- School of Agriculture and Environment M087, University of Western Australia Institute of Agriculture, The University of Western Australia, Crawley, WA, Australia
Phytoestrogens can impact on reproductive health due to their structural similarity to estradiol. Initially identified in sheep consuming estrogenic pasture, phytoestrogens are known to influence reproductive capacity in numerous species. Estrogenic pastures continue to persist in sheep production systems, yet there has been little headway in our understanding of the underlying mechanisms that link phytoestrogens with compromised reproduction in sheep. Here we review the known and postulated actions of phytoestrogens on reproduction, with particular focus on competitive binding with nuclear and non-nuclear estrogen receptors, modifications to the epigenome, and the downstream impacts on normal physiological function. The review examines the evidence that phytoestrogens cause reproductive dysfunction in both the sexes, and that outcomes depend on the developmental period when an individual is exposed to phytoestrogen.
Introduction
The endocrine disrupting effects of phytoestrogens has been of emerging interest in both animal production and human health. The full spectrum of effects on reproduction continue to be elucidated. Initially of interest in human reproduction, the impact of phytoestrogens on reproduction is becoming increasingly relevant in livestock production because several key pasture species are estrogenic. The effects of estrogenic pasture on livestock reproduction are a considerable economic and animal welfare issue.
Several species of subterranean clover (“sub clovers”) were incorporated into Australian livestock grazing systems in the 19th century (1, 2). These sub clovers were widely adopted due to their nutritive value as pasture legumes, their resilient characteristics such as the ability to remain dormant over many seasons, their lower requirement for fertilizer than other pasture species, and their persistence under grazing (1). As a result, sub clover pastures are well integrated into animal production systems in Australia. Many of those species are now recognized to have high levels of the isoflavone formononetin, which is metabolized to equol in the rumen. Equol causes a spectrum of moderate to severe functional and morphological changes to the ewe reproductive tract (3–5) that has been termed “clover disease”. Although it is suspected that clover disease continues to cause fertility and welfare issues in sheep, investigation into it has been dormant since the 1990’s.
The female has been the focus of livestock research into phytoestrogen effects on reproduction. Early investigations into the disruptive effects of estrogenic clover described masculinized genitalia, morphological changes to the cervix, and irregularities in the duration of the estrous cycle (3, 4, 6, 7). While there have been reports on the profound effects of phytoestrogens on male fertility in several species (8–10) and in vitro (11), there has been very little investigation into reproductive compromise in the ram.
The obvious solution to mitigate clover disease in sheep production is pasture renovation to eliminate the sub clover cultivars that are estrogenic. However, pasture renovation can take several years, and does not guarantee that estrogenic sub clover will not persist or re-emerge, given the resilient characteristics of this species (1).
Though the known presentation of clover disease has changed over time, with reduced severity compared to initial reports (4, 12, 13), an impact on sheep reproduction is likely still present (14). With the expansion of research into the impacts of other endocrine disruptors on reproduction (15, 16), new perspectives emerge on the potential mechanisms behind how estrogenic pasture influences on sheep reproduction. The first step toward prevention of the issue, therefore, is to define the severity, nature, and distribution of clover disease in commercial systems, to establish the mechanistic basis behind the phenomenon, and to increase awareness of the potential impacts of estrogenic sub clover within the industry. Accordingly, this review examines phytoestrogens as endocrine disruptors in sheep reproduction, with reference to known and postulated mechanisms of phytoestrogens in both the sexes.
Ovine Clover Disease: The First Evidence for Phytoestrogens as Endocrine Disruptors in Sheep
Ovine clover disease was first detected and described in Western Australia in the 1940’s (17). Decades later, the disease was attributed to the high formononetin content of several cultivars of Trifolium subterraneum, a common pasture legume in sheep production systems in Australia (3, 4, 6, 7, 18). In the rumen, formononetin is converted to the estrogenic metabolite equol, causing masculinized genitalia, morphological changes to the cervix (Figures 1A, B), and irregularities in the duration of the estrous cycle (3, 4, 6, 7). These changes are accompanied by a reduction in the fertility rate, an increase in dystocia, an increase in the number of stillborn lambs, and an increase in the incidence of uterine prolapse after parturition (3, 17–19). Though these studies were critical in identifying the role of estrogenic sub clover in the compromise of sheep reproduction, the mechanisms behind the disease are not fully understood. Though the severe manifestations of clover disease appear to have been eradicated, anecdotal reports indicate that more subtle manifestations of sub-fertility have persisted when sheep graze on sub clover cultivars that are estrogenic.
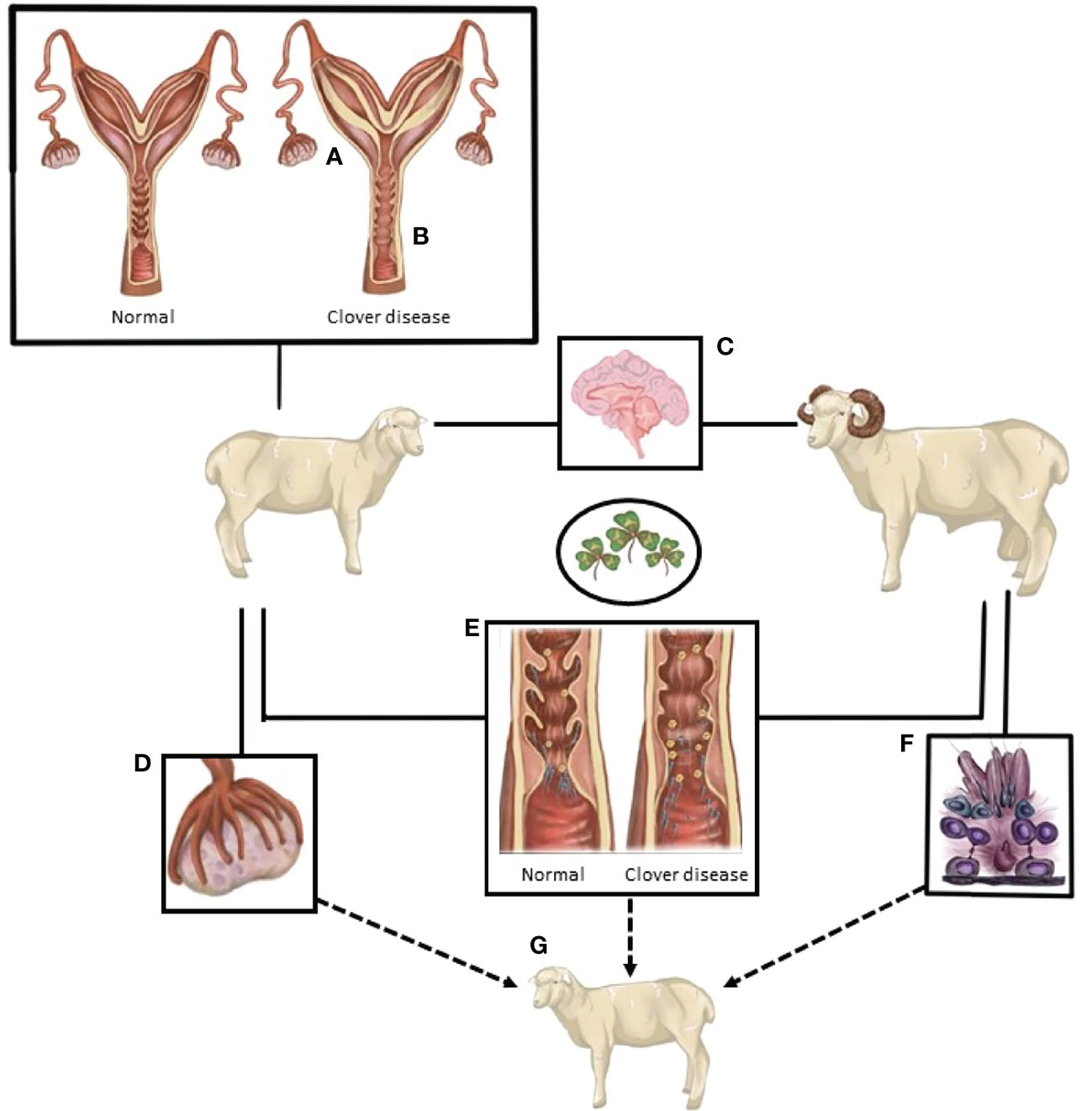
Figure 1 A summary of the known and postulated sites of action of compounds from oestrogenic pasture that lead to compromised sheep reproduction. (A) Endometrial thickening/edema. (B) Loss of cervical folds. (C) Excessive oestrogen-like actions in the neuroendocrine control of reproduction. (D) Follicle development, quality and potentially ovulatory ability are reduced. (E) The interaction between spermatozoa and the female tract is altered. Loss of cervical crypts, changes in mucus composition, and consistency and changes in the female immune response hinder sperm navigation of the female tract. (F) Sperm production and quality is potentially reduced. (G) Exposure of both male and female gametes, and the ovine embryo, may cause differential developmental programming of the subsequent generation.
Despite evidence that clover disease remains an issue in Australian sheep production systems (14), there has been little progress toward understanding the disease or developing solutions that will mitigate the impact on ovine fertility. Investigations in other species have revealed that phytoestrogens operate via diverse pathways, including competitive binding to nuclear estrogen receptors, that can result in both agonistic and antagonistic actions, as well as by modifying the epigenome. While these studies in other species confirm that exposure to phytoestrogens has detrimental effects on reproduction, particularly during some sensitive developmental periods, there remains a lack of comprehensive evidence on the causal pathways.
A Brief Overview of Phytoestrogens and their Predicted Modes of Action
New additions continue to be made regularly to the spectrum of plant-derived estrogenic compounds that are known broadly as phytoestrogens. The predominant classes of phytoestrogens are isoflavones (daidzein, formononetin, genistein, glycitein), flavones (luteolin), flavonoids (quercetin, kaempferol), coumestans (coumestrol), stilbenes (resveratrol), and lignans (lariciresinol, matairesinol, pinoresinol, secoisolariciresinol) (20, 21). When they are consumed by an animal, many of these compounds can be further metabolized by the gut microflora into estrogenic metabolites. An example of particular relevance is the estrogenic by-product equol, resulting from the breakdown of formononetin in the mammalian digestive tract (22, 23).
The mechanism(s) that link phytoestrogens and reproduction remain controversial, though several key pathways have been proposed via which estrogenic compounds could operate. Perhaps the most discussed pathway is the interaction of phytoestrogens with estrogen receptors, albeit with a far lower binding affinity than the endogenous ligand, 17‐β‐estradiol (24, 25). In vertebrates, there are two main subtypes of nuclear estrogen receptor, ERα and ERβ (which are comprehensively reviewed by 26). The biological functions of the two receptor subtypes vary, as does their distribution between tissues, between the sexes, and between species (27–29). When they are activated by a ligand, the nuclear receptors ERα and ERβ are thought to act through either direct genomic modulation or indirect signaling. In the former, when ligand binds to the estrogen-receptor, the receptor dimerizes and binds to estrogen response elements in the promoter region of specific genes, thereby stimulating or repressing the transcription of that gene (30, 31). Indirect signaling leads to changes in transcription without a direct interaction with DNA (32, 33). Importantly, phytoestrogens not only competitively bind with ERα and ERβ, but can promote transcription from this interaction (34, 35).
Many of estrogens actions occur after stimulation of ERα. Stimulation of ERα is known to induce cellular proliferation, including the priming of the uterus via uterine cell production (36) and cancer progression, particularly in breast tissue, reproductive tissues, and bone (37–39). Estrogen- ERα binding is also able to induce estrogen-neuroendocrine feedback in the hypothalamus (40, 41), and increase transcription in most cell types (42). While ERβ is often co-expressed with ERα (26, 43), the stimulation of ERβ is thought to oppose the actions of ERα on gene expression (26, 31, 44–46). Thus, the outcome of estrogen stimulation for a particular cell will depend on the relative expression of ERα and ERβ. Both subtypes are involved in glucose (47–49) and lipid (50, 51) homeostasis in the brain, liver, pancreas, heart, and skeletal muscle (52). Most phytoestrogens have a higher binding affinity for ERβ than ERα (53), though that bias does not clearly translate to predictable physiological outcomes in vivo in mammalian systems. Notably, the expression of both receptor subtypes is increased by increased estrogen exposure (26), which may be a crucial mechanism behind the cumulative pathology resulting from long-term phytoestrogen exposure (3, 6). In the context of estrogenic pasture, an increase in receptor expression could underly the clinical manifestations, whereby long-term phytoestrogen exposure confers permanent infertility in the ewe, rather than the transient infertility that is observed with short-term exposure.
In addition to actions mediated by ERα and ERβ, phytoestrogens can interact with the G protein-coupled estrogen receptor (GPER). While ERα and ERβ are nuclear receptors, and therefore require their ligands to access the nucleus, GPER is a membrane associated receptor that is distributed across a multitude of tissues, at least in humans and rodents (54–57). When it binds ligand, GPER operates through non-genomic signaling, a comprehensive discussion of which can be found in a recent review by Luo and Liu (54). Of note, GPER is present in the reproductive tissues of both sexes, including breast tissue (58–60), the testis (61), prostate (62), ovary (63, 64), and endometrium (65, 66). Exogenous estrogenic compounds, including genistein, bisphenol A, and zearalenone, have a similar binding affinity for GPER as they do for the nuclear estrogen receptors (67). Of interest, some phytoestrogens, including equol and genistein, have been reported to have a greater binding affinity for GPER compared to 17β-estradiol (68). Thus, these extra-nuclear estrogen receptors may be a significant pathway through which exogenous estrogens can exert endocrine-disruptive effects through non-genomic, or indirect transcriptional pathways.
Phytoestrogens could also alter reproductive function via an indirect effect on genome transcription (69) that is often referred to as organizational effects. Modifications to the epigenome, such as DNA methylation, non-coding RNA’s, and histone modification, can influence the expression of the genome without altering the DNA sequence (70, 71). There is evidence that endocrine disrupting agents, and specifically exogenous estrogenic compounds, alter the epigenome in several tissues. For example, in neonatal rodents, coumestrol, genistein, and the estrogenic metabolite equol induce hypermethylation in several regions of the genome, including tissue-specific alterations in the uterus, kidney, and pancreas (72–76), as well as broadly increasing DNA methylation in the epigenome (73), in proto-oncogenes (72), and in dermal tissue (74). In addition to the endocrine disrupting actions of phytoestrogens, it is likely that in utero exposure to estrogenic compounds is detrimental to reproductive outcomes, given that gestation is an important period of epigenetic remodeling. Phytoestrogens can cross the blood-placenta barrier and infiltrate fetal tissues, including the brain (77, 78). To date, the phenotypic consequences of the epigenetic effects of phytoestrogen exposure have not been investigated.
It is therefore possible that epigenetic modifications that follow phytoestrogen exposure will have biologically relevant effects on the ovine reproductive system. Epigenetic changes that are induced by environmental factors, such as by assisted reproductive technologies and climate, are known to modify sperm function (79, 80), reproductive success in both male and female (81, 82), and disease onset (83) across a range of mammalian species. Moreover, these modifications can persist through generations (83, 84). Targeted and broad epigenetic modifications can influence the clinical severity of other reproductive pathologies, such as varicocele (85), polycystic ovary syndrome (86), and endometriosis (87). Theoretically, epigenetic changes in the reproductive system of sheep after exposure to phyto-estrogens could be at least partially responsible for the wide variation in clinical manifestations of clover disease, and could account for the persistence of flock sub-fertility even after sheep are removed from clover pasture.
Thus, the effects of phytoestrogens can be exerted via several mechanisms and pathways. An understanding of the systemic and direct mechanisms that lead from phytoestrogen exposure to reproductive pathology will be essential to find solutions. The developmental period during which an individual is exposed to phytoestrogens is likely critical in the extent of reproductive dysfunction. Developmentally sensitive periods include uterine life, neonatal life, prior to and during puberty, and times when estrogen has an essential biological role, such as during the estrous cycle.
The Timing of Phytoestrogen Exposure Is Critical for Reproductive Outcomes
Maternal Exposure to Phytoestrogens During Pregnancy, in the Neonate, and Prior to the Onset of Puberty
Beneficial and detrimental outcomes on female fertility after exposure to phytoestrogens have been reported. That apparent contradiction is probably due to the timing, duration, and level of phytoestrogen exposure, with consequences dependent on whether the individual is exposed in utero, during the neonatal and prepubertal periods, or during adult life.
The widespread use of soy-based infant formula, which is now known to contain physiologically relevant concentrations of the isoflavones genistein and genistin, has stimulated research on phytoestrogen exposure during key developmental periods in humans, and to a much larger extent in rodents (88, 89). Infants that consume soy-based formula have circulating levels of genistein that are 13,000 to 68,000-fold higher than the normal biological concentration of estradiol in non-exposed infants in the same age bracket (88). While the long term effects of phytoestrogen exposure in human infants are not known, in utero and neonatal exposure to genistein in rodents induces morphological alterations in the reproductive tract, including precocious vaginal opening (90), ovarian follicle atresia (91), increased uterine fluid content (92), and hyperplasia of the endometrium (92–94).
Given those outcomes in humans and rodents, it is plausible that phytoestrogen exposure during the pre-pubertal period could alter the onset of puberty in the ewe-lamb. The onset of puberty in the ewe requires considerable modulation of the hypothalamic-hypophyseal-gonadal axis and is subject to complex interactions between endocrine, metabolic, and genetic pathways (95–97). Prior to the change in amplitude and frequency of the secretion of gonadotropin-releasing hormone (GnRH) from the hypothalamus that defines the initiation of ovine puberty, the hypothalamus of the pre-pubertal ewe is highly sensitive to negative feedback from estradiol. Estradiol, produced primarily from the pre-pubertal ovary, inhibits GnRH secretion and the release of follicle stimulating hormone (FSH) and luteinizing hormone (LH), without which ovulation does not occur. Phytoestrogens, such as equol, may exert a similar inhibitory effect in the ewe-lamb, particularly when it is present in combination with other factors that are known to delay puberty onset, such as poor nutrition, inadequate exposure to short-day photoperiod patterns, or a lack of social interaction (98).
Comparatively, in juvenile rodents, the effects of phytoestrogens on markers of puberty onset appear to be inconsistent, and depend on dosage (99, 100). At concentrations comparable with low level dietary exposure, genistein induces hyperplasia of mammary tissue in the pre-pubertal rat, but at concentrations comparable with high level dietary exposure reduce both alveolar development (99, 100) and the sensitivity of mammary tissue/gland to estradiol (101). In rodents, in cases where sexual maturity is advanced by phytoestrogen exposure, the estrous cycles are very irregular at the commencement of puberty (102–104). While the mechanism that leads to the oligomenorrhea is not known, several studies support the notion that pre-pubertal exposure to estrogen-like compounds, including genistein, alters hypothalamic kisspeptin expression and leads to a lower density of kisspeptin expressing neurons and fibers in the anteroventral periventricular and arcuate nuclei (102, 105). Kisspeptin has a well-established role in the timing of both puberty and the estrous cycle, playing a critical role in estrogen feedback to the hypothalamus. Because GnRH neurons do not express estrogen receptors, the mechanism of steroid feedback remained a mystery until the discovery of kisspeptin (106). The discovery that kisspeptin neurons do express estrogen receptors, and that substances released from kisspeptin neurons (including kisspeptin, neurokinin B, and dynorphin) can stimulate GnRH neurons, provided a mechanism for steroid feedback. Any lowering of the activity of the kisspeptin signaling pathway can compromise the normal steroid feedback to GnRH neurons (107–111). Theoretically, a decrease in the stimulation of GnRH neurons by kisspeptin could account for the delayed pubertal onset and impaired ovulation that is observed after neonatal phytoestrogen exposure (4, 102, 112–116). Investigation of the neuronal circuitry that leads to GnRH release in phytoestrogen-exposed ewe lambs may shed further light on not only whether puberty is physiologically delayed, but also whether there are more permanent effects on the hypothalamic-pituitary-gonadal axis.
In rats, phytoestrogen exposure during the fetal and early neonatal stage is associated with long term alterations to the estrous cycle after the exposed individuals reach puberty, with prolonged suppression of ovarian cycling (117) and inhibition of LH secretion (118). Neonatal exposure to genistein might create a more hostile uterine environment that is less capable of supporting embryo implantation (103, 119). In addition to these reproductive consequences, in utero exposure to estrogenic compounds also impairs immune function in rats (120), increases the incidence of cancerous lesions in the uterus (121, 122), and impairs the development of estrogen-sensitive organs, particular secondary sex organs (123, 124).
Phytoestrogens Have Conflicting Effects on Reproductive Function in Adult Females
The effects of phytoestrogen exposure in the adult female remain unclear, with a spectrum of effects reported across species, ranging from beneficial to injurious. Adult mice exposed to estrogen-like compounds, for example, develop irregular estrous cycles, with more atretic follicles and an absence of corpora lutea (90). In women, dietary isoflavone intake is associated with an abnormally short luteal phase (125). More broadly across species, phytoestrogens have been linked to reproductive abnormalities, including an increase in uterine weight in cheetahs (126), reduced fertility in rhinoceros (127), and altered ova composition and estrous cyclicity in several bird species (112). In the context of clover disease, morphological changes in the follicles, along with reduced fertility, have been observed in mature ewes grazing estrogenic pasture, as outlined further below. Briefly, exposed ewes present excessive numbers of small to medium ovarian follicles with inadequate antrum formation, increased uterine fluid and subacute inflammation of the endometrium, and a reduction in the depth of cervical crypts and cervical squamous metaplasia (3, 6, 13, 128).
In contrast, in adult humans, isoflavone has been reported to have beneficial effects on fertility. In preconception cohort studies, higher intake of isoflavone was associated with improved fecundity and fertility (129, 130) and the re-instatement of ovulation in anovulatory cycles (131). In women with polycystic ovarian syndrome, supplementation with phytoestrogen extract of Cimicifuga racimosa increased plasma progesterone and endometrial thickness, suggesting that the phytoestrogens facilitated ovulation (132). It should be noted that the metabolites that are produced from phytoestrogens vary between ruminant and monogastric species, which may partly account for some of the species-specific outcomes of exposure to phytoestrogens. Several isoflavones, and their metabolites, have been suggested to have antioxidant effects and to mitigate inflammation (for an extensive review, see 133). These properties are likely responsible for the beneficial effects of isoflavones in cancer models (134) and in some cases, improvements in fertility. Reactive oxygen species (ROS) have a dichotomous role in reproduction, whereby particular levels of ROS are necessary for the processes of oocyte maturation, ovulation (135), and for spermatozoa to undergo capacitation and the acrosome reaction (136). Yet excessive levels of ROS are detrimental to gamete function and quality (136–138). In many cases, supplementation with antioxidants can improve gamete function and fertility in vivo (139, 140) and in vitro (141–144). Because phytoestrogens are known to reduce pro-inflammatory cytokines in experimental models of encephalitis (145, 146), any modulation of the immune response by phytoestrogens could either promote or inhibit normal reproductive function. In the female for example, non-specific inflammation can alter endometrial receptivity, impair tissue repair and remodeling, and affect trophoblast-endometrial interaction (147, 148).
A Meta-Analysis of the Effect of Phytoestrogen Exposure on Ovulation in Sexually Mature Females
It is clear that the impact of phytoestrogens on reproduction varies between studies, and that that variability is probably caused by differences in the dose of phytoestrogens and the timing of delivery. To tease apart the factors that determine the outcomes of phytoestrogen exposure, we performed a meta-analysis to clarify the impact of phytoestrogens on ovulation. Our logic was that ovulation is a key determinant of female reproductive performance. Major databases such as Pubmed, Cochrane Library, Google Scholar, and ResearchGate were searched using combinations of the following keywords: phytoestrogens, ovulation, ovary, sheep, clover, clover disease, isoflavones, coumestans, soy (i.e., not all terms were included in every search). The search included articles with at least an abstract in English. The end date of the database search was October 2020. Twenty-five studies were initially selected when the abstract indicated that the data would be relevant. Seven studies were selected for analysis after we applied the inclusion and exclusion criteria that are listed in Table 1, and the information and data extracted are listed in Table 2.
Statistical Analysis
The results of each study were considered as binary (ovulation or no ovulation), and the odds ratio was used as the measure of effect size. A random effect model, with the DerSimonian-Laird (DSL) method (155), was used to evaluate the effect of phytoestrogens on ovulation occurrence because the conditions differed between studies. R studio software (156) was used for this meta-analysis, with the packages rmeta and meta and the function meta.DSL. For odds ratios, computations were carried out on a log scale to maintain symmetry in the analysis. Visualization of the results was done with the functions summary and plot, which were used to confirm heterogeneity, the individual odds ratios, and confidence intervals. A Funnel plot was created to evaluate publication bias, with a test for funnel plot asymmetry, based on rank correlation or linear regression methods (function metabias).
Results
The number of animals in the studies ranged from 4 to 99. Laparoscopy was the most common method used to assess whether ovulation had occurred [one outcome per study, with the exceptionof Smith et al. (114)]. Four of the studies were conducted in sheep, three of which were conducted in Western Australia (7, 114, 149) and the fourth in Egypt (150). There was no bias across the included studies (P = 0.8349, Figure 2).
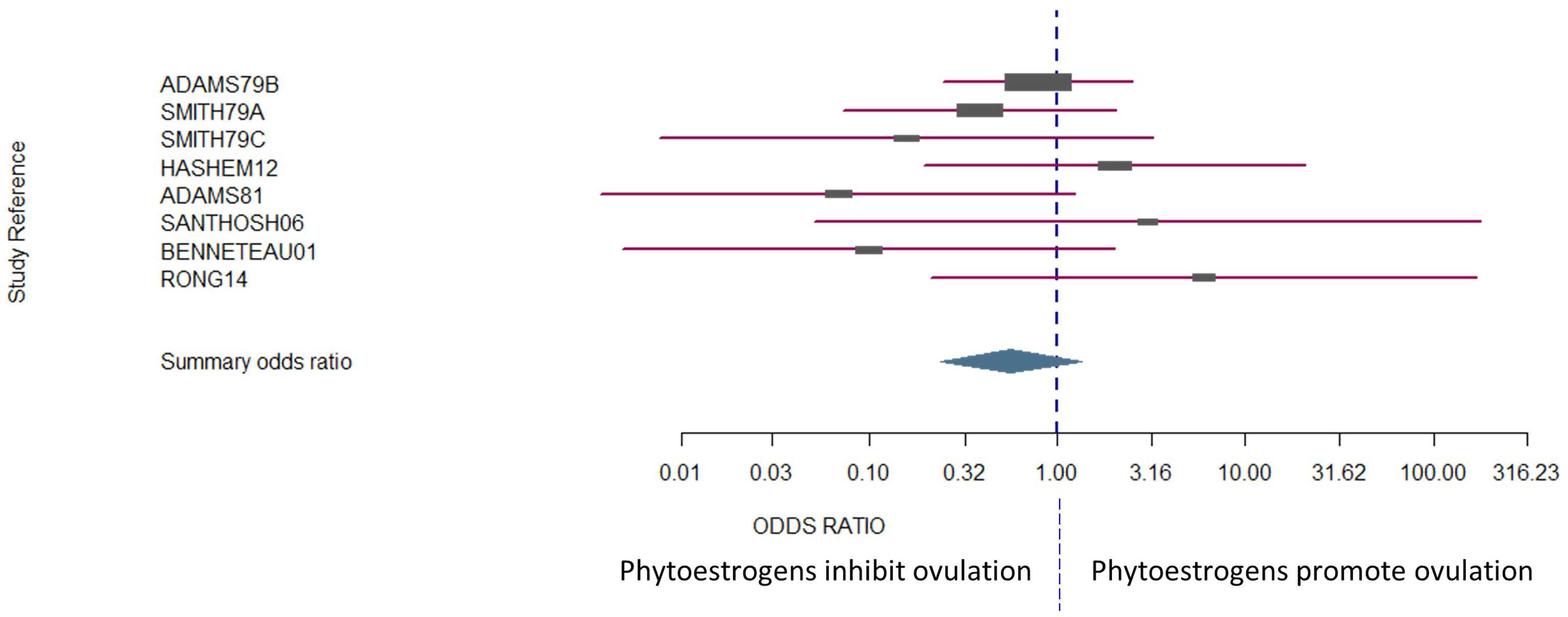
Figure 2 The confidence interval for each study is given by a horizontal line, and the point estimate is given by a square whose height is inversely proportional to the standard error of the estimate. The summary odds ratio is represented by a diamond with horizontal limits at the confidence limits and width inversely proportional to its standard error (R documentation). An odds ratio higher than 1 means that the treatment is more effective than the control and vice versa (whereby 1 means null effect). In the present analysis, the positioning of the summary odds ratio shows that phytoestrogen exposure in sexually mature females inhibits ovulation.
The results exhibited a large heterogeneity between studies (Figure 2), which can be primarily explained by differences in study design, including mainly species and sample size. However, the Woolf’s test for heterogeneity (X2) returned a p-value of 0.3157, and a between-studies variance of 0.23. Confidence intervals were notably wide, particularly for the studies that had fewer subjects in each experimental group (Figure 2).
Conclusions
It can be concluded that, depending on species, there remain contradictions around the impact of phytoestrogens on ovulation. It may be proposed that phytoestrogens inhibit ovulation in sheep, because that outcome seemed to emerge clearly from the data in the largest and the most statistically powerful of the studies. Interestingly, other phytoestrogens, non-flavonoids, have been reported to induce ovulation in women with polycystic ovarian syndrome or with anovulatory cycles (132). The impact of phytoestrogen on ovulation will be clarified only by further studies that are designed to control other external factors that can affect ovulation, such as metabolic status (157, 158).
Male Reproductive Function
Estrogen was once considered a female hormone, but evidence over the past few decades has shown that it is critical for normal male reproductive development and function. In fact, estrogen appears to be essential for normal male reproductive function because mice with the ERα gene knocked out are sterile (159–161). Negative feedback from estrogen contributes to the activity of the hypothalamic-pituitary axis (162), and estrogen signaling plays a role in fluid reabsorption in the epididymis (163). Less is known about the effect of exogenous estrogenic compounds in the male than in the female, though there are several potential mechanisms for these agents to impact on the spermatogenic cycle. In primates and mice, ERβ is present in Leydig cells, Sertoli cells, and in the nuclei of epithelial and stromal cells throughout the reproductive tract, while ERα seems primarily to be present in the accessory sex glands and efferent ductules (164, 165). The disruption of ERα in male mice results in compromised dynamics of fluid resorption in the efferent ductules, an increase in the secretion of chloride ions into seminal fluid, an increase in abnormal sperm morphology, an inhibition of sodium transport in the testis and ultimately reduced fertility (159, 160, 163). Phytoestrogens that can bind to ER receptors would therefore be expected to alter testicular function, sperm production, and sperm quality.
In man, phytoestrogen exposure has a spectrum of effects (Table 3). Although the effects are generally more subtle than the marked changes in females (4, 120, 126), those effects again depend on timing, with exposure during sensitive developmental periods, particularly in utero, leading to compromised reproductive function. Maternal isoflavone intake has been linked to hypospadias in male infants (182, 183) and lower testicular steroidogenic activity in neonatal rats (178). Similarly, as for the female, in the male conflicting results have been reported for the effects of phytoestrogen exposure during adult life. Several studies have been reported no effect in humans (166, 170), rodents (184–186), and rabbits (187), while other studies in the same species have associated phytoestrogen exposure during adult life with decreased sperm production, lower blood testosterone concentration, and reduced testicular weight (167, 168, 173, 174, 188, 189). These conflicting results can probably be attributed to several differences between studies, including phytoestrogen dosage, route of administration, length of exposure and, in the case of human studies, demographic biases including variation in age, ethnicity, and systemic health. Moreover, none of the studies reported the concentration of phytoestrogens in the seminal plasma. Thus it is not clear to what degree these compounds can infiltrate the reproductive tract or whether other indirect pathways are involved.
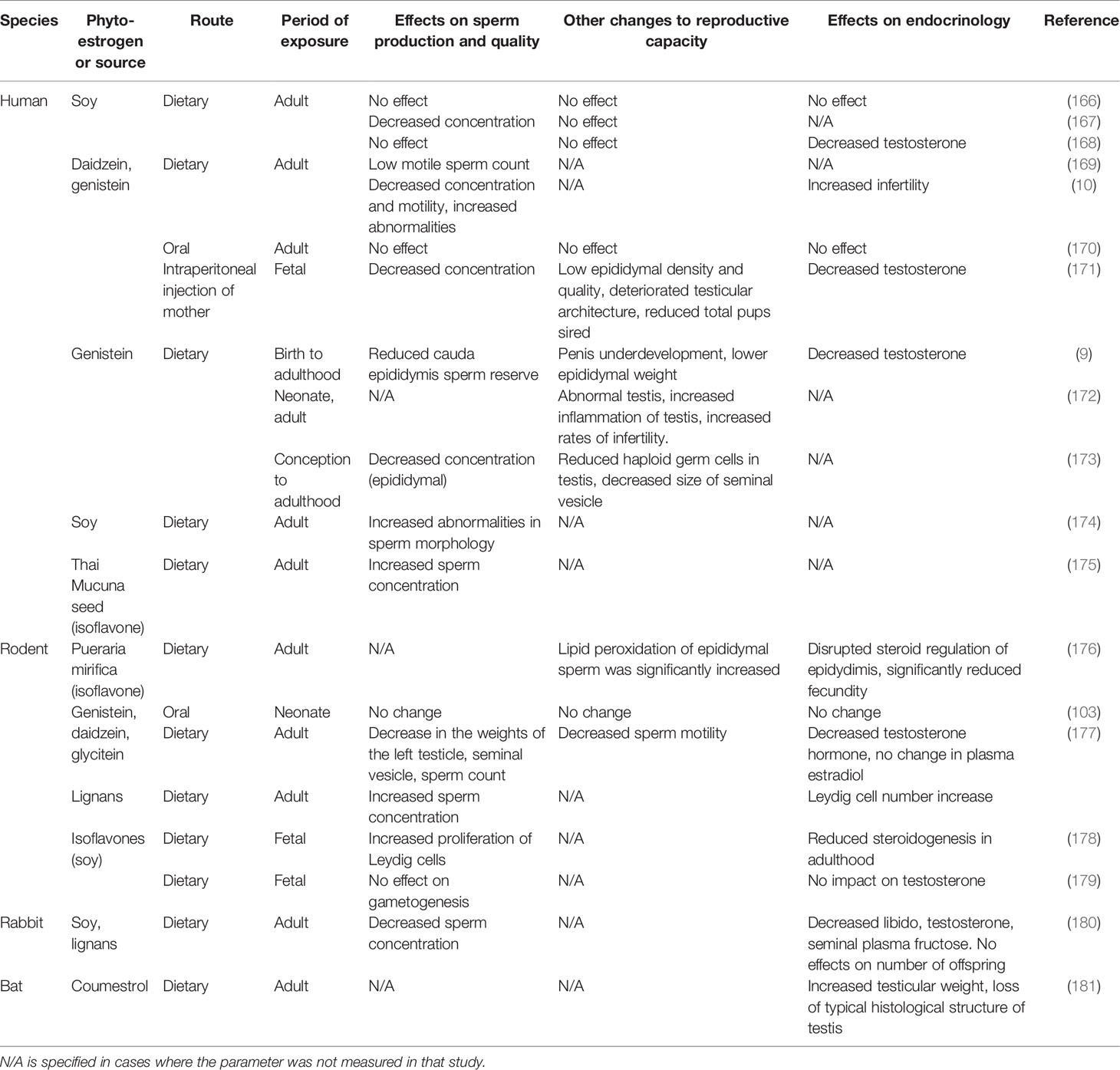
Table 3 The effect of phytoestrogen exposure on male reproductive function across several species and routes of exposure.
While the impact of phytoestrogen exposure on male reproduction is poorly understood, in vitro work suggests several isoflavones and flavonoids can influence the functionality of spermatozoa. The exposure of ram spermatozoa to physiological concentrations of equol in vitro decreases sperm motility, increases ROS, increases membrane fluidity, and increases DNA fragmentation (11). Genistein (8, 190, 191) and myricetin (192) have been reported to cause premature capacitation and acrosome loss in human, boar, and mouse spermatozoa, and inhibit the acrosome reaction in bull spermatozoa. In contrast, other studies have reported that the inclusion of isoflavones in vitro improves sperm viability and function (193–195). One possible confounding factor is that several isoflavones, including the widely tested genistein, can act as an antioxidant and mitigate oxidative stress (196, 197), thus improving sperm function. It is possible that the benefits of the antioxidant action can, at certain concentrations, mitigate any negative impacts from the estrogen-like actions of phytoestrogens.
Clover Disease: Have We Overlooked a Detrimental Impact on the Interaction Between Spermatozoa and the Female Reproductive Tract?
Despite its categorization as a female oriented issue, mounting evidence suggests that the subfertility and infertility that is associated with clover disease is likely far more complex. Since a 1960’s report that wethers on estrogenic pasture exhibited mammary development, lactation, and pathology of the bulbourethral glands (198), there is little investigation into the impact of phytoestrogens on ram reproduction. Similarly, there is no information on how ram spermatozoa perform in the female tract after phytoestrogen exposure in either, or both, sexes. The profound effects of phytoestrogens on male fertility in other species (Table 3) suggests strongly that phytoestrogen impact on ram reproductive function may be a critically overlooked component of clover disease. Of note, human males exposed to estrogenic compounds at relatively high dosages or duration have smaller seminiferous tubules and impaired spermatogenesis (199). Roosters and rats exposed to estrogenic compounds have lower testosterone production (200, 201), more oxidative stress, and reduced sperm function (5). In the boar, estrogenic compounds cause premature capacitation (202) and a stronger immune response to spermatozoa in the female tract (203).
We propose that exposure to phytoestrogens could compromise both the male and the female, as well as interactions between spermatozoa and the female tract, creating conditions within the female reproductive system that are hostile, more so than usual, to spermatozoa. The exposure to exogenous estrogens could drastically impair the ability of spermatozoa to traverse the female tract and successfully fertilize, which could partially account for the poor fertility in sheep that graze estrogenic pasture (4, 204). The addition of physiological concentrations of equol to ram spermatozoa in vitro negatively alters sperm function, with decreased sperm motility, increased ROS, increased membrane fluidity, and greater DNA fragmentation compared to non-exposed ram spermatozoa (11).
One obvious factor that will influence the interaction between spermatozoa and the ewe reproductive tract is the morphological changes to the female reproductive tract that are observed after phytoestrogen exposure (3, 6, 128). Prolonged grazing of estrogenic pasture has been found to induce a loss of crypts and folds in the cervix of ewes (4, 6, 13). The crypts of the ovine cervix represent “privileged pathways”, potential routes where conditions of cervical mucous flow, composition, and viscosity are conducive to the successful progression of spermatozoa toward the oocyte (205, 206). Thus, the morphological changes that are observed in female tract and in the sperm physiology after exposure to phytoestrogens could synergize to compromise the ability of spermatozoa to traverse the female reproductive tract.
The ability of spermatozoa to navigate the female reproductive tract is not merely a function of motility, but rather involves dynamic interactions between the male gamete and the tract environment. Particularly noteworthy in the context of reproduction after animals are exposed estrogenic compounds, is the role of ovarian steroids in the production and composition of cervical mucous, and in the immune response that is stimulated by the presence of the incoming spermatozoa (205, 207). Exogenous hormones, such as those that are used to synchronize the estrous cycle of ewes, are known to vastly alter the composition of cervical mucous thereby impairing the function of spermatozoa, leading to a reduction in fertility (205, 207–210). Infertility in ewes that graze sub clover species that are estrogenic could be partially due to changes in cervical mucous that are induced by phytoestrogens. During the follicular phase, the viscosity of the cervical mucous decreases in response to rising estrogen concentrations, lowering the barrier to sperm migration through the female reproductive tract. That change optimizes the conditions in preparation for ovulation and potential insemination (205, 207). Very early studies in sheep showed that the viscosity of mucus was lower after chronic exposure to estradiol and in ewes with clover disease (12, 211). However, the lower mucus viscosity in ewes with clover disease was also correlated with reduced fertility (212). This suggests that a factor other than viscosity, such as the biochemical composition of mucus following long term phytoestrogen exposure, is less favorable to sperm transport.
While exogenous estrogen is known to alter the female immune response and the composition of seminal plasma in the male (163), it is not known if phytoestrogens elicit similar effects. When seminal plasma enters the female tract immune cells are recruited and activated, releasing cytokines and chemokines that play a role in the sperm selection process in the female tract (213). In mammals, the magnitude of this immune response varies in response to endogenous estrogen (203), exogenous hormones (214), and specifically in sheep, between breeds (205). There are reports of the production of anti-sperm antibodies in the female reproductive tract after insemination, though a predominant focus of immune studies has been the known immunogenic properties of seminal plasma (215, 216). Because some of the constituents of seminal plasma have an immunomodulatory effect (215, 217), anything that influences the composition of the seminal plasma could affect the female immune response, with an impact on sperm survival. Greater numbers of leukocytes in cervical mucus increases sperm capacitation and the acrosome reaction (218). Because leukocytes produce ROS at far higher levels than do spermatozoa (219), the production of ROS might be the underlying mechanism for the promotion of these events. It will be worthwhile to investigate whether phytoestrogens alter the composition of seminal plasma, and if that then modulates the immune response that is mounted by the female after copulation.
In summary, the combination of loss of privileged pathways for sperm in the cervix, changes in the composition of cervical mucous, and changes in the immune response of the female to spermatozoa all represent possible mechanisms that could contribute to the fertility issues that occur in sheep when they graze sub clover (Figure 1). Investigation is warranted into male-female gamete interactions after either sex is exposed to phytoestrogens.
Conclusions and Recommendations for Future Management of Ovine Clover Disease
There are two clear rationale for further research into clover disease; there are clear agricultural benefits to be gained, and this syndrome in sheep presents a unique opportunity to develop a model of the reproductive consequences of phytoestrogen exposure, particularly during critical developmental periods. The review of the known literature points logically to several potential sites of action, and mechanisms of action, of phytoestrogens on sheep reproduction. This includes estrogen receptor binding, genomic and epigenetic alterations and immune modulation, at sites including the reproductive tract, brain and gametes of both the male and female. It is likely that a systemic interplay between those factors is responsible for the spectrum of pathological changes that are observed in ovine clover disease, though that itself requires clarification. In our view, it is necessary not only to understand the consequences of exposure to phytoestrogens on gamete production in both sexes, but also to understand how that exposure alters the interaction of the gametes in the female reproductive tract. An interesting avenue of research would be to explore the physiological and genetic basis of individual susceptibility to phytoestrogens. The identification and strategic breeding of resilient animals is a logical start to address the issue, as well as the removal from breeding of persistently sub-fertile individuals and the early identification of susceptible flocks. Those strategies could be used in parallel with pasture renovation to eliminate estrogenic clover.
Author Contributions
KP drafted, edited and wrote the final manuscript. FC conducted the meta-analysis. DB and JS reviewed, edited and contributed to the manuscript. All authors approved the final version of the manuscript.
Funding
KP is supported by the Lefroy Bequest, and DB was supported by a Meat and Livestock Australia grant (P.PSH.1138).
Conflict of Interest
The authors declare that the research was conducted in the absence of any commercial or financial relationships that could be construed as a potential conflict of interest.
Publisher’s Note
All claims expressed in this article are solely those of the authors and do not necessarily represent those of their affiliated organizations, or those of the publisher, the editors and the reviewers. Any product that may be evaluated in this article, or claim that may be made by its manufacturer, is not guaranteed or endorsed by the publisher.
References
1. Nichols PGH, Foster KJ, Piano E, Pecetti L, Kaur P, Ghamkhar K, et al. Genetic Improvement of Subterranean Clover (Trifolium Subterraneum L.). 1. Germplasm, Traits and Future Prospects. Crop Pasture Sci (2013) 64:312–416. doi: 10.1071/CP13118
2. Nichols PGH, Collins WJ, Gillespie DJ, Barbetti MJ. Developing Improved Varieties of Subterranean Clover. J Agric West Aust (1994) 35:60–5.
3. Adams NR. Pathological Changes in the Tissues of Infertile Ewes With Clover Disease. J Comp Pathol (1976) 86:29–35. doi: 10.1016/0021-9975(76)90024-4
4. Adams NR. Permanent Infertility in Ewes Exposed to Plant Oestrogens. Aust Vet J (1990) 67:197–201. doi: 10.1111/j.1751-0813.1990.tb07758.x
5. Anderson D, Schmid TE, Baumgartner A, Cemeli-Carratala E, Brinkworth MH, Wood JM. Oestrogenic Compounds and Oxidative Stress (in Human Sperm and Lymphocytes in the Comet Assay). Rev Mutat Res (2003) 144:173–8. doi: 10.1016/j.mrrev.2003.06.016
6. Adams NR. Morphological Changes in the Organs of Ewes Grazing Oestrogenic Subterranean Clover. Res Vet Sci (1977) 22:216–21. doi: 10.1016/s0034-5288(18)33290-9
7. Adams NR. Masculinisation of the External Genitalia in Ewes With Clover Disease. Aust Vet J (1979) 55:22–4. doi: 10.1111/j.1751-0813.1979.tb09540.x
8. Menzel VA, Hinsch E, Hägele W, Hinsch KD. Effect of Genistein on Acrosome Reaction and Zona Pellucida Binding Independent of Protein Tyrosine Kinase Inhibition in Bull. Asian J Androl (2007) 9:650–8. doi: 10.1111/j.1745-7262.2007.00240.x
9. Eustache F, Mondon F, Canivenc-Lavier MC, Lesaffre C, Fulla Y, Berges R, et al. Chronic Dietary Exposure to a Low-Dose Mixture of Genistein and Vinclozolin Modifies the Reproductive Axis, Testis Transcriptome, and Fertility. Environ Health Perspect (2009) 117:1272–9. doi: 10.1289/ehp.0800158
10. Xia Y, Chen M, Zhu P, Lu C, Fu G, Zhou X, et al. Urinary Phytoestrogen Levels Related to Idiopathic Male Infertility in Chinese Men. Environ Int (2013) 59:161–7. doi: 10.1016/j.envint.2013.06.009
11. Pool KR, Kent TC, Blache D. Oestrogenic Metabolite Equol Negatively Impacts the Functionality of Ram Spermatozoa In Vitro. Theriogenology (2021) 172:216–22. doi: 10.1016/j.theriogenology.2021.07.005
12. Adams NR, Sanders MR. Persistent Infertility in Ewes After Prolonged Exposure to Oestradiol-17β. J Reprod Fertil (1988) 84:373–8. doi: 10.1530/jrf.0.0840373
13. Adams NR. Morphogenic Change in the Cervix of the Ewe After Prolonged Exposure to Oestradiol-17β. J Reprod Fertil (1986) 76:727–33. doi: 10.1530/jrf.0.0760727
14. Foster K, Ryan M, Martin G, Watts T, Kontoolas M, Durmic Z, et al. Clover Disease Across Southern Australia What We Know Now. In: Proceedings of the 33rd Australian Associationd of Animal Scinces Conference. Clayton, Victoria: CSIRO PUBLISHING (2021).
15. Brehm E, Flaws JA. Transgenerational Effects of Endocrine-Disrupting Chemicals on Male and Female Reproduction. Endocrinology (2019) 160:1421–35. doi: 10.1210/EN.2019-00034
16. Rodprasert W, Toppari J, Virtanen HE. Endocrine Disrupting Chemicals and Reproductive Health in Boys and Men. Front Endocrinol (Lausanne) (2021) 12:706532/BIBTEX. doi: 10.3389/FENDO.2021.706532/BIBTEX
17. Bennetts HW, Uuderwood EJ, Shier FL. A Specific Breeding Problem Of Sheep On Subterranean Clover Pastures In Western Australia. Aust Vet J (1946) 22:2–12. doi: 10.1111/j.1751-0813.1946.tb15473.x
18. Morley FHW, Axelsen A, Bennett D. Recovery of Normal Fertility After Grazing on Oestrogenic Red Clover. Aust Vet J (1966) 42:204–6. doi: 10.1111/j.1751-0813.1966.tb08835.x
19. George JM. The Incidence Of Dystocia In Fine-Wool Merino Ewes. Aust Vet J (1975) 51:262–5. doi: 10.1111/j.1751-0813.1975.tb06931.x
20. Moutsatsou P. The Spectrum of Phytoestrogens in Nature: Our Knowledge is Expanding. Hormones (Athens) (2007) 6:173–93.
21. Jefferson WN, Patisaul HB, Williams CJ. Reproductive Consequences of Developmental Phytoestrogen Exposure. Reproduction (2012) 143:247–60. doi: 10.1530/REP-11-0369
22. Dickinson JM, Smith GR, Randel RD, Pemberton IJ. In Vitro Metabolism of Formononetin and Biochanin A in Bovine Rumen Fluid. J Anim Sci (1988) 66:1969–73. doi: 10.2527/jas1988.6681969x
23. Nilsson A, Hill JL, Lloyd Davies H. An In Vitro Study of Formononetin and Biochanin A Metabolism in Rumen Fluid From Sheep. BBA - Gen Subj (1967) 148:92–8. doi: 10.1016/0304-4165(67)90282-6
24. Shutt DA, Cox RI. Steroid and Phyto-Oestrogen Binding to Sheep Uterine Receptors In Vitro. J Endocrinol (1972) 52:299–310. doi: 10.1677/joe.0.0520299
25. Kuiper GGJM, Lemmen JG, Carlsson B, Corton JC, Safe SH, van der Saag PT, et al. Interaction of Estrogenic Chemicals and Phytoestrogens With Estrogen Receptor β. Endocrinology (1998) 139:4252–63. doi: 10.1210/endo.139.10.6216
26. Matthews J, Gustafsson JA. Estrogen Signaling: A Subtle Balance Between ER Alpha and ER Beta. Mol Interv (2003) 3:281–92. doi: 10.1124/mi.3.5.281
27. Paterni I, Granchi C, Katzenellenbogen JA, Minutolo F. Estrogen Receptors Alpha (Erα) and Beta (Erβ): Subtype-Selective Ligands and Clinical Potential. Steroids (2014) 90:13–29. doi: 10.1016/j.steroids.2014.06.012
28. Lee H-R, Kim T-H, Choi K-C. Functions and Physiological Roles of Two Types of Estrogen Receptors, Erα and Erβ, Identified by Estrogen Receptor Knockout Mouse. Lab Anim Res (2012) 28:71–6. doi: 10.5625/lar.2012.28.2.71
29. Jayachandran M, Preston CC, Hunter LW, Jahangir A, Owen WG, Korach KS, et al. Loss of Estrogen Receptor β Decreases Mitochondrial Energetic Potential and Increases Thrombogenicity of Platelets in Aged Female Mice. Age (Omaha) (2010) 32:109–21. doi: 10.1007/s11357-009-9119-y
30. Björnström L, Sjöberg M. Mechanisms of Estrogen Receptor Signaling: Convergence of Genomic and Nongenomic Actions on Target Genes. Mol Endocrinol (2005) 19:833–42. doi: 10.1210/me.2004-0486
31. Nilsson S, Mäkelä S, Treuter E, Tujague M, Thomsen J, Andersson G, et al. Mechanisms of Estrogen Action. Physiol Rev (2001) 81:1535–65. doi: 10.1152/physrev.2001.81.4.1535
32. Göttlicher M, Heck S, Herrlich P. Transcriptional Cross-Talk, the Second Mode of Steroid Hormone Receptor Action. J Mol Med (1998) 76:480–9. doi: 10.1007/s001090050242
33. Lösel R, Wehling M. Nongenomic Actions of Steroid Hormones. Nat Rev Mol Cell Biol (2003) 4:46–56. doi: 10.1038/nrm1009
34. Dip R, Lenz S, Antignac JP, Le Bizec B, Gmuender H, Naegeli H. Global Gene Expression Profiles Induced by Phytoestrogens in Human Breast Cancer Cells. Endocr Relat Cancer (2008) 15:161–73. doi: 10.1677/ERC-07-0252
35. Gilad LA, Tirosh O, Schwartz B. Phytoestrogen Regulate Transcription and Translation of Vitamin D Receptor in Colon Cancer Cells. J Endocrinol (2006) 191:387–98. doi: 10.1677/joe.1.06930
36. Wipawee W, Hewitt SC, Orvis GD, Behringer RR, Korach KS. Uterine Epithelial Estrogen Receptor α Is Dispensable for Proliferation But Essential for Complete Biological and Biochemical Responses. Proc Natl Acad Sci USA (2010) 107:19272–7. doi: 10.1073/pnas.1013226107
37. Katzenellenbogen JA, Mayne CG, Katzenellenbogen BS, Greene GL, Chandarlapaty S. Structural Underpinnings of Oestrogen Receptor Mutations in Endocrine Therapy Resistance. Nat Rev Cancer (2018) 18:377–88. doi: 10.1038/s41568-018-0001-z
38. Katzenellenbogen BS, Frasor J. Therapeutic Targeting in the Estrogen Receptor Hormonal Pathway. Semin Oncol (2004) 31:28–38. doi: 10.1053/j.seminoncol.2004.01.004
39. Weigelt B, Hu Z, He X, Livasy C, Carey LA, Ewend MG, et al. Molecular Portraits and 70-Gene Prognosis Signature Are Preserved Throughout the Metastatic Process of Breast Cancer. Cancer Res (2005) 65:9155–8. doi: 10.1158/0008-5472.CAN-05-2553
40. Clarke IJ, Cummins JT. Direct Pituitary Effects of Estrogen and Progesterone on Gonadotropin Secretion in the Ovariectomized Ewe. Neuroendocrinology (1984) 39:267–74. doi: 10.1159/000123990
41. Dubois SL, Acosta-Martínez M, DeJoseph MR, Wolfe A, Radovick S, Boehm U, et al. Positive, But Not Negative Feedback Actions of Estradiol in Adult Female Mice Require Estrogen Receptor α in Kisspeptin Neurons. Endocrinology (2015) 156:1111–20. doi: 10.1210/EN.2014-1851
42. Dahlman-Wright K, Koehler K, Gustafsson J-Å. Estrogen Receptor-β Structure and Function. In: Encyclopedia of Hormones. Amsterdam: Elsevier Elsevier (2003). p. 599–608. doi: 10.1016/b0-12-341103-3/00091-7
43. Pettersson K, Delaunay F, Gustafsson JA. Estrogen Receptor β Acts as a Dominant Regulator of Estrogen Signaling. Oncogene (2000) 19:4970–8. doi: 10.1038/sj.onc.1203828
44. Paech K, Webb P, Kuiper GGJM, Nilsson S, Gustafsson JÅ, Kushner PJ, et al. Differential Ligand Activation of Estrogen Receptors Erα and Errβ at AP1 Sites. Sci (80- ) (1997) 277:1508–10. doi: 10.1126/science.277.5331.1508
45. Liu MM, Albanese C, Anderson CM, Hilty K, Webb P, Uht RM, et al. Opposing Action of Estrogen Receptors α and β on Cyclin D1 Gene Expression. J Biol Chem (2002) 277:24353–60. doi: 10.1074/jbc.M201829200
46. Powell E, Xu W. Intermolecular Interactions Identify Ligand-Selective Activity of Estrogen Receptor α/β Dimers. Proc Natl Acad Sci USA (2008) 105:19012–7. doi: 10.1073/pnas.0807274105
47. Mauvais-Jarvis F, Clegg DJ, Hevener AL. The Role of Estrogens in Control of Energy Balance and Glucose Homeostasis. Endocr Rev (2013) 34:309–38. doi: 10.1210/er.2012-1055
48. Barros RPA, Gabbi C, Morani A, Warner M, Gustafsson JÅ. Participation of Erα and Erβ in Glucose Homeostasis in Skeletal Muscle and White Adipose Tissue. Am J Physiol - Endocrinol Metab (2009) 297:124–33. doi: 10.1152/ajpendo.00189.2009
49. Garcia-Arevalo M, Lorza-Gil E, Leite N, Brunetto S, Boschero AC, Carneiro EM. The 17-Beta-Estradiol Improves Insulin Sensitivity in a Rapid Estrogen Receptor Alpha-Dependent Manner in an Animal Model of Malnourishment. J Endocrinol Metab (2019) 9:133–46. doi: 10.14740/jem612
50. Palmisano BT, Zhu L, Stafford JM. Role of Estrogens in the Regulation of Liver Lipid Metabolism. Adv Exp Med Biol (2017) 1043:227–56. doi: 10.1007/978-3-319-70178-3_12
51. Savva C, Korach-André M. Estrogen Receptor Beta (Erβ) Regulation of Lipid Homeostasis—Does Sex Matter? Metabolites (2020) 10:116. doi: 10.3390/metabo10030116
52. Barros RPA, Gustafsson JÅ. Estrogen Receptors and the Metabolic Network. Cell Metab (2011) 14:289–99. doi: 10.1016/j.cmet.2011.08.005
53. Rietjens IMCM, Louisse J, Beekmann K. The Potential Health Effects of Dietary Phytoestrogens. Br J Pharmacol (2017) 174:1263–80. doi: 10.1111/bph.13622
54. Luo J, Liu D. Does GPER Really Function as a G Protein-Coupled Estrogen Receptor In Vivo? Front Endocrinol (Lausanne) (2020) 11. doi: 10.3389/fendo.2020.00148
55. Hazell GGJ, Yao ST, Roper JA, Prossnitz ER, O’Carroll AM, Lolait SJ. Localisation of GPR30, a Novel G Protein-Coupled Oestrogen Receptor, Suggests Multiple Functions in Rodent Brain and Peripheral Tissues. J Endocrinol (2009) 202:223–36. doi: 10.1677/JOE-09-0066
56. Martensson UEA, Salehi SA, Windahl S, Gomez MF, Swärd K, Daszkiewicz-Nilsson J, et al. Deletion of the G Protein-Coupled Receptor 30 Impairs Glucose Tolerance, Reduces Bone Growth, Increases Blood Pressure, and Eliminates Estradiol-Stimulated Insulin Release in Female Mice. Endocrinology (2009) 150:687–98. doi: 10.1210/en.2008-0623
57. Canonaco M, Giusi G, Madeo A, Facciolo RM, Lappano R, Canonaco A, et al. A Sexually Dimorphic Distribution Pattern of the Novel Estrogen Receptor G-Protein-Coupled Receptor 30 in Some Brain Areas of the Hamster. J Endocrinol (2008) 196:131–8. doi: 10.1677/JOE-07-0392
58. Thomas P, Pang Y, Filardo EJ, Dong J. Identity of an Estrogen Membrane Receptor Coupled to a G Protein in Human Breast Cancer Cells. Endocrinology (2005) 146:624–32. doi: 10.1210/en.2004-1064
59. Carmeci C, Thompson DA, Ring HZ, Francke U, Weigel RJ. Identification of a Gene (GPR30) With Homology to the G-Protein-Coupled Receptor Superfamily Associated With Estrogen Receptor Expression in Breast Cancer. Genomics (1997) 45:607–17. doi: 10.1006/geno.1997.4972
60. Wei W, Chen ZJ, Zhang KS, Yang XL, Wu YM, Chen XH, et al. The Activation of G Protein-Coupled Receptor 30 (GPR30) Inhibits Proliferation of Estrogen Receptornegative Breast Cancer Cells In Vitro and In Vivo. Cell Death Dis (2014) 5:e1428. doi: 10.1038/cddis.2014.398
61. Chevalier N, Vega A, Bouskine A, Siddeek B, Michiels JF, Chevallier D, et al. Gpr30, the Non-Classical Membrane G Protein Related Estrogen Receptor, Is Overexpressed in Human Seminoma and Promotes Seminoma Cell Proliferation. PloS One (2012) 7:e34627. doi: 10.1371/journal.pone.0034672
62. Chan QKY, Lam HM, Ng CF, Lee AYY, Chan ESY, Ng HK, et al. Activation of GPR30 Inhibits the Growth of Prostate Cancer Cells Through Sustained Activation of Erk1/2, C-Jun/C-Fos-Dependent Upregulation of P21, and Induction of G2 Cell-Cycle Arrest. Cell Death Differ (2010) 17:1511–23. doi: 10.1038/cdd.2010.20
63. Albanito L, Lappano R, Madeo A, Chimento A, Prossnitz EE, Cappello AR, et al. G-Protein-Coupled Receptor 30 and Estrogen Receptor-α Are Involved in the Proliferative Effects Induced by Atrazine in Ovarian Cancer Cells. Environ Health Perspect (2008) 116:1648–55. doi: 10.1289/ehp.11297
64. Albanito L, Madeo A, Lappano R, Vivacqua A, Rago V, Carpino A, et al. G Protein-Coupled Receptor 30 (GPR30) Mediates Gene Expression Changes and Growth Response to 17β-Estradiol and Selective GPR30 Ligand G-1 in Ovarian Cancer Cells. Cancer Res (2007) 67:1859–66. doi: 10.1158/0008-5472.CAN-06-2909
65. Vivacqua A, Bonofiglio D, Recchia AG, Musti AM, Picard D, Andò S, et al. The G Protein-Coupled Receptor GPR30 Mediates the Proliferative Effects Induced by 17β-Estradiol and Hydroxytamoxifen in Endometrial Cancer Cells. Mol Endocrinol (2006) 20:631–46. doi: 10.1210/me.2005-0280
66. Plante BJ, Lessey BA, Taylor RN, Wang W, Bagchi MK, Yuan L, et al. G Protein-Coupled Estrogen Receptor (GPER) Expression in Normal and Abnormal Endometrium. Reprod Sci (2012) 19:684–93. doi: 10.1177/1933719111431000
67. Thomas P, Dong J. Binding and Activation of the Seven-Transmembrane Estrogen Receptor GPR30 by Environmental Estrogens: A Potential Novel Mechanism of Endocrine Disruption. J Steroid Biochem Mol Biol (2006) 102:175–9. doi: 10.1016/j.jsbmb.2006.09.017
68. Prossnitz ER, Arterburn JB. International Union of Basic and Clinical Pharmacology. XCVII. G Protein–Coupled Estrogen Receptor and Its Pharmacologic Modulators. Pharmacol Rev (2015) 67:505. doi: 10.1124/PR.114.009712
69. Donkin I, Barrès R. Sperm Epigenetics and Influence of Environmental Factors. Mol Metab (2018) 14:1–11. doi: 10.1016/j.molmet.2018.02.006
70. Tang WY, Morey LM, Cheung YY, Birch L, Prins GS, Ho SM. Neonatal Exposure to Estradiol/Bisphenol A Alters Promoter Methylation and Expression of Nsbp1 and Hpcal1 Genes and Transcriptional Programs of Dnmt3a/b and Mbd2/4 in the Ratprostate Gland Throughout Life. Endocrinology (2012) 153:42–55. doi: 10.1210/en.2011-1308
71. Kaneda M, Okano M, Hata K, Sado T, Tsujimoto H, Li E, et al. Essential Role for De Novo DNA Methyltransferase Dnmt3a in Paternal and Maternal Imprinting. Nature (2004) 429:900–3. doi: 10.1038/nature02633
72. Lyn-Cook BD, Blann E, Payne PW, Bo J, Sheehan D, Medlock K. Methylation Profile and Amplification of Proto-Oncogenes in Rat Pancreas Induced With Phytoestrogens. Proc Soc Exp Biol Med (1995) 208:116–9. doi: 10.3181/00379727-208-43842
73. Day J, Bauer AM, Desbordes C, Zhuang Y, Kim BE, Newton LG, et al. Genistein Alters Methylation Patterns in Mice. J Nutr (2002) 132:2419–23. doi: 10.1093/jn/132.8.2419s
74. Dolinoy DC, Weidman JR, Waterland RA, Jirtle RL. Maternal Genistein Alters Coat Color and Protects Avy Mouse Offspring From Obesity by Modifying the Fetal Epigenome. Environ Health Perspect (2006) 114:567–72. doi: 10.1289/ehp.8700
75. Jefferson WN, Doerge D, Padilla-Banks E, Woodling KA, Kissling GE, Newbold R. Oral Exposure to Genistin, the Glycosylated Form of Genistein, During Neonatal Life Adversely Affects the Female Reproductive System. Environ Health Perspect (2009) 117:1883–9. doi: 10.1289/ehp.0900923
76. Tang WY, Newbold R, Mardilovich K, Jefferson W, Cheng RYS, Medvedovic M, et al. Persistent Hypomethylation in the Promoter of Nucleosomal Binding Protein 1 (Nsbp1) Correlates With Overexpression of Nsbp1 in Mouse Uteri Neonatally Exposed to Diethylstilbestrol or Genistein. Endocrinology (2008) 149:5922–31. doi: 10.1210/en.2008-0682
77. Doerge DR. Bioavailability of Soy Isoflavones Through Placental/Lactational Transfer and Soy Food. Toxicol Appl Pharmacol (2011) 24:145–7. doi: 10.1016/j.taap.2010.10.018
78. Doerge DR, Churchwell MI, Chang HC, Newbold RR, Delclos KB. Placental Transfer of the Soy Isoflavone Genistein Following Dietary and Gavage Administration to Sprague Dawley Rats. Reprod Toxicol (2001) 15:105–10. doi: 10.1016/S0890-6238(01)00108-3
79. Francis S, Yelumalai S, Jones C, Coward K. Aberrant Protamine Content in Sperm and Consequential Implications for Infertility Treatment. Hum Fertil (2014) 17:80–9. doi: 10.3109/14647273.2014.915347
80. Montjean D, Zini A, Ravel C, Belloc S, Dalleac A, Copin H, et al. Sperm Global DNA Methylation Level: Association With Semen Parameters and Genome Integrity. Andrology (2015) 3:235–40. doi: 10.1111/andr.12001
81. Dada R, Kumar M, Jesudasan R, Fernández JL, Gosálvez J, Agarwal A. Epigenetics and Its Role in Male Infertility. J Assist Reprod Genet (2012) 29:213–23. doi: 10.1007/s10815-012-9715-0
82. Chamani IJ, Keefe DL. Epigenetics and Female Reproductive Aging. Front Endocrinol (Lausanne) (2019) 10. doi: 10.3389/fendo.2019.00473
83. Dunn GA, Bale TL. Maternal High-Fat Diet Effects on Third-Generation Female Body Size via the Paternal Lineage. Endocrinology (2011) 152:2228–36. doi: 10.1210/en.2010-1461
84. Kaati G, Bygren LO, Pembrey M, Sjöström M. Transgenerational Response to Nutrition, Early Life Circumstances and Longevity. Eur J Hum Genet (2007) 15:784–90. doi: 10.1038/sj.ejhg.5201832
85. Santana VP, Miranda-Furtado CL, de Oliveira-Gennaro FG, dos Reis RM. Genetics and Epigenetics of Varicocele Pathophysiology: An Overview. J Assist Reprod Genet (2017) 34:839–47. doi: 10.1007/s10815-017-0931-5
86. Hosseini E, Shahhoseini M, Afsharian P, Karimian L, Ashrafi M, Mehraein F, et al. Role of Epigenetic Modifications in the Aberrant CYP19A1 Gene Expression in Polycystic Ovary Syndrome. Arch Med Sci (2019) 15:887–95. doi: 10.5114/aoms.2019.86060
87. Borghese B, Zondervan KT, Abrao MS, Chapron C, Vaiman D. Recent Insights on the Genetics and Epigenetics of Endometriosis. Clin Genet (2017) 91:254–64. doi: 10.1111/cge.12897
88. Jefferson WN, Williams CJ. Circulating Levels of Genistein in the Neonate, Apart From Dose and Route, Predict Future Adverse Female Reproductive Outcomes. Reprod Toxicol (2011) 31:272–9. doi: 10.1016/j.reprotox.2010.10.001
89. Setchell KDR, Zimmer-Nechemias L, Cai J, Heubi JE. Exposure of Infants to Phyto-Oestrogens From Soy-Based Infant Formula. Lancet (1997) 350:23–7. doi: 10.1016/S0140-6736(96)09480-9
90. Murthy D, Madhusudana Reddy C, Patil SB. Effect of Benzene Extract of Hibiscus Rosa Sinensis on the Estrous Cycle and Ovarian Activity in Albino Mice. Biol Pharm Bull (1997) 20:756–8. doi: 10.1248/bpb.20.756
91. Awoniyi CA, Roberts D, Rao Veeramachaneni DN, Hurst BS, Tucker KE, Schlaff WD. Reproductive Sequelae in Female Rats After In Utero and Neonatal Exposure to the Phytoestrogen Genistein. Fertil Steril (1998) 70:440–7. doi: 10.1016/S0015-0282(98)00185-X
92. Tinwell H, Soames AR, Foster JR, Ashby J. Estradiol-Type Activity of Coumestrol in Mature and Immature Ovariectomized Rat Uterotrophic Assays. Environ Health Perspect (2000) 108:631–4. doi: 10.1289/ehp.00108631
93. Baker VA, Hepburn PA, Kennedy SJ, Jones PA, Lea LJ, Sumpter JP, et al. Safety Evaluation of Phytosterol Esters. Part 1. Assessment of Oestrogenicity Using a Combination of In Vivo and In Vitro Assays. Food Chem Toxicol (1999) 37:13–22. doi: 10.1016/S0278-6915(98)00101-X
94. Santell RC, Chang YC, Nair MG, Helferich WG. Dietary Genistein Exerts Estrogenic Effects Upon the Uterus, Mammary Gland and the Hypothalamic/Pituitary Axis in Rats. J Nutr (1997) 127:263–9. doi: 10.1093/jn/127.2.263
95. Mahdi D, Khallili K. Relationship Between Follicle Growth and Circulating Gonadotrophin Levels During Postnatal Development of Sheep. Anim Reprod Sci (2008) 106:100–12. doi: 10.1016/j.anireprosci.2007.04.008
96. Huffman LJ, Inskeep EK, Goodman RL. Changes in Episodic Luteinizing Hormone Secretion Leading to Puberty in the Lamb. Biol Reprod (1987) 37:755–61. doi: 10.1095/biolreprod37.4.755
97. Tassell R, Chamley WA, Kennedy JP. Gonadotrophin Levels and Ovarian Development in the Neonatal Ewe Lamb. Aust J Biol Sci (1978) 31:267–74. doi: 10.1071/BI9780267
98. Pool KR, Rickard JP, de Graaf SP. Overcoming Neuroendocrine and Metabolic Barriers to Puberty: The Role of Melatonin in Advancing Puberty in Ewe Lambs. Domest Anim Endocrinol (2020) 72:106457. doi: 10.1016/j.domaniend.2020.106457
99. Delclos KB, Bucci TJ, Lomax LG, Latendresse JR, Warbritton A, Weis CC, et al. Effects of Dietary Genistein Exposure During Development on Male and Female CD (Sprague-Dawley) Rats. Reprod Toxicol (2001) 15:647–63. doi: 10.1016/S0890-6238(01)00177-0
100. Padilla-Banks E, Jefferson WN, Newbold RR. Neonatal Exposure to the Phytoestrogen Genistein Alters Mammary Gland Growth and Developmental Programming of Hormone Receptor Levels. Endocrinology (2006) 147:4871–82. doi: 10.1210/en.2006-0389
101. Molzberger AF, Vollmer G, Hertrampf T, Möller FJ, Kulling S, Diel P. In Utero and Postnatal Exposure to Isoflavones Results in a Reduced Responsivity of the Mammary Gland Towards Estradiol. Mol Nutr Food Res (2012) 56:399–409. doi: 10.1002/mnfr.201100371
102. Bateman HL, Patisaul HB. Disrupted Female Reproductive Physiology Following Neonatal Exposure to Phytoestrogens or Estrogen Specific Ligands Is Associated With Decreased GnRH Activation and Kisspeptin Fiber Density in the Hypothalamus. Neurotoxicology (2008) 29:988–97. doi: 10.1016/j.neuro.2008.06.008
103. Nagao T, Yoshimura S, Saito Y, Nakagomi M, Usumi K, Ono H. Reproductive Effects in Male and Female Rats of Neonatal Exposure to Genistein. Reprod Toxicol (2001) 15:399–411. doi: 10.1016/S0890-6238(01)00141-1
104. Kouki T, Kishitake M, Okamoto M, Oosuka I, Takebe M, Yamanouchi K. Effects of Neonatal Treatment With Phytoestrogens, Genistein and Daidzein, on Sex Difference in Female Rat Brain Function: Estrous Cycle and Lordosis. Horm Behav (2003) 44:140–5. doi: 10.1016/S0018-506X(03)00122-3
105. Patisaul HB, Todd KL, Mickens JA, Adewale HB. Impact of Neonatal Exposure to the Erα Agonist PPT, Bisphenol-A or Phytoestrogens on Hypothalamic Kisspeptin Fiber Density in Male and Female Rats. Neurotoxicology (2009) 30:350–7. doi: 10.1016/J.NEURO.2009.02.010
106. De Roux N, Genin E, Carel JC, Matsuda F, Chaussain JL, Milgrom E. Hypogonadotropic Hypogonadism Due to Loss of Function of the KiSS1-Derived Peptide Receptor GPR54. Proc Natl Acad Sci USA (2003) 100:10972–6. doi: 10.1073/PNAS.1834399100
107. Smith JT, Popa SM, Clifton DK, Hoffman GE, Steiner RA. Kiss1 Neurons in the Forebrain as Central Processors for Generating the Preovulatory Luteinizing Hormone Surge. J Neurosci (2006) 26:6687–94. doi: 10.1523/JNEUROSCI.1618-06.2006
108. Smith JT, Clifton DK, Steiner RA. Regulation of the Neuroendocrine Reproductive Axis by Kisspeptin-GPR54 Signaling. Reproduction (2006) 131:623–30. doi: 10.1530/REP.1.00368
109. Kauffman AS, Clifton DK, Steiner RA. Emerging Ideas About Kisspeptin- GPR54 Signaling in the Neuroendocrine Regulation of Reproduction. Trends Neurosci (2007) 30:504–11. doi: 10.1016/J.TINS.2007.08.001
110. Caraty A, Smith JT, Lomet D, Ben Saiöd S, Morrissey A, Cognie J, et al. Kisspeptin Synchronizes Preovulatory Surges in Cyclical Ewes and Causes Ovulation in Seasonally Acyclic Ewes. Endocrinology (2007) 148:5258–67. doi: 10.1210/EN.2007-0554
111. Castellano JM, Roa J, Luque RM, Dieguez C, Aguilar E, Pinilla L, et al. KiSS-1/Kisspeptins and the Metabolic Control of Reproduction: Physiologic Roles and Putative Physiopathological Implications. Peptides (2009) 30:139–45. doi: 10.1016/j.peptides.2008.06.007
112. Rochester JR, Millam JR. Phytoestrogens and Avian Reproduction: Exploring the Evolution and Function of Phytoestrogens and Possible Role of Plant Compounds in the Breeding Ecology of Wild Birds. Comp Biochem Physiol - A Mol Integr Physiol (2009) 154:279–88. doi: 10.1016/j.cbpa.2009.06.017
113. Jefferson WN. Adult Ovarian Function Can Be Affected by High Levels of Soy. J Nutr (2010) 140:2322S–5S. doi: 10.3945/JN.110.123802
114. Smith JF, Jagusch KT, Brunswick LFC, Kelly RW. Coumestans in Lucerne and Ovulation in Ewes. New Zeal J Agric Res (1979) 22:411–6. doi: 10.1080/00288233.1979.10430768
115. Cox RI, Braden AW. The Metabolism and Physiological Effects of Phyto-Oestrogens in Livestock (1974). Available at: http://www.asap.asn.au/livestocklibrary/1974/Cox74PDFhttp://livestocklibrary.com.au/handle/1234/6691 (Accessed September 21, 2021).
116. Patisaul HB. Effects of Environmental Endocrine Disruptors and Phytoestrogens on the Kisspeptin System. Adv Exp Med Biol (2013) 784:455–79. doi: 10.1007/978-1-4614-6199-9_21
117. Whitten PL, Lewis C, Naftolin F. A Phytoestrogen Diet Induces the Premature Anovulatory Syndrome in Lactationally Exposed Female Rats. Biol Reprod (1993) 49:1117–21. doi: 10.1095/biolreprod49.5.1117
118. Hughes CL, Kaldas RS, Weisinger AS, McCants CE, Basham KB. Acute and Subacute Effects of Naturally Occurring Estrogens on Luteinizing Hormone Secretion in the Ovariectomized Rat: Part 1. Reprod Toxicol (1991) 5:127–32. doi: 10.1016/0890-6238(91)90040-M
119. Salsano S, Pérez-Debén S, Quiñonero A, González-Martín R, Domínguez F. Phytoestrogen Exposure Alters Endometrial Stromal Cells and Interferes With Decidualization Signaling. Fertil Steril (2019) 112:947–58.e3. doi: 10.1016/j.fertnstert.2019.06.014
120. Klein SL, Wisniewski AB, Marson AL, Glass GE, Gearhart JP. Early Exposure to Genistein Exerts Long-Lasting Effects on the Endocrine and Immune Systems in Rats. Mol Med (2002) 8:742–9. doi: 10.1007/bf03402038
121. Newbold RR, Padilla Banks E, Bullock B, Jefferson WN. Uterine Adenocarcinoma in Mice Treated Neonatally With Genistein. Cancer Res (2001) 61:4325–8.
122. Newbold RR, Hanson RB, Jefferson WN, Bullock BC, Haseman J, McLachlan JA. Increased Tumors But Uncompromised Fertility in the Female Descendants of Mice Exposed Developmentally to Diethylstilbestrol. Carcinogenesis (1998) 19:1655–63. doi: 10.1093/carcin/19.9.1655
123. Zin SR, Omar SZ, Ali Khan NL, Musameh NI, Das S, Kassim NM. Effects of the Phytoestrogen Genistein on the Development of the Reproductive System of Sprague Dawley Rats. Clinics (2013) 68:253–62. doi: 10.6061/clinics/2013(02)OA21
124. Burton JL, Wells M. The Effect of Phytoestrogens on the Female Genital Tract. J Clin Pathol (2002) 55:401–7. doi: 10.1136/jcp.55.6.401
125. Andrews MA, Schliep KC, Wactawski-Wende J, Stanford JB, Zarek SM, Radin RG, et al. Dietary Factors and Luteal Phase Deficiency in Healthy Eumenorrheic Women. Hum Reprod (2015) 30:1942–51. doi: 10.1093/humrep/dev133
126. Setchell KDR, Gosselin SJ, Welsh MB, Johnston JO, Balistreri WF, Kramer LW, et al. Dietary Estrogens-A Probable Cause of Infertility and Liver Disease in Captive Cheetahs. Gastroenterology (1987) 93:225–33. doi: 10.1016/0016-5085(87)91006-7
127. Williams CL, Ybarra AR, Meredith AN, Durrant BS, Tubbs CW. Gut Microbiota and Phytoestrogen-Associated Infertility in Southern White Rhinoceros. MBio (2019) 10. doi: 10.1128/mBio.00311-19
128. Lightfoot RJ, Adams NR. Changes in Cervical Histology in Ewes Following Prolonged Grazing on Oestrogenic Subterranean Clover. J Comp Pathol (1979) 89:367–73. doi: 10.1016/0021-9975(79)90026-4
129. Mumford SL, Sundaram R, Schisterman EF, Sweeney AM, Barr DB, Rybak ME, et al. Higher Urinary Lignan Concentrations in Women But Not Men are Positively Associated With Shorter Time to Pregnancy. J Nutr (2014) 144:352–8. doi: 10.3945/jn.113.184820
130. Vanegas JC, Afeiche MC, Gaskins AJ, Mínguez-Alarcón L, Williams PL, Wright DL, et al. Soy Food Intake and Treatment Outcomes of Women Undergoing Assisted Reproductive Technology. Fertil Steril (2015) 103:749–55.e2. doi: 10.1016/j.fertnstert.2014.12.104
131. Kohama T, Kobayashi H, Inoue M. The Effect of Soybeans on the Anovulatory Cycle. J Med Food (2005) 8:550–1. doi: 10.1089/jmf.2005.8.550
132. Kamel H. Role of Phyto-Oestrogens in Ovulation Induction in Women With Polycystic Ovarian Syndrome. Eur J Obstet Gynecol Reprod Biol (2013) 168:60–3. doi: 10.1016/J.EJOGRB.2012.12.025
133. Cady N, Peterson SR, Freedman SN, Mangalam AK. Beyond Metabolism: The Complex Interplay Between Dietary Phytoestrogens, Gut Bacteria, and Cells of Nervous and Immune Systems. Front Neurol (2020) 11. doi: 10.3389/fneur.2020.00150
134. Sahin I, Bilir B, Ali S, Sahin K, Kucuk O. Soy Isoflavones in Integrative Oncology: Increased Efficacy and Decreased Toxicity of Cancer Therapy. Integr Cancer Ther (2019) 18:e1534735419835310. doi: 10.1177/1534735419835310
135. Shkolnik K, Tadmor A, Ben-Dor S, Nevo N, Galiani D, Dekel N. Reactive Oxygen Species are Indispensable in Ovulation. Proc Natl Acad Sci USA (2011) 108:1462. doi: 10.1073/PNAS.1017213108
136. Aitken RJ, Jones KT, Robertson SA. Reactive Oxygen Species and Sperm Function-in Sickness and in Health. J Androl (2012) 33:1096–106. doi: 10.2164/jandrol.112.016535
137. Aitken RJ, Nixon B. Sperm Capacitation: A Distant Landscape Glimpsed But Unexplored. Mol Hum Reprod (2013) 19:785–93. doi: 10.1093/molehr/gat067
138. Hensley K, Robinson KA, Gabbita SP, Salsman S, Floyd RA. Reactive Oxygen Species, Cell Signaling, and Cell Injury. Free Radic Biol Med (2000) 28:1456–62. doi: 10.1016/s0891-5849(00)00252-5
139. Pool KR, Rickard JP, Pini T, de Graaf SP. Exogenous Melatonin Advances the Ram Breeding Season and Increases Testicular Function. Sci Rep (2020) 10:9711. doi: 10.1038/s41598-020-66594-6
140. Sejian V, Singh AK, Sahoo A, Naqvi S. Effect of Mineral Mixture and Antioxidant Supplementation on Growth, Reproductive Performance and Adaptive Capability of Malpura Ewes Subjected to Heat Stress. J Anim Physiol Anim Nutr (Berl) (2014) 98:72–83. doi: 10.1111/JPN.12037
141. Martín M, Macías M, Escames G, León J, Acuña-Castroviejo D. Melatonin But Not Vitamins C and E Maintains Glutathione Homeostasis in T-Butyl Hydroperoxide-Induced Mitochondrial Oxidative Stress. FASEB J (2000) 14:1677–9. doi: 10.1096/fj.99-0865fje
142. Succu S, Pasciu V, Manca ME, Chelucci S, Torres-Rovira L, Leoni GG, et al. Dose-Dependent Effect of Melatonin on Postwarming Development of Vitrified Ovine Embryos. Theriogenology (2014) 81:1058–66. doi: 10.1016/j.theriogenology.2014.01.032
143. Succu S, Berlinguer F, Pasciu V, Satta V, Leoni GG, Naitana S. Melatonin Protects Ram Spermatozoa From Cryopreservation Injuries in a Dose-Dependent Manner. J Pineal Res (2011) 50:310–8. doi: 10.1111/j.1600-079X.2010.00843.x
144. Yang M, Tao J, Chai M, Wu H, Wang J, Li G, et al. Melatonin Improves the Quality of Inferior Bovine Oocytes and Promoted Their Subsequent IVF Embryo Development: Mechanisms and Results. Molecules (2017) 22:2059. doi: 10.3390/molecules22122059
145. Jahromi SR, Arrefhosseini SR, Ghaemi A, Alizadeh A, Tabriz HM, Togha M. Alleviation of Experimental Allergic Encephalomyelitis in C57BL/6 Mice by Soy Daidzein. Iran J Allergy Asthma Immunol (2014) 13:256–64.
146. Castro SBR, Junior COR, Alves CCS, Dias AT, Alves LL, Mazzoccoli L, et al. Immunomodulatory Effects and Improved Prognosis of Experimental Autoimmune Encephalomyelitis After O-Tetradecanoyl-Genistein Treatment. Int Immunopharmacol (2012) 12:465–70. doi: 10.1016/J.INTIMP.2011.12.025
147. Weiss G, Goldsmith LT, Taylor RN, Bellet D, Taylor HS. Inflammation in Reproductive Disorders. Reprod Sci (2009) 16:216. doi: 10.1177/1933719108330087
148. Sargent IL, Borzychowski AM, Redman CWG. NK Cells and Human Pregnancy–An Inflammatory View. Trends Immunol (2006) 27:399–404. doi: 10.1016/J.IT.2006.06.009
149. Adams NR, Oldham CM, Heydon RA. Ovulation Rate and Oocyte Numbers in Ewes After Prolonged Exposure to Oestrogenic Pasture. J Reprod Fertil (1979) 55:87–9. doi: 10.1530/JRF.0.0550087
150. Hashem NM, Sallam SMA. Sexual and Ovarian Activity of Crossbred Ewes Fed Different Types of Roughage During Seasonal Anestrus. Small Rumin Res (2012) 2–3:136–40. doi: 10.1016/J.SMALLRUMRES.2012.04.020
151. Adams NR, Hearnshaw H, Oldham CM. Abnormal Function of the Corpus Luteum in Some Ewes With Phyto-Oestrogenic Infertility. Aust J Biol Sci (1981) 34:61–5. doi: 10.1071/BI9810061
152. Santhosh CR, Shridhar NB, Narayana K, Ramachandra SG, Dinesh S. Studies on the Luteolytic, Oestrogenic and Follicle-Stimulating Hormone Like Activity of Plant Rhaphidophora Pertusa (Roxb.). J Ethnopharmacol (2006) 107:365–9. doi: 10.1016/J.JEP.2006.03.036
153. Bennetau-Pelissero C, Breton BB, Bennetau B, Corraze G, Le Menn F, Davail-Cuisset B, et al. Effect of Genistein-Enriched Diets on the Endocrine Process of Gametogenesis and on Reproduction Efficiency of the Rainbow Trout Oncorhynchus Mykiss. Gen Comp Endocrinol (2001) 121:173–87. doi: 10.1006/GCEN.2000.7585
154. Li R, Zhao F, Diao H, Xiao S, Ye X. Postweaning Dietary Genistein Exposure Advances Puberty Without Significantly Affecting Early Pregnancy in C57BL/6J Female Mice. Reprod Toxicol (2014) 44:85–92. doi: 10.1016/j.reprotox.2013.12.003
155. Schulze R. Meta-Analysis : A Comparison of Approaches / Ralf Schulze (2004). Available at: https://books.google.com.au/books?hl=en&lr=&id=5khfAgAAQBAJ&oi=fnd&pg=PR5&dq=chulze,+R.+(2004)+Meta-analysis:+a+comparison+of+approaches.+Hogrefe+%26+Huber,+Gottingen,+Germany.&ots=61FIQ6Gbf9&sig=niAVgC3CQePBTeN2XV7Q-yLeUg4#v=onepage&q&f=false (Accessed March 11, 2022).
156. R Core Team. R: A Language and Environment for Statistical Computing. Austria: R Found Stat Comput Vienna (2017). Available at: https://wwwR-project.org/https://www.r-project.org/.
157. Kenyon PR, Pinchbeck GL, Perkins NR, Morris ST, West DM. Identifying Factors Which Maximise the Lambing Performance of Hoggets: A Cross Sectional Study. N Z Vet J (2004) 52:371–7. doi: 10.1080/00480169.2004.36454
158. Kenyon PR, Maloney SK, Blache D. Review of Sheep Body Condition Score in Relation to Production Characteristics New Zeal J Agric Res (2014) 57:38–64. doi: 10.1080/00288233.2013.857698
159. Lee KH, Hess RA, Bahr JM, Lubahn DB, Taylor J, Bunick D. Estrogen Receptor α Has a Functional Role in the Mouse Rete Testis and Efferent Ductules. Biol Reprod (2000) 63:1873–80. doi: 10.1095/biolreprod63.6.1873
160. Hess RA, Bunick D, Lubahn DB, Zhou Q, Bouma J. Morphologic Changes in Efferent Ductules and Epididymis in Estrogen Receptor-α Knockout Mice. J Androl (2000) 21:107–21. doi: 10.1002/j.1939-4640.2000.tb03282.x
161. Lee KH, Park JH, Bunick D, Lubahn DB, Bahr JM. Morphological Comparison of the Testis and Efferent Ductules Between Wild-Type and Estrogen Receptor α Knockout Mice During Postnatal Development. J Anat (2009) 214:916–25. doi: 10.1111/j.1469-7580.2009.01080.x
162. Patchev VK, Hayashi S, Orikasa C, Almeida OFX. Implications of Estrogen-Dependent Brain Organization for Gender Differences in Hypothalamo-Pituitary-Adrenal Regulation. FASEB J (1995) 9:419–23. doi: 10.1096/FASEBJ.9.5.7896013
163. Hess RA, Bunick D, Lee KH, Bahr J, Taylor JA, Korach KS, et al. A Role for Oestrogens in the Male Reproductive System. Nature (1997) 390:509–12. doi: 10.1038/37352
164. Saunders PTK, Sharpe RM, Williams K, Macpherson S, Urquart H, Irvine DS, et al. Differential Expression of Oestrogen Receptor α and β Proteins in the Testes and Male Reproductive System of Human and non-Human Primates. Mol Hum Reprod (2001) 7:227–36. doi: 10.1093/molehr/7.3.227
165. Zhou Q, Nie R, Prins GS, Saunders PTK, Katzenellenbogen BS, Hess RA. Localization of Androgen and Estrogen Receptors in Adult Male Mouse Reproductive Tract. J Androl (2002) 23:870–81. doi: 10.1002/j.1939-4640.2002.tb02345.x
166. Habito RC, Montalto J, Leslie E, Ball MJ. Effects of Replacing Meat With Soyabean in the Diet on Sex Hormone Concentrations in Healthy Adult Males. Br J Nutr (2000) 84:557–63. doi: 10.1017/S0007114500001872
167. Chavarro JE, Toth TL, Sadio SM, Hauser R. Soy Food and Isoflavone Intake in Relation to Semen Quality Parameters Among Men From an Infertility Clinic. Hum Reprod (2008) 23:2584–90. doi: 10.1093/humrep/den243
168. Nagata C, Inaba S, Kawakami N, Kakizoe T, Shimizu H. Inverse Association of Soy Product Intake With Serum Androgen and Estrogen Concentrations in Japanese Men. Nutr Cancer (2000) 36:14–8. doi: 10.1207/S15327914NC3601_3
169. Povey AC, Clyma J-A, McNamee R, Moore HD, Baillie H, Pacey AA, et al. Phytoestrogen Intake and Other Dietary Risk Factors for Low Motile Sperm Count and Poor Sperm Morphology. Andrology (2020) 8:1805–14. doi: 10.1111/ANDR.12858
170. Mitchell JH, Cawood E, Kinniburgh D, Provan A, Collins AR, Irvine DS. Effect of a Phytoestrogen Food Supplement on Reproductive Health in Normal Males. Clin Sci (2001) 100:613–8. doi: 10.1042/CS20000212
171. Meena R, Supriya C, Pratap Reddy K, Sreenivasula Reddy P. Altered Spermatogenesis, Steroidogenesis and Suppressed Fertility in Adult Male Rats Exposed to Genistein, a Non-Steroidal Phytoestrogen During Embryonic Development. Food Chem Toxicol (2017) 99:70–7. doi: 10.1016/j.fct.2016.11.020
172. Walker C, Ghazisaeidi S, Collet B, Boisvert A, Culty M. In Utero Exposure to Low Doses of Genistein and Di-(2-Ethylhexyl) Phthalate (DEHP) Alters Innate Immune Cells in Neonatal and Adult Rat Testes. Andrology (2020) 8:943–64. doi: 10.1111/andr.12840
173. Cederroth CR, Zimmermann C, Beny JL, Schaad O, Combepine C, Descombes P, et al. Potential Detrimental Effects of a Phytoestrogen-Rich Diet on Male Fertility in Mice. Mol Cell Endocrinol (2010) 321:152–60. doi: 10.1016/j.mce.2010.02.011
174. Mumford SL, Kim S, Chen Z, Barr DB, Louis GMB. Urinary Phytoestrogens are Associated With Subtle Indicators of Semen Quality Among Male Partners of Couples Desiring Pregnancy. J Nutr (2015) 145:2535–41. doi: 10.3945/jn.115.214973
175. Iamsaard S, Arun S, Burawat J, Yannasithinon S, Tongpan S, Bunsueb S, et al. Evaluation of Antioxidant Capacity and Reproductive Toxicity of Aqueous Extract of Thai Mucuna Pruriens Seeds. J Integr Med (2020) 18:265–73. doi: 10.1016/J.JOIM.2020.03.003
176. Glover A, Assinder SJ. Acute Exposure of Adult Male Rats to Dietary Phytoestrogens Reduces Fecundity and Alters Epididymal Steroid Hormone Receptor Expression. J Endocrinol (2006) 189:565–73. doi: 10.1677/JOE.1.06709
177. Najafizadeh P, Dehghani F, Shahin MP, Taj SH. The Effect of a Hydro-Alcoholic Extract of Olive Fruit on Reproductive Argons in Male Sprague-Dawley Rat. Iran J Reprod Med (2013) 11:293.
178. Napier ID, Simon L, Perry D, Cooke PS, Stocco DM, Sepehr E, et al. Testicular Development in Male Rats Is Sensitive to a Soy-Based Diet in the Neonatal Period. Biol Reprod (2014) 90:40. doi: 10.1095/biolreprod.113.113787
179. Lehraiki A, Messiaen S, Berges R, Canivenc-Lavier MC, Auger J, Habert R, et al. Antagonistic Effects of Gestational Dietary Exposure to Low-Dose Vinclozolin and Genistein on Rat Fetal Germ Cell Development. Reprod Toxicol (2011) 31:424–30. doi: 10.1016/J.REPROTOX.2010.12.005
180. Hashem NM, Abo-elsoud MA, Nour El-Din ANM, Kamel KI, Hassan GA. Prolonged Exposure of Dietary Phytoestrogens on Semen Characteristics and Reproductive Performance of Rabbit Bucks. Domest Anim Endocrinol (2018) 64:84–92. doi: 10.1016/J.DOMANIEND.2018.03.003
181. Serrano H, Pérez-Rivero J-J, Aguilar-Setién A, de-Paz O, Villa-Godoy A, Serrano H, et al. Vampire Bat Reproductive Control by a Naturally Occurring Phytooestrogen. Reprod Fertil Dev (2007) 19:470–2. doi: 10.1071/RD06100
182. Carmichael SL, Cogswell ME, Ma C, Gonzalez-Feliciano A, Olney RS, Correa A, et al. Hypospadias and Maternal Intake of Phytoestrogens. Am J Epidemiol (2013) 178:434–40. doi: 10.1093/aje/kws591
183. Nassar N, Abeywardana P, Barker A, Bower C. Parental Occupational Exposure to Potential Endocrine Disrupting Chemicals and Risk of Hypospadias in Infants. Occup Environ Med (2010) 67:585–9. doi: 10.1136/oem.2009.048272
184. Adachi T, Ono Y, Koh KB, Takashima K, Tainaka H, Matsuno Y, et al. Long-Term Alteration of Gene Expression Without Morphological Change in Testis After Neonatal Exposure to Genistein in Mice: Toxicogenomic Analysis Using cDNA Microarray. Food Chem Toxicol (2004) 42:445–52. doi: 10.1016/j.fct.2003.10.012
185. Awoniyi CA, Roberts D, Chandrashekar V, Veeramachaneni DNR, Hurst BS, Tucker KE, et al. Neonatal Exposure to Coumestrol, a Phytoestrogen, Does Not Alter Spermatogenic Potential in Rats. Endocrine (1997) 7:337–41. doi: 10.1007/bf02801328
186. Casanova M, You L, Gaido KW, Archibeque-Engle S, Janszen DB, Heck HDA. Developmental Effects of Dietary Phytoestrogens in Sprague-Dawley Rats and Interactions of Genistein and Daidzein With Rat Estrogen Receptors α and β In Vitro. Toxicol Sci (1999) 51:236–44. doi: 10.1093/toxsci/51.2.236
187. Cardoso JR, Báo SN. Effects of Chronic Exposure to Soy Meal Containing Diet or Soy Derived Isoflavones Supplement on Semen Production and Reproductive System of Male Rabbits. Anim Reprod Sci (2007) 97:237–45. doi: 10.1016/j.anireprosci.2006.01.014
188. Cline JM, Franke AA, Register TC, Golden DL, Adams MR. Effects of Dietary Isoflavone Aglycones on the Reproductive Tract of Male and Female Mice. Toxicol Pathol (2004) 32:91–9. doi: 10.1080/01926230490265902
189. Weber KS, Setchell KDR, Stocco DM, Lephart ED. Dietary Soy-Phytoestrogens Decrease Testosterone Levels and Prostate Weight Without Altering LH, Prostate 5α-Reductase or Testicular Steroidogenic Acute Regulatory Peptide Levels in Adult Male Sprague-Dawley Rats. J Endocrinol (2001) 170:591–9. doi: 10.1677/joe.0.1700591
190. Fraser LR, Beyret E, Milligan SR, Adeoya-Osiguwa SA. Effects of Estrogenic Xenobiotics on Human and Mouse Spermatozoa. Hum Reprod (2006) 21:1184–93. doi: 10.1093/humrep/dei486
191. Mohamed ESA, Park YJ, Song WH, Shin DH, You YA, Ryu BY, et al. Xenoestrogenic Compounds Promote Capacitation and an Acrosome Reaction in Porcine Sperm. Theriogenology (2011) 75:1161–9. doi: 10.1016/j.theriogenology.2010.11.028
192. Aquila S, Santoro M, De Amicis F, Guido C, Bonofiglio D, Lanzino M, et al. Red Wine Consumption may Affect Sperm Biology: The Effects of Different Concentrations of the Phytoestrogen Myricetin on Human Male Gamete Function. Mol Reprod Dev (2013) 80:155–65. doi: 10.1002/mrd.22145
193. Elsayed DH, El-Shamy AA, Abdelrazek HMA, El-Badry DA. Effect of Genistein on Semen Quality, Antioxidant Capacity, Caspase-3 Expression and DNA Integrity in Cryopreserved Ram Spermmatozoa. Small Rumin Res (2019) 177:50–5. doi: 10.1016/j.smallrumres.2019.06.009
194. Martinez-Soto JC, De Dioshourcade J, Gutiérrez-Adán A, Landeras JL, Gadea J. Effect of Genistein Supplementation of Thawing Medium on Characteristics of Frozen Human Spermatozoa. Asian J Androl (2010) 12:431–41. doi: 10.1038/aja.2009.92
195. Sierens J, Hartley JA, Campbell MJ, Leathem AJC, Woodside JV. In Vitro Isoflavone Supplementation Reduces Hydrogen Peroxide-Induced DNA Damage in Sperm. Teratog Carcinog Mutagen (2002) 22:227–34. doi: 10.1002/tcm.10015
196. Rahman Mazumder MA, Hongsprabhas P. Genistein as Antioxidant and Antibrowning Agents in In Vivo and In Vitro: A Review. BioMed Pharmacother (2016) 82:379–92. doi: 10.1016/j.biopha.2016.05.023
197. Setchell KDR, Brown NM, Lydeking-Olsen E. The Clinical Importance of the Metabolite Equol - A Clue to the Effectiveness of Soy and Its Isoflavones. J Nutr (2002) 132:3577–84. doi: 10.1093/jn/132.12.3577
198. Millington AJ, Francis CM, McKeown NR. Wether Bioassay of Annual Pasture Legumes I Oestrogenic Activity in Medicago Tribuloides Desr Var Cyprus Relative to Four Strains of Trifolium Subterraneum L. Aust J Agric Res (1964) 15:520. doi: 10.1071/AR9640520
199. Leavy M, Trottmann M, Liedl B, Reese S, Stief C, Freitag B, et al. Effects of Elevated β-Estradiol Levels on the Functional Morphology of the Testis - New Insights. Sci Rep (2017) 7:1–11. doi: 10.1038/srep39931
200. Opałka M, Kamińska B, Ciereszko R, Dusza L. Genistein Affects Testosterone Secretion by Leydig Cells in Roosters (Gallus Gallus Domesticus). Reprod Biol (2004) 4:185–93.
201. Akingbemi BT, Braden TD, Kemppainen BW, Hancock KD, Sherrill JD, Cook SJ, et al. Exposure to Phytoestrogens in the Perinatal Period Affects Androgen Secretion by Testicular Leydig Cells in the Adult Rat. Endocrinology (2007) 148:4475–88. doi: 10.1210/en.2007-0327
202. Ded L, Dostalova P, Dorosh A, Dvorakova-Hortova K, Peknicova J. Effect of Estrogens on Boar Sperm Capacitation In Vitro. Reprod Biol Endocrinol (2010) 8:87. doi: 10.1186/1477-7827-8-87
203. Wira CR, Grant-Tschudy KS, Crane-Godreau MA. Epithelial Cells in the Female Reproductive Tract: A Central Role as Sentinels of Immune Protection. Am J Reprod Immunol (2005) 53:65–76. doi: 10.1111/j.1600-0897.2004.00248.x
204. Kelly RW, Lindsay DR, Adams NR. Effect of Coumestans on Reproduction in the Ewe. J Agric Res (1976) 27:253–9. doi: 10.1071/AR9760253
205. Fair S, Meade KG, Reynaud K, Druart X, De Graaf SP. The Biological Mechanisms Regulating Sperm Selection by the Ovine Cervix. Reproduction (2019) 158:R1–R13. doi: 10.1530/REP-18-0595
206. June Mullins K, Saacke RG. Study of the Functional Anatomy of Bovine Cervical Mucosa With Special Reference to Mucus Secretion and Sperm Transport. Anat Rec (1989) 225:106–17. doi: 10.1002/ar.1092250205
207. Maddison JW, Rickard JP, Mooney E, Bernecic NC, Soleilhavoup C, Tsikis G, et al. Oestrus Synchronisation and Superovulation Alter the Production and Biochemical Constituents of Ovine Cervicovaginal Mucus. Anim Reprod Sci (2016) 172:114–22. doi: 10.1016/j.anireprosci.2016.07.008
208. Manes J, Ríos G, Fiorentino MA, Ungerfeld R. Vaginal Mucus From Ewes Treated With Progestogen Sponges Affects Quality of Ram Spermatozoa. Theriogenology (2016) 85:856–61. doi: 10.1016/j.theriogenology.2015.10.033
209. Lewis RA, Taylor D, Natavio MF, Melamed A, Felix J, Mishell D. Effects of the Levonorgestrel-Releasing Intrauterine System on Cervical Mucus Quality and Sperm Penetrability. Contraception (2010) 82:491–6. doi: 10.1016/j.contraception.2010.06.006
210. Maddison JW. Effects of Controlled Breeding on Cervicovaginal Mucus and Fertility of the Merino Ewe. Dissertation (2017).
211. Adams NR. Cervical Mucus Changes in Infertile Ewes Previously Exposed to Oestrogenic Subterranean Clover. Res Vet Sci (1976) 21:59–63. doi: 10.1016/S0034-5288(18)33394-0
212. Adams NR. Cervical Mucus and Reproductive Efficiency in Ewes After Exposure to Oestrogenic Pastures. Aust J Agric Res (1977) 28:481–9. doi: 10.1071/AR9770481
213. Sharkey DJ, Tremellen KP, Jasper MJ, Gemzell-Danielsson K, Robertson SA. Seminal Fluid Induces Leukocyte Recruitment and Cytokine and Chemokine mRNA Expression in the Human Cervix After Coitus. J Immunol (2012) 188:2445–54. doi: 10.4049/jimmunol.1102736
214. Quinlivan T. Studies in Ovine Reproduction: Sperm Transport and Fertilisation in Normal and Progestagen Treated Ewes. Univ Sydney: The University of Sydney (1967).
215. Robertson SA. Seminal Fluid Signaling in the Female Reproductive Tract: Lessons From Rodents and Pigs. J Anim Sci (2007) 85:36–44. doi: 10.2527/jas.2006-578
216. Schjenken JE, Robertson SA. The Female Response to Seminal Fluid. Physiol Rev (2020) 100:1077–117. doi: 10.1152/PHYSREV.00013.2018
217. Leahy T, Rickard JP, Bernecic NC, Druart X, De Graaf SP. Ram Seminal Plasma and Its Functional Proteomic Assessment. Reproduction (2019) 157:R243–56. doi: 10.1530/REP-18-0627
218. Chakroun-Feki N, Therond P, Couturier M, Eustache F, Limea G, Legrand A, et al. Human Sperm Quality and Lipid Content After Migration Into Normal Ovulatory Human Cervical Mucus Containing Low Numbers of Leukocytes. Asian J Androl (2009) 11:308–16. doi: 10.1038/aja.2008.25
Keywords: phytoestrogen, reproduction, endocrine disruptor, clover disease, ruminant
Citation: Pool KR, Chazal F, Smith JT and Blache D (2022) Estrogenic Pastures: A Source of Endocrine Disruption in Sheep Reproduction. Front. Endocrinol. 13:880861. doi: 10.3389/fendo.2022.880861
Received: 22 February 2022; Accepted: 25 March 2022;
Published: 28 April 2022.
Edited by:
Abdel Halim Harrath, King Saud University, Saudi ArabiaReviewed by:
Radoslava Vlčková, University of Veterinary Medicine and Pharmacy in Košice, SlovakiaAlexander Jurkevich, University of Missouri, United States
Copyright © 2022 Pool, Chazal, Smith and Blache. This is an open-access article distributed under the terms of the Creative Commons Attribution License (CC BY). The use, distribution or reproduction in other forums is permitted, provided the original author(s) and the copyright owner(s) are credited and that the original publication in this journal is cited, in accordance with accepted academic practice. No use, distribution or reproduction is permitted which does not comply with these terms.
*Correspondence: Kelsey R. Pool, kelsey.pool@uwa.edu.au