- 1Posgrado en Ciencias Genómicas, Universidad Autónoma de la Ciudad de México (UACM), Mexico City, Mexico
- 2Instituto de Fisiología Celular, Universidad Nacional Autónoma de México (UNAM), Mexico City, Mexico
- 3Neural Signal Transduction, Max Planck Florida Institute for Neuroscience, Jupiter, FL, United States
- 4Instituto Nacional de Cardiología “Ignacio Chávez”, Mexico City, Mexico
Approximately 70% of all breast cancer cases are estrogen receptor-alpha positive (ERα+) and any ERα signaling pathways deregulation is critical for the progression of malignant mammary neoplasia. ERα acts as a transcription factor that promotes the expression of estrogen target genes associated with pro-tumor activity in breast cancer cells. Furthermore, ERα is also part of extranuclear signaling pathways related to endocrine resistance. The regulation of ERα subcellular distribution and protein stability is critical to regulate its functions and, consequently, influence the response to endocrine therapies and progression of this pathology. This minireview highlights studies that have deciphered the molecular mechanisms implicated in controlling ERα stability and nucleo-cytoplasmic transport. These mechanisms offer information about novel biomarkers, therapeutic targets, and promising strategies for breast cancer treatment.
Introduction
Breast cancer is a collection of malignant mammary neoplasms that cause death in women worldwide (1–4). Breast cancer is classified in the subtypes luminal A, luminal B, HER2-overexpression, and basal-like (triple-negative) subtype, based on the detection mainly of ERα, PR, and HER2 expression by immunohistochemistry analysis (5). ERα (ERα+ breast cancer) is expressed in the luminal A/B and represents more than 70% of all cases of breast cancer (6, 7). Therefore, ERα detection is central in breast cancer tumors and is a target of some endocrine therapies, such as selective estrogen receptor downregulators (SERD) and selective estrogen receptor modulators (SERMs). Aromatase inhibitors (AI) are also used in endocrine therapy; however, they control the production of estrogens. A problem with these therapies is that patients develop de novo or acquired resistance (8).
ERα is a 66 kDa protein, a member of the nuclear receptor subfamily that is encoded by the ESR1 gene, displaying conserved domains such as two activation function domains (AF-1 and AF-2), one DNA-binding domain (DBD), and one ligand-binding domain (LBD) (9–12). Furthermore, ERα contains nuclear localization signals (NLS) in the hinge region and nuclear export signals (NES) in DBD and LBD (13–15). The structure and function of ERα are modulated by different posttranslational modifications, such as the phosphorylation of the AF-1 domain induced by E2 (estradiol) but also induced via growth factor signaling (16–19). This minireview is focused mainly on the molecular mechanisms that modulate the nucleo-cytoplasmic transport and stability of ERα in breast cancer.
ERα Signaling and Its Nucleo-Cytoplasmic Dynamics in Breast Cancer
ERα is localized in both the cytoplasm and the nucleus of breast cancer cells. The ERα canonical signaling pathway consists of the binding of E2 to the receptor LBD, triggering its homodimerization, enrichment into the nucleus, binding to estrogen-responsive element (ERE) in enhancers or promoters of E2-responsive genes, and recruitment of coregulators via the AF1/2 domains to induce gene expression (20, 21). Pioneer FTs open up local chromatin, allowing ERα to interact with ERE and recruit coregulators to modulate chromatin structure and gene expression (22). Coregulators are recruited by the AF-1 and AF-2 domains in an E2-independent and -dependent manner, and they are important for the interactions between ERα-dependent enhancers and promoters to synergistically regulate transcription in breast cancer cells (23–25). ERα also acts as a coregulator for diverse TF such as AP-1/c-Jun, ATF-2, NF-kappaB, p53, SP-1, and STAT1, modulating the expression of several genes, including late E2-target genes (16, 26–29). ERα can act as a coregulator when it is phosphorylated in response to growth factors, generating a crosstalk with other signaling pathways (30–35). It has been reported that the levels of DLC1 (dynein light chain 1) are increased in breast cancer and that DYNLL1, also named DLC1, promotes ERα nuclear accumulation and its activity in response to E2 (36) (Figure 1). In addition, ERα is membrane-associated via its palmitoylation, having the ability to respond to E2 at 3-15 min, generating secondary messengers such as Ca2+, cAMP, and nitric oxide. ERα also interacts with transmembrane receptors, such as RTK (receptor tyrosine kinases), GABAB, and mGluR (37–41).
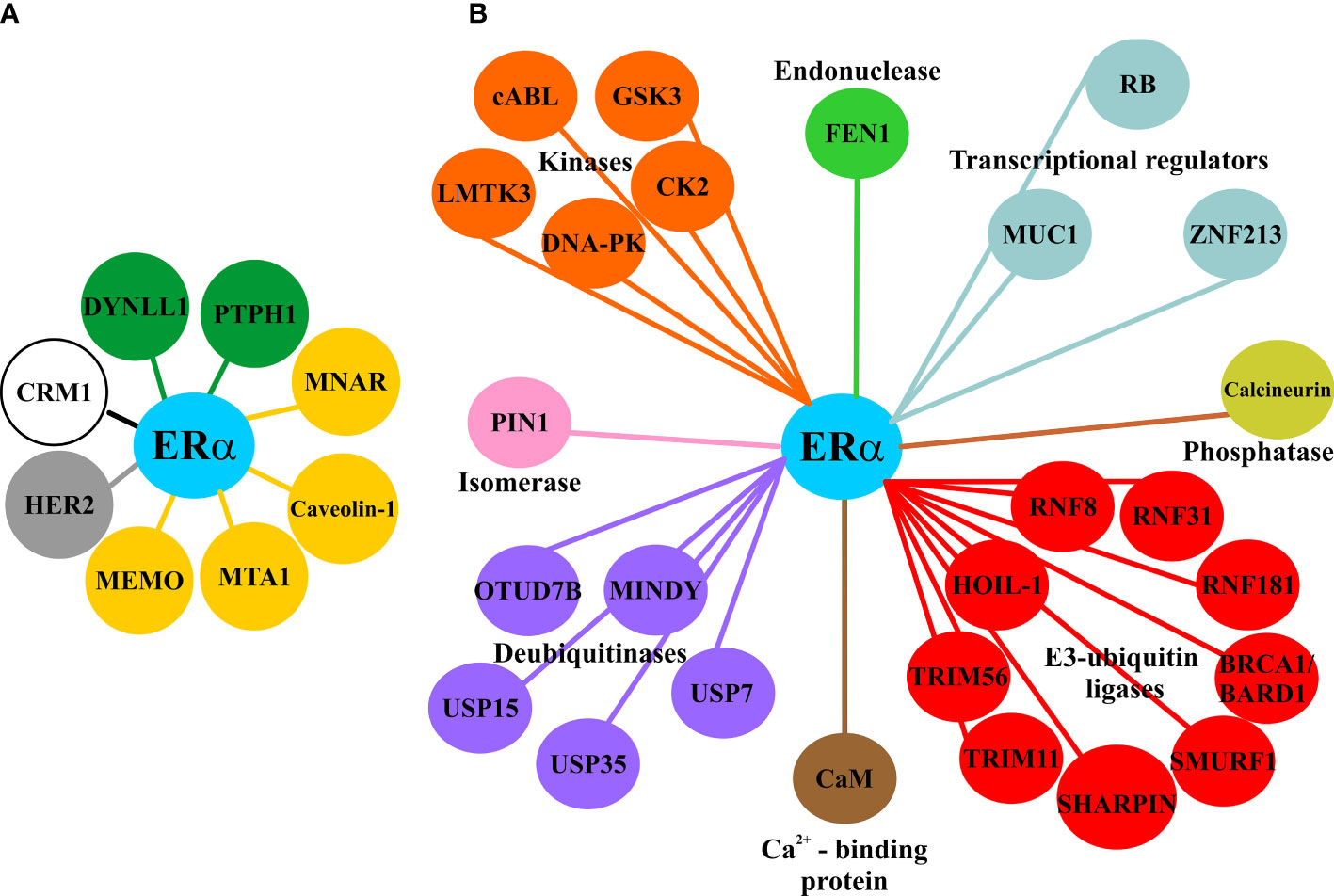
Figure 1 Proteins related to subcellular transport of ERα and its stability in breast cancer cells. (A) Principal proteins involved in the nuclear accumulation of ERα in the nucleus (green), in the extranuclear localization of ERα (yellow), required for the nuclear export (white), and correlated with the nuclear translocation of ERα (gray). (B) Interactome of proteins that increase the stability of ERα in breast cancer cells.
The nuclear export of ERα is mediated by non-canonical NES in the DBD and LBD, which are recognized by CRM-1 exportin, being an E2-dependent process in breast cancer cells (13, 15). The ERα Y537F mutant is unable to associate with CRM-1, resulting in its nuclear accumulation (42). The Y537 is the phosphorylated site by Src, and the treatment with a Src inhibitor (SU6656) or the expression of a dominant-negative Src protein decrease E2-induced ERα phosphorylation and nuclear export (15, 42, 43). In addition, the use of the CRM-1 inhibitor, LMB, decreases ERα transactivation, suggesting that a nucleo-cytoplasmic dynamic is required for ERα nuclear activity (44).
Moreover, E2 induces AKT-dependent phosphorylation of FKHR, promoting the nucleo-cytoplasmic transport of the ERα/FKHR complex (15, 43). In contrast, ATBF1 is another transcription factor enriched in the nucleus of MCF-7 cells in response to E2 hormone and in an ERα-dependent manner, whereas ATB1 is localized in the cytoplasm in those breast cancer cell lines that do not express ERα (45). These data suggest that the subcellular dynamics of some transcription factors may be dependent on ERα status.
In addition, the extranuclear localization of ERα is facilitated by its interaction with proteins such as MEMO (ErbB2-driven cell motility), MNAR (modulation of non-genomic actions of the estrogen receptor), and MTA1 (metastasis-associated 1). MEMO increases Y537 phosphorylation in the ERα and enhances cell proliferation and migration (46) (Figure 1). MNAR and truncated MTA1 sequester ERα and increase its activities out of the nucleus (47, 48). In contrast, the accumulation of ERα in the nucleus is promoted by PTPH1 (protein-tyrosine phosphatase H1) that reverts Src-dependent Y537 phosphorylation, and by the phosphorylation of T311 by p38 MAPK (49–51). Phosphorylated ERα at T311 has been found in human breast tumors (50), and the Y537S, Y537C, and Y537N mutations have been detected in metastatic mammary tumors that are resistant to endocrine therapies (52, 53) (Figure 2). ERα can interact with a signalosome complex that includes c-Src, PI3K, caveolin-1, straitin, and MNAR (54–56).Caveolin-1, a protein enriched mainly in caveolae, interacts with ERα, leading to the trafficking of ERα to caveolae to promote its localization on plasma membrane and the activation of non-genomic pathways (56, 57).
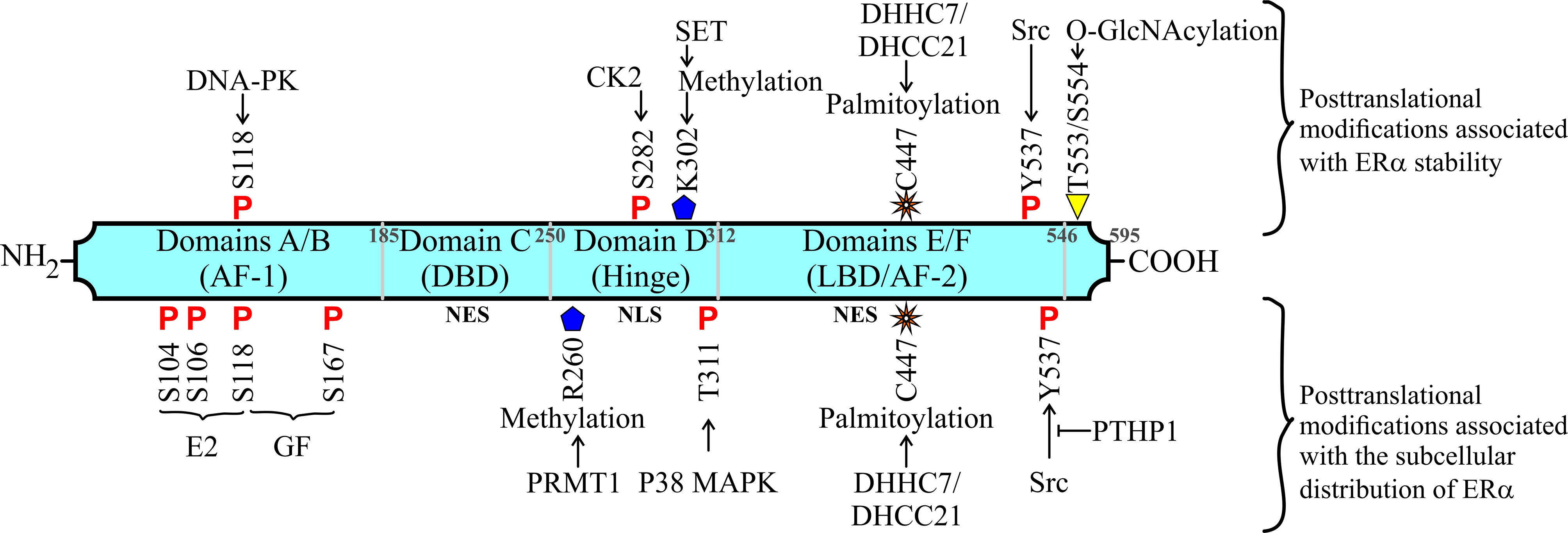
Figure 2 Other posttranslational modifications related to subcellular transport and stability of ERα in breast cancer. Structure of ERα protein and its functional domains. Up: Modifications related to ERα stability. Down: Modifications involved in the subcellular transport in breast cancer cells.
ERα Distribution and Its Relationship With Therapeutic Approaches for Breast Cancer
Some studies suggest that ERα nuclear distribution is associated with the sensitivity of breast cancer cells to endocrine therapy, whereas extranuclear localization is related to endocrine resistance. For example, Selinexor is a CRM-1 inhibitor that combined with tamoxifen can restore the sensitivity of cells to tamoxifen (58). In addition, when PTPH dephosphorylates Y537, ERα is enriched in the nucleus, and breast cancer cells are sensitive to tamoxifen and fulvestrant (49). Another example is the use of Src inhibitors; among them, dasatinib, in combination with tamoxifen, restores the nuclear distribution of ERα and the sensitivity to endocrine therapy of tamoxifen-resistant cells (59, 60). Moreover, MCF-7 breast cancer cells that overexpress HER2 display an ERα translocation nucleo-cytoplasm and are resistant to tamoxifen (61–63). Nuclear redistribution of ERα and re-sensitivity to endocrine therapy are recovered using the HER2 inhibitor AG825 and anti-HER2 monoclonal antibody (61, 62).
Additionally, the methylation of ERα at R260 via PRMT1 (arginine methyltransferase) allows the formation of methyl-ERα/Src/PI3K complex in response to estrogens. The interactions ERα/Src/PI3K is enhanced in aggressive mammary malignant tumors, promoting non-genomic signaling related to resistance to tamoxifen and poor survival. Hence, methyl-ERα/Src/PI3K complex has been proposed as a hallmark of aggressiveness and resistance to tamoxifen. Consequently, the disruption of functional interaction between ERα and PI3K, using the combination of Src or PI3K inhibitors plus tamoxifen or fulvestrant, has been proposed as a strategy in the treatment of ERα+ breast cancer (64, 65).
ERα Is Modulated via Its Mono-Ubiquitination and Polyubiquitination
The polyubiquitination of ERα at K302/K303 is induced by E2 and fulvestrant and is associated with its degradation via the UPS (66). However, the turnover of ERα induced by E2 is also important for its activity, since it has been reported that the inhibition of ERα degradation reduces the recruitment of RNA polymerase II to ERE, and the intranuclear dynamic of ERα is affected by transcriptional or proteasome inhibitors (67, 68). Moreover, ERα coactivators such as E6AP, RNF8, and SKP2 also function as E3-ubiquitin ligases, suggesting an intriguing interplay between ERα transcriptional activity and its polyubiquitination/degradation (29, 67, 69–72).
ERα monoubiquitination at K302/K303 residues modulates cell proliferation induced by E2 (73–76). These monoubiquitinations catalyzed by BRCA-1/BARD1 confers receptor stability under basal conditions (66, 73, 76). It has also been reported that E2 inhibits ERα monoubiquitination (77). In addition, UBD or ubiquitin-binding domains have been identified in the LBD of ERα (L429 and A430 residues), allowing the association of this receptor with ubiquitinated proteins. ERα monoubiquitination and its activity are affected when UBD is mutated (78, 79).
ERα Stability in Breast Cancer
Several studies have identified proteins that interact with ERα and inhibit its polyubiquitination and degradation (Figure 1). The primary functions of the ERα-polyubiquitination inhibitor proteins (EPIP) vary from being transcriptional coregulators, kinases, E3-ubiquitin ligases, or deubiquitinases (Table 1). Most of them are upregulated in breast cancer tissue, promoting ERα stability and breast cancer progression. Thus, proteins promoting ERα stability facilitates higher levels of this receptor, and its actions are associated with the expression of its target genes, cell proliferation, and endocrine resistance (71, 92, 94). One example of those proteins is the endonuclease FEN1, which is increased in tamoxifen-treated breast cancer patients, promoting the transcriptional activity of ERα. Moreover, FEN1 inhibits ERα degradation and maintains its stability to increase the expression of its target genes and cell proliferation. Inhibition of FEN1 decreases ERα activity and proliferation in breast cancer cells resistant to tamoxifen, suggesting the therapeutic potential of FEN1 as a target molecule in endocrine therapy resistance (101). Another example of EPIP is calcineurin, a Ca2+-dependent protein phosphatase, which dephosphorylates the Ser294 in ERα to inhibit its degradation via the UPS. Moreover, calcineurin facilitates the ERα phosphorylation at Ser118 by mTOR to increase its activation. A higher expression of calcineurin is associated with a poor prognosis in patients receiving endocrine therapy, suggesting that it is a key target for breast cancer treatment (102).
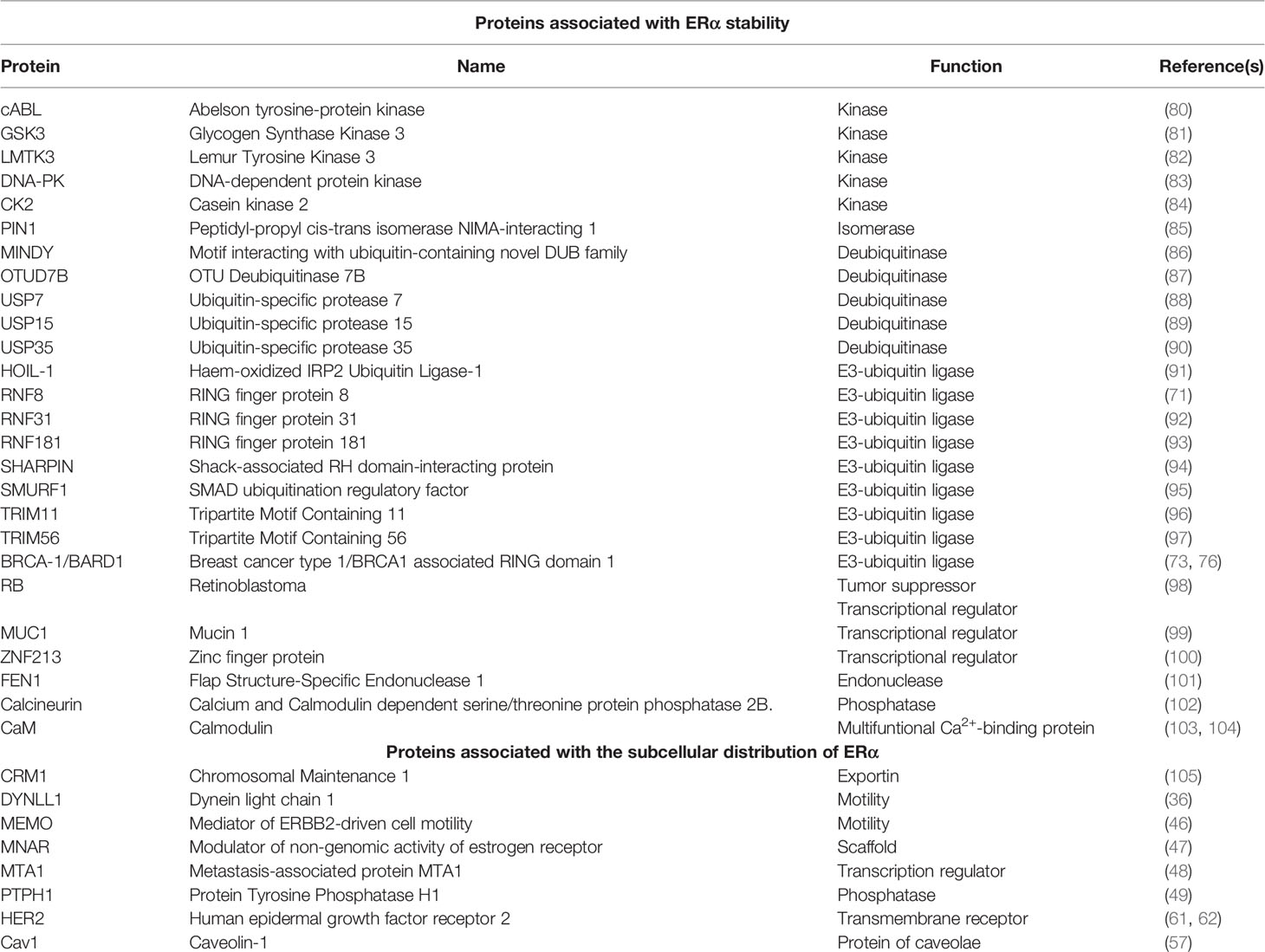
Table 1 Principal proteins involved in the ERα stability and subcellular transport in breast cancer cells.
Some EPIPs are E3-ubiquitin ligases that appear to play a complex role in stabilizing the ERα via different mechanisms. For example, most TRIMs (tripartite motif-containing) act as E3-ligases. In breast cancer, TRIM11 and TRIM56 confer ERα stability (96, 97), whereas TRIM8 increases ERα degradation in the cytoplasm (106). Furthermore, TRIM11, RNF8, RNF31, and SHARPIN catalyze the ERα monoubiquitination and inhibit its degradation (71, 92, 94, 96). Smurf1, TRIM56, and HOIL-1 block ERα degradation by inhibiting K48-specific polyubiquitination (91, 95, 97), whereas RNF181 induces K63-linked ubiquitination, which stabilizes ERα in BC cells (93).
Interestingly, some kinases affect the activity and stability of ERα receptor. For example, the LMTK3, GSK3 and cABL kinases interact with and phosphorylate ERα, avoiding its degradation (80–82). DNA-PK (DNA-dependent protein kinase) phosphorylates ERα at Ser-118 to stabilize it, promoting its transcriptional activity, and the proliferation of breast cancer cells (83). Furthermore, the S282 residue of ERα can be phosphorylated by CK2, resulting in the stability of this receptor in breast cancer cells (84).
Proteins with deubiquitinase activity are also central to regulate ERα stability in breast cancer, such as USP7, USP15, USP35, OTUD7B, and MINDY. For example, MINDY has a positive correlation with ERα levels, and promotes poor prognosis in breast cancer by stabilizing the ERα via the inhibition of its K48-polyubiquitination (87).
Furthermore, the calmodulin (CaM) protein modulates ERα transactivation in a Ca2+-dependent manner (107, 108). The residues Pro-295 to Ser-317 localized between hinge and LBD of ERα are central for binding of CaM. Mutations in these sites decrease the ERα interaction with CaM and the E2-dependent gene transcription (108–110). Studies using a synthetic peptide containing these major determinants (ERα17p: P295-T311) compared to control peptides with Lys-302 and Lys-303 mutated to alanines or glycines (ERα17pAA or ERα17pGG) evidenced that this sequence has an auto-inhibitory activity, which may be relieved by CaM binding (103, 104, 109, 110). Hence, this ERα motif seems to be essential to interact with proteins implicated in its regulation. Interestingly, CaM interacts with ERα and protects it from proteolysis by inhibiting the E6AP-dependent degradation of this receptor (111, 112).
Posttranslational modifications, such as methylation (by SET7 at K302) and palmitoylation (by DHHC7 and DHHC21 at C447) also contribute to ERα stability, inhibiting its degradation (37, 113, 114). In addition, O-GlcNAcylation at T553/S554 residues in ERα mediated by GREB inhibits ZNF598 ubiquitin ligase-dependent degradation, leading to ERα stability (115). In addition, other stimuli, such as the aluminum salts present in antiperspirants, have been associated with ERα stability and accumulation in the nucleus, with an increase in gene expression (116). However, ERα stability is also conferred via indirect mechanisms. For example, PEBP4 (phosphatidyl-ethanolamine-binding protein 4) decreases ERα degradation induced by its Src-dependent phosphorylation, since PEBP4 inhibits the association between Src and ERα (117). The Y537 residue in ERα is phosphorylated by Src kinase to recruit the E6AP protein, which is an E3-Ub ligase that polyubiquitinates ERα for its degradation in breast cancer cells. The interaction of PIN1 with ERα inhibits its phosphorylation (at Y537) and its interaction with E6AP, conferring stability (70, 85, 117).
The proteolysis of ERα can be affected by ERα protein accumulation (118–120), which leads to non-classical mechanisms called concentration-inducible ERα function, where ERα is active in a manner stimuli-independent (E2 signal, or growth factor signals), promoting changes in the expression of its target genes, resulting in new E2-induced genes (121, 122). These data suggest that alterations in the interplay of proteolysis and stability of ERα may have crucial implications in malignant mammary tumors.
Although the higher levels of ERα by increasing its stability are associated with cancer progression and endocrine resistance, the reduction of ERα levels by an increase in its degradation is also related to endocrine resistance, considering that ERα is the target for SERMs and SERDs. Hence, CUEDC2 induces ERα degradation via the UPS, and some malignant mammary tumors with resistance to tamoxifen show high levels of CUEDC2 protein with low levels of ERα (123, 124). In contrast, RB is a protein that stabilizes ERα and protects it from its degradation. Increased ERα degradation through the UPS has been reported in RB-knockdown breast cancer cell lines (98), whereas ERα– mammary tumors display alterations in the expression and function of RB (125, 126).
ERα Stability and Its Relationship With Therapeutic Approaches
Fulvestrant, a SERD clinically used as first-line endocrine therapy to inhibit tumor growth, promotes ERα polyubiquitination and degradation. Other SERDs are being investigated to improve their effects, availability, and administration routes (127–133). Intriguingly, when the expression of large tumor suppressor kinases 1 and 2 (LATS1 and 2) is reduced, the sensitivity to fulvestrant of breast cancer cells is decreased. LATS1/2 (two mediators of the Hippo pathway) are associated with the induction of ERα degradation. High levels of LATS1/2 are detected in patients with breast cancer ERα– and short relapse-free survival (134).
ERα mutations, such as Y537S/N/C, D538G, E380Q, or S463P have been associated with endocrine resistance. In particular, the mutations Y537S, Y537N, Y537C, D538G, and E380Q localized in the LBD of ERα cause an E2-independent activity of ERα (135, 136). These mutations have been detected mainly in metastatic breast cancer (137) and affect gene expression (138) and ERα-dependent cistrome (139). Mutations in the Y537 residue (Y537S, Y537C, and Y537N) can affect the degradation of this receptor, which is associated with metastasis and resistance to endocrine therapy in patients (52, 53, 70, 140). After cells acquire endocrine resistance, Y537C and Y537S are detected, which may be due to long-term E2 deprivation (141).
Mutations in the Y537 residue do not affect fulvestrant and AZD9496 treatments, suggesting the use of SERD to treat endocrine resistance. However, an interesting study showed that when the E2-induced polyubiquitination of ERα is decreased, the ERα stability is increased only in invasive lobular breast carcinoma but not in invasive ductal carcinoma. Fulvestrant was effective in both breast cancer subtypes; however, the SERD AZD9496 does not have the same effect in the reduction of ERα stability in invasive lobular breast carcinoma, suggesting that ERα stability and its functional implications are regulated differentially by SERD therapies in both histological subtypes of breast cancer (142).
In addition to SERDs, other modulators of ERα that diminish its stability are being studied. For example, MHO7 (6-epi-ophiobolin G) is a compound that inhibits the synthesis of ERα mRNA and increases the degradation of this receptor via the UPS, postulating it as a drug candidate to promote ERα downregulation and block breast cancer progression (143).
Discussion
Most cases of breast cancer are ERα+, where this receptor displays pro-tumoral activity, and the molecular mechanisms that regulate its activity are crucial. Some patients with breast cancer have or develop resistance to SERMs and AI, whereas the treatment with SERDs as fulvestrant is not affected by mutations in ERα related to endocrine therapy. The anti-tumor effect of SERDs is based on ERα degradation via the UPS. Interestingly, E2 induces ERα degradation through UPS, both in the cytoplasm and nucleus, whereas fulvestrant induces the degradation of this receptor in the nuclear matrix. Additionally, ERα protein can be downregulated by E2-dependent lysosomal degradation (144), dynamin II-dependent autophagy (145), and via its association with caveolin 1/2 (146), and the clathrin-heavy chain (CHC) endocytic protein (147).
In recent years, many investigations on ERα stability and its nuclear export in breast cancer suggest that these events affect the nuclear and extranuclear activity of this receptor and the cell response to endocrine therapies. For example, Src-dependent phosphorylation at Y537 is required for nuclear export and E6AP-dependent degradation in breast cancer cells, suggesting that ERα subcellular distribution may be associated with its stability (42, 70, 140, 148). Posttranslational modifications of ERα, such as phosphorylation and poly-/mono-ubiquitination, appear to be central for the modulation of its stability, transport, and localization, and some may compete by the same site to modulate ERα stability and activity; for example, K303 is acetylated, mono- and poly-ubiquitinated in breast cancer cells (66, 73, 76, 113, 149), and some mutations at K303 exist in premalignant breast lesions (150, 151). Moreover, many proteins participate to protect ERα from degradation and affect its subcellular distribution in breast cancer, denoting a complex interplay among these elements, and some of them may be potential therapeutic targets. Furthermore, all data indicate that the response to endocrine therapy requires a dynamic in ERα stability/degradation and its subcellular transport.
ERα proteolysis is key to the design of new therapeutic strategies to treat breast cancer, such as PROTACs (proteolysis targeting chimeric) technology, which are modulators of ERα and its mutants (136, 152). PROTACs contain a module for binding to the target protein and another module for the recognition of E3 ligase. Hence, PROTACs bind to their target protein to promote its ubiquitination and degradation, and different PROTACs have been developed to degrade ERα via the UPS in breast cancer cells, exhibiting antitumor activity. PROTACs are being evaluated in patients with metastatic breast cancer and may become promising therapies (153). It is important to consider the implications of ERα stability in malignant mammary neoplasia to avoid some resistance to SERD or PROTAC treatments.
In conclusion, more studies focusing on ERα stability and nuclear export in breast cancer are required. However, several investigations have emerged to date, indicating that therapeutic strategies based on controlling ERα abundance and distribution in breast cancer may improve the status of patients with endocrine resistance.
Author Contributions
All authors listed have made a substantial, direct, and intellectual contribution to the work and approved it for publication.
Funding
Our work is partially supported by institutional budget from UACM and IFC at UNAM.
Conflict of Interest
The authors declare that the research was conducted in the absence of any commercial or financial relationships that could be construed as a potential conflict of interest.
Publisher’s Note
All claims expressed in this article are solely those of the authors and do not necessarily represent those of their affiliated organizations, or those of the publisher, the editors and the reviewers. Any product that may be evaluated in this article, or claim that may be made by its manufacturer, is not guaranteed or endorsed by the publisher.
References
1. Bray F, Ferlay J, Soerjomataram I, Siegel RL, Torre LA, Jemal A. Global Cancer Statistics `2018: GLOBOCAN Estimates of Incidence and Mortality Worldwide for 36 Cancers in 185 Countries. CA Cancer J Clin (2018) 68:394–424. doi: 10.3322/caac.21492
2. Ferlay J, Colombet M, Soerjomataram I, Mathers C, Parkin DM, Piñeros M, et al. Estimating the Global Cancer Incidence and Mortality in 2018: GLOBOCAN Sources and Methods. Int J Cancer (2019) 144:1941–53. doi: 10.1002/ijc.31937
3. Aleskandarany MA, Abduljabbar R, Ashankyty I, Elmouna A, Jerjees D, Ali S, et al. Prognostic Significance of Androgen Receptor Expression in Invasive Breast Cancer: Transcriptomic and Protein Expression Analysis. Breast Cancer Res Treat (2016) 159:215–27. doi: 10.1007/s10549-016-3934-5
4. Turashvili G, Brogi E. Tumor Heterogeneity in Breast Cancer. Front Med (2017) 4:227. doi: 10.3389/fmed.2017.00227
5. Goldhirsch A, Winer EP, Coates AS, Gelber RD, Piccart-Gebhart M, Thürlimann B, et al. Personalizing the Treatment of Women With Early Breast Cancer: Highlights of the St Gallen International Expert Consensus on the Primary Therapy of Early Breast Cancer 2013. Ann Oncol (2013) 24:2206–23. doi: 10.1093/annonc/mdt303
6. Bertucci F, Finetti P, Birnbaum D. Basal Breast Cancer: A Complex and Deadly Molecular Subtype. Curr Mol Med (2011) 12:96–110. doi: 10.2174/156652412798376134
7. Dai X, Xiang L, Li T, Bai Z. Cancer Hallmarks, Biomarkers and Breast Cancer Molecular Subtypes. J Cancer (2016) 7:1281–94. doi: 10.7150/jca.13141
8. Osborne CK, Schiff R. Mechanisms of Endocrine Resistance in Breast Cancer. Annu Rev Med (2011) 62:233–47. doi: 10.1146/annurev-med-070909-182917
9. Kumar R, Zakharov MN, Khan SH, Miki R, Jang H, Toraldo G, et al. The Dynamic Structure of the Estrogen Receptor. J Amino Acids (2011) 2011:1–7. doi: 10.4061/2011/812540
10. Mader S, Chambon P, White JH. Defining a Minimal Estrogen Receptor DNA Binding Domain. Nucleic Acids Res (1993) 21:1125–32. doi: 10.1093/nar/21.5.1125
11. Métivier R, Penot G, Flouriot G, Pakdel F. Synergism Between Erα Transactivation Function 1 (AF-1) and AF-2 Mediated by Steroid Receptor Coactivator Protein-1: Requirement for the AF-1 α-Helical Core and for a Direct Interaction Between the N- and C-Terminal Domains. Mol Endocrinol (2001) 15:1953–70. doi: 10.1210/mend.15.11.0727
12. Zwart W, De Leeuw R, Rondaij M, Neefjes J, Mancini MA, Michalides R. The Hinge Region of the Human Estrogen Receptor Determines Functional Synergy Between AF-1 and AF-2 in the Quantitative Response to Estradiol and Tamoxifen. J Cell Sci (2010) 123:1253–61. doi: 10.1242/jcs.061135
13. Black BE, Holaska JM, Rastinejad F, Paschal BM. DNA Binding Domains in Diverse Nuclear Receptors Function as Nuclear Export Signals. Curr Biol (2001) 11:1749–58. doi: 10.1016/S0960-9822(01)00537-1
14. Burns KA, Li Y, Arao Y, Petrovich RM, Korach KS. Selective Mutations in Estrogen Receptor α D-Domain Alters Nuclear Translocation and non-Estrogen Response Element Gene Regulatory Mechanisms. J Biol Chem (2011) 286:12640–9. doi: 10.1074/jbc.M110.187773
15. Lombardi M, Castoria G, Migliaccio A, Barone MV, Di Stasio R, Ciociola A, et al. Hormone-Dependent Nuclear Export of Estradiol Receptor and DNA Synthesis in Breast Cancer Cells. J Cell Biol (2008) 182:327–40. doi: 10.1083/jcb.200712125
16. Acconcia F, Kumar R. Signaling Regulation of Genomic and Nongenomic Functions of Estrogen Receptors. Cancer Lett (2006) 238:1–14. doi: 10.1016/j.canlet.2005.06.018
17. Atsriku C, Britton DJ, Held JM, Schilling B, Scott GK, Gibson BW, et al. Systematic Mapping of Posttranslational Modifications in Human Estrogen Receptor-α With Emphasis on Novel Phosphorylation Sites. Mol Cell Proteomics (2009) 8:467–80. doi: 10.1074/mcp.M800282-MCP200
18. Britton DJ, Scott GK, Schilling B, Atsriku C, Held JM, Gibson BW, et al. A Novel Serine Phosphorylation Site Detected in the N-Terminal Domain of Estrogen Receptor Isolated From Human Breast Cancer Cells. J Am Soc Mass Spectrom (2008) 19:729–40. doi: 10.1016/j.jasms.2008.02.008
19. Held JM, Britton DJ, Scott GK, Lee EL, Schilling B, Baldwin MA, et al. Ligand Binding Promotes CDK-Dependent Phosphorylation of ER-Alpha on Hinge Serine 294 But Inhibits Ligand-Independent Phosphorylation of Serine 305. Mol Cancer Res (2012) 10:1120–32. doi: 10.1158/1541-7786.MCR-12-0099
20. Hah N, Murakami S, Nagari A, Danko CG, Lee Kraus W. Enhancer Transcripts Mark Active Estrogen Receptor Binding Sites. Genome Res (2013) 23:1210–23. doi: 10.1101/gr.152306.112
21. Hah N, Kraus WL. Hormone-Regulated Transcriptomes: Lessons Learned From Estrogen Signaling Pathways in Breast Cancer Cells. Mol Cell Endocrinol (2014) 382:652–64. doi: 10.1016/j.mce.2013.06.021
22. Manavathi B, Samanthapudi VSK, Gajulapalli VNR. Estrogen Receptor Coregulators and Pioneer Factors: The Orchestrators of Mammary Gland Cell Fate and Development. Front Cell Dev Biol (2014) 2:34. doi: 10.3389/fcell.2014.00034
23. Joseph C, Macnamara O, Craze M, Russell R, Provenzano E, Nolan CC, et al. Mediator Complex (MED) 7: A Biomarker Associated With Good Prognosis in Invasive Breast Cancer, Especially ER+ Luminal Subtypes. Br J Cancer (2018) 118:1142–51. doi: 10.1038/s41416-018-0041-x
24. Panigrahi AK, Foulds CE, Lanz RB, Hamilton RA, Yi P, Lonard DM, et al. SRC-3 Coactivator Governs Dynamic Estrogen-Induced Chromatin Looping Interactions During Transcription. Mol Cell (2018) 70:679–94.e7. doi: 10.1016/j.molcel.2018.04.014
25. Zhang X, Krutchinsky A, Fukuda A, Chen W, Yamamura S, Chait BT, et al. MED1/TRAP220 Exists Predominantly in a TRAP/Mediator Subpopulation Enriched in RNA Polymerase II and is Required for ER-Mediated Transcription. Mol Cell (2005) 19:89–100. doi: 10.1016/j.molcel.2005.05.015
26. Marino M, Galluzzo P, Ascenzi P. Estrogen Signaling Multiple Pathways to Impact Gene Transcription. Curr Genomics (2006) 7:497–508. doi: 10.2174/138920206779315737
27. Björnström L, Sjöberg M. Estrogen Receptor-Dependent Activation of AP-1 via Non-Genomic Signalling. Nucl Recept (2004) 2:1–11. doi: 10.1186/1478-1336-2-3
28. Frasor J, Weaver A, Pradhan M, Dai Y, Miller LD, Lin CY, et al. Positive Cross-Talk Between Estrogen Receptor and NF-κB in Breast Cancer. Cancer Res (2009) 69:8918–25. doi: 10.1158/0008-5472.CAN-09-2608
29. Zhou W, Srinivasan S, Nawaz Z, Slingerland JM. ERα, SKP2 and E2F-1 Form a Feed Forward Loop Driving Late Erα Targets and G1 Cell Cycle Progression. Oncogene (2014) 33:2341–53. doi: 10.1038/onc.2013.197
30. Kato S. Estrogen Receptor-Mediated Cross-Talk With Growth Factor Signaling Pathways. Breast Cancer (2001) 8:3–9. doi: 10.1007/BF02967472
31. Lee AV, Cui X, Oesterreich S. Cross-Talk Among Estrogen Receptor, Epidermal Growth Factor, and Insulin-Like Growth Factor Signaling in Breast Cancer. Clin Cancer Res (2001) 7:4429s–35s.
32. Schiff R, Massarweh SA, Shou J, Bharwani L, Mohsin SK, Osborne CK, et al. Cross-Talk Between Estrogen Receptor and Growth Factor Pathways As A Molecular Target for Overcoming Endocrine Resistance. Clin Cancer Res (2004) 10(Pt 2):331S–6S. doi: 10.1158/1078-0432.CCR-031212
33. Smith CL. Cross-Talk Between Peptide Growth Factor and Estrogen Receptor Signaling Pathways. Biol Reprod (1998) 58(3):627–32. doi: 10.1095/biolreprod58.3.627
34. Le Romancer M, Poulard C, Cohen P, Sentis SP, Renoir JM, Corbo L. Cracking the Estrogen Receptor’s Posttranslational Code in Breast Tumors. Endocr Rev (2011) 32:597–622. doi: 10.1210/er.2010-0016
35. Treviño LS, Weigel NL. Phosphorylation: A Fundamental Regulator of Steroid Receptor Action. Trends Endocrinol Metab (2013) 24:515–24. doi: 10.1016/j.tem.2013.05.008
36. Rayala SK, den Hollander P, Balasenthil S, Yang Z, Broaddus RR, Kumar R. Functional Regulation of Oestrogen Receptor Pathway by the Dynein Light Chain 1. EMBO Rep (2005) 6:538–44. doi: 10.1038/sj.embor.7400417
37. Pedram A, Razandi M, Deschenes RJ, Levin ER. DHHC-7 and -21 are Palmitoylacyltransferases for Sex Steroid Receptors. Mol Biol Cell (2012) 23:188–99. doi: 10.1091/mbc.E11-07-0638
38. Acconcia F, Marino M. Synergism Between Genomic and non Genomic Estrogen Action Mechanisms. IUBMB Life (2003) 55:145–50. doi: 10.1080/1521654031000110172
39. Acconcia F, Ascenzi P, Fabozzi G, Visca P, Marino M. S-Palmitoylation Modulates Human Estrogen Receptor-α Functions. Biochem Biophys Res Commun (2004) 316:878–83. doi: 10.1016/j.bbrc.2004.02.129
40. Soltysik K, Czekaj P. Membrane Estrogen Receptors - is it an Alternative Way of Estrogen Action? J Physiol Pharmacol (2013) 64:129–42.
41. Wessler S, Otto C, Wilck N, Stangl V, Fritzemeier KH. Identification of Estrogen Receptor Ligands Leading to Activation of non-Genomic Signaling Pathways While Exhibiting Only Weak Transcriptional Activity. J Steroid Biochem Mol Biol (2006) 98:25–35. doi: 10.1016/j.jsbmb.2005.08.003
42. Castoria G, Giovannelli P, Lombardi M, De Rosa C, Giraldi T, De Falco A, et al. Tyrosine Phosphorylation of Estradiol Receptor by Src Regulates its Hormone-Dependent Nuclear Export and Cell Cycle Progression in Breast Cancer Cells. Oncogene (2012) 31:4868–77. doi: 10.1038/onc.2011.642
43. Castoria G, Migliaccio A, Auricchio F. Signaling-Dependent Nuclear Export of Estradiol Receptor Controls Cell Cycle Progression in Breast Cancer Cells. Mol Cell Endocrinol (2009) 308:26–31. doi: 10.1016/j.mce.2009.01.006
44. Nonclercq D, Journé F, Laïos I, Chaboteaux C, Toillon RA, Leclercq G, et al. Effect of Nuclear Export Inhibition on Estrogen Receptor Regulation in Breast Cancer Cells. J Mol Endocrinol (2007) 39:105–18. doi: 10.1677/JME-07-0040
45. Li M, Zhang C, Zhong Y, Zhao J. Cellular Localization of ATBF1 Protein and its Functional Implication in Breast Epithelial Cells. Biochem Biophys Res Commun (2017) 490:492–8. doi: 10.1016/j.bbrc.2017.06.068
46. Frei A, MacDonald G, Lund I, Gustafsson JÅ, Hynes NE, Nalvarte I. Memo Interacts With C-Src to Control Estrogen Receptor Alpha Sub-Cellular Localization. Oncotarget (2016) 7:56170–82. doi: 10.18632/oncotarget.10856
47. Vadlamudi RK, Balasenthil S, Sahin AA, Kies M, Weber RS, Kumar R, et al. Novel Estrogen Receptor Coactivator PELP1/MNAR Gene and Erβ Expression in Salivary Duct Adenocarcinoma: Potential Therapeutic Targets. Hum Pathol (2005) 36:670–5. doi: 10.1016/j.humpath.2005.03.016
48. Kumar R, Wang RA, Mazumdar A, Talukder AH, Mandal M, Yang Z, et al. A Naturally Occurring MTA1 Variant Sequesters Oestrogen Receptor-α in the Cytoplasm. Nature (2002) 418:654–7. doi: 10.1038/nature00889
49. Suresh PS, Ma S, Migliaccio A, Chen G. Protein-Tyrosine Phosphatase H1 Increases Breast Cancer Sensitivity to Antiestrogens by Dephosphorylating Estrogen Receptor at Tyr537. Mol Cancer Ther (2013) 13:230–8. doi: 10.1158/1535-7163.MCT-13-0610
50. Lee H, Bai W. Regulation of Estrogen Receptor Nuclear Export by Ligand-Induced and P38-Mediated Receptor Phosphorylation. Mol Cell Biol (2002) 22:5835–45. doi: 10.1128/mcb.22.16.5835-5845.2002
51. Antoon JW, Bratton MR, Guillot LM, Wadsworth S, Salvo VA, Elliott S, et al. Pharmacology and Anti-Tumor Activity of RWJ67657, a Novel Inhibitor of P38 Mitogen Activated Protein Kinase. Am J Cancer Res (2012) 2:446–58.
52. Alluri PG, Speers C, Chinnaiyan AM. Estrogen Receptor Mutations and Their Role in Breast Cancer Progression. Breast Cancer Res (2014) 494:1–8. doi: 10.1186/s13058-014-0494-7
53. Fanning SW, Mayne CG, Dharmarajan V, Carlson KE, Martin TA, Novick SJ, et al. Estrogen Receptor Alpha Somatic Mutations Y537S and D538G Confer Breast Cancer Endocrine Resistance by Stabilizing the Activating Function-2 Binding Conformation. Elife (2016) e12792:1–25. doi: 10.7554/eLife.12792
54. Klinge CM. Estrogenic Control of Mitochondrial Function and Biogenesis. J Cell Biochem (2008) 105:1342–51. doi: 10.1002/jcb.21936
55. Li L, Sacks DB. Functional Interactions Between Calmodulin and Estrogen Receptor-α. Cell Signal (2007) 19:439–43. doi: 10.1016/j.cellsig.2006.08.018
56. Acconcia F, Fiocchetti M, Busonero C, Fernandez VS, Montalesi E, Cipolletti M, et al. The Extra-Nuclear Interactome of the Estrogen Receptors: Implications for Physiological Functions. Mol Cell Endocrinol (2021) 538:111452. doi: 10.1016/j.mce.2021.111452
57. Romano A, Feola A, Porcellini A, Gigantino V, Di Bonito M, Di Mauro A, et al. Estrogen Induces Selective Transcription of Caveolin1 Variants in Human Breast Cancer Through Estrogen Responsive Element-Dependent Mechanisms. Int J Mol Sci (2020) 21:1–15. doi: 10.3390/ijms21175989
58. Wrobel K, Zhao YC, Kulkoyluoglu E, Chen KLA, Hieronymi K, Holloway J, et al. Erα-XPO1 Cross Talk Controls Tamoxifen Sensitivity in Tumors by Altering ERK5 Cellular Localization. Mol Endocrinol (2016) 30:1029–45. doi: 10.1210/me.2016-1101
59. Fan P, Wang J, Santen RJ, Yue W. Long-Term Treatment With Tamoxifen Facilitates Translocation of Estrogen Receptor α Out of the Nucleus and Enhances its Interaction With EGFR in MCF-7 Breast Cancer Cells. Cancer Res (2007) 67:1352–60. doi: 10.1158/0008-5472.CAN-06-1020
60. Guest SK, Ribas R, Pancholi S, Nikitorowicz-Buniak J, Simigdala N, Dowsett M, et al. Src is a Potential Therapeutic Target in Endocrine-Resistant Breast Cancer Exhibiting Low Estrogen Receptor-Mediated Transactivation. PloS One (2016) e0157397:1–15. doi: 10.1371/journal.pone.0157397
61. Pancholi S, Lykkesfeldt AE, Hilmi C, Banerjee S, Leary A, Drury S, et al. ERBB2 Influences the Subcellular Localization of the Estrogen Receptor in Tamoxifen-Resistant MCF-7 Cells Leading to the Activation of AKT and RPS6KA2. Endocr Relat Cancer (2008) 15:985–1002. doi: 10.1677/ERC-07-0240
62. Yang Z, Barnes CJ, Kumar R. Human Epidermal Growth Factor Receptor 2 Status Modulates Subcellular Localization of and Interaction With Estrogen Receptor α in Breast Cancer Cells. Clin Cancer Res (2004) 10:3621–8. doi: 10.1158/1078-0432.CCR-0740-3
63. Shou J, Massarweh S, Osborne CK, Wakeling AE, Ali S, Weiss H, et al. Mechanisms of Tamoxifen Resistance: Increased Estrogen Receptor-HER2/neu Cross-Talk in ER/HER2-Positive Breast Cancer. J Natl Cancer Inst (2004) 96:926–35. doi: 10.1093/jnci/djh166
64. Poulard C, Jacquemetton J, Trédan O, Cohen PA, Vendrell J, Ghayad SE, et al. Oestrogen non-Genomic Signalling is Activated in Tamoxifen-Resistant Breast Cancer. Int J Mol Sci (2019) 2773:1–13. doi: 10.3390/ijms20112773
65. Jacquemetton J, Kassem L, Poulard C, Dahmani A, De Plater L, Montaudon E, et al. Analysis of Genomic and non-Genomic Signaling of Estrogen Receptor in PDX Models of Breast Cancer Treated With a Combination of the PI3K Inhibitor Alpelisib (BYL719) and Fulvestrant. Breast Cancer Res (2021) 57:1–15. doi: 10.1186/s13058-021-01433-8
66. Berry NB, Fan M, Nephew KP. Estrogen Receptor-α Hinge-Region Lysines 302 and 303 Regulate Receptor Degradation by the Proteasome. Mol Endocrinol (2008) 22:1535–51. doi: 10.1210/me.2007-0449
67. Reid G, Hübner MR, Métivier R, Brand H, Denger S, Manu D, et al. Cyclic, Proteasome-Mediated Turnover of Unliganded and Liganded Erα on Responsive Promoters is an Integral Feature of Estrogen Signaling. Mol Cell (2003) 11:695–707. doi: 10.1016/S1097-2765(03)00090-X
68. Stenoien DL, Patel K, Mancini MG, Dutertre M, Smith CL, O’Malley BW, et al. FRAP Reveals That Mobility of Oestrogen Receptor-α is Ligand- and Proteasome-Dependent. Nat Cell Biol (2001) 3:15–23. doi: 10.1038/35050515
69. Helzer KT, Hooper C, Miyamoto S, Alarid ET. Ubiquitylation of Nuclear Receptors: New Linkages and Therapeutic Implications. J Mol Endocrinol (2015) 54:R151–67. doi: 10.1530/JME-14-0308
70. Sun J, Zhou W, Kaliappan K, Nawaz Z, Slingerland JM. Erα Phosphorylation at Y537 by Src Triggers E6-AP-Erα Binding, Erα Ubiquitylation, Promoter Occupancy, and Target Gene Expression. Mol Endocrinol (2012) 26:1567–77. doi: 10.1210/me.2012-1140
71. Wang S, Luo H, Wang C, Sun H, Sun G, Sun N, et al. RNF8 Identified as a Co-Activator of Estrogen Receptor α Promotes Cell Growth in Breast Cancer. Biochim Biophys Acta - Mol Basis Dis (2017) 1863:1615–28. doi: 10.1016/j.bbadis.2017.02.011
72. Métivier R, Reid G, Gannon F. Transcription in Four Dimensions: Nuclear Receptor-Directed Initiation of Gene Expression. EMBO Rep (2006) 7:161–7. doi: 10.1038/sj.embor.7400626
73. Eakin CM, MacCoss MJ, Finney GL, Klevit RE. Estrogen Receptor α is a Putative Substrate for the BRCA1 Ubiquitin Ligase. Proc Natl Acad Sci U S A (2007) 104:5794–9. doi: 10.1073/pnas.0610887104
74. La Rosa P, Acconcia F. Signaling Functions of Ubiquitin in the 17β-Estradiol (E2):estrogen Receptor (ER) α Network. J Steroid Biochem Mol Biol (2011) 127:223–30. doi: 10.1016/j.jsbmb.2011.07.008
75. La Rosa P, Pesiri V, Marino M, Acconcia F. 17β-Estradiol-Induced Cell Proliferation Requires Estrogen Receptor (ER) α Monoubiquitination. Cell Signal (2011) 23:1128–35. doi: 10.1016/j.cellsig.2011.02.006
76. Ma Y, Fan S, Hu C, Meng Q, Fuqua SA, Pestell RG, et al. BRCA1 Regulates Acetylation and Ubiquitination of Estrogen Receptor-α. Mol Endocrinol (2010) 24:76–90. doi: 10.1210/me.2009-0218
77. La Rosa P, Marino M, Acconcia F. 17B-Estradiol Regulates Estrogen Receptor A Monoubiquitination. IUBMB Life (2011) 63:49–53. doi: 10.1002/iub.414
78. Pesiri V, Di Muzio E, Polticelli F, Acconcia F. Selective Binding of Estrogen Receptor α to Ubiquitin Chains. IUBMB Life (2016) 68:569–77. doi: 10.1002/iub.1514
79. Pesiri V, La Rosa P, Stano P, Acconcia F. Identification of an Estrogen Receptor a non Covalent Ubiquitin-Binding Surface: Role in 17β-Estradiolinduced Transcriptional Activity. J Cell Sci (2013) 126:2577–82. doi: 10.1242/jcs.123307
80. He X, Zheng Z, Song T, Wei C, Ma H, Ma Q, et al. C-Abl Regulates Estrogen Receptor α Transcription Activity Through its Stabilization by Phosphorylation. Oncogene (2010) 29:2238–51. doi: 10.1038/onc.2009.513
81. Grisouard J, Medunjanin S, Hermani A, Shukla A, Mayer D. Glycogen Synthase Kinase-3 Protects Estrogen Receptor α From Proteasomal Degradation and is Required for Full Transcriptional Activity of the Receptor. Mol Endocrinol (2007) 21:2427–39. doi: 10.1210/me.2007-0129
82. Giamas G, Filipović A, Jacob J, Messier W, Zhang H, Yang D, et al. Kinome Screening for Regulators of the Estrogen Receptor Identifies LMTK3 as a New Therapeutic Target in Breast Cancer. Nat Med (2011) 17:715–9. doi: 10.1038/nm.2351
83. Medunjanin S, Weinert S, Schmeisser A, Mayer D, Braun-Dullaeus RC. Interaction of the Double-Strand Break Repair Kinase DNA-PK and Estrogen Receptor-α. Mol Biol Cell (2010) 21:1620–8. doi: 10.1091/mbc.E09-08-0724
84. Williams CC, Basu A, El-Gharbawy A, Carrier LM, Smith CL, Rowan BG. Identification of Four Novel Phosphorylation Sites in Estrogen Receptor α: IImpact on Receptor-Dependent Gene Expression and Phosphorylation by Protein Kinase CK2. BMC Biochem (2009) 36:1–8. doi: 10.1186/1471-2091-10-36
85. Rajbhandari P, Schalper KA, Solodin NM, Ellison-Zelski SJ, Ping Lu K, Rimm DL, et al. Pin1 Modulates Erα Levels in Breast Cancer Through Inhibition of Phosphorylation-Dependent Ubiquitination and Degradation. Oncogene (2014) 33:1438–47. doi: 10.1038/onc.2013.78
86. Tang J, Luo Y, Long G, Zhou L. MINDY1 Promotes Breast Cancer Cell Proliferation by Stabilizing Estrogen Receptor α. Cell Death Dis (2021) 12:937. doi: 10.1038/s41419-021-04244-z
87. Tang J, Wu Z, Tian Z, Chen W, Wu G. OTUD7B Stabilizes Estrogen Receptor α and Promotes Breast Cancer Cell Proliferation. Cell Death Dis (2021) 12:534. doi: 10.1038/s41419-021-03785-7
88. Xia X, Liao Y, Huang C, Liu Y, He J, Shao Z, et al. Deubiquitination and Stabilization of Estrogen Receptor α by Ubiquitin-Specific Protease 7 Promotes Breast Tumorigenesis. Cancer Lett (2019) 465:118–28. doi: 10.1016/j.canlet.2019.09.003
89. Xia X, Huang C, Liao Y, Liu Y, He J, Shao Z, et al. The Deubiquitinating Enzyme USP15 Stabilizes ERα and Promotes Breast Cancer Progression. Cell Death Dis (2021) 12. doi: 10.1038/s41419-021-03607-w
90. Cao J, Wu D, Wu G, Wang Y, Ren T, Wang Y, et al. USP35, Regulated by Estrogen and AKT, Promotes Breast Tumorigenesis by Stabilizing and Enhancing Transcriptional Activity of Estrogen Receptor α. Cell Death Dis (2021) 12:619. doi: 10.1038/s41419-021-03904-4
91. Ding J, Kuang P. Regulation of ERα Stability and Estrogen Signaling in Breast Cancer by HOIL-1. Front Oncol (2021) 11:1–11. doi: 10.3389/fonc.2021.664689
92. Zhu J, Zhao C, Kharman-Biz A, Zhuang T, Jonsson P, Liang N, et al. The Atypical Ubiquitin Ligase RNF31 Stabilizes Estrogen Receptor α and Modulates Estrogen-Stimulated Breast Cancer Cell Proliferation. Oncogene (2014) 33:4340–51. doi: 10.1038/onc.2013.573
93. Zhu J, Li X, Su P, Xue M, Zang Y, Ding Y. The Ubiquitin Ligase RNF181 Stabilizes ERα and Modulates Breast Cancer Progression. Oncogene (2020) 39:6776–88. doi: 10.1038/s41388-020-01464-z
94. Zhuang T, Yu S, Zhang L, Yang H, Li X, Hou Y, et al. SHARPIN Stabilizes Estrogen Receptor a and Promotes Breast Cancer Cell Proliferation. Oncotarget (2017) 8:77137–51. doi: 10.18632/oncotarget.20368
95. Yang H, Yu N, Xu J, Ding X, Deng W, Wu G, et al. SMURF1 Facilitates Estrogen Receptor a Signaling in Breast Cancer Cells. J Exp Clin Cancer Res (2018) 37. doi: 10.1186/s13046-018-0672-z
96. Tang J, Luo Y, Tian Z, Liao X, Cui Q, Yang Q, et al. TRIM11 Promotes Breast Cancer Cell Proliferation by Stabilizing Estrogen Receptor α. Neoplasia (United States) (2020) 22:343–51. doi: 10.1016/j.neo.2020.06.003
97. Xue M, Zhang K, Mu K, Xu J, Yang H, Liu Y, et al. Regulation of Estrogen Signaling and Breast Cancer Proliferation by an Ubiquitin Ligase TRIM56. Oncogenesis (2019) 8. doi: 10.1038/s41389-019-0139-x
98. Caligiuri I, Toffoli G, Giordano A, Rizzolio F. pRb Controls Estrogen Receptor Alpha Protein Stability and Activity. Oncotarget (2013) 4:875–83. doi: 10.18632/oncotarget.1036
99. Wei X, Xu H, Kufe D. MUC1 Oncoprotein Stabilizes and Activates Estrogen Receptor α. Mol Cell (2006) 21:295–305. doi: 10.1016/j.molcel.2005.11.030
100. Yang H, Lv X, Li X, Mao L, Niu Z, Wang T, et al. ZNF213 Facilitates ER Alpha Signaling in Breast Cancer Cells. Front Oncol (2021) 11:638751. doi: 10.3389/fonc.2021.638751
101. Flach KD, Periyasamy M, Jadhav A, Dorjsuren D, Siefert JC, Hickey TE, et al. Endonuclease FEN1 Coregulates ERa Activity and Provides a Novel Drug Interface in Tamoxifen-Resistant Breast Cancer. Cancer Res (2020) 80:1914–26. doi: 10.1158/0008-5472.CAN-19-2207
102. Masaki T, Habara M, Sato Y, Goshima T, Maeda K, Hanaki S, et al. Calcineurin Regulates the Stability and Activity of Estrogen Receptor α. Proc Natl Acad Sci (2021) 118:e2114258118. doi: 10.1073/pnas.2114258118
103. Gallo D, Haddad I, Laurent G, Vinh J, Jacquemotte F, Jacquot Y, et al. Regulatory Function of the P295-T311 Motif of the Estrogen Receptor Alpha - Does Proteasomal Degradation of the Receptor Induce Emergence of Peptides Implicated in Estrogenic Responses? Nucl Recept Signal (2008) e007:1–8. doi: 10.1621/nrs.06007
104. Gallo D, Jacquot Y, Laurent G, Leclercq G. Calmodulin, a Regulatory Partner of the Estrogen Receptor Alpha in Breast Cancer Cells. Mol Cell Endocrinol (2008) 291:20–6. doi: 10.1016/j.mce.2008.04.011
105. Kudo N, Wolff B, Sekimoto T, Schreiner EP, Yoneda Y, Yanagida M, et al. Leptomycin B Inhibition of Signal-Mediated Nuclear Export by Direct Binding to CRM1. Exp Cell Res (1998) 242. doi: 10.1006/excr.1998.4136
106. Tian Z, Tang J, Liao X, Gong Y, Yang Q, Wu Y, et al. TRIM8 Inhibits Breast Cancer Proliferation by Regulating Estrogen Signaling. Am J Cancer Res (2020) 10(10):3440–57.
107. Bouhoute A, Lecleroq G. Antagonistic Effect of Triphenylethylenic Antiestrogens on the Association of Estrogen Receptor to Calmodulin. Biochem Biophys Res Commun (1992) 184:1432–40. doi: 10.1016/S0006-291X(05)80043-9
108. Li L, Li Z, Sacks DB. The Transcriptional Activity of Estrogen Receptor-α is Dependent on Ca2+/calmodulin. J Biol Chem (2005) 280:13097–104. doi: 10.1074/jbc.M410642200
109. Gallo D, Jacquemotte F, Cleeren A, Laïos I, Hadiy S, Rowlands MG, et al. Calmodulin-Independent, Agonistic Properties of a Peptide Containing the Calmodulin Binding Site of Estrogen Receptor α. Mol Cell Endocrinol (2007) 268:37–49. doi: 10.1016/j.mce.2007.01.012
110. García Pedrero JM, del Rio B, Martínez-Campa C, Muramatsu M, Lazo PS, Ramos S. Calmodulin Is a Selective Modulator of Estrogen Receptors. Mol Endocrinol (2002) 16:947–60. doi: 10.1210/mend.16.5.0830
111. Li Z, Joyal JL, Sacks DB. Calmodulin Enhances the Stability of the Estrogen Receptor. J Biol Chem (2001) 276:17354–60. doi: 10.1074/jbc.M010238200
112. Li L, Li Z, Howley PM, Sacks DB. E6AP and Calmodulin Reciprocally Regulate Estrogen Receptor Stability. J Biol Chem (2006) 281:1978–85. doi: 10.1074/jbc.M508545200
113. Subramanian K, Jia D, Kapoor-Vazirani P, Powell DR, Collins RE, Sharma D, et al. Regulation of Estrogen Receptor α by the SET7 Lysine Methyltransferase. Mol Cell (2008) 30:336–47. doi: 10.1016/j.molcel.2008.03.022
114. la Rosa P, Pesiri V, Leclercq G, Marino M, Acconcia F. Palmitoylation Regulates 17β-Estradiol-Induced Estrogen Receptor-α Degradation and Transcriptional Activity. Mol Endocrinol (2012) 26:762–74. doi: 10.1210/me.2011-1208
115. Shin EM, Huynh VT, Neja SA, Liu CY, Raju A, Tan K, et al. GREB1: An Evolutionarily Conserved Protein With a Glycosyltransferase Domain Links Erα Glycosylation and Stability to Cancer. Sci Adv (2021) eabe2470:1–8. doi: 10.1126/sciadv.abe2470
116. Gorgogietas VA, Tsialtas I, Sotiriou N, Laschou VC, Karra AG, Leonidas DD, et al. Potential Interference of Aluminum Chlorohydrate With Estrogen Receptor Signaling in Breast Cancer Cells. J Mol Biochem (2018) 7:1–13.
117. Liu H, Qiu J, Li N, Chen T, Cao X. Human Phosphatidylethanolamine-Binding Protein 4 Promotes Transactivation of Estrogen Receptor α (Erα) in Human Cancer Cells by Inhibiting Proteasome-Dependent Erα Degradation via Association With Src. J Biol Chem (2010) 285:21934–42. doi: 10.1074/jbc.M110.109876
118. Alarid ET, Bakopoulos N, Solodin N. Proteasome-Mediated Proteolysis of Estrogen Receptor: A Novel Component in Autologous Down-Regulation. Mol Endocrinol (1999) 13:1522–34. doi: 10.1210/mend.13.9.0337
119. Preisler-Mashek MT, Solodin N, Stark BL, Tyriver MK, Alarid ET. Ligand-Specific Regulation of Proteasome-Mediated Proteolysis of Estrogen Receptor-α. Am J Physiol Endocrinol Metab (2002) 282:E891–8. doi: 10.1152/ajpendo.00353.2001
120. Valley CC, Solodin NM, Powers GL, Ellison SJ, Alarid ET. Temporal Variation in Estrogen Receptor-α Protein Turnover in the Presence of Estrogen. J Mol Endocrinol (2008) 40:23–34. doi: 10.1677/JME-07-0067
121. Fowler AM, Solodin N, Preisler-Mashek MT, Zhang P, Lee AV, Alarid ET. Increases in Estrogen Receptor-α Concentration in Breast Cancer Cells Promote Serine 118/104/106-Independent AF-1 Transactivation and Growth in the Absence of Estrogen. FASEB J (2004) 18:81–93. doi: 10.1096/fj.03-0038com
122. Fowler AM, Solodin NM, Valley CC, Alarid ET. Altered Target Gene Regulation Controlled by Estrogen Receptor-α Concentration. Mol Endocrinol (2006) 20:291–301. doi: 10.1210/me.2005-0288
123. Musgrove EA. Estrogen Receptor Degradation: A CUE for Endocrine Resistance? Breast Cancer Res (2011) 312:1–2. doi: 10.1186/bcr2914
124. Pan X, Zhou T, Tai YH, Wang C, Zhao J, Cao Y, et al. Elevated Expression of CUEDC2 Protein Confers Endocrine Resistance in Breast Cancer. Nat Med (2011) 17:708–14. doi: 10.1038/nm.2369
125. Lehn S, Fernö M, Jirström K, Rydén L, Landberg G. A non-Functional Retinoblastoma Tumor Suppressor (RB) Pathway in Premenopausal Breast Cancer is Associated With Resistance to Tamoxifen. Cell Cycle (2011) 10:956–62. doi: 10.4161/cc.10.6.15074
126. Treré D, Brighenti E, Donati G, Ceccarelli C, Santini D, Taffurelli M, et al. High Prevalence of Retinoblastoma Protein Loss in Triple-Negative Breast Cancers and its Association With a Good Prognosis in Patients Treated With Adjuvant Chemotherapy. Ann Oncol (2009) 20:1818–23. doi: 10.1093/annonc/mdp209
127. Escobar-Hoyos LF, Shah R, Roa-Peña L, Vanner EA, Najafian N, Banach A, et al. Keratin-17 Promotes P27kip1 Nuclear Export and Degradation and Offers Potential Prognostic Utility. Cancer Res (2015) 75:3650–62. doi: 10.1158/0008-5472.CAN-15-0293
128. Garner F, Shomali M, Paquin D, Lyttle CR, Hattersley G. RAD1901: A Novel, Orally Bioavailable Selective Estrogen Receptor Degrader That Demonstrates Antitumor Activity in Breast Cancer Xenograft Models. Anticancer Drugs (2015) 26:948–56. doi: 10.1097/CAD.0000000000000271
129. Wardell SE, Nelson ER, Chao CA, McDonnell DP. Bazedoxifene Exhibits Antiestrogenic Activity in Animal Models of Tamoxifen-Resistant Breast Cancer: Implications for Treatment of Advanced Disease. Clin Cancer Res (2013) 19:2420–31. doi: 10.1158/1078-0432.CCR-12-3771
130. Guo S, Zhang C, Bratton M, Mottamal M, Liu J, Ma P, et al. ZB716, a Steroidal Selective Estrogen Receptor Degrader (SERD), is Orally Efficacious in Blocking Tumor Growth in Mouse Xenograft Models. Oncotarget (2018) 9:6924–37. doi: 10.18632/oncotarget.24023
131. Lai A, Kahraman M, Govek S, Nagasawa J, Bonnefous C, Julien J, et al. Identification of GDC-0810 (ARN-810), an Orally Bioavailable Selective Estrogen Receptor Degrader (SERD) That Demonstrates Robust Activity in Tamoxifen-Resistant Breast Cancer Xenografts. J Med Chem (2015) 58:4888–904. doi: 10.1021/acs.jmedchem.5b00054
132. Tria GS, Abrams T, Baird J, Burks HE, Firestone B, Gaither LA, et al. Discovery of LSZ102, a Potent, Orally Bioavailable Selective Estrogen Receptor Degrader (SERD) for the Treatment of Estrogen Receptor Positive Breast Cancer. J Med Chem (2018) 61:2837–64. doi: 10.1021/acs.jmedchem.7b01682
133. Deeks ED. Fulvestrant: A Review in Advanced Breast Cancer Not Previously Treated With Endocrine Therapy. Drugs (2018) 78:131–7. doi: 10.1007/s40265-017-0855-5
134. Britschgi A, Duss S, Kim S, Couto JP, Brinkhaus H, Koren S, et al. The Hippo Kinases LATS1 and 2 Control Human Breast Cell Fate via Crosstalk With Erα. Nature (2017) 541:541–5. doi: 10.1038/nature20829
135. Reinert T, Gonçalves R, Bines J. Implications of ESR1 Mutations in Hormone Receptor-Positive Breast Cancer. Curr Treat Options Oncol (2018) 24:1–10. doi: 10.1007/s11864-018-0542-0
136. Toy W, Weir H, Razavi P, Lawson M, Goeppert AU, Mazzola AM, et al. Activating ESR1 Mutations Differentially Affect the Efficacy of ER Antagonists. Cancer Discov (2017) 7:277–87. doi: 10.1158/2159-8290.CD-15-1523
137. Angus L, Beije N, Jager A, Martens JWM, Sleijfer S. ESR1 Mutations: Moving Towards Guiding Treatment Decision-Making in Metastatic Breast Cancer Patients. Cancer Treat Rev (2017) 52:33–40. doi: 10.1016/j.ctrv.2016.11.001
138. Bahreini A, Li Z, Wang P, Levine KM, Tasdemir N, Cao L, et al. Mutation Site and Context Dependent Effects of ESR1 Mutation in Genome-Edited Breast Cancer Cell Models. Breast Cancer Res (2017) 60:1–10. doi: 10.1186/s13058-017-0851-4
139. Jeselsohn R, Bergholz JS, Pun M, Cornwell M, Liu W, Nardone A, et al. Allele-Specific Chromatin Recruitment and Therapeutic Vulnerabilities of ESR1 Activating Mutations. Cancer Cell (2018) 33:173–86.e5. doi: 10.1016/j.ccell.2018.01.004
140. Chu I, Arnaout A, Loiseau S, Sun J, Seth A, McMahon C, et al. Src Promotes Estrogen-Dependent Estrogen Receptor α Proteolysis in Human Breast Cancer. J Clin Invest (2007) 117:2205–15. doi: 10.1172/JCI21739
141. Martin LA, Ribas R, Simigdala N, Schuster E, Pancholi S, Tenev T, et al. Discovery of Naturally Occurring ESR1 Mutations in Breast Cancer Cell Lines Modelling Endocrine Resistance. Nat Commun (2017) 1865:1–15. doi: 10.1038/s41467-017-01864-y
142. Sreekumar S, Levine KM, Sikora MJ, Chen J, Tasdemir N, Carter D, et al. Differential Regulation and Targeting of Estrogen Receptor α Turnover in Invasive Lobular Breast Carcinoma. Endocrinology (United States) (2020) 9:1–14. doi: 10.1210/endocr/bqaa109
143. Zhao Y, Zhao C, Lu J, Wu J, Li C, Hu Z, et al. Sesterterpene MHO7 Suppresses Breast Cancer Cells as a Novel Estrogen Receptor Degrader. Pharmacol Res (2019) 104294:1–12. doi: 10.1016/j.phrs.2019.104294
144. Totta P, Pesiri V, Marino M, Acconcia F. Lysosomal Function is Involved in 17β-Estradiol-Induced Estrogen Receptor α Degradation and Cell Proliferation. PloS One (2014) e94880:1–10. doi: 10.1371/journal.pone.0094880
145. Totta P, Busonero C, Leone S, Marino M, Acconcia F. Dynamin II is Required for 17β-Estradiol Signaling and Autophagy-Based Erα Degradation. Sci Rep (2016) 23727:1–10. doi: 10.1038/srep23727
146. Totta P, Gionfra F, Busonero C, Acconcia F. Modulation of 17β-Estradiol Signaling on Cellular Proliferation by Caveolin-2. J Cell Physiol (2016) 231:1219–25. doi: 10.1002/jcp.25218
147. Totta P, Pesiri V, Enari M, Marino M, Acconcia F. Clathrin Heavy Chain Interacts With Estrogen Receptor α and Modulates 17β-Estradiol Signaling. Mol Endocrinol (2015) 29:739–55. doi: 10.1210/me.2014-1385
148. Arnold SF, Vorojeikina DP, Notides AC. Phosphorylation of Tyrosine 537 on the Human Estrogen Receptor is Required for Binding to an Estrogen Response Element. J Biol Chem (1995) 270:30205–12. doi: 10.1074/jbc.270.50.30205
149. Kim SH, Kang HJ, Na H, Lee MO. Trichostatin A Enhances Acetylation as Well as Protein Stability of Erα Through Induction of P300 Protein. Breast Cancer Res (2010) R22:1–8. doi: 10.1186/bcr2562
150. Fuqua SA, Wiltschke C, Zhang QX, Borg A, Castles CG, Friedrichs WE, et al. A Hypersensitive Estrogen Receptor-Alpha Mutation in Premalignant Breast Lesions. Cancer Res (2000) 60:4026–9.
151. Herynk MH, Parra I, Cui Y, Beyer A, Wu MF, Hilsenbeck SG, et al. Association Between the Estrogen Receptor α A908G Mutation and Outcomes in Invasive Breast Cancer. Clin Cancer Res (2007) 13:3235–43. doi: 10.1158/1078-0432.CCR-06-2608
152. Jiang Y, Deng Q, Zhao H, Xie M, Chen L, Yin F, et al. Development of Stabilized Peptide-Based PROTACs Against Estrogen Receptor α. ACS Chem Biol (2018) 13:628–35. doi: 10.1021/acschembio.7b00985
Keywords: estrogen receptor alpha, breast cancer, ERα stability, ERα nucleo-cytoplasmic transport, endocrine resistance, therapeutic approaches
Citation: Tecalco-Cruz AC, Macías-Silva M, Ramírez-Jarquín JO and Ramírez-Jarquín UN (2022) Decoding the Therapeutic Implications of the ERα Stability and Subcellular Distribution in Breast Cancer. Front. Endocrinol. 13:867448. doi: 10.3389/fendo.2022.867448
Received: 01 February 2022; Accepted: 03 March 2022;
Published: 13 April 2022.
Edited by:
Leclercq Guy, Université libre de Bruxelles, BelgiumReviewed by:
Muriel Le Romancer, INSERM U1052 Centre de Recherche en Cancerologie de Lyon, FranceCopyright © 2022 Tecalco-Cruz, Macías-Silva, Ramírez-Jarquín and Ramírez-Jarquín. This is an open-access article distributed under the terms of the Creative Commons Attribution License (CC BY). The use, distribution or reproduction in other forums is permitted, provided the original author(s) and the copyright owner(s) are credited and that the original publication in this journal is cited, in accordance with accepted academic practice. No use, distribution or reproduction is permitted which does not comply with these terms.
*Correspondence: Angeles C. Tecalco-Cruz, YW5nZWxlcy50ZWNhbGNvQHVhY20uZWR1Lm14; Marina Macías-Silva, bW1hY2lhc3NAaWZjLnVuYW0ubXg=