Commentary: Fish and the thyroid: A Janus Bifrons relationship caused by pollutants and the omega-3 polyunsaturated fatty acids
- 1Department of Clinical and Experimental Medicine, University of Messina, Messina, Italy
- 2Department of Human Pathology in Adulthood and Childhood “G. Barresi”, University of Messina, Messina, Italy
- 3Department of Surgical, Medical, Molecular and Critical Area Pathology, University of Pisa, Pisa, Italy
- 4Division of Endocrinology, Dr. Cesar Milstein Hospital, Buenos Aires, Argentina
Benefits of the omega-3 polyunsaturated fatty acids (PUFA) on a number of clinical disorders, including autoimmune diseases, are widely reported in the literature. One major dietary source of PUFA are fish, particularly the small oily fish, like anchovy, sardine, mackerel and others. Unfortunately, fish (particularly the large, top-predator fish like swordfish) are also a source of pollutants, including the heavy metals. One relevant heavy metal is mercury, a known environmental trigger of autoimmunity that is measurable inside the thyroid. There are a number of interactions between the omega-3 PUFA and thyroid hormones, even at the level of the thyroid hormone transport proteins. Concerning the mechanisms behind the protection from/amelioration of autoimmune diseases, including thyroiditis, that are caused by the omega-3 PUFA, one can be the decreased production of chemokines, a decrease that was reported in the literature for other nutraceuticals. Recent studies point also to the involvement of resolvins. The intracellular increase in resolvins is associated with the tissue protection from inflammation that was observed in experimental animals after coadministration of omega-3 PUFA and thyroid hormone. After having presented data on fish consumption at the beginning, we conclude our review by presenting data on the market of the dietary supplements/nutraceuticals. The global omega-3 products market was valued at USD 2.10 billion in 2020, and was projected to go up at a compound annual growth rate of 7.8% from 2020 to 2028. Among supplements, fish oils, which are derived mainly from anchovies, are considered the best and generally safest source of omega-3. Taking into account (i) the anti-autoimmunity and anti-cancer properties of the omega-3 PUFA, (ii) the increasing incidence of both autoimmune thyroiditis and thyroid cancer worldwide, (iii) the predisposing role for thyroid cancer exerted by autoimmune thyroiditis, and (iv) the risk for developing metabolic and cardiovascular disorders conferred by both elevated/trendwise elevated serum TSH levels and thyroid autoimmunity, then there is enough rationale for the omega-3 PUFA as measures to contrast the appearance and/or duration of Hashimoto’s thyroiditis as well as to correct the slightly elevated serum TSH levels of subclinical hypothyroidism.
Introduction
After having reminded the benefits of the omega-3 polyunsaturated fatty acids (PUFA) on several autoimmune diseases, we aim to review their benefits in the thyroid setting both at experimental and clinical levels. We start our work by providing a snapshot of fish consumption since fishes represent a major dietary source of omega-3 PUFA. The other side of the coin (or the other face of Janus, upon comparing fish to this god of the Ancient Roman mythology) is that eating fish means also “eating pollutants”. One relevant pollutant is mercury (Hg), a trigger of autoimmunity that is measurable inside the human thyroid. However, in fish the two faces have unequal size. Indeed, in small oily fish, such as anchovy and sardine, the good face represented by the omega-3 PUFA is much larger than the bad face represented by the pollutants. Just the opposite is true for the large, top predator fish, such as swordfish and tuna. Thus, we also provide a review of studies showing thyroid consequences from eating fish. Finally, after having reminded the interactions between the omega-3 PUFA and thyroid hormones at several levels, and having reminded that other natural compounds have shown benefits in the setting of thyroid autoimmunity, we talk about the commercial side of the benefits of all such substances, namely the expanding market of nutraceuticals. Overall, we think that the use of supplements containing omega-3 in the clinical thyroid setting has enough scientific rationale.
Consumption of fish
The European Market Observatory for Fisheries and Aquaculture Products (EUMOFA) considers fish and seafood as “the aggregation of finfish, crustaceans and mollusks and cephalopods”, regardless of being fresh or chilled and frozen (1). Examples of crustaceans are lobsters, shrimps and crabs; examples of mollusks are oysters and clams; examples of cephalopods are octopus, squid, and cuttlefish (1). Household expenditure on fishery and aquaculture products grew by 17% from 2019 to 2020, much greater than the 2.1% inflation of prices for such products (1). This increase was most likely due to the increased at-home consumption due to the closing of the hotels and restaurants because of the COVID-19 pandemic (1). In 2017, the expenditure on fish in the European Union (EU) was equal to euro 54262 million, which was the highest in the world. However, in terms of per capita expenditure, with euro 106 EU ranked 8th after Iceland, Japan, Korea, Norway, Australia, Israel, and Switzerland (1). Excluding out-of-home consumption, household nominal expenditure on fishery and aquaculture products in 2020 (with % variation over 2019 given in parentheses) was led by Spain (euro 13608 million, +39%) and Italy (euro 12277 million, +3%), with Slovenia (euro 90 million, +1%) and Malta (euro 58 million, +4%) at the bottom of the ranking.
Consolidated data for consumption and other items are available up to 2019 (1). Consumption of fishery and aquaculture products in the EU dropped to 12.30 million tonnes of live weight equivalent (LWE) in 2019, continuing a declining trend that started in 2017. Wild products accounted for 9.41 million tonnes LWE (76% of 12.30), and farm products for 2.89 million tonnes LWE (the remaining 24%). Per capita apparent consumption, estimated at 23.97 kg LWE of mostly wild-caught products, was almost stable in 2019 compared with 2018. Portugal remains the major EU consumer, with 59.91 Kg per capita (-2% vs 2018). Portugal is followed by Spain (46.02 Kg, unchanged), Denmark (42.56 Kg, +6%), France (33.26 Kg, -0.5%), Luxembourg (32.84 Kg, -3%), and Italy (31.21 Kg, +1%). At the other extreme, Hungary and the Czech Republic consume 6.28 and 6.0 Kg (+3% and +7% vs 2018, respectively) (1).
Concerning the most consumed fish, 16 products were considered, and they were listed in decreasing order of consumption: tuna, salmon, cod, Alaska pollock, shrimp, mussel, hake, herring, squid, surimi, sardine mackerel, trout, sprat (brisling), saithe (coalfish) and other products. Illustrative per capita consumption in 2019 (with percentage change vs 2018 given in parentheses) were 3.10 Kg (+ 2%) for tuna, 2.11 Kg (-1%) for cod, 1.02 Kg (+ 2%) for hake, 0.98 Kg (-17%) for herring, 0.58 Kg (+1%) for sardine, 0.53 Kg (-12%) for mackerel, and 6.58 Kg (-2%) for other products (1). In 2020, over 80% of the total volume of fresh fishery and aquaculture products consumed by households in 11 EU countries analyzed was accounted for by Spain, Italy, and France. In decreasing order, the top five fresh fish species consumed by households in 2020 were: hake, salmon, sardine, European seabass, and gilthead seabream in Spain; gilthead seabream, mussels, salmon, European seabass, and anchovy in Italy; salmon, cod, saithe (coalfish), trout and gilthead seabream in France. Data for swordfish, a seafood species that will be mentioned subsequently in our review, were not provided.
Based on a document by CBI (Centre for the Promotion of Imports from developing countries) the swordfish fishery is very important for Southern Europe, especially Spain and Italy, the two European countries with the highest consumption of swordfish (2). With 22,676 tonnes in 2017, Spain is the leading European producer of swordfish, followed by Italy and Portugal. The top importers from non-European countries of frozen swordfish are Portugal, Italy and Spain (6,316, 3,762 and 2,130 tonnes, respectively). Italy’s imports of swordfish have increased by 18% since 2014 with most deliveries coming from Spain. Based on a document by Oceana (3), which is the no-profit largest international ocean conservation organization, Greece, Italy, and Spain are the European countries where swordfish is consumed the most, but numbers were not provided. According to a Spanish paper (4), which in turn reports data published in 1995, the consumption of swordfish by Spanish adults averaged 0.35 g/day, with marked differences between communities, ranging from areas of no swordfish consumption to two areas of highest consumption (1.06 g/day and 1.17 g/day). On an annual basis, the said 0.35, 1.06 and 1.17 g/day correspond to 0.13, 0.39 and 0.40 Kg/year. In Italy, swordfish accounts for 4.9% of the national consumption of fresh fish (5), and in the year 2017 it was the fifth species most consumed (5.7%), following European seabass (17%) (6). Thus, of the 29.80 Kg of fish consumed by Italians in 2017, 1.46 Kg were accounted for by swordfish. A recent Italian survey of 560 consumers (7), led to the identification of 24 seafood species that were commonly purchased. The highest preference was for gilthead bream (Sparus auratus), European seabass (Dicentrarchus labrax), swordfish (Xiphias gladius), and European pilchard (Sardina pilchardus), with approximately 13% of consumers who purchased swordfish.
The Good Face of Janus
There is abundant literature, which is accruing over the years, about the benefits of the omega-3 fatty acids as such or omega-3-rich-foods on some disorders (Table 1), including autoimmune diseases (8–18). An example of the clinical studies that show such benefits for illustrative autoimmune diseases is summarized in Table 2 (19–36), with more details provided for type-1 diabetes (T1D) due to the endocrine nature of this disorder. Table 1 also illustrates the magnitude of the literature on other topics of this review, such as fish consumption.
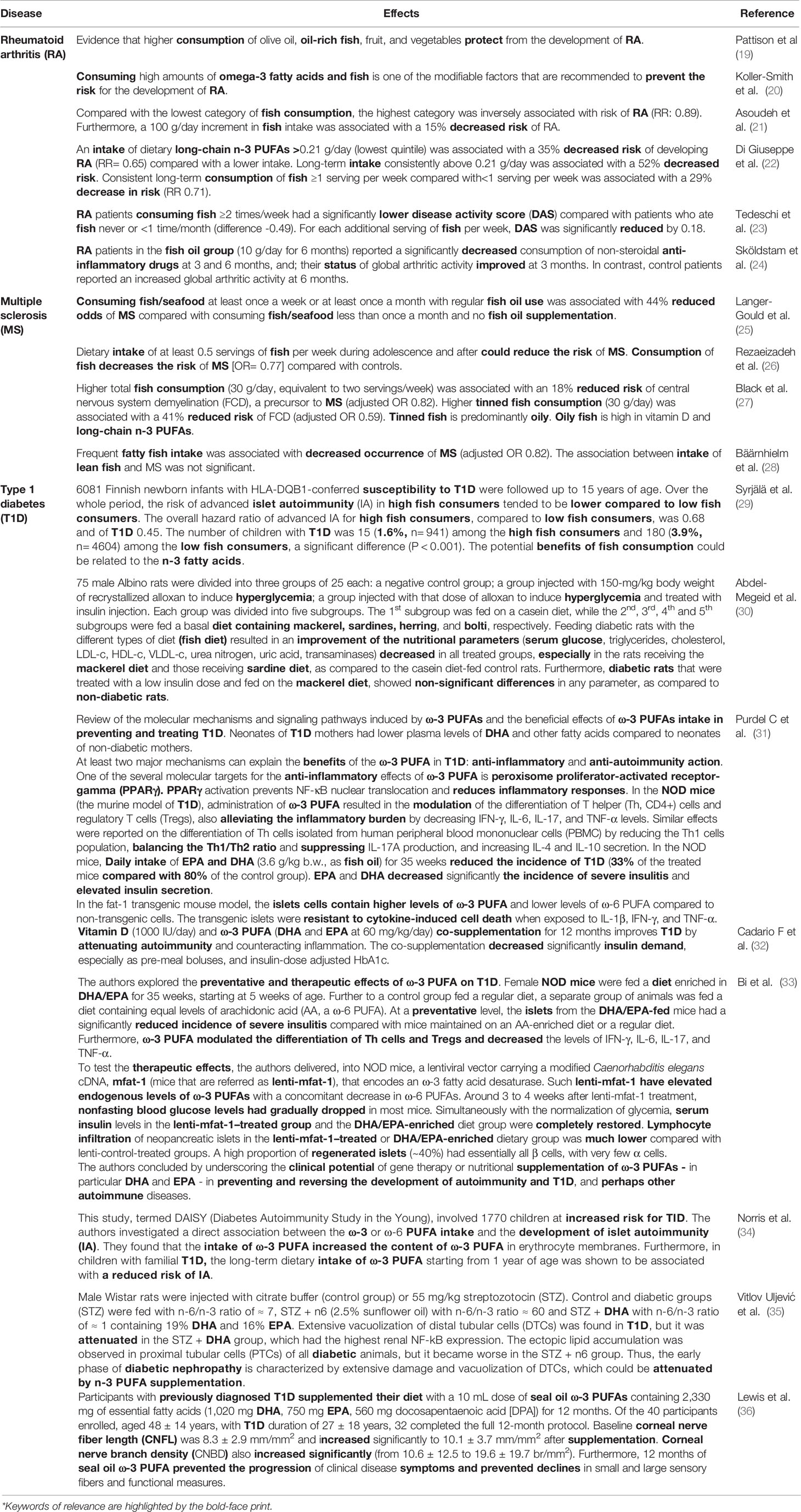
Table 2 Example literature on the effects of fish/fish oil consumption or supplements containing omega-3 polyunsaturated fatty acids (PUFA) on representative autoimmune diseases. *
As well known, fish (particularly seafood) is a good source of high-quality proteins, vitamins, and minerals, including minerals relevant to thyroid physiology (iodine and selenium). As also well known, fish is a major source of the long-chain omega-3 polyunsaturated fatty acids (abbreviated as either LC n-3 PUFA or omega-3 PUFA), particularly docosahexaenoic acid (DHA) and eicosapentaenoic acid (EPA). Figure 1 illustrates the content of EPA and DHA in representative seafood (17) while Figure 2 illustrates the oily fish (with their scientific, English and Italian names), which will be referred to in some sections of this review. Italian names are reported because in Italy we refer to the oily fish as “pesce azzurro” (“azure fish”) due to the blue-green color of the green in the dorsal and later scales (37). In the absence of a scientific definition, their definition is culinary (37). In contrast, the n-6 (omega-6 PUFA), such as arachidonic acid and linoleic acid, are derived largely from plant sources. Plants (and therefore plant oils and seeds) are also a source of another LC n-3 PUFA: α-linolenic acid (ALA). Tissues can convert ALA into EPA and DHA, but because the corresponding conversions are only 1 to 10% and 0.5-5% in human tissues, this conversion process is inefficient in humans (38). Thus, the main dietary source of EPA and DHA is cold-water oily fish such as mackerel, salmon, herring, sardines, anchovies, etc…. Canned fish contains omega-3 PUFA, but some amounts may be removed during processing (39). A similar decline in content of the omega-3 PUFA occurs with frying (39, 40).
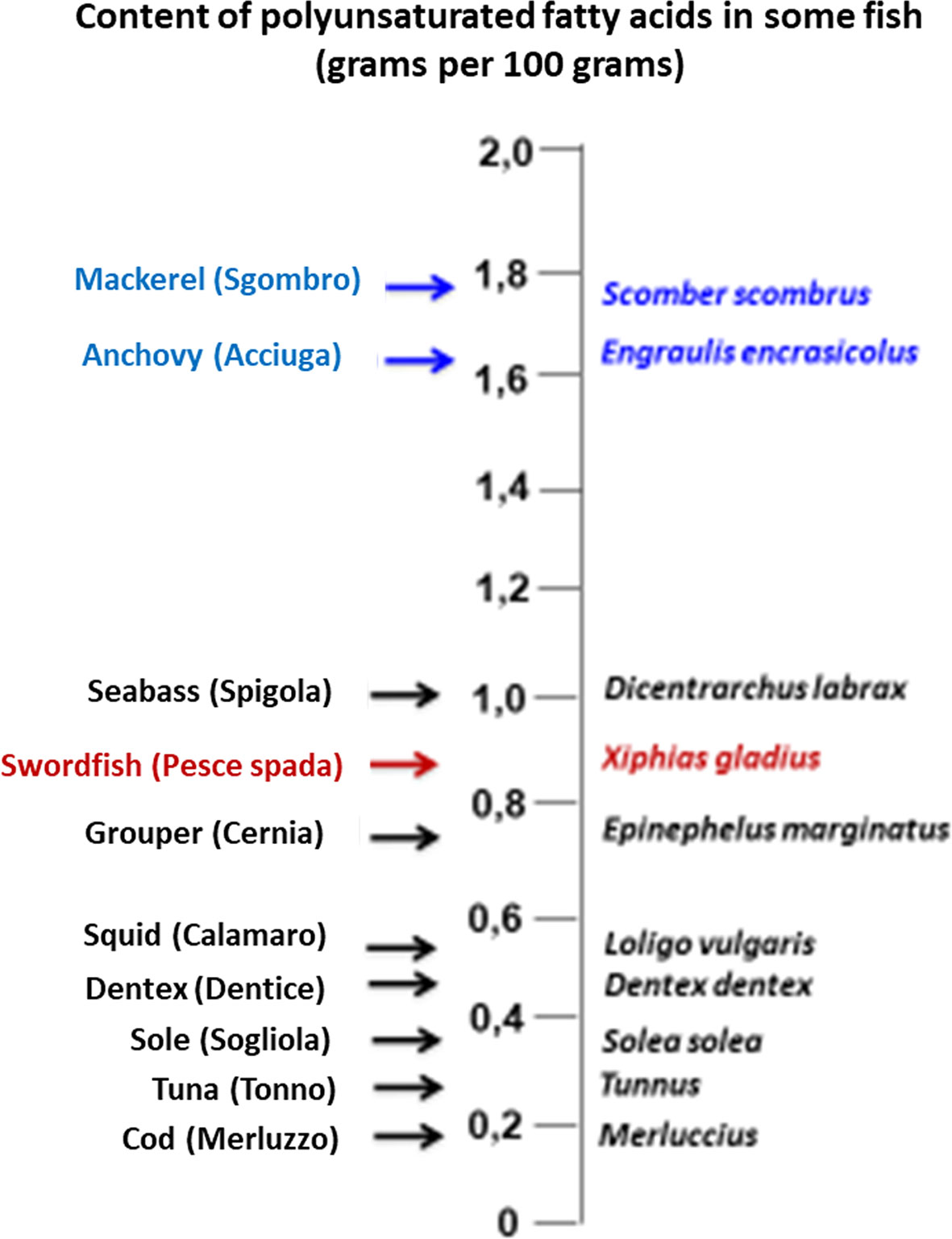
Figure 1 Content of polyunsaturated fatty acids in illustrative species of fish. Oily fish (which are referred to as “pesce azzurro” [“azure fish”] in Italy) are typed in blue, while swordfish (the top predator fish that is mentioned more frequently in our review) is typed in red.
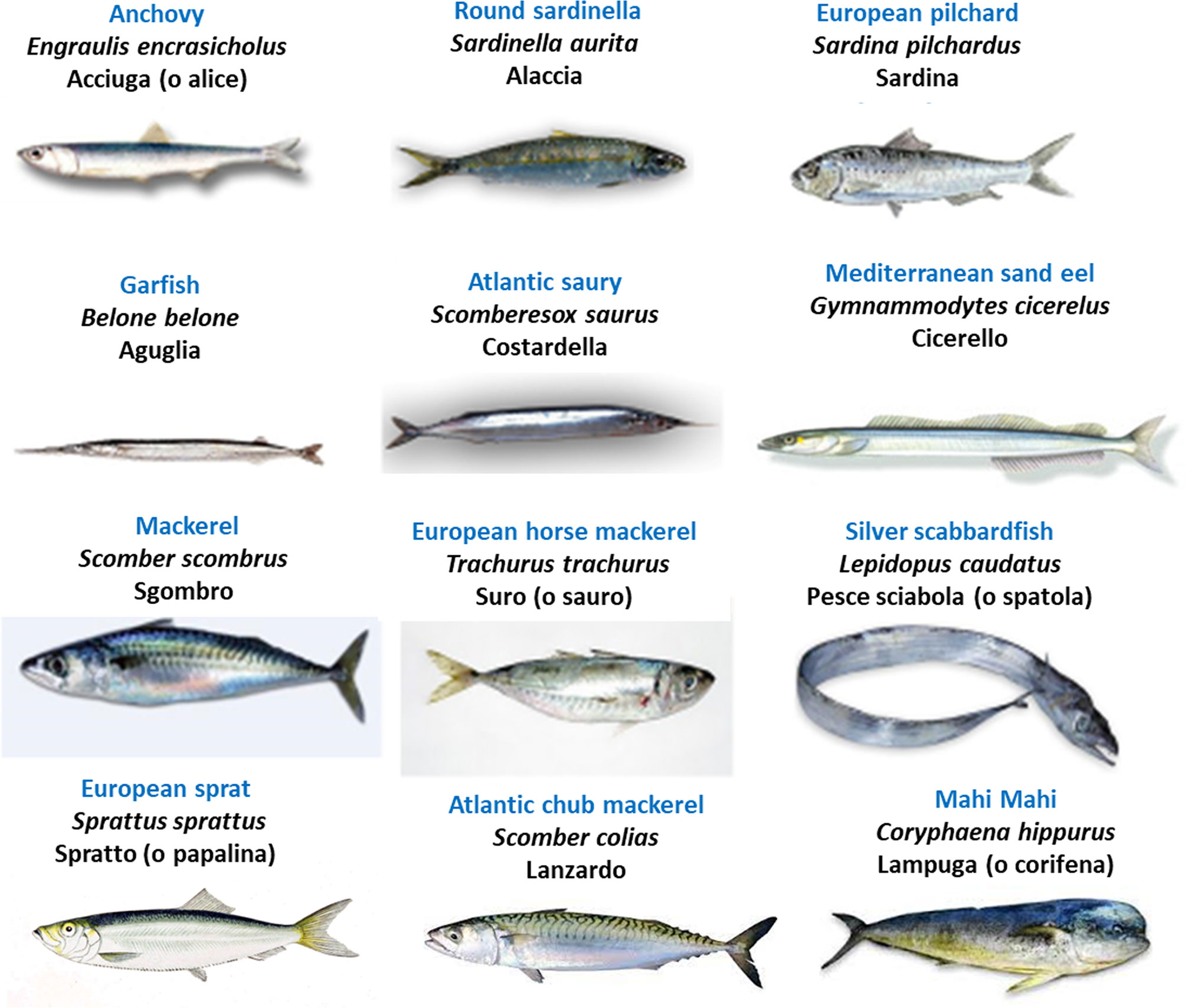
Figure 2 Illustration of oily fish (“pesce azzurro” [“azure fish”] in Italy), with the English names typed in blue, Italian in black, and scientific/Latin name in italics. Source: https://it.wikipedia.org/wiki/Pesce_azzurro.
The Bad Face of Janus
Fish and seafood contain many contaminants, since anthropic activities (e.g., agriculture, industry, mining) increase their concentration in the aquatic ecosystem (17, 41–43). Clearly, because of biomagnification, pollutants are mostly concentrated in the longer-living and larger predatory fish species such as swordfish, tuna, and shark. Classical fish contaminants are the heavy metals, with Hg being worthy of mention in this review because it acts as an environmental trigger of autoimmunity (17, 44–51), a mechanism that has also been observed for cadmium (Cd) (51–55). In predatory marine fish, approximately 90% of Hg is methylated (methylmercury), while the remainder consists of minimal amounts of inorganic mercury, ethylmercury, and phenylmercury (4).
A recent Italian study on the muscle tissue of 30 Mediterranean swordfish determined that the rank order of toxic metals was Hg, Cd and lead (Pb), while the rank order of essential metals was zinc (Zn), copper (Cu), nickel (Ni) and chrome (Cr) (56). Particularly, Hg, Cd, and Pb levels exceeded the respective critical values 1.02, 0.30 and 0.25 μg/wet weight (that is, the legal safety limits established by the European Community) in eight, three, and two of the swordfish specimens examined, respectively. In a Canadian study, Hg was detected in all samples of swordfish, tuna, marlin, and shark purchased from major supermarket outlets and fish retailers, with swordfish containing the greatest concentration (57). In a Food and Drug Administration document on “Mercury Levels in Commercial Fish and Shellfish”, where 68 types of fish are ranked based on their mean content of Hg, the 68th, 67th and 66th positions are occupied by tilefish (1.123 parts per million [ppm]), swordfish (0.995 ppm) and shark (0.979 ppm]) (58). In contrast, sardine and anchovy occupy the 5th and 8th positions with a mere 0.013 and 0.016 ppm concentration of Hg, respectively (58). Thus, it is no surprise that the blood Hg concentrations of 285 adult seafood consumers in Long Island (NY, USA) were positively associated with weekly tuna steak or sushi intake and monthly or weekly swordfish, shark, or marlin intake (59).
With particular reference to thyroid autoimmunity, associations between total blood Hg and positive thyroid autoantibodies (thyroglobulin autoantibodies [TgAb] and thyroperoxidase autoantibodies [TPOAb]) were evaluated using the National Health and Nutrition Examination Survey (NHANES), 2007-2008 (60). Such associations were searched in 2,047 non-pregnant, non-lactating women. Compared to women with the lowest Hg levels (≤0.40 μg/L), those with Hg levels >1.81 μg/L (upper quintile) had 2.24 greater odds (95% CI=1.22, 4.12) for TgAb positivity. In contrast, no significant association was found for TPOAb positivity (60). One study from the Czech Republic in patients with Hg hypersensitivity (61) concluded that removal of Hg-containing dental amalgam may help to successfully treat autoimmune thyroiditis (AIT). In this study, 27/39 AIT patients had Hg hypersensitivity (with amalgam fillings removed only in 15/27), while 12/39 AIT patients had not (controls). Serum TgAb and TPOAb were measured both at baseline and six months later. Compared to baseline, the 15 patients in whom amalgam fillings were removed had a significant decrease in the serum levels of both TgAb and TPOAb (P=0.0007). In contrast, both TgAb and TPOAb did not change in the other two groups (61). Also, for Cd, the other heavy metal that we mentioned above, a direct relationship with thyroid autoantibodies was reported (53). In Chinese women, natural log-transformed blood levels of Cd correlated directly with natural log-transformed serum levels of TgAb (53).
There is evidence for the Hg presence in the thyroid. In a very recent autopsy-based Australian study (62), the presence of intracellular inorganic Hg was searched in paraffin-embedded thyroid tissue blocks from 115 persons (68 males, 47 females; mean age = 54 years, median age = 47 years, range= 1 to 104) with varied clinicopathological conditions. Using autometallography, Hg was found in the thyrocytes with an age-dependent frequency: 4%, 9%, and 38% of persons in the age band 1-29, 30-59, and 60-104 years, respectively. The frequency of thyroid samples containing Hg was similar in males (18%) and females (21%). Laser ablation-inductively coupled plasma-mass spectrometry not only confirmed the presence of Hg, but also detected other metals in six selected samples: cadmium (n= 6), iron (n= 5), lead (n= 4), nickel (n= 2), and silver (n= 2). The authors concluded that Hg can trigger genotoxicity, autoimmunity, oxidative damage, and be involved in the pathogenesis of AIT, hypothyroidism, and thyroid cancer (TC) (62). A previous Italian study on thyroid tissue samples removed at surgery from 77 euthyroid subjects showed that Hg and Cd are significantly more concentrated in the thyroid than in the adjacent muscle and fat of the same individual (63). Worthy of note, this Italian group (64) compared the urine concentration of several metals in a Sicilian area that features an incidence of thyroid-cancer two-fold greater than a control area. The authors found that the geometric mean value in the first area was at least two-fold higher than that in the second area for eight metals, two of which being Hg and Cd (64).
Having mentioned TC is not improper, given the abundant literature on the important predisposing role for such malignancy (particularly, papillary TC) and its advanced stages exerted not only by Hashimoto’s thyroiditis (HT) but also by serum TSH per se, even by TSH levels that are within the upper values of the reference limits (65–88). In regard to TC, it is also pertinent to remind the involvement of chemokines (89–98) and the peroxisome proliferator-activated receptors (PPARs) in the molecular oncogenesis (95–106), so that both types of molecules may serve as novel targets of TC precision therapy or prevention. Quite interestingly, the beneficial health effects of DHA and EPA on metabolic diseases are thought to arise from their binding to and activation of PPARs (107), as it was shown illustratively for T1D in Table 2.
There is cross-talk between the omega-3 PUFA and the thyroid hormone pathways, as exemplified by the augmented thyroid hormone signaling pathways in the liver, this being one mechanism used by n-3 PUFAs to affect lipid metabolism (108). For instance, specific steps of TH signaling in lipid metabolism that are influenced by n-3 PUFA include higher liver expression of the thyroid hormone nuclear receptor TRβ1 and mitochondrial α-glycerophosphate dehydrogenase (109). Starting from their previous observation showing that plasma free fatty acids concentration in some hypothyroid patients is above the normal range and that this higher concentration is associated with less severe symptoms of hypothyroidism, Makino et al. investigated the effect of highly purified EPA ethyl ester (EPA-E) derived from fish oil on thyroid function in rats with methimazole-induced hypothyroidism (110). They found that oral administration of EPA-E inhibited the reduction of thyroid hormone levels and the change of thyroid follicles in the hypothyroid rats, suggesting that n-3 PUFA may prevent methimazole-induced hypothyroidism. In a Dutch study on 13 patients with hypothyroidism caused by the ablation treatment for well-differentiated TC (111), induction of hypothyroidism decreased PUFA levels in plasma, erythrocytes and polymorphonuclear leukocytes. Another site of interaction between PUFA and thyroid hormones can be the thyroid hormone plasma transport proteins. Several studies have shown that PUFA inhibit thyroid hormone binding to such carrier proteins (112, 113), one practical consequence being increased tissue availability of the biologically active protein-unbound, free thyroid hormone. In addition, studies on the brain of aged rats that were fed fish oil (27% DHA content) for one month showed an approximately 10-fold increase in the expression of transthyretin (114). Transthyretin is the second major thyroid hormone plasma carrier, which is synthesized also in the central nervous system. Since transthyretin also operates as an amyloid-beta protein scavenger, transthyretin overexpression could prevent the formation of amyloid aggregates (114). This study is relevant because decreased PUFA levels, particularly DHA, were detected in elderly subjects and patients with Alzheimer’s disease (AD), and because there is epidemiological evidence for an association between fish consumption and low prevalence of AD (114). Of interest, AD is also characterized by brain inflammation and decreased local concentration of specialized pro-resolving mediators (SPM) (115). SPM are derived from PUFA and are key in the resolution of inflammation. Because of the technical difficulties in investigating the microglia function directly, Wang et al. took advantage of the useful model of peripheral blood mononuclear cells (PBMC) (115). In their randomized, double-blind, and placebo-controlled trial on 204 AD patients, Wang et al. administered a placebo or a supplement of DHA (1.7 g) and EPA (0.6 g) daily for 6 months. At the end of treatment, in those who received the n-3 PUFA, the plasma levels of DHA and EPA levels increased. When the culture medium of PBMC incubated with amyloid-β 1-40 was analyzed, levels of the SPMs lipoxin A 4 and resolvin D1 secreted by PBMCs were decreased in the patients supplemented with placebo, but unchanged in the patients supplemented with n-3 PUFA. Changes in the levels of SPM secreted by PBMC were positively correlated to changes in plasma transthyretin, and to cognitive changes as well (115). In the setting of tissue protection by PUFA, experimental studies in rats by Videla and colleagues showed that the combination of DHA plus thyroid hormone (T3) protects from liver injury through a synergistic action that also involves causing increased intrahepatic levels of resolvins (116–120).
Table 3 summarizes human studies that link thyroid disorders with the consumption of contaminated fish (17, 18, 121–127). Overall, the consequences are impairment of thyroid function, as measured by serum levels of thyroid hormones and/or TSH, and triggering of thyroid autoimmunity, as measured by serum levels of thyroid autoantibodies. Only studies by Benvenga and colleagues addressed Hg contamination (17, 18). The group of pregnant women who consumed swordfish selectively or predominantly among other fish species ingested the greatest amounts of Hg and had the greatest both serum levels and rates of positivity for thyroid autoantibodies throughout pregnancy compared to other groups of women (17, 18). As a result, the swordfish eaters had the greatest rate of postpartum thyroiditis, since positivity for thyroid autoantibodies is a major risk factor for such autoimmune type of thyroiditis that develops within the first 12 months after parturition (17, 18).
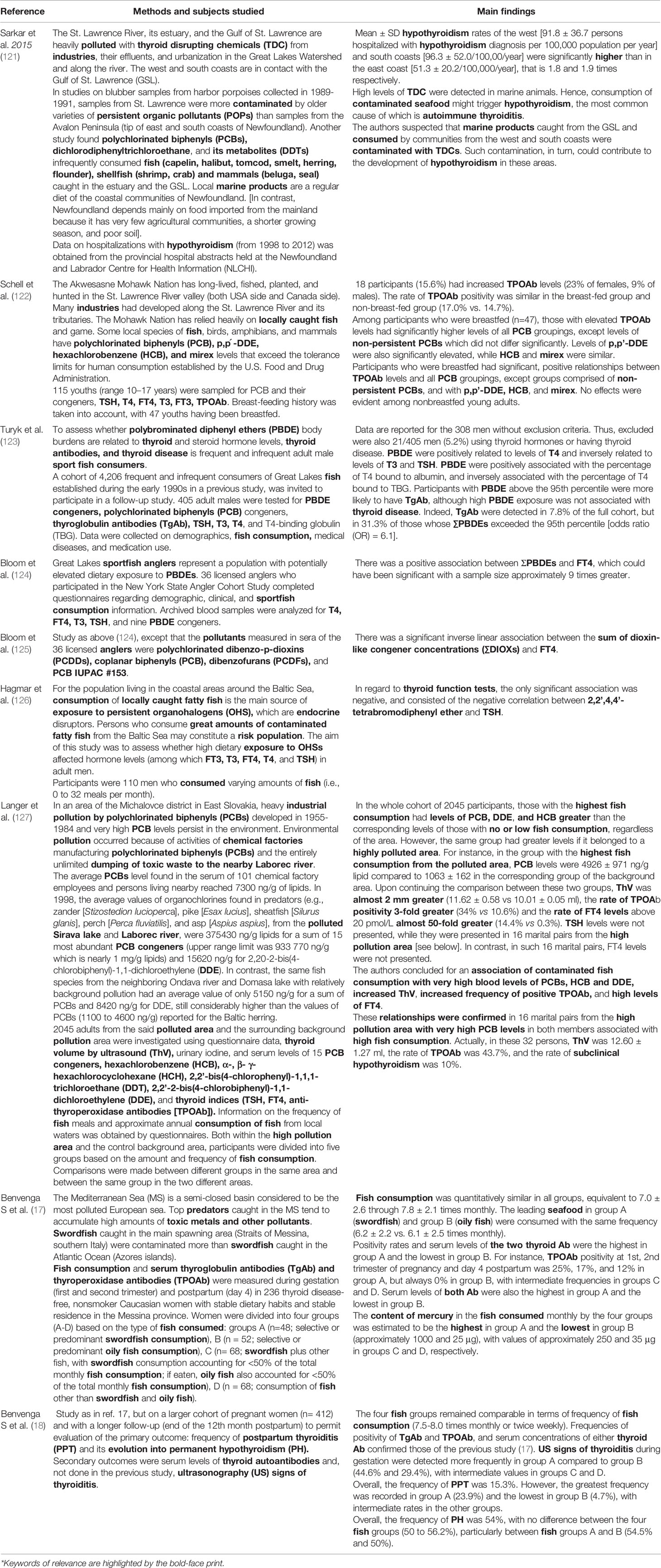
Table 3 Studies on the relationship between consumption of contaminated fish and thyroid disorders in humans. *
Fish and Omega-3 PUFA as Protection From Thyroid Disorders
In the preceding section, we have reminded the role of Hg as an autoimmunity trigger (17, 44–51). However, omega-3 PUFA antagonize this effect of Hg (128, 129). Gill et al. (129) showed that dietary ingestion of n-3 PUFA (fish oil) promotes CD95 signaling by upregulating caspase 8 activation and that DHA counteracts the negative effect of Hg on CD95 signaling in T lymphocytes (128).
The clinical benefit of thyroid autoimmune disorders given by consuming Hg-poor, omega-3-rich oily fish or by taking omega-3-based supplements (17, 18, 130–132) is summarized in Table 4. The group of pregnant women who consumed oily fish, selectively or predominantly among other species of fish, ingested the lowest amount of Hg but the greatest amount of omega- PUFA; this group of women also had the lowest both serum levels and rates of positivity for thyroid autoantibodies throughout pregnancy compared to other groups of women (17, 18). As a result, the oily fish eaters had the lowest rate of postpartum thyroiditis.
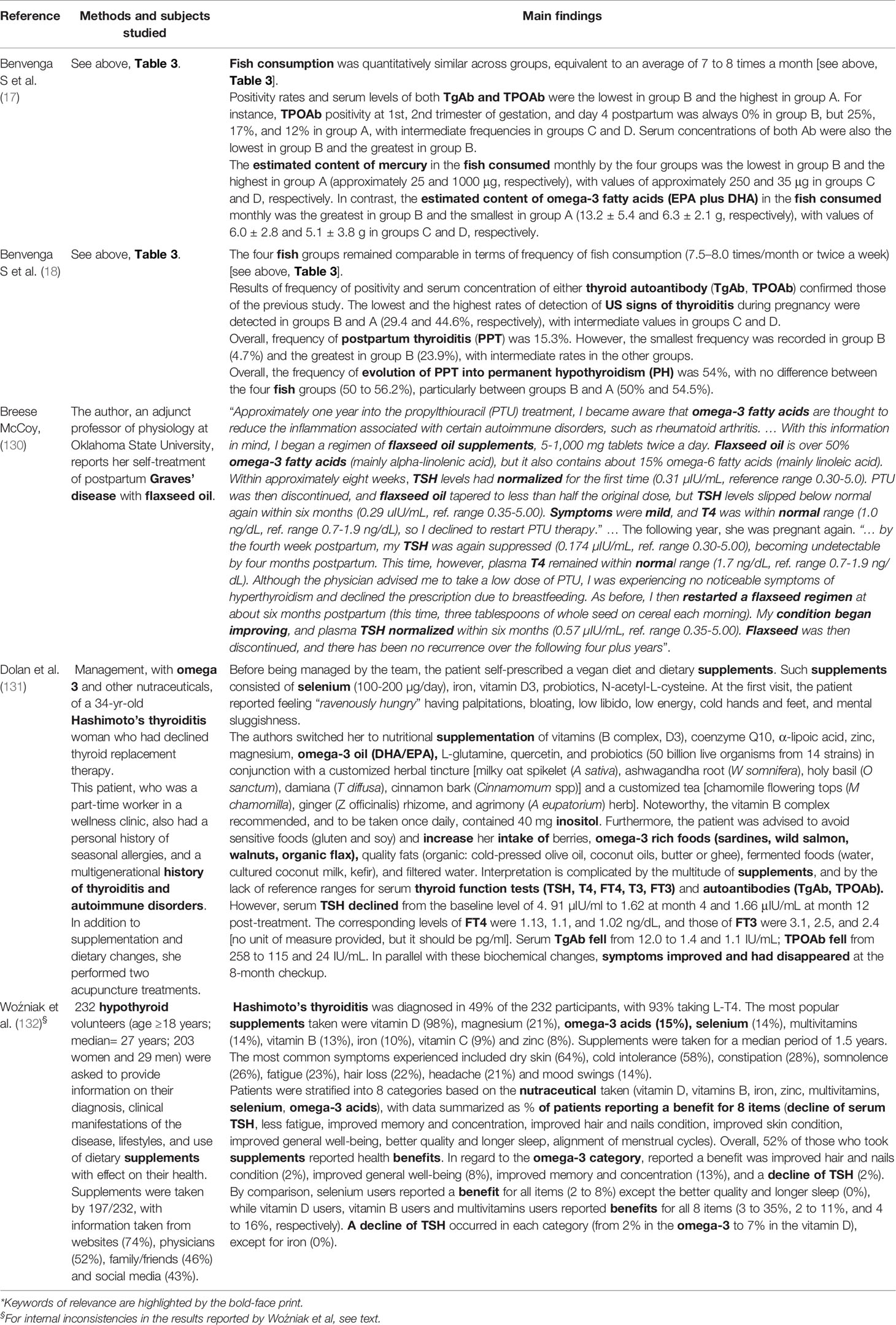
Table 4 Studies/reports on the relationship between consuming Hg-poor, omega-3-rich oily fish or by taking omega-3-based supplements and the clinical benefit towards thyroid autoimmune disorders. * §
Concerning Breese McCoy’s study (130), one comment is the limitation given by the fact that only thyroid function tests were monitored, while thyroid autoantibodies were not.
Some comments deserve the questionnaire-based study on 232 hypothyroid patients from Poland (132), a country where 15.8% of women and 2.5% of men suffer from thyroid disease, with an increase of 4.0 percent points in the year 2019 compared to 2014 (132). First, as properly described by the authors, 24% of participants were diagnosed with additional diseases (8% with polycystic ovary syndrome, 4% with depression, and 2% with insulin resistance). Second, there was enormous variability in the 197/232 hypothyroid patients who took supplements. Various was not only the spectrum of supplements taken (Table 4 of their paper) but also the periodicity of taking them (every day, 74%; irregularly, 16%; every other day, 10%). Concerning the main source of information on supplements, patients chose websites (74%), physicians (52%), family and friends (46%), and social media (43%). About two-thirds of participants took supplements according to the leaflet (71%), while one-third according to physicians’ or pharmacists’ guidelines. Results for the 197 participants (85% of 232) who took the supplements are illustrated in Table 1 of their paper (132). In that table, patients were stratified into 8 categories based on the nutraceutical taken (vitamin D, vitamins B, iron, zinc, multivitamins, selenium, omega-3 acids), with data summarized as a percent of patients reporting a benefit for 8 items, the denominator being the said 197 participants. We think that the results of this study (132) should be interpreted cautiously, mainly because it is not possible to distinguish the effect of a given nutraceutical taken alone from that of the same nutraceutical taken in association with others, and because the numbers presented in the said Table 1 are internally inconsistent. For instance, in the initial text of the Results section, the authors state that zinc supplementation was taken by 8% of the participants, therefore by 16 participants (0.08 x 197). However, upon adding the percentages reported in Table 1, the sum is 26, meaning that the number of participants claiming a benefit from zinc supplementation was 51 (0.26 x 197). For the omega-3 users, the sum of percentages is 23, meaning that the number of participants claiming a benefit from such supplementation was 45. Yet, from the initial text in the Results section, omega-3 users had to be 29 or 30 (0.15 x 197 = 29.5). Other obvious limitations of this Polish study (132) are the lack of details on the degree of hypothyroidism (overt, subclinical, either), the magnitude of change in serum levels of TSH, and having neglected to obtain information on changes in serum thyroid autoantibodies.
Concerning the mechanism behind the protection from/amelioration of AIT and the decrease in serum levels of thyroid autoantibodies caused by the omega-3 PUFA, two recent studies point to the involvement of resolvins (133, 134). Resolvins, lipoxins, maresins, and protectins are specialized pro-resolving mediators (SPM), which are downstream derivatives of PUFA (133). Resolvins of the E series (RVE1-RVE3) derive from EPA, while resolvins of the D series (RVD1-RVD6) derive from DHA. Concerning RVE-1 (5S,12R,18R-trihydroxy-eicosapentaenoic acid), which is the resolvin investigated by Song et al. (133), it triggers all aspects of the pro-resolution cascade, from inhibiting lymphocyte aggregation at the inflammation site to efferocytosis (that is, the process by which apoptotic cells are removed by phagocytic cells). The cell receptor for REV-1 is chemokine-like receptor 1 (chemR23 or CMKLR) (135), with the ligand-receptor interaction triggering anti-inflammatory responses that include marked inhibition of proinflammatory cytokines and chemokines (136). In one article, Song et al. (133) measured serum levels of REV-1 and serum thyroid parameters (TSH, T4, FT4, FT3, TPOAb, and TgAb) in 30 untreated HT patients (median and interquartile range [IQR] of TSH= 2.62, 1.95–3.05 µIU/ml) and 27 age- and sex-matched healthy controls (TSH= 2.38, IQR= 1.54–2.94, P= 0.178 vs. patients). Levels of RVE1 in patients were significantly lower compared to controls (median and IQR= 24.09, 15.76–34.38 pg/mL vs 28.51, 20.76–51.23 pg/mL). RVE1 levels correlated inversely with increasing TgAb levels in both the unadjusted model (OR= 0.945, P= 0.002) and adjusted models (OR= 0.938, P= 0.005). RVE1 levels were the lowest (19.21, 13.81–27.34 pg/mL) in the highest quartile group of TgAb levels (TgAb >361 IU/mL), and the highest (37.70, 24.66–99.16 pg/mL) in the lowest quartile group (TgAb <34 IU/mL) (19.21, 13.81–27.34 pg/mL vs 37.70, 24.66–99.16 pg/mL). Concerning TPOAb, the association with serum levels of REV1 was an inverted U-shaped curve with the lowest RVE1 levels (median and IQR= 19.21, 15.11–26.01) in the highest quartile of TPOAb levels (>431 U/mL) and the highest 31.39 (23.79–62.31) in the second quartile of TPOAb levels (13.6–106 U/mL). RVE1 levels correlated negatively also with T3 and FT3 (133). In another article, Song et al. (134) addressed RVD1 (7S, 8R, 17S-trihydroxy-4Z, 9E, 11E, 13Z, 15E, 19Z-docosahexaenoic acid) by measuring its serum levels in 30 patients with HT and 33 healthy controls. Serum concentrations of RVD1 in patients were significantly lower than in controls (median and IQR= 134.76, 85.35-201.36 pg/mL vs 187.64, 131.01-326.85 pg/mL). There was a significantly negative correlation between the TPOAb levels and the RVD1 levels. The authors also measured serum levels of the inflammatory chemokine IP-10/CXCL-10, and found that this chemokine had a significant negative association with TPOAb in the patients (134). Noteworthy, the administration of DHA (daily doses of 300 mg/kg) combined with T3 (0.05 mg/kg) to rats increased significantly the content of RVD1 and RVD2 in the liver, but left unchanged that of RVE1 and RVE2, indicating a synergistic effect compared to treatment with DHA alone and T3 alone (118). The authors concluded that co-administration of T3 and DHA enhances the capacity of liver for the resolution of inflammation by increasing RVD1 and RVD2 availability, so such co-administration constitutes an important hepatoprotective protocol for clinical purposes (118).
Omega-3 PUFA-Based Nutraceuticals in the Setting of Thyroid Disorders: Experimental Setting
Experimental studies that have evaluated the thyroidal effects of the omega-3 PUFA (137–143) are summarized in Table 5. Omega-3 PUFA were shown to have a neuroprotective (138, 139) and cardioprotective (143) action on unfavorable effects caused by thyroid hormone deficiency. On the other hand, the omega-3 PUFA mitigate, ameliorate certain hyperthyroidism-induced unfavorable effects, such as arrhythmias (141) and hepatic dysfunction (137). Such favorable effects of the omega-3 PUFA on consequences in the peripheral tissues that are caused by opposite states of thyroid dysfunction confer to the omega-3 PUFA a modulatory role that is reminiscent of another dietary nutrient, L-carnitine. Indeed, beneficial effects of L-carnitine were reported both in the hyperthyroidism setting (144, 145) and in the hypothyroidism setting (146, 147), with a modulatory role also demonstrated in the tissue glucocorticoid hormone action (148).
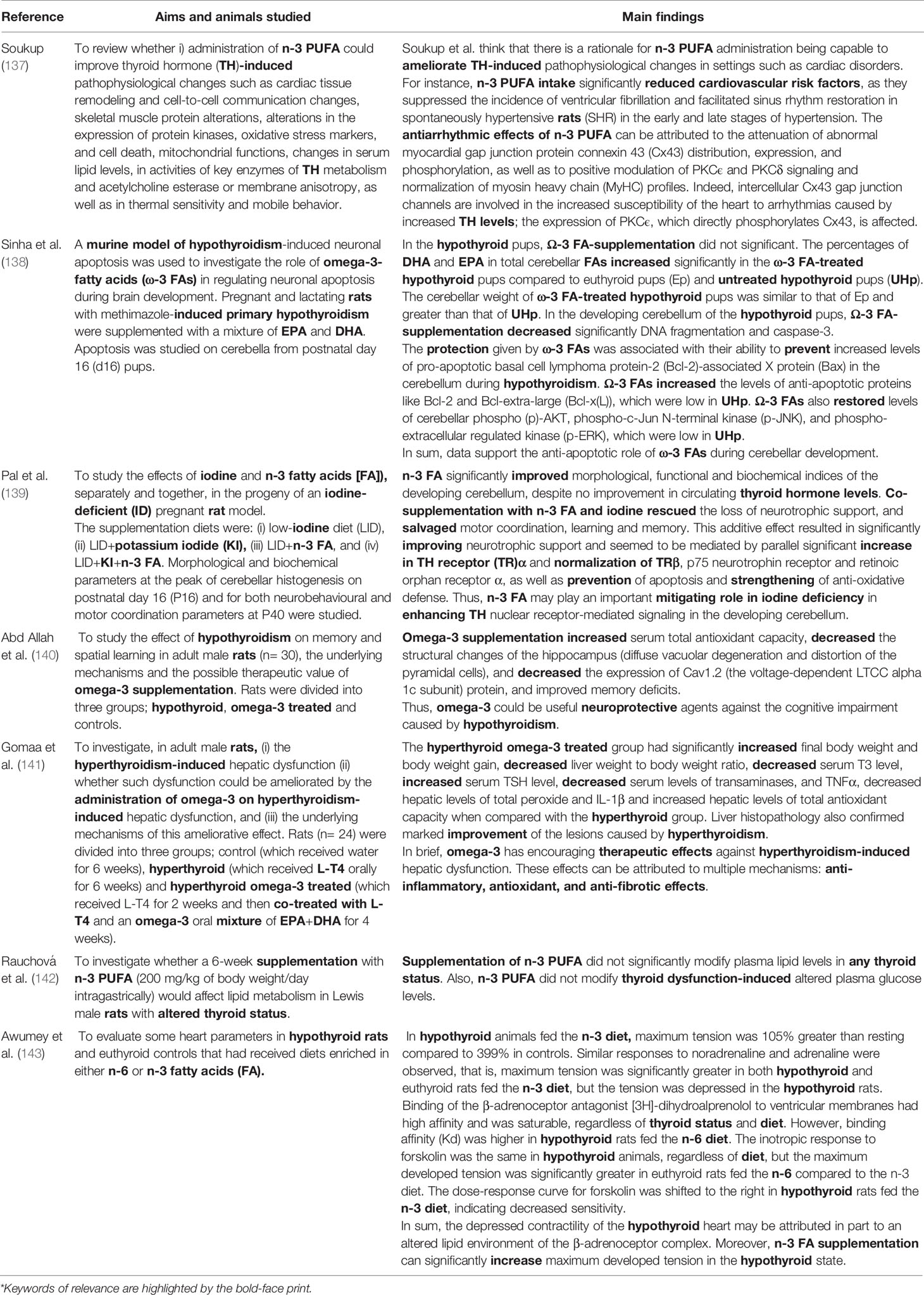
Table 5 Studies in experimental animals on the effects of the omega-3 polyunsaturated fatty acids (PUFA) in the thyroid setting. *
Protection From Thyroid Disorders Given by Nutraceuticals Other Than the Omega-3 PUFA
The very few studies available in the English-language literature on the omega-3 PUFA-based nutraceuticals in the clinical setting of autoimmune thyroid disorders (130, 131) (Table 4) contrast with the large number of studies that have tested seleno-L-methionine alone (149–151) or seleno-L-methionine combined with myo-inositol (151–157), which is a hexahydroxycyclohexane (C6H12O6) and one of the nine stereoisomers of inositol that plays a pivotal role in many metabolic pathways (158). In the studies with seleno-L-methionine combined with myo-inositol, the benefit was generally greater than that given by seleno-L-methionine alone or myo-inositol alone. Such benefit was based on the reduction of TSH, TgAb, TPOAb levels, and, when studied (154), on the reduction of serum chemokine levels (CXCL10/IP-10). Myo-inositol+seleno-L-methionine treatment protected blood mononuclear cells (PBMC) of either HT or healthy patients from H2O2–induced stress. Furthermore, the association of myo-inositol and seleno-L-methionine decreased the expression of the chemokines CXCL10/IP-10, CCL2/MCP-1 and CXCL9 (159). In another study, which evaluated the thyroid toxicity of cadmium and protection from this toxicity by nutraceuticals (160), Cd induced a marked overexpression MCP-1/CCL2 and CXCL10 in the thyroid. Again, the protection given by seleno-L-methionine combined with myo-inositol was significantly greater than that of either nutraceutical alone (160). In vitro experiments on blood cells or other cells have shown that omega-3 PUFA decrease the secretion of CXCL10/IP-10 (161–163), CXCL9 (164) and CCL2/MCP-1 (11, 165–176).
Another nutraceutical, Aloe vera (also known as Aloe barbadensis), decreased serum levels of thyroid antibodies and improved subclinical hypothyroidism (177). It is pertinent to remind Aloe vera because, among the several nutritional substances contained in its leaves, there are selenium and PUFA, including the omega-6 linoleic acid and the omega-3 linolenic acid, the latter being the second most abundant after one saturated fatty acid (palmitic acid) (178). Noteworthy, in the Aloe vera juice used in the study by Metro et al. (177) the fat content is 0.2%, with saturated fatty acids being absent. As written in that paper (177), “one of the authors decided to take Aloe Barbadensis Miller juice (ABMJ), at the dose of 50 ml every morning on an empty stomach, as a skin soother and laxative”. This author had a history of HT-associated subclinical hypothyroidism, which she monitored with periodical checks of serum thyroid function tests and thyroid autoantibodies. “At the biochemical check performed three months after having started taking ABMJ, she was struck by the remarkable improvement of all indices (Table 1). The improvement was even more impressive six months later” (177). Based on this experience, the effects of ABMJ administration were tested, in a 9-month duration trial, on HT women with levothyroxine-untreated subclinical hypothyroidism and high levels of TPOAb (n= 30). Controls were 15 HT women with untreated subclinical hypothyroidism who were matched for age and baseline levels of TPOAb, TSH, FT4 and FT3. In the Aloe-treated group, TSH, FT4 and TPOAb improved significantly already at month 3 and further (−61%, +23% and −56%, respectively) at month 9. At baseline, 100% of women had TSH > 4.0 mU/L and TPOAb > 400 U/ml, but at month 9 rates fell to 0% and 37%, respectively. In contrast, the control group had no significant changes in any index (177).
Of the three aforementioned chemokines (CXCL10/IP-10, CCL2/MCP-1 and CXCL9), only one (MCP-1) was evaluated in terms of response to Aloe vera, and only by two studies (179, 180). In one study (179), a wound dressing containing an Aloe vera extract plus gelatin decreased the production of MCP-1 by 75% in human mesenchymal stem cells treated with TGFβ. In the other study (180) the oral administration of two antidiabetic phytosterols isolated from Aloe vera lophenol (lophenol and cycloartanol) decreased serum and hepatic concentrations of MCP-1 in Zucker diabetic fatty (ZDF) rats. Nevertheless, there is abundant literature on Aloe vera being able to decrease the production of pro-inflammatory cytokines and chemokines (181–188).
Omega-3 PUFA and Oily Fish in the Nutraceutical Market
Based on an article of May 2018 by the main Italian press agency ANSA (189), Italy is the first European country for nutraceutical products based on per capita expenditure, that is, euro 40 compared to euro 28 of the EU. Italians spend more than euro 3.2 billion on dietary/supplements/nutraceutical products. A major driver for this expenditure is wishing to prevent and/or treat metabolic diseases (189) Based on the survey “The Italian food supplement supply chain, 2019-2020” by the FederSalus Research Centre (that is the national agency of the Italian producers and distributors of food supplements), in late 2019, the food supplement market in Italy reached a value of approximately euro 3.6 billion (190), corresponding to 27% of the euro 13.2 billion value of the European market, thus preceding Germany and France (18 and 8%, respectively). In particular, 32 million persons used supplements (65% of the adult Italian population) and 261 million packs (equal to 8 per capita) were sold in 2019 (190). At Italian pharmacies, the main sales channel, supplements are confirmed to be the second category after prescription drugs and give the greatest boost to growth, with 28.6 million medical prescriptions issued for food supplements in 2019 (190). Interestingly, the leading medical category that accounts for most of the 28.6 million medical prescriptions is represented by the general practitioners (21%) (190). Endocrinologists are absent in the first 8 positions, but the document fails to specify which categories are represented in the 9th category (“others”), a category that accounts for 12% of the prescriptions (190). In terms of comparison with the United States, based on data from a decade ago, annual supplement sales were USD 23 billion, with approximately 40,000 supplements products being on the market (191). In 2015, the American market for supplements was valued at USD 37 billion (151), but expected to reach USD 56.7 billion by 2024 (192) or USD 117.92 billion by 2027 (193).
With regard to the omega-3 products, their global market was valued at USD 2.10 billion in 2020 (USD 554.8 million in the US alone) (194), and it is projected to go up at a compound annual growth rate (CAGR) of 7.4% during the from 2020 to 2025 (195) and 7.8% from 2020 to 2028 (194). The main factors responsible for such increased consumption include the rising frequency of cardiovascular diseases (CVDs), changing dietary habits, the rising importance of immunity development post-COVID-19 and an increasing number of omega-3-based pharmaceutical product launches. DHA dominated the market in 2020, but EPA is expected to grow faster because of the increasing demand for immunity-boosting supplements (194).
The marine source segment of omega-3 supplements held the largest revenue share of over 82.8% in 2020. Fish oil (which contains both DHA and EPA) is the major marine source, and it is derived mainly from anchovy fish. Prices of omega-3 PUFA reflect the extraction and processing costs of fish oil, and they change depending on the availability of anchovy fish. Increasing contamination of fish by Hg and other pollutants is expected to negatively affect the prices of fish oil (194). Fish oils are currently considered the best and generally a safe source of omega-3 (196). However, the declining fish population due to overfishing has led to searching for more sustainable sources. Krill oil (which contains both DHA and EPA) and algae oil (which contains only DHA) have gained greater importance in recent years as alternatives, with algae serving as an option for the vegetarian population (197). In the US market, 9% of grocery shoppers buy high-omega-3 food or beverages in a typical shopping trip, with the proportion of adults who take fish oil supplements have increased from 8% in 2006 to 17% in 2011 (198). Omega-3 PUFA are also derived from plant sources including walnuts, pumpkin seeds, soybean oil, flaxseed oil, and canola oil, with plant oils being major sources of alpha-linolenic (ALA).
By comparison, the European market of the omega-3 products during the period (2019–2024) is expected to reach USD 14.61 billion by 2024 growing at a CAGR of 7% (199). The European demand for DHA is forecasted to increase significantly due to the favorable regulations in the European Union, which made DHA a mandatory ingredient in infant formula from 2020. Chia seeds are gaining popularity in Europe because of their nutritional and health properties that derive from their content in omega-3. Germany is the top European importer of chia seeds, preceding the Netherlands, Spain, and the United Kingdom (199).
In 2010, Friend of the Sea (FoS), an international nongovernment organization with the mission of promoting environmental conservation, introduced specific standards for producers of fish oil, fishmeal, fish feed and omega-3 supplements (200). Accredited third-party certification bodies certify that the oil originates from fisheries that are compliant with FoS sustainable fishing requirements, and that a full chain of custody occurs throughout the supply and the production chain (200). As of September 2018, 439 companies (compared to only 76 in 2015) adhere voluntarily to FoS standards for fish oil, fishmeal, fish feed and omega-3 supplement. Certified oils originate mostly from approved Peruvian anchovy fisheries and fleet (Engraulis ringens, 29%), Antarctic krill (Euphausia superba, 22%), European sardine (Sardina pilchardus, 8%), European anchovy (Engraulis encrasicolus, 7%), Chub mackerel (Scomber japonicus, 7%), Atlantic cod (Gadus morhua, 3%) (195). FoS presence in the nutraceutical industry has grown considerably in the United States, where it now accounts for over 50% of total FoS certified supplements (268 companies) (195). The United States are followed by France, Canada, Norway, United Kingdom and Italy, these six countries representing the top 6 countries for FoS labeled supplements.
Some studies are described now to illustrate the bioavalability of different types of omega-3 products. A 4-week randomized, placebo-controlled, double-blinded Icelandic study on 99 adults (of whom 77 completed the study) investigated the bioavailability of LC n-3 PUFAs from microencapsulated powder compared with ready-to-eat meals enriched with liquid fish oil (201). Participants were randomized into three groups 38 received 1.5 g/d EPA and DHA as meals enriched with liquid fish oil; 30 received the same amount of these LC n-3 PUFA as microencapsulated fish oil powder and regular meals; and 31 (controls) received placebo powder and regular meals. The authors found similar bioavailability between ready-to-eat meals enriched with liquid fish oil and LC n-3 PUFAs in encapsulated powder (201).
Starting from the fact that no conclusive information had been published on the relative bioavailability of omega-3 supplements taken as fish oil or krill oil, with few studies suggesting that the phospholipid form (krill) is absorbed better than the fish oil ethyl ester (EE) or triglyceride (TG) forms, Yurko-Mauro et al. (202) compared the oral bioavailability of the same dose of both DHA and EPA in fish oil-EE vs. fish oil-TG vs. krill oil after a four-week supplementation. In this double-blind, randomized, parallel study, 66 healthy adults were supplemented with a 1.3 g/day dose of EPA+DHA (approximately 816 mg/d EPA + 522 mg/d DHA, regardless of formulation) as either fish oil-EE (n=22 participants), fish oil-TG (n=22) or krill oil capsules (n=22). The authors found similar plasma and red blood cell levels of EPA+DHA across fish oil and krill oil products when matched for dose, DHA and EPA concentrations, indicating comparable oral bioavailability irrespective of formulation (197). For instance, mean total plasma DHA+EPA at 672 h were 90.9 ± 41 µg/mL (fish oil-EE), 108 ± 40 µg/mL fish oil-TG (fish oil-TG), and 118.5 ± 48 krill oil (krill oil), with a probability value just borderline significant (P= 0.052). Indeed, upon perusing figures in this paper (202), a decreasing hierarchy is evident for both plasma and red blood cell levels of DHA+EPA across all time points: krill oil > fish oil-TG > fish oil-EE. Furthermore, in a Canadian double-blinded, randomized, placebo-controlled, crossover trial on 24 volunteers, krill oil resulted more effective than fish oil in increasing n-3 PUFA both in plasma (P = 0.0043) and in red blood cells (P = 0.0011) (203). This study consisted of three treatment phases including krill or fish oil, each providing 600 mg of n-3 PUFA or placebo control, corn oil in capsule form (203).
DHA and EPA are added to several commercially available foods, such as infant and pet formulas, and they are also supplemented in animal feed to incorporate them in consumer dairy, meat, and poultry products (204). The main sources of EPA and DHA are fish oils or purified preparations from microalgae (204). In a one-month duration Australian study (205), 16 healthy males were provided with a range of foodstuffs naturally containing LC n-3 PUFA (fresh and canned fish, canola oil, flaxseed meal) and items fortified with fish oil (sausages, milk, margarine spread, luncheon meat, French onion dip); food choices were left to the discretion of each participant. Blood and cell levels of ALA, EPA and DHA increased highly significantly after 4 weeks (P<0.001) (205).
Conclusions
Because of the systemic action of thyroid hormones and their pleiotropic effects, thyroid disrupting chemicals (such as those mentioned in Table 3) represent a public health issue, making the comprehension of the mechanisms through which they interfere on thyroid homeostasis considerably importance.
Considering (i) the increasing incidence of both HT and TC worldwide (206–213); (ii) the aforementioned predisposing role for TC (particularly, papillary TC) and its advanced stages exerted not only by HT but also by even trendwise high serum TSH levels per se (65–88); (iii) the risk for developing metabolic and cardiovascular disorders conferred by both elevated/trendwise elevated serum TSH levels and thyroid autoimmunity (214–230), then it would be beneficial to contrast the appearance and/or duration of HT as well as to correct the slightly elevated serum TSH levels of subclinical hypothyroidism, the leading etiology of which is AIT. Furthermore, HT is frequently associated with other endocrine and nonendocrine autoimmune diseases, on which omega-3 PUFA proved to be beneficial [see above, Introduction]. For instance, 19.5% of 3,069 HT patients had evidence of at least one other autoimmune disease compared to 3.6% of 1,023 patients with multinodular goiter (231). As a corollary, there would be the place for the use of nutraceuticals to prevent/delay/minimize the onset/burden of autoimmune thyroiditis and the magnitude of TSH elevation. Taking into account their aforementioned anti-autoimmunity and anti-cancer (including TC) properties, the omega-3 PUFA would be appropriate nutraceuticals to be used, either alone or combined with other supplements.
Author Contributions
Writing - original draft: SB and FF. Writing - review and editing: SB, FF, LP, AA, GB, FV and MM. Supervision: SB, FV and MM. All authors have read and approved the final manuscript and all materials before submission.
Conflict of Interest
The authors declare that the research was conducted in the absence of any commercial or financial relationships that could be construed as a potential conflict of interest.
The reviewer SF declared a past collaboration with the authors AA, SB to the handling editor.
Publisher’s Note
All claims expressed in this article are solely those of the authors and do not necessarily represent those of their affiliated organizations, or those of the publisher, the editors and the reviewers. Any product that may be evaluated in this article, or claim that may be made by its manufacturer, is not guaranteed or endorsed by the publisher.
References
1. EUMOFA. THE EU FISH MARKET 2021 EDITION (2022). Available at: https://www.eumofa.eu/documents/20178/477018/EN_The+EU+fish+market_2021.pdf/27a6d912-a758-6065-c973-c1146ac93d30?t=1636964632989 (Accessed January 19, 2022).
2. CBI Ministry of Foreign Affairs. The European Market Potential for Tuna by-Catch Species (2022). Available at: https://www.cbi.eu/market-information/fish-seafood/tuna/market-potential (Accessed January 19, 2022).
3. Europe Oceana. ICCAT: SWORDFISH (2022) . Available at: https://europe.oceana.org/en/our-work/swordfish/overview (Accessed January 19, 2022).
4. Torres-Escribano S, Vélez D, Montoro R. Mercury and Methylmercury Bioaccessibility in Swordfish. Food Addit Contam Part A Chem Anal Control Expo Risk Assess (2010) 27:327–237. doi: 10.1080/19440040903365272
5. ASA (Associazione Stampa Agroalimentare Italiana). PRESS. LA BORSA DELLA SPESA (2022). Available at: https://www.asa-press.com/archivio/r-spesa/borsa2.html (Accessed January 19, 2022).
6. ESSERE ANIMALI. SPADARE, ARPIONI, PALANGARI: NON C’è TREGUA PER IL PESCE SPADA . Available at: https://www.essereanimali.org/2020/08/spadare-arpioni-palangari-consumo-pesce-spada/ (Accessed January 19, 2022).
7. Giosuè C, Gancitano V, Sprovieri M, Bono G, Vitale S. A Responsible Proposal for Italian Seafood Consumers. Eur J Sustain Dev (2018) 7:523–44. doi: 10.14207/ejsd.2018.v7n3p523
8. Maulu S, Nawanzi K, Abdel-Tawwab M, Khalil HS. Fish Nutritional Value as an Approach to Children's Nutrition. Front Nutr (2021) 8:780844. doi: 10.3389/fnut.2021.780844
9. Li J, Pora BLR, Dong K, Hasjim J. Health Benefits of Docosahexaenoic Acid and its Bioavailability: A Review. Food Sci Nutr (2021) 9:5229–43. doi: 10.1002/fsn3.2299
10. Kapoor B, Kapoor D, Gautam S, Singh R, Bhardwaj S. Dietary Polyunsaturated Fatty Acids (PUFAs): Uses and Potential Health Benefits. Curr Nutr Rep (2021) 10:232–42. doi: 10.1007/s13668-021-00363-3
11. Pisaniello AD, Psaltis PJ, King PM, Liu G, Gibson RA, Tan JT, et al. Omega-3 Fatty Acids Ameliorate Vascular Inflammation: A Rationale for Their Atheroprotective Effects. Atherosclerosis (2021) 324:27–37. doi: 10.1016/j.atherosclerosis.2021.03.003
12. Chen C, Huang H, Dai QQ, Ren J, Cai HH, Hu WJ, et al. Fish Consumption, Long-Chain Omega-3 Fatty Acids Intake and Risk of Stroke: An Updated Systematic Review and Meta-Analysis. Asia Pac J Clin Nutr (2021) 30:140–52. doi: 10.6133/apjcn.202103_30(1).0017
13. Chen J, Jayachandran M, Bai W, Xu B. A Critical Review on the Health Benefits of Fish Consumption and its Bioactive Constituents. Food Chem (2022) 369:130874. doi: 10.1016/j.foodchem.2021.130874
14. Mendivil CO. Fish Consumption: A Review of Its Effects on Metabolic and Hormonal Health. Nutr Metab Insights (2021) 14:11786388211022378. doi: 10.1177/11786388211022378
15. Liu C, Ralston NVC. Seafood and Health: What You Need to Know? Adv Food Nutr Res (2021) 97:275–318. doi: 10.1016/bs.afnr.2021.04.001
16. Li X, Bi X, Wang S, Zhang Z, Li F, Zhao AZ. Therapeutic Potential of ω-3 Polyunsaturated Fatty Acids in Human Autoimmune Diseases. Front Immunol (2019) 10:2241. doi: 10.3389/fimmu.2019.02241
17. Benvenga S, Vigo MT, Metro D, Granese R, Vita R, Le Donne M. Type of Fish Consumed and Thyroid Autoimmunity in Pregnancy and Postpartum. Endocrine (2016) 52:120–9. doi: 10.1007/s12020-015-0698-3
18. Benvenga S, Vita R, Di Bari F, Granese R, Metro D, Le Donne M. Stable Consumption of Swordfish Favors, Whereas Stable Consumption of Oily Fish Protects From, Development of Postpartum Thyroiditis. Endocrine (2019) 65:94–101. doi: 10.1007/s12020-019-01882-4
19. Pattison DJ, Harrison RA, Symmons DP. The Role of Diet in Susceptibility to Rheumatoid Arthritis: A Systematic Review. J Rheumatol (2004) 31:1310–9.
20. Koller-Smith L, Mehdi AM, March L, Tooth L, Mishra G, Thomas R. Rheumatoid Arthritis is a Preventable Disease: 11 Ways to Reduce Your Patients' Risk. Intern Med J (2021). doi: 10.1111/imj.15537
21. Asoudeh F, Jayedi A, Kavian Z, Ebrahimi-Mousavi S, Nielsen SM, Mohammadi H. A Systematic Review and Meta-Analysis of Observational Studies on the Association Between Animal Protein Sources and Risk of Rheumatoid Arthritis. Clin Nutr (2021) 40:4644–52. doi: 10.1016/j.clnu.2021.05.026
22. Di Giuseppe D, Wallin A, Bottai M, Askling J, Wolk A. Long-Term Intake of Dietary Long-Chain N-3 Polyunsaturated Fatty Acids and Risk of Rheumatoid Arthritis: A Prospective Cohort Study of Women. Ann Rheum Dis (2014) 73:1949–53. doi: 10.1136/annrheumdis-2013-203338
23. Tedeschi SK, Bathon JM, Giles JT, Lin TC, Yoshida K, Solomon DH. Relationship Between Fish Consumption and Disease Activity in Rheumatoid Arthritis. Arthritis Care Res (Hoboken) (2018) 70:327–32. doi: 10.1002/acr.23295
24. Sköldstam L, Börjesson O, Kjällman A, Seiving B, Akesson B. Effect of Six Months of Fish Oil Supplementation in Stable Rheumatoid Arthritis. A Double-Blind, Controlled Study. Scand J Rheumatol (1992) 21:178–85. doi: 10.3109/03009749209099218
25. Langer-Gould A, Black LJ, Waubant E, Smith JB, Wu J, Gonzales EG, et al. Seafood, Fatty Acid Biosynthesis Genes, and Multiple Sclerosis Susceptibility. Mult Scler (2020) 26:1476–85. doi: 10.1177/1352458519872652
26. Rezaeizadeh H, Mohammadpour Z, Bitarafan S, Harirchian MH, Ghadimi M, Homayon IA. Dietary Fish Intake and the Risk of Multiple Sclerosis: A Systematic Review and Meta-Analysis of Observational Studies. Nutr Neurosci (2022) 25:681–9. doi: 10.1080/1028415X.2020.1804096
27. Black LJ, Zhao Y, Peng YC, Sherriff JL, Lucas RM, van der Mei I, et al. Higher Fish Consumption and Lower Risk of Central Nervous System Demyelination. Eur J Clin Nutr (2020) 74:818–24. doi: 10.1038/s41430-019-0476-z
28. Bäärnhielm M, Olsson T, Alfredsson L. Fatty Fish Intake is Associated With Decreased Occurrence of Multiple Sclerosis. Mult Scler (2014) 20:726–32. doi: 10.1177/1352458513509508
29. Syrjälä E, Nevalainen J, Peltonen J, Takkinen HM, Hakola L, Åkerlund M, et al. A Joint Modeling Approach for Childhood Meat, Fish and Egg Consumption and the Risk of Advanced Islet Autoimmunity. Sci Rep (2019) 9:7760. doi: 10.1038/s41598-019-44196-1
30. Abdel-Megeid AA, Ael-R A, SS E, Ibrahim AM. Effect of Different Types of Fish on Rats Suffering From Diabetes. Nutr Health (2008) 19:257–71. doi: 10.1177/026010600801900402
31. Purdel C, Ungurianu A, Margina D. Metabolic and Metabolomic Insights Regarding the Omega-3 PUFAs Intake in Type 1 Diabetes Mellitus. Front Mol Biosci (2021) 8:783065. doi: 10.3389/fmolb.2021.783065
32. Cadario F, Pozzi E, Rizzollo S, Stracuzzi M, Beux S, Giorgis A, et al. Vitamin D and ω-3 Supplementations in Mediterranean Diet During the 1st Year of Overt Type 1 Diabetes: A Cohort Study. Nutrients (2019) 11:2158. doi: 10.3390/nu11092158
33. Bi X, Li F, Liu S, Jin Y, Zhang X, Yang T, et al. ω-3 Polyunsaturated Fatty Acids Ameliorate Type 1 Diabetes and Autoimmunity. J Clin Invest (2017) 127:1757–71. doi: 10.1172/JCI87388
34. Norris JM, Yin X, Lamb MM, Barriga K, Seifert J, Hoffman M, et al. Omega-3 Polyunsaturated Fatty Acid Intake and Islet Autoimmunity in Children at Increased Risk for Type 1 Diabetes. JAMA (2007) 298:1420–8. doi: 10.1001/jama.298.12.1420
35. Vitlov Uljević M, Starčević K, Mašek T, Bočina I, Restović I, Kević N, et al. Dietary DHA/EPA Supplementation Ameliorates Diabetic Nephropathy by Protecting From Distal Tubular Cell Damage. Cell Tissue Res (2019) 378:301–17. doi: 10.1007/s00441-019-03058-y
36. Lewis EJH, Perkins BA, Lovblom LE, Bazinet RP, Wolever TMS, Bril V. Effect of Omega-3 Supplementation on Neuropathy in Type 1 Diabetes: A 12-Month Pilot Trial. Neurology (2017) 88:2294–301. doi: 10.1212/WNL.0000000000004033
37. WIKIPEDIA. PESCE AZZURRO (2022). Available at: https://it.wikipedia.org/wiki/Pesce_azzurro (Accessed January 19, 2022).
38. Greupner T, Kutzner L, Nolte F, Strangmann A, Kohrs H, Hahn A, et al. Effects of a 12-Week High-α-Linolenic Acid Intervention on EPA and DHA Concentrations in Red Blood Cells and Plasma Oxylipin Pattern in Subjects With a Low EPA and DHA Status. Food Funct (2018) 9:1587–600. doi: 10.1039/c7fo01809f
39. Stephen NM, Jeya Shakila R, Jeyasekaran G, Sukumar D. Effect of Different Types of Heat Processing on Chemical Changes in Tuna. J Food Sci Technol (2010) 47:174–81. doi: 10.1007/s13197-010-0024-2
40. Zotos A, Kotaras A, Mikras E. Effect of Baking of Sardine (Sardina Pilchardus) and Frying of Anchovy (Engraulis Encrasicholus) in Olive and Sunflower Oil on Their Quality. Food Sci Technol Int (2013) 19:11–23. doi: 10.1177/1082013212442179
41. Zuluaga Rodríguez J, Gallego Ríos SE, Ramírez Botero CM. Content of Hg, Cd, Pb and As in Fish Species: A Review. Vitae (2015) 22:148–59. doi: 10.17533/udea.vitae.v22n2a09
42. Al Taee SK, Al-Mallah K, Ismail HHK. Review On Some Heavy Metals Toxicity On Freshwater Fishes. J Appl Vet Sci (2020) 5:78–86. doi: 10.21608/javs.2020.100157
43. Yousifi RA, Choudhary MI, Ahmed S, Ahmed Q. Bioaccumulation of Heavy Metals in Fish and Other Aquatic Organisms From Karachi Coast, Pakistan. Nusantara Biosci (2021) 13:73–84. doi: 10.13057/nusbiosci/n130111
44. Amirhosseini M, Alkaissi H, Hultman PA, Havarinasab S. Autoantibodies in Outbred Swiss Webster Mice Following Exposure to Gold and Mercury. Toxicol Appl Pharmacol (2021) 412:115379. doi: 10.1016/j.taap.2020.115379
45. Wacewicz-Muczyńska M, Socha K, Soroczyńska J, Niczyporuk M, Borawska MH. Cadmium, Lead and Mercury in the Blood of Psoriatic and Vitiligo Patients and Their Possible Associations With Dietary Habits. Sci Total Environ (2021) 757:143967. doi: 10.1016/j.scitotenv.2020.143967
46. Kern JK, Geier DA, Mehta JA, Homme KG, Geier MR. Mercury as a Hapten: A Review of the Role of Toxicant-Induced Brain Autoantibodies in Autism and Possible Treatment Considerations. J Trace Elem Med Biol (2020) 62:126504. doi: 10.1016/j.jtemb.2020.126504
47. Bjørklund G, Peana M, Dadar M, Chirumbolo S, Aaseth J, Martins N. Mercury-Induced Autoimmunity: Drifting From Micro to Macro Concerns on Autoimmune Disorders. Clin Immunol (2020) 213:108352. doi: 10.1016/j.clim.2020.108352
48. Lu M, Khera S. Autoimmune Manifestations of Acute Mercury Toxicity. Clin Pediatr (Phila) (2020) 59:816–8. doi: 10.1177/0009922820915885
49. Pollard KM, Cauvi DM, Toomey CB, Hultman P, Kono DH. Mercury-Induced Inflammation and Autoimmunity. Biochim Biophys Acta Gen Subj (2019) 1863:129299. doi: 10.1016/j.bbagen.2019.02.001
50. Crowe W, Allsopp PJ, Watson GE, Magee PJ, Strain JJ, Armstrong DJ, et al. Mercury as an Environmental Stimulus in the Development of Autoimmunity - A Systematic Review. Autoimmun Rev (2017) 16:72–80. doi: 10.1016/j.autrev.2016.09.020
51. Kindgren E, Guerrero-Bosagna C, Ludvigsson J. Heavy Metals in Fish and its Association With Autoimmunity in the Development of Juvenile Idiopathic Arthritis: A Prospective Birth Cohort Study. Pediatr Rheumatol Online J (2019) 17:33. doi: 10.1186/s12969-019-0344-3
52. Popov Aleksandrov A, Mirkov I, Tucovic D, Kulas J, Zeljkovic M, Popovic D, et al. Immunomodulation by Heavy Metals as a Contributing Factor to Inflammatory Diseases and Autoimmune Reactions: Cadmium as an Example. Immunol Lett (2021) 240:106–22. doi: 10.1016/j.imlet.2021.10.003
53. Nie X, Chen Y, Chen Y, Chen C, Han B, Li Q, et al. Lead and Cadmium Exposure, Higher Thyroid Antibodies and Thyroid Dysfunction in Chinese Women. Environ Pollut (2017) 230:320–8. doi: 10.1016/j.envpol.2017.06.052
54. Ohsawa M. Heavy Metal-Induced Immunotoxicity and its Mechanisms. Yakugaku Zasshi (2009) 129:305–19. doi: 10.1248/yakushi.129.305
55. Leffel EK, Wolf C, Poklis A, White KL Jr. Drinking Water Exposure to Cadmium, an Environmental Contaminant, Results in the Exacerbation of Autoimmune Disease in the Murine Model. Toxicology (2003) 188:233–50. doi: 10.1016/s0300-483x(03)00092-1
56. Barone G, Dambrosio A, Storelli A, Garofalo R, Busco VP, Storelli MM. Estimated Dietary Intake of Trace Metals From Swordfish Consumption: A Human Health Problem. Toxics (2018) 6:22. doi: 10.3390/toxics6020022
57. Forsyth DS, Casey V, Dabeka RW, McKenzie A. Methylmercury Levels in Predatory Fish Species Marketed in Canada. Food Addit Contam (2004) 21:849–56. doi: 10.1080/02652030400004259
58. U.S. Food and Drug Administration. Mercury Levels in Commercial Fish and Shellfish 1990-2012 (2022). Available at: https://www.fda.gov/food/metals-and-your-food/mercury-levels-commercial-fish-and-shellfish-1990-2012 (Accessed January 19, 2022).
59. Karimi R, Silbernagel S, Fisher NS, Meliker JR. Elevated Blood Hg at Recommended Seafood Consumption Rates in Adult Seafood Consumers. Int J Hyg Environ Health (2014) 217:758–864. doi: 10.1016/j.ijheh.2014.03.007
60. Gallagher CM, Meliker JR. Mercury and Thyroid Autoantibodies in U.S. Women, NHANES 2007-2008. Environ Int (2012) 40:39–43. doi: 10.1016/j.envint.2011.11.014
61. Sterzl I, Prochazkova J, Hrda P, Matucha P, Bartova J, Stejskal V. Removal of Dental Amalgam Decreases Anti-TPO and Anti-Tg Autoantibodies in Patients With Autoimmune Thyroiditis. Neuro Endocrinol Lett (2006) 27:25–30.
62. Pamphlett R, Doble PA, Bishop DP. Mercury in the Human Thyroid Gland: Potential Implications for Thyroid Cancer, Autoimmune Thyroiditis, and Hypothyroidism. PloS One (2021) 16:e0246748. doi: 10.1371/journal.pone.0246748
63. Malandrino P, Russo M, Ronchi A, Moretti F, Gianì F, Vigneri P, et al. Concentration of Metals and Trace Elements in the Normal Human and Rat Thyroid: Comparison With Muscle and Adipose Tissue and Volcanic Versus Control Areas. Thyroid (2020) 30:290–9. doi: 10.1089/thy.2019.0244
64. Malandrino P, Russo M, Gianì F, Pellegriti G, Vigneri P, Belfiore A, et al. Increased Thyroid Cancer Incidence in Volcanic Areas: A Role of Increased Heavy Metals in the Environment? Int J Mol Sci (2020) 21:3425. doi: 10.3390/ijms21103425
65. Feldt-Rasmussen U. Hashimoto's Thyroiditis as a Risk Factor for Thyroid Cancer. Curr Opin Endocrinol Diabetes Obes (2020) 27:364–71. doi: 10.1097/MED.0000000000000570
66. Vita R, Ieni A, Tuccari G, Benvenga S. The Increasing Prevalence of Chronic Lymphocytic Thyroiditis in Papillary Microcarcinoma. Rev Endocr Metab Disord (2018) 19:301–9. doi: 10.1007/s11154-018-9474-z
67. Zirilli G, Salzano G, Corica D, Pajno GB, Mignosa C, Pepe G, et al. Thyrotropin Serum Levels and Coexistence With Hashimoto's Thyroiditis as Predictors of Malignancy in Children With Thyroid Nodules. Ital J Pediatr (2019) 45:96. doi: 10.1186/s13052-019-0693-z
68. Penta L, Cofini M, Lanciotti L, Leonardi A, Principi N, Esposito S. Hashimoto's Disease and Thyroid Cancer in Children: Are They Associated? Front Endocrinol (Lausanne) (2018) 9:565. doi: 10.3389/fendo.2018.00565
69. Abbasgholizadeh P, Naseri A, Nasiri E, Sadra V. Is Hashimoto Thyroiditis Associated With Increasing Risk of Thyroid Malignancies? A Systematic Review and Meta-Analysis. Thyroid Res (2021) 14:26. doi: 10.1186/s13044-021-00117-x
70. Hanege FM, Tuysuz O, Celik S, Sakallıoglu O, Arslan Solmaz O. Hashimoto's Thyroiditis in Papillary Thyroid Carcinoma: A 22-Year Study. Acta Otorhinolaryngol Ital (2021) 41:142–5. doi: 10.14639/0392-100X-N1081
71. Resende de Paiva C, Grønhøj C, Feldt-Rasmussen U, von Buchwald C. Association Between Hashimoto's Thyroiditis and Thyroid Cancer in 64,628 Patients. Front Oncol (2017) 7:53. doi: 10.3389/fonc.2017.00053
72. Ieni A, Vita R, Magliolo E, Santarpia M, Di Bari F, Benvenga S, et al. One-Third of an Archivial Series of Papillary Thyroid Cancer (Years 2007-2015) Has Coexistent Chronic Lymphocytic Thyroiditis, Which Is Associated With a More Favorable Tumor-Node-Metastasis Staging. Front Endocrinol (Lausanne) (2017) 8:337. doi: 10.3389/fendo.2017.00337
73. Uhliarova B, Hajtman A. Hashimoto's Thyroiditis - an Independent Risk Factor for Papillary Carcinoma. Braz J Otorhinolaryngol (2018) 84:729–35. doi: 10.1016/j.bjorl.2017.08.012
74. Graceffa G, Patrone R, Vieni S, Campanella S, Calamia S, Laise I, et al. Association Between Hashimoto's Thyroiditis and Papillary Thyroid Carcinoma: A Retrospective Analysis of 305 Patients. BMC Endocr Disord (2019) 19:26. doi: 10.1186/s12902-019-0351-x
75. Ye ZQ, Gu DN, Hu HY, Zhou YL, Hu XQ, Zhang XH. Hashimoto's Thyroiditis, Microcalcification and Raised Thyrotropin Levels Within Normal Range are Associated With Thyroid Cancer. World J Surg Oncol (2013) 11:56. doi: 10.1186/1477-7819-11-56
76. Bircan HY, Koc B, Akarsu C, Demiralay E, Demirag A, Adas M, et al. Is Hashimoto's Thyroiditis a Prognostic Factor for Thyroid Papillary Microcarcinoma? Eur Rev Med Pharmacol Sci (2014) 18:1910–5.
77. Boelaert K, Horacek J, Holder RL, Watkinson JC, Sheppard MC, Franklyn JA. Serum Thyrotropin Concentration as a Novel Predictor of Malignancy in Thyroid Nodules Investigated by Fine-Needle Aspiration. J Clin Endocrinol Metab (2006) 91:4295–301. doi: 10.1210/jc.2006-0527
78. Fiore E, Rago T, Provenzale MA, Scutari M, Ugolini C, Basolo F, et al. Lower Levels of TSH are Associated With a Lower Risk of Papillary Thyroid Cancer in Patients With Thyroid Nodular Disease: Thyroid Autonomy may Play a Protective Role. Endocr Relat Cancer (2009) 16:1251–60. doi: 10.1677/ERC-09-0036
79. Huang H, Rusiecki J, Zhao N, Chen Y, Ma S, Yu H, et al. Thyroid-Stimulating Hormone, Thyroid Hormones, and Risk of Papillary Thyroid Cancer: A Nested Case-Control Study. Cancer Epidemiol Biomarkers Prev (2017) 26:1209–18. doi: 10.1158/1055-9965.EPI-16-0845
80. Hu MJ, Zhang C, Liang L, Wang SY, Zheng XC, Zhang Q, et al. Fasting Serum Glucose, Thyroid-Stimulating Hormone, and Thyroid Hormones and Risk of Papillary Thyroid Cancer: A Case-Control Study. Head Neck (2019) 41:2277–84. doi: 10.1002/hed.25691
81. Kim HI, Jang HW, Ahn HS, Ahn S, Park SY, Oh YL, et al. High Serum TSH Level Is Associated With Progression of Papillary Thyroid Microcarcinoma During Active Surveillance. J Clin Endocrinol Metab (2018) 103:446–51. doi: 10.1210/jc.2017-01775
82. Tam AA, Ozdemir D, Aydın C, Bestepe N, Ulusoy S, Sungu N, et al. Association Between Preoperative Thyrotrophin and Clinicopathological and Aggressive Features of Papillary Thyroid Cancer. Endocrine (2018) 59:565–72. doi: 10.1007/s12020-018-1523-6
83. Golbert L, de Cristo AP, Faccin CS, Farenzena M, Folgierini H, Graudenz MS, et al. Serum TSH Levels as a Predictor of Malignancy in Thyroid Nodules: A Prospective Study. PloS One (2017) 12:e0188123. doi: 10.1371/journal.pone.0188123
84. Soleimanisardoo L, Rouhani M, Sardoo FS, Gozashti MH. The Effect of Thyroid Stimulating Hormone on Stage of Differentiated Thyroid Carcinoma. Endocrinol Diabetes Metab (2021) 4:e00266. doi: 10.1002/edm2.266
85. Mao A, An N, Wang J, Wu Y, Wang T, Wang Z, et al. Association Between Preoperative Serum TSH and Tumor Status in Patients With Papillary Thyroid Microcarcinoma. Endocrine (2021) 73:617–24. doi: 10.1007/s12020-021-02690-5
86. Zhang X, Zhang X, Chang Z, Wu C, Guo H. Correlation Analyses of Thyroid-Stimulating Hormone and Thyroid Autoantibodies With Differentiated Thyroid Cancer. J BUON (2018) 23:1467–71.
87. Wu X, Lun Y, Jiang H, Gang Q, Xin S, Duan Z, et al. Coexistence of Thyroglobulin Antibodies and Thyroid Peroxidase Antibodies Correlates With Elevated Thyroid-Stimulating Hormone Level and Advanced Tumor Stage of Papillary Thyroid Cancer. Endocrine (2014) 46:554–60. doi: 10.1007/s12020-013-0121-x
88. Duccini K, de Souza MVL, Delfim R, Aguiar AP, Teixeira P, Vaisman M. High Serum Thyrotropin Concentrations Within the Reference Range: A Predictor of Malignancy in Nodular Thyroid Disease. Med Princ Pract (2018) 27:272–7. doi: 10.1159/000488196
89. Qin XJ, Lin X, Xue G, Fan HL, Wang HY, Wu JF, et al. CXCL10 is a Potential Biomarker and Associated With Immune Infiltration in Human Papillary Thyroid Cancer. Biosci Rep (2021) 41:BSR20203459. doi: 10.1042/BSR20203459
90. Lee SA, Choi JH, Cho SJ, Chang JW, Maeng YH. The Clinical Usefulness of Chemokine C-X-C Motif Ligand 12 as a Diagnostic Marker for Papillary Thyroid Carcinoma. Indian J Pathol Microbiol (2020) 63:544–50. doi: 10.4103/IJPM.IJPM_722_19
91. Wu W, Ren F, Guo M, Yang J, Xiao Y, Liu W. Increased Expression of CX3CL1 and CX3CR1 in Papillary Thyroid Carcinoma. Histol Histopathol (2020) 35:1189–96. doi: 10.14670/HH-18-265
92. Kim MJ, Sun HJ, Song YS, Yoo SK, Kim YA, Seo JS, et al. CXCL16 Positively Correlated With M2-Macrophage Infiltration, Enhanced Angiogenesis, and Poor Prognosis in Thyroid Cancer. Sci Rep (2019) 9:13288. doi: 10.1038/s41598-019-49613-z
93. Wang W, Chu HY, Zhong ZM, Qi X, Cheng R, Qin RJ, et al. Platelet-Secreted CCL3 and its Receptor CCR5 Promote Invasive and Migratory Abilities of Anaplastic Thyroid Carcinoma Cells via MMP-1. Cell Signal (2019) 63:109363. doi: 10.1016/j.cellsig.2019.109363
94. Coperchini F, Croce L, Marinò M, Chiovato L, Rotondi M. Role of Chemokine Receptors in Thyroid Cancer and Immunotherapy. Endocr Relat Cancer (2019) 26:R465–78. doi: 10.1530/ERC-19-0163
95. Fallahi P, Ferrari SM, Piaggi S, Luconi M, Cantini G, Gelmini S, et al. The Paramount Role of Cytokines and Chemokines in Papillary Thyroid Cancer: A Review and Experimental Results. Immunol Res (2018) 66:710–22. doi: 10.1007/s12026-018-9056-x
96. Ferrari SM, Elia G, Piaggi S, Baldini E, Ulisse S, Miccoli M, et al. CCL2 Is Modulated by Cytokines and PPAR-γ in Anaplastic Thyroid Cancer. Anticancer Agents Med Chem (2018) 18:458–66. doi: 10.2174/1871520617666170719152349
97. Cui D, Zhao Y, Xu J. Activated CXCL5-CXCR2 Axis Promotes the Migration, Invasion and EMT of Papillary Thyroid Carcinoma Cells via Modulation of β-Catenin Pathway. Biochimie (2018) 148:1–11. doi: 10.1016/j.biochi.2018.02.009
98. Yapa S, Mulla O, Green V, England J, Greenman J. The Role of Chemokines in Thyroid Carcinoma. Thyroid (2017) 27:1347–59. doi: 10.1089/thy.2016.0660
99. Ferrari SM, Materazzi G, Baldini E, Ulisse S, Miccoli P, Antonelli A, et al. Antineoplastic Effects of Pparγ Agonists, With a Special Focus on Thyroid Cancer. Curr Med Chem (2016) 23:636–49. doi: 10.2174/0929867323666160203114607
100. Jin JQ, Han JS, Ha J, Baek HS, Lim DJ. Lobeglitazone, A Peroxisome Proliferator-Activated Receptor-Gamma Agonist, Inhibits Papillary Thyroid Cancer Cell Migration and Invasion by Suppressing P38 MAPK Signaling Pathway. Endocrinol Metab (Seoul) (2021) 36:1095–110. doi: 10.3803/EnM.2021.1155
101. Chen JY, Wang JJ, Lee HC, Chi CW, Lee CH, Hsu YC. Combination of Peroxisome Proliferator-Activated Receptor Gamma and Retinoid X Receptor Agonists Induces Sodium/Iodide Symporter Expression and Inhibits Cell Growth of Human Thyroid Cancer Cells. J Chin Med Assoc (2020) 83:923–30. doi: 10.1097/JCMA.0000000000000389
102. Copland JA, Marlow LA, Kurakata S, Fujiwara K, Wong AK, Kreinest PA, et al. Novel High-Affinity PPARgamma Agonist Alone and in Combination With Paclitaxel Inhibits Human Anaplastic Thyroid Carcinoma Tumor Growth via P21waf1/CIP1. Oncogene (2006) 25:2304–17. doi: 10.1038/sj.onc.1209267
103. Ohta K, Endo T, Haraguchi K, Hershman JM, Onaya T. Ligands for Peroxisome Proliferator-Activated Receptor Gamma Inhibit Growth and Induce Apoptosis of Human Papillary Thyroid Carcinoma Cells. J Clin Endocrinol Metab (2001) 86:2170–7. doi: 10.1210/jcem.86.5.7493
104. Hayashi N, Nakamori S, Hiraoka N, Tsujie M, Xundi X, Takano T, et al. Antitumor Effects of Peroxisome Proliferator Activate Receptor Gamma Ligands on Anaplastic Thyroid Carcinoma. Int J Oncol (2004) 24:89–95. doi: 10.3892/ijo.24.1.89
105. Bonofiglio D, Qi H, Gabriele S, Catalano S, Aquila S, Belmonte M, et al. Peroxisome Proliferator-Activated Receptor Gamma Inhibits Follicular and Anaplastic Thyroid Carcinoma Cells Growth by Upregulating P21cip1/WAF1 Gene in a Sp1-Dependent Manner. Endocr Relat Cancer (2008) 15:545–57. doi: 10.1677/ERC-07-0272
106. Yousefnia S, Momenzadeh S, Seyed Forootan F, Ghaedi K, Nasr Esfahani MH. The Influence of Peroxisome Proliferator-Activated Receptor γ (Pparγ) Ligands on Cancer Cell Tumorigenicity. Gene (2018) 649:14–22. doi: 10.1016/j.gene.2018.01.018
107. Gani OA. Are Fish Oil Omega-3 Long-Chain Fatty Acids and Their Derivatives Peroxisome Proliferator-Activated Receptor Agonists? Cardiovasc Diabetol (2008) 7:6. doi: 10.1186/1475-2840-7-6
108. Souza LL, Nunes MO, Paula GS, Cordeiro A, Penha-Pinto V, Neto JF, et al. Effects of Dietary Fish Oil on Thyroid Hormone Signaling in the Liver. J Nutr Biochem (2010) 21:935–40. doi: 10.1016/j.jnutbio.2009.07.008
109. Souza LL, Cordeiro A, Oliveira LS, de Paula GS, Faustino LC, Ortiga-Carvalho TM, et al. Thyroid Hormone Contributes to the Hypolipidemic Effect of Polyunsaturated Fatty Acids From Fish Oil: In Vivo Evidence for Cross Talking Mechanisms. J Endocrinol (2011) 211:65–72. doi: 10.1530/JOE-11-0142
110. Makino M, Oda N, Miura N, Imamura S, Yamamoto K, Kato T, et al. Effect of Eicosapentaenoic Acid Ethyl Ester on Hypothyroid Function. J Endocrinol (2001) 171:259–65. doi: 10.1677/joe.0.1710259
111. van Doormaal JJ, Muskiet FA, Martini IA, Doorenbos H. Changes in Fatty Acid Profiles of Plasma, Erythrocytes and Polymorphonuclear Leukocytes in Induced Hypothyroidism in Man: Indirect Evidence for Altered Delta 6 Desaturase Activity. Clin Chim Acta (1986) 156:299–313. doi: 10.1016/0009-8981(86)90073-2
112. Benvenga S, Li Calzi L, Robbins J. Effect of Free Fatty Acids and Nonlipid Inhibitors of Thyroid Hormone Binding in the Immunoradiometric Assay of Thyroxin-Binding Globulin. Clin Chem (1987) 33:1752–5. doi: 10.1093/clinchem/33.10.1752
113. Tabachnick M, Korcek L. Effect of Long-Chain Fatty Acids on the Binding of Thyroxine and Triiodothyronine to Human Thyroxine-Binding Globulin. Biochim Biophys Acta (1986) 881:292–6. doi: 10.1016/0304-4165(86)90016-4
114. Puskás LG, Kitajka K, Nyakas C, Barcelo-Coblijn G, Farkas T. Short-Term Administration of Omega 3 Fatty Acids From Fish Oil Results in Increased Transthyretin Transcription in Old Rat Hippocampus. Proc Natl Acad Sci USA (2003) 100:1580–5. doi: 10.1073/pnas.0337683100
115. Wang X, Hjorth E, Vedin I, Eriksdotter M, Freund-Levi Y, Wahlund LO, et al. Effects of N-3 FA Supplementation on the Release of Proresolving Lipid Mediators by Blood Mononuclear Cells: The OmegAD Study. J Lipid Res (2015) 56:674–81. doi: 10.1194/jlr.P055418
116. Videla LA, Fernández V, Vargas R, Cornejo P, Tapia G, Varela N, et al. Upregulation of Rat Liver Pparα-FGF21 Signaling by a Docosahexaenoic Acid and Thyroid Hormone Combined Protocol. Biofactors (2016) 42:638–46. doi: 10.1002/biof.1300
117. Videla LA. Combined Docosahexaenoic Acid and Thyroid Hormone Supplementation as a Protocol Supporting Energy Supply to Precondition and Afford Protection Against Metabolic Stress Situations. IUBMB Life (2019) 71:1211–20. doi: 10.1002/iub.2067
118. Videla LA, Vargas R, Valenzuela R, Muñoz P, Corbari A, Hernandez-Rodas MC. Combined Administration of Docosahexaenoic Acid and Thyroid Hormone Synergistically Enhances Rat Liver Levels of Resolvins RvD1 and Rvd2. Prostaglandins Leukot Essent Fatty Acids (2019) 140:42–6. doi: 10.1016/j.plefa.2018.11.013
119. Mardones M, Valenzuela R, Romanque P, Covarrubias N, Anghileri F, Fernández V, et al. Prevention of Liver Ischemia Reperfusion Injury by a Combined Thyroid Hormone and Fish Oil Protocol. J Nutr Biochem (2012) 23:1113–20. doi: 10.1016/j.jnutbio.2011.06.004
120. Vargas R, Riquelme B, Fernández J, Álvarez D, Pérez IF, Cornejo P, et al. Docosahexaenoic Acid-Thyroid Hormone Combined Protocol as a Novel Approach to Metabolic Stress Disorders: Relation to Mitochondrial Adaptation via Liver PGC-1α and Sirtuin1 Activation. Biofactors (2019) 45:271–8. doi: 10.1002/biof.1483
121. Sarkar A, Knight JC, Babichuk NA, Mulay S. Skewed Distribution of Hypothyroidism in the Coastal Communities of Newfoundland, Canada. Environ Int (2015) 83:171–5. doi: 10.1016/j.envint.2015.05.017
122. Schell LM, Gallo MV, Ravenscroft J, DeCaprio AP. Persistent Organic Pollutants and Anti-Thyroid Peroxidase Levels in Akwesasne Mohawk Young Adults. Environ Res (2009) 109:86–92. doi: 10.1016/j.envres.2008.08.015
123. Turyk ME, Persky VW, Imm P, Knobeloch L, Chatterton R, Anderson HA. Hormone Disruption by PBDEs in Adult Male Sport Fish Consumers. Environ Health Perspect (2008) 116:1635–41. doi: 10.1289/ehp.11707
124. Bloom M, Spliethoff H, Vena J, Shaver S, Addink R, Eadon G. Environmental Exposure to PBDEs and Thyroid Function Among New York Anglers. Environ Toxicol Pharmacol (2008) 25:386–92. doi: 10.1016/j.etap.2007.12.004
125. Bloom M, Vena J, Olson J, Moysich K. Chronic Exposure to Dioxin-Like Compounds and Thyroid Function Among New York Anglers. Environ Toxicol Pharmacol (2006) 21:260–7. doi: 10.1016/j.etap.2005.09.001
126. Hagmar L, Björk J, Sjödin A, Bergman A, Erfurth EM. Plasma Levels of Persistent Organohalogens and Hormone Levels in Adult Male Humans. Arch Environ Health (2001) 56:138–43. doi: 10.1080/00039890109604065
127. Langer P, Kocan A, Tajtaková M, Petrík J, Chovancová J, Drobná B, et al. Fish From Industrially Polluted Freshwater as the Main Source of Organochlorinated Pollutants and Increased Frequency of Thyroid Disorders and Dysglycemia. Chemosphere (2007) 67:S379–385. doi: 10.1016/j.chemosphere.2006.05.132
128. Gill R, Lanni L, Jen KL, McCabe MJ Jr, Rosenspire A. Docosahexaenoic Acid Counteracts Attenuation of CD95-Induced Cell Death by Inorganic Mercury. Toxicol Appl Pharmacol (2015) 282:61–7. doi: 10.1016/j.taap.2014.11.005
129. Gill R, Jen KL, McCabe MJ, Rosenspire A. Dietary N-3 PUFAs Augment Caspase 8 Activation in Staphylococcal Aureus Enterotoxin B Stimulated T-Cells. Toxicol Appl Pharmacol (2016) 309:141–8. doi: 10.1016/j.taap.2016.09.002
130. Breese McCoy SJ. Coincidence of Remission of Postpartum Graves' Disease and Use of Omega-3 Fatty Acid Supplements. Thyroid Res (2011) 4:16. doi: 10.1186/1756-6614-4-16
131. Dolan K, Finley H, Gasta M, Houseman S. Managing Hashimoto's Thyroiditis Through Personalized Care: A Case Report. Altern Ther Health Med (2018) 24:56–61.
132. Woźniak D, Drzymała S, Przysławski J, Drzymała-Czyż S. Dietary Supplements in Hypothyroidism. Acta Sci Pol Technol Aliment (2021) 20:375–81. doi: 10.17306/J.AFS.2021.0985
133. Song J, Sun R, Zhang Y, Ke J, Zhao D. Serum Resolvin E1 Levels and its Relationship With Thyroid Autoimmunity in Hashimoto's Thyroiditis: A Preliminary Study. BMC Endocr Disord (2021) 21:66. doi: 10.1186/s12902-021-00730-9
134. Song J, Sun R, Zhang Y, Fu Y, Zhao D. Role of the Specialized Pro-Resolving Mediator Resolvin D1 in Hashimoto's Thyroiditis. Exp Clin Endocrinol Diabetes (2021) 129:791–7. doi: 10.1055/a-1345-0173
135. Arita M, Bianchini F, Aliberti J, Sher A, Chiang N, Hong S, et al. Stereochemical Assignment, Antiinflammatory Properties, and Receptor for the Omega-3 Lipid Mediator Resolvin E1. J Exp Med (2005) 201:713–22. doi: 10.1084/jem.20042031
136. Bannenberg GL, Chiang N, Ariel A, Arita M, Tjonahen E, Gotlinger KH, et al. Molecular Circuits of Resolution: Formation and Actions of Resolvins and Protectins. J Immunol (2005) 174:4345–55. doi: 10.4049/jimmunol.174.7.4345
137. Soukup T. Effects of Long-Term Thyroid Hormone Level Alterations, N-3 Polyunsaturated Fatty Acid Supplementation and Statin Administration in Rats. Physiol Res (2014) 63:S119–131. doi: 10.33549/physiolres.932623
138. Sinha RA, Khare P, Rai A, Maurya SK, Pathak A, Mohan V, et al. Anti-Apoptotic Role of Omega-3-Fatty Acids in Developing Brain: Perinatal Hypothyroid Rat Cerebellum as Apoptotic Model. Int J Dev Neurosci (2009) 27:377–83. doi: 10.1016/j.ijdevneu.2009.02.003
139. Pal A, Mohan V, Modi DR, Sinha RA, Rastogi L, Kumar P, et al. Iodine Plus N-3 Fatty Acid Supplementation Augments Rescue of Postnatal Neuronal Abnormalities in Iodine-Deficient Rat Cerebellum. Br J Nutr (2013) 110:659–70. doi: 10.1017/S0007114512005569
140. Abd Allah ES, Gomaa AM, Sayed MM. The Effect of Omega-3 on Cognition in Hypothyroid Adult Male Rats. Acta Physiol Hung (2014) 101:362–76. doi: 10.1556/APhysiol.101.2014.3.11
141. Gomaa AM, Abd El-Aziz EA. Omega-3 Fatty Acids Decreases Oxidative Stress, Tumor Necrosis Factor-Alpha, and Interleukin-1 Beta in Hyperthyroidism-Induced Hepatic Dysfunction Rat Model. Pathophysiology (2016) 23:295–301. doi: 10.1016/j.pathophys.2016.10.001
142. Rauchová H, Vokurková M, Pavelka S, Behuliak M, Tribulová N, Soukup T. N-3 Polyunsaturated Fatty Acids Supplementation Does Not Affect Changes of Lipid Metabolism Induced in Rats by Altered Thyroid Status. Horm Metab Res (2013) 45:507–12. doi: 10.1055/s-0033-1334944
143. Awumey EM, Paton DM, Pehowich DJ. Thyroid Status and Dietary Fatty Acids Affect Beta-Adrenoceptor Agonist Stimulation of Tension Development in Rat Myocardium. J Auton Pharmacol (1995) 15:73–84. doi: 10.1111/j.1474-8673.1995.tb00293.x
144. Benvenga S. Effects of L-Carnitine on Thyroid Hormone Metabolism and on Physical Exercise Tolerance. Horm Metab Res (2005) 37:566–71. doi: 10.1055/s-2005-870424
145. Chee R, Agah R, Vita R, Benvenga S. L-Carnitine Treatment in a Seriously Ill Cancer Patient With Severe Hyperthyroidism. Hormones (Athens) (2014) 13:407–12. doi: 10.14310/horm.2002.149
146. An JH, Kim YJ, Kim KJ, Kim SH, Kim NH, Kim HY, et al. L-Carnitine Supplementation for the Management of Fatigue in Patients With Hypothyroidism on Levothyroxine Treatment: A Randomized, Double-Blind, Placebo-Controlled Trial. Endocr J (2016) 63:885–95. doi: 10.1507/endocrj.EJ16-0109
147. Benvenga S, Sindoni A. L-Carnitine Supplementation for the Management of Fatigue in Patients With Hypothyroidism on Levothyroxine Treatment. Endocr J (2016) 63:937–8. doi: 10.1507/endocrj.EJ16-0374
148. Alesci S, De Martino MU, Mirani M, Benvenga S, Trimarchi F, Kino T, et al. L-Carnitine: A Nutritional Modulator of Glucocorticoid Receptor Functions. FASEB J (2003) 17:1553–5. doi: 10.1096/fj.02-1024fje
149. Duntas LH, Benvenga S. Selenium: An Element for Life. Endocrine (2015) 48:756–75. doi: 10.1007/s12020-014-0477-6
150. Wichman J, Winther KH, Bonnema SJ, Hegedüs L. Selenium Supplementation Significantly Reduces Thyroid Autoantibody Levels in Patients With Chronic Autoimmune Thyroiditis: A Systematic Review and Meta-Analysis. Thyroid (2016) 26:1681–92. doi: 10.1089/thy.2016.0256
151. Benvenga S, Feldt-Rasmussen U, Bonofiglio D, Asamoah E. Nutraceutical Supplements in the Thyroid Setting: Health Benefits Beyond Basic Nutrition. Nutrients (2019) 11:2214. doi: 10.3390/nu11092214
152. Nordio M, Pajalich R. Combined Treatment With Myo-Inositol and Selenium Ensures Euthyroidism in Subclinical Hypothyroidism Patients With Autoimmune Thyroiditis. J Thyroid Res (2013) 2013:424163. doi: 10.1155/2013/424163
153. Nordio M, Basciani S. Myo-Inositol Plus Selenium Supplementation Restores Euthyroid State in Hashimoto’s Patients With Subclinical Hypothyroidism. Eur Rev Med Pharmacol Sci (2017) 21:51–9. doi: 10.1155/2017/2549491
154. Ferrari SM, Fallahi P, Di Bari F, Vita R, Benvenga S, Antonelli A. Myo-Inositol and Selenium Reduce the Risk of Developing Overt Hypothyroidism in Patients With Autoimmune Thyroiditis. Eur Rev Med Pharmacol Sci (2017) 21:36–42.
155. Pace C, Tumino D, Russo M, Le Moli R, Naselli A, Borzì G, et al. Role of Selenium and Myo-Inositol Supplementation on Autoimmune Thyroiditis Progression. Endocr J (2020) 67:1093–8. doi: 10.1507/endocrj.EJ20-0062
156. Porcaro G, Angelozzi P. Myo-Inositol and Selenium Prevent Subclinical Hypothyroidism During Pregnancy: An Observational Study. IJMDAT (2018) 1:e164.
157. Benvenga S, Nordio M, Laganà AS, Unfer V. The Role of Inositol in Thyroid Physiology and in Subclinical Hypothyroidism Management. Front Endocrinol (Lausanne) (2021) 12:662582. doi: 10.3389/fendo.2021.662582
158. Dinicola S, Unfer V, Facchinetti F, Soulage CO, Greene ND, Bizzarri M, et al. Inositols: From Established Knowledge to Novel Approaches. Int J Mol Sci (2021) 22:10575. doi: 10.3390/ijms221910575
159. Benvenga S, Vicchio T, Di Bari F, Vita R, Fallahi P, Ferrari SM, et al. Favorable Effects of Myo-Inositol, Selenomethionine or Their Combination on the Hydrogen Peroxide-Induced Oxidative Stress of Peripheral Mononuclear Cells From Patients With Hashimoto's Thyroiditis: Preliminary In Vitro Studies. Eur Rev Med Pharmacol Sci (2017) 21:89–101.
160. Benvenga S, Marini HR, Micali A, Freni J, Pallio G, Irrera N, et al. Protective Effects of Myo-Inositol and Selenium on Cadmium-Induced Thyroid Toxicity in Mice. Nutrients (2020) 12:1222. doi: 10.3390/nu12051222
161. Jensen KN, Omarsdottir SY, Reinhardsdottir MS, Hardardottir I, Freysdottir J. Docosahexaenoic Acid Modulates NK Cell Effects on Neutrophils and Their Crosstalk. Front Immunol (2020) 11:570380. doi: 10.3389/fimmu.2020.570380
162. Li Z, Choi JH, Oh HJ, Park SH, Lee JB, Yoon KC. Effects of Eye Drops Containing a Mixture of Omega-3 Essential Fatty Acids and Hyaluronic Acid on the Ocular Surface in Desiccating Stress-Induced Murine Dry Eye. Curr Eye Res (2014) 39:871–8. doi: 10.3109/02713683.2014.884595
163. Saedisomeolia A, Wood LG, Garg ML, Gibson PG, Wark PA. Anti-Inflammatory Effects of Long-Chain N-3 PUFA in Rhinovirus-Infected Cultured Airway Epithelial Cells. Br J Nutr (2009) 101:533–40. doi: 10.1017/S0007114508025798
164. Henao Agudelo JS, Baia LC, Ormanji MS, Santos ARP, Machado JR, Saraiva Câmara NO, et al. Fish Oil Supplementation Reduces Inflammation But Does Not Restore Renal Function and Klotho Expression in an Adenine-Induced CKD Model. Nutrients (2018) 10:1283. doi: 10.3390/nu10091283
165. Baker EJ, Valenzuela CA, De Souza CO, Yaqoob P, Miles EA, Calder PC. Comparative Anti-Inflammatory Effects of Plant- and Marine-Derived Omega-3 Fatty Acids Explored in an Endothelial Cell Line. Biochim Biophys Acta Mol Cell Biol Lipids (2020) 1865:158662. doi: 10.1016/j.bbalip.2020.158662
166. Harari A, Leikin Frenkel A, Barshack I, Sagee A, Cohen H, Kamari Y, et al. Addition of Fish Oil to Atherogenic High Fat Diet Inhibited Atherogenesis While Olive Oil did Not, in LDL Receptor KO Mice. Nutr Metab Cardiovasc Dis (2020) 30:709–16. doi: 10.1016/j.numecd.2019
167. Serini S, Cassano R, Facchinetti E, Amendola G, Trombino S, Calviello G. Anti-Irritant and Anti-Inflammatory Effects of DHA Encapsulated in Resveratrol-Based Solid Lipid Nanoparticles in Human Keratinocytes. Nutrients (2019) 11:1400. doi: 10.3390/nu11061400
168. Ferguson JF, Roberts-Lee K, Borcea C, Smith HM, Midgette Y, Shah R. Omega-3 Polyunsaturated Fatty Acids Attenuate Inflammatory Activation and Alter Differentiation in Human Adipocytes. J Nutr Biochem (2019) 64:45–9. doi: 10.1016/j.jnutbio.2018.09.027
169. Khadge S, Thiele GM, Sharp JG, McGuire TR, Klassen LW, Black PN, et al. Long-Chain Omega-3 Polyunsaturated Fatty Acids Modulate Mammary Gland Composition and Inflammation. J Mammary Gland Biol Neoplasia (2018) 23:43–58. doi: 10.1007/s10911-018-9391-5
170. Liang P, Henning SM, Schokrpur S, Wu L, Doan N, Said J, et al. Effect of Dietary Omega-3 Fatty Acids on Tumor-Associated Macrophages and Prostate Cancer Progression. Prostate (2016) 76:1293–302. doi: 10.1002/pros.23218
171. Yoshihara T, Shimada K, Fukao K, Sai E, Sato-Okabayashi Y, Matsumori R, et al. Omega 3 Polyunsaturated Fatty Acids Suppress the Development of Aortic Aneurysms Through the Inhibition of Macrophage-Mediated Inflammation. Circ J (2015) 79:1470–8. doi: 10.1253/circj.CJ-14-0471
172. Monk JM, Liddle DM, De Boer AA, Brown MJ, Power KA, Ma DW, et al. Fish-Oil-Derived N-3 PUFAs Reduce Inflammatory and Chemotactic Adipokine-Mediated Cross-Talk Between Co-Cultured Murine Splenic CD8+ T Cells and Adipocytes. J Nutr (2015) 145:829–38. doi: 10.3945/jn.114.205443
173. Baranowski M, Enns J, Blewett H, Yakandawala U, Zahradka P, Taylor CG. Dietary Flaxseed Oil Reduces Adipocyte Size, Adipose Monocyte Chemoattractant Protein-1 Levels and T-Cell Infiltration in Obese, Insulin-Resistant Rats. Cytokine (2012) 59:382–91. doi: 10.1016/j.cyto.2012.04.004
174. Diaz Encarnacion MM, Warner GM, Cheng J, Gray CE, Nath KA, Grande JP. N-3 Fatty Acids Block TNF-α-Stimulated MCP-1 Expression in Rat Mesangial Cells. Am J Physiol Renal Physiol (2011) 300:F1142–1151. doi: 10.1152/ajprenal.00064.2011
175. Meijerink J, Plastina P, Vincken JP, Poland M, Attya M, Balvers M, et al. The Ethanolamide Metabolite of DHA, Docosahexaenoylethanolamine, Shows Immunomodulating Effects in Mouse Peritoneal and RAW264.7 Macrophages: Evidence for a New Link Between Fish Oil and Inflammation. Br J Nutr (2011) 105:1798–807. doi: 10.1017/S0007114510005635
176. An WS, Kim HJ, Cho KH, Vaziri ND. Omega-3 Fatty Acid Supplementation Attenuates Oxidative Stress, Inflammation, and Tubulointerstitial Fibrosis in the Remnant Kidney. Am J Physiol Renal Physiol (2009) 297:F895–903. doi: 10.1152/ajprenal.00217.2009
177. Metro D, Cernaro V, Papa M, Benvenga S. Marked Improvement of Thyroid Function and Autoimmunity by Aloe Barbadensis Miller Juice in Patients With Subclinical Hypothyroidism. J Clin Transl Endocrinol (2018) 11:18–25. doi: 10.1016/j.jcte.2018.01.003
178. Ahmad M, Nangyal H, Sherwani S, Islam Z, Shah S. Effect of Heat Stress on Fatty Acids Profiles of Aloe Vera and Bryophyllum Pinnatum Leaves. World Appl Sci J (2013) 28:1592–6. doi: 10.5829/idosi.wasj.2013.28.11.14128
179. Woeller CF, Woodroof A, Cottler PS, Pollock SJ, Haidaris CG, Phipps RP. In Vitro Characterization of Variable Porosity Wound Dressing With Anti-Scar Properties. Eplasty (2018) 18:e21.
180. Misawa E, Tanaka M, Nomaguchi K, Nabeshima K, Yamada M, Toida T, et al. Oral Ingestion of Aloe Vera Phytosterols Alters Hepatic Gene Expression Profiles and Ameliorates Obesity-Associated Metabolic Disorders in Zucker Diabetic Fatty Rats. J Agric Food Chem (2012) 60:2799–806. doi: 10.1021/jf204465j
181. Sánchez M, González-Burgos E, Iglesias I, Gómez-Serranillos MP. Pharmacological Update Properties of Aloe Vera and its Major Active Constituents. Molecules (2020) 25:1324. doi: 10.3390/molecules25061324
182. Klaikeaw N, Wongphoom J, Werawatganon D, Chayanupatkul M, Siriviriyakul P. Anti-Inflammatory and Anti-Oxidant Effects of Aloe Vera in Rats With non-Alcoholic Steatohepatitis. World J Hepatol (2020) 12:363–77. doi: 10.4254/wjh.v12.i7.363
183. Seo JM, Cheng S, Soliman N, Nabi Z, Pan L. The Blend of Taurine and Aloe Vera Extract Boosts Action Against Skin Irritation: In Vitro and Clinical Evaluations. J Cosmet Sci (2018) 69:213–28.
184. Na HS, Song YR, Kim S, Heo JY, Chung HY, Chung J. Aloin Inhibits Interleukin (IL)-1β-Stimulated IL-8 Production in KB Cells. J Periodontol (2016) 87:e108–115. doi: 10.1902/jop.2016.150447
185. Radha MH, Laxmipriya NP. Evaluation of Biological Properties and Clinical Effectiveness of Aloe Vera: A Systematic Review. J Tradit Complement Med (2014) 5:21–6. doi: 10.1016/j.jtcme.2014.10.006
186. Budai MM, Varga A, Milesz S, Tőzsér J, Benkő S. Aloe Vera Downregulates LPS-Induced Inflammatory Cytokine Production and Expression of NLRP3 Inflammasome in Human Macrophages. Mol Immunol (2013) 56:471–9. doi: 10.1016/j.molimm.2013.05.005
187. Shin E, Shim KS, Kong H, Lee S, Shin S, Kwon J, et al. Dietary Aloe Improves Insulin Sensitivity via the Suppression of Obesity-Induced Inflammation in Obese Mice. Immune Netw (2011) 11:59–67. doi: 10.4110/in.2011.11.1.59
188. Langmead L, Makins RJ, Rampton DS. Anti-Inflammatory Effects of Aloe Vera Gel in Human Colorectal Mucosa. vitro. Aliment Pharmacol Ther (2004) 19:521–7. doi: 10.1111/j.1365-2036.2004.01874.x
189. ANSA. L'Italia Prima Nell'ue Per Gli Acquisti Di Nutraceutical (2022). Available at: https://www.ansa.it/cibus_2018/notizie/salute_benessere/2018/05/07/italia-prima-in-ue-acquisti-nutraceutica_3db89be3-de8d-4920-88b1-7d5d22f61e05.html (Accessed January 19, 2022).
190. FEDERSALUS. 5th Industry Survey. The Italian Food Supplement Supply Chain 2019-2020 (2022). Available at: https://www.federsalus.it/wp-content/uploads/2020/06/Report_5th-Industry-survey-2.pdf (Accessed January 19, 2022).
191. Wootan GD, Phillips MB. Detox Diets for Dummies. Hoboken, NJ, (USA: Wiley Publishing, Inc (2010). 88 p.
192. STATISTA. Total U.S. Dietary Supplements Market Size From 2016 to 2024 (2022). Available at: https://www.statista.com/statistics/828481/total-dietary-supplements-market-size-in-the-us/ (Accessed January 19, 2022).
193. GLOBE NEWS WIRE. Dietary Supplements Market Worth USD 117.92 Billion by 2027 (2022). Available at: https://www.globenewswire.com/news-release/2021/10/22/2319238/0/en/Dietary-Supplements-Market-Worth-USD-117-92-Billion-by-2027-Fortune-Business-Insights.html (Accessed January 19, 2022).
194. GRAND VIEW RESEARCH. Omega 3 Market Size (2022). Available at: https://www.grandviewresearch.com/industry-analysis/omega-3-market (Accessed January 19, 2022).
195. MONDOR INTELLIGENCE. Omega-3 Products Market (2022). Available at: https://www.mordorintelligence.com/industry-reports/omega-3-product-market (Accessed January 19, 2022).
196. ALLIED MARKET RESEARCH. Omega-3 Market (2022). Available at: https://www.alliedmarketresearch.com/omega-3-market (Accessed January 19, 2022).
197. FORTUNE BUSINESS INSIGHTS. Food Additives & Ingredients - Omega-3 Fatty Acids Market (2022). Available at: https://www.fortunebusinessinsights.com/industry-reports/omega-3-fatty-acids-market-100248 (Accessed January 19, 2022).
198. AOCS. Omega-3 Fatty Acids: $13 Billion Global Market (2022). Available at: https://www.aocs.org/stay-informed/inform-magazine/featured-articles/omega-3-fatty-acids-13-billion-global-market-october-2011?SSO=True (Accessed January 19, 2022).
199. BUSINESS WIRE. The European Omega-3 Products Market to 2024 - $14.6 Billion Opportunity Analysis Featuring Unilever, Amway Corporation and Nestle (2022). Available at: https://www.businesswire.com/news/home/20190523005353/en/The-European-Omega-3-Products-Market-to-2024—14.6-Billion-Opportunity-Analysis-Featuring-Unilever-Amway-Corporation-and-Nestle—ResearchAndMarkets.com (Accessed January 19, 2022).
200. FRIEND OF THE SEA. Sustainable Omega 3 Consumption: A Positive Trend Set to Increase (2022). Available at: https://friendofthesea.org/it/sustainable-omega-3-consumption-a-positive-trend-set-to-increase/(Accessed January 19, 2022).
201. Hinriksdottir HH, Jonsdottir VL, Sveinsdottir K, Martinsdottir E, Ramel A. Bioavailability of Long-Chain N-3 Fatty Acids From Enriched Meals and From Microencapsulated Powder. Eur J Clin Nutr (2015) 69:344–8. doi: 10.1038/ejcn.2014.250
202. Yurko-Mauro K, Kralovec J, Bailey-Hall E, Smeberg V, Stark JG, Salem N Jr. Similar Eicosapentaenoic Acid and Docosahexaenoic Acid Plasma Levels Achieved With Fish Oil or Krill Oil in a Randomized Double-Blind Four-Week Bioavailability Study. Lipids Health Dis (2015) 14:99. doi: 10.1186/s12944-015-0109-z
203. Ramprasath VR, Eyal I, Zchut S, Jones PJ. Enhanced Increase of Omega-3 Index in Healthy Individuals With Response to 4-Week N-3 Fatty Acid Supplementation From Krill Oil Versus Fish Oil. Lipids Health Dis (2013) 12:178. doi: 10.1186/1476-511X-12-178
204. Ganesan B, Brothersen C, McMahon DJ. Fortification of Foods With Omega-3 Polyunsaturated Fatty Acids. Crit Rev Food Sci Nutr (2014) 54:98–114. doi: 10.1080/10408398.2011.578221
205. Metcalf RG, James MJ, Mantzioris E, Cleland LG. A Practical Approach to Increasing Intakes of N-3 Polyunsaturated Fatty Acids: Use of Novel Foods Enriched With N-3 Fats. Eur J Clin Nutr (2003) 57:1605–12. doi: 10.1038/sj.ejcn.1601731
206. Benvenga S, Trimarchi F. Changed Presentation of Hashimoto's Thyroiditis in North-Eastern Sicily and Calabria (Southern Italy) Based on a 31-Year Experience. Thyroid (2008) 18:429–41. doi: 10.1089/thy.2007.0234
207. Rizzo M, Rossi RT, Bonaffini O, Scisca C, Altavilla G, Calbo L, et al. Increased Annual Frequency of Hashimoto's Thyroiditis Between Years 1988 and 2007 at a Cytological Unit of Sicily. Ann Endocrinol (Paris) (2010) 71:525–34. doi: 10.1016/j.ando.2010.06.006
208. Caturegli P, De Remigis A, Chuang K, Dembele M, Iwama A, Iwama S. Hashimoto's Thyroiditis: Celebrating the Centennial Through the Lens of the Johns Hopkins Hospital Surgical Pathology Records. Thyroid (2013) 23:142–50. doi: 10.1089/thy.2012.0554
209. Miranda-Filho A, Lortet-Tieulent J, Bray F, Cao B, Franceschi S, Vaccarella S, et al. Thyroid Cancer Incidence Trends by Histology in 25 Countries: A Population-Based Study. Lancet Diabetes Endocrinol (2021) 9:225–34. doi: 10.1016/S2213-8587(21)00027-9
210. Kitahara CM, Sosa JA. Understanding the Ever-Changing Incidence of Thyroid Cancer. Nat Rev Endocrinol (2020) 16:617–8. doi: 10.1038/s41574-020-00414-9
211. Cheng F, Xiao J, Shao C, Huang F, Wang L, Ju Y, et al. Burden of Thyroid Cancer From 1990 to 2019 and Projections of Incidence and Mortality Until 2039 in China: Findings From Global Burden of Disease Study. Front Endocrinol (Lausanne) (2021) 12:738213. doi: 10.3389/fendo.2021.738213
212. Lim H, Devesa SS, Sosa JA, Check D, Kitahara CM. Trends in Thyroid Cancer Incidence and Mortality in the United States, 1974-2013. JAMA (2017) 317:1338–48. doi: 10.1001/jama.2017.2719
213. Pellegriti G, Frasca F, Regalbuto C, Squatrito S, Vigneri R. Worldwide Increasing Incidence of Thyroid Cancer: Update on Epidemiology and Risk Factors. J Cancer Epidemiol (2013) 2013:965212. doi: 10.1155/2013/965212
214. Park HT, Cho GJ, Ahn KH, Shin JH, Hong SC, Kim T, et al. Thyroid Stimulating Hormone is Associated With Metabolic Syndrome in Euthyroid Postmenopausal Women. Maturitas (2009) 62:301–5. doi: 10.1016/j.maturitas.2009.01.007
215. He J, Lai Y, Yang J, Yao Y, Li Y, Teng W, et al. The Relationship Between Thyroid Function and Metabolic Syndrome and Its Components: A Cross-Sectional Study in a Chinese Population. Front Endocrinol (Lausanne) (2021) 12:661160. doi: 10.3389/fendo.2021.661160
216. Kalra S, Aggarwal S, Khandelwal D. Thyroid Dysfunction and Dysmetabolic Syndrome: The Need for Enhanced Thyrovigilance Strategies. Int J Endocrinol (2021) 2021:9641846. doi: 10.1155/2021/9641846
217. Tang K, Zhang Q, Peng NC, Zhang M, Xu SJ, Li H, et al. Epidemiology of Metabolic Syndrome and its Components in Chinese Patients With a Range of Thyroid-Stimulating Hormone Concentrations. J Int Med Res (2020) 48:300060520966878. doi: 10.1177/0300060520966878
218. Morini E, Catalano A, Lasco A, Morabito N, Benvenga S. In Thyroxine-Replaced Hypothyroid Postmenopausal Women Under Simultaneous Calcium Supplementation, Switch to Oral Liquid or Softgel Capsule L-Thyroxine Ensures Lower Serum TSH Levels and Favorable Effects on Blood Pressure, Total Cholesterolemia and Glycemia. Endocrine (2019) 65:569–79. doi: 10.1007/s12020-019-01979-w
219. Deshmukh V, Farishta F, Bhole M. Thyroid Dysfunction in Patients With Metabolic Syndrome: A Cross-Sectional, Epidemiological, Pan-India Study. Int J Endocrinol (2018) 2018:2930251. doi: 10.1155/2018/2930251
220. Zhou YC, Fang WH, Kao TW, Wang CC, Chang YW, Peng TC, et al. Exploring the Association Between Thyroid- Stimulating Hormone and Metabolic Syndrome: A Large Population-Based Study. PloS One (2018) 13:e0199209. doi: 10.1371/journal.pone.0199209
221. Janovsky CCPS, Cesena FH, Valente VAT, Conceição RDO, Santos RD, Bittencourt MS. Association Between Thyroid-Stimulating Hormone Levels and Non-Alcoholic Fatty Liver Disease Is Not Independent From Metabolic Syndrome Criteria. Eur Thyroid J (2018) 7:302–7. doi: 10.1159/000492324
222. Hak AE, Pols HA, Visser TJ, Drexhage HA, Hofman A, Witteman JC. Subclinical Hypothyroidism is an Independent Risk Factor for Atherosclerosis and Myocardial Infarction in Elderly Women: The Rotterdam Study. Ann Intern Med (2000) 132:270–8. doi: 10.7326/0003-4819-132-4-200002150-00004
223. Chaker L, Baumgartner C, den Elzen WP, Ikram MA, Blum MR, Collet TH, et al. Subclinical Hypothyroidism and the Risk of Stroke Events and Fatal Stroke: An Individual Participant Data Analysis. J Clin Endocrinol Metab (2015) 100:2181–1291. doi: 10.1210/jc.2015-1438
224. Rodondi N, den Elzen WP, Bauer DC, Cappola AR, Razvi S, Walsh JP, et al. Subclinical Hypothyroidism and the Risk of Coronary Heart Disease and Mortality. JAMA (2010) 304:1365–74. doi: 10.1001/jama.2010.1361
225. Ashizawa K, Imaizumi M, Usa T, Tominaga T, Sera N, Hida A, et al. Metabolic Cardiovascular Disease Risk Factors and Their Clustering in Subclinical Hypothyroidism. Clin Endocrinol (Oxf) (2010) 72:689–95. doi: 10.1111/j.1365-2265.2009.03697.x
226. Singh S, Duggal J, Molnar J, Maldonado F, Barsano CP, Arora R. Impact of Subclinical Thyroid Disorders on Coronary Heart Disease, Cardiovascular and All-Cause Mortality: A Meta-Analysis. Int J Cardiol (2008) 125:41–8. doi: 10.1016/j.ijcard.2007.02.027
227. Siemińska L, Wojciechowska C, Walczak K, Borowski A, Marek B, Nowak M, et al. Associations Between Metabolic Syndrome, Serum Thyrotropin, and Thyroid Antibodies Status in Postmenopausal Women, and the Role of Interleukin-6. Endokrynol Pol (2015) 66:394–403. doi: 10.5603/EP.2015.0049
228. Cengiz H, Demirci T, Varim C, Tamer A. The Effect of Thyroid Autoimmunity on Dyslipidemia in Patients With Euthyroid Hashimoto Thyroiditis. Pak J Med Sci (2021) 37:1365–70. doi: 10.12669/pjms.37.5.3883
229. Wu Y, Shi X, Tang X, Li Y, Tong N, Wang G, et al. The Correlation Between Metabolic Disorders And Tpoab/Tgab: A Cross-Sectional Population-Based Study. Endocr Pract (2020) 26:869–82. doi: 10.4158/EP-2020-0008
230. Chen Y, Zhu C, Chen Y, Wang N, Li Q, Han B, et al. Are Thyroid Autoimmune Diseases Associated With Cardiometabolic Risks in a Population With Normal Thyroid-Stimulating Hormone? Mediators Inflammation (2018) 2018:1856137. doi: 10.1155/2018/1856137
Keywords: fish, nutraceuticals, omega-3 polyunsaturated fatty acids, eicosapentaenoic acid (EPA), docosahexaenoic acid (DHA), autoimmune thyroid diseases, endocrine disruptors, mercury
Citation: Benvenga S, Famà F, Perdichizzi LG, Antonelli A, Brenta G, Vermiglio F and Moleti M (2022) Fish and the Thyroid: A Janus Bifrons Relationship Caused by Pollutants and the Omega-3 Polyunsaturated Fatty Acids. Front. Endocrinol. 13:891233. doi: 10.3389/fendo.2022.891233
Received: 07 March 2022; Accepted: 21 April 2022;
Published: 27 May 2022.
Edited by:
Loredana Pagano, University of Turin, ItalyReviewed by:
Daniela Gallo, University of Insubria, ItalySilvia Martina Ferrari, University of Pisa, Italy
Copyright © 2022 Benvenga, Famà, Perdichizzi, Antonelli, Brenta, Vermiglio and Moleti. This is an open-access article distributed under the terms of the Creative Commons Attribution License (CC BY). The use, distribution or reproduction in other forums is permitted, provided the original author(s) and the copyright owner(s) are credited and that the original publication in this journal is cited, in accordance with accepted academic practice. No use, distribution or reproduction is permitted which does not comply with these terms.
*Correspondence: Fausto Famà, ZmZhbWFAdW5pbWUuaXQ=; ZmFtYWZhdXN0b0B5YWhvby5pdA==