- 1Department of Otolaryngology-Head and Neck Surgery, E-Da Hospital, Kaohsiung, Taiwan
- 2School of Medicine, College of Medicine, I-Shou University, Kaohsiung, Taiwan
- 3Department of Otolaryngology, E-Da Cancer Hospital, Kaohsiung, Taiwan
- 4Department of Otorhinolaryngology-Head and Neck Surgery, International Thyroid Surgery Center, Kaohsiung Medical University Hospital, Faculty of Medicine, College of Medicine, Kaohsiung Medical University, Kaohsiung, Taiwan
- 5Division of General Surgery, Endocrine Surgery Section, Istituto Auxologico Italiano IRCCS, Milan, Italy
- 6Department of Pathophysiology and Transplantation, University of Milan, Milan, Italy
Objectives: Traction injury is the most common type of recurrent laryngeal nerve (RLN) injury in thyroid surgery. Intraoperative neuromonitoring (IONM) facilitates early detection of adverse electromyography (EMG) effect, and this corrective maneuver can reduce severe and repeated nerve injury. This study aimed to evaluate intraoperative patterns and outcomes of EMG decrease and recovery by traction injury.
Methods: 644 patients received nerve monitored thyroidectomy with 1142 RLNs at risk were enrolled. Intermittent IONM with stimulating dissecting instrument (real-time during surgical procedure) and trans-thyroid cartilage EMG recording method (without electrode malpositioning issue) were used for nerve stimulation and signal recording. When an EMG amplitude showed a decrease of >50% during RLN dissection, the surgical maneuver was paused immediately. Nerve dissection was restarted when the EMG amplitude was stable.
Results: 44/1142 (3.9%) RLNs exhibited a >50% EMG amplitude decrease during RLN dissection and all (100%) showed gradual progressive amplitude recovery within a few minutes after releasing thyroid traction (10 recovered from LOS; 34 recovered from a 51-90% amplitude decrease). Three EMG recovery patterns were noted, A-complete EMG recovery (n=14, 32%); B-incomplete EMG recovery with an injury point (n=16, 36%); C-incomplete EMG recovery without an injury point (n=14, 32%). Patients with postoperative weak or fixed vocal cord mobility in A, B, and C were 0(0%), 7(44%), and 2(14%), respectively. Complete EMG recovery was found in 14 nerves, and incomplete recovery was found in another 30 nerves. Temporary vocal cord palsy was found in 6 nerves due to unavoidable repeated traction.
Conclusion: Early detection of traction-related RLN amplitude decrease allows monitoring of intraoperative EMG signal recovery during thyroid surgery. Different recovery patterns show different vocal cord function outcomes. To elucidate the recovery patterns can assist surgeons in the intraoperative decision making and postoperative management.
Introduction
Routine identification of the recurrent laryngeal nerve (RLN) has been accepted as the gold standard of care during thyroid surgery to decrease RLN palsy rates (1–4). During the RLN identification and dissection phase, the RLN can be injured by the surgical maneuvers of traction, clamping, electrocauterization, mechanical trauma, and transection. However, traction injury is the most common cause of RLN injury, with a rate of up to 70-80% among all maneuvers (5–8). Medial traction of the thyroid lobe is a necessary step in the surgical procedure to identify RLN. The RLN can be overstretched and injured by a dense fibrous band or surrounding blood vessels at the region of Berry’s ligament during medial thyroid traction. Loss of signal (LOS) was found after nerve dissection with the application of intraoperative neuromonitoring (IONM) in thyroid surgery, even though visual anatomical integrity of the RLN was confirmed intraoperatively (8–10).
For stimulating methods, intermittent IONM (I-IONM) applied with a hand-held stimulating probe is still mainstream internationally due to its convenience and inexpensiveness. However, it is challenging to detect early between the intervals of nerve stimulation using the conventional I-IONM, the adverse electromyography (EMG) change, and LOS is consistently recognized after the occurrence of traction injury (5–8). Animal and clinical studies of RLN traction injury with the application of continuous IONM (C-IONM) (11–14) revealed an EMG amplitude decrease during RLN traction and progressive recovery after releasing traction stress. C-IONM is reported to be helpful for the early detection of EMG amplitude decreases caused by traction distress (15–17). Stimulating dissecting instrument (SDI) is a novel and real-time I-IONM stimulating method during surgical procedure. It can maximally reduce the intervals of the nerve stimulation while maintaining the advantages of I-IONM convenience, simplicity and affordability. For recording methods, unstable and variable EMG amplitudes during operation have been reported using traditional EMG tubes for EMG recoding (18, 19). It will be challenging to differentiate an actual decrease in EMG signal caused by traction stress on the RLN from a false decrease caused by EMG tube displacement. The trans-thyroid cartilage EMG recording method can avoid the electrode malpositioning issue and obtain high-quality EMG signals.
IONM facilitates early detection of adverse EMG effect, and the corrective maneuver can reduce severe and repeated nerve injury. However, intraoperative patterns and outcomes of EMG recovery are less studied in the literature. In this study, SDI and the trans-thyroid cartilage EMG recording method were used for nerve stimulation and signal recording. With these two new IONM techniques, we aimed to determine the feasibility of early detection of a significant EMG amplitude decrease during the phase of RLN dissection and the possibility of intraoperative EMG recovery after releasing thyroid traction, which might be helpful for avoiding signal deterioration to complete LOS and postoperative vocal cord (VC) palsy.
Materials and methods
Patients
This retrospective study was conducted at a tertiary referral academic medical center, Kaohsiung Medical University Hospital, Taiwan. The data were collected from Oct. 2015 to Oct. 2019 regarding 664 patients (118 men and 546 women; ages ranging from 19 to 81 years; mean age, 50.9 years) who underwent operations for various thyroid diseases treated by the same surgeon (F.-Y. Chiang). There were 173 thyroid lobectomies and 491 total thyroidectomies (397 benign and 267 malignant thyroid diseases). Thirteen nerves were excluded from this study due to preoperative cord palsy (9 nerves) and intentional sacrifice due to cancer encasement (4 nerves). Thus, 1142 nerves at risk were enrolled in this study. (Figure 1)
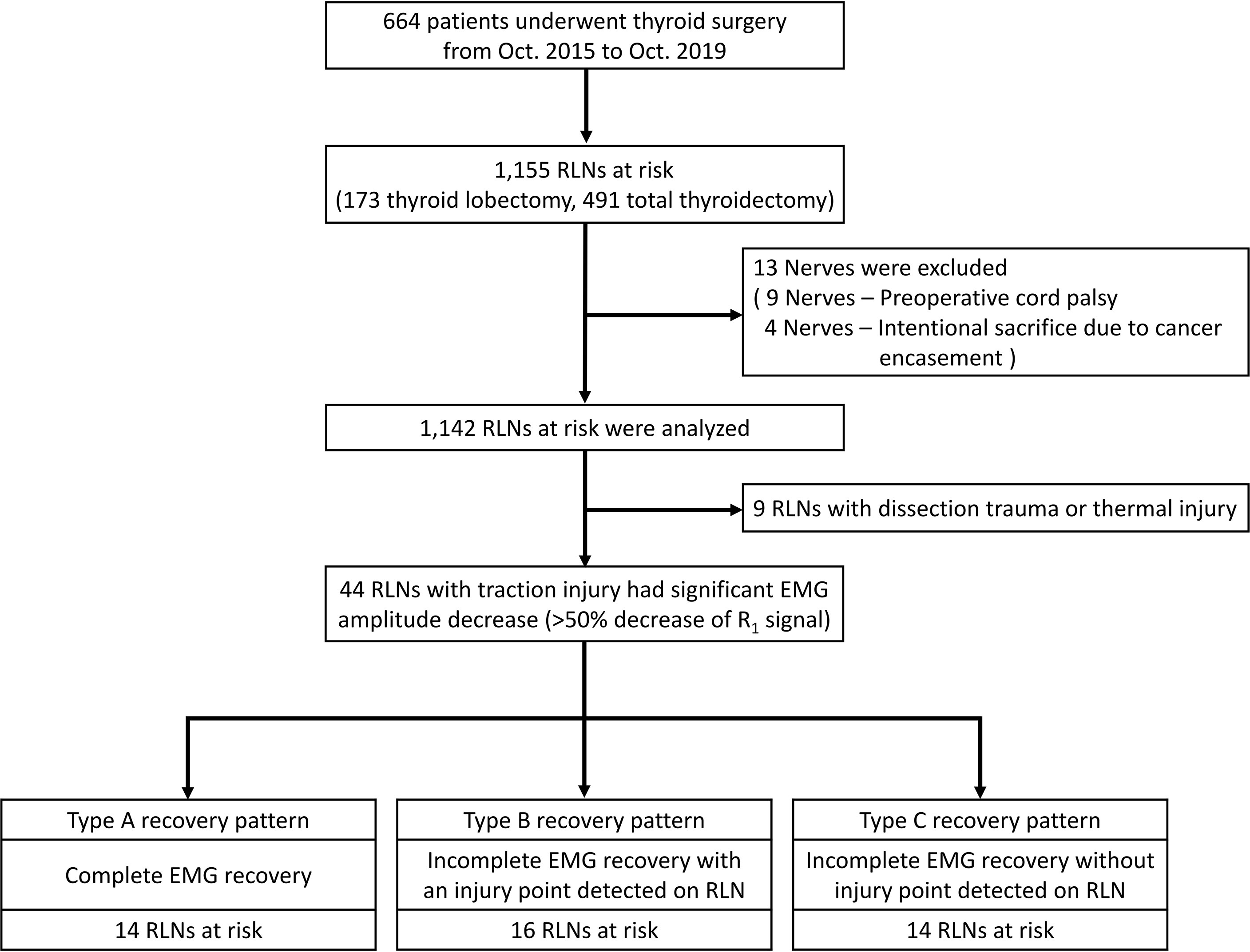
Figure 1 Flowchart of the procedure for the inclusion and exclusion of patients and the patterns, definitions, and patient distribution summarized from this study. RLN= Recurrent laryngeal nerve.
IONM setup and procedures
General anesthesia was induced with lidocaine (1 mg/kg), propofol (2–3 mg/kg), a single dose of rocuronium (0.3 mg/kg), and a bolus of fentanyl (50 μg) as necessary. Regular oral endotracheal tubes were used for all patients. Anesthesia was maintained with sevoflurane and propofol target-controlled infusion. We routinely elevated the skin flap to the upper level of the thyroid cartilage and dissected the pyramidal lobe and prelaryngeal lymph nodes to place the electrodes correctly. Two paired subdermal electrodes (length, 12.0 mm; diameter, 0.4 mm; Medtronic, Jacksonville, FL) were inserted into the subperichondrium of the middle thyroid lamina for EMG recording (Figure 2A). The conventional dissecting forceps was connected by the stimulation wire to use it as SDI (Figures 2B, C), which was used for vagus nerve (VN) and RLN stimulation.
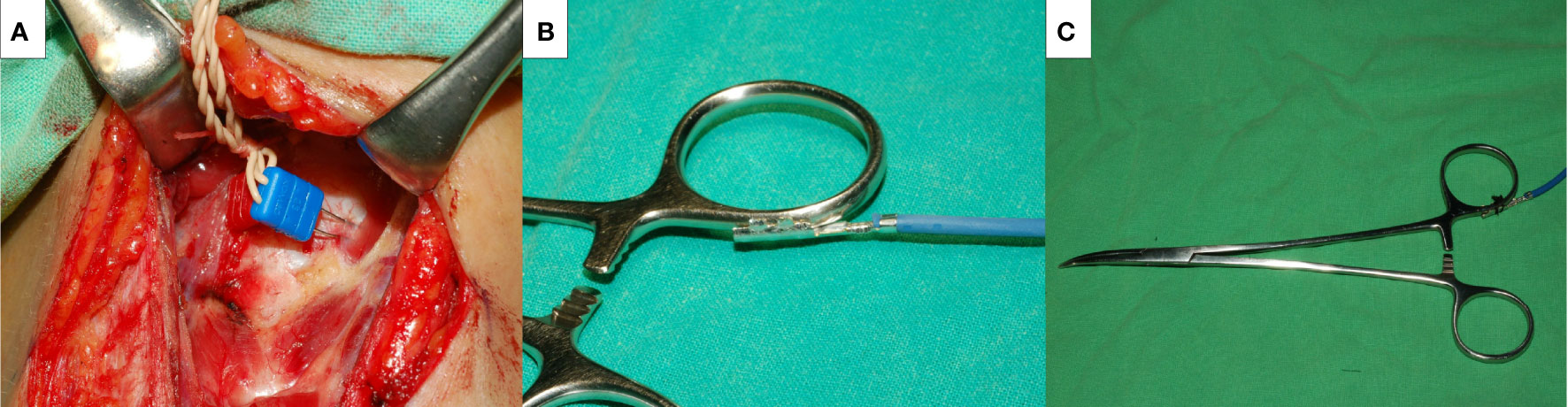
Figure 2 The novel intermittent intraoperative neuromonitoring method in this study. Medtronic two paired subdermal electrodes were inserted into the subperichondrium of the middle thyroid lamina for the EMG recording (A). A Medtronic stimulation wire was connected to the conventional dissecting forceps (B), and it became a stimulating dissecting instrument (C).
During the operation, standard IONM procedures (20) were strictly followed. The VN (without dissecting the carotid sheath to expose the VN) was stimulated with a 5-10 mA stimulus current before and after RLN dissection, and V1 and V2 signals were obtained. At the first identification, the RLN was stimulated with 3-5 mA, and the R1 signal was obtained. After complete RLN dissection, the exposed RLN was stimulated at the most proximal and distal ends near the laryngeal entry point, and R2p and R2d signals were obtained. The largest amplitudes of these five EMG signals (V1-R1-R2p-R2d-V2) were registered in all cases.
The EMG amplitude was monitored for changes during the phase of medial thyroid traction and RLN dissection. An EMG amplitude decrease >50% of the R1 signal was defined as a significant EMG amplitude decrease. When a significant EMG amplitude decrease was detected, the surgical maneuver was immediately stopped, and the mechanism (traction injury, thermal injury, and dissection trauma) of nerve injury was immediately determined and recovery of EMG amplitudes was closely monitored. Nerve dissection was restarted with gentle thyroid traction after the EMG amplitude recovered and reached a stable amplitude. A stable amplitude is defined as less than 10% amplitude change in one minute. Secondary significant EMG amplitude decreases can be observed and recorded in some nerves. After finishing RLN dissection, the amplitudes of R2p and R2d signals were compared. If the R2p/R2d ratio reduction was over 10%, the whole exposed RLN was mapped to identify the injured point with 1 mA. The difference in the R2p and R2d signals within ±10% is regarded as the normal variation in the monitoring system. Before closing the wound, we repeated the same procedure to confirm and record the final data.
LOS was defined as the absence of EMG signals (amplitude less than 100 µV) after nerve stimulation. Type 1 LOS was defined as a localized injury point detected on the RLN, and Type 2 LOS was defined as no injury point on the whole exposed RLN. All patients received pre- and postoperative video recordings of VC function. Symmetric VC mobility was regarded as a normal VC function. Weak VC mobility was defined as asymmetric VC movement (VC still moved and was approximated well). VC palsy was defined as fixation of VC mobility. When VC palsy was found, VC function was examined every 2 weeks initially and every 4 weeks after that until complete recovery was achieved. VC palsy was considered permanent if it persisted for more than 6 months postoperatively.
The study was approved by the Institutional Review Board (IRB) of Kaohsiung Medical University Hospital (KMUHIRB-E(I)-20210358).
Results
Forty-four of 1142 nerves (3.9%) exhibited a significant EMG amplitude decrease (>50% decrease in R1 signal) during RLN dissection, and all (100%) showed gradual progressive amplitude recovery within a few minutes after releasing thyroid traction (Figure 3). Ten nerves recovered from LOS, and another 34 nerves recovered from a 51-90% amplitude decrease.
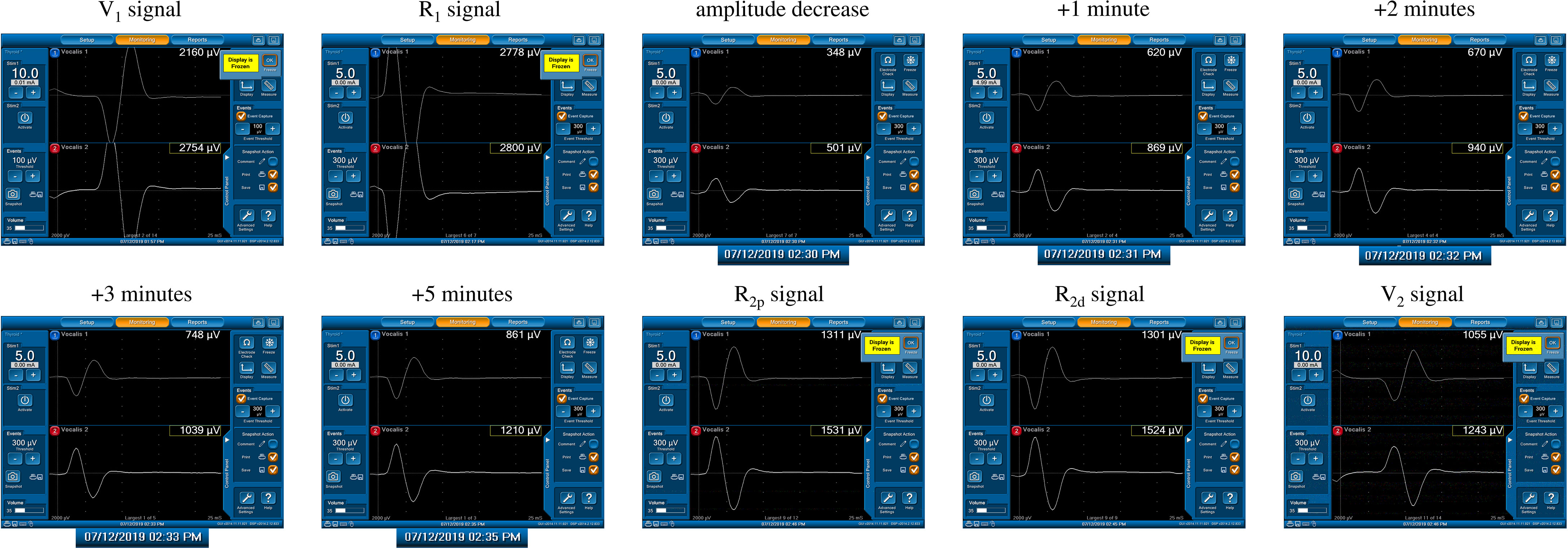
Figure 3 A significant amplitude decrease (channel 2) from 2800 µV (R1 signal) to 501 µV was detected. The actual operation time of the neuromonitoring screenshots is magnified and displayed below, according to amplitude decrease, +1, +2, +3, +5 minutes after amplitude decrease, respectively. Gradual and progressive amplitude recovery was found within a few minutes after releasing thyroid traction. The amplitudes between the R2p and R2d signals showed no difference, but there was a 66% amplitude decrease between the R2p and R1 signals. This is a case of a Type C recovery pattern after traction injury.
After restarting the surgical maneuver, a secondary significant EMG amplitude decrease occurred in 6 (13.6%) of 44 nerves. A substantial R2p/R2d ratio reduction (81% and 92%) in 2 nerves and unrecovered LOS in 4 nerves occurred due to inevitable repeated traction, and all 6 nerves developed temporary VC palsy.
Three types of EMG recovery patterns were summarized. Type A recovery pattern showed complete EMG amplitude recovery in 14 (32%) nerves; Type B showed incomplete recovery with an injury point detected on the RLN in 16 (36%) nerves. Type C showed incomplete recovery without an injury point detected in 14 (32%) nerves (Figure 1). Regarding Type A recovery pattern, 2 of 14 nerves had LOS during the surgical maneuver. No (0%) patient had postoperative weak or fixed VC mobility (Table 1). Of the nerves with the Type B recovery pattern, 6 of 16 nerves had LOS during the surgical maneuver. Seven (44%) patients had abnormal postoperative VC mobility, including 2 patients had weak VC mobility and 5 patients had fixed VC mobility. All 16 nerve injury points were located in the upper portion of exposed RLN (at the region of Berry’s ligament or near the laryngeal entry point) (Table 2). Of the nerves with Type C recovery pattern, 2 of 14 nerves had LOS during the surgical maneuver. Two (14%) patients had abnormal postoperative VC mobility, including one patient had weak VC mobility, and one patient had fixed VC mobility (Table 3).
In this study, a substantial EMG amplitude decrease was also found in 9 nerves caused by dissection trauma or thermal injury but without showing the feature of progressive recovery. Complete LOS was found in 5 nerves, and incomplete LOS was found in 4 nerves. Temporary VC palsy occurred in 7 nerves, and permanent VC palsy occurred in 2 nerve with thermal injury.
Discussion
RLN traction injury frequently occurs at the region of Berry’s ligament since the anatomical relationship between RLN, Berry’s ligament and surrounding vessels is highly variable (21–24) The RLN is located not only posterolateral to Berry’s ligament but also posteromedial to it, and the anterior motor branch of the RLN may penetrate the ligament. Additionally, blood vessels often intertwine with the RLN at the region of Berry’s ligament. The RLN can be overstretched during medial thyroid traction by Berry’s ligament or an intertwined vessel (Figures 4A–C).
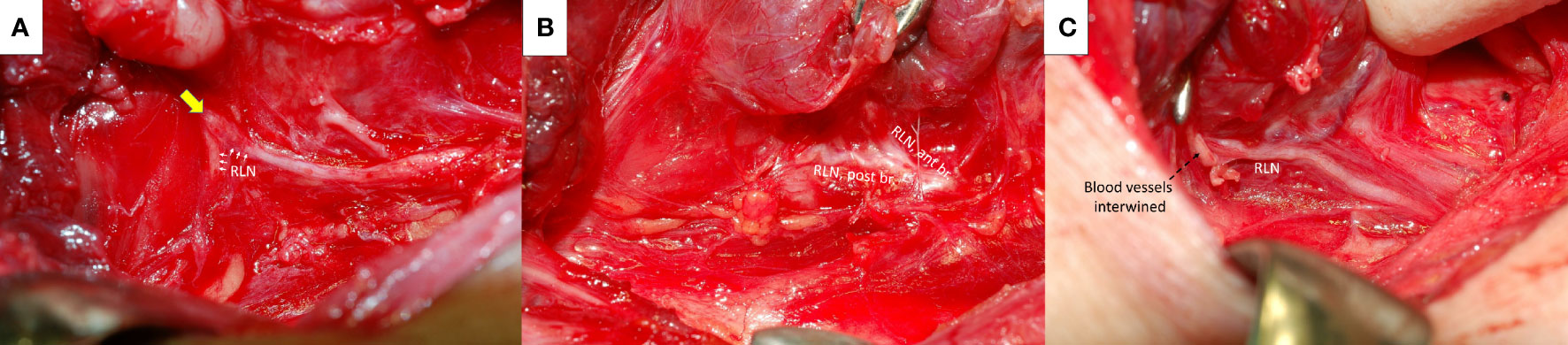
Figure 4 Recurrent laryngeal nerve (RLN) and Berry’s ligament. The RLN (white arrows) was severely stretched upward at the region of Berry’s ligament (yellow arrow) (A). The anterior motor branch of the RLN penetrates Berry’s ligament (B). A surrounding blood vessel intertwined (black dotted arrows) with the RLN at the region of Berry’s ligament (C). ant br., anterior branch; post br., posterior branch.
When applying C-IONM in thyroid surgery, a progressive decrease in EMG amplitude is the characteristic feature of traction distress on the RLN (11–14). Prolonged traction may result in unrecovered LOS and postoperative VC palsy. Therefore, the weakness of conventional I-IONM compared to C-IONM is that early detection of imminent RLN injury and relief of traction distress are less effective to avoid signals deteriorating to an unrecoverable level (13). In this study, SDI was used for nerve stimulation and tissue dissection. It is a simple and effective way to real-time monitor nerve function during the highest risk phase for RLN injury (25, 26). In our experience, SDI is not inferior to C-IONM in early detection of nerve injury, furthermore, C-IONM cannot replace I-IONM in locating the nerve injury point. Additionally, we chose the trans-thyroid cartilage recording method for EMG signal recording. It provides high initial EMG amplitudes during VN and RLN stimulation, and the amplitudes remain stable during the entire course of the operation (27–29). This technique solves the major limitation of the EMG tube recording method. A significant EMG amplitude decrease can also occur due to EMG tube displacement during surgical manipulation of the thyroid lobe or trachea. Using an EMG tube for signal recording make it difficult to tell an actual EMG amplitude decrease from a false one. In this study, applying these two novel IONM techniques, we detected 44 nerves with a significant EMG amplitude decrease during RLN dissection and gradual progressive amplitude recovery after releasing thyroid traction. The EMG signal recovers from LOS in 10 nerves and a 51-90% amplitude decrease in 34 nerves. The results suggest a high chance of signal recovery after a substantial amplitude decrease, even after LOS, if traction strain on the RLN was relieved in time. In cases with large tumors or complicated anatomical relationships between the RLN and Berry’s ligament, a secondary EMG amplitude decrease still occurred after restarting the surgical maneuver with gentle thyroid traction and careful nerve dissection due to unavoidable repeated traction. A persistent substantial amplitude decrease was found in 6 nerves after finishing RLN dissection (2 nerves with 81% and 92% R2p/R2d ratio reduction; 4 nerves with unrecovered LOS). All 6 nerves developed temporary VC palsy. The results indicate that the RLN is highly vulnerable to repetitive stretching.
From the results of the five EMG signals (V1-R1-R2p-R2d-V2) obtained from VN and RLN stimulation in this study, we found 3 types of EMG recovery patterns. Type A recovery showed complete EMG amplitude recovery, and the amplitudes of the R2p, R2d and R1 signals were similar (Table 1). Type B recovery showed incomplete EMG recovery when comparing R2p with R1 signals, and it was combined with an injury point on the RLN and a significant reduction in the R2p/R2d ratio (Table 2). Type C recovery showed incomplete EMG recovery, but without an injury point detected on the RLN (Table 3). In Type C recovery pattern, we can see that the amplitudes of the R2p and R2d signals are similar, but the amplitudes show a significant decrease compared with the R1 signal (Figure 3). Case No. 2 in Table 3 did not show a reduction of the R2p/R2d ratio. Still, it had a 66% amplitude decrease compared to the R2p and R1 signals (509 vs. 1515 µV), and this case developed weak VC mobility postoperatively. This kind of nerve injury was unrecognized when using the EMG tube recording method since the amplitude change would be mistaken for EMG tube displacement. Overall, different recovery patterns have different vocal cord function outcomes, to elucidate the recovery patterns can assist surgeons in the intraoperative decision making and postoperative management (i.e. speech therapy and injection laryngoplasty).
Regarding LOS after traction injury, Chiang (7) reported that nerve injuries caused by overstretching of Berry’s ligament could be divided into type 1 and type 2 stretch injuries. A type 1 stretch injury was caused by direct distress on the nerve and featured an injury point on the nerve. A type 2 stretch injury could be caused by pulling down the distal part of the RLN when the RLN was stretched excessively at the region of Berry’s ligament, and the nerve might be injured at a higher position above the laryngeal entry point. From the evidence of type B and type C EMG recovery patterns, we find that type 1 or type 2 nerve injury occurs not only in LOS (7, 9, 30) but also in incomplete LOS. In this study, there were 3 nerves with type 1 LOS and 1 nerve with type 2 LOS. In addition, there were 13 nerves with a type 1 incomplete LOS with a weak point of nerve conduction detected on the RLN (case No. 4-16 in Table 2) and 13 nerves with a type 2 incomplete LOS with no weak point detected (case No. 2-14 in Table 3).
In our clinical experience, some patients with very late recovery of muscle tone under neuromuscular blockade agent and the accuracy of the amplitudes of V1 and R1 may be questionable. In this study, the time from the administration of rocuronium (0.3 mg/kg), neck positioning, skin preparation to skin incision is around 20 minutes. And the time from skin incision, skin flap elevation, pyramidal dissection, superior thyroid pole dissection to V1 and R1 signals takes another 30 minutes, which is even longer. From the results of our patients without nerve injury, the data of V1-R1-R2p-R2d-V2 showed stable. It indicates that neuromuscular blockade has been almost completely recovered through 50 minutes. Therefore, the use of R1 signal as basic reference baseline should be reliable, although more studies are necessary to prove it.
Several limitations of this study were noted. First, this was an observational study and lacked a control group. Second, the comparisons between conventional I-IONM, SDI, and C-IONM were not studied. Furthermore, the combined SDI and C-IONM technique is an optimal procedure (least missed EMG changes) with distinct advantages that merit evaluation by a specific design study. Third, the recovery time might differ between type A, B, and C EMG recovery patterns in this study. When the nerve recovers quickly, the surgeon tends to wait and observe until the signal reaches a stable amplitude; when the nerve recovers slowly, the surgeon tends to perform surgical dissection of the contralateral or other regions first. Therefore, no exact recovery time can be provided in the current study, but a gentle thyroid traction and nerve dissection is strongly recommended after adverse EMG event. Fourth, the patient number of each recovery type is relatively small; however, the characteristics of each recovery type can be clearly shown in this study. Further large-scale studies in multiple centers are helpful for the analysis of the clinical presentation in the different recovery types.
Conclusion
A significant EMG amplitude decrease and progressive recovery after releasing thyroid traction is the characteristic feature of traction strain on the RLN during thyroid surgery. In this study, early detection and release of thyroid traction results in intraoperative EMG recovery and helps to avoid a signal deterioration to an unrecoverable LOS. Gentle traction of the thyroid lobe is recommended during the entire course of the operation, and frequent stimulation of the RLN is necessary for the early detection of EMG amplitude changes, particularly when using a stimulating probe for nerve stimulation. Overall, different recovery patterns result in different vocal cord function outcomes which elucidate that the recovery patterns can assist surgeons in the intraoperative decision making and postoperative management.
Data availability statement
The original contributions presented in the study are included in the article. Further inquiries can be directed to the corresponding authors.
Ethics statement
Ethical approval of this study was obtained from the Kaohsiung Medical University Hospital Institutional Review Board (KMUHIRB-E(I)-20210358). Written informed consent for participation was not required for this study in accordance with the national legislation and the institutional requirements.
Author contributions
Supervision – T-ZH, W-HY, C-WW, GD, and F-YC; Materials – C-FL, T-ZH, Y-CS, T-YH, and F-YC; Data Collection and Processing – K-LC, ChihCW, ChieCW , and Y-CS; Analysis and Interpretation – K-LC, C-FL, W-HVY, T-YH, and F-YC; Literature Search – K-LC, ChihCW, ChieCW , T-YH, and F-YC; Writing Manuscript – All authors. All authors have read and agreed to the published version of the manuscript.
Funding
This study was supported by grants from Kaohsiung Medical University Hospital, Kaohsiung Medical University (KMUH110-0R51), and Ministry of Science and Technology (MOST 110-2314-B-037-104-MY2, MOST 110-2314-B-037-120, MOST 111-2628-B-037-007), Taiwan.
Acknowledgments
The authors would like to thank all the patients included in this study.
Conflict of interest
The authors declare that the research was conducted in the absence of any commercial or financial relationships that could be construed as a potential conflict of interest.
Publisher’s note
All claims expressed in this article are solely those of the authors and do not necessarily represent those of their affiliated organizations, or those of the publisher, the editors and the reviewers. Any product that may be evaluated in this article, or claim that may be made by its manufacturer, is not guaranteed or endorsed by the publisher.
References
1. Karlan MS, Catz B, Dunkelman D, Uyeda RY, Gleischman S. A safe technique for thyroidectomy with complete nerve dissection and parathyroid preservation. Head Neck Surg (1984) 6(6):1014–9. doi: 10.1002/hed.2890060606
2. Jatzko GR, Lisborg PH, Müller M, Wette VM. Recurrent nerve palsy after thyroid operations–principal nerve identification and a literature review. Surgery (1994) 115(2):139–44.
3. Steurer M, Passler C, Denk DM, Schneider B, Niederle B, Bigenzahn W. Advantages of recurrent laryngeal nerve identification in thyroidectomy and parathyroidectomy and the importance of preoperative and postoperative laryngoscopic examination in more than 1000 nerves at risk. Laryngosc (2002) 112(1):124–33. doi: 10.1097/00005537-200201000-00022
4. Chiang F-Y, Wang L-F, Huang Y-F, Lee K-W, Kuo W-R. Recurrent laryngeal nerve palsy after thyroidectomy with routine identification of the recurrent laryngeal nerve. Surgery (2005) 137(3):342–7. doi: 10.1016/j.surg.2004.09.008
5. Snyder SK, Lairmore TC, Hendricks JC, Roberts JW. Elucidating mechanisms of recurrent laryngeal nerve injury during thyroidectomy and parathyroidectomy. J Am Coll Surg (2008) 206(1):123–30. doi: 10.1016/j.jamcollsurg.2007.07.017
6. Dionigi G, Wu C-W, Kim HY, Rausei S, Boni L, Chiang F-Y. Severity of recurrent laryngeal nerve injuries in thyroid surgery. World J Surg (2016) 40(6):1373–81. doi: 10.1007/s00268-016-3415-3
7. Chiang F-Y, Lu I-C, Kuo W-R, Lee K-W, Chang N-C, Wu C-W. The mechanism of recurrent laryngeal nerve injury during thyroid surgery—the application of intraoperative neuromonitoring. Surgery (2008) 143(6):743–9. doi: 10.1016/j.surg.2008.02.006
8. Liu M-Y, Chang C-P, Hung C-L, Hung C-J, Huang S-M. Traction injury of recurrent laryngeal nerve during thyroidectomy. World J Surg (2020) 44(2):402–7. doi: 10.1007/s00268-019-05178-6
9. Wu C-W, Hao M, Tian M, Dionigi G, Tufano RP, Kim HY, et al. Recurrent laryngeal nerve injury with incomplete loss of electromyography signal during monitored thyroidectomy—evaluation and outcome. Langenb Arch Surg (2017) 402(4):691–9. doi: 10.1007/s00423-016-1381-8
10. Liu N, Chen B, Li L, Zeng Q, Sheng L, Zhang B, et al. Mechanisms of recurrent laryngeal nerve injury near the nerve entry point during thyroid surgery: A retrospective cohort study. Int J Surg (2020) 83:125–30. doi: 10.1016/j.ijsu.2020.08.058
11. Wu C-W, Dionigi G, Sun H, Liu X, Kim HY, Hsiao P-J, et al. Intraoperative neuromonitoring for the early detection and prevention of rln traction injury in thyroid surgery: A porcine model. Surgery (2014) 155(2):329–39. doi: 10.1016/j.surg.2013.08.015
12. Brauckhoff K, Aas T, Biermann M, Husby P. EMG changes during continuous intraoperative neuromonitoring with sustained recurrent laryngeal nerve traction in a porcine model. Langenb Arch Surg (2017) 402(4):675–81. doi: 10.1007/s00423-016-1419-y
13. Schneider R, Randolph GW, Sekulla C, Phelan E, Thanh PN, Bucher M, et al. Continuous intraoperative vagus nerve stimulation for identification of imminent recurrent laryngeal nerve injury. Head Neck (2013) 35(11):1591–8. doi: 10.1002/hed.23187
14. Schneider R, Bures C, Lorenz K, Dralle H, Freissmuth M, Hermann M. Evolution of nerve injury with unexpected emg signal recovery in thyroid surgery using continuous intraoperative neuromonitoring. World J Surg (2013) 37(2):364–8. doi: 10.1007/s00268-012-1853-0
15. Ku D, Hui M, Cheung P, Chow O, Smith M, Riffat F, et al. Meta-analysis on continuous nerve monitoring in thyroidectomies. Head Neck (2021) 43(12):3966–78. doi: 10.1002/hed.26828
16. Kandil E, Mohsin K, Murcy MA, Randolph GW. Continuous vagal monitoring value in prevention of vocal cord paralysis following thyroid surgery. Laryngosc (2018) 128(10):2429–32. doi: 10.1002/lary.27144
17. Schneider R, Randolph GW, Barczynski M, Dionigi G, Wu C-W, Chiang F-Y, et al. Continuous intraoperative neural monitoring of the recurrent nerves in thyroid surgery: A quantum leap in technology. Gland Surg (2016) 5(6):607. doi: 10.21037/gs.2016.11.10
18. Barber SR, Liddy W, Kyriazidis N, Cinquepalmi M, Lin BM, Modi R, et al. Changes in electromyographic amplitudes but not latencies occur with endotracheal tube malpositioning during intraoperative monitoring for thyroid surgery: Implications for guidelines. Laryngosc (2017) 127(9):2182–8. doi: 10.1002/lary.26392
19. Zhang D, Wu C-W, Wang T, Zhao Y, Kim HY, Pino A, et al. Drawbacks of neural monitoring troubleshooting algorithms in transoral endoscopic thyroidectomy. Langenb Arch Surg (2021) 406(7):2433–40. doi: 10.1007/s00423-021-02217-6
20. Chiang F-Y, Lee K-W, Chen H-C, Chen H-Y, Lu I-C, Kuo W-R, et al. Standardization of intraoperative neuromonitoring of recurrent laryngeal nerve in thyroid operation. World J Surg (2010) 34(2):223–9. doi: 10.1007/s00268-009-0316-8
21. Yalçın B, Ozan H. Detailed investigation of the relationship between the inferior laryngeal nerve including laryngeal branches and ligament of berry. J Am Coll Surg (2006) 202(2):291–6. doi: 10.1016/j.jamcollsurg.2005.09.025
22. Yalcxin B. Anatomic configurations of the recurrent laryngeal nerve and inferior thyroid artery. Surgery (2006) 139(2):181–7. doi: 10.1016/j.surg.2005.06.035
23. John A, Etienne D, Klaassen Z, Shoja MM, Tubbs RS, Loukas M. Variations in the locations of the recurrent laryngeal nerve in relation to the ligament of berry. Am Surg (2012) 78(9):947–51. doi: 10.1177/000313481207800933
24. Chiang F-Y, Lu I-C, Chen H-C, Chen H-Y, Tsai C-J, Hsiao P-J, et al. Anatomical variations of recurrent laryngeal nerve during thyroid surgery: How to identify and handle the variations with intraoperative neuromonitoring. Kaohsiung J Med Sci (2010) 26(11):575–83. doi: 10.1016/S1607-551X(10)70089-9
25. Chiang FY, Lu IC, Chang PY, Sun H, Wang P, Lu XB, et al. Stimulating dissecting instruments during neuromonitoring of rln in thyroid surgery. Laryngosc (2015) 125(12):2832–7. doi: 10.1002/lary.25251
26. Sung E-S, Lee J-C, Shin S-C, Choi S-W, Jung D-W, Lee B-J. Development of a novel detachable magnetic nerve stimulator for intraoperative neuromonitoring. World J Surg (2018) 42(1):137–42. doi: 10.1007/s00268-017-4159-4
27. Chiang F-Y, Wu C-W, Chang P-Y, Wu S-H, Chen H-Y, Lin Y-C, et al. Trans-thyroid cartilage recording for neural monitoring of the recurrent laryngeal nerve in thyroid surgery. Laryngosc (2020) 130(4):E280–E3. doi: 10.1002/lary.28049
28. Liddy W, Lawson BR, Barber SR, Kamani D, Shama M, Soylu S, et al. Anterior laryngeal electrodes for recurrent laryngeal nerve monitoring during thyroid and parathyroid surgery: New expanded options for neural monitoring. Laryngosc (2018) 128(12):2910–5. doi: 10.1002/lary.27362
29. Chiang FY, Lu IC, Chang PY, Dionigi G, Randolph GW, Sun H, et al. Comparison of EMG signals recorded by surface electrodes on endotracheal tube and thyroid cartilage during monitored thyroidectomy. Kaohsiung J Med Sci (2017) 33(10):503–9. doi: 10.1016/j.kjms.2017.06.014
Keywords: recurrent laryngeal nerve (RLN), intraoperative neuromonitoring (IONM), thyroid surgery, electromyography (EMG), traction injury
Citation: Chiu K-L, Lien C-F, Wang C-C, Wang C-C, Hwang T-Z, Shih Y-C, Yu W-HV, Wu C-W, Dionigi G, Huang T-Y and Chiang F-Y (2022) Intraoperative EMG recovery patterns and outcomes after RLN traction-related amplitude decrease during monitored thyroidectomy. Front. Endocrinol. 13:888381. doi: 10.3389/fendo.2022.888381
Received: 02 March 2022; Accepted: 27 June 2022;
Published: 11 August 2022.
Edited by:
Kang Dae Lee, Kosin University Gospel Hospital, South KoreaReviewed by:
Byungjoo Lee, Pusan National University Hospital, South KoreaKyung Tae, Hanyang, University, South Korea
Yong Bae Ji, Hanyang University, South Korea
Copyright © 2022 Chiu, Lien, Wang, Wang, Hwang, Shih, Yu, Wu, Dionigi, Huang and Chiang. This is an open-access article distributed under the terms of the Creative Commons Attribution License (CC BY). The use, distribution or reproduction in other forums is permitted, provided the original author(s) and the copyright owner(s) are credited and that the original publication in this journal is cited, in accordance with accepted academic practice. No use, distribution or reproduction is permitted which does not comply with these terms.
*Correspondence: Tzu-Yen Huang, dHlodWFuZy5lbnRAZ21haWwuY29t; Feng-Yu Chiang, ZnljaGlhbmdAa211LmVkdS50dw==