- 1Department of Biological Sciences, Graduate School of Science and Technology, Kumamoto University, Kumamoto, Japan
- 2Department of Biochemistry, Asahikawa Medical University, Asahikawa, Japan
- 3International Research Center for Agricultural and Environmental Biology, Graduate School of Science and Technology, Kumamoto University, Kumamoto, Japan
Medaka (Oryzias latipes) is a teleost fish with an XX/XY sex determination system. Sex reversal from female-to-male (masculinization of XX fish) can be induced through cortisol elevation from exposure to environmental stress such as high temperature during sexual differentiation. However, the effects of oxidative stress, generated via metabolic reactions and biological defense mechanisms, on the sexual differentiation of medaka are unclear. Here, we investigated the effect of oxidative stress on medaka sexual differentiation using hydrogen peroxide (H2O2), which induces oxidative stress in vertebrates. H2O2 treatment from 0 to 5 days post-hatching induced masculinization of wild-type XX medaka, but not of gonadal soma-derived growth factor (gsdf) or peroxisome proliferator-activated receptor alpha-a (pparaa) knockout XX fish. Co-treatment with an oxidative stress inhibitor caused masculinization recovery but co-treatment with a cortisol synthesis inhibitor did not. H2O2 treatment significantly upregulated gsdf and pparaa expression in XX medaka. However, H2O2 did not elevate cortisol levels in medaka larvae during sexual differentiation. These results strongly indicate that oxidative stress induces masculinization of XX medaka without causing elevation of cortisol.
Introduction
In the offspring of many vertebrates sex is determined genetically through sex chromosomes inherited from their parents—with parthenogenesis being the exception (1–3). Sex determination systems differ across species. For example, most mammals exhibit a male heterozygous XX/XY system, and birds and some reptiles exhibit a female heterozygous ZZ/ZW system. Sex determination can also be influenced by environmental stresses (e.g., temperature and pH) during the sexual differentiation period in some reptiles (4, 5), amphibians (6, 7), and fish (8, 9). However, the molecular mechanisms underlying environmental sex determination in these species are poorly understood.
Medaka (Oryzias latipes) is commonly used as a model laboratory organism because it has a short generation interval, a small genome size, and is easy to handle and rear. dmy/dmrt1bY, the medaka sex-determining gene located on the Y chromosome, has been identified (10, 11). Medaka also make an excellent vertebrate model organism for molecular biology and genetic experiments because transgenic techniques and gene knockout (KO) systems using transcription activator-like effector nuclease (TALEN), or clustered regularly interspaced short palindromic repeat (CRISPR)/CRISPR-associated protein 9 (Cas9) have been established in this species (12–15).
Exposure to high temperature (HT) causes female-to-male sex reversal (masculinization) of medaka during the sexual differentiation period (16–18). Exposure to HT elevates cortisol levels and increases expression of gonadal soma-derived growth factor (gsdf), a TGF-beta superfamily gene related to testis differentiation in teleosts, and decreases the expression of cyp19a1a, which encodes cytochrome P450 aromatase, an ovary differentiation factor (19). Therefore, it was concluded that HT promotes male development and suppresses female development. Moreover, it was recently reported that peroxisome proliferator-activated receptor alpha-a (pparaa), which regulates the expression of fatty acid-related genes, is activated by HT and cortisol and its activation leads to the masculinization of XX medaka (20). Recently, it was reported that lipid metabolism may regulate female-to-male sex reversal in starved medaka (21). Hence, changes in the lipid metabolism system, induced as a stress response, may also be involved in the masculinization of XX medaka.
Reactive oxygen species (ROS) generated by metabolic reactions (e.g., fatty acid oxidation and the mitochondrial respiratory chain) have a physiological function in intercellular signaling, but excessive ROS cause oxidative damage in various cellular molecules (e.g., DNA, RNA, proteins, and lipids) (22–26). For example, oxidative stress from excessive ROS has been linked to diseases such as alcoholic liver disease (27–29). Zhang et al. (2016) reported that inorganic mercury (Hg) exposure caused oxidative stress and histological damage in the gonads of adult zebrafish, and altered sex hormone levels by disrupting the transcription of genes involved in the hypothalamic-pituitary-gonadal axis. (30). However, the effect of oxidative stress on sexual differentiation was not examined.
In the present study, we investigated the effect of oxidative stress on the sexual differentiation of medaka using hydrogen peroxide (H2O2), which induces oxidative stress in vertebrates (31). We then assessed sex-reversal ratio and fertility in the adults, and investigated cortisol levels and expression patterns of sex-related genes in medaka larvae. Finally, we studied the relationship between masculinization by oxidative stress and GSDF or PPARα function using gsdf and pparaa KO medaka.
Materials and Methods
Ethics Statement
This study was performed using protocols approved by the Animal Care and Use Committee of Kumamoto University (A2020-014). All methods were carried out in accordance with the relevant guidelines and regulations. The study was performed according to the ARRIVE guidelines along with the general guidelines and ethical approval.
Animals
Wild-type, pparaa KO (20), and gsdf KO lines (32) originating from an FLFII medaka line (33) were used in this study. pparaa KO and gsdf KO lines were generated using the CRISPR/Cas9 system and maintained by brother-sister mating of the genetically homogenous medaka (20, 32). pparaa KO and gsdf KO XX medaka do not masculinize with cortisol treatment (20, 32), while some gsdf KO but not pparaa KO XY fish become females having ovaries under normal conditions, while others become normal males having testes (34). Fish embryos and larvae were maintained in embryo-rearing medium (ERM: 17 mM NaCl, 0.4 mM KCl, 0.27 mM CaCl2.2H2O, 0.66 mM MgSO4, pH 7) at 26°C under a 14-h light and 10-h dark cycle.
Experimental Treatment
To confirm the masculinization of XX medaka by oxidative stress, H2O2 treatments were performed with 0.75 and 2 mM H2O2 (purity 30%, CAS RN: 7722-84-1; Wako Pure Chemical, Osaka, Japan) using wild-type larvae from 0 to 5 days post-hatching (dph) in 6-well culture plates (Corning, Glendale, AZ) with the water being changed daily. A rescue test to reduce oxidative stress through antioxidant supplementation was conducted with either 1 or 10 μM N-acetyl-L-cysteine (NAC; Wako) dissolved in Dimethyl sulfoxide (DMSO; Sigma-Aldrich, Saint Louis, MO) as previously described (20), using larvae from 0 to 5 dph in 6-well culture plates with the water being changed daily.
To investigate whether other XX medaka lines undergo masculinization from oxidative stress, pparaa KO, and gsdf KO medaka larvae were treated with 2 mM H2O2 under the conditions outlined above. Finally, treatment with 5 μM metyrapone (Sigma-Aldrich) dissolved in ethanol (Wako) as previously described (17), was used in conjunction with 2 mM H2O2 in FLFII medaka larvae to investigate the relationship between cortisol and oxidative stress-induced masculinization. The survival rates and the body sizes in adults are shown in Supplementary Tables S1, S2, respectively.
Genetic Sexing
The genetic sex of the adults (about 2 months old) was determined by genomic PCR. The PCR was performed using specific primers for dmy/dmrt1bY as previously described (17). PCR conditions were as follows: preheating at 95°C for 10 min, 40 cycles of 94°C for 30 sec, 59°C for 30 sec, 72°C for 1 min, and a final extension at 72°C for 5 min. The genetic sex of the larvae at 5 dph was determined by the appearance of leucophores as previously described (33).
Histological Analysis of Gonads
Histological analysis was performed as previously described (17). Adult fish were prepared for examining the gonadal phenotype and calculating sex ratios. The tissue was fixed in Bouin’s solution at 4°C overnight, embedded in paraffin, sectioned serially at a thickness of 5 μm, stained with hematoxylin and eosin, and then imaged with an Eclipse Ci-E microscope (Nikon, Tokyo, Japan).
Fertility Assessment
The fertility of adult XX male medaka (approximately 2 months old) after H2O2 treatment from 0 to 5 dph was assessed by natural mating with fertile partners (wild-type XX female medaka). A minimum of 80 eggs were analyzed to determine the fertilization rate. Adult XY male medaka (approximately 2 months old) without H2O2 treatment were used as the control group. Fertility assessment was conducted with three mating pairs.
Quantitative Real-Time PCR
Gene expression analysis by quantitative real-time PCR was used to further investigate the mechanism of XX medaka masculinization by oxidative stress. Total RNA was extracted from the gonad regions of wild-type larvae (N = 4, 10 pooled samples) treated with or without 2 mM H2O2 from 0 to 5 dph using ISOGEN (Nippon Gene, Tokyo, Japan) as previously described (19). Briefly, reverse transcription was performed at 37°C for 30 min using a ReverTra Ace® qPCR RT Master Mix (Toyobo, Osaka, Japan). Quantitative real-time PCR was performed with specific primers (Supplementary Table S3) on a LightCycler 480 (Roche, Mannheim, Germany) using SYBR Green I Master Mix (Roche). The PCR conditions were as follows: 95°C for 5 min, then 45 cycles of 95°C for 5 min, 59°C for 10 s, and 72°C for 10 s. Relative gene expression levels were calculated using a delta-CT method. The RefFinder tool, which integrates four specific algorithms [GeNorm (35), NormFinder (36), BestKeeper (37), and the comparative delta-Ct method (38)], was used for the assessment and screening of three candidate reference genes [elongation factor 1 alpha (ef1α), β-actin, and glyceraldehyde-3-phosphate dehydrogenase (gapdh)]. ef1a was the most stably expressed gene and was used as a reference gene (Supplementary Table S4).
Cortisol Measurement
To confirm the stress level in each treatment, a cortisol measurement was performed. Wild-type medaka larvae were treated with or without 2 mM H2O2 from 0 to 5 dph. Metyrapone (5 μM) was added to the 2 mM H2O2 treatment group and cortisol (5 μM) treatment was used as a positive control.
Steroid hormones were extracted as previously described (39). Briefly, five pooled larvae were homogenized in phosphate-buffered saline (137 mM NaCl, 2.68 mM KCl, 8.1 mM Na2HPO4, 1.47 mM KH2PO4; pH 7.4). Steroids were extracted three times from the homogenates into diethyl ether according to previous methods used for teleosts (40–42), and cortisol levels were measured using a cortisol EIA kit (Cayman Chemical, Ann Arbor, MI) according to the manufacturer’s instructions. Individual cortisol levels (per fish) were determined by dividing the average measurement by the number of pooled samples.
Statistical Analysis
Statistical analysis was performed using Statcel 3 with Excel (OMS, Saitama, Japan). Significant differences in sex-reversal ratio among the treatments were determined by the chi-squared test. Student’s t-test was used to detect significant differences in fertility. One-way ANOVA, Tukey-Kramer, and Scheffe’s F tests were used to statistically analyze the gene expression and cortisol measurement data.
Results
The Effect of Oxidative Stress on Sex Differentiation of XX Medaka
To investigate whether oxidative stress causes the masculinization of XX medaka, we treated medaka larvae with H2O2 from 0 to 5 dph, which is the shortest temperature-sensitive period determined by our previous study (17, 19). The sex-reversal ratio (at approximately 2 months of age) for each treatment is shown in Table 1. H2O2 treatment (0.75 mM H2O2; 14.3%, 2 mM H2O2; 35%) caused the masculinization of wild-type XX medaka in a concentration-dependent manner. Histological analysis showed that control XY males had normal testes with productive spermatogenesis Figure 1A) and XX female medaka had normal ovaries with young oocytes (Figure 1B). H2O2-treated, sex-reversed XX individuals had normal testes that included spermatocytes (Figure 1C), while other H2O2-treated XX individuals had normal ovaries, as in control females (Figure 1D). This was also observed after treatment with 2 mM H2O2 and metyrapone, a cortisol synthesis inhibitor (Figure 1E). Treatment with 2 mM H2O2 and NAC, an oxidative stress inhibitor, produced less masculinization (15.4%) than with 2 mM H2O2 alone (Figure 1F). No masculinization was observed in the gsdf or pparaa KO XX medaka treated with 2 mM H2O2 (Figures 1G, H), similar to the untreated gsdf and pparaa KO XX medaka (20, 32). There was a significant difference in sex-reversal ratio between XX controls and XX individuals treated with 2 mM H2O2 or XX individuals treated with 2 mM H2O2 and metyrapone (Table 1).
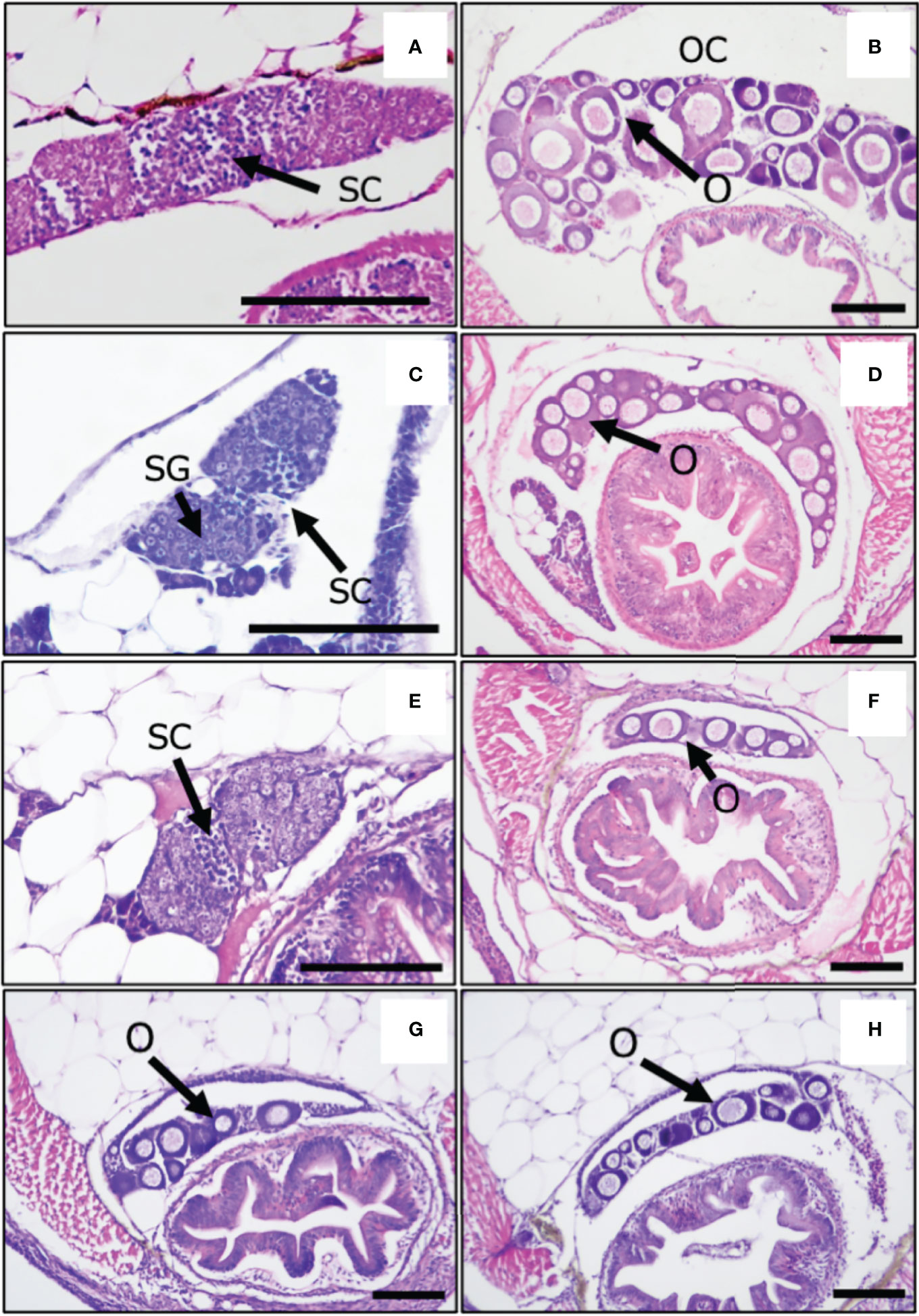
Figure 1 Gonads of adult medaka at 2 months post-hatching. (A) XY male, control, (B) XX female, control, (C) sex-reversal XX male, (D) no sex-reversal XX female with H2O2 treatment, (E) H2O2 + metyrapone, (F) H2O2 + NAC, (G) gsdf KO XX female, (H) pparaa KO XX female with H2O2 treatment. SC, spermatocytes; SG, spermatogonia; O, oocytes; OC, ovarian cavities; Scale bars: 100 μm.
Wild-type XY males and sex-reversal XX males (from H2O2 treatment) had similar fertilization rates after mating with XX females (Figure 2). Every fertilized egg developed into a normal larva.
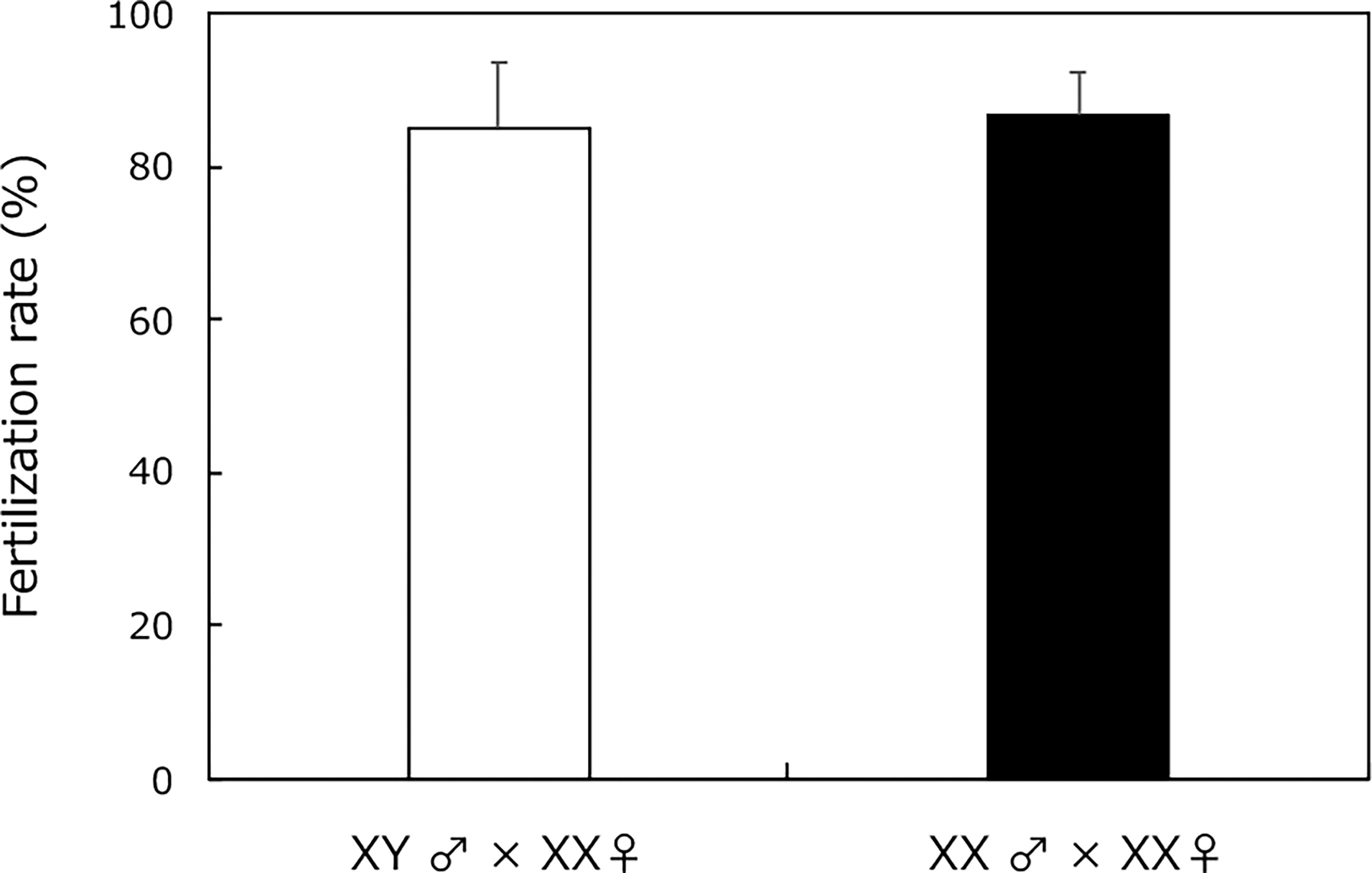
Figure 2 Fertility assessment of adult XY male without H2O2 treatment (white box) and sex-reversal XX male medaka with H2O2 treatment from 0 to 5 dph (black box) using natural mating with fertile partners (XX female medaka). Vertical bar: mean ± standard error of triplicate samples.
Effects of Oxidative Stress on Gene Expression During Sex Differentiation
We analyzed the expression pattern of male-related genes [gsdf and anti-Müllerian hormone (amh)], female-related genes (cyp19a1b), which is expressed in the larval gonads and regulated by cortisol (20), and pparaa in 5-dph larvae exposed to H2O2-induced oxidative stress using quantitative real-time PCR. gsdf expression levels were significantly higher in XY medaka than in XX fish and the expression was increased in both sexes by H2O2 treatment (Figure 3A). amh expression was detected in both sexes to the same extent, similar to the findings in a previous study (43), and the expression in XX fish was significantly increased by H2O2 treatment (Figure 3B). cyp19a1b expression levels were significantly higher in XX medaka than in XY fish and the expression was significantly increased in both sexes by H2O2 treatment (Figure 3C). pparaa expression levels were detected in both sexes to the same extent and the expression in XX fish was significantly increased by H2O2 treatment (Figure 3D).
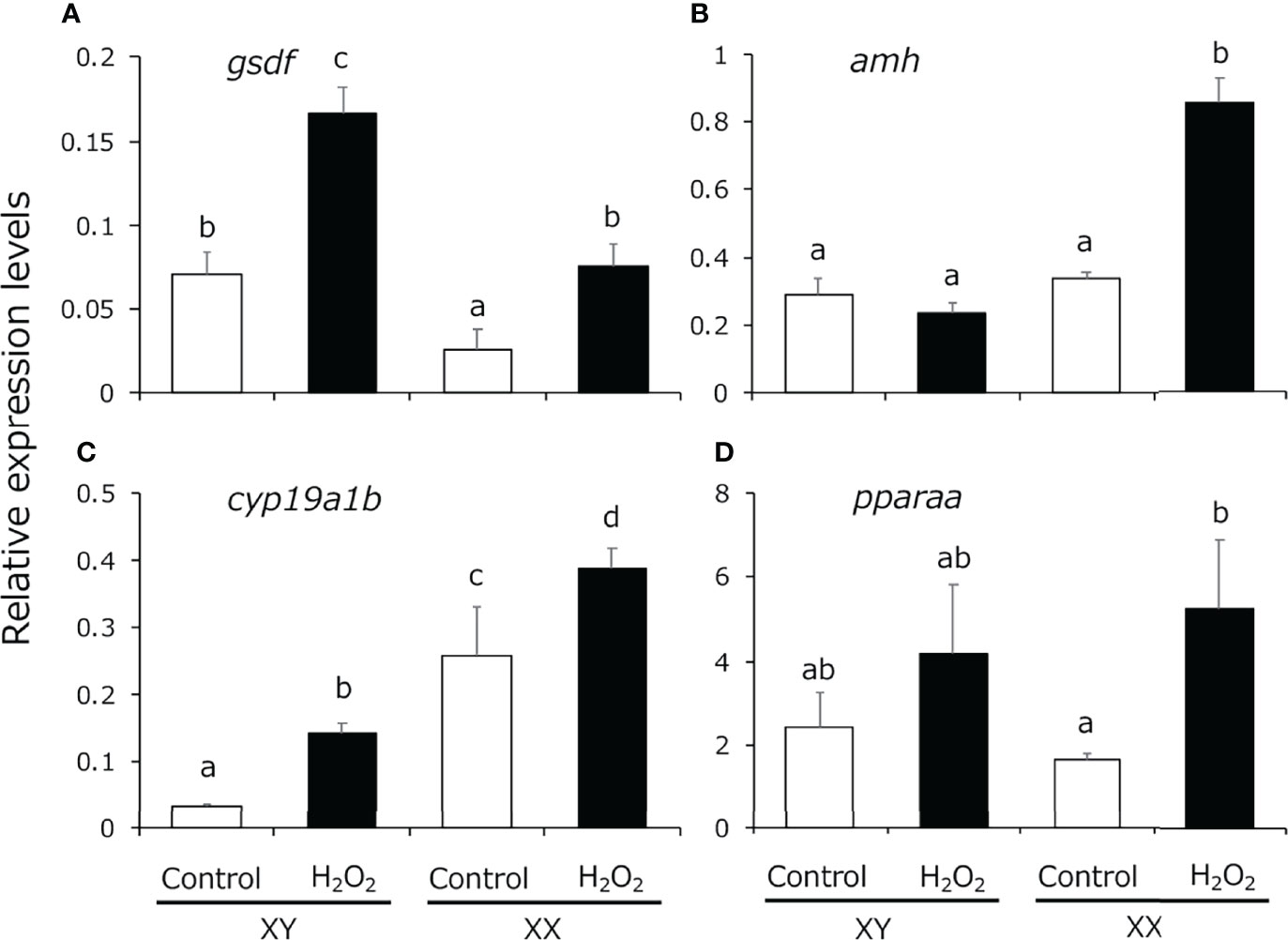
Figure 3 Quantitative real-time PCR analysis of the expression of (A) gsdf, (B) amh, (C) cyp19a1b and (D) pparaa in the gonadal region of controls (white box) and H2O2-treated medaka (black box) at 5 dph. Relative expression levels of the target genes were normalized to that of ef1α. Vertical bar: mean ± standard error of quadruplicate samples; a, b, c, d, and ab: significant difference (p < 0.05).
Change of Cortisol Levels in Medaka Exposed to Oxidative Stress
To investigate if oxidative stress caused cortisol elevation in XX medaka (in a similar way to high temperature treatment), we assessed cortisol levels in wild-type medaka treated with: 0 or 2 mM H2O2, metyrapone, or cortisol, from 0 to 5 dph (Figure 4). The cortisol levels were similar in the H2O2-, and metyrapone-treated groups, in contrast to those in HT-treated medaka (17). Significant differences were detected between the cortisol treated group and the other groups.
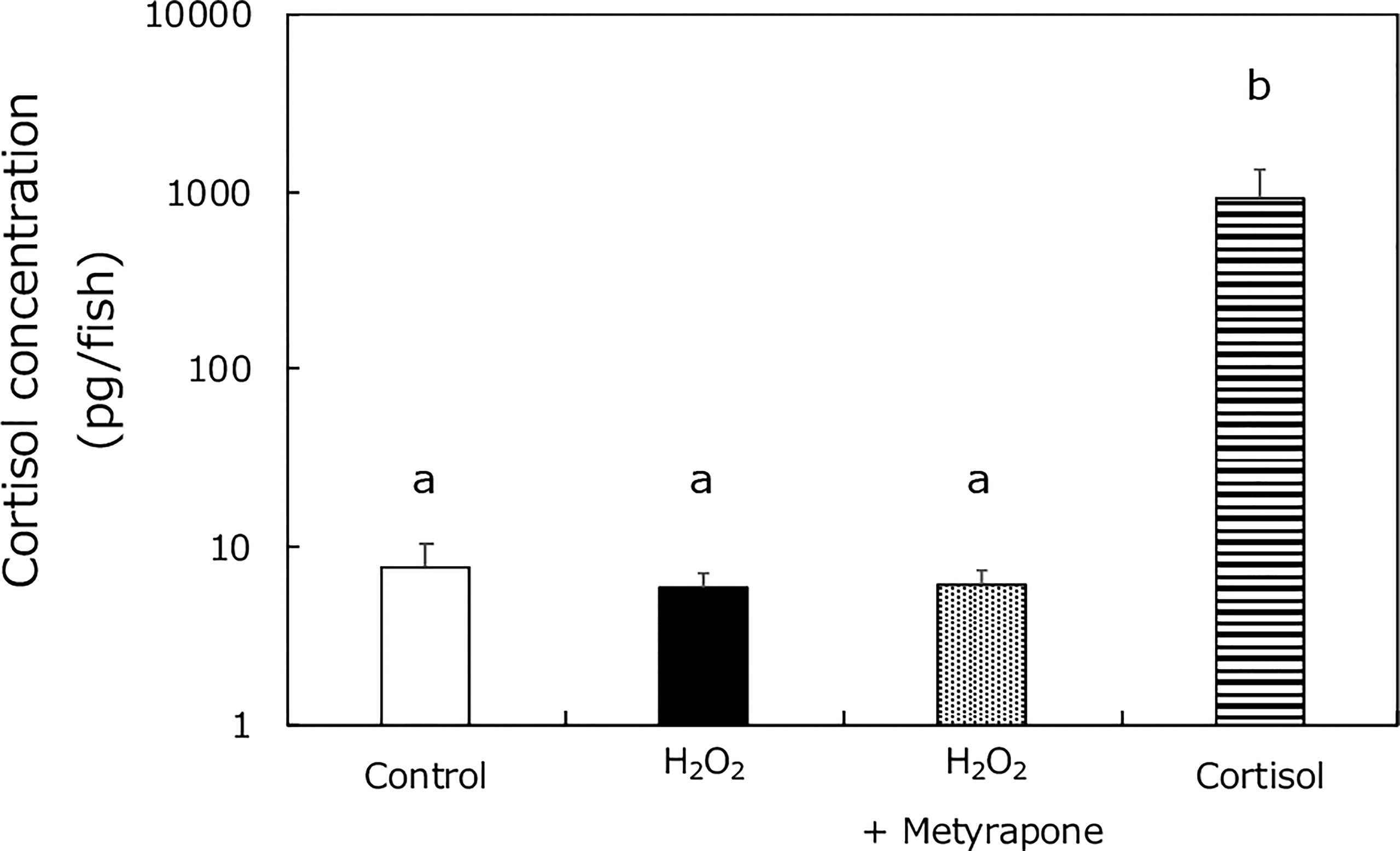
Figure 4 Cortisol levels in medaka larvae at 5 dph. Control (white box), H2O2 (black box), H2O2 with metyrapone (dotted box), and cortisol treatment (stripe box) groups. Vertical bar: mean ± standard error of quadruplicate samples; a and b: significant difference (p < 0.01).
Discussion
To assess the effect of oxidative stress on sex differentiation of medaka, we analyzed histological changes of the gonad, cortisol levels, fertility rate, and sex-related gene expression using adults or larvae treated with H2O2 from 0 to 5 dph. Previous studies have shown that cortisol and HT treatments were more effective during the 5 days after hatching than before hatching (17, 20), although sexually dimorphic proliferation of germ cells has occurred by the hatching stage (44). Therefore, in this study, we treated larvae with H2O2 for 5 days after hatching. Our results indicate that oxidative stress causes masculinization of XX medaka. To our knowledge, this is the first report to show that oxidative stress can cause masculinization of a vertebrate species. Recently, it was reported that in a teleost, Atherinopsidae, whose sex can be determined by temperature, masculinization by continuous illumination was accompanied by significant increases in the expression of the stress axis activation gene, crf, and ROS antagonist effector genes, gsr and cat, indicating that both the stress axis and ROS response mechanisms are activated at this time (45). Therefore, although the masculinizing effect of oxidative stress in other species remains uncertain, there may be a link between continuous light, oxidative stress, and environmental sex determination in vertebrates.
The fertilization rate was similar between matings of H2O2-treated, sex-reversed XX males with XX females, and of control XY males with XX females. Moreover, the fertilized eggs developed into normal individuals. A previous study reported that Hg exposure induced oxidative stress in adult gonads and caused alterations in gonadal histology, sex hormone production, and sex-related gene expression in zebrafish (30). Additionally, atretic oocytes, and a loss of contact between the oocyte cell membrane and the follicular cell layer was observed. While the fertilization rate was not confirmed, the structure of the gonads was adversely affected and it was proposed that fertility would be disrupted (30). In the present study, we found that XX males, induced by oxidative stress from H2O2 treatment, exhibited normal fertility, which indicates that sex reversal by H2O2 treatment resulted in a normal masculinization cascade and had no toxic effects.
Previously, we showed that masculinization of XX medaka was caused by elevated cortisol levels because masculinization by HT was inhibited by treatment with metyrapone, an inhibitor of cortisol synthesis (17). Although metyrapone completely inhibits cortisol synthesis in medaka embryos (17), this drug did not decrease cortisol levels of fish larvae after hatching in this study. Therefore, higher concentrations of metyrapone may be needed to block completely cortisol synthesis in the larvae. However, masculinization of XX medaka by H2O2 treatment was not suppressed by treatment with metyrapone and no increase in cortisol levels was observed in the H2O2-treated group. These results strongly indicate that oxidative stress causes masculinization of XX medaka without elevating cortisol. Moreover, we found that H2O2 treatment dose-dependently induced masculinization of XX medaka but not all fish were masculinized, similar to cortisol-induced masculinization (16–18, 46). Some studies have been reported that cortisol induces oxidative stress in teleosts (47, 48). Taken together, these results strongly suggest that oxidative stress may act downstream of cortisol signaling in the process of stress-induced masculinization, although further investigation is needed.
In our previous studies, pparaa or gsdf KO XX medaka were not masculinized by cortisol treatment, which strongly indicated that pparaa and gsdf are involved in masculinization of XX medaka (20, 32). Consistently, in this study, masculinization of XX medaka by H2O2 treatment was completely suppressed in pparaa KO and gsdf KO fish, suggesting that masculinization of XX medaka by oxidative stress also occurred via PPARα and GSDF function. The gsdf is predominantly expressed in Sertoli cells and granulosa cells in mature medaka gonads (49). Moreover, deletion of gsdf results in phenotypic sex reversal of males to females (34), while gsdf transgenic XX medaka show masculinization (50). PPARα acts as a transcription factor that regulates the expression of genes related to fatty acid oxidation (51, 52). Hara et al. (2020) revealed that agonist-activation of PPARα induces the masculinization of XX medaka whereas treatment of pparaa KO medaka with cortisol or the agonist did not induce masculinization of XX medaka (20). In the present study, the expression levels of gsdf and pparaa were significantly elevated in XX larvae by H2O2 treatment. Surprisingly, the expression of amh, which is detected to the same extent in both sexes (17), and cyp19a1b, which is more highly expressed in XX individuals than in XY individuals (20), were also significantly induced in XX larvae by H2O2 treatment. Since molecular mechanisms and implications that oxidative stress induces the expression of these genes remain unclear at this time, it will be necessary to analyze them using amh and cyp19a1b KO medaka in the future. Thus, oxidative stress appears to cause masculinization of XX medaka by regulating the expression of these sex-related genes during the sex differentiation period.
Although this study shows that oxidative stress induces masculinization of XX medaka, the molecular pathway of stress-mediated masculinization was not fully elucidated. Previously, it has been reported that ROS interact with low-density lipoproteins to activate PPARα and subsequently limit inflammation, as indicated by PPAR-dependent repression of inducible nitric oxide synthase gene transcription (53). Therefore, oxidative stress is likely to induce masculinization of XX medaka through activation of PPARα, which in turn causes induction of the expression of male-related genes. Future studies will focus on the molecular pathway of masculinization mediated by stress.
In summary, H2O2 treatment induced masculinization of wild-type XX medaka but not of gsdf or pparaa KO XX fish. The masculinization could be prevented by co-treatment with the oxidative stress inhibitor, NAC, but not with the cortisol synthesis inhibitor, metyrapone. Moreover, H2O2 treatment significantly upregulated gsdf and pparaa expression in XX medaka. Notably, H2O2 did not elevate cortisol levels in medaka larvae during sexual differentiation. These results strongly indicate that oxidative stress induces masculinization of XX medaka without causing elevation of cortisol.
Data Availability Statement
The original contributions presented in the study are included in the article/Supplementary Material. Further inquiries can be directed to the corresponding author.
Ethics Statement
The animal study was reviewed and approved by the Animal Care and Use Committee of Kumamoto University (A2020-014). Written informed consent was obtained from the owners for the participation of their animals in this study.
Author Contributions
TK obtained funding and designed the study. KM, SH, KS, RN and TY performed the experiments and collected the data. KM, RN and TK wrote the manuscript. All authors contributed to the article and approved the submitted version.
Conflict of Interest
The authors declare that the research was conducted in the absence of any commercial or financial relationships that could be construed as a potential conflict of interest.
Publisher’s Note
All claims expressed in this article are solely those of the authors and do not necessarily represent those of their affiliated organizations, or those of the publisher, the editors and the reviewers. Any product that may be evaluated in this article, or claim that may be made by its manufacturer, is not guaranteed or endorsed by the publisher.
Acknowledgments
We thank Tamara Leahy, PhD, from Edanz (https://jp.edanz.com/ac) for editing a draft of this manuscript. This work was supported in part by JSPS KAKENHI Grant Number 19H03052 (to TK) from the Ministry of Education, Culture, Sports, Science and Technology of Japan.
Supplementary Material
The Supplementary Material for this article can be found online at: https://www.frontiersin.org/articles/10.3389/fendo.2022.878286/full#supplementary-material
References
1. Chapman DD, Shivji MS, Louis E, Sommer J, Fletcher H, Prodohl PA. Virgin Birth in a Hammerhead Shark. Biol Lett (2007) 3:425–7. doi: 10.1098/rsbl.2007.0189
2. Kottler V, Schartl M. The Colorful Sex Chromosomes of Teleost Fish. Genes (2018) 9:233. doi: 10.3390/genes9050233
3. Martinez P, Vinas AM, Sanchez L, Diaz N, Ribas L, Piferrer F. Genetic Architecture of Sex Determination in Fish: Applications to Sex Ratio Control in Aquaculture. Front Genet (2014) 5:340. doi: 10.3389/fgene.2014.00340
4. Bull JJ, Vogt RC. Temperature-Dependent Sex Determination in Turtles. Science (1979) 206:1186–8. doi: 10.1126/science.505003
5. Gutzke WHN, Crews D. Embryonic Temperature Determines Adult Sexuality in a Reptile. Nature (1988) 332:832–4. doi: 10.1038/332832a0
6. Dournon C, Houillon C, Pieau C. Temperature Sex-Reversal in Amphibians and Reptiles. Int J Dev Biol (1990) 34:81–92.
7. Wallace H, Badawy GM, Wallace BM. Amphibian Sex Determination and Sex Reversal. Cell Mol Life Sci (1999) 55:901–9. doi: 10.1007/s000180050343
8. Conover DO, Kynard BE. Environmental Sex Determination: Interaction of Temperature and Genotype in a Fish. Science (1981) 213:577–9. doi: 10.1126/science.213.4507.577
9. Rubin DA. Effect of pH on Sex Ratio in Cichlids and a Poecilliid (Teleostei). Copeia (1985) 1985:233–5. doi: 10.2307/1444818
10. Matsuda M, Nagahama Y, Shinomiya A, Sato T, Matsuda C, Kobayashi T, et al. DMY is a Y-Specific DM-Domain Gene Required for Male Development in the Medaka Fish. Nature (2002) 417:559–63. doi: 10.1038/nature751
11. Nanda I, Kondo M, Hornung U, Asakawa S, Winkler C, Shimizu A, et al. A Duplicated Copy of DMRT1 in the Sex-Determining Region of the Y Chromosome of the Medaka. Oryzias latipes Proc Natl Acad Sci USA (2002) 99:11778–83. doi: 10.1073/pnas.182314699
12. Ozato K, Kondoh H, Inohara H, Iwamatsu T, Wakamatsu Y, Okada TS. Production of Transgenic Fish: Introduction and Expression of Chicken Delta-Crystallin Gene in Medaka Embryos. Cell Differ (1986) 19:237–44. doi: 10.1016/0045-6039(86)90100-4
13. Ansai S, Sakuma T, Yamamoto T, Ariga H, Uemura N, Takahashi R, et al. Efficient Targeted Mutagenesis in Medaka Using Custom-Designed Transcription Activator-Like Effector Nucleases. Genetics (2013) 193:739–49. doi: 10.1534/genetics.112.147645
14. Matsuzaki Y, Sakuma T, Yamamoto T, Saya H. Establishment of Pten Knockout Medaka With Transcription Activator-Like Effector Nucleases (TALENs) as a Model of PTEN Deficiency Disease. PLoS One (2017) 12:e0186878. doi: 10.1371/journal.pone.0186878
15. Sawamura R, Osafune N, Murakami T, Furukawa F, Kitano T. Generation of Biallelic F0 Mutants in Medaka Using the CRISPR/Cas9 System. Genes Cells (2017) 22:756–63. doi: 10.1111/gtc.12511
16. Sato T, Endo T, Yamahira K, Hamaguchi S, Sakaizumi M. Induction of Female-to-Male Sex Reversal by High Temperature Treatment in Medaka. Oryzias latipes Zoolog Sci (2005) 22:985–8. doi: 10.2108/zsj.22.985
17. Hayashi Y, Kobira H, Yamaguchi T, Shiraishi E, Yazawa T, Hirai T, et al. High Temperature Causes Masculinization of Genetically Female Medaka by Elevation of Cortisol. Mol Reprod Dev (2010) 77:679–86. doi: 10.1002/mrd.21203
18. Adolfi MC, Fischer P, Herpin A, Regensburger M, Kikuchi M, Tanaka M, et al. Increase of Cortisol Levels After Temperature Stress Activates Dmrt1a Causing Female-to-Male Sex Reversal and Reduced Germ Cell Number in Medaka. Mol Reprod Dev (2019) 86:1405–17. doi: 10.1002/mrd.23177
19. Kitano T, Hayashi Y, Shiraishi E, Kamei Y. Estrogen Rescues Masculinization of Genetically Female Medaka by Exposure to Cortisol or High Temperature. Mol Reprod Dev (2012) 79:719–26. doi: 10.1002/mrd.22080
20. Hara S, Furukawa F, Mukai K, Yazawa T, Kitano T. Peroxisome Proliferator-Activated Receptor Alpha Is Involved in the Temperature-Induced Sex Differentiation of a Vertebrate. Sci Rep (2020) 10:11672. doi: 10.1038/s41598-020-68594-y
21. Sakae Y, Oikawa A, Sugiura Y, Mita M, Nakamura S, Nishimura T, et al. Starvation Causes Female-to-Male Sex Reversal Through Lipid Metabolism in the Teleost Fish, Medaka (Olyzias latipes). Biol Open (2020) 9:bio050054. doi: 10.1242/bio.050054
22. Fridovich I. The Biology of Oxygen Radicals. Science (1978) 201:875–80. doi: 10.1126/science.210504
23. Johnson KJ, Fantone J, Kaplan J, Ward PA. In Vivo Damage of Rat Lungs by Oxygen Metabolites. J Clin Invest (1981) 67:983–93. doi: 10.1172/jci110149
24. Halliwell B, Gutteridge JM. Oxygen Toxicity, Oxygen Radicals, Transition Metals and Disease. Biochem J (1984) 219:1–14. doi: 10.1042/bj2190001
25. Thannickal VJ, Fanburg BL. Reactive Oxygen Species in Cell Signaling. Am J Physiol Lung Cell Mol Physiol (2000) 279:L1005–28. doi: 10.1152/ajplung.2000.279.6.L1005
26. D'Autréaux B, Toledano MB. ROS as Signalling Molecules: Mechanisms That Generate Specificity in ROS Homeostasis. Nat Rev Mol Cell Biol (2007) 8:813–24. doi: 10.1038/nrm2256
27. Mello T, Polvani S, Galli A. Peroxisome Proliferator-Activated Receptor and Retinoic X Receptor in Alcoholic Liver Disease. PPAR Res (2009) 2009:748174. doi: 10.1155/2009/748174
28. Neuschwander-Tetri BA. Hepatic Lipotoxicity and the Pathogenesis of Nonalcoholic Steatohepatitis: The Central Role of Nontriglyceride Fatty Acid Metabolites. Hepatology (2010) 52:774–88. doi: 10.1002/hep.23719
29. Zhu D-M, Shi J, Liu S, Liu Y, Zheng D. HIV Infection Enhances TRAIL-Induced Cell Death in Macrophage by Down-Regulating Decoy Receptor Expression and Generation of Reactive Oxygen Species. PLoS One (2011) 6:e18291. doi: 10.1371/journal.pone.0018291
30. Zhang QF, Li YW, Liu ZH, Chen QL. Reproductive Toxicity of Inorganic Mercury Exposure in Adult Zebrafish: Histological Damage, Oxidative Stress, and Alterations of Sex Hormone and Gene Expression in the Hypothalamic-Pituitary-Gonadal Axis. Aquat Toxicol (2016) 177:417–24. doi: 10.1016/j.aquatox.2016.06.018
31. Cho SH, Heo SJ, Yang HW, Ko EY, Jung MS, Cha SH, et al. Protective Effect of 3-Bromo-4,5-Dihydroxybenzaldehyde From Polysiphonia Morrowii Harvey Against Hydrogen Peroxide-Induced Oxidative Stress In Vitro and In Vivo. J Microbiol Biotechnol (2019) 29:1193–203. doi: 10.4014/jmb.1904.04062
32. Hara S, Sawamura R, Kitano T. Cortisol Induces Masculinization of XX Medaka Through Gonadal Soma-Derived Growth Factor (GSDF) and Anti-Müllerian Hormone Receptor Type 2 (AMHR2). Fish Sci (2021) 87:85–91. doi: 10.1007/s12562-020-01479-1
33. Wakamatsu Y, Inoue C, Hayashi H, Mishima N, Sakaizumi M, Ozato K. Establishment of New Medaka (Oryzias latipes) Stock Carrying Genotypic Sex Markers. Environ Sci (2003) 10:291–302.
34. Imai T, Saino K, Matsuda M. Mutation of Gonadal Soma-Derived Factor Induces Medaka XY Gonads to Undergo Ovarian Development. Biochem Biophys Res Commun (2015) 467:109–14. doi: 10.1016/j.bbrc.2015.09.112
35. Vandesompele J, De Preter K, Pattyn F, Poppe B, Van Roy N, De Paepe A, et al. Accurate Normalization of Real-Time Quantitative RT-PCR Data by Geometric Averaging of Multiple Internal Control Genes. Genome Biol (2002) 3:research0034.1. doi: 10.1186/gb-2002-3-7-research0034
36. Andersen CL, Jensen JL, Orntoft TF. Normalization of Real-Time Quantitative Reverse Transcription-PCR Data: A Model-Based Variance Estimation Approach to Identify Genes Suited for Normalization, Applied to Bladder and Colon Cancer Data Sets. Cancer Res (2004) 64:5245–50. doi: 10.1158/0008-5472.CAN-04-0496
37. Pfaffl MW, Tichopad A, Prgomet C, Neuvians TP. Determination of Stable Housekeeping Genes, Differentially Regulated Target Genes and Sample Integrity: Bestkeeper–Excel-Based Tool Using Pair-Wise Correlations. Biotechnol Lett (2004) 26:509–15. doi: 10.1023/b:bile.0000019559.84305.47
38. Silver N, Best S, Jiang J, Thein SL. Selection of Housekeeping Genes for Gene Expression Studies in Human Reticulocytes Using Real-Time PCR. BMC Mol Biol (2006) 7:33. doi: 10.1186/1471-2199-7-33
39. Kitano T, Takamune K, Kobayashi T, Nagahama Y, Abe SI. Suppression of P450 Aromatase Gene Expression in Sex-Reversed Males Produced by Rearing Genetically Female Larvae at a High Water Temperature During a Period of Sex Differentiation in the Japanese Flounder (Paralichthys olivaceus). J Mol Endocrinol (1999) 23:167–76. doi: 10.1677/jme.0.0230167
40. de Jesus EG, Hirano T. Changes in Whole Body Concentrations of Cortisol, Thyroid Hormones, and Sex Steroids During Early Development of the Chum Salmon. Oncorhynchus keta Gen Comp Endocrinol (1992) 85:55–61. doi: 10.1016/0016-6480(92)90171-f
41. Feist G, Schreck CB. Ontogeny of the Stress Response in Chinook Salmon. Oncorhynchus tshawytscha Fish Physiol Biochem (2001) 25:31–40. doi: 10.1023/a:1019709323520
42. Ramsay JM, Feist GW, Varga ZM, Westerfield M, Kent ML, Schreck CB. Whole-Body Cortisol is an Indicator of Crowding Stress in Adult Zebrafish. Danio rerio Aquacult (2006) 258:565–74. doi: 10.1016/j.aquaculture.2006.04.020
43. Kluver N, Pfennig F, Pala I, Storch K, Schlieder M, Froschauer A, et al. Differential Expression of Anti-Mullerian Hormone (Amh) and Anti-Mullerian Hormone Receptor Type II (amhrII) in the Teleost Medaka. Dev Dyn (2007) 236:271–81. doi: 10.1002/dvdy.20997
44. Hamaguchi S. A Light- and Electron-Microscopic Study on the Migration of Primordial Germ Cells in the Teleost, Oryzias latipes. Cell Tissue Res (1982) 227:139–51. doi: 10.1007/BF00206337
45. Corona-Herrera GA, Arranz SE, Martinez-Palacios CA, Navarrete-Ramirez P, Toledo-Cuevas EM, Valdez-Alarcon JJ, et al. Experimental Evidence of Masculinization by Continuous Illumination in a Temperature Sex Determination Teleost (Atherinopsidae) Model: Is Oxidative Stress Involved? J Fish Biol (2018) 93:229–37. doi: 10.1111/jfb.13651
46. Yamaguchi T, Yoshinaga N, Yazawa T, Gen K, Kitano T. Cortisol is Involved in Temperature-Dependent Sex Determination in the Japanese Flounder. Endocrinology (2010) 151:3900–8. doi: 10.1210/en.2010-0228
47. Nakano T, Kameda M, Shoji Y, Hayashi S, Yamaguchi T, Sato M. Effect of Severe Environmental Thermal Stress on Redox State in Salmon. Redox Biol (2014) 2:772–6. doi: 10.1016/j.redox.2014.05.007
48. Espinoza MB, Aedo JE, Zuloaga R, Valenzuela C, Molina A, Valdés JA. Cortisol Induces Reactive Oxygen Species Through a Membrane Glucocorticoid Receptor in Rainbow Trout Myotubes. J Cell Biochem (2017) 118:718–25. doi: 10.1002/jcb.25676
49. Shibata Y, Paul-Prasanth B, Suzuki A, Usami T, Nakamoto M, Matsuda M, et al. Expression of Gonadal Soma Derived Factor (GSDF) is Spatially and Temporally Correlated With Early Testicular Differentiation in Medaka. Gene Expr Patterns (2010) 10:283–9. doi: 10.1016/j.gep.2010.06.005
50. Zhang X, Guan G, Li M, Zhu F, Liu Q, Naruse K, et al. Autosomal Gsdf Acts as a Male Sex Initiator in the Fish Medaka. Sci Rep (2016) 6:19738. doi: 10.1038/srep19738
51. Braissant O, Foufelle F, Scotto C, Dauca M, Wahli W. Differential Expression of Peroxisome Proliferator-Activated Receptors (PPARs): Tissue Distribution of Ppar-Alpha, -Beta, and -Gamma in the Adult Rat. Endocrinology (1996) 137:354–66. doi: 10.1210/endo.137.1.8536636
52. Devchand PR, Ziouzenkova O, Plutzky J. Oxidative Stress and Peroxisome Proliferator-Activated Receptors: Reversing the Curse? Circ Res (2004) 95:1137–9. doi: 10.1161/01.RES.0000151331.69399.b2
Keywords: oxidative stress, cortisol, masculinization, environmental sex determination, medaka Oryzias latipes
Citation: Mukai K, Hara S, Sakima K, Nozu R, Yazawa T and Kitano T (2022) Oxidative Stress Causes Masculinization of Genetically Female Medaka Without Elevating Cortisol. Front. Endocrinol. 13:878286. doi: 10.3389/fendo.2022.878286
Received: 17 February 2022; Accepted: 13 May 2022;
Published: 16 June 2022.
Edited by:
Honoo Satake, Suntory Foundation for Life Sciences, JapanReviewed by:
Kataaki Okubo, The University of Tokyo, JapanToshiya Yamaguchi, National Research Institute of Aquaculture, Fisheries Research and Education Agency, Japan
Copyright © 2022 Mukai, Hara, Sakima, Nozu, Yazawa and Kitano. This is an open-access article distributed under the terms of the Creative Commons Attribution License (CC BY). The use, distribution or reproduction in other forums is permitted, provided the original author(s) and the copyright owner(s) are credited and that the original publication in this journal is cited, in accordance with accepted academic practice. No use, distribution or reproduction is permitted which does not comply with these terms.
*Correspondence: Takeshi Kitano, dGtpdGFub0BrdW1hbW90by11LmFjLmpw