. First, we observed the formation of endogenous SST5 homo-dimers in GH3, A7, and M2 cells. Using the PLA approach combined with epitope tagging, we detected homo-dimers of human SST2 in GH3, A7, and M2 cells transiently co-expressing HA- and SNAP-tagged SST2. SST2 and SST5 can also form endogenous hetero-dimers in these cells. Interestingly, FLNA absence reduced the basal number of hetero-dimers (-36.8 ± 6.3% reduction of PLA events in M2, P < 0.05 vs. A7), and octreotide but not pasireotide promoted hetero-dimerization in both A7 and M2 (+20.0 ± 11.8% and +44.1 ± 16.3% increase of PLA events in A7 and M2, respectively, P < 0.05 vs. basal). Finally, immunofluorescence data showed that SST2 and SST5 recruitment at the plasma membrane and internalization are similarly induced by octreotide and pasireotide in GH3 and A7 cells. On the contrary, in M2 cells, octreotide failed to internalize both receptors whereas pasireotide promoted robust receptor internalization at shorter times than in A7 cells. In conclusion, we demonstrated that in GH3 cells SST2 and SST5 can form both homo- and hetero-dimers and that FLNA plays a role in the formation of SST2/SST5 hetero-dimers. Moreover, we showed that FLNA regulates SST2 and SST5 intracellular trafficking induced by octreotide and pasireotide.
Introduction
G protein-coupled receptors (GPCRs) are the largest family of membrane receptors. Because of their heavy involvement in human physiology and disease, they are major drug targets (1, 2). The biochemical and pharmacological properties of GPCRs have been extensively characterized. One emerging aspect of GPCR signaling is their ability to form homo- and hetero-dimers, which has been implicated in the modulation of ligand binding affinity, signal transduction, and intracellular trafficking (3, 4). Whereas constitutive dimerization is essential for the correct functioning of class C GPCRs (5, 6), its consequences for the function of other GPCRs can vary considerably (7, 8). Of note, hetero-dimerization between two GPCRs of the same or different groups may endow the resulting hetero-dimer with unique signaling and pharmacological properties (9–15).
Somatostatin receptors (SSTs) comprise five family A GPCRs, named SST1–SST5, which are abundantly expressed in the endocrine system where they exert inhibitory actions on hormone secretion and cell proliferation (16–19). Of these, SST2 and SST5 are the prevalent subtypes in the pituitary (20, 21). Specifically, SST2 and SST5 are considered the main pharmacological targets of somatostatin analogs (SSAs) octreotide and pasireotide in the treatment of acromegaly caused by GH-secreting pituitary tumors (22). Octreotide displays a higher binding affinity for SST2 (IC50 = 0.38 nM), and lower for SST5 (6.3 nM), SST3 (7.1 nM), and SST1 (280 nM). On the contrary, pasireotide preferentially binds SST5 (0.16 nM) and shows lower affinity for SST2 (1 nM), SST3 (1.5 nM), and SST1 (9.3 nM) (23).
To date, whether SSTs are capable to form homo- and hetero-dimers in the pituitary as well as whether octreotide or pasireotide may play any modulatory effects on these events has not been investigated, yet. Indeed, the field of SST dimerization has been only partially explored in simple cell systems transfected with different SSTs from diverse species (12, 13, 24–27, revised in 28). Moreover, the exact mechanisms governing GPCR dimerization are, with a few exceptions, largely unknown.
An involvement of molecular chaperones such as 14-3-3, HSP70, or receptor activity-modifying proteins (RAMPs) has been proposed (29). Scaffolding proteins and cytoskeletal elements, which are already implicated in the formation of specialized signaling subdomains at the plasma membrane, receptor mobility, cluster assembly, and internalization, may also be involved (30, 31). Among these, the actin-binding protein filamin A (FLNA) might be a good candidate. Thanks to its flexible V shape, its actin-binding domain, and its scaffolding domains, FLNA is involved in crosslinking of actin filaments and anchoring transmembrane receptors to the subcortical cytoskeleton, thus providing a scaffold platform for receptor spatial organization and signaling (32). By means of single-molecule imaging and in situ proximity ligation assay (PLA), we have previously demonstrated that SST2 and SST5 directly interact with FLNA (33, 34). In pituitary tumoral cell lines, the association of FLNA with both SST2 and SST5 is crucial for an efficient SSA-induced signal transduction (34, 35). In addition, FLNA expression is required for SST2 internalization, recycling to the plasma membrane and protein stability after prolonged agonist stimulation (35), and to maintain a stable amount of SST5 in basal conditions by preventing both lysosomal and proteosomal degradation (34). However, whether FLNA may participate to SST dimeric assembly remains to be assessed.
In the present work, we used a PLA-based methodology for the in situ visualization and quantification of SST2/SST5 dimerization in rat GH-secreting pituitary cells (GH3). Moreover, in order to investigate the role of FLNA, we used the human melanoma cell models either expressing (A7) or lacking (M2) FLNA.
In situ PLA represents a powerful strategy to study receptor dimerization also in its natural context at physiological expression levels (36, 37). Moreover, we performed immunofluorescence experiments to demonstrate the specific FLNA-dependent modulation of SST2 and SST5 intracellular trafficking induced by octreotide and pasireotide.
Materials and methods
Cell culture
Rat pituitary tumoral GH-secreting cells (GH3 cells, ATCC CCL-82.1) were purchased from ATCC (Manassas, VA, USA) and cultured in F12K medium supplemented with 15% horse serum (HS), 2.5% fetal bovine serum (FBS), and antibiotics (Life Technologies, Carlsbad, CA, USA).
M2 is a spontaneously FLNA-deficient cell line, established from the tumor of a patient with malignant melanoma (38). A7 is a stably transfected cell line derived from the M2 cell line. A7 and M2 cells were kindly provided by Dr. Fumihiko Nakamura (School of Pharmaceutical Science and Technology, Tianjin University, Tianjin, China) and were grown in Eagle’s Minimum Essential Medium (EMEM) (ATCC, Manassas, VA, USA) supplemented with 8% Newborn Calf serum (NBCS), 2% fetal bovine serum (FBS), and antibiotics (Life Technologies, Carlsbad, CA, USA). For A7 cells, 200 µg/ml G418 was added (Merck KGaA, Darmstadt, DE). Cells were kept at 37°C in a humidified atmosphere with 5% CO2. Octreotide and pasireotide were provided by Novartis Pharma AG (Basel, CH) and used at 100-nM concentration.
Plasmid transfection
Expression vectors coding for human HA-SSTR2 (influenza hemagglutinin-tagged SSTR2) and SNAP-SSTR2 (SSTR2 fused to SNAP tag, a 20-kDa protein derived from the enzyme O6-alkylguanine-DNA alkyltransferase) were previously described (33). These vectors were transiently co-transfected in GH3 cells for 6 h in order to achieve low expression levels, resembling those of endogenous SSTR2. Lipofectamine 2000 was used as transfection reagent (Invitrogen, Thermo Fisher Scientific, Waltham, MA, USA) according to the manufacturer’s instruction.
In situ proximity ligation assay
GH3, A7, and M2 cells were seeded on 13-mm poly-L-lysine-coated coverslips at a density of 1.25 × 105 cells/well in 24-well plates and grown at 37°C for 18 h. The following day, cells were exposed or not with pasireotide 100 nM or octreotide 100 nM for 5 min. In case of SSTR2/SSTR2 homo-dimer evaluation, cells were transiently transfected with HA-tagged SSTR2 and SNAP-tagged SSTR2, as described above. Cells were fixed with 4% paraformaldehyde (Merck KGaA, Darmstadt, DE) for 10 min at room temperature, washed three times with PBS, and incubated for 1 h at room temperature with blocking buffer (5% FBS, 0.3% Triton™ X-100, in PBS). To test the presence of SST2/SST5 hetero-dimers, coverslips were incubated overnight at 4°C with primary rabbit anti-SST2 UMB1 #ab134152 (1:50, Abcam, Cambridge, UK) and primary mouse anti-SST5 #6675-1-Ig (1:200, Proteintech, Rosemont, IL, USA) antibodies. To test the presence of SST5/SST5 homo-dimers, two different primary antibodies against SST5 were used: a rabbit anti-SST5 #PA3-209 (Thermo Fisher Scientific, CA, USA) and a mouse anti-SST5 #6675-1-Ig (Proteintech, Rosemont, IL, USA), both diluted 1:200. To test the presence of SST2/SST2 homo-dimers, primary mouse anti-HA #26183 (1:250, Thermo Fisher Scientific, CA, USA) and primary rabbit anti-SNAP #CAB4255 (1:800, Thermo Fisher Scientific, CA, USA) antibodies were used. All antibodies were diluted in antibody Diluent Reagent Solution (Life Technologies, Thermo Fisher, CA). As negative controls to detect potential unspecific signal, one of the primary antibodies was removed. We used the Duolink In Situ PLA kit from Sigma-Aldrich (Merck KGaA, Darmstadt, DE). Briefly, Duolink Anti-Rabbit PLUS Probe (DUO92002, Sigma-Aldrich) and Duolink Anti-Mouse MINUS Probe (DUO92040, Sigma-Aldrich) were added and incubated for 1 h at 37°C. Then, Duolink In Situ Detection Reagents Green (Duolink, DUO92014) was used. Ligation-Ligase solution was added and incubated for 30 min at 37°C. Amplification-polymerase solution was subsequently added and incubated for 2 h (for SST2/SST2 homo-dimer detection) or 18 h (for SST2/SST5 hetero-dimer and SST5/SST5 homo-dimer detection) at 37°C. Coverslips were mounted on glass slides with EverBrite™ Hardset Mounting Medium with DAPI (Biotium, Fremont, CA, USA) for subsequent observation under epifluorescence microscope. Proximity ligation events were quantified with NIH ImageJ software after image deconvolution. The average number of PLA puncta per cell are related to images acquired from randomly chosen fields per condition from three independent experiments and quantified as previously described (34).
Immunofluorescence
GH3, A7, and M2 cells were seeded on 13-mm poly-L-lysine-coated coverslips at a density of 1.25 × 105 cells/well in 24-well plates and grown at 37°C for 18 h. The following day, cells were incubated with pasireotide 100 nM or octreotide 100 nM for 0, 5, or 30 min. For immunofluorescence analysis of SST2 and SST5 localization in GH3, A7, and M2 cells, rabbit anti-SST2 UMB1 #ab134152 (1:50, Abcam, Cambridge, UK) and mouse anti-SST5 #6675-1-Ig (1:200, Proteintech, Rosemont, IL, USA) antibodies were used and incubated o/n at 4°C. Anti-mouse Alexa Fluor™ 546-conjugated secondary antibody (1:500, Thermo Fisher Scientific, CA, USA) and anti-rabbit Alexa Fluor™ 488-conjugated secondary antibody (1:500, Thermo Fisher Scientific, CA, USA) were incubated at room temperature for 2 h. All antibodies were diluted in Antibody Diluent Reagent Solution (Life Technologies, Thermo Fisher, CA, USA). Coverslips were mounted on glass slides with EverBrite™ Hardset Mounting Medium with DAPI (Biotium, Fremont, CA, USA) for subsequent observation under epifluorescence microscope. The NIH ImageJ software was used to merge single-channel images before and after deconvolution.
Statistical analysis
The results are expressed as the mean ± SD. A paired two-tailed Student’s t-test was applied to assess the significance between two series of data. Statistical analysis was performed by GraphPad Prism 7.0 software, and P < 0.05 was accepted as statistically significant.
Results
Detection of SST2/SST2 and SST5/SST5 homodimers in somatotroph and melanoma cells
In order to examine the occurrence of SST2/SST2 homo-dimers in rat pituitary GH-secreting cells (GH3) and human melanoma cells A7 (FLNA-expressing cells) and M2 (FLNA-lacking cells), we combined the PLA approach with epitope tagging. Cells were transiently co-transfected with human HA-tagged SST2 and SNAP-tagged SST2, and antibodies against HA and SNAP tags were then used for PLA experiments. Indeed, in preliminary experiments, we did not find a pair of anti-SST2 antibodies raised in two different species, suitable for this application. To detect endogenous SST5/SST5 homo-dimers in GH3, A7, and M2 cells, two separate anti-SST5 antibodies raised in mouse and rabbit were used.
In GH3 cells, SST2/SST2 homo-dimers and SST5/SST5 homo-dimers were detected under basal conditions (Figure 1A). The presence of SST2/SST2 homo-dimers and SST5/SST5 homo-dimers were observed in both A7 and M2 cells, regardless of FLNA expression (Figures 1B, C). There were no SST2/SST2 or SST5/SST5 dimer signals in negative controls (Supplementary Figure 1).
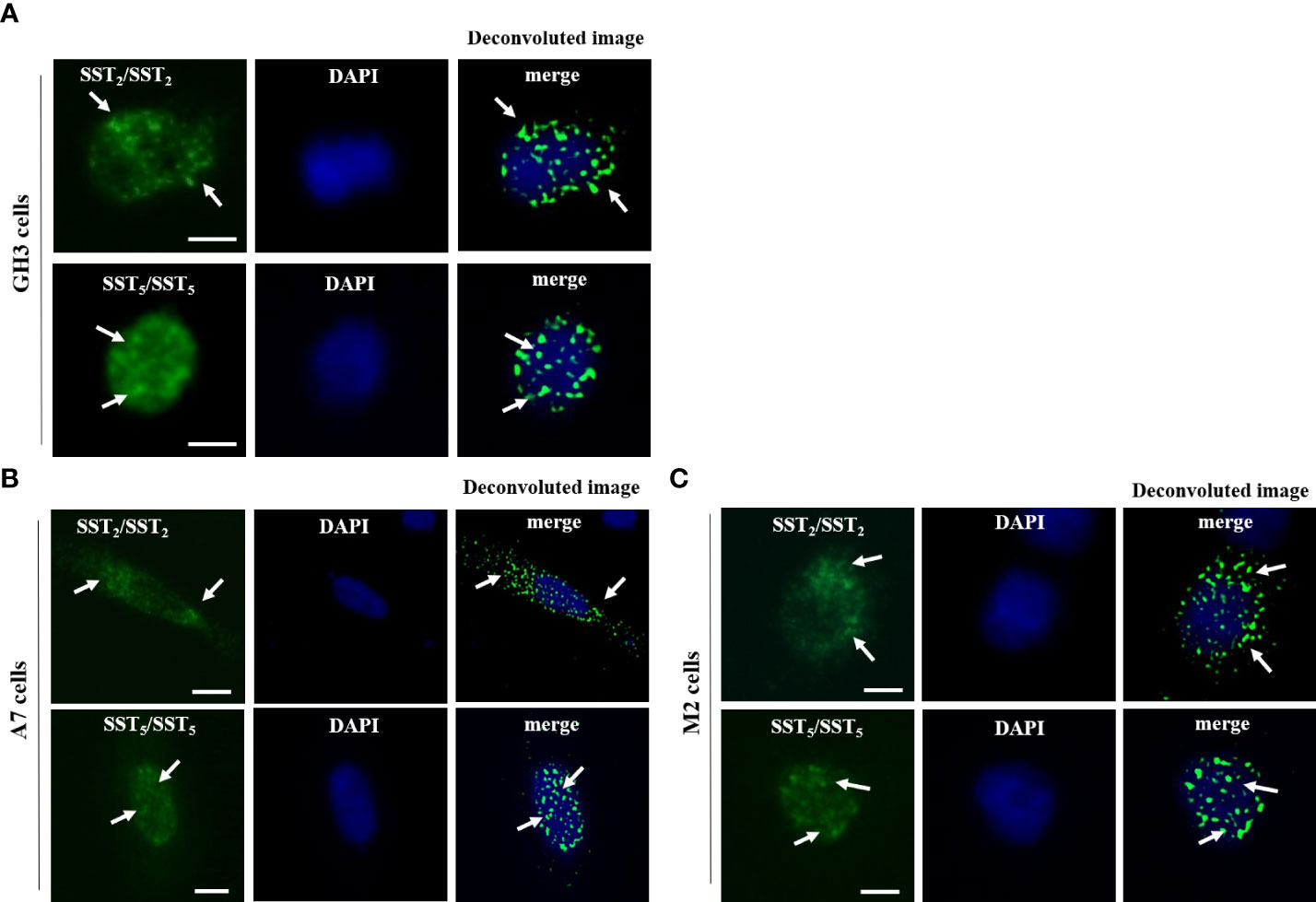
Figure 1 In situ detection of SST2/SST2 and SST5/SST5 homo-dimers. Representative in situ PLA experiment performed in GH3 (A), A7 cells (B), and M2 cells (C) showing SST2/SST2 (upper panels) and SST5/SST5 (lower panels) homo-dimers. For SST2/SST2 homo-dimers, mouse anti-HA and rabbit anti-SNAP antibodies were used. For SST5/SST5 homo-dimers, mouse and rabbit anti-SST5 antibodies were used. PLA puncta representing homo-dimers are shown as green dots and nuclei are stained with DAPI in blue. A deconvoluted image of PLA events merged with DAPI is shown. White arrows indicated the localization of PLA puncta. Scale bars: 10 μm.
Effect of SSAs and FLNA on SST2/SST5 hetero-dimerization in somatotroph and melanoma cells
Then, we investigated the presence of endogenous SST2/SST5 hetero-dimers in GH3 cells by means of PLA analysis and tested the possible effects exerted by octreotide and pasireotide on this receptor dimeric state. As shown in Figure 2A, SST2/SST5 hetero-dimers were detected under basal conditions and after treatment with 100 nM octreotide or pasireotide for 5 min. No effect on the number of PLA puncta corresponding to SST2/SST5 hetero-dimers was observed.
To decipher the role of FLNA in the formation of SST2/SST5 hetero-dimers and in the modulation of SSA-dependent effects on receptor assembly, PLA experiments were repeated in A7 and M2 cells. Our results showed that SST2 and SST5 were able to form hetero-dimers in A7 and M2 cells under basal physiological conditions. However, the absence of FLNA significantly impaired the amount of SST2/SST5 hetero-dimers (-36.8 ± 6.3% reduction of PLA events in M2 cells, P < 0.05 vs. A7 cells). In both cell lines, a significant increase in the PLA events was observed after 5 min of incubation with octreotide (+20.0 ± 11.8% increase of PLA events in A7 cells, P < 0.05 vs. basal, and +44.1 ± 16.3% increase of PLA events in M2 cells, P < 0.05 vs. basal) but not pasireotide (Figure 2B). No SST2/SST5 dimer signals were detected in negative controls (Supplementary Figure 2).
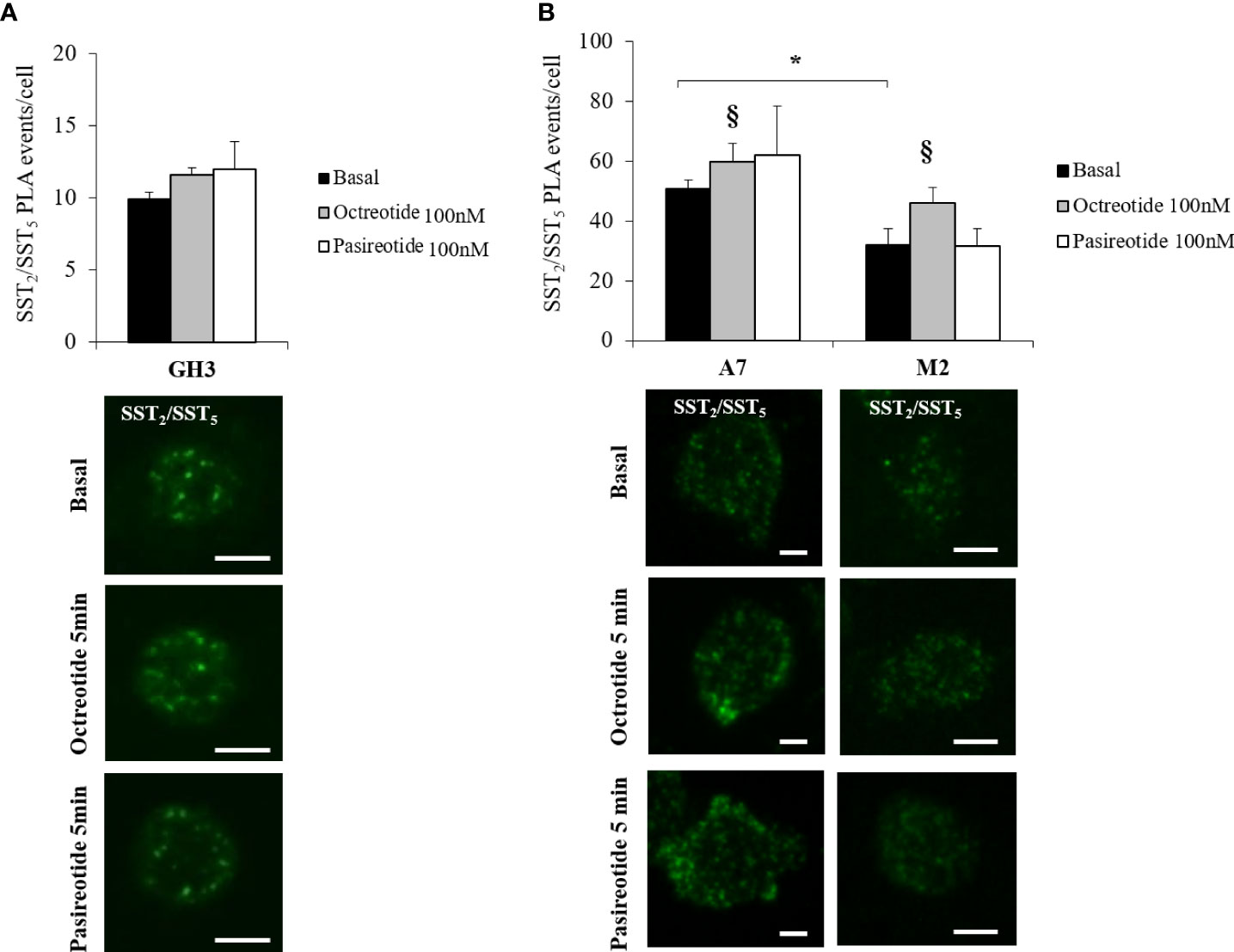
Figure 2 In situ detection of SST2/SST5 hetero-dimers. Representative in situ PLA experiment showing SST2/SST5 hetero-dimers in GH3 (A) and melanoma cells (B) before and after treatments with 100 nM octreotide or pasireotide for 5 min. Rabbit anti-SST2 and mouse-anti SST5 antibodies were used. Green dots represent PLA events and indicate close proximity between SST2 and SST5. Graphs resulting from the quantification of total SST2/SST5 puncta representing PLA events are shown for each cell line. For (B), a reduction in basal SST2/SST5 hetero-dimers in M2 cells compared to A7 cells and an increase in SST2/SST5 hetero-dimers in A7 and M2 cells treated with octreotide compared to basal are shown (n = 3, number of PLA puncta per cells was quantified for 150 cells randomly chosen from different fields per condition, *p < 0.05 vs. basal A7 cells; §p < 0.05 vs. corresponding basal). Scale bars: 10 μm.
FLNA-dependent modulation of SSA-induced intracellular trafficking of SST2 and SST5
Next, we used immunofluorescence to follow SSA-induced intracellular trafficking of endogenous SST2 and SST5 in GH3. Our imaging data showed that SST2 and SST5 colocalize throughout the cell body in the absence of stimuli. Cells’ exposure to octreotide or pasireotide for 5 min resulted in rapid receptor translocation to the plasma membrane. SST2 and SST5 were then similarly internalized by longer-time stimulation with octreotide and pasireotide, as shown by the intracellular colocalization signal (Figure 3A).
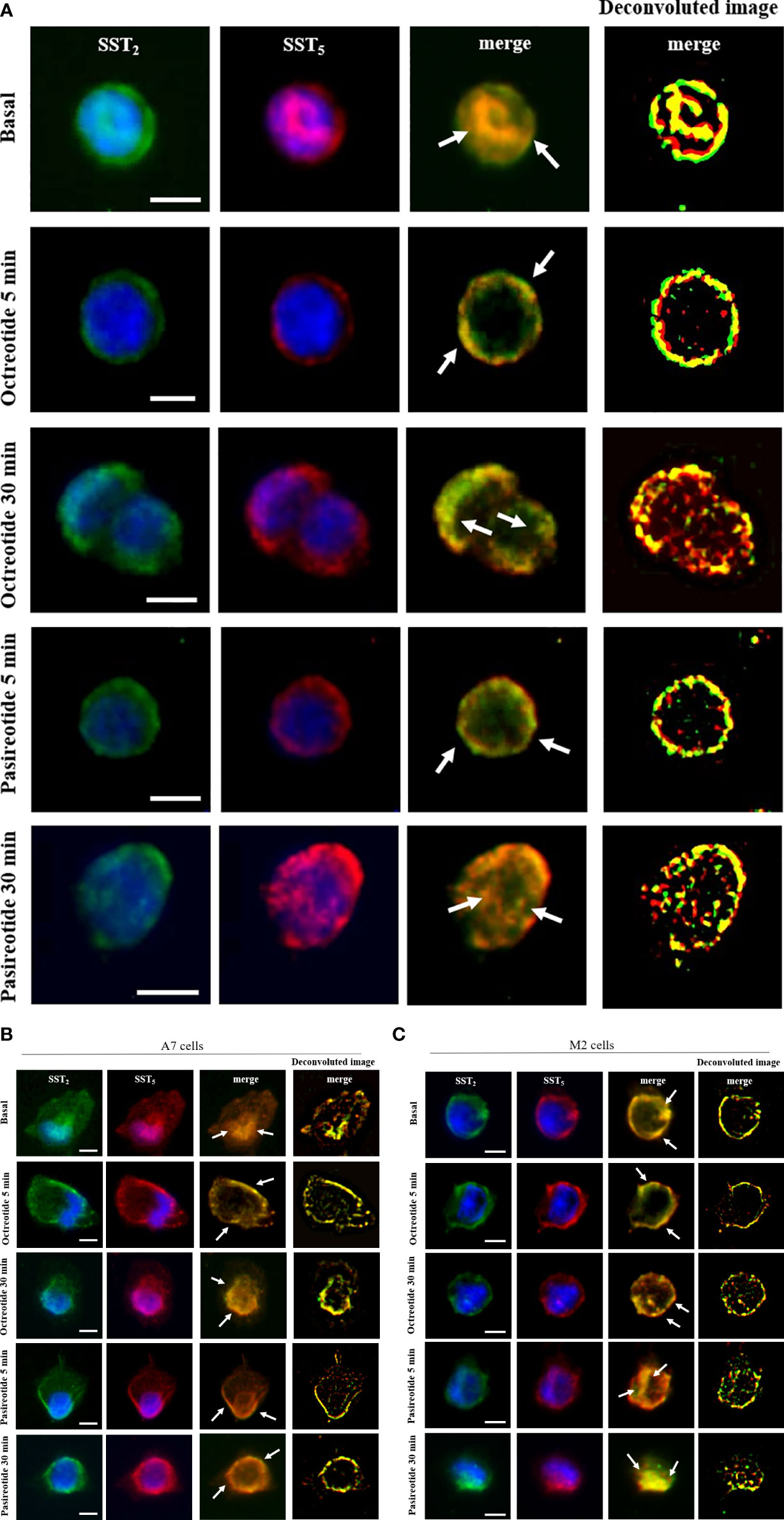
Figure 3 SST2 and SST5 intracellular trafficking. Representative immunofluorescence experiment showing subcellular localization of SST2 (green) and SST5 (red) in GH3 cells (A), A7 cells (B), and M2 cells (C) stimulated or not with 100 nM octreotide or pasireotide for the indicated times. Nuclei are stained with DAPI in blue. Overlay of green and red channels is shown, and white arrows indicate SST2 and SST5 subcellular colocalization. Deconvolution algorithm was applied to further show SST2 and SST5 colocalization areas (yellow signals, right columns). Scale bars: 10 μm.
To investigate the role of FLNA in the colocalization of human SST2 and SST5 during internalization experiments, A7 and M2 cells were used. Under basal conditions, SST2 and SST5 share the same localization at intracellular sites and on the plasma membrane in both cell lines. In A7 cells, stimulation with 100 nM octreotide and pasireotide induced a rapid cell membrane recruitment of both receptors (at 5 min) and receptor accumulation in a perinuclear region (at 30 min) (Figure 3B). In M2 cells, whereas a 5-min exposure to octreotide resulted in complete SST2 and SST5 translocation to the plasma membrane, similarly to what is observed in A7 cells, pasireotide also promoted a rapid internalization of a subset of both receptors. After 30 min of stimulation, only pasireotide led to SST2 and SST5 accumulation in a perinuclear region, while octreotide failed to internalize both receptors, as shown by the persistence of membrane signals (Figure 3C).
Discussion
SST2 and SST5 are the most abundantly expressed SSTs in the pituitary, where they mediate octreotide and pasireotide inhibitory effects on hormone secretion and cell proliferation, representing the main pharmacological targets for GH-secreting pituitary tumors in acromegaly (22). FLNA is known to directly bind SST2 and SST5 influencing their expression, signaling, and intracellular trafficking (34–36). To date, the co-expression of SST2 and SST5 has been reported in pituitary tumor cells (17, 39). However, the occurrence of SSTs homo- and hetero-dimerization and the modulation by SSAs as well as a possible involvement of FLNA in this process have been only postulated (40, 41). In the present study, we provided evidence of SST2 and SST5 homo and hetero-dimer assembly in pituitary somatotroph GH3 cells and melanoma cells and the impact of FLNA and octreotide, but not pasireotide, in the modulation of SST2/SST5 hetero-dimer formation. Moreover, we uncovered a novel role of FLNA in SSA-dependent SST2 and SST5 internalization.
First, by in situ PLA we detected the presence of homo-dimers of transiently transfected human SST2 in GH3 cells. This finding is consistent with previous immunoprecipitation and fluorescence resonance energy transfer (FRET) results on human, rat, and porcine SST2 in transfected in CHO and HEK293 cells, which revealed interspecies variations in the response to somatostatin (13, 24, 26). Here, we showed that SST2/SST2 homo-dimer assembly also occurs in melanoma cells. Moreover, our results suggest an involvement of FLNA-independent processes, since both A7 and M2 cell lines displayed PLA signals under basal conditions. As regards SST5, our PLA experiments showed that endogenous rat and human receptors assemble to form homo-dimers in GH3 and melanoma cells both under basal and stimulated conditions, respectively. This is in apparent contrast to previous studies which suggested that human SST5 does not dimerize after its synthesis but rather following somatostatin treatment (25). However, there is evidence that the stringent protein solubilization conditions used to study dimerization with co-immunoprecipitation may induce dimer dissociation, whereas agonist binding may stabilize receptor dimers (42). Since PLA does not require protein solubilization and has a higher sensitivity, it is plausible that our approach was able to reveal constitutive SST5 dimers that could not be detected in previous biochemical studies.
Since SST5/SST5 homo-dimers were present in both A7 and M2 cells, our results rule out a role of FLNA in SST5 homo-dimerization. Unfortunately, we could not perform a quantitative analysis of possible effects exerted by SSAs on SST2/SST2 and SST5/SST5 homo-dimers due to technical limitations. Specifically, in the case of SST2/SST2 homo-dimers, the difference in SST2 transfection efficiency among cells represented a bias, that, in turn, could have affected the PLA outcome; in the case of SST5/SST5 homo-dimers, the PLA coalescent signal (a consequence of the use of two different antibodies directed toward the same protein) could have rendered the puncta counting step unreliable.
Regarding GPCR hetero-dimerization, this process may be critical for the correct functionality of the GPCR, as is the case of the and the γ-aminobutyric acid (GABAB) receptor (5, 6), or, in some instances, may result in the alteration of the single receptor functioning (9–15). Here, the occurrence of endogenous SST2/SST5 hetero-dimers was reported for GH3 cells, although they remained insensitive to octreotide and pasireotide stimulation. In melanoma cells, SST2/SST5 hetero-dimers were also observed, but, interestingly, the absence of FLNA resulted in a significant reduction of their amount under basal conditions, indicating that FLNA is required but not essential for bringing SST2 and SST5 in close proximity to dimerize. Hetero-dimer formation was enhanced by octreotide but not pasireotide in both A7 and M2 cells, suggesting that activation of SST2 but not SST5 is an FLNA-independent driving factor for receptors to assemble. It has to be noticed that the effect of octreotide in M2 cells seemed even more pronounced. This observation raises the hypothesis of a less organized and more random occurrence of receptor interactions in the absence of FLNA. Indeed, in previous single-molecule studies, the disrupted FLNA–SST2 interaction resulted in upregulation of freely diffusing SST2 in CHO cells (33). However, in line with our finding of octreotide-promoting SST2/SST5 hetero-dimerization, co-immunoprecipitation and FRET data published by Grant and colleagues already documented an upregulation of human SST2/SST5 hetero-dimers in HEK293 cells following the selective activation of SST2 but not SST5 or their co-stimulation, with implications on receptor dynamics such as association of β-arrestin to SST2, receptor recycling, and signaling transduction efficiency (27).
An issue that should be considered is that the experiments testing SST2/SST2 homo-dimerization were performed by transient transfection of human SST2 in rat GH3 cells, since a pair of anti-SST2 antibodies raised in two different species, suitable for this application, and a human tumoral pituitary cell line were unavailable. Admittedly, a limitation of the present study is the lack of primary cell cultures from GH-secreting pituitary tumors.
Moreover, further studies analyzing a possible differential function of dimers compared to receptor monomers in terms of enhanced response to SSA treatment or activation of different intracellular pathways will open the way for the development of new therapeutic strategies for pituitary tumors based on drugs targeting the formation of SST dimers.
Finally, we studied SST2 and SST5 trafficking in order to highlight a potential role of FLNA in the modulation of ligand-mediated receptor internalization. We first observed a similar efficiency of octreotide and pasireotide in the recruitment of SST2 and SST5 at the plasma membrane and subsequent internalization in GH3 cells and A7 cells, supporting the idea of an efficient activation of these receptors exerted by both compounds in these specific cell models. These findings were only partially in line with data present in the literature reporting different effects on SST dynamics promoted by octreotide and pasireotide (43, 44). As already reported for SST2 in experiments of FLNA silencing in somatotroph cells (45), here we observed a lesser extent of octreotide-induced internalization not only of SST2 but also of SST5 in M2 cells compared to A7 cells. In addition, we documented a faster mobilization and internalization of both SST2 and SST5 in M2 cells compared to A7 cells promoted by pasireotide. These data pointed out a specific FLNA-dependent modulation of SST2 and SST5 intracellular trafficking induced by octreotide and pasireotide.
In conclusion, this work provides novel insights into the molecular mechanisms modulating SST assembly and functioning. Although the SST dimer interfaces remain to be established, such studies may open the way for the design of drugs targeting specific interactions between receptors of the SSTs family as well as interaction between scaffold proteins and SSTs.
Data availability statement
The original contributions presented in the study are included in the article/Supplementary Material. Further inquiries can be directed to the corresponding author.
Author contributions
Conceptualization: EP and GMan; investigation: DT, GMar, GeM, RC, FM, and EE; software: DT; writing—original draft preparation: DT; writing—review and editing: EP, DC, and GMan; supervision: MA; funding acquisition: EP, DC, and GMan. All authors contributed to the article and approved the submitted version.
Funding
This work was supported by the Italian Ministry of Health grant to GM (PE-2016-02361797), by an AIRC (Associazione Italiana Ricerca Cancro) grant to GM (IG 2017–20594), and by a Progetti di Ricerca di Interesse Nazionale (PRIN) grant to EP (2017N8CK4K). DC is a recipient of a Wellcome Trust Senior Research Fellowship (212313/Z/18/Z).
Conflict of interest
The authors declare that the research was conducted in the absence of any commercial or financial relationships that could be construed as a potential conflict of interest.
Publisher’s note
All claims expressed in this article are solely those of the authors and do not necessarily represent those of their affiliated organizations, or those of the publisher, the editors and the reviewers. Any product that may be evaluated in this article, or claim that may be made by its manufacturer, is not guaranteed or endorsed by the publisher.
Supplementary material
The Supplementary Material for this article can be found online at: https://www.frontiersin.org/articles/10.3389/fendo.2022.892668/full#supplementary-material
References
1. Ferré S, Casadó V, Devi LA, Filizola M, Jockers R, Lohse MJ, et al. G Protein–coupled receptor oligomerization revisited: functional and pharmacological perspectives. Pharmacol Rev (2014) 66:413–34. doi: 10.1124/pr.113.008052
2. Hauser AS, Attwood MM, Rask-Andersen M, Schiöth HB, Gloriam DE. Trends in GPCR drug discovery: new agents, targets and indications. Nat Rev Drug Discov (2017) 16(12):829–42. doi: 10.1038/nrd.2017.178
3. Terrillon S, Bouvier M. Roles of G-protein-coupled receptor dimerization. EMBO Rep (2004) 5:30–4. doi: 10.1038/sj.embor.7400052
4. Lohse MJ. Dimerization in GPCR mobility and signaling. Curr Opin Pharmacol (2010) 10:53–8. doi: 10.1016/j.coph.2009.10.007
5. Jones KA, Borowsky B, Tamm JA, Craig DA, Durkin MM, Dai M, et al. GABAB receptors function as a heteromeric assembly of the subunits GABABR1 and GABABR2. Nature (1998) 396:674–9. doi: 10.1038/25348
6. White JH, Wisw A, Main MJ, Green A, Fraser NJ, Disney GH, et al. Heterodimerization is required for the formation of a functional GABAB receptor. Nature (1998) 396:679–82. doi: 10.1038/25354
7. Milligan G. G Protein-coupled receptor dimerization: function and ligand pharmacology. Mol Pharmacol (2004) 66:1–7. doi: 10.1124/mol.104.000497
8. Pfleger KDG, Eidne KA. Monitoring the formation of dynamic gprotein-coupled receptor-protein complexes in living cells. Biochem J (2005) 385:625–37. doi: 10.1042/BJ20041361
9. AbdAlla S, Lother H, Quitterer U. AT1-receptor heterodimers show enhanced G-protein activation and altered receptor sequestration. Nature (2000) 407:94–8. doi: 10.1038/35024095
10. George SR, Fan T, Xie Z, Tse R, Tam V, Varghese G, et al. Oligomerization of mu- and delta-opioid receptors. generation of novel functional properties. J Biol Chem (2000) 275:26128–35. doi: 10.1074/jbc.M000345200
11. Rocheville M, Lange DC, Kumar U, Patel SC, Patel RC, Patel YC. Receptors for dopamine and somatostatin: formation of heterooligomers with enhanced functional activity. Science (2000) 288:154–7. doi: 10.1126/science.288.5463.154
12. Rocheville M, Lange DC, Kumar U, Sasi R, Patel RC, Patel YC. Subtypes of the somatostatin receptor assemble as functional homo- and heterodimers. J Biol Chem (2000) 275:7862–9. doi: 10.1074/jbc.275.11.7862
13. Pfeiffer M, Koch T, Schroder H, Klutzny M, Kirscht S, Kreienkamp HJ, et al. Homo- and heterodimerization of somatostatin receptor subtypes. inactivation of sst(3) receptor function by heterodimerization with sst(2A). J Biol Chem (2001) 276:14027–36. doi: 10.1074/jbc.M006084200
14. Yoshioka K, Saitoh O, Nakata H. Heteromeric association creates a P2Y-like adenosine receptor. Proc Nat Acad Sci USA (2001) 98:7617–22. doi: 10.1073/pnas.121587098
15. Pfeiffer M, Koch T, Schroder H, Laugsch M, Hollt V, Schulz S. Heterodimerization of somatostatin and opioid receptors cross-modulates phosphorylation, internalization, and desensitization. J Biol Chem (2002) 277:19762–72. doi: 10.1074/jbc.M110373200
16. Patel YC. Somatostatin and its receptor family. Front Neuroendocrinol (1999) 20:157–98. doi: 10.1006/frne.1999.0183
17. Moller LN, Stidsen CE, Hartmann B, Holst JJ. Somatostatin receptors. Biochim Biophys (2003) 1616:1–84. doi: 10.1016/s0005-2736(03)00235-9
18. Schonbrunn A. Selective agonism in somatostatin receptor signaling and regulation. Mol Cell Endocrinol (2008) 286:35–9. doi: 10.1016/j.mce.2007.09.009
19. Siehler S, Nunn C, Hannon JP, Feuerbach D, Hoyer D. Pharmacological profile of somatostatin and cortistatin receptors. Mol Cell Endocrinol (2008) 286:26–34. doi: 10.1016/j.mce.2007.12.007
20. Fischer T, Doll C, Jacobs S, Kolodziej A, Stumm R, Schulz S. Reassessment of sst2 somatostatin receptor expression in human normal and neoplastic tissues using the novel rabbit monoclonal antibody UMB-1. J Clin Endocrinol Metab (2008) 93:4519–24. doi: 10.1210/jc.2008-1063
21. Lupp A, Hunder A, Petrich A, Nagel F, Doll C, Schulz S. Reassessment of sst(5) somatostatin receptor expression in normal and neoplastic human tissues using the novel rabbit monoclonal antibody UMB-4. Neuroendocrinology (2011) 94:255–64. doi: 10.1159/000329876
22. Ben-Shlomo A, Melmed S. Pituitary somatostatin receptor signaling. Trends Endocrinol Metab (2010) 21:123–33. doi: 10.1016/j.tem.2009.12.003
23. Bruns C, Lewis I, Briner U, Meno-Tetang G, Weckbecker G. SOM230: a novel somatostatin peptidomimetic with broad somatotropin release inhibiting factor (SRIF) receptor binding and a unique antisecretory profile. Eur J Endocrinol (2002) 146(5):707–16. doi: 10.1530/eje.0.1460707
24. Grant M, Collier B, Kumar U. Agonist-dependent dissociation of human somatostatin receptor 2 dimers: a role in receptor trafficking. J Biol Chem (2004) 279:36179–83. doi: 10.1074/jbc.M407310200
25. Grant M, Patel RC, Kumar U. The role of subtype-specific ligand binding and the c-tail domain in dimer formation of human somatostatin receptors. J Biol Chem (2004) 279:38636–43. doi: 10.1074/jbc.M406276200
26. Duran-Prado M, Bucharles C, Gonzalez BJ, Vazquez-Martinez R, Martinez-Fuentes AJ, Garcia-Navarro S, et al. Porcine somatostatin receptor 2 displays typical pharmacological sst2 features but unique dynamics of homodimerization and internalization. Endocrinology (2007) 148:411–21. doi: 10.1210/en.2006-0920
27. Grant M, Alturaihi H, Jaquet P, Collier B, Kumar U. Cell growth inhibition and functioning of human somatostatin receptor type 2 are modulated by receptor heterodimerization. Mol Endocrinol (2008) 22:2278–92. doi: 10.1210/me.2007-0334
28. Durán-Prado M, Malagón MM, Gracia-Navarro F, Castaño JP. Dimerization of G protein-coupled receptors: new avenues for somatostatin receptor signalling, control and functioning. Mol Cell Endocrinol (2008) 286(1-2):63–8. doi: 10.1016/j.mce.2007.12.006
29. Bulenger S, Marullo S, Bouvier M. Emerging role of homo- and heterodimerization in G-protein-coupled receptor biosynthesis and maturation. Trends Pharmacol Sci (2005) 26:131–37. doi: 10.1016/j.tips.2005.01.004
30. Hall RA, Lefkowitz RJ. Regulation of G protein-coupled receptor signaling by scaffold proteins. Circ Res (2002) 91:672–80. doi: 10.1161/01.res.0000037000.74258.03
31. Fehon RG, McClatchey AI, Bretscher A. Organizing the cell cortex: the role of ERM proteins. Nat Rev Mol Cell Biol (2010) 11:276–87. doi: 10.1038/nrm2866
32. Nakamura F, Stossel TP, Hartwig JH. The filamins: organizers of cell structure and function. Cell Adh Migr (2011) 2:160–9. doi: 10.4161/cam.5.2.14401
33. Treppiedi D, Jobin ML, Peverelli E, Giardino E, Sungkaworn T, Zabel U, et al. Single-molecule microscopy reveals dynamic FLNA interactions governing SSTR2 clustering and internalization. Endocrinology (2018) 159:2953–65. doi: 10.1210/en.2018-00368
34. Treppiedi D, Di Muro G, Mangili F, Catalano R, Giardino E, Barbieri AM, et al. Filamin a is required for somatostatin receptor type 5 expression and pasireotide-mediated signaling in pituitary corticotroph tumor cells. Mol Cell Endocrinol (2021) 524:111159. doi: 10.1016/j.mce.2021.111159
35. Peverelli E, Giardino E, Treppiedi D, Vitali E, Cambiaghi V, Locatelli M, et al. (FLNA) plays an essential role in somatostatin receptor 2 (SST2) signaling and stabilization after agonist stimulation in human and rat somatotroph tumor cells. Endocrinology (2014) 155:2932–41. doi: 10.1210/en.2014-1063
36. Gajadhar A, Guha A. A proximity ligation assay using transiently transfected, epitope-tagged proteins: application for in situ detection of dimerized receptor tyrosine kinases. Biotechniques (2010) 48:145–52. doi: 10.2144/000113354
37. Gomes I, Sierra S, Devi LA. Detection of receptor heteromerization using in situ proximity ligation assay. Curr Protoc Pharmacol (2016) 75:2.16.1–2.16.31. doi: 10.1002/cpph.15
38. Cunningham CC, Gorlin JB, Kwiatkowski DJ, Hartwig JH, Janmey PA, Byers HR, et al. Actin-binding protein requirement for cortical stability and efficient locomotion. Science (1992) 255(5042):325–7. doi: 10.1126/science.1549777
39. Olias G, Viollet C, Kusserow H, Epelbaum J, Meyerhof W. Regulation and function of somatostatin receptors. J Neurochem (2004) 89:1057– 91. doi: 10.1111/j.1471-4159.2004.02402.x
40. Ren SG, Taylor J, Dong J, Yu R, Culler MD, Melmed S. Functional association of somatostatin receptor subtypes 2 and 5 in inhibiting human growth hormone secretion. J Clin Endocrinol Metab (2003) 88:4239–45. doi: 10.1210/jc.2003-030303
41. Ben-Shlomo A, Wawrowsky KA, Proekt I, Wolkenfeld NM, Ren SG, Taylor J, et al. Somatostatin receptor type 5 modulates somatostatin receptor type 2 regulation of adrenocorticotropin secretion. J Biol Chem (2005) 280:24011–21. doi: 10.1074/jbc.M501998200
42. Vilardaga JP, Bünemann M, Krasel C, Castro M, Lohse MJ. Measurement of the millisecond activation switch of G protein-coupled receptors in living cells. Nat Biotechnol (2003) 21:807–12. doi: 10.1038/nbt838
43. Poll F, Lehmann D, Illing S, Ginj M, Jacobs S, Lupp A, et al. Pasireotide and octreotide stimulate distinct patterns of sst2A somatostatin receptor phosphorylation. Mol Endocrinol (2010) 24:436–46. doi: 10.1210/me.2009-0315
44. Amarù J, Barbieri F, Arvigo M, Solari A, Bajetto A, Nista F, et al. Octreotide and pasireotide combination treatment in somatotroph tumor cells: predominant role of SST2 in mediating ligand effects. Cancers (Basel) (2021) 8:1816. doi: 10.3390/cancers13081816
45. Treppiedi D, Mangili F, Giardino E, Catalano R, Locatelli M, Lania AG, et al. Cytoskeleton protein filamin a is required for efficient somatostatin receptor type 2 internalization and recycling through Rab5 and Rab4 sorting endosomes in tumor somatotroph cells. Neuroendocrinology (2020) 110:642–52. doi: 10.1159/000503791
Keywords: GPCR dimerization, in situ PLA, somatostatin analogs, FLNA, SST2, SST5
Citation: Treppiedi D, Marra G, Di Muro G, Catalano R, Mangili F, Esposito E, Calebiro D, Arosio M, Peverelli E and Mantovani G (2022) Dimerization of GPCRs: Novel insight into the role of FLNA and SSAs regulating SST2 and SST5 homo- and hetero-dimer formation. Front. Endocrinol. 13:892668. doi: 10.3389/fendo.2022.892668
Received: 17 March 2022; Accepted: 08 July 2022;
Published: 05 August 2022.
Edited by:
Federico Gatto, San Martino Hospital (IRCCS), ItalyReviewed by:
Marica Arvigo, University of Genoa, ItalyJusto P. Castaño, Maimonides Biomedical Research Institute of Cordoba (IMIBIC), Spain
Copyright © 2022 Treppiedi, Marra, Di Muro, Catalano, Mangili, Esposito, Calebiro, Arosio, Peverelli and Mantovani. This is an open-access article distributed under the terms of the Creative Commons Attribution License (CC BY). The use, distribution or reproduction in other forums is permitted, provided the original author(s) and the copyright owner(s) are credited and that the original publication in this journal is cited, in accordance with accepted academic practice. No use, distribution or reproduction is permitted which does not comply with these terms.
*Correspondence: Erika Peverelli, ZXJpa2EucGV2ZXJlbGxpQHVuaW1pLml0