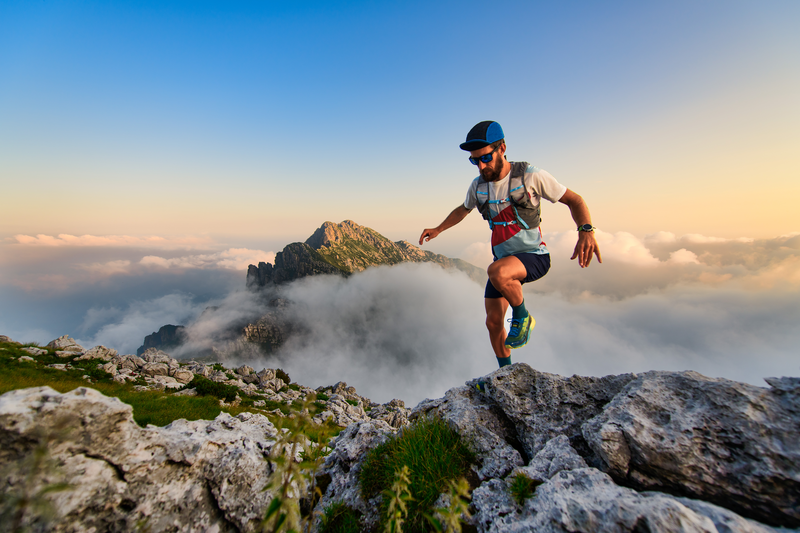
95% of researchers rate our articles as excellent or good
Learn more about the work of our research integrity team to safeguard the quality of each article we publish.
Find out more
REVIEW article
Front. Endocrinol. , 10 June 2022
Sec. Bone Research
Volume 13 - 2022 | https://doi.org/10.3389/fendo.2022.876269
Postmenopausal osteoporosis (PMOP) is characterized by the uncoupling of bone resorption and bone formation induced by estrogen deficiency, which is a complex outcome related to estrogen and the immune system. The interaction between bone and immune cells is regarded as the context of PMOP. Macrophages act differently on bone cells, depending on their polarization profile and secreted paracrine factors, which may have implications for the development of PMOP. PMOP, rheumatoid arthritis (RA), and Alzheimer’s disease (AD) might have pathophysiological links, and the similarity of their pathological mechanisms is partially visible in altered macrophages and cytokines in the immune system. This review focuses on exploring the pathological mechanisms of PMOP, RA, and AD through the roles of altered macrophages and cytokines secretion. First, the multiple effects on cytokines secretion by bone-bone marrow (BM) macrophages in the pathological mechanism of PMOP are reviewed. Then, based on the thought of “different tissue-same cell type-common pathological molecules-disease pathological links-drug targets” and the methodologies of “molecular network” in bioinformatics, highlight that multiple cytokines overlap in the pathological molecules associated with PMOP vs. RA and PMOP vs. AD, and propose that these overlaps may lead to a pathological synergy in PMOP, RA, and AD. It provides a novel strategy for understanding the pathogenesis of PMOP and potential drug targets for the treatment of PMOP.
Postmenopausal osteoporosis (PMOP) is a systemic chronic bone metabolic disease caused by the uncoupling of bone resorption and bone formation with estrogen deficiency (1, 2). Estrogen is also involved in the control of immune function, leading to a chronic low-grade pro-inflammatory phenotype under estrogen deficiency with altered cytokine expression and immune cell profiles in PMOP (3–5). Bone and immune system are functionally linked by complex molecular networks, in which accumulating evidence suggests that macrophages either directly or indirectly through the secretion of various cytokines, coordinate the coupling between osteoblasts and osteoclasts (6, 7). The relationship among estrogen, macrophages, and the skeleton could help in understanding the complex mechanism of PMOP.
The complex crosstalk between bone and immune cells plays an indispensable role in the pathogenesis of PMOP. Immunomodulatory imbalances and functional alterations are also part of the pathological conditions of PMOP, rheumatoid arthritis (RA), and Alzheimer’s disease (AD). Immunological studies have demonstrated that different tissue-resident cells of the macrophage lineage, such as bone-bone marrow (BM) macrophages, synovial macrophages, and microglia, are responsible for pathological changes in PMOP, RA, and AD, respectively. The existence of pathological links in PMOP, RA, and AD may be explained by searching for the common molecular network mediated by bone-BM macrophages, synovial macrophages, and microglia to provide a novel strategy for potential drug targets for the treatment of PMOP.
Macrophages, which are immune cells with heterogeneous phenotypes and complex functions, can be divided into circulating and resident macrophages (8, 9). Primitive hematopoiesis is a source of macrophages in embryos, and the majority of resident macrophages originate from yolk sac erythro-myeloid progenitors (10, 11). Bone-BM macrophages, synovial macrophages, and microglia play a key role in maintaining tissue homeostasis; phagocytosis and removal of cellular debris and foreign substances; tissue repair, regeneration, and remodeling; and the development and resolution of inflammation (12–15). Under physiological conditions, the bone-BM contains multiple different resident macrophage populations, including osteal macrophages, hematopoietic stem cell niche macrophages, and erythroblast island macrophages (16, 17). Macrophages have remarkable plasticity that allows them to respond efficiently to environmental signals and change their phenotypes.
Macrophages are activated, polarized, and subsequently secreted various cytokines (Supplementary Table S1) that are involved in coupling of bone resorption and bone formation under exposure to various types of stimuli. Under exposure to lipopolysaccharide (LPS) or T-helper 1 cytokines, such as interferon-gamma or granulocyte macrophage-colony stimulating factor, alone or in combination, macrophages are activated towards an M1 functional program to produce toxic effector molecules (such as inflammatory cytokines, reactive oxygen, and nitrogen species), which participate in polarized T-helper 1 responses, regulate oxidative stress, and evoke inflammatory responses (18–21). Conversely, T-helper 2 cytokines, such as interleukin (IL)-4 or IL-13, can induce macrophages to polarize into the M2 type including M2a, M2b, M2c, and M2d, and play a central role in polarized T-helper 2 responses, the dampening of inflammation, angiogenesis, immunoregulation, and the remodeling of tissues (11, 22–25). The diversity of the phenotypes and functions of macrophages makes them play important different roles in inflammatory, immune, and metabolic diseases, such as PMOP, RA, and AD.
Macrophages play a pivotal role in the coupling of bone resorption and bone formation. Paracrine cytokines, such as transforming growth factor-β (TGF-β), bone morphogenetic protein (BMP)-2, BMP-4, BMP-6, and osteopontin, are secreted by activated macrophages, which have a direct and critical impact on the physiological and pathological regulation of bone (Supplementary Table S2). On the one hand, fusion between cells of the monocyte/macrophage lineage leads to the formation of osteoclasts, which are the only cells with the ability to dissolve bone tissue (26). On the other hand, ablation of macrophages leads to loss of endosteal osteoblasts, reduction in the number of bone marrow mesenchymal stem cells (BMSCs), decrease in the ability of BMSCs to differentiate into osteoblasts, and attenuation of parathyroid hormone-induced trabecular bone anabolism (27–30). The macrophage-osteoclast axis plays an essential role in osteoimmunity, regulating the coupling of bone resorption and bone formation (31).
Although macrophages in bone-BM are not directly adjacent to osteoblasts, they can alter the BM microenvironment by mediating an inflammatory response to affect bone synthesis and catabolism. Osteocytes are regulated by macrophages that secrete inflammatory mediators to control the coupling of bone resorption and bone formation (Supplementary Table S3). Macrophages are vital modulators of inflammation that rapidly change their phenotypes and functions in response to local microenvironmental signals and also play various roles in both the induction and resolution of inflammation, such as clearing dead cells and debris, presenting antigens, and recruiting, and activating other immune cells (7, 32–34). Skeletal homeostasis depends on the balance between the classically active M1 type and the alternatively active M2 type (35, 36). M2 activation appears to be blunted in macrophages from postmenopausal women, leading to an increased M1/M2 response ratio (32). In ovariectomized (OVX) mice, polarization of M1 macrophages was increased whereas polarization of M2 macrophages was disturbed (37). Therefore, changes in macrophage-derived cytokines and their phenotypes linked with inflammation are critical regulators of bone resorption and bone formation, supporting the theory that the immune system significantly contributes to the pathological mechanism of inflammation-mediated bone-loss.
After menopause, due to the influence of estrogen deficiency, the level of oxidative stress in the body increases, which causes the imbalance in bone reconstruction and leads to osteoporosis (38–40). Macrophages secrete regulatory factors related to oxidative stress, such as reactive oxygen species (ROS), nitric oxide (NO), and inducible nitric oxide synthase, which induce pathological changes in the differentiation process and activity of bone cells, ultimately leading to the uncoupling of bone resorption and bone formation (Supplementary Table S4). The oxidative stress level in PMOP depends on the relationship between ROS and the endogenous antioxidant defense system (41, 42). One of the most damaging effects of ROS is lipid peroxidation, whose end product, malondialdehyde, is a potential biomarker of oxidative stress (43). NO, catalyzed by nitric oxide synthase, is also an integral part of the response to oxygen deprivation and has been confirmed to be a key regulator of bone homeostasis (44–46). The effects of these factors highlight the diversity of the roles of macrophages in regulating bone homeostasis. Further studies are needed to clarify the molecular mechanisms underlying the relationship among macrophages, oxidative stress and PMOP.
Bone is a highly vascularized tissue, and bone homeostasis depends on the coupling between bone and blood vessels. The skeletal microvasculature system plays an important role in the metabolism of BM microenvironment, osteogenesis, and maintenance of the balance between bone formation and bone resorption. Basic and clinical studies have found that the decrease in local blood supply is related to PMOP. In the OVX mouse model, the number of microvessels, the type H vessels, and the expression of vascular endothelial growth factor (VEGF) are all significantly reduced (47, 48). Macrophages are key cellular components in the BM microenvironment that regulate bone homeostasis and angiogenesis. In bone repair, macrophages can remove dead neutrophils at the injured site after fracture, and release cytokines, such as VEGF, erythropoietin, platelet-derived growth factor-BB, matrix metallopeptidase 2 (MMP2), MMP9, and fibroblast growth factor 2 (FGF2) (Supplementary Table S5), so as to initiate the repair cascade that suppresses the pro-inflammatory responses and promotes angiogenic responses (49, 50). During the inflammatory phase of bone repair, the recruitment of macrophages is related to angiogenesis, and their numbers are strongly correlated with the density of blood vessels (51). Moreover, the coordinated conversion of the pro-inflammatory M1 and anti-inflammatory M2 phenotypes in macrophages determines the efficiency of bone regeneration to a great extent (52). Given their intimate involvement in vascular formation, an understanding of the multilayered contributions of macrophages to bone repair and fracture healing is also accumulating.
Because the physiological and immune functions are reduced in postmenopausal women, in addition to the need to prevent osteoporosis, the prevalence of RA and AD is also quite noteworthy. A large number of clinical and basic studies have confirmed the association in PMOP, RA, and AD. With the help of bioinformatics analysis methods (Supplementary Methods of Bioinformatics), we integrated multiple databases to screen the differential genes of PMOP, and then performed enrichment analysis of the Kyoto Encyclopedia of Genes and Genomes (KEGG) pathway, which was also enriched in the RA and AD pathways (Figure 1 and Table 1). Simultaneously, the two signaling pathways of neuroactive ligand-receptor interaction and cytokine-cytokine receptor interaction also undergo significant changes. Like PMOP, the pathological mechanisms of RA and AD are also closely associated with two resident macrophages: synovial macrophages and brain microglia, respectively. Therefore, we pose the question of what role do macrophages play in the “two-pairs of disease links”. Searching for significant common-targets in PMOP, RA, and AD may have a particularly practical meaning in providing guidance for the prevention and control of PMOP, RA, and AD.
Figure 1 Top 20 Kyoto Encyclopedia of Genes and Genomes (KEGG) pathway enrichment candidate targets of differential genes in postmenopausal osteoporosis. Pathways with significant changes (false discovery rate [FDR] < 0.05) were identified. The vertical coordinates represent the KEGG pathway with significant enrichment, and the horizontal coordinates represent the gene ratio, which refers to the ratio of enriched genes to all target genes. The color of the bubble graph indicates the significance of the enriched KEGG pathway, the color gradient represents the size of the P-value, and the size of each dot represents the number of genes.
The immune cells involved in RA, macrophages, are the most numerous immune cells found in the RA synovium and play a key role in immune/inflammatory reactions and bone loss by paracrine signaling or via direct cell-cell contact (53–56). In addition, synovial macrophages are involved in pathological processes such as matrix degradation, oxidative stress, and angiogenesis in RA (57–59).
Among other features, RA is characterized by systemic bone loss, and the risk of osteoporosis is high in patients with RA, especially in postmenopausal women (60–62). Therefore, to clarify the pathological links between PMOP and RA, we used bioinformatics analysis methods (Supplementary Methods of Bioinformatics) to search for common pathological molecules between them.
The results are summarized as follows: among biological processes, it is enriched in leukocyte migration, cell chemotaxis, leukocyte chemotaxis, myeloid, mononuclear cell migration, granulocyte chemotaxis, monocyte chemotaxis, and other processes. (Figure 2A). Among molecular functions, it is enriched in cytokine receptor binding, cytokine activity, growth factor binding, growth factor activity, immune receptor activity, growth factor receptor binding, cytokine receptor activity, cytokine binding, TGF-β receptor binding, insulin-like growth factor (IGF) binding, IGF-1 binding, and other functions (Figure 2B). The results show that the immune system and immune cells play important regulatory roles in the occurrence of PMOP and RA. Although the results did not directly enrich macrophage-related functions in the top 20 Gene Ontology functions, the related functions enriched in cells (such as TGF-β and IGF-1) still had great directivity. Cytokines secreted by macrophages, including TGF-β1, IL-1β, IL-2, IL-4, IL-6, IL-10, tumor necrosis factor (TNF), IGF-1, VEGFA, FGF2 and MMP2, which are associated with the functions of regulation of immune system process, bone remodeling, regulation of inflammatory response, response to oxidative stress, and angiogenesis, were screened from the protein–protein interaction (PPI) core network, as described above (Figure 2C).
Figure 2 Core cytokine networks of pathological crosstalk in postmenopausal osteoporosis (PMOP) vs. rheumatoid arthritis (RA) and PMOP vs. Alzheimer’s disease (AD). Gene ontology functional enrichment analysis of common differential genes in PMOP vs. RA and PMOP vs. AD was performed, including biological processes (A, D) and molecular functions (B, E). Protein–protein interaction (PPI) network topology analysis was performed for common differential genes in PMOP vs. RA and PMOP vs. AD, and biological process enrichment analysis of core network genes was completed (C, F).
As in PMOP, an imbalanced network of cytokines secreted by synovial macrophages plays a key role in the pathogenesis of RA. Among them, secretion of TNF-α, IL-1β, IL-2, and IL-6, or a combined deficiency of IL-4 and IL-10, promotes and sustains inflammation, while also acting to promote bone erosion (63, 64). In addition, TGF-β, TNF-α, IGF-1, VEGF, FGF2, and MMP2 are involved in the hypervascularization as well as the pannus formation observed in RA (65–67). As a result, the imbalanced cytokine network could provide clues to identify pathological links between the two diseases and potentially suggest some shared pharmacological prevention and treatment.
Microglia may play a significant role in the pathogenesis of AD, which is characterized by deposition of β-amyloid plaques, hyperphosphorylation of tau protein, oxidative damage, neuroinflammation, vascular remodeling, autophagy, and mitochondrial dysfunction (68–71). AD and PMOP are frequently seen to coincide in clinical practice, and their possible relationship, concurrent occurrence, and linking mechanism have recently been highlighted (72–74). Prevention of osteoporosis should be considered as part of the treatment of patients with AD, especially in postmenopausal women, and conversely, prevention of AD should be considered in patients with various degrees of bone loss.
Microglia show altered morphology and reduced arborization, and their activation increases with the progression of AD (75, 76). Activated microglia exhibit many morphologic and immunophenotypic features of peripheral macrophages, such as pro-inflammatory M1 and immunosuppressive M2 phenotypes (77, 78). Activated microglia assume diverse phenotypes, which mediate the different pathological processes of AD by releasing various substances, such as inflammatory cytokines, growth factors, chemokines, neurotrophins, and superoxide (79–82).
We also used bioinformatics analysis methods (Supplementary Methods of Bioinformatics) to search for common pathological molecules between PMOP and AD. Among biological processes, it’s enriched in the regulation of inflammatory response, response to LPS, ROS metabolic process, and other processes (Figure 2D). Among molecular function, it is enriched in the cytokine receptor binding, cytokine activity, growth factor activity, growth factor binding and receptor binding, and other functions (Figure 2E). Cytokines secreted by macrophages, including TGF-β1, IL-1β, IL-2, IL-4, IL-6, IL-10, IL-18, TNF, IGF-1, C-X-C motif chemokine ligand 8 (CXCL8), VEGFA, FGF2, MMP2, and MMP9, which are also associated with the functions of regulation of immune system process, bone remodeling, regulation of inflammatory response, response to oxidative stress, and angiogenesis, were screened from the PPI core network, as described above (Figure 2F). The results indicated that the pathological changes in bone-BM macrophage-mediated PMOP are partially similar to the pathological changes in microglia-mediated AD.
The delicate balance between their pro-inflammatory and anti-inflammatory actions and their neurotoxic and neuroprotective actions determines the role of microglia in AD. Microglia activate and drive inflammatory processes by inducing the pro-inflammatory molecules, such as IL-1β, IL-6, IL-18, and TNF-α, leading to accumulation of extracellular amyloid-β peptides, tau hyperphosphorylation, and activation of other inflammatory participants (83, 84). They also produce various anti-inflammatory, chemokines and growth factors, such as IL-2, IL-4, IL-10, CXCL8, FGF2, IGF-1, and TGF-β1, which have been shown to exert neuroprotective effects against amyloid-β-induced neurodegeneration (85–88). Other microglia-derived factors such as VEGFA, MMP2, and MMP9, are associated with disruption of the blood-brain barrier, leading to neuroinflammation and progression of AD (89–91). Combined with the role of macrophages in PMOP mentioned above, simultaneous tracing of the common pathological molecular network associated with cytokines (bone-BM macrophages and microglia) in PMOP and AD may reveal the key pathological links between the two diseases.
Because of the common pathological molecules of PMOP, RA, and AD, it is of great importance to seek effective drugs to prevent the occurrence of complications. Chinese herbs have anti-PMOP, anti-RA and anti-AD properties due to their actions against multiple targets, pathways, and systems. Therefore, taking the cytokines secreted by macrophages as the entry point, combined with the results of bioinformatics analysis, we summarized potential active components extracted from Chinese herbs, such as icariin, querzcetin, and naringin, which were simultaneously applied in the treatment of PMOP, RA, and AD (Tables 2 and 3). From the side, this also reflects the roles of altered macrophages and cytokines on PMOP, RA, and AD.
PMOP is caused by dysregulation of the homeostatic connection between bone and the immune system, leading to bone loss. This review has outlined the direct and indirect effects of cytokines secreted by bone-BM macrophages on the coupling of bone resorption and bone formation. The principal mechanisms of these effects include inflammatory/immune responses, angiogenesis, and oxidative stress. Some overlapping cytokines of PMOP, RA, and AD in bioinformatics analysis may immunologically link two diseases, serving as either shared susceptibility factors or molecular links. Therefore, based on the thought of “different tissue (bone-BM, synovial, and brain)-same cell type (macrophages)-common pathological molecules (cytokines)-disease pathological links (PMOP vs. RA and PMOP vs. AD)-drug targets (active compounds extracted from Chinese herbs)” and the methodologies of “molecular network” in bioinformatics, may lead to a paradigm shift in the understanding of the pathogenesis, prophylaxis, and treatment of PMOP.
XHL and YX identified the focus and overall direction of the review. Funding acquisition XHL and HY; Sources, XHL; Methodology, XZ, JZ, YH, HZ, DX, XL, WC, XW, SW, and XHL; Supervision, XHL; Writing—original draft, YX, HY, and XHL; Writing—review & editing, YX, HY, XZ, JZ, YH, HZ, DX, XL, WC, XW, SW, and XHL. All authors contributed to the article and approved the submitted version.
This work was funded by the National Natural Science Foundation of China (No. 82074461), the Chen Keji Development Fund of Integrative Medicine (No. 2020004) and the Research Start-up Fund of Fujian University of Traditional Chinese Medicine (No. X2020009-Talent).
The authors declare that the research was conducted in the absence of any commercial or financial relationships that could be construed as a potential conflict of interest.
All claims expressed in this article are solely those of the authors and do not necessarily represent those of their affiliated organizations, or those of the publisher, the editors and the reviewers. Any product that may be evaluated in this article, or claim that may be made by its manufacturer, is not guaranteed or endorsed by the publisher.
We would like to thank Dr. Ziqing Li and three reviewers for their helpful suggestions, which have help us to improve the manuscript.
The Supplementary Material for this article can be found online at: https://www.frontiersin.org/articles/10.3389/fendo.2022.876269/full#supplementary-material
1. Møller A, Delaissé JM, Olesen JB, Madsen JS, Canto LM, Bechmann T, et al. Aging and Menopause Reprogram Osteoclast Precursors for Aggressive Bone Resorption. Bone Res (2020) 8:27–37. doi: 10.1038/s41413-020-0102-7
2. Pacifici R. Estrogen, Cytokines, and Pathogenesis of Postmenopausal Osteoporosis. J Bone Miner Res (1996) 11(8):1043–51. doi: 10.1002/jbmr.5650110802
3. Khosla S, Oursler MJ, Monroe DG. “Estrogen and the Skeleton”. Trends Endocrinol Metab (2012) 23(11):576–81. doi: 10.1016/j.tem.2012.03.008
4. Ralston SH. Analysis of Gene Expression in Human Bone Biopsies by Polymerase Chain Reaction: Evidence for Enhanced Cytokine Expression in Postmenopausal Osteoporosis. J Bone Miner Res (1994) 9(6):883–90. doi: 10.1002/jbmr.5650090614
5. D’Amelio P. The Immune System and Postmenopausal Osteoporosis. Immunol Invest (2013) 42(7):544–54. doi: 10.3109/08820139.2013.822764
6. Yang DH, Yang MY. The Role of Macrophage in the Pathogenesis of Osteoporosis. Int J Mol Sci (2019) 20(9):2093–107. doi: 10.3390/ijms20092093
7. Muñoz J, Akhavan NS, Mullins AP, Arjmandi BH. Macrophage Polarization and Osteoporosis: A Review. Nutrients (2020) 12(10):2999–3104. doi: 10.3390/nu12102999
8. Guilliams M, Scott CL. Does Niche Competition Determine the Origin of Tissue-Resident Macrophages? Nat Rev Immunol (2017) 17(7):451–60. doi: 10.1038/nri.2017.42
9. Okabe Y, Medzhitov R. Tissue Biology Perspective on Macrophages. Nat Immunol (2016) 17(1):9–17. doi: 10.1038/ni.3320
10. Perdiguero EG, Geissmann F. The Development and Maintenance of Resident Macrophages. Nat Immunol (2016) 17(1):2–8. doi: 10.1038/ni.3341
11. Franken L, Schiwon M, Kurts C. Macrophages: Sentinels and Regulators of the Immune System. Cell Microbiol (2016) 18(4):475–87. doi: 10.1111/cmi.12580
12. Casanova-Acebes M, Dalla E, Leader AM, LeBerichel J, Nikolic J, Morales BM, et al. Tissue-Resident Macrophages Provide a Pro-Tumorigenic Niche to Early NSCLC Cells. Nature (2021) 595(7868):578–84. doi: 10.1038/s41586-021-03651-8
13. Italiani P, Boraschi D. New Insights Into Tissue Macrophages: From Their Origin to the Development of Memory. Immune Netw (2015) 15(4):167–76. doi: 10.4110/in.2015.15.4.167
14. Wynn TA, Vannella KM. Macrophages in Tissue Repair, Regeneration, and Fibrosis. Immunity (2016) 44(3):450–62. doi: 10.1016/j.immuni.2016.02.015
15. Dey A, Allen J, Hankey-Giblin PA. Ontogeny and Polarization of Macrophages in Inflammation: Blood Monocytes Versus Tissue Macrophages. Front Immunol (2014) 5:683. doi: 10.3389/fimmu.2014.00683
16. Chen K, Jiao Y, Liu L, Huang M, He C, He W, et al. Communications Between Bone Marrow Macrophages and Bone Cells in Bone Remodeling. Front Cell Dev Biol (2020) 8:598263. doi: 10.3389/fcell.2020.598263
17. Kaur S, Raggatt LJ, Batoon L, Hume DA, Levesque JP, Pettit AR. Role of Bone Marrow Macrophages in Controlling Homeostasis and Repair in Bone and Bone Marrow Niches. Semin Cell Dev Biol (2017) 61:12–21. doi: 10.1016/j.semcdb.2016.08.009
18. Martinez FO, Gordon S. The M1 and M2 Paradigm of Macrophage Activation: Time for Reassessment. F1000Prime Rep (2014) 6:13–25. doi: 10.12703/P6-13
19. Muraille E, Leo O, Moser M. TH1/TH2 Paradigm Extended: Macrophage Polarization as an Unappreciated Pathogen-Driven Escape Mechanism? Front Immunol (2014) 5:603. doi: 10.3389/fimmu.2014.00603
20. Fresta CG, Fidilio A, Lazzarino G, Musso N, Grasso M, Merlo S, et al. Modulation of Pro-Oxidant and Pro-Inflammatory Activities of M1 Macrophages by the Natural Dipeptide Carnosine. Int J Mol Sci (2020) 21(3):776–97. doi: 10.3390/ijms21030776
21. Karkossa I, Raps S, von Bergen M, Schubert K. Systematic Review of Multi-Omics Approaches to Investigate Toxicological Effects in Macrophages. Int J Mol Sci (2020) 21(24). doi: 10.3390/ijms21249371
22. Shapouri-Moghaddam A, Mohammadian S, Vazini H, Taghadosi M, Esmaeili SA, Mardani F, et al. Macrophage Plasticity, Polarization, and Function in Health and Disease. J Cell Physiol (2018) 233(9):6425–40. doi: 10.1002/jcp.26429
23. Sica A, Mantovani A. Macrophage Plasticity and Polarization: In Vivo Veritas. J Clin Invest (2012) 122(3):787–95. doi: 10.1172/JCI59643
24. Jetten N, Verbruggen S, Gijbels MJ, Post MJ, De Winther MP, Donners MM. Anti-Inflammatory M2, But Not Pro-Inflammatory M1 Macrophages Promote Angiogenesis In Vivo. Angiogenesis (2014) 17(1):109–18. doi: 10.1007/s10456-013-9381-6
25. Ramirez-GarciaLuna JL, Rangel-Berridi K, Olasubulumi OO, Rosenzweig DH, Henderson JE, Gawri R, et al. Enhanced Bone Remodeling After Fracture Priming. Calcif Tissue Int (2021) 110(3):349–66. doi: 10.1007/s00223-021-00921-5
26. Pereira M, Petretto E, Gordon S, Bassett J, Williams GR, Behmoaras J. Common Signalling Pathways in Macrophage and Osteoclast Multinucleation. J Cell Sci (2018) 131(11):jcs216267. doi: 10.1242/jcs.216267
27. Winkler IG, Sims NA, Pettit AR, Barbier V, Nowlan B, Helwani F, et al. Bone Marrow Macrophages Maintain Hematopoietic Stem Cell (HSC) Niches and Their Depletion Mobilizes HSCs. Blood (2010) 116(23):4815–28. doi: 10.1182/blood-2009-11-253534
28. Jacobsen RN, Forristal CE, Raggatt LJ, Nowlan B, Barbier V, Kaur S, et al. Mobilization With Granulocyte Colony-Stimulating Factor Blocks Medullar Erythropoiesis by Depleting F4/80(+)VCAM1(+)CD169(+)ER-HR3(+)Ly6G(+) Erythroid Island Macrophages in the Mouse. Exp Hematol (2014) 42(7):547–61.e4. doi: 10.1016/j.exphem.2014.03.009
29. Vi L, Baht GS, Whetstone H, Ng A, Wei Q, Poon R, et al. Macrophages Promote Osteoblastic Differentiation in-Vivo: Implications in Fracture Repair and Bone Homeostasis. J Bone Miner Res (2015) 30(6):1090–102. doi: 10.1002/jbmr.2422
30. Cho SW, Soki FN, Koh AJ, Eber MR, Entezami P, Park SI, et al. Osteal Macrophages Support Physiologic Skeletal Remodeling and Anabolic Actions of Parathyroid Hormone in Bone. Proc Natl Acad Sci USA (2014) 111(4):1545–50. doi: 10.1073/pnas.1315153111
31. Yao Y, Cai X, Ren F, Ye Y, Wang F, Zheng C, et al. The Macrophage-Osteoclast Axis in Osteoimmunity and Osteo-Related Diseases. Front Immunol (2021) 12664871:664871. doi: 10.3389/fimmu.2021.664871
32. Fischer V, Haffner-Luntzer M. Interaction Between Bone and Immune Cells: Implications for Postmenopausal Osteoporosis. Semin Cell Dev Biol (2021)123:14–21. doi: 10.1016/j.semcdb.2021.05.014
33. Tysoe O. Osteal Macrophages Implicated in Osteoporosis. Nat Rev Endocrinol (2021) 17(10):577. doi: 10.1038/s41574-021-00548-4
34. Schlundt C, Fischer H, Bucher CH, Rendenbach C, Duda GN, Schmidt-Bleek K. The Multifaceted Roles of Macrophages in Bone Regeneration: A Story of Polarization, Activation and Time. Acta Biomater (2021) 133:46–57. doi: 10.1016/j.actbio.2021.04.052
35. Sun Y, Li J, Xie X, Gu F, Sui Z, Zhang K, et al. Macrophage-Osteoclast Associations: Origin, Polarization, and Subgroups. Front Immunol (2021) 12:778078. doi: 10.3389/fimmu.2021.778078
36. Gong L, Zhao Y, Zhang Y, Ruan Z. The Macrophage Polarization Regulates MSC Osteoblast Differentiation In Vitro. Ann Clin Lab Sci (2016) 46(1):65–71.
37. Toniolo A, Fadini GP, Tedesco S, Cappellari R, Vegeto E, Maggi A, et al. Alternative Activation of Human Macrophages Is Rescued by Estrogen Treatment In Vitro and Impaired by Menopausal Status. J Clin Endocrinol Metab (2015) 100(1):E50–8. doi: 10.1210/jc.2014-2751
38. Manolagas SC. From Estrogen-Centric to Aging and Oxidative Stress: A Revised Perspective of the Pathogenesis of Osteoporosis. Endocr Rev (2010) 31(3):266–300. doi: 10.1210/er.2009-0024
39. Banfi G, Iorio EL, Corsi MM. Oxidative Stress, Free Radicals and Bone Remodeling. Clin Chem Lab Med (2008) 46(11):1550–5. doi: 10.1515/CCLM.2008.302
40. Mohamad NV, Ima-Nirwana S, Chin KY. Are Oxidative Stress and Inflammation Mediators of Bone Loss Due to Estrogen Deficiency? A Review of Current Evidence. Endocr Metab Immune Disord Drug Targets (2020) 20(9):1478–87. doi: 10.2174/1871530320666200604160614
41. Wauquier F, Leotoing L, Coxam V, Guicheux J, Wittrant Y. Oxidative Stress in Bone Remodelling and Disease. Trends Mol Med (2009) 15(10):468–77. doi: 10.1016/j.molmed.2009.08.004
42. Kimball JS, Johnson JP, Carlson DA. Oxidative Stress and Osteoporosis. J Bone Joint Surg Am (2021) 103(15):1451–61. doi: 10.2106/JBJS.20.00989
43. Barrera G, Pizzimenti S, Daga M, Dianzani C, Arcaro A, Cetrangolo GP, et al. Lipid Peroxidation-Derived Aldehydes, 4-Hydroxynonenal and Malondialdehyde in Aging-Related Disorders. Antioxid (Basel) (2018) 7(8). doi: 10.3390/antiox7080102
44. Blokhina O, Fagerstedt KV. Oxidative Metabolism, ROS and NO Under Oxygen Deprivation. Plant Physiol Biochem (2010) 48(5):359–73. doi: 10.1016/j.plaphy.2010.01.007
45. Joshua J, Kalyanaraman H, Marathe N, Pilz RB. Nitric Oxide as a Mediator of Estrogen Effects in Osteocytes. Vitam Horm (2014) 96:247–63. doi: 10.1016/B978-0-12-800254-4.00010-6
46. Wimalawansa SJ. Nitric Oxide and Bone. Ann NY Acad Sci (2010) 1192:391–403. doi: 10.1111/j.1749-6632.2009.05230.x
47. Ding WG, Wei ZX, Liu JB. Reduced Local Blood Supply to the Tibial Metaphysis Is Associated With Ovariectomy-Induced Osteoporosis in Mice. Connect Tissue Res (2011) 52(1):25–9. doi: 10.3109/03008201003783011
48. Wang L, Ma R, Guo Y, Sun J, Liu H, Zhu R, et al. Antioxidant Effect of Fructus Ligustri Lucidi Aqueous Extract in Ovariectomized Rats Is Mediated Through Nox4-ROS-NF-κb Pathway. Front Pharmacol (2017) 8:266. doi: 10.3389/fphar.2017.00266
49. Claes L, Recknagel S, Ignatius A. Fracture Healing Under Healthy and Inflammatory Conditions. Nat Rev Rheumatol (2012) 8(3):133–43. doi: 10.1038/nrrheum.2012.1
50. Hu K, Olsen BR. Vascular Endothelial Growth Factor Control Mechanisms in Skeletal Growth and Repair. Dev Dyn (2017) 246(4):227–34. doi: 10.1002/dvdy.24463
51. Hu K, Olsen BR. Osteoblast-Derived VEGF Regulates Osteoblast Differentiation and Bone Formation During Bone Repair. J Clin Invest (2016) 126(2):509–26. doi: 10.1172/JCI82585
52. Zheng Z, Chen Y, Hong H, Shen Y, Wang Y, Sun J, et al. The “Yin and Yang” of Immunomodulatory Magnesium-Enriched Graphene Oxide Nanoscrolls Decorated Biomimetic Scaffolds in Promoting Bone Regeneration. Adv Healthc Mater (2021) 10(2):e2000631. doi: 10.1002/adhm.202000631
53. Ardura JA, Rackov G, Izquierdo E, Alonso V, Gortazar AR, Escribese MM. Targeting Macrophages: Friends or Foes in Disease? Front Pharmacol (2019) 10:1255. doi: 10.3389/fphar.2019.01255
54. Chen Z, Bozec A, Ramming A, Schett G. Anti-Inflammatory and Immune-Regulatory Cytokines in Rheumatoid Arthritis. Nat Rev Rheumatol (2019) 15(1):9–17. doi: 10.1038/s41584-018-0109-2
55. Tardito S, Martinelli G, Soldano S, Paolino S, Pacini G, Patane M, et al. Macrophage M1/M2 Polarization and Rheumatoid Arthritis: A Systematic Review. Autoimmun Rev (2019) 18(11):102397. doi: 10.1016/j.autrev.2019.102397
56. Kinne RW, Bräuer R, Stuhlmüller B, Palombo-Kinne E, Burmester GR. Macrophages in Rheumatoid Arthritis. Arthritis Res (2000) 2(3):189–202. doi: 10.1186/ar86
57. Elshabrawy HA, Chen Z, Volin MV, Ravella S, Virupannavar S, Shahrara S. The Pathogenic Role of Angiogenesis in Rheumatoid Arthritis. Angiogenesis (2015) 18(4):433–48. doi: 10.1007/s10456-015-9477-2
58. Yang Z, Shen Y, Oishi H, Matteson EL, Tian L, Goronzy JJ, et al. Restoring Oxidant Signaling Suppresses Proarthritogenic T Cell Effector Functions in Rheumatoid Arthritis. Sci Transl Med (2016) 8(331):331ra38. doi: 10.1126/scitranslmed.aad7151
59. Szekanecz Z, Koch AE. Macrophages and Their Products in Rheumatoid Arthritis. Curr Opin Rheumatol (2007) 19(3):289–95. doi: 10.1097/BOR.0b013e32805e87ae
60. Sapir-Koren R, Livshits G. Postmenopausal Osteoporosis in Rheumatoid Arthritis: The Estrogen Deficiency-Immune Mechanisms Link. Bone (2017) 103:102–15. doi: 10.1016/j.bone.2017.06.020
61. Haugeberg G, Uhlig T, Falch JA, Halse JI, Kvien TK. Bone Mineral Density and Frequency of Osteoporosis in Female Patients With Rheumatoid Arthritis: Results From 394 Patients in the Oslo County Rheumatoid Arthritis Register. Arthritis Rheum (2000) 43(3):522–30. doi: 10.1002/1529-0131(200003)43:3<522::AID-ANR7>3.0.CO;2-Y
62. Mirzaei A, Jahed SA, Amini KA, Zabihiyeganeh M. Risk of Infection in Postmenopausal Women With Rheumatoid Arthritis and Osteoporosis Taking Denosumab and bDMARDS. Med J Islam Repub Iran (2021) 35(12):12–5. doi: 10.47176/mjiri.35.12
63. Boutet MA, Courties G, Nerviani A, Le Goff B, Apparailly F, Pitzalis C, et al. Novel Insights Into Macrophage Diversity in Rheumatoid Arthritis Synovium. Autoimmun Rev (2021) 20(3):102758. doi: 10.1016/j.autrev.2021.102758
64. Ambarus CA, Noordenbos T, de Hair MJ, Tak PP, Baeten DL. Intimal Lining Layer Macrophages But Not Synovial Sublining Macrophages Display an IL-10 Polarized-Like Phenotype in Chronic Synovitis. Arthritis Res Ther (2012) 14(2):R74. doi: 10.1186/ar3796
65. Maruotti N, Annese T, Cantatore FP, Ribatti D. Macrophages and Angiogenesis in Rheumatic Diseases. Vasc Cell (2013) 5(1):11. doi: 10.1186/2045-824X-5-11
66. Zhou M, Qin S, Chu Y, Wang F, Chen L, Lu Y. Immunolocalization of MMP-2 and MMP-9 in Human Rheumatoid Synovium. Int J Clin Exp Pathol (2014) 7(6):3048–56.
67. Suzuki S, Morimoto S, Fujishiro M, Kawasaki M, Hayakawa K, Miyashita T, et al. Inhibition of the Insulin-Like Growth Factor System is a Potential Therapy for Rheumatoid Arthritis. Autoimmunity (2015) 48(4):251–8. doi: 10.3109/08916934.2014.976631
68. Henstridge CM, Hyman BT, Spires-Jones TL. Beyond the Neuron-Cellular Interactions Early in Alzheimer Disease Pathogenesis. Nat Rev Neurosci (2019) 20(2):94–108. doi: 10.1038/s41583-018-0113-1
69. Bhatia V, Sharma S. Role of Mitochondrial Dysfunction, Oxidative Stress and Autophagy in Progression of Alzheimer’s Disease. J Neurol Sci (2021) 421:117253–75. doi: 10.1016/j.jns.2020.117253
70. Galasko D, Montine TJ. Biomarkers of Oxidative Damage and Inflammation in Alzheimer’s Disease. Biomark Med (2010) 4(1):27–36. doi: 10.2217/bmm.09.89
71. Thurgur H, Pinteaux E. Microglia in the Neurovascular Unit: Blood-Brain Barrier-Microglia Interactions After Central Nervous System Disorders. Neuroscience (2019) 405:55–67. doi: 10.1016/j.neuroscience.2018.06.046
72. Ebrahimpur M, Sharifi F, Shadman Z, Payab M, Mehraban S, Shafiee G, et al. Osteoporosis and Cognitive Impairment Interwoven Warning Signs: Community-Based Study on Older Adults-Bushehr Elderly Health (BEH) Program. Arch Osteoporos (2020) 15(1):140. doi: 10.1007/s11657-020-00817-1
73. Amouzougan A, Lafaie L, Marotte H, Dẻnariẻ D, Collet P, Pallot-Prades B, et al. High Prevalence of Dementia in Women With Osteoporosis. Joint Bone Spine (2017) 84(5):611–4. doi: 10.1016/j.jbspin.2016.08.002
74. Liu D, Zhou H, Tao Y, Tan J, Chen L, Huang H, et al. Alzheimer’s Disease is Associated With Increased Risk of Osteoporosis: The Chongqing Aging Study. Curr Alzheimer Res (2016) 13(10):1165–72. doi: 10.2174/15672050113109990149
75. Davies DS, Ma J, Jegathees T, Goldsbury C. Microglia Show Altered Morphology and Reduced Arborization in Human Brain During Aging and Alzheimer’s Disease. Brain Pathol (2017) 27(6):795–808. doi: 10.1111/bpa.12456
76. Xiang Z, Haroutunian V, Ho L, Purohit D, Pasinetti GM. Microglia Activation in the Brain as Inflammatory Biomarker of Alzheimer’s Disease Neuropathology and Clinical Dementia. Dis Markers (2006) 22(1-2):95–102. doi: 10.1155/2006/276239
77. Tang Y, Le W. Differential Roles of M1 and M2 Microglia in Neurodegenerative Diseases. Mol Neurobiol (2016) 53(2):1181–94. doi: 10.1007/s12035-014-9070-5
78. Sarlus H, Heneka MT. Microglia in Alzheimer’s Disease. J Clin Invest (2017) 127(9):3240–9. doi: 10.1172/JCI90606
79. Schwab C, McGeer PL. Inflammatory Aspects of Alzheimer Disease and Other Neurodegenerative Disorders. J Alzheimers Dis (2008) 13(4):359–69. doi: 10.3233/jad-2008-13402
80. Luo XG, Chen SD. The Changing Phenotype of Microglia From Homeostasis to Disease. Transl Neurodegener (2012) 1(1):9. doi: 10.1186/2047-9158-1-9
81. Varnum MM, Ikezu T. The Classification of Microglial Activation Phenotypes on Neurodegeneration and Regeneration in Alzheimer’s Disease Brain. Arch Immunol Ther Exp (Warsz) (2012) 60(4):251–66. doi: 10.1007/s00005-012-0181-2
82. Wilkinson BL, Landreth GE. The Microglial NADPH Oxidase Complex as a Source of Oxidative Stress in Alzheimer’s Disease. J Neuroinflamm (2006) 3:30–41. doi: 10.1186/1742-2094-3-30
83. Wang WY, Tan MS, Yu JT, Tan L. Role of Pro-Inflammatory Cytokines Released From Microglia in Alzheimer’s Disease. Ann Transl Med (2015) 3(10):136. doi: 10.3978/j.issn.2305-5839.2015.03.49
84. Kaur D, Sharma V, Deshmukh R. Activation of Microglia and Astrocytes: A Roadway to Neuroinflammation and Alzheimer’s Disease. Inflammopharmacology (2019) 27(4):663–77. doi: 10.1007/s10787-019-00580-x
85. Alves S, Churlaud G, Audrain M, Michaelsen-Preusse K, Fol R, Souchet B, et al. Interleukin-2 Improves Amyloid Pathology, Synaptic Failure and Memory in Alzheimer’s Disease Mice. Brain (2017) 140(3):826–42. doi: 10.1093/brain/aww330
86. Ashutosh KW, Cotter R, Borgmann K, Wu L, Persidsky R, et al. CXCL8 Protects Human Neurons From Amyloid-β-Induced Neurotoxicity: Relevance to Alzheimer’s Disease. Biochem Biophys Res Commun (2011) 412(4):565–71. doi: 10.1016/j.bbrc.2011.07.127
87. Gasparini L, Xu H. Potential Roles of Insulin and IGF-1 in Alzheimer’s Disease. Trends Neurosci (2003) 26(8):404–6. doi: 10.1016/S0166-2236(03)00163-2
88. Caruso G, Fresta CG, Musso N, Giambirtone M, Grasso M, Spampinato SF, et al. Carnosine Prevents Aβ-Induced Oxidative Stress and Inflammation in Microglial Cells: A Key Role of TGF-β1. Cells (2019) 8(1):64–86. doi: 10.3390/cells8010064
89. Ogunmokun G, Dewanjee S, Chakraborty P, Valupadas C, Chaudhary A, Kolli V, et al. The Potential Role of Cytokines and Growth Factors in the Pathogenesis of Alzheimer’s Disease. Cells (2021) 10(10):2790–816. doi: 10.3390/cells10102790
90. Durmanova V, Javor J, Parnicka Z, Minarik G, Ocenasova A, Vaseckova B, et al. Impact of MMP2 Rs243865 and MMP3 Rs3025058 Polymorphisms on Clinical Findings in Alzheimer’s Disease Patients. Mediat Inflamm (2021) 2021:5573642. doi: 10.1155/2021/5573642
91. Wang XX, Tan MS, Yu JT, Tan L. Matrix Metalloproteinases and Their Multiple Roles in Alzheimer’s Disease. BioMed Res Int (2014) 2014:908636–43. doi: 10.1155/2014/908636
92. Hsieh TP, Sheu SY, Sun JS, Chen MH. Icariin Inhibits Osteoclast Differentiation and Bone Resorption by Suppression of MAPKs/NF-κb Regulated HIF-1α and PGE(2) Synthesis. Phytomedicine (2011) 18(2-3):176–85. doi: 10.1016/j.phymed.2010.04.003
93. Zhang D, Zhang J, Fong C, Yao X, Yang M. Herba Epimedii Flavonoids Suppress Osteoclastic Differentiation and Bone Resorption by Inducing G2/M Arrest and Apoptosis. Biochimie (2012) 94(12):2514–22. doi: 10.1016/j.biochi.2012.06.033
94. Ma HP, Ma XN, Ge BF, Zhen P, Zhou J, Gao YH, et al. Icariin Attenuates Hypoxia-Induced Oxidative Stress and Apoptosis in Osteoblasts and Preserves Their Osteogenic Differentiation Potential In Vitro. Cell Prolif (2014) 47(6):527–39. doi: 10.1111/cpr.12147
95. Wu ZM, Luo J, Shi XD, Zhang SX, Zhu XB, Guo J. Icariin Alleviates Rheumatoid Arthritis via Regulating miR-223-3p/NLRP3 Signalling Axis. Autoimmunity (2020) 53(8):450–8. doi: 10.1080/08916934.2020.1836488
96. Liu T, Zhao M, Zhang Y, Qiu Z, Zhang Y, Zhao C, et al. Pharmacokinetic-Pharmacodynamic Modeling Analysis and Anti-Inflammatory Effect of Wangbi Capsule in the Treatment of Adjuvant-Induced Arthritis. BioMed Chromatogr (2021) 35(7):e5101. doi: 10.1002/bmc.5101
97. Chi L, Gao W, Shu X, Lu X. A Natural Flavonoid Glucoside, Icariin, Regulates Th17 and Alleviates Rheumatoid Arthritis in a Murine Model. Mediat Inflamm (2014) 2014:392062. doi: 10.1155/2014/392062
98. Luo H, Zhang R. Icariin Enhances Cell Survival in Lipopolysaccharide-Induced Synoviocytes by Suppressing Ferroptosis via the Xc-/GPX4 Axis. Exp Ther Med (2021) 21(1):72. doi: 10.3892/etm.2020.9504
99. Choi EM. Modulatory Effects of Luteolin on Osteoblastic Function and Inflammatory Mediators in Osteoblastic MC3T3-E1 Cells. Cell Biol Int (2007) 31(9):870–7. doi: 10.1016/j.cellbi.2007.01.038
100. Sun YW, Bao Y, Yu H, Chen QJ, Lu F, Zhai S, et al. Anti-Rheumatoid Arthritis Effects of Flavonoids From Daphne Genkwa. Int Immunopharmacol (2020) 83:106384. doi: 10.1016/j.intimp.2020.106384
101. Abd EA, Fathy MM, Ali ZY, El-Garawany A, Mohamed EK. Enhanced Therapeutic Benefit of Quercetin-Loaded Phytosome Nanoparticles in Ovariectomized Rats. Chem Biol Interact (2017) 271:30–8. doi: 10.1016/j.cbi.2017.04.026
102. Niu YB, Yang YY, Xiao X, Sun Y, Zhou YM, Zhang YH, et al. Quercetin Prevents Bone Loss in Hindlimb Suspension Mice via Stanniocalcin 1-Mediated Inhibition of Osteoclastogenesis. Acta Pharmacol Sin (2020) 41(11):1476–86. doi: 10.1038/s41401-020-00509-z
103. Shen P, Lin W, Ba X, Huang Y, Chen Z, Han L, et al. Quercetin-Mediated SIRT1 Activation Attenuates Collagen-Induced Mice Arthritis. J Ethnopharmacol (2021) 279:114213. doi: 10.1016/j.jep.2021.114213
104. Shangguan WJ, Zhang YH, Li ZC, Tang LM, Shao J, Li H. Naringin Inhibits Vascular Endothelial Cell Apoptosis via Endoplasmic Reticulum Stress− and Mitochondrial−Mediated Pathways and Promotes Intraosseous Angiogenesis in Ovariectomized Rats. Int J Mol Med (2017) 40(6):1741–9. doi: 10.3892/ijmm.2017.3160
105. Cao X, Lin W, Liang C, Zhang D, Yang F, Zhang Y, et al. Naringin Rescued the TNF-α-Induced Inhibition of Osteogenesis of Bone Marrow-Derived Mesenchymal Stem Cells by Depressing the Activation of NF-Кb Signaling Pathway. Immunol Res (2015) 62(3):357–67. doi: 10.1007/s12026-015-8665-x
106. Lee JH, Kim GH. Evaluation of Antioxidant and Inhibitory Activities for Different Subclasses Flavonoids on Enzymes for Rheumatoid Arthritis. J Food Sci (2010) 75(7):H212–7. doi: 10.1111/j.1750-3841.2010.01755.x
107. Aihaiti Y, Song CY, Tuerhong X, Ni YY, Ma Y, Shi ZH, et al. Therapeutic Effects of Naringin in Rheumatoid Arthritis: Network Pharmacology and Experimental Validation. Front Pharmacol (2021) 12:672054. doi: 10.3389/fphar.2021.672054
108. Zhai YK, Guo XY, Ge BF, Zhen P, Ma XN, Zhou J, et al. Icariin Stimulates the Osteogenic Differentiation of Rat Bone Marrow Stromal Cells via Activating the PI3K-AKT-eNOS-NO-cGMP-PKG. Bone (2014) 66:189–98. doi: 10.1016/j.bone.2014.06.016
109. Hsieh TP, Sheu SY, Sun JS, Chen MH, Liu MH. Icariin Isolated From Epimedium Pubescens Regulates Osteoblasts Anabolism Through BMP-2, SMAD4, and Cbfa1 Expression. Phytomedicine (2010) 17(6):414–23. doi: 10.1016/j.phymed.2009.08.007
110. Guo J, Li F, Wu Q, Gong Q, Lu Y, Shi J. Protective Effects of Icariin on Brain Dysfunction Induced by Lipopolysaccharide in Rats. Phytomedicine (2010) 17(12):950–5. doi: 10.1016/j.phymed.2010.03.007
111. Sze SC, Tong Y, Ng TB, Cheng CL, Cheung HP. Herba Epimedii: Anti-Oxidative Properties and Its Medical Implications. Molecules (2010) 15(11):7861–70. doi: 10.3390/molecules15117861
112. Luo Y, Nie J, Gong QH, Lu YF, Wu Q, Shi JS. Protective Effects of Icariin Against Learning and Memory Deficits Induced by Aluminium in Rats. Clin Exp Pharmacol Physiol (2007) 34(8):792–5. doi: 10.1111/j.1440-1681.2007.04647.x
113. Meng X, Fu M, Wang S, Chen W, Wang J, Zhang N. Naringin Ameliorates Memory Deficits and Exerts Neuroprotective Effects in a Mouse Model of Alzheimer’s Disease by Regulating Multiple Metabolic Pathways. Mol Med Rep (2021) 23(5):332–44. doi: 10.3892/mmr.2021.11971
114. Sachdeva AK, Kuhad A, Chopra K. Naringin Ameliorates Memory Deficits in Experimental Paradigm of Alzheimer’s Disease by Attenuating Mitochondrial Dysfunction. Pharmacol Biochem Behav (2014) 127:101–10. doi: 10.1016/j.pbb.2014.11.002
115. Wong SK, Chin KY, Ima-Nirwana S. Quercetin as an Agent for Protecting the Bone: A Review of the Current Evidence. Int J Mol Sci (2020) 21(17):6448–84. doi: 10.3390/ijms21176448
116. Yuan Z, Min J, Zhao Y, Cheng Q, Wang K, Lin S, et al. Quercetin Rescued TNF-Alpha-Induced Impairments in Bone Marrow-Derived Mesenchymal Stem Cell Osteogenesis and Improved Osteoporosis in Rats. Am J Transl Res (2018) 10(12):4313–21.
117. Lu J, Wu DM, Zheng YL, Hu B, Zhang ZF, Shan Q, et al. Quercetin Activates AMP-Activated Protein Kinase by Reducing PP2C Expression Protecting Old Mouse Brain Against High Cholesterol-Induced Neurotoxicity. J Pathol (2010) 222(2):199–212. doi: 10.1002/path.2754
Keywords: postmenopausal osteoporosis, macrophages, cytokines, rheumatoid arthritis, Alzheimer’s disease
Citation: Xu Y, Yan H, Zhang X, Zhuo J, Han Y, Zhang H, Xie D, Lan X, Cai W, Wang X, Wang S and Li X (2022) Roles of Altered Macrophages and Cytokines: Implications for Pathological Mechanisms of Postmenopausal Osteoporosis, Rheumatoid Arthritis, and Alzheimer’s Disease. Front. Endocrinol. 13:876269. doi: 10.3389/fendo.2022.876269
Received: 15 February 2022; Accepted: 05 April 2022;
Published: 10 June 2022.
Edited by:
Ziqing Li, Shandong Provincial Hospital Affiliated to Shandong First Medical University, ChinaReviewed by:
Giacomina Brunetti, University of Bari Aldo Moro, ItalyCopyright © 2022 Xu, Yan, Zhang, Zhuo, Han, Zhang, Xie, Lan, Cai, Wang, Wang and Li. This is an open-access article distributed under the terms of the Creative Commons Attribution License (CC BY). The use, distribution or reproduction in other forums is permitted, provided the original author(s) and the copyright owner(s) are credited and that the original publication in this journal is cited, in accordance with accepted academic practice. No use, distribution or reproduction is permitted which does not comply with these terms.
*Correspondence: Xihai Li, bGl4aWhhaWZ6QDE2My5jb20=
†These authors have contributed equally to this work
Disclaimer: All claims expressed in this article are solely those of the authors and do not necessarily represent those of their affiliated organizations, or those of the publisher, the editors and the reviewers. Any product that may be evaluated in this article or claim that may be made by its manufacturer is not guaranteed or endorsed by the publisher.
Research integrity at Frontiers
Learn more about the work of our research integrity team to safeguard the quality of each article we publish.