- 1Department of Cell and Molecular Biology, Fluminense Federal University, Niterói, Brazil
- 2Laboratory of Immunopharmacology, Federal University of the State of Rio de Janeiro, Rio de Janeiro, Brazil
- 3Postgraduate Program in Biotechnology, Fluminense Federal University, Rio de Janeiro, Brazil
Muscle and adipose tissue produce irisin during exercise. Irisin is thermogenic adipomyokine, improves glucose and lipid metabolism, and ameliorates the effects of obesity-driven inflammation, metabolic syndrome, and diabetes. In addition, exercise-induced irisin activates anti-inflammatory pathways and may play an essential role in improving the outcomes of inflammatory conditions, such as coronavirus disease (COVID-19). COVID-19 infection can activate different intracellular receptors and modulate various pathways during the course of the disease. The cytokine release storm (CRS) produced is significant because it promotes the context for systemic inflammation, which increases the risk of mortality in patients with severe acute respiratory syndrome coronavirus 2 (SARS-CoV2). In addition, viral infection and the resulting organ damage may stimulate the mitogen-activated protein kinase(MAPK) and toll-like receptor 4 (TLR4)/toll interleukin receptor (TIR)-domain-containing adaptor (MyD88) pathways while negatively modulating the AMP-activated protein kinase (AMPK) pathway, leading to increased inflammatory cytokine production. Exercise-induced irisin may counteract this inflammatory modulation by decreasing cytokine production. Consequently, increased irisin levels, as found in healthy patients, may favor a better prognosis in patients with SARS-CoV2. This review aims to explore the molecular mechanisms underlying the anti-inflammatory properties of irisin in mitigating CRS and preventing severe outcomes due to infection with SARS-CoV2.
Introduction
In 2012, a group of researchers reported the discovery of irisin, a previously uncharacterized hormone derived from the transmembrane protein fibronectin type III domain-containing protein 5 (FNDC5), which is widely expressed in numerous tissues (1). Based on the functional characterization of irisin, it may be defined as a myokine (1) and an adipokine released during exercise (2). Further research has found that irisin mediates the conversion of white adipose tissue to brown adipose tissue, resulting in a thermogenic effect (1). In addition, irisin improves glucose and lipid metabolism (3–5) and ameliorates the effects of obesity-driven inflammation, metabolic syndrome, and diabetes (6–8). As a result, several beneficial effects on human health derived from exercise, such as reduced inflammation in pathological states, may be ascribed in part to irisin secretion (9, 10). Given these positive effects of exercise-induced irisin, it may play a role in mitigating the effects of other conditions that trigger systemic inflammation.
Coronavirus disease 2019 (COVID-19) is a highly infectious disease caused by severe acute respiratory syndrome coronavirus 2 (SARS-CoV2), which has reached alarming proportions during the pandemic (11). Its pathological features include exacerbated inflammation and induction of a cytokine storm, which contribute to the development of severe immune reactions (12–14). Therefore, one of the main therapeutic strategies targets the hyperactivation of immune responses (12). Multiple studies hypothesize exercise to be a protective factor against COVID-19 through the downregulation of the cytokine storm and reduction of sequelae (15, 16). It can also mitigate the progress of the infection, improve immune response and even work as a coadjutant therapy for COVID-19 (17). In this way, one observational study was able to associate moderate levels of exercise with a lower prevalence of COVID-19-related hospitalization (18). However, the role of irisin as a potential mechanism mediating exercise’s benefits in COVID-19 outcomes has yet to be uncovered.
In this review, we aim to discuss the anti-inflammatory effects of irisin, focusing on the MAPK, AMPK, and TLR4/MyD88 intracellular pathways, and examine how exercise-induced irisin may improve the outcomes of inflammatory conditions, such as COVID-19.
Irisin
Irisin has 112 amino acid residues and is a proteolytic cleavage subproduct of the FNDC5 protein (1). FNDC5 is a type 1 membrane protein that contains 209 amino acids in mice and 212 in humans. The FNDC5 precursor has five domains: the signal sequence (29 aa), fibronectin type III (94 aa), functionally unidentified region (28 aa), transmembrane (19 aa), and cytoplasmic (39 aa) domains. The N-terminal signal sequence is located in the extracellular environment, and the cytoplasmic domain is located at the C-terminus (1, 19, 20).
Irisin has a very similar structure to fibronectin type III (FNIII) domains: a β-sandwich domain with four β-strands on one side and three on the other (19). However, irisin forms continuous antiparallel eight-stranded β-sheet dimers, a feature rarely found in other FNIII domains. Thus, irisin dimerization is associated with structural stability, receptor binding, and triggering signaling cascades (19).
In rodents, irisin was identified in different tissues: muscle, lung, liver pancreas, adrenal glands, kidneys, and even in the central nervous system (21, 22). In humans, FNDC5 is predominantly expressed in muscle tissue, and its expression patterns establish a few primary predictors of circulating irisin, such as muscle mass and age (23). Therefore, increased muscle tissue due to a resistance training program could be a way to increase circulating irisin levels, and these observations are substantiated by studies showing an increase in circulating irisin levels after aerobic and resistance exercise sessions (24, 25). Furthermore, lower circulating irisin levels in older people have been noted because human muscle tissue mass decreases annually after 50 years of age (26).
Because older adults (> 60 years old) have lower physiological irisin levels, their circulating irisin levels tend to increase at a higher rate than in younger adults after training sessions, with a higher increase noted after intense training (24). Although exercise frequency is positively associated with increased irisin blood levels (25) populations that frequently exercise did not receive equal benefits from training interventions (23, 24). Thus, further research is necessary to understand the relationship between exercise and circulating irisin levels in trained and untrained populations. Interestingly, participants with a greater body mass index were less influenced by the acute irisin response due to exercise, a condition termed “irisin resistance syndrome” (25). Therefore, subjects who showed lower body mass index (BMI) levels throughout the intervention also experienced notable increases in circulating irisin levels (24).
Both irisin and its precursor are N-glycosylated proteins (1). In FNDC5, two asparagines (Asn36 and Asn81) were identified as the glycosylation sites. The modification is associated with critical functions such as cellular stability and localization of FNDC5. In addition, glycosylation increases the half-life and membrane incorporation of the protein. In contrast, unglycosylated FNDC5 has a shorter half-life and is abundantly retained in the endoplasmic reticulum (27).
Irisin has two additional potentially glycosylated asparagine residues (Asn7 and Asn52). Glycosylation may stimulate irisin secretion because FNDC5 membrane incorporation depends on it. In addition, adipose tissue browning is intensified with glycosylated irisin, and glycosylation does not impact irisin dimerization (19, 27, 28).
The mechanisms associated with FNDC5 cleavage into irisin and its release are still uncertain, but a disintegrin and metalloproteinase (ADAM) family member are purportedly involved. ADAM family inhibitors caused irisin levels to decrease, even though FNDC5 expression did not change (29). The first characterized binding partners for irisin were found in adipocytes and osteocytes, and irisin was identified as a potential modulator of bone metabolism (30). In binding assays, integrins such as αV/β5, and to a lesser extent α1/β1, possess a high affinity for irisin. Furthermore, the expression of integrin-like signaling mediators increased with irisin stimulation. Conversely, irisin signaling decreased with the use of integrin inhibitors. Thus, the proposed receptors for irisin in tissues are the αV-integrin family of proteins (30, 31).
Irisin may act through an alternative pathway in the lungs of mice with ischemia/reperfusion-induced injury. Irisin may enter injured cells, protecting them by targeting the mitochondrial uncoupling protein 2 (UCP2), reducing cellular oxidative stress. Remarkably, this effect appears to be mediated by lipid raft endocytosis of irisin from the bloodstream, indicating that irisin may act regardless of the presence of the receptor (32).
FNDC5 and irisin expression are regulated by the transcriptional coactivator peroxisome proliferator-activated receptor-gamma coactivator 1 alpha (PGC1-α). An increase in PGC1-α expression in the muscle of transgenic mice is associated with the browning of white adipose tissue (WAT) in a process mediated by irisin (1). Additionally, PGC1-α is associated with exercise-mediated benefits and energy metabolism, leading to the hypothesis that exercise may stimulate irisin release (1)
PGC1-α interacts with several transcription factors to regulate FNDC5 synthesis during exercise. In particular, the transcription factors estrogen-related receptor alpha (ERRα) and cAMP response element-binding protein (CREB) are two presumed PGC1-α partners that increase FNDC5 synthesis (33, 34). Furthermore, nuclear hormone receptors such as glucocorticoid receptor (GR), constitutive androstane receptor (CAR), and farnesoid X receptor (FXR) also increase FNDC5 mRNA levels in cell cultures. Notably, cortisol can modulate FNDC5/irisin transcription in some human hepatoma cell lines (35–37). Because cortisol suppresses inflammation, we asked whether irisin could function alongside cortisol as an anti-inflammatory myokine in response to high-intensity exercise or inflammation.
Further, mothers against decapentaplegic homolog 3 (SMAD3) reduced FNDC5/irisin and PGC1-α expression because SMAD3 ablated mice showed increased serum irisin and high PGC1-α and FNDC5 expression in muscle. Additionally, TGF-β signaling activates SMAD3 signaling and blocks FNDC5 and PGC1-α transcription (38).
COVID-19
In 2020, the world witnessed the rise of a new infectious disease caused by viral pneumonia, named COVID-19. Disease outbreaks began in 2019 in Wuhan, China, and spread to other countries and continents (39). As a result, the World Health Organization declared the disease a public health emergency in January 2020 and a pandemic in March 2020. As the pandemic progressed, over 400 million cases of COVID-19 and over 5.5 million deaths have been reported worldwide (11). Although a few infected individuals remain healthy and asymptomatic, the clinical spectrum of COVID-19 varies greatly, with increasing severity found in groups presenting comorbidities, including obesity, hypertension, diabetes, cardiovascular diseases, and old age (40, 41). The main clinical symptoms include fever, cough, respiratory distress/pneumonia, headache, anosmia, and ageusia, although atypical symptoms may also occur (39, 42).
SARS-CoV-2 causes the COVID-19 (42–44). It is a single-stranded RNA virus capable of infecting humans and other mammals, and it promotes disease due to the virus’s features and interaction with the host’s immune system (45). The interaction of the viral capsid protein S (spike protein) and human receptor proteins, mainly ACE-2 (angiotensin-converting enzyme 2) and TMPRSS2 (transmembrane protease, serine 2) enable virus entry into host cells (46). Indeed, ACE-2 levels are correlated with SARS-CoV-2 invasiveness potential and tropism in specific tissues. Tissues and organs expressing ACE-2, such as the lungs, heart, adipose tissue, and gastrointestinal tract, are the preferred targets for viral invasion (39, 47).
After viral uptake and fusion, its genetic material is uncoated and expressed, resulting in viral life cycle progression and replication (46). In the replication state, few or no symptoms are prevalent because tissue damage and immune responses are minimal, whereas organ damage and increasing severity of symptoms may occur as the infection progresses (14, 48). With immune cell recruitment, cytokine production begins to occur during immune response exacerbation. The expression of several types of cytokines may be increased in patients with COVID-19, such as IL1β, IL1RA, IL6, IL7, IL8, IL9, IL10, IFNγ, and TNFα (49, 50). Hence, one of the hallmarks of COVID-19 pathophysiology, hyperimmune activation, leads to a cytokine storm that is directly linked to disease severity (51). Although considerable research has been done, the COVID-19 literature evolves with new data released, and further research will continue while the pandemic persists.
COVID-19-Related Inflammatory Pathways Modulated by Irisin
AMP-Activated Protein Kinase Pathway
Studies have investigated the intracellular signaling cascades involved in irisin activity. For example, one study associated the AMPK/mTOR signaling pathway with irisin’s reported effects such as improved glucose uptake by muscle cells, reduced inflammation and insulin resistance, reduced blood pressure, conversion of WAT to brown adipose tissue (BAT), promotion of autophagy, and inhibition of pancreatic cancer cell growth (52). Herein, we will focus on the signaling pathways involved in the modulation of immune responses, leading to reduced inflammation and cytokine release. Furthermore, we hypothesized that irisin treatment could mitigate the effects of the cytokine storm in patients with severe COVID-19 by modulating the AMPK pathway (53).
The AMPK pathway is activated in response to an increase in adenosine monophosphate (AMP) concentration which can result from several circumstances, such as exercise, decreased adenosine triphosphate (ATP) production, and activation by crosstalk with other pathways. AMPK activation leads to the phosphorylation of intracellular targets, regulating glucose and lipid metabolism, cell growth, gene transcription, and other functions such as mTOR activation blockage, which is an intracellular pathway linked to irisin signaling (54). Furthermore, integrin αV/β5 signaling may be involved in irisin-mediated AMPK activation (55), and the pathway is associated with the protective role of irisin against cellular damage/cytokines, in addition to suppressing inflammation (56). Exercise activates AMPK pathway. However, the effect magnitude will depend on exercise intensity, duration, glycogen availability, and training status (57). Interestingly, high-intensity training did not improve AMPK activation in hypertensive rats, which contrasts with low- and medium-intensity exercise (58).
Xiong et al. (2018) described the effects of FNDC5/irisin on inflammation and macrophage function in mouse adipose tissues. FNDC5 ablation resulted in inflammation, AMPK inhibition, and M1 polarization. The administration of FNDC5 and its overexpression alleviated these effects. M1 macrophages support inflammatory responses through proinflammatory cytokine secretion of factors such as tumor necrosis factor-α, and interleukin-1β (59). Macrophage dysregulation is observed in chronic inflammatory diseases, such as obesity, and the induction of M1 polarization triggers cytokine release, impairing adipose tissue function (60). Obesity and other chronic proinflammatory conditions are COVID-19 comorbidities that influence disease severity. Therefore, avoiding M1 polarization via irisin-AMPK pathway activation could protect against COVID-19 disease development.
The AMPK and mTOR signaling pathways are intimately related, with opposite effects on immune system regulation (61). Irisin activates AMPK and blocks the mTOR pathway (29), resulting in decreased NF-κB activity and a consequent decrease in innate and adaptive immune responses (62, 63). Therefore, these pathways were linked to COVID-19 hyper inflammation and may serve as a potential therapeutic target (64), and the anti-inflammatory effects of irisin may result due to AMPK/mTOR regulation.
Mitogen-Activated Protein Kinase Pathway
The MAPK pathway is extensively studied in association with irisin, comprising p38 and extracellular signal-regulated kinase (ERK)-mediated signaling. These pathways are related to several beneficial effects such as adipose tissue browning, glucose uptake, decreased insulin resistance, bone cell proliferation, and neural differentiation (52).
The activation of p38 MAPK induces cytokine release in individuals with COVID-19 (13, 65, 66). In addition, MAPKs are upregulated under several proinflammatory conditions and induce IL-6, IL-1β, and TNF-α secretion (67). Remarkably, irisin reduced inflammation and alleviated lung injury and acute respiratory distress syndrome in mice by decreasing the levels of the same cytokines released through MAPK pathway activation (68). Furthermore, irisin treatment decreased p38 and NF-κB activation induced by lipopolysaccharide (LPS) (68). Zhang et al. (2016) also reported anti-inflammatory effects of irisin treatment in treatment in mice for atherosclerosis, and found that IL-6 expression was reduced by p38 MAPK/NF-κB suppression (69).
Curiously, exercise triggers MAPK and NF-κB signaling pathways (70–75), and both promote proinflammatory states (67). P38 MAPK is an essential component in the cellular stress response that may act in physiological and pathological conditions, such as exercise and inflammatory states (67, 73). In addition, nonesterified fatty acid concentration may increase during exercise, stimulating TLR4/MAPK pathway, with no effect on NF-kB (73). Conversely, exercise may downregulate MAPK and NF-kB pathways. For instance, aerobic exercise attenuated proinflammatory macrophage polarization while decreased NF-kB and MAPK activation in LPS-exposed mice (76). Also, decreased p-p38 levels in rats’ lung inflammation submitted to aerobic exercise-trained (77). In addition, resistance training decreased MAPK activation after exercise (78, 79). Different aspects will change MAPK levels during and after exercise, such as age and exercise modality (80). The effects of irisin and exercise on MAPK/NF-kB are still controversial. However, a recent study sheds light on this debate: exercise-induced irisin counteracted inflammatory states by disturbing MD2-TLR4 complex formation and inhibiting MAPK and NF-kB pathways activation (81).
Toll-Like Receptor 4/MyD88 Pathway
Toll-like receptors (TLRs) in immune cells recognize pathogen-associated molecular patterns (PAMPs) or danger-associated molecular patterns (DAMPs) (82). For example, TLR4 recognizes LPS present on the wall of Gram-negative bacteria, whereas viral dsDNA activates TLR3 (83, 84). In addition, the Toll interleukin receptor (TIR)-domain-containing adaptor (MyD88) is part of the TLR signaling pathway in all TLR family members and triggers inflammatory responses (84). However, TLRs are known to act differently. For instance, TLR3 and TLR4 activate MyD88-independent signaling pathways to induce proinflammatory responses, whereas cells from MyD88-knockout mice are unresponsive to TLR7 activation (84, 85).
Another signaling pathway reportedly modulated by irisin and related to the regulation of inflammatory responses is the TLR4/MyD88 pathway (86, 87). High concentrations of irisin in mice decreased TLR4 and MyD88 mRNA levels, consequently reducing NF-κB activation (86, 87). In this context, a decrease in proinflammatory cytokine secretion for IL-1β, IL-6, and TNF-α in macrophages was observed (86). Studies have shown that irisin can reduce brain ischemic/reperfusion injury and the proinflammatory activity of macrophages (86, 87). Hence, through the negative modulation of the TLR4/MyD88 pathway, irisin could improve the condition of patients with COVID-19 and their outcomes.
With the progression of SARS-CoV2 infection, a CRS may occur, which is significantly associated with the mortality of patients with COVID-19 (13). CRS is generated by the release of viral components in infected cells, leading to the uncontrolled activation of inflammatory signaling pathways (88). CRS causes oxidative stress, disseminated intravascular coagulation, severe metabolic acidosis, and multi-organ failure, inducing “viral sepsis” (13).
The second stage of injury, induced by CRS during influenza infections, can be mediated by DAMP-TLR4 dependent activation that results in pulmonary injury (89). We hypothesized that similar events occur during SARS-CoV2 infection, in which DAMP released during the second stage of SARS-CoV2 infection can further damage the lungs (88–90). Therefore, as irisin reduces TLR4 expression levels, which could decrease patient immune responses to PAMPs and DAMPs, and maybe COVID-19 patient prognosis. A similar therapeutic approach has been proposed to enhance adenosine signaling and inhibit the TLR/NF-κB pathway, diminishing the associated adverse effects (88). Additionally, the SARS-CoV2 spike protein can activate TLR4 and induce IL-1β production (91). Therefore, irisin effects may be extended, potentially acting on the prevention of patient conditions deterioration and interfering with macrophage viral recognition.
Obesity is a comorbidity of SARSCoV2 infection, resulting in a poor prognosis that implicates other signaling pathways. First, the TLR4 pathway, which is activated by saturated fatty acids, is one of the primary triggers for obesity-induced inflammation (92–95). Second, obese patients show increased levels of fatty acids in the serum, making them susceptible to systemic non-esterified fatty acid (NEFA) cytotoxicity which is responsible for increased oxidative stress and tissue damage (93). In addition, NEFA cytotoxicity may be further accentuated in obese patients with severe COVID-19 due to decreased albumin levels, contributing to more free fatty acids in serum because these are transported in the plasma by albumin (93, 96). In addition to obesity, hypertension also is clinical comorbidity associated with higher mortality risk in COVID-19 patients (97). In hypertension, the renin-angiotensin-aldosterone system (98, 99) and LPS-TLR4-MyD88 pathway are activated (100–102) and might be a propensity to inflammatory events during SARS-CoV-2 infection. Also, proinflammatory cytokines may trigger TLR4/MyD88/NF-kB pathways activation, contributing to hypertensive response in the hypothalamus (103). Considering exercise effects on hypertension, low- and medium-intensity exercise effectively improved blood pressure through TLR4/NF-kB suppression (58) and improved hypertension in rats (103). Exercise modulates TLR4 and NF-kB expressions, depending on the training intensity: acute, moderate, low, and on the training frequency: acute and regular (104). Acute training could be potentially inflammatory, stimulating an increase in TLR4 levels on the cell surface (104, 105); while resistance training presents an anti-inflammatory pattern, leading to MyD88 and TLR4 downregulation of mRNA expression and protein levels (106, 107). Moderate regular exercise has anti-inflammatory properties, downregulating TLR4 and MyD88 (108–110). TLR4 downregulation by irisin could play a prominent role in preventing or decreasing hyperinflammation in obese and hypertensive patients, and healthy individuals who undergo regular moderate exercise. Thus, we suggest that activation of the TLR4 pathway in patients with COVID-19 may be linked to poor patient outcomes (3, 111).
Pseudomonas aeruginosa is the most common bacterial coinfection in patients with COVID-19 (112). P. aeruginosa is a Gram-negative bacterium associated with severe hospital-acquired infections and is a fundamental cause of sepsis (Ramachandran, 2014; Shafran et al., 2021). Irisin can ameliorate inflammation in mice with LPS-induced lung injury (68), which may be critical for patients with COVID-19 because they are prone to contracting bacterial infections during hospitalization (90, 112). Thus, irisin may prevent secondary Gram-negative bacterial immune response exacerbation and sepsis.
Besides regulating the activation of inflammatory pathways, irisin can modulate gene transcription and may potentially reduce the transcriptional levels of several genes related to COVID-19 (113). For example, irisin decreased the expression of TLR3, which is upregulated in COVID-19 patients, which prevented the hyperactivation of innate immunity. TLR3 ablation in mice resulted in increased susceptibility to other coronavirus infections, indicating the prevalence of unwanted outcomes for the irisin/TLR3 relationship (113, 114).
Discussion
Figure 1 briefly summarizes the inflammatory pathways that irisin may modulate during SARS-CoV2 infection, while Table 1 assembles the different effects of COVID-19, irisin, exercise, and sedentarism/aging over the inflammatory pathways discussed in this paper. In the first stage of the disease, SARS-CoV2 stimulates an innate immune response mediated specifically by macrophages, through pathogen-associated molecular patterns associated with TLR3, TLR7, and TLR8. In addition, the SARS-CoV-2 spike glycoprotein binds and activates TLR4 (3). Irisin can reduce TLR3 and TLR4 expression and possibly alleviates the innate immune response in the first stage of the disease, inhibiting the release of proinflammatory cytokines (Figure 1A) (29, 86).
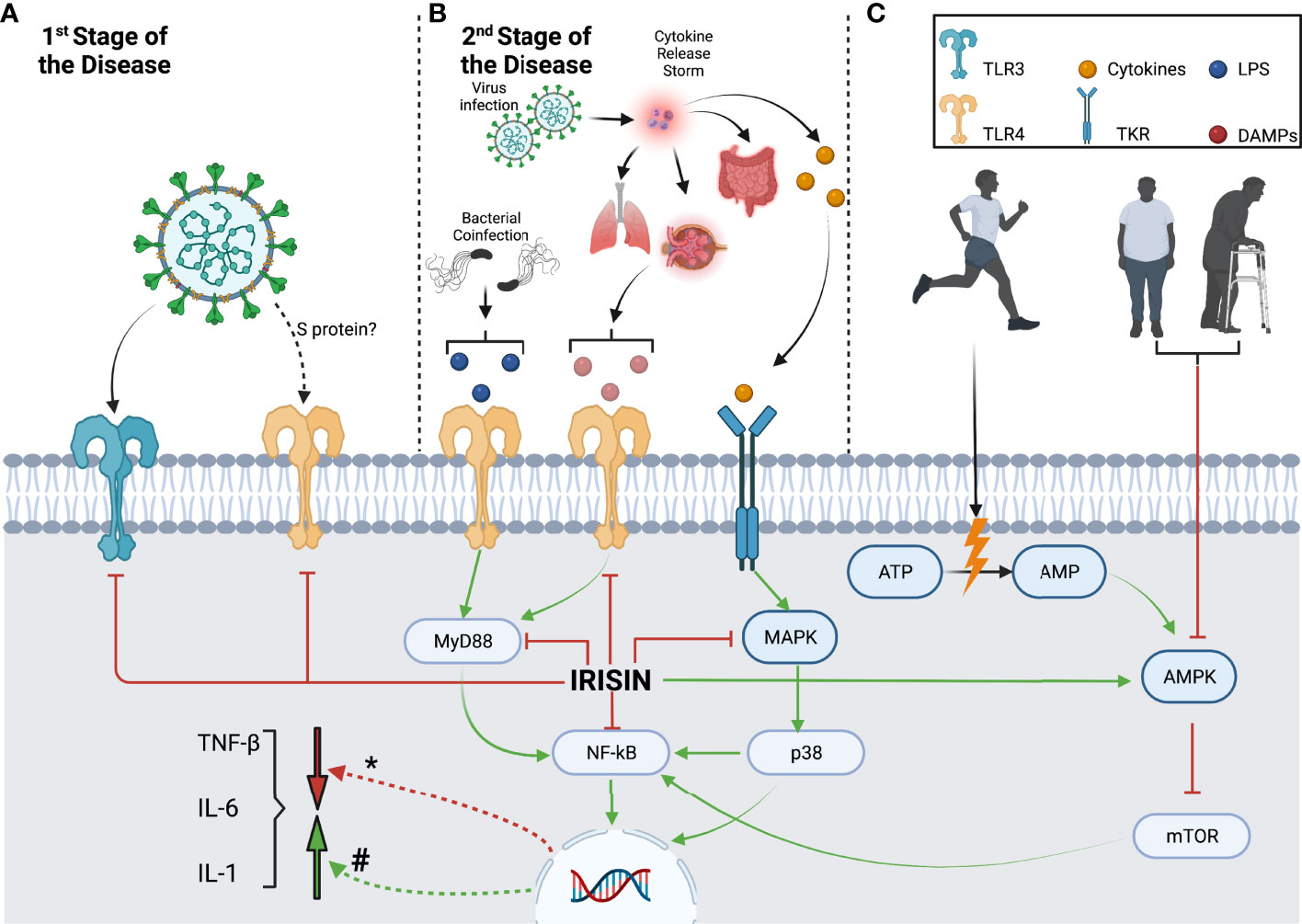
Figure 1 Macrophage inflammatory pathways modulated by irisin during different SARS-CoV2 infection stages and lifestyle contexts. (A) The first stage of SARS-CoV2 infection activates innate immune responses through the recognition of PAMPs by TLR3 and TLR4, which are downregulated by irisin. (B) The second stage of SARS-CoV2 infection causes cellular damage and induces a cytokine release storm, which activates the MAPK/p38/NF-kB pathway through TKR. CRS results in further organ damage, leading to DAMP release and MyD88/NF-kB pathway activation. Irisin can downregulate both these pathways, decreasing inflammatory cytokine production. In addition, the TLR4 and MyD88/NF-kB pathways can be stimulated by LPS, which can be released due to potential bacterial coinfection. (C) Although sedentarism and aging lead to AMPK downregulation and consequent mTOR activation, exercise produces the opposite effect. Exercise-derived AMP and irisin can stimulate AMPK, inhibiting mTOR and downregulating the NF-kB pathway while decreasing cytokine production (#) Cytokine transcription modulation by inflammatory pathways. (*) Cytokine transcription modulation by irisin.
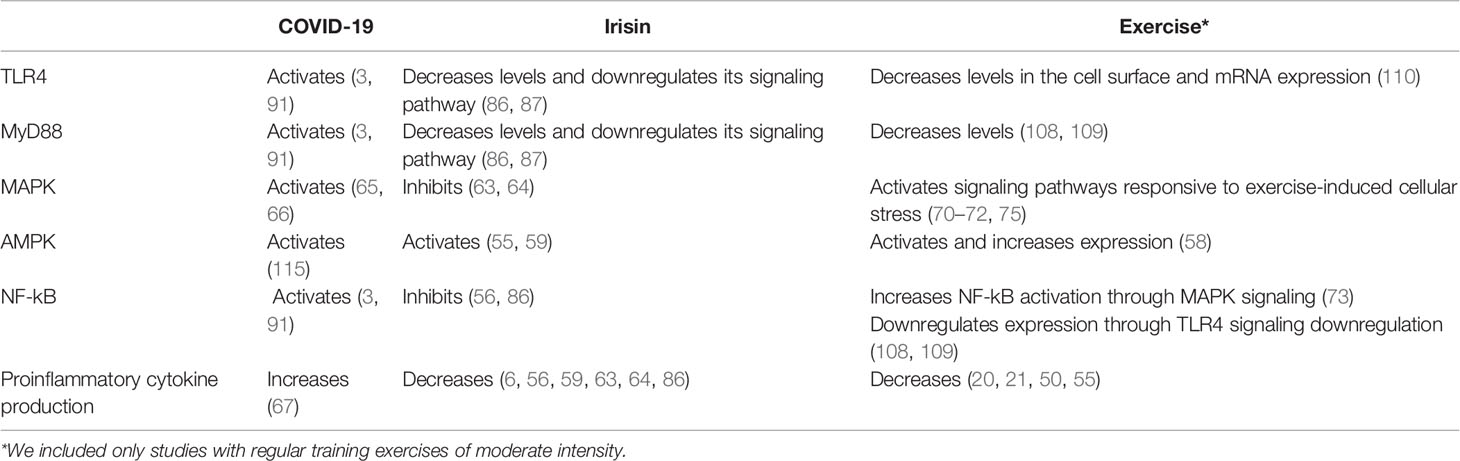
Table 1 COVID-19, Irisin, Exercise, Sedentarism/Aging, and Obesity influence related inflammatory pathways: TLR4, MyD88, MAPK, AMPK, NF-kB, and cytokine production.
In the second stage, the viral infection may result in a cytokine release storm that produces severe systemic inflammation. Increased cytokine levels activate tyrosine kinase receptors in macrophages, inducing MAPK pathway activation (67). In addition, severe systemic inflammation causes organ damage, inducing the production of DAMPs, which further activates the TLR4/MyD88 pathway (88, 90). Both the TLR4/MyD88 and MAPK pathways stimulate NF-kB, inducing an increase in the production of proinflammatory cytokines (Figure 1B) (13). Irisin has several modulation targets, including TLR4, MyD88, MAPK, and NF-κB. By reducing their expression levels, irisin reduces proinflammatory cytokine production (Figure 1B) (68). In addition, patients with COVID-19 may develop a bacterial coinfection that releases LPS and activates the TLR4/MyD88 pathway (112). Irisin causes a decrease in TLR4 and MyD88 levels, which could prevent the development of sepsis (Figure 1B).
Exercise increases energy expenditure and AMP levels, activates AMPK, and suppresses the mTOR pathway (54). On the other hand, sedentarism and aging may have the opposite effects, with a decrease in AMPK activation observed, whereas mTOR activation and proinflammatory cytokine production are induced (Figure 1C). Thus, irisin modulation is of particular interest as it counteracts the suppression of the AMPK pathway. In addition, irisin may also ameliorate the proinflammatory state by reducing mTOR and NF-κB activation (Figure 1C) (29, 59).
Even though irisin levels may vary following muscle mass, age, and involvement in physical activities, healthy and active people would benefit from irisin’s counterbalance mechanism, considering they would at least present physiological levels. For instance, obese, elderly, and sedentary subjects present loss of muscle mass and consequently lower irisin levels. These groups present a higher risk of developing COVID-19, with a lack of inflammatory counterbalance mechanism. Thus we suggest a potential role of irisin in preventing COVID-19 complications.
One limitation of the present work is that exercise, irisin, and inflammatory status are unveiled. Therefore, further studies have to be made to fulfill the gap in the current knowledge in the field. Furthermore, despite data on irisin and inflammation, COVID-19, and inflammation, the relations between irisin and COVID-19 are still poor. Furthermore, the knowledge about COVID-19 pathophysiology continues to evolve concerning the differences between current and future variants. So further studies will help to understand the role of irisin on COVID-19 outcome. Therefore, this review highlights the potential relation between irisin, inflammation, and COVID-19.
Together, increased irisin levels may counteract the adverse effects of COVID-19 during the various disease stages. Therefore, we hypothesized that irisin could prevent excessive inflammation, perhaps decreasing organ damage and dysfunction and secondary bacterial infection during hospitalization. Irisin’s anti-inflammatory modulation properties may also explain, at least in part, why healthy patients generally have a better prognosis for SARS-CoV2 infection. Further research is needed to understand the role of irisin on COVID-19 patients and may help develop new prevention and treatment strategies.
Author Contributions
Conceptualization, writing—original draft preparation and editing, and funding acquisition: HA, GL, CF, Gd-A and PB. Final approval: PB and CG. All authors have read and agreed to the published version of the manuscript. All authors contributed to the article and approved the submitted version.
Funding
This work was supported by grants from Universidade Federal Fluminense (PROPPI/UFF), Coordenação de Aperfeiçoamento de Pessoal de Nível Superior (CAPES) Grant [001], Programa de Biotecnologia da Universidade Federal Fluminense (UFF), Programa de Pós Graduação em Biologia Molecular Celular (UNIRIO), Universidade Federal do Estado do Rio de Janeiro (UNIRIO), Fundação Carlos Chagas Filho de Amparo à Pesquisa do Estado do Rio de Janeiro (FAPERJ) Grants [E-26/010.000983/2019, E-26/203.290/2017, and E-26/2010.592/2019, E-26/201.448/2021], Conselho Nacional de Desenvolvimento Científico e Tecnológico (CNPq), and Instituto Oswaldo Cruz, FIOCRUZ. We also acknowledge financial support by the European Community’s Seventh Framework Programme (FP7-2007-2013) under grant agreement [HEALTH-F4-2011-282095] (TARKINAID).
Conflict of Interest
The authors declare that the research was conducted in the absence of any commercial or financial relationships that could be construed as a potential conflict of interest.
Publisher’s Note
All claims expressed in this article are solely those of the authors and do not necessarily represent those of their affiliated organizations, or those of the publisher, the editors and the reviewers. Any product that may be evaluated in this article, or claim that may be made by its manufacturer, is not guaranteed or endorsed by the publisher.
References
1. Bostrom P, Wu J, Jedrychowski MP, Korde A, Ye L, Lo JC, et al. A PGC1-Alpha-Dependent Myokine That Drives Brown-Fat-Like Development of White Fat and Thermogenesis. Nature (2012) 481:463–8. doi: 10.1038/nature10777
2. Roca-Rivada A, Castelao C, Senin LL, Landrove MO, Baltar J, Belen Crujeiras A, et al. FNDC5/irisin Is Not Only a Myokine But Also an Adipokine. PloS One (2013) 8:e60563. doi: 10.1371/journal.pone.0060563
3. Aboudounya MM, Heads RJ. COVID-19 and Toll-Like Receptor 4 (TLR4): SARS-CoV-2 May Bind and Activate TLR4 to Increase ACE2 Expression, Facilitating Entry and Causing Hyperinflammation. Mediators Inflamm (2021) 2021:8874339. doi: 10.1155/2021/8874339
4. So WY, Leung PS. Irisin Ameliorates Hepatic Glucose/Lipid Metabolism and Enhances Cell Survival in Insulin-Resistant Human HepG2 Cells Through Adenosine Monophosphate-Activated Protein Kinase Signaling. Int J Biochem Cell Biol (2016) 78:237–47. doi: 10.1016/j.biocel.2016.07.022
5. Perakakis N, Triantafyllou GA, Fernandez-Real JM, Huh JY, Park KH, Seufert J, et al. Physiology and Role of Irisin in Glucose Homeostasis. Nat Rev Endocrinol (2017) 13:324–37. doi: 10.1038/nrendo.2016.221
6. Cao RY, Zheng H, Redfearn D, Yang J. FNDC5: A Novel Player in Metabolism and Metabolic Syndrome. Biochimie (2019) 158:111–6. doi: 10.1016/j.biochi.2019.01.001
7. Liu TY, Shi CX, Gao R, Sun HJ, Xiong XQ, Ding L, et al. Irisin Inhibits Hepatic Gluconeogenesis and Increases Glycogen Synthesis via the PI3K/Akt Pathway in Type 2 Diabetic Mice and Hepatocytes. Clin Sci (Lond) (2015) 129:839–50. doi: 10.1042/CS20150009
8. Polyzos SA, Anastasilakis AD, Efstathiadou ZA, Makras P, Perakakis N, Kountouras J, et al. Irisin in Metabolic Diseases. Endocrine (2018) 59:260–74. doi: 10.1007/s12020-017-1476-1
9. Metsios GS, Moe RH, Kitas GD. Exercise and Inflammation. Best Pract Res Clin Rheumatol (2020) 34:101504. doi: 10.1016/j.berh.2020.101504
10. Pedersen BK. Anti-Inflammatory Effects of Exercise: Role in Diabetes and Cardiovascular Disease. Eur J Clin Invest (2017) 47:600–11. doi: 10.1111/eci.12781
11. Li J, Lai S, Gao GF, Shi W. The Emergence, Genomic Diversity and Global Spread of SARS-CoV-2. Nature (2021) 600:408–18. doi: 10.1038/s41586-021-04188-6
12. Anka AU, Tahir MI, Abubakar SD, Alsabbagh M, Zian Z, Hamedifar H, et al. Coronavirus Disease 2019 (COVID-19): An Overview of the Immunopathology, Serological Diagnosis and Management. Scand J Immunol (2021) 93:e12998. doi: 10.1111/sji.12998
13. Choudhary S, Sharma K, Silakari O. The Interplay Between Inflammatory Pathways and COVID-19: A Critical Review on Pathogenesis and Therapeutic Options. Microb Pathog (2021) 150:104673. doi: 10.1016/j.micpath.2020.104673
14. Garcia LF. Immune Response, Inflammation, and the Clinical Spectrum of COVID-19. Front Immunol (2020) 11:1441. doi: 10.3389/fimmu.2020.01441
15. Fernandez-Lazaro D, Gonzalez-Bernal JJ, Sanchez-Serrano N, Navascues LJ, Ascaso-Del-Rio A, Mielgo-Ayuso J. Physical Exercise as a Multimodal Tool for COVID-19: Could It Be Used as a Preventive Strategy? Int J Environ Res Public Health (2020) 17:8496. doi: 10.3390/ijerph17228496
16. Heffernan KS, Ranadive SM, Jae SY. Exercise as Medicine for COVID-19: On PPAR With Emerging Pharmacotherapy. Med Hypotheses (2020) 143:110197. doi: 10.1016/j.mehy.2020.110197
17. Filgueira TO, Castoldi A, Santos LER, de Amorim GJ, de Sousa Fernandes MS, Anastacio W, et al. The Relevance of a Physical Active Lifestyle and Physical Fitness on Immune Defense: Mitigating Disease Burden, With Focus on COVID-19 Consequences. Front Immunol (2021) 12:587146. doi: 10.3389/fimmu.2021.587146
18. de Souza FR, Motta-Santos D, Dos Santos Soares D, de Lima JB, Cardozo GG, Guimaraes LSP, et al. Association of Physical Activity Levels and the Prevalence of COVID-19-Associated Hospitalization. J Sci Med Sport (2021) 24:913–8. doi: 10.1016/j.jsams.2021.05.011
19. Schumacher MA, Chinnam N, Ohashi T, Shah RS, Erickson HP. The Structure of Irisin Reveals a Novel Intersubunit Beta-Sheet Fibronectin Type III (FNIII) Dimer: Implications for Receptor Activation. J Biol Chem (2013) 288:33738–44. doi: 10.1074/jbc.M113.516641
20. Panati K, Narala VR, Narasimha VR, Derangula M, Arva Tatireddigari VRR, Yeguvapalli S. Expression, Purification and Biological Characterisation of Recombinant Human Irisin (12.5kda). J Genet Eng Biotechnol (2018) 16:459–66. doi: 10.1016/j.jgeb.2018.06.007
21. Gur FM, Timurkaan S, Yalcin MH, Girgin A, Gencer Tarakci B. Immunohistochemical Localization of Irisin in Mole Rats (Spalax Leucodon). Biotechnic Histochem Off Publ Biol Stain Commission (2017) 92:245–51. doi: 10.1080/10520295.2017.1303194
22. Timurkaan S, Gur FM, Gencer Tarakci B, Yalcin MH, Girgin M. Identification of Irisin Immunoreactivity in Porcupine (Hystrix Cristata) Adrenal Glands and Kidneys. Anatomia Histologia Embryologia (2018) 47:405–9. doi: 10.1111/ahe.12371
23. Huh JY, Panagiotou G, Mougios V, Brinkoetter M, Vamvini MT, Schneider BE, et al. FNDC5 and Irisin in Humans: I. Predictors of Circulating Concentrations in Serum and Plasma and II. mRNA Expression and Circulating Concentrations in Response to Weight Loss and Exercise. Metabolism (2012) 61:1725–38. doi: 10.1016/j.metabol.2012.09.002
24. Cosio PL, Crespo-Posadas M, Velarde-Sotres A, Pelaez M. Effect of Chronic Resistance Training on Circulating Irisin: Systematic Review and Meta-Analysis of Randomized Controlled Trials. Int J Environ Res Public Health (2021) 18:2476. doi: 10.3390/ijerph18052476
25. Fox J, Rioux BV, Goulet EDB, Johanssen NM, Swift DL, Bouchard DR, et al. Effect of an Acute Exercise Bout on Immediate Post-Exercise Irisin Concentration in Adults: A Meta-Analysis. Scand J Med Sci Sports (2018) 28:16–28. doi: 10.1111/sms.12904
26. von Haehling S, Morley JE, Anker SD. An Overview of Sarcopenia: Facts and Numbers on Prevalence and Clinical Impact. J Cachexia Sarcopenia Muscle (2010) 1:129–33. doi: 10.1007/s13539-010-0014-2
27. Nie Y, Liu D. N-Glycosylation is Required for FDNC5 Stabilization and Irisin Secretion. Biochem J (2017) 474:3167–77. doi: 10.1042/BCJ20170241
28. Zhang Y, Li R, Meng Y, Li S, Donelan W, Zhao Y, et al. Irisin Stimulates Browning of White Adipocytes Through Mitogen-Activated Protein Kinase P38 MAP Kinase and ERK MAP Kinase Signaling. Diabetes (2014) 63:514–25. doi: 10.2337/db13-1106
29. Yu Q, Kou W, Xu X, Zhou S, Luan P, Xu X, et al. FNDC5/Irisin Inhibits Pathological Cardiac Hypertrophy. Clin Sci (2019) 133:611–27. doi: 10.1042/CS20190016
30. Greenhill C. Irisin Receptor in Osteocytes Identified. Nat Rev Endocrinol (2019) 15:63. doi: 10.1038/s41574-018-0151-9
31. Kim H, Wrann CD, Jedrychowski M, Vidoni S, Kitase Y, Nagano K, et al. Irisin Mediates Effects on Bone and Fat via alphaV Integrin Receptors. Cell (2018) 175:1756–1768.e17. doi: 10.1016/j.cell.2018.10.025
32. Chen K, Xu Z, Liu Y, Wang Z, Li Y, Xu X, et al. Irisin Protects Mitochondria Function During Pulmonary Ischemia/Reperfusion Injury. Sci Transl Med (2017) 9:eaao6298. doi: 10.1126/scitranslmed.aao6298
33. Wrann CD, White JP, Salogiannnis J, Laznik-Bogoslavski D, Wu J, Ma D, et al. Exercise Induces Hippocampal BDNF Through a PGC-1alpha/FNDC5 Pathway. Cell Metab (2013) 18:649–59. doi: 10.1016/j.cmet.2013.09.008
34. Yang XY, Tse MCL, Hu X, Jia WH, Du GH, Chan CB. Interaction of CREB and PGC-1alpha Induces Fibronectin Type III Domain-Containing Protein 5 Expression in C2C12 Myotubes. Cell Physiol Biochem (2018) 50:1574–84. doi: 10.1159/000494655
35. Kim HK, Jeong YJ, Song IS, Noh YH, Seo KW, Kim M, et al. Glucocorticoid Receptor Positively Regulates Transcription of FNDC5 in the Liver. Sci Rep (2017) 7:43296. doi: 10.1038/srep43296
36. Li H, Shen J, Wu T, Kuang J, Liu Q, Cheng S, et al. Irisin Is Controlled by Farnesoid X Receptor and Regulates Cholesterol Homeostasis. Front Pharmacol (2019) 10:548. doi: 10.3389/fphar.2019.00548
37. Mo L, Shen J, Liu Q, Zhang Y, Kuang J, Pu S, et al. Irisin Is Regulated by CAR in Liver and Is a Mediator of Hepatic Glucose and Lipid Metabolism. Mol Endocrinol (2016) 30:533–42. doi: 10.1210/me.2015-1292
38. Tiano JP, Springer DA, Rane SG. SMAD3 Negatively Regulates Serum Irisin and Skeletal Muscle FNDC5 and Peroxisome Proliferator-Activated Receptor Gamma Coactivator 1-Alpha (PGC-1alpha) During Exercise. J Biol Chem (2015) 290:7671–84. doi: 10.1074/jbc.M114.617399
39. Zou X, Chen K, Zou J, Han P, Hao J, Han Z. Single-Cell RNA-Seq Data Analysis on the Receptor ACE2 Expression Reveals the Potential Risk of Different Human Organs Vulnerable to 2019-Ncov Infection. Front Med (2020) 14:185–92. doi: 10.1007/s11684-020-0754-0
40. Brodin P. Immune Determinants of COVID-19 Disease Presentation and Severity. Nat Med (2021) 27:28–33. doi: 10.1038/s41591-020-01202-8
41. Ejaz H, Alsrhani A, Zafar A, Javed H, Junaid K, Abdalla AE, et al. COVID-19 and Comorbidities: Deleterious Impact on Infected Patients. J Infect Public Health (2020) 13:1833–9. doi: 10.1016/j.jiph.2020.07.014
42. Wu F, Zhao S, Yu B, Chen YM, Wang W, Song ZG, et al. A New Coronavirus Associated With Human Respiratory Disease in China. Nature (2020) 579:265–9. doi: 10.1038/s41586-020-2008-3
43. Domingo JL. What We Know and What We Need to Know About the Origin of SARS-CoV-2. Environ Res (2021) 200:111785. doi: 10.1016/j.envres.2021.111785
44. Zhou P, Yang XL, Wang XG, Hu B, Zhang L, Zhang W, et al. A Pneumonia Outbreak Associated With a New Coronavirus of Probable Bat Origin. Nature (2020) 579:270–3. doi: 10.1038/s41586-020-2012-7
45. Trougakos IP, Stamatelopoulos K, Terpos E, Tsitsilonis OE, Aivalioti E, Paraskevis D, et al. Insights to SARS-CoV-2 Life Cycle, Pathophysiology, and Rationalized Treatments That Target COVID-19 Clinical Complications. J BioMed Sci (2021) 28:9. doi: 10.1186/s12929-020-00703-5
46. V'Kovski P, Kratzel A, Steiner S, Stalder H, Thiel V. Coronavirus Biology and Replication: Implications for SARS-CoV-2. Nat Rev Microbiol (2021) 19:155–70. doi: 10.1038/s41579-020-00468-6
47. Hamming I, Timens W, Bulthuis ML, Lely AT, Navis G, van Goor H. Tissue Distribution of ACE2 Protein, the Functional Receptor for SARS Coronavirus. A First Step in Understanding SARS Pathogenesis. J Pathol (2004) 203:631–7. doi: 10.1002/path.1570
48. Wang Z, Zhou M, Fu Z, Zhao L. The Pathogenic Features of Severe Acute Respiratory Syndrome Coronavirus 2 (SARS-CoV-2): Possible Mechanisms for Immune Evasion? Front Immunol (2021) 12:693579. doi: 10.3389/fimmu.2021.693579
49. Huang C, Wang Y, Li X, Ren L, Zhao J, Hu Y, et al. Clinical Features of Patients Infected With 2019 Novel Coronavirus in Wuhan, China. Lancet (2020) 395:497–506. doi: 10.1016/S0140-6736(20)30183-5
50. Wiersinga WJ, Rhodes A, Cheng AC, Peacock SJ, Prescott HC. Pathophysiology, Transmission, Diagnosis, and Treatment of Coronavirus Disease 2019 (COVID-19): A Review. JAMA (2020) 324:782–93. doi: 10.1001/jama.2020.12839
51. Fajgenbaum DC, June CH. Cytokine Storm. N Engl J Med (2020) 383:2255–73. doi: 10.1056/NEJMra2026131
52. Rabiee F, Lachinani L, Ghaedi S, Nasr-Esfahani MH, Megraw TL, Ghaedi K. New Insights Into the Cellular Activities of Fndc5/Irisin and its Signaling Pathways. Cell Biosci (2020) 10:51. doi: 10.1186/s13578-020-00413-3
53. Kim JS, Lee JY, Yang JW, Lee KH, Effenberger M, Szpirt W, et al. Immunopathogenesis and Treatment of Cytokine Storm in COVID-19. Theranostics (2021) 11:316–29. doi: 10.7150/thno.49713
54. Mihaylova MM, Shaw RJ. The AMPK Signalling Pathway Coordinates Cell Growth, Autophagy and Metabolism. Nat Cell Biol (2011) 13:1016–23. doi: 10.1038/ncb2329
55. Bi J, Zhang J, Ren Y, Du Z, Li T, Wang T, et al. Irisin Reverses Intestinal Epithelial Barrier Dysfunction During Intestinal Injury via Binding to the Integrin Alphavbeta5 Receptor. J Cell Mol Med (2020) 24:996–1009. doi: 10.1111/jcmm.14811
56. Bi J, Zhang J, Ren Y, Du Z, Zhang Y, Liu C, et al. Exercise Hormone Irisin Mitigates Endothelial Barrier Dysfunction and Microvascular Leakage-Related Diseases. JCI Insight (2020) 5:e136277. doi: 10.1172/jci.insight.136277
57. Rothschild JA, Islam H, Bishop DJ, Kilding AE, Stewart T, Plews DJ. Factors Influencing AMPK Activation During Cycling Exercise: A Pooled Analysis and Meta-Regression. Sports Med (2021). doi: 10.1007/s40279-021-01610-x
58. Luo M, Cao C, Niebauer J, Yan J, Ma X, Chang Q, et al. Effects of Different Intensities of Continuous Training on Vascular Inflammation and Oxidative Stress in Spontaneously Hypertensive Rats. J Cell Mol Med (2021) 25:8522–36. doi: 10.1111/jcmm.16813
59. Xiong XQ, Geng Z, Zhou B, Zhang F, Han Y, Zhou YB, et al. FNDC5 Attenuates Adipose Tissue Inflammation and Insulin Resistance via AMPK-Mediated Macrophage Polarization in Obesity. Metabolism (2018) 83:31–41. doi: 10.1016/j.metabol.2018.01.013
60. Russo L, Lumeng CN. Properties and Functions of Adipose Tissue Macrophages in Obesity. Immunology (2018) 155:407–17. doi: 10.1111/imm.13002
61. Jeon SM. Regulation and Function of AMPK in Physiology and Diseases. Exp Mol Med (2016) 48:e245. doi: 10.1038/emm.2016.81
62. Cavalli G, Dinarello CA. Suppression of Inflammation and Acquired Immunity by IL-37. Immunol Rev (2018) 281:179–90. doi: 10.1111/imr.12605
63. Kracht M, Muller-Ladner U, Schmitz ML. Mutual Regulation of Metabolic Processes and Proinflammatory NF-KappaB Signaling. J Allergy Clin Immunol (2020) 146:694–705. doi: 10.1016/j.jaci.2020.07.027
64. Maiese K. The Mechanistic Target of Rapamycin (mTOR): Novel Considerations as an Antiviral Treatment. Curr Neurovasc Res (2020) 17:332–7. doi: 10.2174/1567202617666200425205122
65. Grimes JM, Grimes KV. P38 MAPK Inhibition: A Promising Therapeutic Approach for COVID-19. J Mol Cell Cardiol (2020) 144:63–5. doi: 10.1016/j.yjmcc.2020.05.007
66. Hussman JP. Cellular and Molecular Pathways of COVID-19 and Potential Points of Therapeutic Intervention. Front Pharmacol (2020) 11:1169. doi: 10.3389/fphar.2020.01169
67. Zarubin T, Han J. Activation and Signaling of the P38 MAP Kinase Pathway. Cell Res (2005) 15:11–8. doi: 10.1038/sj.cr.7290257
68. Shao L, Meng D, Yang F, Song H, Tang D. Irisin-Mediated Protective Effect on LPS-Induced Acute Lung Injury via Suppressing Inflammation and Apoptosis of Alveolar Epithelial Cells. Biochem Biophys Res Commun (2017) 487:194–200. doi: 10.1016/j.bbrc.2017.04.020
69. Zhang Y, Mu Q, Zhou Z, Song H, Zhang Y, Wu F, et al. Protective Effect of Irisin on Atherosclerosis via Suppressing Oxidized Low Density Lipoprotein Induced Vascular Inflammation and Endothelial Dysfunction. PloS One (2016) 11:e0158038. doi: 10.1371/journal.pone.0158038
70. Akimoto T, Pohnert SC, Li P, Zhang M, Gumbs C, Rosenberg PB, et al. Exercise Stimulates Pgc-1alpha Transcription in Skeletal Muscle Through Activation of the P38 MAPK Pathway. J Biol Chem (2005) 280:19587–93. doi: 10.1074/jbc.M408862200
71. Wen C, Ying Y, Zhao H, Jiang Q, Gan X, Wei Y, et al. Resistance Exercise Affects Catheter-Related Thrombosis in Rats Through miR-92a-3p, Oxidative Stress and the MAPK/NF-kappaB Pathway. BMC Cardiovasc Disord (2021) 21:440. doi: 10.1186/s12872-021-02233-w
72. Bouviere J, Fortunato RS, Dupuy C, Werneck-de-Castro JP, Carvalho DP, Louzada RA. Exercise-Stimulated ROS Sensitive Signaling Pathways in Skeletal Muscle. Antioxidants (2021) 10:537. doi: 10.3390/antiox10040537
73. Kramer HF, Goodyear LJ, Exercise MAPK. And NF-kappaB Signaling in Skeletal Muscle. J Appl Physiol (2007) 103:388–95. doi: 10.1152/japplphysiol.00085.2007
74. Holm L, van Hall G, Rose AJ, Miller BF, Doessing S, Richter EA, et al. Contraction Intensity and Feeding Affect Collagen and Myofibrillar Protein Synthesis Rates Differently in Human Skeletal Muscle. Am J Physiol Endocrinol Metab (2010) 298:E257–69. doi: 10.1152/ajpendo.00609.2009
75. Galpin AJ, Fry AC, Chiu LZ, Thomason DB, Schilling BK. High-Power Resistance Exercise Induces MAPK Phosphorylation in Weightlifting Trained Men. Appl Physiol Nutrition Metab = Physiologie Appliquee Nutr metabolisme (2012) 37:80–7. doi: 10.1139/h11-131
76. Shi Y, Liu T, Nieman DC, Cui Y, Li F, Yang L, et al. Aerobic Exercise Attenuates Acute Lung Injury Through NET Inhibition. Front Immunol (2020) 11:409. doi: 10.3389/fimmu.2020.00409
77. Qin F, Fan Z, Xu M, Wang Z, Dong Y, Qu C, et al. Amelioration of Ambient Particulate Matter (PM2.5)-Induced Lung Injury in Rats by Aerobic Exercise Training. Front Physiol (2021) 12:731594. doi: 10.3389/fphys.2021.731594
78. Takegaki J, Sase K, Fujita S. Repeated Bouts of Resistance Exercise Attenuate Mitogen-Activated Protein-Kinase Signal Responses in Rat Skeletal Muscle. Biochem Biophys Res Commun (2019) 520:73–8. doi: 10.1016/j.bbrc.2019.09.050
79. Coffey VG, Zhong Z, Shield A, Canny BJ, Chibalin AV, Zierath JR, et al. Early Signaling Responses to Divergent Exercise Stimuli in Skeletal Muscle From Well-Trained Humans. FASEB J Off Publ Fed Am Societies Exp Biol (2006) 20:190–2. doi: 10.1096/fj.05-4809fje
80. Williamson D, Gallagher P, Harber M, Hollon C, Trappe S. Mitogen-Activated Protein Kinase (MAPK) Pathway Activation: Effects of Age and Acute Exercise on Human Skeletal Muscle. J Physiol (2003) 547:977–87. doi: 10.1113/jphysiol.2002.036673
81. Zhu W, Sahar NE, Javaid HMA, Pak ES, Liang G, Wang Y, et al. Exercise-Induced Irisin Decreases Inflammation and Improves NAFLD by Competitive Binding With MD2. Cells (2021) 10:3306. doi: 10.3390/cells10123306
82. Jeong E, Lee JY. Intrinsic and Extrinsic Regulation of Innate Immune Receptors. Yonsei Med J (2011) 52:379–92. doi: 10.3349/ymj.2011.52.3.379
83. Maldonado RF, Sa-Correia I, Valvano MA. Lipopolysaccharide Modification in Gram-Negative Bacteria During Chronic Infection. FEMS Microbiol Rev (2016) 40:480–93. doi: 10.1093/femsre/fuw007
84. Kawasaki T, Kawai T. Toll-Like Receptor Signaling Pathways. Front Immunol (2014) 5:461. doi: 10.3389/fimmu.2014.00461
85. Deguine J, Barton GM. MyD88: A Central Player in Innate Immune Signaling. F1000Prime Rep (2014) 6:97. doi: 10.12703/P6-97
86. Mazur-Bialy AI, Pochec E, Zarawski M. Anti-Inflammatory Properties of Irisin, Mediator of Physical Activity, Are Connected With TLR4/MyD88 Signaling Pathway Activation. Int J Mol Sci (2017) 18:701. doi: 10.3390/ijms18040701
87. Yu Q, Li G, Ding Q, Tao L, Li J, Sun L, et al. Irisin Protects Brain Against Ischemia/Reperfusion Injury Through Suppressing TLR4/MyD88 Pathway. Cerebrovasc Dis (2020) 49:346–54. doi: 10.1159/000505961
88. Silva-Lagos LA, Pillay J, van Meurs M, Smink A, van der Voort PHJ, de Vos P. DAMPening COVID-19 Severity by Attenuating Danger Signals. Front Immunol (2021) 12:720192. doi: 10.3389/fimmu.2021.720192
89. Shirey KA, Blanco JCG, Vogel SN. Targeting TLR4 Signaling to Blunt Viral-Mediated Acute Lung Injury. Front Immunol (2021) 12:705080. doi: 10.3389/fimmu.2021.705080
90. Brandao SCS, Ramos JOX, Dompieri LT, Godoi E, Figueiredo JL, Sarinho ESC, et al. Is Toll-Like Receptor 4 Involved in the Severity of COVID-19 Pathology in Patients With Cardiometabolic Comorbidities? Cytokine Growth Factor Rev (2021) 58:102–10. doi: 10.1016/j.cytogfr.2020.09.002
91. Zhao Y, Kuang M, Li J, Zhu L, Jia Z, Guo X, et al. SARS-CoV-2 Spike Protein Interacts With and Activates TLR41. Cell Res (2021) 31:818–20. doi: 10.1038/s41422-021-00495-9
92. Rogero MM, Calder PC. Obesity, Inflammation, Toll-Like Receptor 4 and Fatty Acids. Nutrients (2018) 10:818–820. doi: 10.3390/nu10040432
93. Goncalves-de-Albuquerque CF, Barnese MRC, Soares MA, Castro-Faria MV, Silva AR, de Castro-Faria-Neto HC, et al. Serum Albumin Saturation Test Based on non-Esterified Fatty Acids Imbalance for Clinical Employment. Clin Chim Acta (2019) 495:422–8. doi: 10.1016/j.cca.2019.05.003
94. Carvalho MV, Goncalves-de-Albuquerque CF, Silva AR. PPAR Gamma: From Definition to Molecular Targets and Therapy of Lung Diseases. Int J Mol Sci (2021) 22. doi: 10.3390/ijms22020805
95. Silva AR, Moraes BPT, Goncalves-de-Albuquerque CF. Mediterranean Diet: Lipids, Inflammation, and Malaria Infection. Int J Mol Sci (2020) 21. doi: 10.3390/ijms21124489
96. Violi F, Cangemi R, Romiti GF, Ceccarelli G, Oliva A, Alessandri F, et al. Is Albumin Predictor of Mortality in COVID-19? Antioxid Redox Signal (2021) 35:139–42. doi: 10.1089/ars.2020.8142
97. Lippi G, Wong J, Henry BM. Hypertension in Patients With Coronavirus Disease 2019 (COVID-19): A Pooled Analysis. Polish Arch Internal Med (2020) 130:304–9. doi: 10.20452/pamw.15272
98. Peng M, He J, Xue Y, Yang X, Liu S, Gong Z. Role of Hypertension on the Severity of COVID-19: A Review. J Cardiovasc Pharmacol (2021) 78:e648–55. doi: 10.1097/FJC.0000000000001116
99. Trump S, Lukassen S, Anker MS, Chua RL, Liebig J, Thurmann L, et al. Hypertension Delays Viral Clearance and Exacerbates Airway Hyperinflammation in Patients With COVID-19. Nat Biotechnol (2021) 39:705–16. doi: 10.1038/s41587-020-00796-1
100. Biancardi VC, Bomfim GF, Reis WL, Al-Gassimi S, Nunes KP. The Interplay Between Angiotensin II, TLR4 and Hypertension. Pharmacol Res (2017) 120:88–96. doi: 10.1016/j.phrs.2017.03.017
101. Grylls A, Seidler K, Neil J. Link Between Microbiota and Hypertension: Focus on LPS/TLR4 Pathway in Endothelial Dysfunction and Vascular Inflammation, and Therapeutic Implication of Probiotics. Biomed Pharmacother = Biomed Pharmacotherapie (2021) 137:111334. doi: 10.1016/j.biopha.2021.111334
102. Singh MV, Cicha MZ, Nunez S, Meyerholz DK, Chapleau MW, Abboud FM. Angiotensin II-Induced Hypertension and Cardiac Hypertrophy Are Differentially Mediated by TLR3- and TLR4-Dependent Pathways. Am J Physiol Heart Circulatory Physiol (2019) 316:H1027–38. doi: 10.1152/ajpheart.00697.2018
103. Qi J, Yu XJ, Fu LY, Liu KL, Gao TT, Tu JW, et al. Exercise Training Attenuates Hypertension Through TLR4/MyD88/NF-kappaB Signaling in the Hypothalamic Paraventricular Nucleus. Front Neurosci (2019) 13:1138. doi: 10.3389/fnins.2019.01138
104. Schnohr P, O'Keefe JH, Marott JL, Lange P, Jensen GB. Dose of Jogging and Long-Term Mortality: The Copenhagen City Heart Study. J Am Coll Cardiol (2015) 65:411–9. doi: 10.1016/j.jacc.2014.11.023
105. Fernandez-Gonzalo R, De Paz JA, Rodriguez-Miguelez P, Cuevas MJ, Gonzalez-Gallego J. Effects of Eccentric Exercise on Toll-Like Receptor 4 Signaling Pathway in Peripheral Blood Mononuclear Cells. . J Appl Physiol (1985) (2012) 112:2011–8. doi: 10.1152/japplphysiol.01499.2011
106. McFarlin BK, Flynn MG, Campbell WW, Stewart LK, Timmerman KL. TLR4 Is Lower in Resistance-Trained Older Women and Related to Inflammatory Cytokines. Med Sci sports Exercise (2004) 36:1876–83. doi: 10.1249/01.MSS.0000145465.71269.10
107. Flynn MG, McFarlin BK, Phillips MD, Stewart LK, Timmerman KL. Toll-Like Receptor 4 and CD14 mRNA Expression Are Lower in Resistive Exercise-Trained Elderly Women. J Appl Physiol (1985) (2003) 95:1833–42. doi: 10.1152/japplphysiol.00359.2003
108. Soltani N, Marandi SM, Kazemi M, Esmaeil N. Combined All-Extremity High-Intensity Interval Training Regulates Immunometabolic Responses Through Toll-Like Receptor 4 Adaptors and A20 Downregulation in Obese Young Females. Obes facts (2020) 13:415–31. doi: 10.1159/000509132
109. Fernandez-Gonzalo R, De Paz JA, Rodriguez-Miguelez P, Cuevas MJ, Gonzalez-Gallego J. TLR4-Mediated Blunting of Inflammatory Responses to Eccentric Exercise in Young Women. Mediators Inflammation (2014) 2014:479395. doi: 10.1155/2014/479395
110. Cavalcante PAM, Gregnani MF, Henrique JS, Ornellas FH, Araujo RC. Aerobic But Not Resistance Exercise Can Induce Inflammatory Pathways via Toll-Like 2 and 4: A Systematic Review. Sports Med - Open (2017) 3:42. doi: 10.1186/s40798-017-0111-2
111. Albashir AAD. The Potential Impacts of Obesity on COVID-19. Clin Med (2020) 20:e109–13. doi: 10.7861/clinmed.2020-0239
112. Shafran N, Shafran I, Ben-Zvi H, Sofer S, Sheena L, Krause I, et al. Secondary Bacterial Infection in COVID-19 Patients Is a Stronger Predictor for Death Compared to Influenza Patients. Sci Rep (2021) 11:12703. doi: 10.1038/s41598-021-92220-0
113. de Oliveira M, De Sibio MT, Mathias LS, Rodrigues BM, Sakalem ME, Nogueira CR. Irisin Modulates Genes Associated With Severe Coronavirus Disease (COVID-19) Outcome in Human Subcutaneous Adipocytes Cell Culture. Mol Cell Endocrinol (2020) 515:110917. doi: 10.1016/j.mce.2020.110917
114. Totura AL, Whitmore A, Agnihothram S, Schafer A, Katze MG, Heise MT, et al. Toll-Like Receptor 3 Signaling via TRIF Contributes to a Protective Innate Immune Response to Severe Acute Respiratory Syndrome Coronavirus Infection. mBio (2015) 6:e00638–15. doi: 10.1128/mBio.00638-15
Keywords: COVID-19, irisin, exercise, inflammation, adipomyokine
Citation: Alves HR, Lomba GSB, Gonçalves-de-Albuquerque CF and Burth P (2022) Irisin, Exercise, and COVID-19. Front. Endocrinol. 13:879066. doi: 10.3389/fendo.2022.879066
Received: 18 February 2022; Accepted: 28 April 2022;
Published: 17 June 2022.
Edited by:
Emilio J. Vélez, Institut National de recherche pour l’agriculture, l’alimentation et l’environnement (INRAE), FranceReviewed by:
Dewan Md. Sumsuzzman, Inje University, South KoreaS. Anand Narayanan, Florida State University, United States
Sema Timurkaan, Firat University, Turkey
Copyright © 2022 Alves, Lomba, Gonçalves-de-Albuquerque and Burth. This is an open-access article distributed under the terms of the Creative Commons Attribution License (CC BY). The use, distribution or reproduction in other forums is permitted, provided the original author(s) and the copyright owner(s) are credited and that the original publication in this journal is cited, in accordance with accepted academic practice. No use, distribution or reproduction is permitted which does not comply with these terms.
*Correspondence: Patricia Burth, cGJ1cnRoQGlkLnVmZi5icg==; Cassiano Felippe Gonçalves-de-Albuquerque, Y2Fzc2lhbm8uYWxidXF1ZXJxdWVAdW5pcmlvLmJy
†These authors have contributed equally to this work