- 1College of Animal Science and Technology, China Agricultural University, Beijing, China
- 2Key Laboratory of Fertility Preservation and Maintenance of Ministry of Education, and Key Laboratory of Reproduction and Genetics of Ningxia Hui Autonomous Region, School of Basic Medical Science, Ningxia Medical University, Yinchuan, China
- 3National Health Commission of China (NHC) Key Laboratory of Human Disease Comparative Medicine, Institute of Laboratory Animal Sciences, Chinese Academy of Medical Sciences and Comparative Medicine Center, Peking Union Medical College, Beijing, China
There are many organochlorine pollutants in the environment, which can be directly or indirectly exposed to by mothers, and as estrogen endocrine disruptors can cause damage to the lactation capacity of the mammary gland. In addition, because breast milk contains a lot of nutrients, it is the most important food source for new-born babies. If mothers are exposed to organochlorine pesticides (OCPs), the lipophilic organochlorine contaminants can accumulate in breast milk fat and be passed to the infant through breast milk. Therefore, it is necessary to investigate organochlorine contaminants in human milk to estimate the health risks of these contaminants to breastfed infants. In addition, toxic substances in the mother can also be passed to the fetus through the placenta, which is also something we need to pay attention to. This article introduces several types of OCPs, such as dichlorodiphenyltrichloroethane (DDT), methoxychlor (MXC), hexachlorocyclohexane (HCH), endosulfan, chlordane, heptachlorand and hexachlorobenzene (HCB), mainly expounds their effects on women’s lactation ability and infant health, and provides reference for maternal and infant health. In addition, some measures and methods for the control of organochlorine pollutants are also described here.
Introduction
Pesticides are one of the most widely used chemicals in the world and are divided into four chemical groups: organochlorine, organophosphate, carbamate and pyrethroid (1, 2). However, approximately 95% of pesticides may adversely affect non-target organisms during the application process (3). Organochlorine pesticides (OCPs) and their me7olites have toxic effects on higher organisms due to the presence of chlorine atoms in the compounds, as well as their low solubility and a tendency to preferentially partition into the lipophilic phase (4). Although some persistent organic pollutants (POPs) were banned or restricted decades ago (5), they are still often found in humans. They are resistant to microbial degradation, and their half-life ranges from several months to several years. They can be transported over long distances, and their presence can even be detected in the Arctic (6). They can biomagnify throughout the food chain and cause high concentrations in top predators including humans (7). OCPs are considered to be endocrine disrupting chemicals (EDCs) that can work at very low doses and are compounds that change hormones and homeostasis systems (8). They interfere with important life processes, such as sexual development, growth and reproduction, and the development of live fetuses (9).
OCPs can enter the human body through the absorption of the respiratory system, digestive system, skin and eyes (10). They are distributed throughout the body through metabolism, excretion and storage processes (11). Human exposure to OCPs, even at low exposure levels, can cause a variety of diseases. In addition to carcinogenic risks, neurotoxicity and genotoxicity, it can also have destructive effects on the endocrine, reproductive and immune systems (3, 12). Due to its lipophilicity and high persistence, OCPs accumulate in lipophilic human body parts, especially in fatty tissues and other lipid-rich tissues (13).
The breast is a hormone-dependent tissue. Its growth and differentiation require many hormones including estradiol, progesterone, and prolactin to coordinate (14–16). During pregnancy and lactation, breasts mainly secrete and store breast milk in response to the complex effects of hormones such as estrogen, progesterone and prolactin. OCPs can cause damage to the mammary glands and also affect the lactation ability of lactating women.
Breast milk provides almost all the necessary nutrients for babies under 6 months of age. It is a complete food that can improve and promote the growth and development of babies (17). About 60% of the lipids in breast milk come from the mother’s adipose tissue. Some OCPs stored in the mother’s adipose tissue will accumulate in breast milk and transfected to the baby through breastfeeding, which brings hidden dangers to the health of the baby (18). In addition, the developing fetus is at risk from OCPs via placental transfer (19). Therefore, breast milk is best used to represent the baby’s exposure to OCPs after birth. OCPs may affect infant anthropometric development (20), gut microbial function (21), and early childhood behaviour (22). OCPs exposure has a negative impact on infants’ neurological function (23). In addition, it has also been found that the OCPs in breast milk are closely related to cryptorchidism in male children (23). Compared with the after milk, the foremilk has a lower fat content. Compared with maternal serum, the content of DDT and HCH in post-milk is 80% higher (23). The lipid-adjusted concentration of OCPs will not continue to decrease during lactation, and there is no significant difference between the organochlorine content in colostrum and the organochlorine content in mature milk samples (24, 25). The residual level of persistent organic pollutants in breast milk may reflect the mother’s physical burden (26–28) and can be used to further examine the relationship between OCPs and maternal health and the possible health risks to breastfeeding offspring (29). Thus, the residue levels of POPs in breast milk and their related health consequences on infants are of high concern (30, 31).
This article reviews several main OCPs in the body fluids of pregnant women, such as dichlorodiphenyltrichloroethane (DDT), methoxychlor (MXC), hexachlorocyclohexane (HCH), endosulfan, chlordane, heptachlorand and hexachlorobenzene (HCB). On the one hand, it starts with the effect of estrogen endocrine disruptors on the mammary glands. On the other hand, it will provide some references for preventing maternal and infant diseases caused by OCPs.
Main types of OCPs
Here we divided OCPs into 4 main sub-groups of OCPs viz: DDT and its analogues, HCH and its isomers, cyclodienes and HCB. Most OCPs can accumulate in breast milk. DDT, HCH and HCB are the main OCPs found in breast milk (32). The following is an introduction to several major OCPs (Table 1).
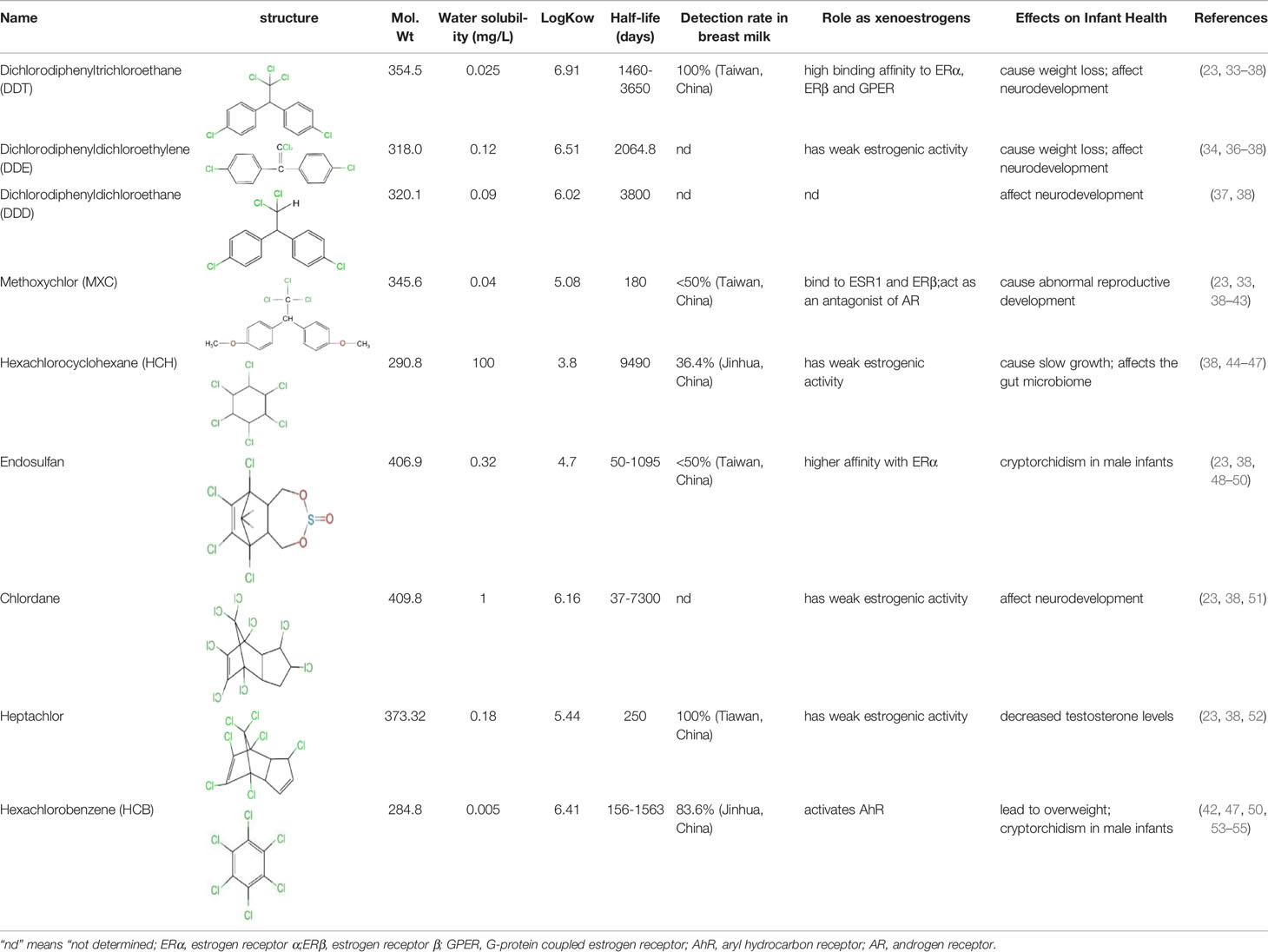
Table 1 Structure, physicochemical properties and biological effects of several organochlorine pesticides.
Dichlorodiphenyltrichloroethane and Its Analogues
DDT and Its Isomers
Dichlorodiphenyltrichloroethane (DDT, 1,1,1-trichloro-2,2-bis(ρ-chlorophenyl) ethane), is a synthetic pesticide widely used for disease-vector control and agriculture. This compound can stimulate the release of gonadotropin-releasing hormones in a manner similar to that induced by estrogen interaction with estrogen receptors (ERs) (56, 57). DDT products usually consist of a mixture with 77% p, p’-isomers, 15% o, p’-isomers and traces of o,o′-DDT. The o, p’-DDT isomer is deemed to be the more estrogenic isomer and has a 100 times higher binding affinity for ERα than p, p’ -DDT (33). Dichlorodiphenyldichloroethylene (DDE) is the main metabolite of DDT and can be considered as an environmental contaminant (58). The half-life of DDT and its main metabolite (DDE) in humans is at least 5 years, the longest can even reach 10 years (34, 35). Humans are primarily exposed to p,p’-DDT and o,p’-DDT through direct exposure to pesticide application and dietary exposure, whereas the source of p,p’-DDE exposure depends on whether p,p’-DDT exposure is effective. In addition, p,p’-DDE can also be directly exposed through diet and other aspects. The higher detectability and levels of p,p’-DDE in humans may be due to the longer half-life of this compound in the environment (36). Dichlorodiphenyldichloroethane (DDD) is also an important metabolite, and DDT can also be reductively dechlorinated to DDD under reducing conditions (59). Reductive dechlorination (RD) is also the main mechanism by which microorganisms convert o,p’-DDT and p,p’-DDT isomers to DDD (4). Effects of DDT include liver and central nervous system toxicity, estrogenic and antiandrogenic effects, and possible carcinogenicity (60). Some epidemiological evidence suggests that DDT and its metabolites exposure increases preterm and small-for-gestational-age births and shortens lactation (61, 62).
(DDT, 1,1,1-trichloro-2,2-bis(ρ‑chlorophenyl) ethane)
Because DDT was banned long ago, MXC emerged as an alternative to DDT (63). MXC, a structural analogy of DDT, has been found in human tissue samples and breast milk (23). Its half-life is shorter than that of DDT, about half a year (38). MXC has a chlorinated double ring structure. It can bind to ER. The purity of MXC has an effect on its binding ability on ER, and there are obvious differences in the binding of MXC with different purities on ER. 95% MXC has a weak affinity for ER, while 99% MXC has no competitive effect on ER (39). Its metabolite (2,2-bis-(p-hydroxyphenyl)-1,1,1-trichloroethane) (HPTE) can bind to ESR1 (Estrogen Receptor 1) and ERβ and has antiestrogenic activity. Also acts as an antagonist of androgen receptor (AR) (40, 41). It adversely affects female fertility, early pregnancy and uterine development (63).
Hexachlorocyclohexane and Its Isomers
Industrial grade HCH is highly stable and resistant to degradation, and has been used to kill parasites such as mosquitoes for malaria or typhoid control for a long time (64, 65). Although these compounds were banned in the 1990s, they are still used in some developing countries. The production of such saturated cyclic compounds leads to the production of several isomers with different spatial arrangements of chlorine atoms around the cyclohexane ring. There are five stable isomers, including α: 60%-70%, β: 5%-12%, γ: 10%-12%, δ: 6%-10% and ϵ: 3%-4% (66). HCH can persist in the environment for a long time, for example, the predicted half-life of γ-HCH in water is 191 days (44). The average half-life of γ- and α-HCH around the Great Lakes region is about 3 to 4 years (38). It was later found that only γ-HCH (also known as lindane) has insecticidal activity (67). Lindane production process is very inefficient, producing 1 ton of lindane produces about 8-12 tons of HCH waste consisting of α-, β-, δ- and ϵ-isomers (68). Although only the γ-HCH is insecticidal, industrial grade HCH is widely used as an inexpensive and effective insecticide in developing countries (67).
In the environment, some bacteria can slowly isomerize lindane into α-, β- and δ-HCH, mainly under anaerobic conditions (69). The α-, β- and δ- isomers are in many ways more problematic than lindane itself. Of all isomers, β-HCH was noted most commonly, which is a result of its high stability and persistence. The β-isomer is usually present in higher concentrations than those of α- and γ-HCH, which metabolize into β-HCH in the human body (66). Among the isomeric forms of HCH, β-HCH has a more stubborn chemical structure, which makes it more resistant to biodegradation. Once ingested or inhaled, it leaves the body very slowly due to its higher lipophilicity. Therefore, in the case of long-term exposure, β-HCH is more toxic than the other isomers and has a significant adverse effect on fetal growth (70).
Cyclodienes
Endosulfan
Endosulfan (6,7,8,9,10,10-hexachloro-1,5,5a,6,9,9ahexahydro-6,9-methano-2,4,3-benzodioxathiepin-3-oxide) consists of α- and β-isomers in a ratio of approximately 7:3 (42, 71). This insecticide is used to control pests on fruits, vegetables and tea, as well as non-food crops such as tobacco and cotton. It is also used as a wood preservative. Because of its high efficiency, low cost and environmental stability, it is widely used mainly in developing countries (71). Endosulfan is semi-volatile and relatively persistent. It has a half-life of 6 years. Once endosulfan is released into the environment, it undergoes a transformation process that regulates its presence in soil, sediment, water, and biota (48). In addition, he also has a higher affinity with ERα (49), long-term effects on female fertility and differentiation of uterine function in early pregnancy (71).
Chlordane
Chlordane is a cyclopentadiene-derived insecticide that was once used to control termites and borers. Industrial chlordane is a complex mixture of structurally related chemicals, including cis-chlordane, trans-chlordane, and heptachlor (72). Due to its toxicity and persistence, it can remain in the soil for up to 20 years, so chlordane and its related compounds can still be detected in the environment (51). Chlordane initially accumulates in the liver and kidneys and then redistributes to adipose tissue (73). Chlordane has toxic effects on the nervous system, causing headaches, confusion, convulsions, and even fatal symptoms (74).
Many cyclopentadiene pesticides are chiral and exist as non-superimposable mirror-image pairs of enantiomers (72, 75). Chlordane mixtures are racemic (they contain two pairs of each chiral component 1:1 mixture of enantiomers). Chiral organochlorine compounds can cause enantioselective and even enantiospecific biological effects (76, 77).
Heptachlor
Chlorinated cyclodiene heptachlor was registered in 1952 as an agricultural and household insecticide, mainly for the control of termites and fire ants (78). In vivo studies have shown that heptachloro epoxide is the major metabolite. In just a few hours, about 20% of the heptachlor in the body is degraded to epoxy heptachlor. Formed as a product of a mixed-function oxidase system, 1-hydroxychloride is the major soil metabolite (78). In breast milk, higher levels of heptachlor residues are present. Heptachlor was detected in 100% of 55 breast milk samples in a study in southern Taiwan (23). Heptachlor exposure has been found to be associated with neurodegenerative and neurobehavioral disorders such as Parkinson’s disease (79) and depression (80).
Hexachlorobenzene
HCBs are widely present in the environment around us, mainly used as a fungicide. At present, HCB is no longer used as a fungicide, but it is a by-product produced in the commercial chlorination process (81). It adheres strongly to soil and degrades very slowly (53). It is poorly soluble in water, and once there, it accumulates in sediments (54). Its half-life is about 2 years in air and 6 years in water and sediments (82). The main route of exposure of the general population to HCB is through foods such as fatty fish. HCB is a hormone disruptor as a weak ligand for dioxin-like compounds and the aryl hydrocarbon receptor (AhR) (83). At the molecular level, HCB first activates the AhR. Subsequently, AhR will form heterodimerization with AhR nuclear transporter (ARNt) and bind to specific gene regulatory sequences of xenobiotic response element (XRE) (42, 84). There are many adverse effects of long-term exposure to HCB, including neurological symptoms, immune disorders and thyroid dysfunction (82).
Factors Influencing the Level of Organochlorine Pesticides in Breast Milk
The pollution rate of humans mainly depends on individual exposure and accumulation, and is affected by local soil and air pollution, diet, exposure duration, age, metabolic elimination ability, and breast milk production (13, 85) (Figure 1).
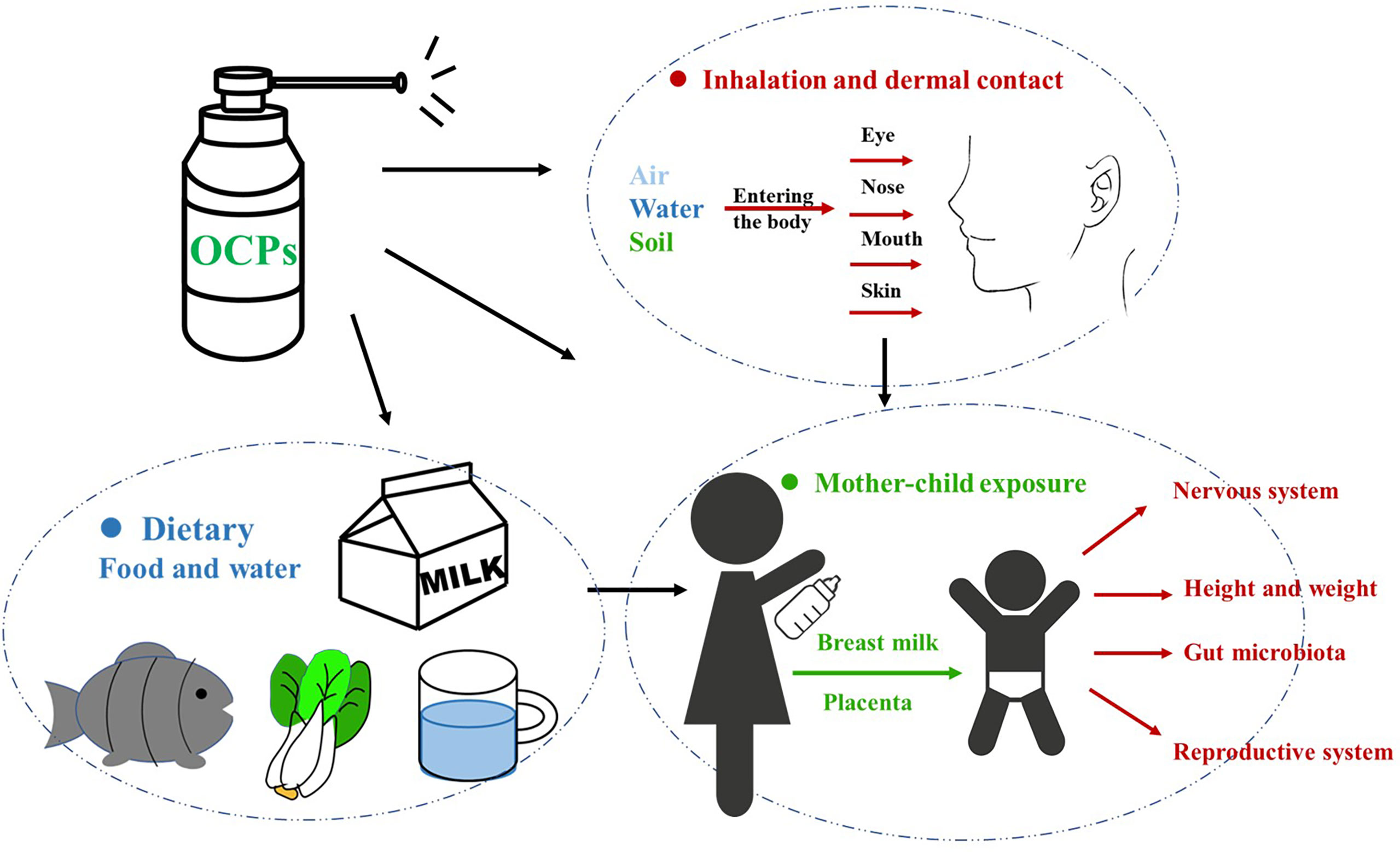
Figure 1 Mothers before and after pregnancy can be directly or indirectly exposed to OCPs, which are passed to the fetus/infants through the placenta/breast milk, causing damage to the growth and development of the babies. OCPs, organochlorine pesticides.
Food
Eating habit is an important factor that affects the level of OCPs in breast milk and women’s related risks. Dietary exposure accounts for more than 90% of the total body burden of organochlorines (32). OCPs tend to slowly bioaccumulate in the food chain, such that they are eventually ingested by women and enriched in adipose tissues; in these tissues, OCPs can persist for a long period of time (86). The highest residues of these compounds are found in fish, meat, poultry, eggs, milk and dairy products, as well as vegetable oils, nuts, avocados, sesame or olives (87–89). For example, fruits and grains are the main sources of DDE (90). The level of DDT in breast milk is closely related to the consumption of animal-derived food and aquatic food (91). Of these, bioaccumulation of organic compounds in fish and other animals and their products (meat and dairy) contributes to substantial exposure to OCPs in humans through ingestion due to their high fat solubility (92). It has been observed that vegetarians (i.e. those who consume vegetables, fruits and grains without animal products) have significantly lower levels of oral contraceptives compared to those who consume animal products (93). However, it is not to say that vegetables do not cause the accumulation of OCPs. In one study, food preferences in vegetables were found to be correlated with HCB, HCHs (ΣHCHs, β-HCH, γ-HCH), p,p’-DDE and heptachlor (Σ heptachlor and trans-heptachlor epoxides) in breast milk concentrations were significantly positively correlated (94). In addition to contamination of dairy products through the animals themselves, a major source of dairy contamination may be the presence of OCPs such as HCH and DDT in dry and green feeds (19).Untreated agricultural wastewater (including pesticides, etc.) released into the water may cause the accumulation of organochlorines, leading to pollution of the aquatic ecosystem (95). At the same time, it also led to the pollution of aquatic organisms such as fish and shrimp. Humans feed on these, and as the food chain accumulates, they are ultimately the most harmed by OCPs. Fish consumption has been found to be an important factor leading to elevated OCP levels in human breast milk. β-HCH, δ-HCH, p’-DDT, p’-endosulfan, HCB, p,p’-DDT, cis-chlordane and methoxychlor in breast milk of fish-eating mothers are generally higher than that of mothers who do not eat fish (32). Studies have also found that heat treatment of fish, especially grilling, can reduce the level of OCPs residues in fish (95). The reason for this may be that the heat treatment causes changes in the lipid content of fish meat, which eventually leads to a decrease in OCP levels.
Region
Residents of developed countries (North America and Western Europe) have lower levels of OCPs in breast milk than those of developing countries, which may reflect differences in exposure (96). The reason for this may be that OCPs have long been banned in most developed countries, but they are still widely used in many third world countries because they are cheap and readily available. Or the time of ban in developing countries is later than that in developed countries. It was found heptachlor-epoxide isomer B in breast milk collected between the second and eighth weeks of lactation in samples from rural and urban Australia. The average lipid of samples from rural areas was 16.7 ng/g lipids, while the average in samples from urban areas was 2.21 ng/g lipids (69, 97). This may be related to the use of pesticides in rural areas. In two surveys of the levels of persistent OCPs in breast milk of Chinese mothers, significant differences were found between rural and urban areas in 1998, while the results in 2011 showed little difference. Presumably due to the rapid urbanization and industrialization of rural areas, this difference has been reduced (98, 99). However, some studies have found that the place of residence has nothing to do with the concentration of DDT or HCH in breast milk (69). Studies have also found that mothers who work or live near industrial plants or where factories used to be more susceptible to contamination (100).
Physiological Factors
Some studies have found that the concentration of OCPs (DDT, diphenylether, HCB, βHCH, dieldrin and MXC, etc) in breast milk increases with the mother’s age (30, 101), but some studies have not found this situation (69). Older mothers, who have longer lifetime exposures, may transfer more OCPs to their first infants through breastfeeding than mothers of younger maternal age. Therefore, first births from older mothers may be at higher risk for OCPs (102). The increase in the concentration of OCPs with age may be caused by eating habits. On the other hand, pesticides are resistant to metabolic processes in the body, and they will bioaccumulate with age (103, 104). And this lack of correlation may be due to the short exposure period or the disappearance of these organics in the environment (69). Because the breast milk of prenatally exposed mothers may have higher levels of organochlorine, prenatal exposure is probably the most critical window of exposure, more critical than any other period of postnatal life (105). Studies have found that women’s weight before and after pregnancy affects changes in the concentration of organochlorines in breast milk (101, 106), residues in breast milk are due to accumulation of fat. A study found that women with low gestational weight gain retained higher levels of contaminants in their colostrum (107). This is because increases in body weight and fat have the effect of diluting OCPs concentrations (107). In addition, insufficient levels of maternal fat may lead to higher rates of mobilization of maternal fat stores in the last trimester of pregnancy (107), which may trigger the release of oral contraceptives into the bloodstream and ultimately into colostrum and breast milk. The colostrum of low gestational weight mothers transmits more contaminants to the baby (108). Some OCPs, such as cis- Chlordane and γ-HCH, are also related to menstrual characteristics, hormone intake, and treatment of infertility (109). Women with irregular menstrual cycles have observed higher levels of dioxins and polybrominated diphenyl ethers (110, 111). This also shows that OCPs may interfere with hormonal balance, thereby destroying menstrual characteristics. Due to differences in metabolism between individuals, large inter-individual differences in pesticide concentrations in breast milk were also found (50).
The Health Effect of OCPs on Mother and Baby
The Effect of OCPs on Lactation
OCPs, including DDE, interfere with the mother’s ability to lactate, possibly because of its estrogenic properties (112). In women, the endocrine system is responsible for hormonal balance and reproductive potential; chemicals that share similar structures with estrogen, act as agonists/antagonists of ERs, or induce ER-mediated signalling can be considered estrogen endocrine disruptors (EEDs, also called xenoestrogens). The expression of ERα and ERβ was found in both mammary epithelial cells and mesenchymal cells. ERα is essential for mammary gland development and lactation; ERβ is more involved in lobular acinar development (113). Because of its aromatic A ring and C3-hydroxyl that provide properties of both hydrogen-bond donor and acceptor, 17β-estradiol (E2) can interact with Arg394 and Glu353 in ERα or with Arg346 and Glu305 in ERβ (114, 115). Moreover, unlike testosterone, E2 lacks a C19 methyl group, which ensures that the interface between ring A and B is flat, resulting in closer contact with ERs. The C17-hydroxyl on the D ring is of equal importance during binding of E2 to ERs; it exhibits stable contact with His524 in ERα and His475 in Erβ (114, 116, 117). Presumably, chemicals containing two rings properly spaced (i.e., in the manner present in E2) can bind to ERs and disrupt normal estrogenic physiology. But in most cases the relative binding affinity (RBA) was at least 1000-fold lower than that of E2 (118). While most OCPs are generally classified as “weak” estrogens, they act “additively” to endogenous estrogens, and when ingested in sufficient quantities, they can affect the endocrine system (119, 120). OCPs can act as estrogen ligands and cause damage to female reproductive physiology, especially lactation. During early pregnancy, the ductal system dilates into adipose tissue in response to an increase in estrogen. Estrogen also stimulates the pituitary gland, leading to increased prolactin levels (121).. Later in pregnancy, the mammary glands are fully developed to produce milk due to prolactin stimulation. High levels of circulating prolactin and estrogen can increase the surface area of the glands and ducts in the breast, impairing milk synthesis. Only at term, and when estrogen begins to decline, does prolactin begin to boost milk synthesis. Weak but persistent estrogen analogs like OCPs such as DDE interfere with milk synthesis, causing early weaning (122). In addition, OCPs can compete for prolactin receptors (PRLR), thereby inhibiting the lactation effect of PRL.
Prolactin (PRL) is generally regarded as a pituitary hormone that stimulates and maintains milk secretion (123). Specifically, PRL induces cell proliferation by activating the cyclin D1 promoter through the JAK2/STAT pathway, promoting cell growth and differentiation of alveolar cells in the mammary gland (124). The secretion of PRL is also related to cell proliferation, cellular immune response and hormonal regulation of parental behavior (125–127). Excessive stimulation or inappropriate stimulation at the developmental stage or reproductive cycle stage may inhibit the secretion of PRL, thereby destroying normal reproductive function (128). The rapid response to EED involves the mobilization of various second messengers, such as cyclic adenosine monophosphate (cAMP) and calcium ions (Ca2+), and G-protein coupled estrogen receptor (GPER) can mediate these rapid effects to activate various signaling cascades (129). The response of Ca2+ to extracellular stimuli can lead to cell movement, intracellular and extracellular signal transduction processes, and rapid secretion of hormones through exocytosis, among which prolactin can be secreted in this way (130) Studies have found that several OCPs, including DDE and endosulfan, can quickly induce Ca2+ influx at very low concentrations (picomolar to nanomolar), leading to PRL secretion and undermining endocrine function (128). Studies have found that HCB treatment significantly reduces plasma prolactin concentrations, which may lead to reproductive changes. But it cannot be determined whether HCB directly affects the glands or reduces the release of prolactin from the pituitary gland by changing the concentration of dopamine (131). In addition, the level of oxytocin has a certain effect on new-born babies. Some studies have also found that new-borns with lower levels of prolactin in cord blood have an increased risk of respiratory distress syndrome than new-borns with higher levels of prolactin (132, 133).
Gonadotropin-releasing hormone (GnRh) is a neurohormone secreted by the hypothalamus that regulates reproduction in both sexes through the secretion of pituitary gonadotropins. Expression of GnRH and its receptors has also been found in various peripheral tissues (134). GnRH can promote the pituitary gland to secrete luteinizing hormone (LH), follicle-stimulating hormone (FSH) and prolactin (PRL). Prolactin acts on the mammary gland to promote mammary gland development and milk production. ANXA5 is a member of the annexin family of proteins, grouped by its structural similarity and affinity to calcium and phospholipids (135, 136). ANXA5 is thought to be involved in GnRH receptor signaling (136), and reduction of PRL increases GnRH in breast tissue, leading to ANXA5 expression, which enhances epithelial cell apoptosis and reduces milk production and weight (135).
Another target pathway by which OCPs affect lactation is through activation of the AhR. Activation of AhR, an environment-aware transcription factor, leads to severely impaired mammary gland differentiation during pregnancy, including reduced ductal branching, poor alveolar structure formation, blocked milk protein expression, and impaired lactation capacity (137). E-cadherin is a cell adhesion molecule that is directly involved in the development and differentiation of epithelial cells in various tissues including the mammary gland (138). In late pregnancy, activation of AhR reduces the expression level of E-cadherin in mammary cells, thereby affecting mammary gland development. Defects in mammary gland differentiation and lactogenesis result from direct effects on mammary epithelial cells (MECs). AhR-mediated reduction of cyclin D1 provides a possible mechanism for reducing pregnancy-related MEC proliferation (137) (Figure 2).
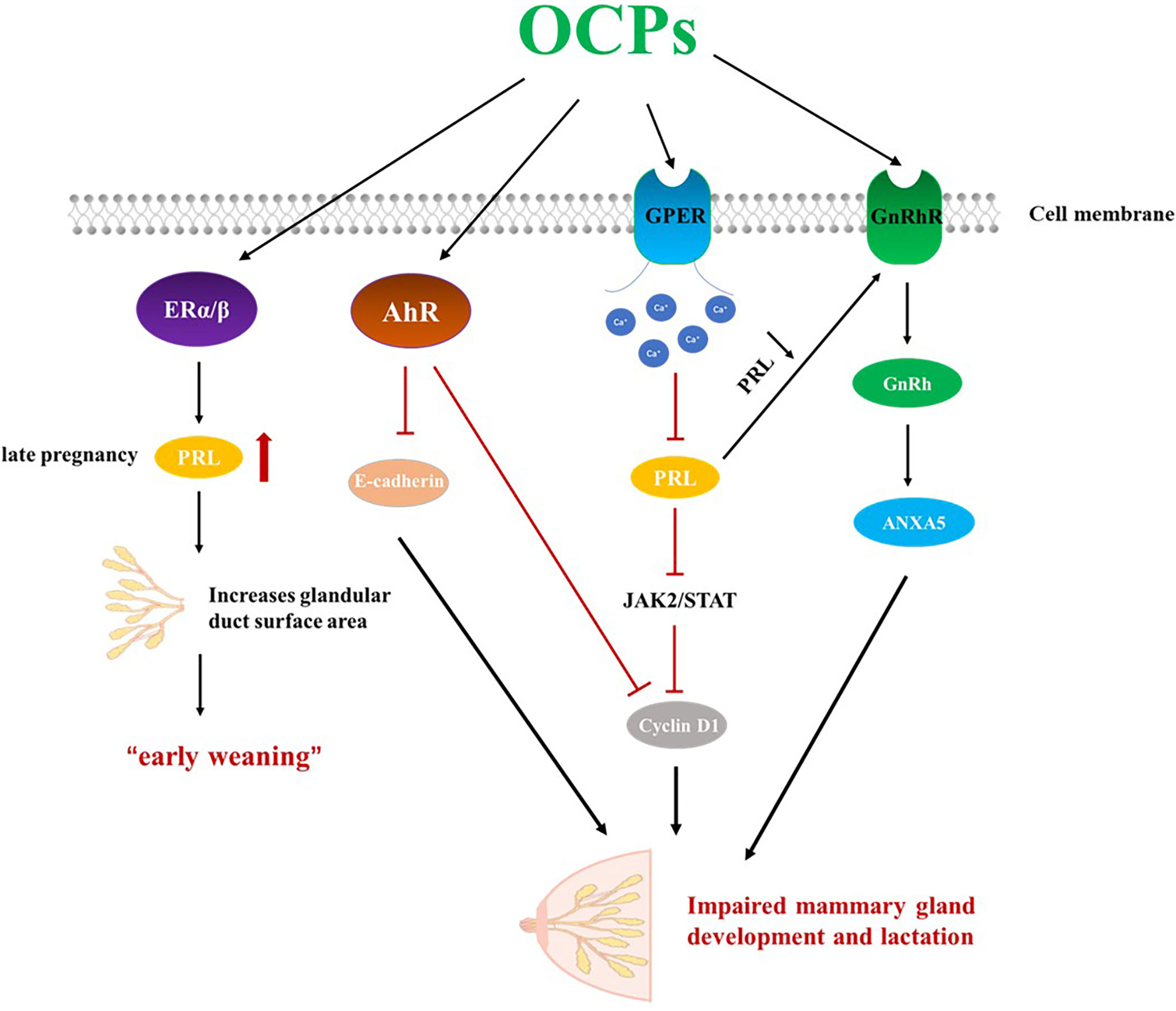
Figure 2 OCPs can act as estrogen endocrine disruptors and impair lactation. OCPs, organochlorine pesticides; ERα, estrogen receptors α; ERβ, estrogen receptors β; GPER, G-protein coupled estrogen receptor; AhR, aryl hydrocarbon receptor; GnRhR, Gonadotropin-releasing hormone receptor; GnRh, Gonadotropin-releasing hormone; PRL, Prolactin.
Effects of OCPs in Breast Milk on Infants
Some evidence suggests that fetuses and infants may be more sensitive to OCPs than adults (139). Because children’s metabolic pathways are immature, their detoxification is much poorer than in adults, especially during the fetal period and the first year of life. Additionally, developmental processes during these periods are also more susceptible to disruption due to their higher body surface area relative to their body size (140). In general, OCPs with molecular weight <800 D present in plasma enter breast tissue by passive transport (141). Early life exposure through breastfeeding significantly increases the physical burden of oral contraceptives in children and is thought to be a major determinant of blood levels in children before age 7 years (25, 142) (Figure 1).
Effects on Neonatal Growth and Development
Breastfeeding is very important for the health effects of postpartum exposure to infants. OCPs will affect the growth and development of babies. In Norway, there is an association between an increase in HCB in breast milk and a decrease in birth weight (BW), crown heel length (CHL) and head circumference (HC) (143). There is a correlation between higher β-HCH in female breast milk and lower bio-organic weight (144), Early exposure to β-HCH is also associated with slower growth (45). In addition, residual DDT in breast milk also has a significant impact on fetal growth (BW, CHL, HC and CC) (20). OCPs are associated with lipogenesis and weight gain, and they may increase the risk of childhood obesity (145). Prenatal exposure to HCB increases the risk of being overweight in children under the age of 6 (55). OCPs in breast milk were positively associated with rapid growth and obesity in the first 6 months of life (146). However, studies have also found that o,p’-DDT and p,p’-DDE are associated with decreased birth weight at a given p,p’-DDT exposure level (36).
Effects on the Development of the Nervous System
Exposure to OCPs in breast milk negatively affects infants’ neurological function. At present, the exact mechanism of the negative effects of EDCs such as OCPs on the neurodevelopment of infants and young children is unclear. However, several possible mechanisms can be proposed. First, the neurotransmitter γ-aminobutyric acid (GABA) is a key mediator of neural development. It acts on all neuron types through GABA receptors located on adjacent neurons to promote neurogenesis, neuronal migration, and synapse formation, among others (147). The primary goal of OCPs such as endosulfan is to inhibit GABA receptors, which can affect multiple aspects of neuronal circuits in the frontal cortex, resulting in impaired cognitive function and behavioral deficits (148). In addition, OCPs exposure disrupts thyroid hormones with adverse consequences on developing brain nerves (149). Finally, disruption of calcium signalling, activation of peroxisome proliferator-activated receptors (PPARs), and lipid metabolism can also be used to explain the adverse neurodevelopmental effects of some OCPs (150). DDT and its metabolites DDE and DDD will pass through the placenta and contaminate breast milk, causing toxicity related to infant neurodevelopment (37). Studies have found in samples of breast milk consumed by 18-month-old infants that high levels of chlordecone can cause neuronal damage, such as poor fine motor scores in boys (151). After adjusting for confounding factors such as maternal age, pre-pregnancy body mass index (BMI), and baby gender, Kao et al. (23) used a logistic regression model and found that the breast milk levels of 4,4′-DDT were associated with cognition and language, while trans-Chlordane were associated with socioemotional scores. Significantly negative correlation. In addition, the amount and duration of breastfeeding may be a covariate related to infant neurodevelopment when breastfed infants are exposed to OCPs postpartum (23). studies have shown that exposure to heptachlor is associated with disruption of the dopamine system (152). A study in Japan also found a significant negative association between low prenatal exposure to cis-heptachloroepoxide and Mental Development Index (MDI) at 18 months of age (153). In addition, heptachloroepoxide had the highest negative correlation with the adaptive behavior scale (23).
Effects on the Gut Microbiome
The gut microbiota (GM) is essential for the development and maturation of the gastrointestinal tract (154, 155). Early disruption of microbial communities may have lifelong health consequences. The developing infant’s gut microbiome is directly exposed to OCPs-contaminated breast milk. There are many studies on whether OCPs in breast milk affect the composition and function of the infant’s gut microbiome (21, 156). HCH alters the colonization of the infant gut by altering the microbial composition in human colostrum (46). In addition, studies investigating whether environmental pollutants (including organochlorines) in breast milk affect the homeostasis of the infant gut microbiota at one month revealed differences in the abundance of some Firmicutes strains (21).
Effects on Reproductive System Development
As an endocrine disruptor, OCPs can adversely affect the reproductive system of infants, which has been demonstrated in many studies (157, 158). Breast tissue is not fully developed at birth, so hormones are critical for its development at this stage (159). Furthermore, cells are in a state of rapid growth and differentiation during this period. Exposure to OCPs at this stage may increase breast cancer incidence (160). Early exposure to OCPs also affected normal prostate function and structure, with a notable combination being DDT and its metabolite DDE. Low amounts of DDT were able to inhibit PSA at the mRNA and protein levels (161). Congenital cryptorchidism is a genital malformation disorder that occurs in newborn boys and is associated with decreased semen quality and an increased risk of testicular cancer (162). There is an association between congenital cryptorchidism and some persistent OCPs(p,p′-DDT, p,p′-DDE, p,p′-DDD, o,p′-DDT, HCH (α, β, γ), HCB, α -endosulfan)present in the mother’s breast milk, which can adversely affect testicular descent in boys (50, 163).
Effects of OCP on the Baby Through the Placenta
Fetal exposure to OCPs and other POPs is primarily through placental transfusion (99). The placenta is an important barrier for fetal protection during pregnancy. The placenta produces human chorionic gonadotropin (hCG), which is involved in the exchange of gases, nutrients, and waste between mother and fetus (164). Estimated levels of OCPs in breast milk, placenta, and fetal tissue were 16.7, 10.1, and 5.3 (ng/kg lipid) (66, 165). Despite the lower burden, transplacental exposure of the developing fetus remains important for the child’s physical development and cognitive functions, even more so than postpartum exposure through breast milk (166). Organic chloride pollutants have high affinity for hydrocarbon (Ah) receptors (167). A combination of strongly induced cytochrome P4501A1 (CYP1A1) genes encoding cytochrome P-450 1A1 enzymes involved in the metabolism of organochlorines (168). The metabolism of organochlorine in the body produces a large amount of reactive oxygen species, and the antioxidant system cannot eliminate these reactive oxygen species, thereby damaging the DNA chain and affecting mitochondrial function. CYP1A1 is involved in human placental metabolism; abnormal expression of this enzyme may disrupt placental detoxification mechanisms (164, 169). During pregnancy, OCPs can enter the maternal circulation and reach the placenta (170). These substances can interfere with the placenta, such as the production and release of hormones and enzymes, the transport of nutrients, and the production of waste, and then disrupt fetal development and the final stages of placental life (171). Higher concentrations of β-HCH in cord blood increase the risk of preterm birth. In addition, researchers noted that serum γ-HCH levels were positively associated with habitual miscarriage in women (172, 173). MXC is thought to be a testosterone trigger, and exposure to OCPs during pregnancy may disrupt maternal hormones that regulate offspring sex, resulting in higher rates of boy production (2, 174). Furthermore, MXC crosses the placenta and induces abnormal reproductive development (43). Prenatal exposure to alpha endosulfan and heptachlor increases estradiol and sex hormone binding globulin (SHBG), thereby reducing testosterone levels in infants at birth (52).
The Method of Prevention
Overall, in the past 30 years, OCPs in breast milk have decreased in many countries. For example, in the case of DDT, since the 1990s, lipids have dropped from 2000-32,000 ng/g lipid to 0.1 ng/g lipid (69). However, the harm of OCPs to mothers and babies is still very serious, and it is very necessary to find suitable prevention methods.
At present, although most countries and regions have explicitly banned the use of OCPs, in some developing countries, such as African, Asian and Latin American countries, there is no or only partial control, and polychlorinated biphenyls and other chemicals can still be found. Concentrations are high (175). The use of pesticides can be dealt with from a socio-economic perspective, and an explicit prohibition can be made, if necessary, which is the most fundamental means to solve the problem of OCPs pollution. Since OCPs are difficult to degrade, they still circulate in water, sediment and soil, affecting the health and safety of humans, especially mothers and infants.
The governance of OCPs in environments such as water and soil is necessary. Numerous techniques are available to treat existing organochlorine compounds in the environment, involving physical, chemical, and biological methods such as adsorption, oxidation, catalytic degradation, membrane filtration, and biological treatment (38). Biological treatment is an ideal approach, but due to the stubborn structure of OCPs, few specific bacterial and fungal species can degrade them, and finding them is cumbersome (176, 177). Currently, many researchers focus on the development and integration of electrochemical oxidation techniques. The main advantages of this approach are that no chemicals or other products need to be added, and the process can be easily connected to renewable energy sources (178). However, when dealing with large volumes of wastewater, the size of equipment and devices increases with energy consumption and waste generation, in addition to unavoidable side reactions and mass transfer limitations (179, 180). The use of nanoparticles to remove this type of contaminant has also been well studied (38). Lindane was removed from aqueous solutions using FeS nanoparticles stabilized by biopolymers (181). In addition, most nanomaterials are semiconductive in nature and can easily photocatalyzed the degradation of OCPs. The conditions of the photodegradation process simulate real environmental conditions and can be applied to soils on a large scale.
Soybean isoflavones are a kind of plant estrogen, extracted in soybean, mainly including genistein and daidzein. There is an interaction effect between OCPs and isoflavones (182). Several studies suggest that flavonoids such as soy may be added to infant supplements as protective molecules to counteract the effects of OCPs in infants (161). However, studies have found that genistein mixed with common pollutants (DDT, DDE, endosulfan) can affect the mammary gland (183). Conversely, prepubertal exposure to genistein prevents the development of breast tumors (184). Melatonin (N-acetyl-5-methoxytryptamine), which protects against cellular damage caused by reactive oxygen species (185), has some preventive functions. Studies have found that melatonin reduces lesions in epithelial compartments, tubular atrophy and vascular congestion (186), and has a protective function in the prostate. Green tea is a non-oxidized and non-fermented form of tea that contains several polyphenols, including green tea catechins. In preclinical studies, green tea extract and polyphenols have been shown to reduce the toxicity of DDT (187).
In addition, several studies have shown that cooking food in different forms can effectively reduce the content of organochlorine. Research on animal meat samples such as cows, goats and chickens. Among the raw meats, beef samples were found to have the highest levels of contamination and chicken samples to have the lowest levels of contamination. Subsequent decontamination studies have shown that cooking is the best option for reducing pesticide levels in raw meat samples. Cooked chicken is the safest food to eat (188). Heat-treated fish can also effectively reduce the level of OCPs residues (95). Milk is an ideal solvent for fat-soluble OCPs. Comparing the levels of OCPs in fresh raw milk and pasteurized milk, the levels found in raw milk were higher than pasteurized milk, and the results verified the efficient effect of heat treatment on the degradation of OCPs (189).
Finally, Information and education can be provided to the public to minimize exposure to possible OCPs, educate and awareness among farmers about the harmful effects of OCPs and how to handle them properly, and use less harmful or non-hazardous alternatives where possible.
Conclusion
This review details several OCPs, including DDT, HCH, HCB, Cyclodienes, etc. Today, people can be directly or indirectly exposed to OCP through a variety of routes including skin, eyes, nose, mouth, and more. Among them, diet is the most important influencing factor. The negative impact of OCPs toxicity on maternal and infant health is a serious issue that must be addressed. First, OCPs is a type of EED with weak estrogenic activity, and mothers exposed to OCPs before and after pregnancy will cause their accumulation in the body and affect lactation. In addition, OCPs can affect fetal development through the placenta. OCPs can also accumulate in the fat-rich mammary gland, and breast milk is the main source of nutrition for newborns. Transfection of OCPs to infants through breastfeeding brings hidden dangers to the health of infants, including: effects on their height, weight, Effects on the development of the nervous system, effects on the composition of the intestinal flora, effects on the development of the reproductive system, etc. As the most important developmental stage in life, babies should be protected from all toxic and harmful substances. The effects of OCPs on the mother through the mammary gland and on the infant/fetus through breast milk/placenta may raise concerns about how to reduce the health damage of OCPs during pregnancy and the postpartum period and encourage early preventive measures. At present, many methods have been studied for the treatment of OCPs, including the treatment of residual OCPs in water, soil and other environments through various physical, chemical and biological methods. It is also possible to relieve residual OCPs in the body by eating some beneficial foods. Food is the main way that mothers/infants are exposed to OCPs, and heat treatment of them can help reduce the harm of OCPs. However, these methods have their own advantages and disadvantages, and more perfect methods need to be explored to solve this problem. Also, I think public awareness should be raised about the harmful effects of OCPs and how to properly handle them, and where possible use less harmful or harmless alternatives, especially in some developing countries. Further research on OCPs is currently underway, but there are still many unknowns about the mechanism of action of OCPs. We hope this brief review will inform mothers and children health and disease and how to properly manage OCPs.
Authors Contributions
S-LD conceptualization, S-YQ and X-LX writing original draft preparation, KY and S-LD editing technical review, S-YQ and X-LX visualization, W-ZM and Z-XL supervision. All authors have read and agreed to the published version of the manuscript.
Funding
This work was supported by National Natural Science Foundation of China (32072722, 32072721, 81860266) and National Transgenic Creature Breeding Grand Project (2016zx08008-003).
Conflict of Interest
The authors declare that the research was conducted in the absence of any commercial or financial relationships that could be construed as a potential conflict of interest.
Publisher’s Note
All claims expressed in this article are solely those of the authors and do not necessarily represent those of their affiliated organizations, or those of the publisher, the editors and the reviewers. Any product that may be evaluated in this article, or claim that may be made by its manufacturer, is not guaranteed or endorsed by the publisher.
References
1. Kass L, Gomez AL, Altamirano GA. Relationship Between Agrochemical Compounds and Mammary Gland Development and Breast Cancer. Mol Cell Endocrinol (2020) 508:110789. doi: 10.1016/j.mce.2020.110789
2. Hamid ERA, Sharaf NE, Ahmed HH, Ahmed A, Mossa ATH. In Utero Exposure to Organochlorine Pesticide Residues and Their Potential Impact on Birth Outcomes and Fetal Gender. Environ Sci Pollut R (2020) 27:33703–11. doi: 10.1007/s11356-020-09411-x
3. Kuang L, Hou Y, Huang F, Hong H, Sun H, Deng W, et al. Pesticide Residues in Breast Milk and the Associated Risk Assessment: A Review Focused on China. Sci Total Environ (2020) 727:138412. doi: 10.1016/j.scitotenv.2020.138412
4. Sudharshan S, Naidu R, Mallavarapu M, Bolan N. DDT Remediation in Contaminated Soils: A Review of Recent Studies. Biodegradation (2012) 23:851–63. doi: 10.1007/s10532-012-9575-4
5. Hung H, Katsoyiannis AA, Guardans R. Ten Years of Global Monitoring Under the Stockholm Convention on Persistent Organic Pollutants (POPs): Trends, Sources and Transport Modelling Preface. Environ Pollut (2016) 217:1–3. doi: 10.1016/j.envpol.2016.05.035
6. Dietz R, Riget FF, Sonne C, Born EW, Bechshoft T, McKinney MA, et al. Three Decades (1983-2010) of Contaminant Trends in East Greenland Polar Bears (Ursus Maritimus). Part 1: Legacy Organochlorine Contaminants. Environ Int (2013) 59:485–93. doi: 10.1016/j.envint.2012.09.004
7. Govaerts A, Verhaert V, Covaci A, Jaspers VLB, Berg OK, Addo-Bediako A, et al. Distribution and Bioaccumulation of POPs and Mercury in the Ga-Selati River (South Africa) and the Rivers Gudbrandsdalslagen and Rena (Norway). Environ Int (2018) 121:1319–30. doi: 10.1016/j.envint.2018.10.058
8. Kavlock RJ, Daston GP, DeRosa C, Fenner-Crisp P, Gray LE, Kaattari S, et al. Research Needs for the Risk Assessment of Health and Environmental Effects of Endocrine Disruptors: A Report of the U.S. EPA-Sponsored Workshop. Environ Health Perspect (1996) 104 Suppl 4:715–40. doi: 10.1289/ehp.96104s4715
9. Kabir ER, Rahman MS, Rahman IA. Review on Endocrine Disruptors and Their Possible Impacts on Human Health. Environ Toxicol Phar (2015) 40:241–58. doi: 10.1016/j.etap.2015.06.009
10. Kim KH, Kabir E, Jahan SA. Exposure to Pesticides and the Associated Human Health Effects. Sci Total Environ (2017) 575:525–35. doi: 10.1016/j.scitotenv.2016.09.009
11. Needham LL, Patterson DG Jr., Barr DB, Grainger J, Calafat AM. Uses of Speciation Techniques in Biomonitoring for Assessing Human Exposure to Organic Environmental Chemicals. Anal Bioanal Chem (2005) 381:397–404. doi: 10.1007/s00216-004-2975-5
12. Persson EC, Graubard BI, Evans AA, London WT, Weber JP, LeBlanc A, et al. Dichlorodiphenyltrichloroethane and Risk of Hepatocellular Carcinoma. Int J Cancer (2012) 131:2078–84. doi: 10.1002/ijc.27459
13. Waliszewski SM, Melo-Santiesteban G, Villalobos-Pietrini R, Gomez-Arroyo S, Amador-Munoz O, Herrero-Mercado M, et al. Breast Milk Excretion Kinetic of Beta-HCH, Pp'dde and Pp'ddt. B Environ Contam Tox (2009) 83:869–73. doi: 10.1007/s00128-009-9796-3
14. Imagawa W, Bandyopadhyay GK, Nandi S. Regulation of Mammary Epithelial Cell Growth in Mice and Rats. Endocr Rev (1990) 11:494–523. doi: 10.1210/edrv-11-4-494
15. Rosen JM, Wyszomierski SL, Hadsell D. Regulation of Milk Protein Gene Expression. Annu Rev Nutr (1999) 19:407–36. doi: 10.1146/annurev.nutr.19.1.407
16. Neville MC, McFadden TB, Forsyth I. Hormonal Regulation of Mammary Differentiation and Milk Secretion. J Mammary Gland Biol Neoplasia (2002) 7:49–66. doi: 10.1023/a:1015770423167
17. Kishikawa N, Kuroda N. Evaluation of Organic Environmental Pollutants Detected in Human Milk. J Health Sci (2009) 55:1–10. doi: 10.1248/jhs.55.1
18. Solomon GM, Weiss PM. Chemical Contaminants in Breast Milk: Time Trends and Regional Variability. Environ Health Perspect (2002) 110:A339–347. doi: 10.1289/ehp.021100339
19. Keswani C, Dilnashin H, Birla H, Roy P, Tyagi RK, Singh D, et al. Global Footprints of Organochlorine Pesticides: A Pan-Global Survey. Environ Geochem Health (2022) 44:149–77. doi: 10.1007/s10653-021-00946-7
20. Dewan P, Jain V, Gupta P, Banerjee BD. Organochlorine Pesticide Residues in Maternal Blood, Cord Blood, Placenta, and Breastmilk and Their Relation to Birth Size. Chemosphere (2013) 90:1704–10. doi: 10.1016/j.chemosphere.2012.09.083
21. Iszatt N, Janssen S, Lenters V, Dahl C, Stigum H, Knight R, et al. Environmental Toxicants in Breast Milk of Norwegian Mothers and Gut Bacteria Composition and Metabolites in Their Infants at 1 Month. Microbiome (2019) 7:34. doi: 10.1186/s40168-019-0645-2
22. Forns J, Mandal S, Iszatt N, Polder A, Thomsen C, Lyche JL, et al. Novel Application of Statistical Methods for Analysis of Multiple Toxicants Identifies DDT as a Risk Factor for Early Child Behavioral Problems. Environ Res (2016) 151:91–100. doi: 10.1016/j.envres.2016.07.014
23. Kao CC, Que DE, Bongo SJ, Tayo LL, Lin YH, Lin CW, et al. Residue Levels of Organochlorine Pesticides in Breast Milk and Its Associations With Cord Blood Thyroid Hormones and the Offspring's Neurodevelopment. Int J Env Res Pub He (2019) 16:1438. doi: 10.3390/ijerph16081438
24. Yu Z, Palkovicova L, Drobna B, Petrik J, Kocan A, Trnovec T, et al. Comparison of Organochlorine Compound Concentrations in Colostrum and Mature Milk. Chemosphere (2007) 66:1012–8. doi: 10.1016/j.chemosphere.2006.07.043
25. Lakind JS, Berlin CM, SjöDin A, Turner W, Wang RY, Needham LL, et al. Do Human Milk Concentrations of Persistent Organic Chemicals Really Decline During Lactation? Chemical Concentrations During Lactation and Milk/Serum Partitioning. Environ Health Persp (2009) 117:1625–31. doi: 10.1289/ehp.0900876
26. Zhou Y, Yuan B, Nyberg E, Yin G, Bignert A, Glynn A, et al. Chlorinated Paraffins in Human Milk From Urban Sites in China, Sweden, and Norway. Environ Sci Technol (2020) 54:4356–66. doi: 10.1021/acs.est.9b06089
27. Chan JK, Xing GH, Xu Y, Liang Y, Chen LX, Wu SC, et al. Body Loadings and Health Risk Assessment of Polychlorinated Dibenzo-P-Dioxins and Dibenzofurans at an Intensive Electronic Waste Recycling Site in China. Environ Sci Technol (2007) 41:7668–74. doi: 10.1021/es071492j
28. Lee S, Kim S, Park J, Kim HJ, Choi G, Choi S, et al. Perfluoroalkyl Substances (PFASs) in Breast Milk From Korea: Time-Course Trends, Influencing Factors, and Infant Exposure. Sci Total Environ (2018) 612:286–92. doi: 10.1016/j.scitotenv.2017.08.094
29. Ribas-Fito N, Cardo E, Sala M, Eulalia de Muga M, Mazon C, Verdu A, et al. Breastfeeding, Exposure to Organochlorine Compounds, and Neurodevelopment in Infants. Pediatrics (2003) 111:e580–5. doi: 10.1542/peds.111.5.e580
30. Mamontova EA, Tarasova EN, Mamontov AA. PCBs and OCPs in Human Milk in Eastern Siberia, Russia: Levels, Temporal Trends and Infant Exposure Assessment. Chemosphere (2017) 178:239–48. doi: 10.1016/j.chemosphere.2017.03.058
31. Roots O, Simm M, Kiviranta H, Rantakokko P. Persistent Organic Pollutants (POPs): Food Safety Control in Estonia. Springer Netherlands (2008) 173–85. doi: 10.1007/978-1-4020-6642-9_14
32. Lu D, Wang D, Ni R, Lin Y, Feng C, Xu Q, et al. Organochlorine Pesticides and Their Metabolites in Human Breast Milk From Shanghai, China. Environ Sci Pollut Res Int (2015) 22:9293–306. doi: 10.1007/s11356-015-4072-z
33. Kojima H, Katsura E, Takeuchi S, Niiyama K, Kobayashi K. Screening for Estrogen and Androgen Receptor Activities in 200 Pesticides by In Vitro Reporter Gene Assays Using Chinese Hamster Ovary Cells. Environ Health Perspect (2004) 112:524–31. doi: 10.1289/ehp.6649
34. Wolff MS. Half-Lives of Organochlorines (OCs) in Humans. Arch Environ Contam Toxicol (1999) 36:504. doi: 10.1007/pl00006624
35. Toan VD, Thao VD, Walder J, Ha CT. Residue, Temporal Trend and Half-Life Time of Selected Organochlorine Pesticides (OCPs) in Surface Soils From Bacninh, Vietnam. B Environ Contam Tox (2009) 82:516–21. doi: 10.1007/s00128-008-9629-9
36. Kezios KL, Liu X, Cirillo PM, Cohn BA, Kalantzi OI, Wang Y, et al. Dichlorodiphenyltrichloroethane (DDT), DDT Metabolites and Pregnancy Outcomes. Reprod Toxicol (2013) 35:156–64. doi: 10.1016/j.reprotox.2012.10.013
37. Saeedi Saravi SS, Dehpour AR. Potential Role of Organochlorine Pesticides in the Pathogenesis of Neurodevelopmental, Neurodegenerative, and Neurobehavioral Disorders: A Review. Life Sci (2016) 145:255–64. doi: 10.1016/j.lfs.2015.11.006
38. Rani M, Shanker U, Jassal V. Recent Strategies for Removal and Degradation of Persistent & Toxic Organochlorine Pesticides Using Nanoparticles: A Review. J Environ Manage (2017) 190:208–22. doi: 10.1016/j.jenvman.2016.12.068
39. Blair RM, Fang H, Branham WS, Hass BS, Dial SL, Moland CL, et al. The Estrogen Receptor Relative Binding Affinities of 188 Natural and Xenochemicals: Structural Diversity of Ligands. Toxicol Sci (2000) 54:138–53. doi: 10.1093/toxsci/54.1.138
40. Gaido KW, Leonard LS, Maness SC, Hall JM, McDonnell DP, Saville B, et al. Differential Interaction of the Methoxychlor Metabolite 2,2-Bis-(P-Hydroxyphenyl)-1,1,1-Trichloroethane With Estrogen Receptors Alpha and Beta. Endocrinology (1999) 140:5746–53. doi: 10.1210/endo.140.12.7191
41. Maness SC, McDonnell DP, Gaido KW. Inhibition of Androgen Receptor-Dependent Transcriptional Activity by DDT Isomers and Methoxychlor in HepG2 Human Hepatoma Cells. Toxicol Appl Pharmacol (1998) 151:135–42. doi: 10.1006/taap.1998.8431
42. Poland A, Knutson JC. 2,3,7,8-Tetrachlorodibenzo-Para-Dioxin and Related Halogenated Aromatic-Hydrocarbons - Examination of the Mechanism of Toxicity. Annu Rev Pharmacol (1982) 22:517–54. doi: 10.1146/annurev.pa.22.040182.002505
43. Umeda K, Kotake Y, Miyara M, Ishida K, Sanoh S, Ohta S. Methoxychlor and Fenvalerate Induce Neuronal Death by Reducing GluR2 Expression. J Toxicol Sci (2016) 41:255–64. doi: 10.2131/jts.41.255
44. Cortes DR, Hites RA. Detection of Statistically Significant Trends in Atmospheric Concentrations of Semivolatile Compounds. Environ Sci Technol (2000) 34:2826–9. doi: 10.1021/es990466l
45. Criswell R, Lenters V, Mandal S, Stigum H, Iszatt N, Eggesbo M. Persistent Environmental Toxicants in Breast Milk and Rapid Infant Growth. Ann Nutr Metab (2017) 70:210–6. doi: 10.1159/000463394
46. Tang M, Xu C, Chen K, Yan Q, Mao W, Liu W, et al. Hexachlorocyclohexane Exposure Alters the Microbiome of Colostrum in Chinese Breastfeeding Mothers. Environ Pollut (2019) 254:112900. doi: 10.1016/j.envpol.2019.07.068
47. Kuang L, Hou Y, Huang F, Guo A, Deng W, Sun H, et al. Pesticides in Human Milk Collected From Jinhua, China: Levels, Influencing Factors and Health Risk Assessment. Ecotoxicol Environ Saf (2020) 205:111331. doi: 10.1016/j.ecoenv.2020.111331
48. van der Oost R, Beyer J, Vermeulen NPE. Fish Bioaccumulation and Biomarkers in Environmental Risk Assessment: A Review. Environ Toxicol Phar (2003) 13:57–149. doi: 10.1016/S1382-6689(02)00126-6
49. Gale WL, Patino R, Maule AG. Interaction of Xenobiotics With Estrogen Receptors Alpha and Beta and a Putative Plasma Sex Hormone-Binding Globulin From Channel Catfish (Ictalurus Punctatus). Gen Comp Endocrinol (2004) 136:338–45. doi: 10.1016/j.ygcen.2004.01.009
50. Damgaard IN, Skakkebaek NE, Toppari J, Virtanen HE, Shen H, Schramm KW, et al. Nordic Cryptorchidism Study, G. Persistent Pesticides in Human Breast Milk and Cryptorchidism. Environ Health Perspect (2006) 114:1133–8. doi: 10.1289/ehp.8741
51. Bidleman TF, Jantunen LM, Helm PA, Brorstrom-Lunden E, Juntto S. Chlordane Enantiomers and Temporal Trends of Chlordane Isomers in Arctic Air. Environ Sci Technol (2002) 36:539–44. doi: 10.1021/es011142b
52. Araki A, Miyashita C, Mitsui T, Goudarzi H, Mizutani F, Chisaki Y, et al. Prenatal Organochlorine Pesticide Exposure and the Disruption of Steroids and Reproductive Hormones in Cord Blood: The Hokkaido Study. Environ Int (2018) 110:1–13. doi: 10.1016/j.envint.2017.10.006
53. Basterrechea M, Lertxundi A, Iniguez C, Mendez M, Murcia M, Mozo I, et al. Prenatal Exposure to Hexachlorobenzene (HCB) and Reproductive Effects in a Multicentre Birth Cohort in Spain. Sci Total Environ (2014) 466:770–6. doi: 10.1016/j.scitotenv.2013.07.053
54. Burton MAS, Bennett BG. Exposure of Man to Environmental Hexachlorobenzene (Hcb) - An Exposure Commitment Assessment. Sci Total Environ (1987) 66:137–46. doi: 10.1016/0048-9697(87)90083-0
55. Tang-Peronard JL, Andersen HR, Jensen TK, Heitmann BL. Endocrine-Disrupting Chemicals and Obesity Development in Humans: A Review. Obes Rev (2011) 12:622–36. doi: 10.1111/j.1467-789X.2011.00871.x
56. Vasiliu O, Muttineni J, Karmaus W. In Utero Exposure to Organochlorines and Age at Menarche. Hum Reprod (2004) 19:1506–12. doi: 10.1093/humrep/deh292
57. Kuhl AJ, Manning S, Brouwer M. Brain Aromatase in Japanese Medaka (Oryzias Latipes): Molecular Characterization and Role in Xenoestrogen-Induced Sex Reversal. J Steroid Biochem Mol Biol (2005) 96:67–77. doi: 10.1016/j.jsbmb.2005.01.029
58. Calaf GM, Ponce-Cusi R, Aguayo F, Munoz JP, Bleak TC. Endocrine Disruptors From the Environment Affecting Breast Cancer. Oncol Lett (2020) 20:19–32. doi: 10.3892/ol.2020.11566
59. Gao B, Liu WB, Jia LY, Xu L, Xie J. Isolation and Characterization of an Alcaligenes Sp. Strain DG-5 Capable of Degrading DDTs Under Aerobic Conditions. J Environ Sci Health B (2011) 46:257–63. doi: 10.1080/03601234.2011.540534
60. Chen A, Rogan WJ. Nonmalarial Infant Deaths and DDT Use for Malaria Control. Emerg Infect Dis (2003) 9:960–4. doi: 10.3201/eid0908.030082
61. Longnecker MP, Klebanoff MA, Zhou HB, Brock JW. Association Between Maternal Serum Concentration of the DDT Metabolite DDE and Preterm and Small-For-Gestational-Age Babies at Birth. Lancet (2001) 358:110–4. doi: 10.1016/S0140-6736(01)05329-6
62. Gladen BC, Rogan WJ. Dde and Shortened Duration of Lactation in a Northern Mexican Town. Am J Public Health (1995) 85:504–8. doi: 10.2105/AJPH.85.4.504
63. Cummings AM. Methoxychlor as a Model for Environmental Estrogens. Crit Rev Toxicol (1997) 27:367–79. doi: 10.3109/10408449709089899
64. Hassine SB, Ameur WB, Gandoura N, Driss MR. Determination of Chlorinated Pesticides, Polychlorinated Biphenyls, and Polybrominated Diphenyl Ethers in Human Milk From Bizerte (Tunisia) in 2010. Chemosphere (2012) 89:369–77. doi: 10.1016/j.chemosphere.2012.05.035
65. Ennaceur S, Gandoura N, Driss MR. Distribution of Polychlorinated Biphenyls and Organochlorine Pesticides in Human Breast Milk From Various Locations in Tunisia: Levels of Contamination, Influencing Factors, and Infant Risk Assessment. Environ Res (2008) 108:86–93. doi: 10.1016/j.envres.2008.05.005
66. Willett KL, Ulrich EM, Hites RA. Differential Toxicity and Environmental Fates of Hexachlorocyclohexane Isomers. Environ Sci Technol (1998) 32:2197–207. doi: 10.1021/es9708530
67. Lal R, Pandey G, Sharma P, Kumari K, Malhotra S, Pandey R, et al. Biochemistry of Microbial Degradation of Hexachlorocyclohexane and Prospects for Bioremediation. Microbiol Mol Biol Rev (2010) 74:58–80. doi: 10.1128/MMBR.00029-09
68. Nayyar N, Sangwan N, Kohli P, Verma H, Kumar R, Negi V, et al. Hexachlorocyclohexane: Persistence, Toxicity and Decontamination. Rev Environ Health (2014) 29:49–52. doi: 10.1515/reveh-2014-0015
69. Witczak A, Pohorylo A, Abdel-Gawad H. Endocrine-Disrupting Organochlorine Pesticides in Human Breast Milk: Changes During Lactation. Nutrients (2021) 13:229. doi: 10.3390/nu13010229
70. ATSDR (2005) Toxicological Profile for Hexachlorocyclohexanes. U.S. Department of Health and Human Services. Public Health Service. Agency for Toxic Substances and Disease Registry. (2005). Available at: http://www.atsdr.cdc.gov/toxprofiles/tp43.html
71. Milesi MM, Durando M, Lorenz V, Gastiazoro MP, Varayoud J. Postnatal Exposure to Endosulfan Affects Uterine Development and Fertility. Mol Cell Endocrinol (2020) 511:110855. doi: 10.1016/j.mce.2020.110855
72. Kania-Korwel I, Lehmler HJ. Chlordane and Heptachlor are Metabolized Enantioselectively by Rat Liver Microsomes. Environ Sci Technol (2013) 47:8913–22. doi: 10.1021/es401916a
73. Singh K, Nong A, Feeley M, Chan HM. Development of Biomonitoring Equivalents for Chlordane and Toxaphene With Application to the General Canadian Population. Regul Toxicol Pharmacol (2019) 106:262–9. doi: 10.1016/j.yrtph.2019.05.015
74. Wu Q, Du X, Feng X, Cheng H, Chen Y, Lu C, et al. Chlordane Exposure Causes Developmental Delay and Metabolic Disorders in Drosophila Melanogaster. Ecotoxicol Environ Saf (2021) 225:112739. doi: 10.1016/j.ecoenv.2021.112739
75. Muller MD, Buser HR. Identification of the (+)-Enantiomers and (-)-Enantiomers of Chiral Chlordane Compounds Using Chiral High-Performance Liquid-Chromatography Chiroptical Detection and Chiral High-Resolution Gas-Chromatography Mass-Spectrometry. Anal Chem (1994) 66:2155–62. doi: 10.1021/ac00085a034
76. Wang C, Li Z, Zhang Q, Zhao M, Liu W. Enantioselective Induction of Cytotoxicity by O,P'-DDD in PC12 Cells: Implications of Chirality in Risk Assessment of POPs Metabolites. Environ Sci Technol (2013) 47:3909–17. doi: 10.1021/es3049306
77. Hoekstra PF, Burnison BK, Neheli T, Muir DC. Enantiomer-Specific Activity of O,P'-DDT With the Human Estrogen Receptor. Toxicol Lett (2001) 125:75–81. doi: 10.1016/s0378-4274(01)00410-6
78. Fendick EA, Mathermihaich E, Houck KA, Stclair MB, Faust JB, Rockwell CH, et al. Ecological Toxicology and Human Health-Effects of Heptachlor. Rev Environ Contam T (1990) 111:61–142. doi: 10.1007/978-1-4612-3340-4_2
79. Richardson JR, Caudle WM, Wang MZ, Dean ED, Pennell KD, Miller GW. Developmental Heptachlor Exposure Increases Susceptibility of Dopamine Neurons to N-Methyl-4-Phenyl-1,2,3,6-Tetrahydropyridine (MPTP)in a Gender-Specific Manner. Neurotoxicology (2008) 29:855–63. doi: 10.1016/j.neuro.2008.05.007
80. Beseler CL, Stallones L, Hoppin JA, Alavanja MCR, Blair A, Keefe T, et al. Depression and Pesticide Exposures Among Private Pesticide Applicators Enrolled in the Agricultural Health Study. Environ Health Persp (2008) 116:1713–9. doi: 10.1289/ehp.11091
81. Courtney KD. Hexachlorobenzene (HCB): A Review. Environ Res (1979) 20:225–66. doi: 10.1016/0013-9351(79)90001-x
82. Miret NV, Pontillo CA, Zarate LV, Kleiman de Pisarev D, Cocca C, Randi AS. Impact of Endocrine Disruptor Hexachlorobenzene on the Mammary Gland and Breast Cancer: The Story Thus Far. Environ Res (2019) 173:330–41. doi: 10.1016/j.envres.2019.03.054
83. Starek-Swiechowicz B, Budziszewska B, Starek A. Hexachlorobenzene as a Persistent Organic Pollutant: Toxicity and Molecular Mechanism of Action. Pharmacol Rep (2017) 69:1232–9. doi: 10.1016/j.pharep.2017.06.013
84. Poland A, Knutson JC. 2,3,7,8-Tetrachlorodibenzo-P-Dioxin and Related Halogenated Aromatic Hydrocarbons: Examination of the Mechanism of Toxicity. Annu Rev Pharmacol Toxicol (1982) 22:517–54. doi: 10.1146/annurev.pa.22.040182.002505
85. Croes K, Colles A, Koppen G, Govarts E, Bruckers L, Van de Mieroop E, et al. Persistent Organic Pollutants (POPs) in Human Milk: A Biomonitoring Study in Rural Areas of Flanders (Belgium). Chemosphere (2012) 89:988–94. doi: 10.1016/j.chemosphere.2012.06.058
86. Evanthia DK, Jean-Pierre B, Giudice LC, Russ H, Prins GS, Soto AM, et al. Endocrine-Disrupting Chemicals: An Endocrine Society Scientific Statement. Endocr Rev (2009) 30:293–342. doi: 10.1210/er.2009-0002
87. Benbrook CM. Organochlorine Residues Pose Surprisingly High Dietary Risks. J Epidemiol Community Health (2002) 56:822–3. doi: 10.1136/jech.56.11.822
88. Chung SW, Kwong KP, Yau JC. Dietary Exposure to DDT of Secondary School Students in Hong Kong. Chemosphere (2008) 73:65–9. doi: 10.1016/j.chemosphere.2008.05.049
89. Darnerud PO, Atuma S, Aune M, Bjerselius R, Glynn A, Grawe KP, et al. Dietary Intake Estimations of Organohalogen Contaminants (Dioxins, PCB, PBDE and Chlorinated Pesticides, E.G. DDT) Based on Swedish Market Basket Data. Food Chem Toxicol (2006) 44:1597–606. doi: 10.1016/j.fct.2006.03.011
90. Ibarluzea J, Alvarez-Pedrerol M, Guxens M, Marina LS, Basterrechea M, Lertxundi A, et al. Sociodemographic, Reproductive and Dietary Predictors of Organochlorine Compounds Levels in Pregnant Women in Spain. Chemosphere (2011) 82:114–20. doi: 10.1016/j.chemosphere.2010.09.051
91. Zhou J, Zeng X, Zheng K, Zhu X, Ma L, Xu Q, et al. Musks and Organochlorine Pesticides in Breast Milk From Shanghai, China: Levels, Temporal Trends and Exposure Assessment. Ecotoxicol Environ Saf (2012) 84:325–33. doi: 10.1016/j.ecoenv.2012.08.011
92. Pandit GG, Sharma S, Srivastava PK, Sahu SK. Persistent Organochlorine Pesticide Residues in Milk and Dairy Products in India. Food Addit Contam (2002) 19:153–7. doi: 10.1080/02652030110081155
93. Tian H. Determination of Chloramphenicol, Enrofloxacin and 29 Pesticides Residues in Bovine Milk by Liquid Chromatography-Tandem Mass Spectrometry. Chemosphere (2011) 83:349–55. doi: 10.1016/j.chemosphere.2010.12.016
94. Dong Y, Yin S, Zhang J, Guo F, Aamir M, Liu S, et al. Exposure Patterns, Chemical Structural Signatures, and Health Risks of Pesticides in Breast Milk: A Multicenter Study in China. Sci Total Environ (2022) 830:154617. doi: 10.1016/j.scitotenv.2022.154617
95. Saleh EA, Nassar AMK, Amer HH. Organochlorine Pesticide Residues in Raw and Grilled Freshwater Fish (Oreochromis Niloticus) Collected From Various Locations Along the Nile Basin in Egypt. Environ Monit Assess (2021) 193:673. doi: 10.1007/s10661-021-09455-7
96. Klincic D, Herceg Romanic S, Matek Saric M, Grzunov J, Dukic B. Polychlorinated Biphenyls and Organochlorine Pesticides in Human Milk Samples From Two Regions in Croatia. Environ Toxicol Pharmacol (2014) 37:543–52. doi: 10.1016/j.etap.2014.01.009
97. Mueller JF, Harden F, Toms LM, Symons R, Furst P. Persistent Organochlorine Pesticides in Human Milk Samples From Australia. Chemosphere (2008) 70:712–20. doi: 10.1016/j.chemosphere.2007.06.037
98. Sun SJ, Zhao JH, Koga M, Ma YX, Liu DW, Nakamura M, et al. Persistent Organic Pollutants in Human Milk in Women From Urban and Rural Areas in Northern China. Environ Res (2005) 99:285–93. doi: 10.1016/j.envres.2005.05.007
99. Zhou P, Wu Y, Yin S, Li J, Zhao Y, Zhang L, et al. National Survey of the Levels of Persistent Organochlorine Pesticides in the Breast Milk of Mothers in China. Environ Pollut (2011) 159:524–31. doi: 10.1016/j.envpol.2010.10.014
100. Cerna M, Bencko V, Brabec M, Smid J, Krskova A, Jech L. Exposure Assessment of Breast-Fed Infants in the Czech Republic to Indicator PCBs and Selected Chlorinated Pesticides: Area-Related Differences. Chemosphere (2010) 78:160–8. doi: 10.1016/j.chemosphere.2009.09.062
101. Dimitriadou L, Malarvannan G, Covaci A, Iossifidou E, Tzafettas J, Zournatzi-Koiou V, et al. Levels and Profiles of Brominated and Chlorinated Contaminants in Human Breast Milk From Thessaloniki, Greece. Sci Total Environ (2016) 539:350–8. doi: 10.1016/j.scitotenv.2015.08.137
102. Kunisue T, Someya M, Kayama F, Jin Y, Tanabe S. Persistent Organochlorines in Human Breast Milk Collected From Primiparae in Dalian and Shenyang, China. Environ Pollut (2004) 131:381–92. doi: 10.1016/j.envpol.2004.03.008
103. Minh NH, Someya M, Minh TB, Kunisue T, Iwata H, Watanabe M, et al. Persistent Organochlorine Residues in Human Breast Milk From Hanoi and Hochiminh City, Vietnam: Contamination, Accumulation Kinetics and Risk Assessment for Infants. Environ Pollut (2004) 129:431–41. doi: 10.1016/j.envpol.2003.11.012
104. Sudaryanto A, Kunisue T, Kajiwara N, Iwata H, Adibroto TA, Hartono P, et al. Specific Accumulation of Organochlorines in Human Breast Milk From Indonesia: Levels, Distribution, Accumulation Kinetics and Infant Health Risk. Environ Pollut (2006) 139:107–17. doi: 10.1016/j.envpol.2005.04.028
105. Gascon M, Verner MA, Guxens M, Grimalt JO, Forns J, Ibarluzea J, et al. Evaluating the Neurotoxic Effects of Lactational Exposure to Persistent Organic Pollutants (POPs) in Spanish Children. Neurotoxicology (2013) 34:9–15. doi: 10.1016/j.neuro.2012.10.006
106. Mueller MHB, Polder A, Brynildsrud OB, Karimi M, Lie E, Manyilizu WB, et al. Organochlorine Pesticides (OCPs) and Polychlorinated Biphenyls (PCBs) in Human Breast Milk and Associated Health Risks to Nursing Infants in Northern Tanzania. Environ Res (2017) 154:425–34. doi: 10.1016/j.envres.2017.01.031
107. Haggarty P. Fatty Acid Supply to the Human Fetus. Annu Rev Nutr (2010) 30:237–55. doi: 10.1146/annurev.nutr.012809.104742
108. Grimalt JO, Gari M, Santa-Marina L, Ibarluzea J, Sunyer J. Influence of Gestational Weight Gain on the Organochlorine Pollution Content of Breast Milk. Environ Res (2022) 209: 112783. doi: 10.1016/j.envres.2022.112783
109. Chen MW, Santos HM, Que DE, Gou YY, Tayo LL, Hsu YC, et al. Association Between Organochlorine Pesticide Levels in Breast Milk and Their Effects on Female Reproduction in a Taiwanese Population. Int J Environ Res Public Health (2018) 15:931. doi: 10.3390/ijerph15050931
110. Chao HR, Shy CG, Wang SL, Chen SC, Koh TW, Chen FA, et al. Impact of non-Occupational Exposure to Polybrominated Diphenyl Ethers on Menstruation Characteristics of Reproductive-Age Females. Environ Int (2010) 36:728–35. doi: 10.1016/j.envint.2010.05.007
111. Chao HR, Wang SL, Lin LY, Lee WJ, Papke O. Placental Transfer of Polychlorinated Dibenzo-P-Dioxins, Dibenzofurans, and Biphenyls in Taiwanese Mothers in Relation to Menstrual Cycle Characteristics. Food Chem Toxicol (2007) 45:259–65. doi: 10.1016/j.fct.2006.07.032
112. Rogan WJ, Gladen BC, McKinney JD, Carreras N, Hardy P, Thullen J, et al. Polychlorinated Biphenyls (PCBs) and Dichlorodiphenyl Dichloroethene (DDE) in Human Milk: Effects on Growth, Morbidity, and Duration of Lactation. Am J Public Health (1987) 77:1294–7. doi: 10.2105/ajph.77.10.1294
113. Jia M, Dahlman-Wright K, Gustafsson JA. Estrogen Receptor Alpha and Beta in Health and Disease. Best Pract Res Clin Endocrinol Metab (2015) 29:557–68. doi: 10.1016/j.beem.2015.04.008
114. Brzozowski AM, Pike AC, Dauter Z, Hubbard RE, Bonn T, Engstrom O, et al. Molecular Basis of Agonism and Antagonism in the Oestrogen Receptor. Nature (1997) 389:753–8. doi: 10.1038/39645
115. Mocklinghoff S, Rose R, Carraz M, Visser A, Ottmann C, Brunsveld L. Synthesis and Crystal Structure of a Phosphorylated Estrogen Receptor Ligand Binding Domain. Chembiochem (2010) 11:2251–4. doi: 10.1002/cbic.201000532
116. Eiler S, Gangloff M, Duclaud S, Moras D, Ruff M. Overexpression, Purification, and Crystal Structure of Native ER Alpha LBD. Protein Expr Purif (2001) 22:165–73. doi: 10.1006/prep.2001.1409
117. Baker ME, Lathe R. The Promiscuous Estrogen Receptor: Evolution of Physiological Estrogens and Response to Phytochemicals and Endocrine Disruptors. J Steroid Biochem Mol Biol (2018) 184:29–37. doi: 10.1016/j.jsbmb.2018.07.001
118. Kuiper GG, Lemmen JG, Carlsson B, Corton JC, Safe SH, van der Saag PT, et al. Interaction of Estrogenic Chemicals and Phytoestrogens With Estrogen Receptor Beta. Endocrinology (1998) 139:4252–63. doi: 10.1210/endo.139.10.6216
119. Singleton DW, Khan SA. Xenoestrogen Exposure and Mechanisms of Endocrine Disruption. Front Biosci-Landmrk (2003) 8:S110–8. doi: 10.2741/1010
120. Degen GH, Bolt HM. Endocrine Disruptors: Update on Xenoestrogens. Int Arch Occ Env Hea (2000) 73:433–41. doi: 10.1007/s004200000163
121. Alex A, Bhandary E, McGuire KP. Anatomy and Physiology of the Breast During Pregnancy and Lactation. Adv Exp Med Biol (2020) 1252:3–7. doi: 10.1007/978-3-030-41596-9_1
122. Rogan WJ, Ragan NB. Some Evidence of Effects of Environmental Chemicals on the Endocrine System in Children. Int J Hyg Environ Health (2007) 210:659–67. doi: 10.1016/j.ijheh.2007.07.005
123. Freeman ME, Kanyicska B, Lerant A, Nagy G. Prolactin: Structure, Function, and Regulation of Secretion. Physiol Rev (2000) 80:1523–631. doi: 10.1152/physrev.2000.80.4.1523
124. Brisken C. Hormonal Control of Alveolar Development and its Implications for Breast Carcinogenesis. J Mammary Gland Biol (2002) 7:39–48. doi: 10.1023/A:1015718406329
125. Krown KA, Wang YF, Ho TW, Kelly PA, Walker AM. Prolactin Isoform 2 as an Autocrine Growth Factor for GH3 Cells. Endocrinology (1992) 131:595–602. doi: 10.1210/endo.131.2.1639009
126. DiMattia GE, Gellersen B, Bohnet HG, Friesen HG. A Human B-Lymphoblastoid Cell Line Produces Prolactin. Endocrinology (1988) 122:2508–17. doi: 10.1210/endo-122-6-2508
127. Lucas BK, Ormandy CJ, Binart N, Bridges RS, Kelly PA. Null Mutation of the Prolactin Receptor Gene Produces a Defect in Maternal Behavior. Endocrinology (1998) 139:4102–7. doi: 10.1210/endo.139.10.6243
128. Wozniak AL, Bulayeva NN, Watson CS. Xenoestrogens at Picomolar to Nanomolar Concentrations Trigger Membrane Estrogen Receptor-Alpha-Mediated Ca2+ Fluxes and Prolactin Release in GH3/B6 Pituitary Tumor Cells. Environ Health Perspect (2005) 113:431–9. doi: 10.1289/ehp.7505
129. Xu XL, Deng SL, Lian ZX, Yu K. Estrogen Receptors in Polycystic Ovary Syndrome. Cells-Basel (2021) 10:161–71. doi: 10.3390/cells10020459
130. Watson CS, Norfleet AM, Pappas TC, Gametchu B. Rapid Actions of Estrogens in GH3/B6 Pituitary Tumor Cells via a Plasma Membrane Version of Estrogen Receptor-Alpha. Steroids (1999) 64:5–13. doi: 10.1016/s0039-128x(98)00107-x
131. Alvarez L, Randi A, Alvarez P, Piroli G, Chamson-Reig A, Lux-Lantos V, et al. Reproductive Effects of Hexachlorobenzene in Female Rats. J Appl Toxicol (2000) 20:81–7. doi: 10.1002/(sici)1099-1263(200001/02)20:1<81::aid-jat629>3.0.co;2-z
132. Parker CR Jr., MacDonald PC, Guzick DS, Porter JC, Rosenfeld CR, Hauth JC. Prolactin Levels in Umbilical Cord Blood of Human Infants: Relation to Gestational Age, Maternal Complications, and Neonatal Lung Function. Am J Obstet Gynecol (1989) 161:795–802. doi: 10.1016/0002-9378(89)90404-3
133. Padvi NV, Narkhede HR, Pawar AP, Mhatre PN. Prolactin Level in Umbilical Cord Blood of Newborn and its Relation to Respiratory Distress Syndrome. J Int J Reprod Contracept Obstet Gynecol (2016) 6:1–6. doi: 10.18203/2320-1770.ijrcog20164798
134. Harrison GS, Wierman ME, Nett TM, Glode LM. Gonadotropin-Releasing Hormone and its Receptor in Normal and Malignant Cells. Endocr Relat Cancer (2004) 11:725–48. doi: 10.1677/erc.1.00777
135. Rieanrakwong D, Laoharatchatathanin T, Terashima R, Yonezawa T, Kurusu S, Hasegawa Y, et al. Prolactin Suppression of Gonadotropin-Releasing Hormone Initiation of Mammary Gland Involution in Female Rats. Endocrinology (2016) 157:2750–8. doi: 10.1210/en.2016-1180
136. Kawaminami M, Etoh S, Miyaoka H, Sakai M, Nishida M, Kurusu S, et al. Annexin 5 Messenger Ribonucleic Acid Expression in Pituitary Gonadotropes is Induced by Gonadotropin-Releasing Hormone (GnRH) and Modulates GnRH Stimulation of Gonadotropin Release. Neuroendocrinology (2002) 75:2–11. doi: 10.1159/000048216
137. Lew BJ, Manickam R, Lawrence BP. Activation of the Aryl Hydrocarbon Receptor During Pregnancy in the Mouse Alters Mammary Development Through Direct Effects on Stromal and Epithelial Tissues. Biol Reprod (2011) 84:1094–102. doi: 10.1095/biolreprod.110.087544
138. Knudsen KA, Wheelock MJ. Cadherins and the Mammary Gland. J Cell Biochem (2005) 95:488–96. doi: 10.1002/jcb.20419
139. Landrigan P, Garg A, Droller DB. Assessing the Effects of Endocrine Disruptors in the National Children's Study. Environ Health Perspect (2003) 111:1678–82. doi: 10.1289/ehp.5799
140. Barouki R, Gluckman PD, Grandjean P, Hanson M, Heindel JJ. Developmental Origins of non-Communicable Disease: Implications for Research and Public Health. Environ Health (2012) 11:42. doi: 10.1186/1476-069X-11-42
141. Massart F, Harrell JC, Federico G, Saggese G. Human Breast Milk and Xenoestrogen Exposure: A Possible Impact on Human Health. J Perinatol (2005) 25:282–8. doi: 10.1038/sj.jp.7211251
142. Barr DB, Weihe P, Davis MD, Needham LL, Grandjean P. Serum Polychlorinated Biphenyl and Organochlorine Insecticide Concentrations in a Faroese Birth Cohort. Chemosphere (2006) 62:1167–82. doi: 10.1016/j.chemosphere.2005.06.063
143. Eggesbo M, Stigum H, Longnecker MP, Polder A, Aldrin M, Basso O, et al. Levels of Hexachlorobenzene (HCB) in Breast Milk in Relation to Birth Weight in a Norwegian Cohort. Environ Res (2009) 109:559–66. doi: 10.1016/j.envres.2009.04.001
144. Schade G, Heinzow B. Organochlorine Pesticides and Polychlorinated Biphenyls in Human Milk of Mothers Living in Northern Germany: Current Extent of Contamination, Time Trend From 1986 to 1997 and Factors That Influence the Levels of Contamination. Sci Total Environ (1998) 215:31–9. doi: 10.1016/s0048-9697(98)00008-4
145. Gautam B, Rogge MM, Acharya N, Keesari R, Almekdash MH. Obesogenic Toxicants in Breast Milk of Lactating Women: Investigation of a Risk Factor for Childhood Obesity. Biol Res Nurs (2020) 22:295–301. doi: 10.1177/1099800420909151
146. Smink A, Ribas-Fito N, Garcia R, Torrent M, Mendez MA, Grimalt JO, et al. Exposure to Hexachlorobenzene During Pregnancy Increases the Risk of Overweight in Children Aged 6 Years. Acta Paediatr (2008) 97:1465–9. doi: 10.1111/j.1651-2227.2008.00937.x
147. Akerman CJ, Cline HT. Refining the Roles of GABAergic Signaling During Neural Circuit Formation. Trends Neurosci (2007) 30:382–9. doi: 10.1016/j.tins.2007.06.002
148. Wilson WW, Shapiro LP, Bradner JM, Caudle WM. Developmental Exposure to the Organochlorine Insecticide Endosulfan Damages the Nigrostriatal Dopamine System in Male Offspring. Neurotoxicology (2014) 44:279–87. doi: 10.1016/j.neuro.2014.07.008
149. Laurberg P. Thyroid Function: Thyroid Hormones, Iodine and the Brain-An Important Concern. Nat Rev Endocrinol (2009) 5:475–6. doi: 10.1038/nrendo.2009.155
150. Miodovnik A, Edwards A, Bellinger DC, Hauser R. Developmental Neurotoxicity of Ortho-Phthalate Diesters: Review of Human and Experimental Evidence. Neurotoxicology (2014) 41:112–22. doi: 10.1016/j.neuro.2014.01.007
151. Boucher O, Simard MN, Muckle G, Rouget F, Kadhel P, Bataille H, et al. Exposure to an Organochlorine Pesticide (Chlordecone) and Development of 18-Month-Old Infants. Neurotoxicology (2013) 35:162–8. doi: 10.1016/j.neuro.2013.01.007
152. Gilbert ME, Rovet J, Chen Z, Koibuchi N. Developmental Thyroid Hormone Disruption: Prevalence, Environmental Contaminants and Neurodevelopmental Consequences. Neurotoxicology (2012) 33:842–52. doi: 10.1016/j.neuro.2011.11.005
153. Yamazaki K, Araki A, Nakajima S, Miyashita C, Ikeno T, Itoh S, et al. Association Between Prenatal Exposure to Organochlorine Pesticides and the Mental and Psychomotor Development of Infants at Ages 6 and 18 Months: The Hokkaido Study on Environment and Children's Health. Neurotoxicology (2018) 69:201–8. doi: 10.1016/j.neuro.2017.11.011
154. Joly Condette C, Bach V, Mayeur C, Gay-Queheillard J, Khorsi-Cauet H. Chlorpyrifos Exposure During Perinatal Period Affects Intestinal Microbiota Associated With Delay of Maturation of Digestive Tract in Rats. J Pediatr Gastroenterol Nutr (2015) 61:30–40. doi: 10.1097/MPG.0000000000000734
155. Arrieta MC, Finlay BB. The Commensal Microbiota Drives Immune Homeostasis. Front Immunol (2012) 3:33. doi: 10.3389/fimmu.2012.00033
156. Giambo F, Teodoro M, Costa C, Fenga C. Toxicology and Microbiota: How Do Pesticides Influence Gut Microbiota? A Review. Int J Environ Res Public Health (2021) 18:5510. doi: 10.3390/ijerph18115510
157. Edwards TM, Moore BC, Guillette LJ Jr. Reproductive Dysgenesis in Wildlife: A Comparative View. Int J Androl (2006) 29:109–21. doi: 10.1111/j.1365-2605.2005.00631.x
158. Vos JG, Dybing E, Greim HA, Ladefoged O, Lambre C, Tarazona JV, et al. Health Effects of Endocrine-Disrupting Chemicals on Wildlife, With Special Reference to the European Situation. Crit Rev Toxicol (2000) 30:71–133. doi: 10.1080/10408440091159176
159. Brody JG, Rudel RA. Environmental Pollutants and Breast Cancer. Environ Health Perspect (2003) 111:1007–19. doi: 10.1289/ehp.6310
160. Cohn BA, La Merrill M, Krigbaum NY, Yeh G, Park JS, Zimmermann L, et al. DDT Exposure in Utero and Breast Cancer. J Clin Endocrinol Metab (2015) 100:2865–72. doi: 10.1210/jc.2015-1841
161. Bleak TC, Calaf GM. Breast and Prostate Glands Affected by Environmental Substances (Review). Oncol Rep (2021) 45:20. doi: 10.3892/or.2021.7971
162. Cortes D, Thorup JM, Visfeldt J. Cryptorchidism: Aspects of Fertility and Neoplasms. A Study Including Data of 1,335 Consecutive Boys Who Underwent Testicular Biopsy Simultaneously With Surgery for Cryptorchidism. Horm Res (2001) 55:21–7. doi: 10.1159/000049959
163. Krysiak-Baltyn K, Toppari J, Skakkebaek NE, Jensen TS, Virtanen HE, Schramm KW, et al. Association Between Chemical Pattern in Breast Milk and Congenital Cryptorchidism: Modelling of Complex Human Exposures. Int J Androl (2012) 35:294–302. doi: 10.1111/j.1365-2605.2012.01268.x
164. Tang ZR, Xu XL, Deng SL, Lian ZX, Yu K. Oestrogenic Endocrine Disruptors in the Placenta and the Fetus. Int J Mol Sci (2020) 21:1519. doi: 10.3390/ijms21041519
165. Schecter A, Kassis I. Papke, O. Partitioning of Dioxins, Dibenzofurans, and Coplanar PCBS in Blood, Milk, Adipose Tissue, Placenta and Cord Blood From Five American Women. Chemosphere (1998) 37:1817–23. doi: 10.1016/s0045-6535(98)00247-1
166. Przyrembel H, Heinrich-Hirsch B, Vieth B. Exposition to and Health Effects of Residues in Human Milk. Adv Exp Med Biol (2000) 478:307–25. doi: 10.1007/0-306-46830-1_27
167. Bandiera SM, Torok SM, Letcher RJ, Norstrom RJ. Immunoquantitation of Cytochromes P450 1A and P450 2B and Comparison With Chlorinated Hydrocarbon Levels in Archived Polar Bear Liver Samples. Chemosphere (1997) 34:1469–79. doi: 10.1016/s0045-6535(97)00443-8
168. Docea AO, Vassilopoulou L, Fragou D, Arsene AL, Fenga C, Kovatsi L, et al. CYP Polymorphisms and Pathological Conditions Related to Chronic Exposure to Organochlorine Pesticides. Toxicol Rep (2017) 4:335–41. doi: 10.1016/j.toxrep.2017.05.007
169. Mrema EJ, Rubino FM, Brambilla G, Moretto A, Tsatsakis AM, Colosio C. Persistent Organochlorinated Pesticides and Mechanisms of Their Toxicity. Toxicology (2013) 307:74–88. doi: 10.1016/j.tox.2012.11.015
170. Lopez-Espinosa MJ, Granada A, Carreno J, Salvatierra M, Olea-Serrano F, Olea N. Organochlorine Pesticides in Placentas From Southern Spain and Some Related Factors. Placenta (2007) 28:631–8. doi: 10.1016/j.placenta.2006.09.009
171. Kumar S. Occupational Exposure Associated With Reproductive Dysfunction. J Occup Health (2004) 46:1–19. doi: 10.1539/joh.46.1
172. Pathak R, Mustafa M, Ahmed RS, Tripathi AK, Guleria K, Banerjee BD. Association Between Recurrent Miscarriages and Organochlorine Pesticide Levels. Clin Biochem (2010) 43:131–5. doi: 10.1016/j.clinbiochem.2009.09.019
173. Anand M, Singh L, Agarwal P, Saroj R, Taneja A. Pesticides Exposure Through Environment and Risk of Pre-Term Birth: A Study From Agra City. Drug Chem Toxicol (2019) 42:471–7. doi: 10.1080/01480545.2017.1413107
174. Shen H, Main KM, Virtanen HE, Damggard IN, Haavisto AM, Kaleva M, et al. From Mother to Child: Investigation of Prenatal and Postnatal Exposure to Persistent Bioaccumulating Toxicants Using Breast Milk and Placenta Biomonitoring. Chemosphere (2007) 67:S256–62. doi: 10.1016/j.chemosphere.2006.05.106
175. Lewis-Mikhael AM, Olmedo-Requena R, Martinez-Ruiz V, Bueno-Cavanillas A, Jimenez-Moleon JJ. Organochlorine Pesticides and Prostate Cancer, Is There an Association? A Meta-Analysis of Epidemiological Evidence. Cancer Causes Control (2015) 26:1375–92. doi: 10.1007/s10552-015-0643-z
176. Kwon GS, Sohn HY, Shin KS, Kim E, Seo BI. Biodegradation of the Organochlorine Insecticide, Endosulfan, and the Toxic Metabolite, Endosulfan Sulfate, by Klebsiella Oxytoca KE-8. Appl Microbiol Biotechnol (2005) 67:845–50. doi: 10.1007/s00253-004-1879-9
177. Bhalerao TS, Puranik PR. Biodegradation of Organochlorine Pesticide, Endosulfan, by a Fungal Soil Isolate, Aspergillus Niger. Int Biodeterior Biodegradation (2007) 59:315–21. doi: 10.1016/j.ibiod.2006.09.002
178. Raschitor A, Llanos J, Rodrigo MA, Canizares P. Combined Electrochemical Processes for the Efficient Degradation of non-Polar Organochlorine Pesticides. J Environ Manage (2019) 248:109289. doi: 10.1016/j.jenvman.2019.109289
179. Comninellis C, Kapalka A, Malato S, Parsons SA, Poulios L, Mantzavinos D. Advanced Oxidation Processes for Water Treatment: Advances and Trends for R&D. J Chem Technol Biotechnol (2008) 83:769–76. doi: 10.1002/jctb.1873
180. Panizza M, Michaud PA, Cerisola G, Comninellis C. Anodic Oxidation of 2-Naphthol at Boron-Doped Diamond Electrodes. J Electroanal Chem (2001) 507:206–14. doi: 10.1016/S0022-0728(01)00398-9
181. Paknikar KM, Nagpal V, Pethkar AV, Rajwade JM. Degradation of Lindane From Aqueous Solutions Using Iron Sulfide Nanoparticles Stabilized by Biopolymers. Sci Technol Adv Mater (2005) 6:370–4. doi: 10.1016/j.stam.2005.02.016
182. Zhang Y, Guo J, Zhang X, Guo J, Zhang M, Yang Y, et al. Interaction Effects Between Organochlorine Pesticides and Isoflavones In Vitro and In Vivo. BioMed Res Int (2016) 2016:6861702. doi: 10.1155/2016/6861702
183. Badger TM, Gilchrist JM, Pivik RT, Andres A, Shankar K, Chen JR, et al. The Health Implications of Soy Infant Formula. Am J Clin Nutr (2009) 89:1668S–72S. doi: 10.3945/ajcn.2009.26736U
184. Foster WG, Younglai EV, Boutross-Tadross O, Hughes CL, Wade MG. Mammary Gland Morphology in Sprague-Dawley Rats Following Treatment With an Organochlorine Mixture. Utero Neonatal Genistein Toxicol Sci (2004) 77:91–100. doi: 10.1093/toxsci/kfg247
185. Andrabi SS, Parvez S, Tabassum H. Melatonin and Ischemic Stroke: Mechanistic Roles and Action. Adv Pharmacol Sci (2015) 2015:384750. doi: 10.1155/2015/384750
186. Olukole SG, Ajani SO, Ola-Davies EO, Lanipekun DO, Aina OO, Oyeyemi MO, et al. Melatonin Ameliorates Bisphenol A-Induced Perturbations of the Prostate Gland of Adult Wistar Rats. BioMed Pharmacother (2018) 105:73–82. doi: 10.1016/j.biopha.2018.05.125
187. Chen L, Mo H, Zhao L, Gao W, Wang S, Cromie MM, et al. Therapeutic Properties of Green Tea Against Environmental Insults. J Nutr Biochem (2017) 40:1–13. doi: 10.1016/j.jnutbio.2016.05.005
188. Sengupta D, Aktar MW, Alam S, Chowdhury A. Impact Assessment and Decontamination of Pesticides From Meat Under Different Culinary Processes. Environ Monit Assess (2010) 169:37–43. doi: 10.1007/s10661-009-1148-6
Keywords: organochlorine pesticides, breast milk, lactation, infant, estrogen endocrine disruptors
Citation: Qi S-Y, Xu X-L, Ma W-Z, Deng S-L, Lian Z-X and Yu K (2022) Effects of Organochlorine Pesticide Residues in Maternal Body on Infants. Front. Endocrinol. 13:890307. doi: 10.3389/fendo.2022.890307
Received: 05 March 2022; Accepted: 22 April 2022;
Published: 09 June 2022.
Edited by:
Angel I. Melo, Universidad Autónoma de Tlaxcala, MexicoReviewed by:
Arely Anaya-Hernández, Autonomous University of Tlaxcala, MexicoMosudi Sosan, Obafemi Awolowo University, Nigeria
Copyright © 2022 Qi, Xu, Ma, Deng, Lian and Yu. This is an open-access article distributed under the terms of the Creative Commons Attribution License (CC BY). The use, distribution or reproduction in other forums is permitted, provided the original author(s) and the copyright owner(s) are credited and that the original publication in this journal is cited, in accordance with accepted academic practice. No use, distribution or reproduction is permitted which does not comply with these terms.
*Correspondence: Wen-Zhi Ma, mawenzhi126@126.com; Kun Yu, yukun@cau.edu.cn; Zheng-Xing Lian, lianzhx@cau.edu.cn
†These authors have contributed equally to this work