- 1Key Laboratory of Sustainable Development of Marine Fisheries, Ministry of Agriculture and Rural Affairs, Yellow Sea Fisheries Research Institute, Chinese Academy of Fishery Sciences, Qingdao, China
- 2Laboratory for Marine Fisheries and Food Production Processes, Pilot National Laboratory for Marine Science and Technology (Qingdao), Qingdao, China
- 3Instituto de Investigaciones en Biodiversidad y Biotecnología (INBIOTEC-CONICET), Mar del Plata, Argentina
- 4Fundación para Investigaciones Biológicas Aplicadas (FIBA), Mar del Plata, Argentina
- 5Instituto Tecnológico de Chascomús (CONICET-UNSAM), Chascomús, Argentina
In the last two decades, kisspeptin (Kiss) has been identified as an important player in the regulation of reproduction and other physiological functions in vertebrates, including several fish species. To date, two ligands (Kiss1, Kiss2) and three kisspeptin receptors (Kissr1, Kissr2, Kissr3) have been identified in teleosts, likely due to whole-genome duplication and loss of genes that occurred early in teleost evolution. Recent results in zebrafish and medaka mutants have challenged the notion that the kisspeptin system is essential for reproduction in fish, in marked contrast to the situation in mammals. In this context, this review focuses on the role of kisspeptins at three levels of the reproductive, brain-pituitary-gonadal (BPG) axis in fish. In addition, this review compiled information on factors controlling the Kiss/Kissr system, such as photoperiod, temperature, nutritional status, sex steroids, neuropeptides, and others. In this article, we summarize the available information on the molecular diversity and evolution, tissue expression and neuroanatomical distribution, functional significance, signaling pathways, and gene regulation of Kiss and Kissr in teleost fishes. Of particular note are recent advances in understanding flatfish kisspeptin systems, which require further study to reveal their structural and functional diversity.
Introduction
Eighteen years have passed since the first paper on kisspeptin in a teleost species was published. In that study, the complementary DNA (cDNA) of a kisspeptin receptor (referred to then as GPR54 and now as kissr2) was isolated in the Nile tilapia, Oreochromis niloticus (1). The interest in studying the kisspeptin system in fish came from its key role in mammalian reproduction (2–4). A clear example of this is that more than 250 papers have been published to date on kisspeptin and kisspeptin receptors in teleosts, as shown in the Scopus database.
It is now generally accepted that the kisspeptin system in most teleost species consists of two ligands, known as Kiss1 and Kiss2, and two receptors, Kissr2 and Kissr3. However, only one element of this system (either the ligand and/or the receptor) has been detected in Pleuronectiformes, such as kissr2 in Atlantic halibut, Hippoglossus hippoglossus (5); both kiss2 and kissr2 in the Senegalese sole, Solea senegalensis (6, 7), half-smooth tongue sole, Cynoglossus semilaevis (8), and Japanese flounder, Paralichthys olivaceus (9). Of note, the European eel, Anguilla anguilla, is the only teleost species having two kiss genes (kiss1 and kiss2) and three kissr types (kissr1, kissr2, and kissr3) reported to date (10).
In recent years, a considerable number of studies have suggested that the kisspeptin system is the “master system” controlling the BPG axis in mammals by exerting its action on gonadotropin-releasing hormone (Gnrh) neurons (3, 11, 12). However, in fish, several studies have considered the Gnrh system as the main system and, the kisspeptin system as a complementary system in controlling fish reproduction (13–15). First, the anatomical association of kisspeptin and Gnrh neurons is not obvious, or almost absent in many teleost species (16–21). Similarly, in zebrafish (Danio rerio) and medaka (Oryzias latipes) kiss and/or kissr knockouts display normal reproduction (20, 22, 23). However, it must be considered that, surprisingly, similar results have been obtained with gnrh knockouts because, at least in zebrafish, single gnrh3 mutants and gnrh3 plus 2 kiss gene triple mutants can normally reproduce (23–26). However, in the same species, laser ablation of Gnrh cells at the larval stage resulted in the loss of reproduction in adult fish (27), suggesting that the cellular integrity of Gnrh cells is essential and the Gnrh system is a key and essential player for normal reproduction. Then, it was suggested that the unaltered normal reproductive capacity of mutant fish is compensated by the action of other neuropeptides known to affect gonadotropin secretion (15, 24, 25). In this context, it would be interesting to investigate whether similar mechanisms occur in other teleost species and to clearly determine which peptide(s) are involved in these compensatory mechanisms.
The aim of this review is to examine the entire literature on the kisspeptin system in teleost fishes, with particular emphasis on diversity and evolution, central and peripheral distribution, physiological effects on reproduction, intracellular signaling pathways and regulatory mechanisms.
Kisspeptin Genes and Peptides
Kisspeptins were initially considered to be members of the RFamide peptide family (10). However, other studies demonstrated that kisspeptins are far from the RF-amide family and were proposed to be members of the Kisspeptin/Galanin/Spexin family (28). Given the low conservation of kisspeptin ligands among fish species, their characterization took longer compared to kisspeptin receptors (7). The first Kiss1 orthologs in fish to be characterized were those of zebrafish, spotted pufferfish (Tetraodon nigroviridis), Japanese pufferfish (Fugu rubripes) and medaka (29, 30). Shortly after, Kiss2 was characterized in zebrafish and medaka (31).
In humans, Kiss1 prepropeptide consists of 145 amino acids (aa) in length, with a major cleavage product of 54 aa (originally named as metastin) and three shorter peptides of 14, 13 and 10 amino acids in length. All these peptides bind to their cognate G protein-coupled receptor today known as kisspeptin receptor (32). It was then demonstrated that the 10 aa peptide was conserved across vertebrates (33), suggesting that it plays an important role in different taxa (34). However, the situation is not as conserved in teleosts. For example, the Kiss1 precursor contains a conserved putative cleavage site six amino acids upstream of the core sequence, suggesting that the mature form of Kiss1 is a pentadecapeptide (17, 35, 36). In addition, the kiss2 gene produces a mature dodecapeptide in several species (17, 34–36). Moreover, several studies have shown that Kiss1-15 and Kiss2-12 peptides are more effective than Kiss1/2-10 for receptor activation in teleosts (34).
Kisspeptin Receptor Genes and Proteins
Kisspeptin receptors are membrane receptors that belong to the superfamily of G protein-coupled receptors (GPCRs) (37). These receptors have a highly conserved structure of seven transmembrane domains (TMDs) that has facilitated their cloning and characterization in vertebrates, including teleosts. As mentioned earlier, the first kissr to be characterized in fish was found in the Nile tilapia (1). Soon after, several kissr2 were characterized in other fish species, such as cobia (Rachycentron canadum) (38), grey mullet (Mugil cephalus) (39), fathead minnow (Pimephales promelas) (40) and two flatfish species, Senegalese Sole (6) and Atlantic halibut (5). The zebrafish genome then helped Biran and coworkers (41) to identify for the first time two kisspeptin receptors in a fish species, then named kiss1ra and kiss1rb and now known as kissr2 and kissr3, according to the nomenclature introduced by Pasquier et al. (10). Since then, two kisspeptin receptors have been discovered in most teleost fish species studied (15, 42, 43). However, not all teleost species have two kisspeptin receptors. For example, only kissr2 has been found in the three-spined stickleback (Gasterosteus aculeatus), fugu (Takifugu niphobles), and spotted pufferfish (44). Mechaly and coworkers (5, 6) also failed to detect kissr3 by PCR in Pleuronectiformes and suggested that kissr3 may have been lost during evolution of this order (45).
Evolution of the Kisspeptinergic Systems in Fish
The first phylogenetic studies on the kisspeptin system genes were essentially obtained from cloned and characterized sequences (1, 5–7, 31, 33, 46). However, advances in next-generation sequencing (NGS) technologies and genomics have made available dozens of transcriptomes and genomes from a wide range of teleosts and allowed to re-examine the diversity, origin, and evolution of kisspeptins and their receptors not only in fish but also in other vertebrates (10, 44, 47). For example, sequences related to kisspeptin have been identified from genome databases in the European eel (44) and pejerrey fish, Odontesthes bonariensis (36), among others.
In teleosts, several studies have performed phylogenetic studies on the nucleotide and/or amino acid sequences of kisspeptins and kisspeptin receptors (10, 44). Since the appearance of this large dataset, more complete phylogenetic studies on the evolution of the kisspeptin systems have been conducted. The situation is similar for flatfishes, and the currently available genomes and/or transcriptomes from half-smooth tongue sole (48), turbot, Scophthalmus maximus (49), Senegalese sole (50, 51), common sole, Solea solea (50, 51), Japanese flounder (52), black flounder, Paralichthys orbignyanus (53), and more recently the available genomes of 11 flatfish species, representing 9 pleuronectiform families (54) have added data to understand kisspeptin phylogeny in teleosts. A summary of all the available information is shown in Figure 1A.
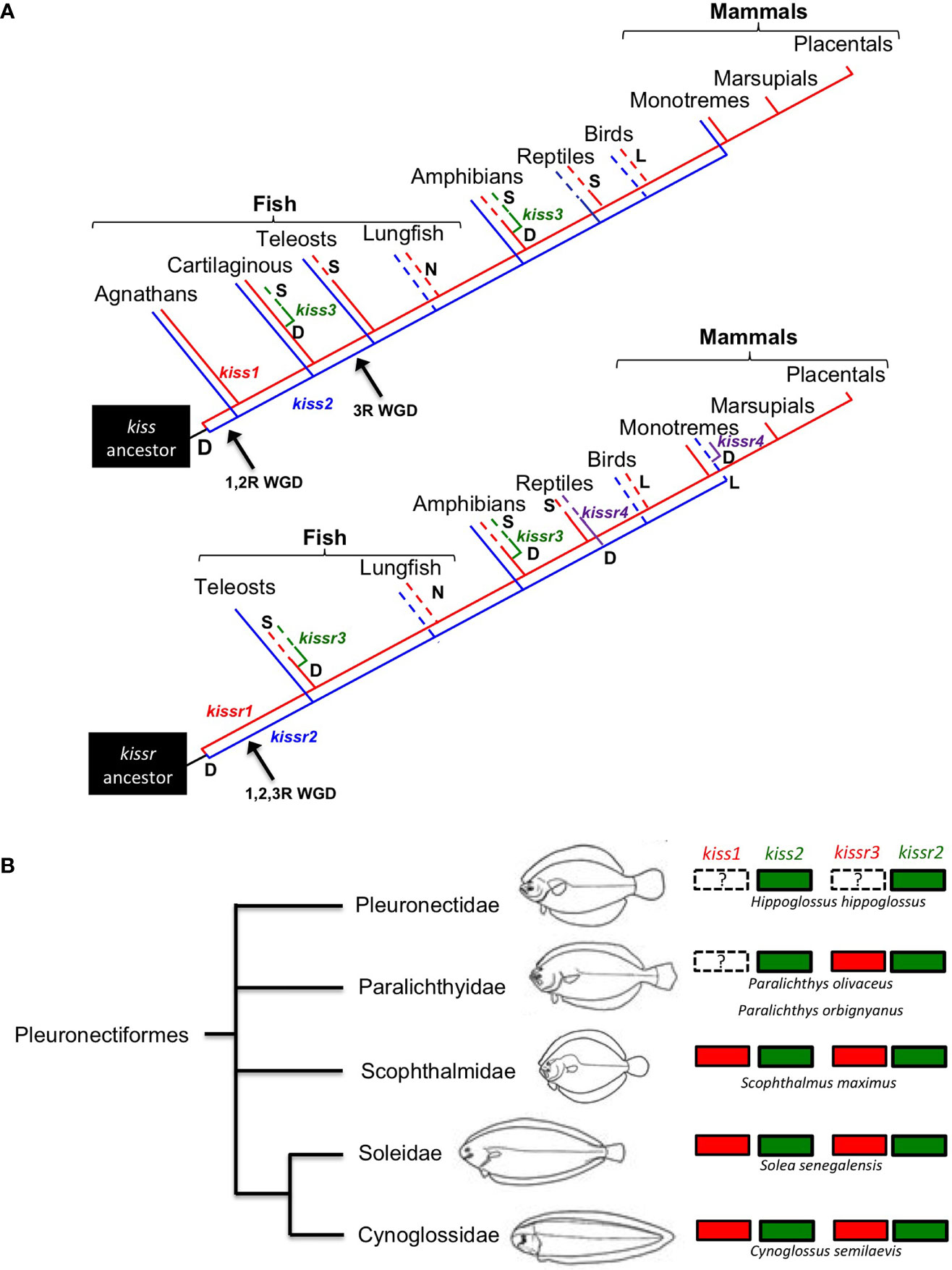
Figure 1 (A) kiss and kissr gene evolution in vertebrates derived from available information. D = gene duplication, L = gene lost, S = gene lost in some species, N = gene not searched. (Modified from 7). (B) Consensus tree of flatfish relationships proposed by Chapleau (55), figure modified from Chanet et al. (56).
Within this framework, another aim of the review is to highlight some relevant aspects of the kisspeptinergic system in flatfishes, as conflicting information on the presence of kisspeptin genes has been reported in this group. One example is the absence of kiss1 and kissr3, as suggested by Mechaly et al. (45) for the Senegalese sole and Atlantic halibut. However, using the current genomic information of both species, we found either complete or partial kissr3 sequences in Senegalese sole (Figure 1B). However, we could not find kiss1 sequences in the black and Japanese flounders. Both genomes have high sequencing coverage and identified 25,231 protein-coding genes in black flounder (53) and 21,787 protein-coding genes in the case of Japanese flounder (52). However, the absence of kiss1 annotation in flounder genomes does not necessarily mean that this gene is missing in these species, as “missing” genes can often occur in unassembled reads or contigs (57).
To make a definitive conclusion about the Kiss1 situation, PCR analysis must be performed in both cases. However, it must be kept in mind that this does not guarantee the detection of the gene, as has already been shown in Senegalese sole and Atlantic halibut (5–7). To test whether kiss1 and/or kissr3 have been lost in some pleuronectiform species, a more comprehensive comparative sequence analysis needs to be performed. With this in mind, a syntenic analysis of kiss and kissr neighboring genes in Pleuronectiformes was performed (Figure 2). It is always possible that mutations and/or translocations may have occurred to explain the absence of a particular gene in the genome. For example, a kiss1-like transcript was already found in the red seabream, Pagrus major (58). A similar situation has also been observed in primates, where a Kiss2-like gene was in human, chimpanzee, and gorilla genome databases (59).
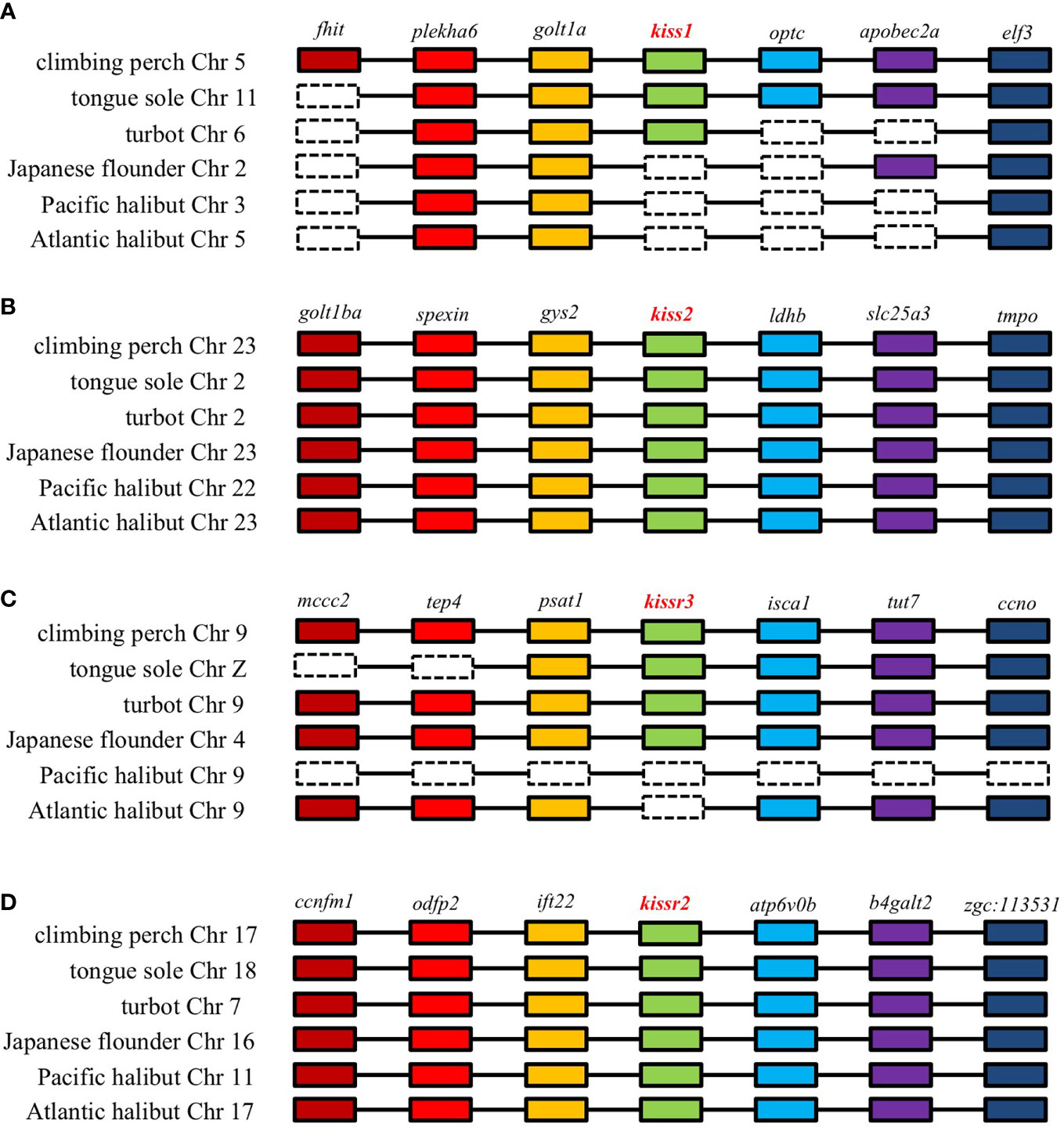
Figure 2 Genomic synteny analysis of kisspeptin (A, B) and its receptor (C, D) genes in different teleost species.
Tissue Expression and Neuroanatomical Distribution of the Kiss/Kissr Systems in Fish
While studies in mammals have shown that kisspeptin has pleiotropic effects (60, 61), the situation in teleosts has not been studied in detail, as most of the studies have focused on the central regulation of reproduction (15, 42, 45). However, kisspeptin transcripts and proteins are widely distributed in various brain areas and tissues of fish, but no clear roles have been associated with these extra brain kisspeptins. These tissues/organs, include the pituitary gland, the spinal cord, the intestine, the gonads, and the liver (62–65). The presence of kisspeptin in blood has been detected in mammals (66). However, to the best of our knowledge there are no available data on kisspeptins levels in fish plasma. This is also the case in flatfishes, where kisspeptin elements have been detected in various tissues, organs and brain areas. As mentioned earlier, only kiss2 and kissr2 have been described in all flatfishes studied to date (45). However, to the best of our knowledge, no functions have been assigned to Kiss2 in these tissues/organs. With the genomes available today this situation has changed, as kiss1 and kissr3 sequences have been found in several pleuronectiform species, but no functional studies of kiss1-kissr3 tissue expression have been performed to date. In this context, the advent of RNA sequencing technology (RNA-seq) will allow us to clarify this situation. However, future studies are needed to clarify the pleiotropic role of kisspeptins not only in Pleuronectiforms but also in other teleost species.
It is important to mention that alternative splice variants are frequently observed in mammals when analyzing GPCR tissue distribution (67), and spliced variants of kisspeptin have also been detected in several teleosts. For example, the presence of alternative splice variants for kissr2 and/or kissr3 was observed in Senegalese sole (6, 45), southern bluefin tuna, Thunnus maccoyii (68), yellowtail kingfish, Seriola lalandi (68), zebrafish (69), European eel (44) and pejerrey fish (65) through intron retention. Mechaly et al. (6, 45, 65) indicate that truncated and likely non-functional proteins are produced in this manner. In two species, yellowtail kingfish (68) and zebrafish (69) alternative spliced variants were also generated by deletion of exons. For a detailed description of the splicing events detected in kisspeptin receptors in fish see Mechaly et al. (45, 65). In this regards, future studies are needed to evaluate whether truncated proteins are generated and whether this represents regulation by unproductive splicing, as has been described for arginine-serine-rich (SR) splicing factors in several organisms (70). To the best of our knowledge, alternative spliced variants have been detected in a kisspeptin ligand in the specific case of Pleuronectiformes and identified only in the kiss2 gene of Senegalese sole (7).
Functional Significance of the Kiss/Kissr System in Fish
In fishes, the functional roles of kisspeptinergic systems in regulating reproduction is not always clear and is sometimes contradictory. For example, Kiss1 has been shown to significantly increase the levels of messengers of luteinizing hormone b subunit (lhb), growth hormone (gh) and prolactin (prl) in goldfish (Carassius auratus) pituitary cells (71). However, in European eel, four different kisspeptin analogues (Kiss1-10, Kiss1-15, Kiss2-10 and Kiss2-12) are able to specifically inhibit lhb expression in a dose-dependent manner without affecting follicle-stimulating hormone b (fshb) mRNA levels when acting on pituitary cells (72). But, as mentioned earlier, kiss/kissr mutated fish showed almost normal fertility and gonadal maturation, suggesting that kisspeptin systems are not strictly required for reproduction, at least in some teleosts (20, 22) or compensatory mechanisms may take over the role of kisspeptins in reproduction (20, 22, 43, 73, 74).
Moreover, connection of kisspeptin nerve terminals and Gnrh cells is not really clear in all teleost species. For example, in the Nile tilapia, a kisspeptin receptor has been shown to be expressed in Ghrh cells (1), and a small number of Gnrh neurons receive kisspeptin innervation in zebrafish (16, 21), striped bass (Morone saxatilis) (17) and the cichlid Astatotilapia burtoni (75). Conversely, in medaka (19) and European sea bass (Dicentrarchus labrax) (18), the presence of kisspeptin receptors on Gnrh neurons could not be detected. However, in zebrafish, Kiss2 nerve terminals reach the pituitary gland (76) and Kiss2 cell bodies and fiber-like projections are found in the proximal pars distalis (PPD) with a distribution like Gnrh3 nerve terminals (77), supporting the possibility of an intrapituitary kisspeptinergic regulation of pituitary function. Thus, the physiological significance and functions of kisspeptin in fish reproduction remain controversial. Table 1 summarizes the physiological effects of kisspeptins in teleosts fish.
Kisspeptins’ Actions at Brain Level
The biological effects of kisspeptin on Gnrh neurons have been demonstrated in several teleost species at different levels. Kiss1 stimulates the electrical activity of terminal nerve-Gnrh3 neurons in adult medaka (97). Kiss1 also stimulates the electrical activity of the preoptic area (POA) and hypothalamic Gnrh3 neurons in adult zebrafish, while Kiss2 inhibits their neuronal activity (98). In the orange-spotted grouper, Epinephelus coioides, intraperitoneal (ip) injection of Kiss2 leads to upregulation of hypothalamic expression of gnrh1 (91). Similarly, Kiss2, but not Kiss1, significantly stimulates gnrh1 expression in striped bass brain slices (82). Stimulatory effects of Kiss2 on gnrh1 expression in the brain and hypothalamus are also observed in the black porgy, Acanthopagrus schlegelii (99), Nile tilapia (92), and Japanese flounder (100). In hybrid bass, a differential and gonadal stage-dependent role of kisspeptins on gnrh1 expression in the brain was observed; both Kiss1 and Kiss2 increase gnrh1 expression in pre-pubertal fish, while Kiss2 reduces gnrh1 expression in gonadal recrudescencing fish (17).
Chronic administration of Kiss1 and Kiss2 leads to a decrease in gnrh1, gnrh2, and/or gnrh3 transcript levels in the brain of female striped bass (81). An inhibitory effect of Kiss1 and Kiss2 on gnrh1 and gnrh2 expression in the forebrain and midbrain is also found in male European sea bass (83). On the other hand, Kiss2 does not alter gnrh2 and gnrh3 mRNA expression in the hypothalamus of the half-smooth tongue sole in vitro (89). Likewise, injection with Kiss1 and/or Kiss2 peptides induce no significant differences in gnrh mRNA levels in other teleosts, such as zebrafish brain gnrh2 and gnrh3 (31), hybrid bass brain gnrh2 and gnrh3 (17), orange-spotted grouper hypothalamic gnrh3 (91), yellowtail kingfish brain and hypothalamic gnrh1 (88), lined seahorse hypothalamic gnrh3 (93), European sea bass hypothalamic gnrh1 and forebrain-midbrain gnrh3 (83). However, Kiss2 has both stimulatory and inhibitory effects on gnrh1 mRNA levels in the brain of female chub mackerel (Scomber japonicus), depending on the mode of administration. Subcutaneous and slow release of Kiss2 increases gnrh1 expression (86), whereas intracerebroventricular (icv) administration of Kiss2 suppresses gnrh1 expression (87). Taken together, these data suggest that the mode of actions of Kiss1 and Kiss2 on Gnrh neurons are different among fish species and depend not only on gonadal status but also on the way of administration.
On the other hand, the LPXRFa system (the piscine ortholog of gonadotropin-inhibitory hormone, Gnih) is also a target for the central effects of kisspeptin in fish. In hypothalamic explants of half-smooth tongue sole, Kiss2 exerts a stimulatory effect on lpxra transcript levels, while apparently reducing lpxrfa-r mRNA levels (89). To our knowledge, this is the first evidence for the involvement of kisspeptin in the LPXRFa system in any fish species investigated so far. In addition, autoregulation of the kisspeptin system has been observed in several teleosts. For details, see the section on neuropeptides (see below).
Kisspeptins´ Actions on the Pituitary
The physiological roles of both Kiss1 and Kiss2 do not appear to follow a common pattern in teleosts. Previous in vitro studies indicate a direct stimulatory effect of kisspeptin on gonadotropins in different species. For example, Kiss1 significantly triggers Lh release from primary pituitary cell cultures of goldfish (71, 101), and Kiss2 has a stimulatory effect on both Lh and Fsh release in pituitary cells of European sea bass (84). In striped bass, both Kiss1 and Kiss2 stimulate Fsh release in vitro, whereas only Kiss2 is able to exert a stimulatory effect on Lh release (82). Moreover, ip injection of Kiss1, but not Kiss2, significantly increases serum Lh levels in goldfish (33). An increase in plasma Lh and Fsh levels is observed in European sea bass after injection of both Kiss1 and Kiss2 (46, 83). However, Kiss2 is more effective than Kiss1 in triggering gonadotropin secretion in this species (46). Similarly, intramuscular injection of Kiss2 stimulates secretion of Fsh and Lh in Senegalese sole of both sexes (94). Moreover, a differential and gonadal stage-dependent roles of kisspeptin on Lh release was observed in hybrid bass: Kiss1 increases plasma LH levels during gonadal recrudescence in vivo, whereas Kiss2 stimulates the release of LH during at pre-puberty and gonadal recrudescence (17).
In addition, injection of Kiss2 triggers an increase in pituitary fshb and lhb mRNA expression in zebrafish (31) and chub mackerel (87). Treatment of orange-spotted grouper with Kiss2 results in an increase in fshb mRNA abundance in vivo (91). Moreover, half-smooth tongue sole Kiss2 apparently induces an increase in gtha and fshb mRNA levels, without affecting lhb mRNA transcripts in vitro (90). In zebrafish, Kiss2 significantly stimulates fshb and lhb expression in the female pituitary gland in vitro (77).
However, other teleost studies have reported some inhibitory effects of kisspeptins on gonadotropins. For example, chronic treatment with Kiss2 results in a decrease in plasma Lh and Fsh levels in vivo in striped bass (81). Both heterologous and homologous kisspeptin peptides inhibit lhb mRNA levels in vitro, without affecting fshb expression in European eel (72, 102). An inhibitory effect of Kiss1 on lhb expression is also observed in striped bass (82) and female chub mackerel (85). However, no effect of Kiss1 treatment on the relative abundances of lhb and fshb is observed in zebrafish (31) and chub mackerel (86, 87). Kiss2 also dose not alter lhb and fshb mRNA levels in yellowtail kingfish (88), chub mackerel (85), striped bass (81) and European sea bass (84).
In addition to the effects on gonadotropins, kisspeptins have also been shown to be involved in regulating the synthesis and/or release of other pituitary hormones in fish. Kiss1 in goldfish directly stimulates the secretion of Prl and Gh as well as gene expression in vitro (71). Similarly, Kiss1 enhances the release of somatolactin-a (Sla) in goldfish pituitary cells (78). Kiss2 in zebrafish significantly stimulates the expression of prl1 in the female pituitary in vitro without affecting the mRNA levels of prl2, pro-opiomelanocortin-a (pomca) and pomcb (77). At the pituitary level, injection of Kiss1, but not Kiss2, significantly increases pituitary levels of Gnrh1 in European sea bass (83). In addition, an inhibitory effect of kisspeptin on gnrhr expression is observed in European eel (72), European sea bass (83) and striped bass (81). As mentioned earlier, it is also important to emphasize that unidentified Kiss2 cells and projections are found in the PPD, as well as the distribution of Gnrh3 fibers (77), suggesting the possibility of a paracrine/autocrine intrapituitary kisspeptinergic system.
Kisspeptins´ Actions on the Gonads
To date, there are a few reports on the effects or functions of kisspeptins at the gonadal level in teleosts. An initial study in yellowtail kingfish showed that chronic treatment with Kiss1 and Kiss2 could stimulate gonadal development in prepubertal males (103). Further studies in the same species showed that Kiss1 is more effective in stimulating gonadal development during the breeding season, while the effects of Kiss2 is more pronounced during the nonbreeding season (88). Kiss1 is also able to accelerate puberty onset in juvenile male white bass (Morone chrysops) (104). Plasma levels of 11-ketotestosterone (11-KT) and 17b-estradiol are increased, and spermatogenesis and the onset of vitellogenesis are observed in sexually immature adult chub mackerel over 6–7 weeks following subcutaneous implantation of Kiss1, but not Kiss2 (85). Furthermore, subcutaneous injection of Kiss1 also accelerates spermatogenesis in prepubertal male chub mackerel (86).
On the other hand, only Kiss2 stimulates plasma levels of testosterone (T) and 11-KT in male European sea bass, causing an increase in cumulative milt, sperm density and sperm motility parameters (83). Similarly, plasma levels of 11-KT in males and E2 in females are significantly increased in immature Nile tilapia treated with Kiss2, and Kiss2 apparently accelerates the process of spermatogenesis (92). Recently, Kiss2 was shown to stimulate T secretion in both sexes of Senegalese sole (94). All these data suggest an effect on the gonads, probably mediated by gonadotropins, but a direct effect of kisspeptins on the gonads have been less considered.
It is now known that there is intra-gonadal expression of kisspeptins and kisspeptin receptors in fish gonads, suggesting a local action on fish gonads (36, 42, 62, 63, 105–110). In this context, the intra-gonadal roles of kisspeptin in fish are poorly understood. For example, Kiss1 was recently detected in the gonads of Asian catfish (Clarias batrachus) and it was suggested that it could locally regulate gonadal steroidogenesis (111, 112). In addition, Chaube et al. (95) found that kisspeptins in female stinging catfish, Heteropneustes fossilis, act not only at the brain or pituitary level but also on the ovary to stimulate ovarian maturation and ovulation, demonstrating the potential of these peptides for aquaculture.
Taken together, these results suggest that kisspeptins may regulate the reproductive axis by acting not only at the brain and pituitary level but also at the gonadal level in teleost species.
Other Physiological Roles
Less explored and beyond the control of reproduction, kisspeptins are involved in other physiological processes in fish. For example, mammalian kisspeptin increases the expression of pituitary gh, sl, melatonin receptor (mt), and hepatic insulin growth factor-1 (igf-1), along with higher levels of plasma Gh, Igf-1, and melatonin in the cinnamon clownfish (Amphiprion melanopus), suggesting a role in controlling growth in this species (113). On the other hand, intracranial administration of Kiss1 suppresses the fear response elicited by an alarm substance (AS) in zebrafish, representing a unique role for the Kiss1 system in the brain of teleosts (114). Further studies in the same species showed that Kiss1 reduces the AS-triggered fear response via serotonin receptors (115).
However, whether and how kisspeptins are involved in the control of food intake and energy balance in fish remains unknown and represents a promising area for future research, as nutritional status has a profound effect on kiss/kissr gene expression in some teleosts (7, 65, 116).
Signaling Pathways Activated by the Kiss/Kissr Systems in Fish
Despite the importance of studying the involvement of kisspeptins in the regulation of reproduction in fish, the detailed intracellular signaling pathways mediating the effects of the Kiss/Kissr systems have not been fully elucidated (2, 10, 117). In these studies, mainly heterologous mammalian cell lines transfected with fish cognate receptors were used together with cAMP-responsive element-dependent luciferase (CRE-luc) or serum responsive element-dependent luciferase (SRE-luc) reporter assays to investigate the possible involvement of the protein kinase A (PKA) or protein kinase C (PKC) pathways, respectively (118, 119).
Analysis of zebrafish Kissr3 signal transduction in COS-7 cells reveals a clear stimulation of CRE-luc activity and SRE-luc activity by Kiss1, suggesting that zebrafish Kissr3 signal can be transduced via both PKA and PKC pathways, whereas Kissr2 transduces its activity through the PKC pathway (41). Similarly, in zebrafish, both Kiss1 and Kiss2 induce a concentration-dependent increase in SRE-luc activity in CV-1 cells, CHO-K1 cells, and HEK293 cells expressing their cognate receptors (35, 69). In chub mackerel and medaka, however, Kissr3 activity is transduced via the PKC pathway, whereas Kissr2 signaling is transduced via both the PKA and PKC pathways (19, 120). Similar results are also observed for Kissr3 signaling in Pacific bluefin tuna, Thunnus orientalis and Japanese Spanish mackerel, Scomberomorus niphonius (121).
On the other hand, in striped bass, both Kissr2 and Kissr3 are signaling through the PKC pathway rather than the PKA pathway (82). Interestingly, in goldfish and European sea bass Kissr2 and Kissr3 signals can be transduced via both the PKA and PKC pathways (33, 122). To date, only the Kissr2 type has been identified in orange-spotted grouper, half-smooth tongue sole, yellowtail kingfish and Southern bluefin tuna, and differential activation of the signal transduction pathways has been demonstrated. In the case of orange-spotted grouper, Kiss2 activates the PKC pathway, but not the PKA pathway (91). However, in the other three species, Kissr2 signaling is shown to be transduced via both the PKC and PKA pathways (68, 123). In addition, blockade of the PKC and PKA pathways by specific inhibitors significantly reduces the stimulatory effects induced by half-smooth tongue sole Kiss2, further confirming the participation of these two signaling pathways in the action of Kissr2 (123).
It is worth noting that the coexistence of two Kiss/Kissr systems in a single fish species indicates differential ligand selectivity for the two cognate receptors. In general, Kissr2 and Kissr3 exhibit higher affinity for Kiss2 and Kiss1, respectively, as observed in zebrafish (69), chub mackerel (120), medaka (19), and European sea bass (122). However, in goldfish, Kissr3 is more efficiently activated by Kiss2, whereas Kissr2 is preferentially activated by Kiss1 (33). In striped bass, Kissr3 is activated almost equally by Kiss1 and Kiss2, and Kissr2 is activated more efficiently by Kiss2 than by Kiss1 (82). It is noteworthy that the longer ligand forms show a stronger efficacy in activating the receptors than the core decapeptide (35, 82, 120–122).
In addition, the possible involvement of intracellular Ca2+ was also evaluated among post-receptor signaling events evoked by kisspeptin, showing that all European eel kisspeptin forms are able to increase intracellular Ca2+ in CHO-K1 cells stably transfected with the rat Kissr1 (72). It should be noted that the European eel is the only teleost species that possesses three different kisspeptin receptors (Kissr1, Kissr2, and Kissr3) that have been studied to date. However, there is no information on the signaling pathways triggered by homologous Kiss peptides across each Kissr type of eel (10, 72). On the other hand, other studies were performed using primary cultured pituitary cells to investigate the molecular mechanisms of the effects of the Kiss/Kissr system on target cells. Consistent with the results obtained with the heterologous systems mentioned above, goldfish Kiss1 may act directly at the pituitary level to increase SLa release via the PKA and PKC pathways and subsequent activation of Ca2+-dependent cascades (78). Goldfish Kiss1 also directly stimulates the secretion of Lh and Gh from primary cultures of pituitary cells in a Ca2+-dependent manner (101). Moreover, Kiss2 is shown to increase phosphorylation levels of ERK and Akt in female pituitary explants in zebrafish (77).
Currently, there is limited information on the interaction between kisspeptins and other neuroendocrine factors in cell signaling (124). In zebrafish, none of the three LPXRFa peptides (LPXRFa-1, LPXRFa-2, and LPXRFa-3) alters SRE-luc activity in COS-7 cells transfected with any of the three cognate LPXRFa receptors (LPXRFa-R1, LPXRFa-R2, and LPXRFa-R3), however, both LPXRFa-2 and LPXRFa-3 exert an inhibitory effect on Kiss2 activation of Kissr2, which involves the PKC pathway (125). Moreover, LPXRFa-2, but not LPXRFa-3, also inhibits Kiss1 activation of Kissr3, which involves the PKC pathway (125). Similarly, half-smooth tongue sole LPXRFa-1 and LPXRFa-2 can also antagonize the action of Kissr2 by inhibiting the PKC pathway (90). Because half-smooth tongue sole LPXRFa-R is coupled to Gαi protein (126), whereas its Kissr2 is coupled to Gαs protein (123), thus LPXRFa-2 also exerts an inhibitory effect on Kissr2 signaling involving the PKA pathway (123). Of note, Kissr3, LPXRFa-R2, and LPXRFa-R3 all transduce their activity through the PKA pathway in zebrafish (41, 125), but no comparative studies have been conducted. Given that activation of Kissr1 in mammals is coupled to multiple signals (10, 12, 127), further studies are needed to investigate previously unknown intracellular mechanisms by which kisspeptin exerts its physiological functions in teleosts, as well as possible interactions of kisspeptins with other factors (Figure 3).
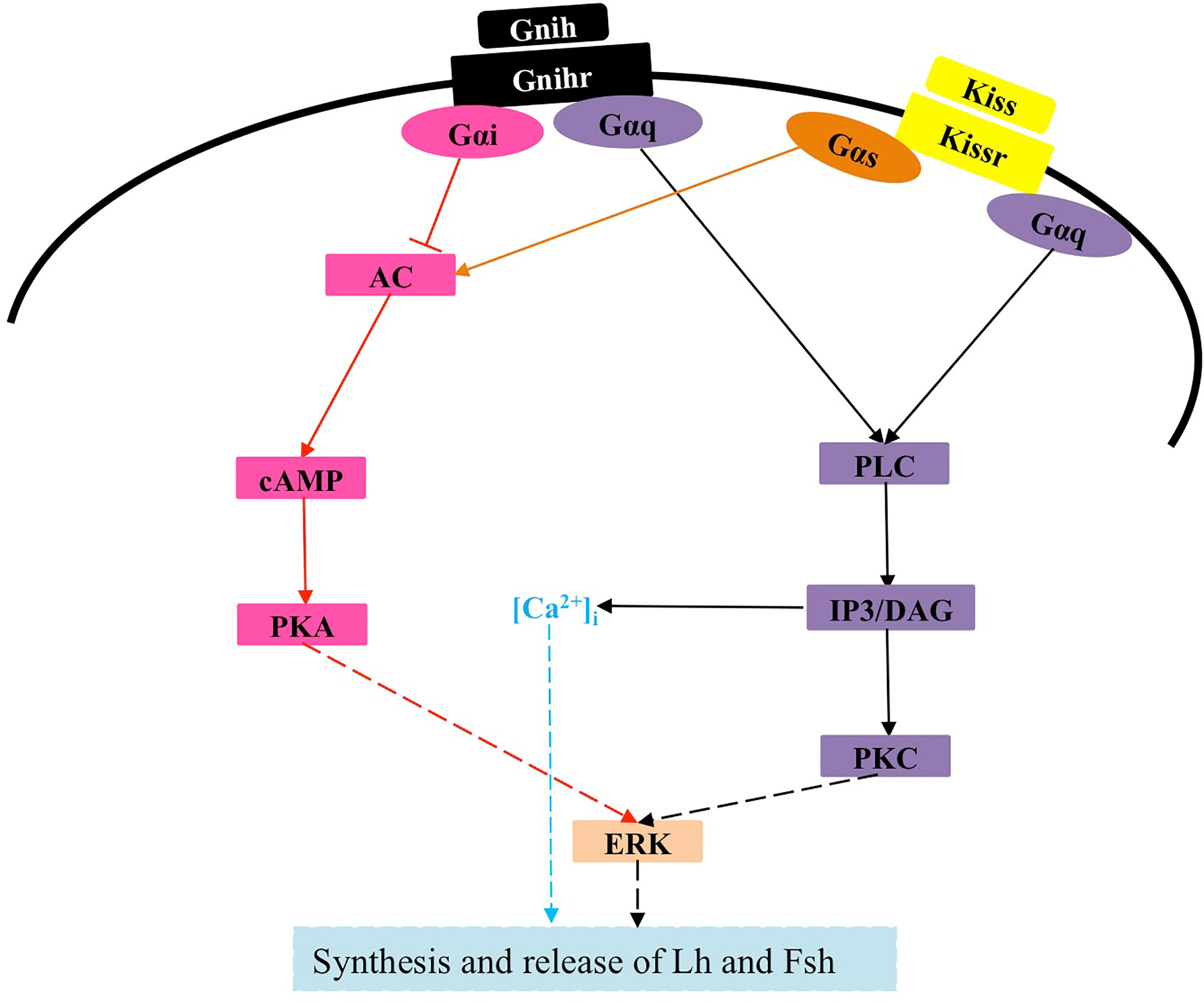
Figure 3 Signaling pathway of Kissr and possible interaction with Gnih in teleosts. The solid lines represent confirmed effects, whereas the dashed lines indicate very limited evidence or possible pathways and interactions that merit further investigation. Kiss, kisspeptin; Kissr, Kiss receptor; Gnih, gonadotropin-inhibitory hormone; Gnihr, Gnih receptor; Gαq, Gαs and Gαi, heterotrimeric G proteins; PLC, phospholipase C; IP3, inositol 1,4,5-trisphosphate; DAG, diacylglycerol; PKC, protein kinase C; ERK, extracellular signal-regulated kinase; AC, adenylyl cyclase; PKA, protein kinase A; Lh, luteinizing hormone; Fsh, follicle-stimulating hormone.
Regulation of the Kiss/Kissr Systems in Fish
Photoperiod (Melatonin)
In mammals, kisspeptin is recognized as a mediator of photoperiodic control of reproduction, and the effects of photoperiod are mainly by melatonin produced in the pineal gland during the night (128, 129). Nevertheless, studies on the effects of photoperiod on the kisspeptin system are still scarce and in some way contradictories in teleosts. For example, an initial study in Nile tilapia showed that continuous illumination reduces brain kissr2 expression levels, suggesting a possible link between environmental stimuli and the kisspeptin system (130). In contrast, continuous light increases hypothalamic kissr2 expression levels in Atlantic salmon (64). On the other hand, there is no clear relationship between kiss2/kissr2 expression and photoperiod in Atlantic cod (131).
In medaka, a long-day (LD) breeder, the number of kiss1 neurons located in the Nucleus ventral tuberis (NVT) in the LD condition is larger than that in the short-day (SD) condition, whereas the kiss2 neurons located in the Nucleus recessus lateralis (NRL) are not altered (29, 132). On the contrary, kiss2, but not kiss1, transcript levels in the brain of striped bass/white bass hybrid, a SD spawner, increase in the SD regime compared to the LD regime (133). In zebrafish, a LD breeder, constant darkness increases brain melatonin concentrations, and melatonin stimulates kiss1 and kiss2 gene expression in the brain (134, 135). Similarly, melatonin elicits a significant increase in kiss1, kiss2 and kissr2 mRNA abundance in the hypothalamus of male European sea bass (136), while an inhibitory effect of melatonin on kiss1 and kiss2 mRNA levels is observed in the dorsal brain of male European sea bass (136) and in the whole brain of female sapphire devil, Chrysiptera cyanea (137). Furthermore, continuous light results in the loss of forebrain-midbrain kiss1/kissr3 seasonal rhythms in male European sea bass, which apparently prevents further normal testicular development (138). Taken together, these results indicate that the effects of photoperiod mediated by melatonin can regulate the kiss/kissr systems. This appeared to be species- and tissue-specific, and the mechanisms of action remain to be studied in detail in fish.
Temperature
Temperature, especially in ectothermic vertebrates is one of the most important environmental factors regulating reproduction. However, the mechanism by which temperature affects reproduction remains unclear in teleosts. Preliminary evidence has indicated that kisspeptin systems may be involved in mediating the effects of temperature on reproduction. For example, in sexually mature male zebrafish temperature differentially modulated gene expression of the two kisspeptin systems (139). A low temperature of 15°C, but not a high temperature of 35°C, significantly increases kiss1 mRNA levels in the whole brain, as well as kissr3 mRNA levels in the habenula and the brain region containing nucleus of the medial longitudinal fascicle, the oculomotor nucleus, and the interpeduncular nucleus. However, kiss2 mRNA levels in the whole brain and kissr2 mRNA levels in the caudal zone of the periventricular hypothalamus and the posterior tuberal nucleus is significantly decreased when exposed to both low and high temperatures. Interestingly, kissr2 mRNA levels in the nucleus of the medial longitudinal fascicle, the oculomotor nucleus, and interpeduncular nucleus show an increase when animals were exposed to low temperatures compared with the normal rearing temperature for this species, 27°C. These results suggest that the kiss1/kissr3 system is activated by low temperatures, whereas the kiss2/kissr2 system is inhibited by both low and high temperatures, suggesting that these two kisspeptin systems may be involved in different aspects of zebrafish physiology (139).
Similarly, an inhibitory effect on the expression of kiss2 and kissr2 genes is also observed in the diencephalon/midbrain of mature male grass puffer, that spawns on the beach in semilunar cycles during spring tide in early summer, when exposed to both low and high temperatures (140). Notably, although brain melatonin concentrations are significantly increased at high temperatures, high temperatures do not affect kiss2 mRNA levels in the hypothalamus of adult male zebrafish (135). On the other hand, high temperature results in an increase in kiss2 transcripts in the head of pejerrey larvae at week 4 after hatching. It is important to note that pejerrey is a fish with strong temperature-dependent sex determination, and high temperatures can result in 100% male offspring. These data suggest that kiss2 may play an important role in the process of sex differentiation in this species (36).
Nutritional Status
In mammals, the reproductive axis is known that be regulated by energy balance, and the kisspeptin system appears to play a key role in linking energy balance and reproduction (141). Fasting has been shown to decrease hypothalamic kiss1 and kissr1 mRNA levels in mouse and rhesus monkey, Macaca mulatta (142, 143). Moreover, fasting in rat results in a concomitant decrease in hypothalamic kiss1 and an increase in kissr1 mRNA levels (144).
In teleosts, kisspeptin systems also appear to be associated with nutritional status. For example, in Senegalese sole, 15 days of starvation results in a significant increase in kiss2 and kissr2 mRNA levels in the hypothalamus, but no changes are observed for these two genes in the stomach (7). Similarly, two alternative variants for kissr3 (kissr3_v1 and kissr3_v2) and kissr2 (kissr2_v1 and kissr2_v2) are identified in pejerrey, and fasting also increases hypothalamic kiss2 and kissr2_v1 mRNA levels, whereas kissr2_v2 shows no expression in the hypothalamus (65). However, food deprivation has no significant effect on the expression levels of kissr2_v1 and kissr2_v2 in the testis and habenula of pejerrey compared to the control group (65).
Also, a longer period of food restriction (14 months) results in an increase in mRNA levels of kiss1, kiss2, kissr2 and kissr3 in the hypothalamus of European sea bass (116). Overall, it appears that the neuroendocrine mechanisms mediating the effect of negative energy balance on reproduction may differ between mammals and teleosts. It is noteworthy that kisspeptin reduces appetite in several mammalian species (145–148). However, whether and how kisspeptins are involved in the regulation of food intake and energy balance in teleosts requires further investigation.
Sex Steroids
Sex steroids, estrogens and androgens, are important for the differential expression of the elements of the kisspeptin systems. For example, in female medaka, the number of kiss1 neurons in the NVT, but not in the nucleus preopticus periventricularis (NPPv), is significantly reduced after ovariectomy (OVX) compared with the sham-operated group, and basal levels are restored after E2 treatment (29). In addition, double-labeling in situ hybridization showed that estrogen receptor alfa (Erα) is expressed together with kiss1 in NVT neurons, suggesting that these neurons are involved in the positive feedback regulation of the BPG axis in this species (132). However, the number of NRL kiss2 neurons is not altered after OVX, and no ERα transcripts are detected in or in close association to the NRL kiss2 neurons (132). In contrast, in goldfish, the number of kiss2 neurons in the POA is downregulated after OVX and is restored by E2 administration, and kiss2 neurons in the POA express all three ER types (149).
In OVX orange-spotted grouper, the expression of kiss2 but not kiss1 is significantly increased in the brain, and E2 substitution could reverse this effect (150). Bioinformatics analysis of the promoter of kisspeptins and kisspeptin receptors in yellowtail kingfish and zebrafish reveals high abundance of several regulatory elements such as AP-1, Sp1, ER, AR and PR (151), suggesting possible regulation of Kiss genes and their receptors by steroids, especially E2. It was also demonstrated that E2 is able to positively feedback on the expression of kiss1 and kiss2 in goldfish through different ERα pathways (152), and similar results are observed in orange-spotted grouper (150). On the other hand, E2 treatment causes a significant increase in mRNA expression of kiss1, kiss2, and kissr2 in zebrafish brain, but kissr3 transcript levels are not altered (16). In addition, a positive effect of E2 on the expression of kiss2 but not kiss1 is observed in the brain of the sapphire devil, Chrysiptera cyanea (153) and in the hypothalamus of the Dabry’s sturgeon, Acipenser dabryanus (154).
Kisspeptin receptors are also regulated by gonadal steroids in fish. E2 also increases expression of the kissr2 and kissr3 genes in the sapphire devil brain (155) and European sea bass pituitary cells (84). Hypothalamic kissr3 but not kissr2 transcripts are upregulated in Dabry’s sturgeon after E2 injection (154), whereas neither kiss2 nor kissr2 mRNA levels are altered by E2 in the hypothalamus of half-smooth tongue sole (156). Interestingly, no significant changes in hypothalamic kiss1, kiss2 and their receptors mRNA levels are observed in European sea bass by E2 treatment after OVX, as determined by qRT-PCR. However, the number of kiss1 and kiss2 expressing cells is reduced in some brain regions, and E2 replacement prevents this effect, as revealed by in situ hybridization (157).
Androgens have also been shown to mediate feedback on the regulation of kisspeptin neurons. Transcript levels of kiss1, kiss2, and kissr2 in the brain are reduced by T treatment of OVX female striped bass during mid-vitellogenesis (158). Similarly, T administration reduces mRNA levels of kiss1, kiss2, and kissr2 in the brain of gonadectomized (GDX) at mid-gonadal development of male striped bass. In contrast, pubertal males responds to T replacement by up-regulation of kiss1 and kiss2, whereas no changes are observed in juvenile and recrudescent males, suggesting a differential and gonadal stage-dependent role of T in regulating mRNA levels of kiss1 and kiss2 (133). On the other hand, a negative feedback effects of T on hypothalamic kiss2 expression is observed in GDX European sea bass males, without affecting kiss1, kissr2 and kissr3 mRNA levels (157). However, T has no effect on the expression of the elements of kisspeptin system in the hypothalamus of half-smooth tongue sole (156) and midbrain of goldfish (159). A stimulatory effect of T on mRNA levels of kissr2 and kissr3 is detected in primary cultured pituitary cells of European sea bass (84). Taken together, these results suggest that the regulation of genes encoding kisspeptins and their receptor by gonadal steroids in teleosts depends on the species, tissue, gene, reproductive stage, and route of administration and that needs to be investigated in each individual species.
Neuropeptides Related to Reproduction
In teleosts, negative and positive feedbacks were described for kisspeptins on their own expression. For example, Kiss1 administration decreases the amount of kiss1 mRNA in the habenula of zebrafish (160) and induces a higher expression of kissr2 in the brain of fathead minnow (40). Similarly, Kiss2 stimulates kissr2 mRNA levels in primary cultured brain cells of Japanese flounder (100). Both kiss2 and kissr2 transcript levels are significantly increased in the hypothalamus of black porgy, Acanthopagrus schlegelii, after injection with Kiss2 (99). In addition, exogenous administration of Kiss2 increases gene expression of reproduction-related genes (gnrh3, kissr2, fshb, lhb, ar, and er2), sex hormone levels (E2 and T), and accelerates the onset of puberty in largemouth bass, Micropterus salmoides (96). On the other hand, Kiss2 increases hypothalamic kiss2 expression in half-smooth tongue sole, and decreases kissr2 mRNA levels (89). In addition, a negative effect of Kiss2 is found on the mRNA abundance of kissr2_v1 and kissr2_v5 in the brain of male yellowtail kingfish, while the mRNA levels of kissr2_v4 are significantly increased (68).
Injection of Kiss2 does not alter kissr2 mRNA levels in the hypothalamus of lined seahorse, Hippocampus erectus (93). Neither Kiss1 nor Kiss2 alters the transcript levels of kissr2 and kissr3 mRNAs in the hypothalamus of European sea bass, and kissr3 mRNA levels in the forebrain-midbrain are not altered by these two peptides (83). However, kissr2 gene expression is increased in the forebrain-midbrain after exposure to Kiss1 and Kiss2 (83). Interestingly, a differential and gonadal stage-dependent roles of Kiss1 and Kiss2 in regulating kissr2 expression in hybrid bass brain is observed (17). Transcript levels of kissr2 are increased only by Kiss2 in prepuberty, whereas a significant decrease in mRNA levels is observed after treatment with Kiss1 and Kiss2 in recrudescence (17).
LPXRFa may also induce differential effects on the expression of kisspeptins and their receptor in teleosts. Intracerebroventricular (icv) injection of LPXRFa-2 suppresses kiss1, kiss2, and kissr3 transcripts in the brain of male European sea bass, without affecting kissr2 expression (161). However, intramuscular (im) injection of LPXRFa-2 significantly increases kissr3 expression and has no effect on the expression of the other genes (162). On the other hand, no changes in the expression of these four kisspeptin genes (kiss1, kiss2, kissr2, and kissr3) are observed after administration of LPXRFa-1 in the same studies (161, 162). In half-smooth tongue sole, neither LPXRFa-1 nor LPXRFa-2 alters hypothalamic kiss2 mRNA levels in vitro (163). Similarly, im injection of LPXRFa-2 and LPXRFa-3 does not alter kiss2 gene expression in the brain of Senegalese sole (164), and none of the three LPXRFa peptides alters hypothalamic kiss1 and kiss2 mRNA levels in orange-spotted grouper (165).
In mammals, kisspeptin is considered an upstream regulator of Gnrh secretion, and although the situation is clearly different in teleosts, Gnrh may exert feedback on gene expression of kisspeptin systems. A mammalian GnRH analog, [D-Ala6, Pro9Net]-mGnRHa, has a stimulatory effect on the expression of kiss2 in European sea bass pituitary cell cultures but has no effect on the mRNA levels of kissr2 and kissr3 (84). Furthermore, no significant differences in hypothalamic abundance of kiss2 and kissr2 mRNAs are observed after exposure to the aforementioned GnRHa in half-smooth tongue sole (89). Similarly, treatment with GnRHa has no effects on the expression levels of kissr2 in the brain, pituitary gland, and gonads in male yellowtail kingfish (88). Overall, these results suggest a complex control of the kisspeptin system, and each neuropeptide exerts a differential effect on kisspeptin gene regulation, which could depend on the species, sex, tissues, reproductive stages of the animals, peptides used, dose, route of administration, and elapsed time after treatment.
Other Factors
Thyroid hormones (T3 and T4) play an important role in the control of growth, morphogenesis, metabolism, and reproduction in several species, including fish (166, 167). Moreover, T3 ip administration significantly increases hypothalamic kiss2 gene in sexually mature male Nile tilapia, whereas this gene is suppressed under a hypothyroid condition induced by methimazole treatment (168).
Endocrine disrupting chemicals (EDCs) can also affect reproductive regulation, in part by affecting kisspeptins system, which is a clear example of neuroendocrine disruption (169). For example, bisphenol-A shows a greatly increased expression of kiss1, kiss2, and kissr2 in the brains of pubertal Catla (Catla catla) without affecting mRNA levels of kissr3 (170). In addition, bisphenol-F leads to an increase in the expression of kiss1 and kissr3 in the brain of zebrafish but has no effect on the mRNA levels of kiss2 and kissr2 (171). In adult male goldfish exposed to vinclozolin, a pesticide that acts as an antiandrogen and impairs reproduction in mammals, kiss1 but not kiss2 mRNA levels are increased in the midbrain (159). Similarly, the antiandrogen flutamide also induces kiss1 and kiss2 gene expression in the midbrain of goldfish (159). All these data suggest that these EDCs act on steroid receptors and/or steroid balance.
On the other hand, semicarbazide (SMC), an industrially produced synthetic hydrazine compound, significantly downregulates mRNA expression of kiss2 and kissr2 in the brain of female Japanese flounder (172). An inhibitory effect of SMC on kissr2 expression in the brain is also observed in male Japanese flounder (173). Moreover, mRNA levels of kissr2 and kissr3 are significantly reduced in the brain of adult female Japanese medaka after chronic exposure to Roundup, a glyphosate-based herbicide. However, neither kiss1 nor kiss2 transcripts are altered (174). Moreover, these EDCs may act on the kisspeptins system by mimicking the effects of gonadal steroids, as plasma E2 and T levels can be altered by EDCs (93, 173).
Interestingly, other less studied factors, such as social status may also regulate mRNA levels of kissr in the entire brain of mouthbrooding cichlids, with higher mRNA levels of kissr2 observed in high-status territorial males compared to non-territorial males (75).
Conclusions and Future Directions
In fish, kisspeptins may exert their functions by acting at multiple levels of the brain-pituitary-gonadal axis. Two recent reviews focusing on fish and vertebrates highlighted the different pathways by which kisspeptins may be involved in reproduction, discussed the levels and nature of action, and interaction with Gnrh and other neuropeptides (15, 43). In this review, attention was focused on the whole reproductive brain-pituitary-gonadal axis. Unlike mammals, kiss/kissr null zebrafish and medaka can reproduce normally, suggesting that kisspeptin is either not essential for reproduction or that there are compensation mechanisms exerted by other neuropeptides. Teleost are known for their neuroplasticity and multifactorial control of reproduction, with new reproductive neuropeptides emerging (175–177).
With respect to Kiss/Kissr diversity and evolution, we focused particularly on Pleuronectiformes because this order is a good model from an evolutionary perspective and multiple genomes are currently available. Moreover, in Pleuronectiformes, previous studies have mentioned that the kisspeptin-1 system seems to have been lost during evolution (8, 15, 45), but recent synteny and phylogenetic analysis has shown that this is not so clear for all species in this group. In addition, four rounds of genome duplication are known to have occurred in salmonids (178), but no additional kiss or kissr have been found to date. Therefore, it will be interesting to search for orthologous pseudogenes in salmonid genomes.
Most studies on kisspeptin in fish have focused on reproduction, while the role of the kisspeptin system in peripheral tissues is still unclear and there are important questions to be addressed. For example, kisspeptin suppresses food intake in some mammalian species, such as mice, rats, and desert jerboas (145–148). Whether and how kisspeptin regulates appetite and energy balance in teleosts is not yet clear and requires further investigation. Further studies are also needed to elucidate the roles of the kisspeptin systems in development, metabolism, and behavior, as well as to explore the intracellular signaling pathways involved in kisspeptin actions and possible interactions with other neuroendocrinological factors in teleosts.
Author Contributions
BW, ASM, and GMS contributed equally to the manuscript. All authors contributed to the article and approved the submitted version.
Funding
This study was carried out with financial support from a project funded by the Agencia Nacional de Promoción Científica y Tecnológica (ANPCYT, Argentina) PICT-2015-2783 to GMS. and PICT-2017- 2839 to ASM, the National Natural Science Foundation of China 32072949 and the Laboratory for Marine Fisheries and Food Production Processes, Pilot National Laboratory for Marine Science and Technology (Qingdao) ZZ-B06 to BW, who also received a scholarship supported by the China Scholarship Council (CSC, File No. 201903260004).
Conflict of Interest
The authors declare that the research was conducted in the absence of any commercial or financial relationships that could be construed as a potential conflict of interest.
Publisher’s Note
All claims expressed in this article are solely those of the authors and do not necessarily represent those of their affiliated organizations, or those of the publisher, the editors and the reviewers. Any product that may be evaluated in this article, or claim that may be made by its manufacturer, is not guaranteed or endorsed by the publisher.
References
1. Parhar IS, Ogawa S, Sakuma Y. Laser-Captured Single Digoxigenin-Labeled Neurons of Gonadotropin-Releasing Hormone Types Reveal a Novel G Protein-Coupled Receptor (Gpr54) During Maturation in Cichlid Fish. Endocrinology (2004) 145:3613–8. doi: 10.1210/en.2004-0395
2. Tena-Sempere M, Felip A, Gomez A, Zanuy S, Carrillo M. Comparative Insights of the Kisspeptin/Kisspeptin Receptor System: Lessons From Non-Mammalian Vertebrates. Gen Comp Endocrinol (2012) 175:234–43. doi: 10.1016/j.ygcen.2011.11.015
3. Kirilov M, Clarkson J, Liu X, Roa J, Campos P, Porteous R, et al. Dependence of Fertility on Kisspeptin–Gpr54 Signaling at the GnRH Neuron. Nat Commun (2013) 4:2492. doi: 10.1038/ncomms3492
4. Ruohonen ST, Poutanen M, Tena-Sempere M. Role of Kisspeptins in the Control of the Hypothalamic-Pituitary-Ovarian Axis: Old Dogmas and New Challenges. Fertil Steril (2020) 114:465–74. doi: 10.1016/j.fertnstert.2020.06.038
5. Mechaly AS, Viñas J, Murphy C, Reith M, Piferrer F. Gene Structure of the Kiss1 Receptor-2 (Kiss1r-2) in the Atlantic Halibut: Insights Into the Evolution and Regulation of Kiss1r Genes. Mol Cell Endocrinol (2010) 317:78–89. doi: 10.1016/j.mce.2009.11.005
6. Mechaly AS, Viñas J, Piferrer F. Identification of Two Isoforms of the Kisspeptin-1 Receptor (Kiss1r) Generated by Alternative Splicing in a Modern Teleost, the Senegalese Sole (Solea Senegalensis). Biol Reprod (2009) 80:60–9. doi: 10.1095/biolreprod.108.072173
7. Mechaly AS, Viñas J, Piferrer F. Gene Structure Analysis of Kisspeptin-2 (Kiss2) in the Senegalese Sole (Solea Senegalensis): Characterization of Two Splice Variants of Kiss2, and Novel Evidence for Metabolic Regulation of Kisspeptin Signaling in non-Mammalian Species. Mol Cell Endocrinol (2011) 339:14–24. doi: 10.1016/j.mce.2011.03.004
8. Song H, Wang M, Wang Z, Liu J, Qi J, Zhang Q. Characterization of Kiss2 and Kissr2 Genes and the Regulation of Kisspeptin on the HPG Axis in Cynoglossus Semilaevis. Fish Physiol Biochem (2017) 43:731–53. doi: 10.1007/s10695-016-0328-x
9. Song H, Wang M, Zhongkai W, Yu H, Wang Z, Zhang Q. Identification and Characterization of Kiss2 and Kissr2 Homologs in Paralichthys Olivaceus. Fish Physiol Biochem (2016) 42:1073–92. doi: 10.1007/s10695-016-0199-1
10. Pasquier J, Kamech N, Lafont AG, Vaudry H, Rousseau K, Dufour S. Molecular Evolution of GPCRs: Kisspeptin/kisspeptin Receptors. J Mol Endocrinol (2014) 52:T101–17. doi: 10.1530/JME-13-0224
11. Roa J, Aguilar E, Dieguez C, Pinilla L, Tena-Sempere M. New Frontiers in Kisspeptin/GPR54 Physiology as Fundamental Gatekeepers of Reproductive Function. Front Neuroendocrinol (2008) 29:48–69. doi: 10.1016/j.yfrne.2007.07.002
12. Pinilla L, Aguilar E, Dieguez C, Millar RP, Tena-Sempere M. Kisspeptins and Reproduction: Physiological Roles and Regulatory Mechanisms. Physiol Rev (2012) 92:1235–316. doi: 10.1152/physrev.00037.2010
13. Kah O. A 45-Years Journey Within the Reproductive Brain of Fish. Gen Comp Endocrinol (2020) 288:113370. doi: 10.1016/j.ygcen.2019.113370
14. Muñoz-Cueto JA, Zmora N, Paullada-Salmerón JA, Marvel M, Mañanos E, Zohar Y. The Gonadotropin-Releasing Hormones: Lessons From Fish. Gen Comp Endocrinol (2020) 291:113422. doi: 10.1016/j.ygcen.2020.113422
15. Somoza GM, Mechaly AS, Trudeau VL. Kisspeptin and GnRH Interactions in the Reproductive Brain of Teleosts. Gen Comp Endocrinol (2020) 298:113568. doi: 10.1016/j.ygcen.2020.113568
16. Servili A, Le Page Y, Leprince J, Caraty A, Escobar S, Parhar IS, et al. Organization of Two Independent Kisspeptin Systems Derived From Evolutionary-Ancient Kiss Genes in the Brain of Zebrafish. Endocrinology (2011) 152:1527–40. doi: 10.1210/en.2010-0948
17. Zmora N, Stubblefield J, Zulperi Z, Biran J, Levavi-Sivan B, Munoz-Cueto JA, et al. Differential and Gonad Stage-Dependent Roles of Kisspeptin1 and Kisspeptin2 in Reproduction in the Modern Teleosts, Morone Species. Biol Reprod (2012) 86:177. doi: 10.1095/biolreprod.111.097667
18. Escobar S, Servili A, Espigares F, Gueguen MM, Brocal I, Felip A, et al. Expression of Kisspeptins and Kiss Receptors Suggests a Large Range of Functions for Kisspeptin Systems in the Brain of the European Sea Bass. PloS One (2013) 8:e70177. doi: 10.1371/journal.pone.0070177
19. Kanda S, Akazome Y, Mitani Y, Okubo K, Oka Y. Neuroanatomical Evidence That Kisspeptin Directly Regulates Isotocin and Vasotocin Neurons. PloS One (2013) 8:e62776. doi: 10.1371/journal.pone.0062776
20. Nakajo M, Kanda S, Karigo T, Takahashi A, Akazome Y, Uenoyama Y, et al. Evolutionarily Conserved Function of Kisspeptin Neuronal System is Nonreproductive Regulation as Revealed by Nonmammalian Study. Endocrinology (2018) 159:163–83. doi: 10.1210/en.2017-00808
21. Ogawa S, Sivalingam M, Anthonysamy R, Parhar IS. Distribution of Kiss2 Receptor in the Brain and its Localization in Neuroendocrine Cells in the Zebrafish. Cell Tiss Res (2020) 379:349–72. doi: 10.1007/s00441-019-03089-5
22. Tang H, Liu Y, Luo D, Ogawa S, Yin Y, Li S, et al. The Kiss/Kissr Systems are Dispensable for Zebrafish Reproduction: Evidence From Gene Knockout Studies. Endocrinology (2015) 156:589–99. doi: 10.1210/en.2014-1204
23. Liu Y, Tang H, Xie R, Li S, Liu X, Lin H, et al. Genetic Evidence for Multifactorial Control of the Reproductive Axis in Zebrafish. Endocrinology (2017) 158:604–11. doi: 10.1210/en.2016-1540
24. Spicer OS, Wong TT, Zmora N, Zohar Y. Targeted Mutagenesis of the Hypophysiotropic Gnrh3 in Zebrafish (Danio Rerio) Reveals No Effects on Reproductive Performance. PloS One (2016) 11:e0158141. doi: 10.1371/journal.pone.0158141
25. Marvel M, Spicer OS, Wong TT, Zmora N, Zohar Y. Knockout of the Gnrh Genes in Zebrafish: Effects on Reproduction and Potential Compensation by Reproductive and Feeding-Related Neuropeptides. Biol Reprod (2018) 99:565–77. doi: 10.1093/biolre/ioy078
26. Whitlock KE, Postlethwait J, Ewer J. Neuroendocrinology of Reproduction: Is Gonadotropin-Releasing Hormone (GnRH) Dispensable? Front Neuroendocrinol (2019) 53:100738. doi: 10.1016/j.yfrne.2019.02.002
27. Abraham E, Palevitch O, Gothilf Y, Zohar Y. Targeted Gonadotropin-Releasing Hormone-3 Neuron Ablation in Zebrafish: Effects on Neurogenesis, Neuronal Migration, and Reproduction. Endocrinology (2010) 151:332–240. doi: 10.1210/en.2009-0548
28. Yun S, Kim DK, Furlong M, Hwang JI, Vaudry H, Seong JY. Does Kisspeptin Belong to the Proposed RF-Amide Peptide Family? Front Endocrinol (Lausanne) (2014) 5:134. doi: 10.3389/fendo.2014.00134
29. Kanda S, Akazome Y, Matsunaga T, Yamamoto N, Yamada S, Tsukamura H, et al. Identification of KiSS-1 Product Kisspeptin and Steroid-Sensitive Sexually Dimorphic Kisspeptin Neurons in Medaka (Oryzias Latipes). Endocrinology (2008) 149:2467–76. doi: 10.1210/en.2007-1503
30. van Aerle R, Kille P, Lange A, Tyler CR. Evidence for the Existence of a Functional Kiss1/Kiss1 Receptor Pathway in Fish. Peptides (2008) 29:57–64. doi: 10.1016/j.peptides.2007.10.018
31. Kitahashi T, Ogawa S, Parhar IS. Cloning and Expression of Kiss2 in the Zebrafish and Medaka. Endocrinology (2009) 150:821–31. doi: 10.1210/en.2008-0940
32. Kotani M, Detheux M, Vandenbogaerde A, Communi D, Vanderwinden JM, Le Poul E, et al. The Metastasis Suppressor Gene KiSS-1 Encodes Kisspeptins, the Natural Ligands of the Orphan G Protein-Coupled Receptor GPR54. J Biol Chem (2001) 276:34631–6. doi: 10.1074/jbc.M104847200
33. Li S, Zhang Y, Liu Y, Huang X, Huang W, Lu D, et al. Structural and Functional Multiplicity of the Kisspeptin/GPR54 System in Goldfish (Carassius Auratus). J Endocrinol (2009) 201:407–18. doi: 10.1677/joe-09-0016
34. Ohga H, Adachi H, Kitano H, Yamaguchi A, Matsuyama M. Kiss1 Hexadecapeptide Directly Regulates Gonadotropin-Releasing Hormone 1 in the Scombroid Fish, Chub Mackerel. Biol Reprod (2017) 96:376–88. doi: 10.1095/biolreprod.116.142083
35. Lee YR, Tsunekawa K, Moon MJ, Um HN, Hwang JI, Osugi T, et al. Molecular Evolution of Multiple Forms of Kisspeptins and GPR54 Receptors in Vertebrates. Endocrinology (2009) 150:2837–46. doi: 10.1210/en.2008-1679
36. Tovar-Bohórquez MO, Mechaly AS, Hughes LC, Campanella D, Orti G, Canosa LF, et al. Kisspeptin System in Pejerrey Fish (Odontesthes Bonariensis). Characterization and Gene Expression Pattern During Early Developmental Stages. Comp Bioch Physiol (2017) 204A:146–56. doi: 10.1016/j.cbpa.2016.11.014
37. Clements MK, McDonald TP, Wang RP, Xie GC, O’Dowd BF, George SR, et al. FMRFamide-Related Neuropeptides are Agonists of the Orphan G-Protein-Coupled Receptor GPR54. Biochem Biophys Res Commun (2001) 284:1189–93. doi: 10.1006/bbrc.2001.5098
38. Mohamed JS, Benninghoff AD, Holt GJ, Khan IA. Developmental Expression of the G Protein-Coupled Receptor 54 and Three GnRH mRNAs in the Teleost Fish Cobia. J Mol Endocrinol (2007) 38:235–44. doi: 10.1677/jme.1.02182
39. Nocillado JN, Levavi-Sivan B, Carrick F, Elizur A. Temporal Expression of G-Protein-Coupled Receptor 54 (GPR54), Gonadotropin-Releasing Hormones (GnRH), and Dopamine Receptor D2 (Drd2) in Pubertal Female Grey Mullet, Mugil Cephalus. Gen Comp Endocrinol (2007) 150:278–87. doi: 10.1016/j.ygcen.2006.09.008
40. Filby AL, van Aerle R, Duitman J, Tyler CR. The Kisspeptin/Gonadotropin-Releasing Hormone Pathway and Molecular Signaling of Puberty in Fish. Biol Reprod (2008) 78:278–89. doi: 10.1095/biolreprod.107.063420
41. Biran J, Ben-Dor S, Levavi-Sivan B. Molecular Identification and Functional Characterization of the Kisspeptin/Kisspeptin Receptor System in Lower Vertebrates. Biol Reprod (2008) 79:776–86. doi: 10.1095/biolreprod.107.066266
42. Ohga H, Selvaraj S, Matsuyama M. The Roles of Kisspeptin System in the Reproductive Physiology of Fish With Special Reference to Chub Mackerel Studies as Main Axis. Front Endocrinol (Lausanne) (2018) 9:147. doi: 10.3389/fendo.2018.00147
43. Sivalingam OS, Trudeau VL, Parhar IS. Conserved Functions of Hypothalamic Kisspeptin in Vertebrates. Gen Comp Endocrinol (2022) 317:113973. doi: 10.1016/j.ygcen.2021.113973
44. Pasquier J, Lafont AG, Jeng SR, Morini M, Dirks R, van den Thillart G, et al. Multiple Kisspeptin Receptors in Early Osteichthyans Provide New Insights Into the Evolution of This Receptor Family. PloS One (2012) 7:e48931. doi: 10.1371/journal.pone.0048931
45. Mechaly AS, Viñas J, Piferrer F. The Kisspeptin System Genes in Teleost Fish, Their Structure and Regulation, With Particular Attention to the Situation in Pleuronectiformes. Gen Comp Endocrinol (2013) 188:258–68. doi: 10.1016/j.ygcen.2013.04.010
46. Felip A, Zanuy S, Pineda R, Pinilla L, Carrillo M, Tena-Sempere M, et al. Evidence for Two Distinct KiSS Genes in non-Placental Vertebrates That Encode Kisspeptins With Different Gonadotropin-Releasing Activities in Fish and Mammals. Mol Cell Endocrinol (2009) 312:61–71. doi: 10.1016/j.mce.2008.11.017
47. Dufour S, Quérat B, Tostivint H, Pasqualini C, Vaudry H, Rousseau K. Origin and Evolution of the Neuroendocrine Control of Reproduction in Vertebrates, With Special Focus on Genome and Gene Duplications. Physiol Rev (2020) 100(2):869–943. doi: 10.1152/physrev.00009.2019
48. Chen S, Zhang G, Shao C, Huang Q, Liu G, Zhang P, et al. Whole-Genome Sequence of a Flatfish Provides Insights Into ZW Sex Chromosome Evolution and Adaptation to a Benthic Lifestyle. Nat Genet (2014) 46:253–60. doi: 10.1038/ng.2890
49. Figueras A, Robledo D, Corvelo A, Hermida M, Pereiro P, Rubiolo JA, et al. Whole Genome Sequencing of Turbot (Scophthalmus Maximus; Pleuronectiformes): A Fish Adapted to Demersal Life. DNA Res (2016) 23:181–92. doi: 10.1093/dnares/dsw007
50. Benzekri H, Armesto P, Cousin X, Rovira M, Crespo D, Merlo MA, et al. De Novo Assembly, Characterization and Functional Annotation of Senegalese Sole (Solea Senegalensis) and Common Sole (Solea Solea) Transcriptomes: Integration in a Database and Design of a Microarray. BMC Genom (2014) 15:952. doi: 10.1186/1471-2164-15-952
51. Manchado M, Planas JV, Cousin X, Rebordinos L, Claros MG. Current Status in Other Finfish Species: Description of Current Genomic Resources for the Gilthead Seabream (Sparus Aurata) and Soles (Solea Senegalensis and Solea Solea). In: Mackenzie S, Jentoft S, editors. Genomics in Aquaculture. Amsterdam: Elsevier (2016). p. 195–221. doi: 10.1016/B978-0-12-801418-9.00008-1
52. Shao C, Bao B, Xie Z, Chen X, Li B, Jia X, et al. The Genome and Transcriptome of Japanese Flounder Provide Insights Into Flatfish Asymmetry. Nat Genet (2017) 49:119–24. doi: 10.1038/ng.3732
53. Burguener G, Viñas J, Martinez P, Boccanfuso JJ, Somoza GM, Turjanski A, et al. Sequencing and First Draft Assembly of the Genome of the Flounder Paralichthys Orbignyanus. In: IX Argentinian Congress of Bioinformatics and Computational Biology. Mar del Plata, Argentina (2018).
54. Lü Z, Gong L, Ren Y, Chen Y, Wang Z, Liu L, et al. Large-Scale Sequencing of Flatfish Genomes Provides Insights Into the Polyphyletic Origin of Their Specialized Body Plan. Nat Genet (2021) 53(5):742–51. doi: 10.1038/s41588-021-00836-9
55. Chapleau F. Pleuronectiform Relationships: A Cladistic Reassessment. Bull Mar Sci (1993) 52(1):516–40.
56. Chanet B, Mondéjar Fernández J, Lecointre G. Flatfishes interrelationships revisited based on anatomical characters2+)/calmodulin-Dependent Cascades. Int J Ichthyol (2020) 44:009–18. doi: 10.26028/cybium/2020-441-002
57. Baptista RP, Kissinger JC. Is Reliance on an Inaccurate Genome Sequence Sabotaging Your Experiments? PloS Pathog (2019) 15:e1007901. doi: 10.1371/journal.ppat.1007901
58. Shimizu Y, Tomikawa J, Hirano K, Nanikawa Y, Akazome Y, Kanda S, et al. Central Distribution of Kiss2 Neurons and Peri-Pubertal Changes in Their Expression in the Brain of Male and Female Red Seabream Pagrus Major. Gen Comp Endocrinol (2012) 175:432–42. doi: 10.1016/j.ygcen.2011.11.038
59. Osugi T, Ohtaki N, Sunakawa Y, Son YL, Ohkubo M, Iigo M, et al. Molecular Evolution of Kiss2 Genes and Peptides in Vertebrates. Endocrinology (2013) 154:4270–80. doi: 10.1210/en.2012-2267
60. Uenoyama Y, Pheng V, Tsukamura H, Maeda KI. The Roles of Kisspeptin Revisited: Inside and Outside the Hypothalamus. J Reprod Dev (2016) 62:537–45. doi: 10.1262/jrd.2016-083
61. Wolfe A, Hussain MA. The Emerging Role(s) for Kisspeptin in Metabolism in Mammals. Front Endocrinol (Lausanne) (2018) 9:184. doi: 10.3389/fendo.2018.00184
62. Fairgrieve MR, Shibata Y, Smith EK, Hayman ES, Luckenbach JA. Molecular Characterization of the Gonadal Kisspeptin System: Cloning, Tissue Distribution, Gene Expression Analysis and Localization in Sablefish (Anoplopoma Fimbria). Gen Comp Endocrinol (2016) 225:212–23. doi: 10.1016/j.ygcen.2015.07.015
63. Saha A, Pradhan A, Sengupta S, Nayak M, Samanta M, Sahoo L, et al. Molecular Characterization of Two Kiss Genes and Their Expression in Rohu (Labeo Rohita) During Annual Reproductive Cycle. Comp Biochem Physiol (2016) 191B:135–45. doi: 10.1016/j.cbpb.2015.10.008
64. Chi L, Li X, Liu Q, Liu Y. Photoperiod Regulate Gonad Development via Kisspeptin/Kissr in Hypothalamus and Saccus Vasculosus of Atlantic Salmon (Salmo Salar). PloS One (2017) 12:e0169569. doi: 10.1371/journal.pone.0169569
65. Mechaly AS, Tovar-Bohórquez MO, Mechaly AE, Suku E, Perez MR, Giorgetti A, et al. Evidence of Alternative Splicing as a Regulatory Mechanism for Kissr2 in Pejerrey Fish. Front Endocrinol (Lausanne) (2018) 9:604. doi: 10.3389/fendo.2018.00604
66. Horikoshi Y, Matsumoto H, Takatsu Y, Ohtaki T, Kitada C, Usuki S. Dramatic Elevation of Plasma Metastin Concentrations in Human Pregnancy: Metastin as a Novel Placenta-Derived Hormone in Humans. J Clin Endocrinol Metab (2003) 88:914–9. doi: 10.1210/jc.2002-021235
67. Oladosu FA, Maixner W, Nackley AG. Alternative Splicing of G Protein–Coupled Receptors: Relevance to Pain Management. Mayo Clin Proc (2015) 90:1135–51. doi: 10.1016/j.mayocp.2015.06.010
68. Nocillado JN, Biran J, Lee YY, Levavi-Sivan B, Mechaly AS, Zohar Y, et al. The Kiss2 Receptor (Kiss2r) Gene in Southern Bluefin Tuna, Thunnus Maccoyii and in Yellowtail Kingfish, Seriola Lalandi - Functional Analysis and Isolation of Transcript Variants. Mol Cell Endocrinol (2012) 362:211–20. doi: 10.1016/j.mce.2012.06.024
69. Onuma TA, Duan C. Duplicated Kiss1 Receptor Genes in Zebrafish: Distinct Gene Expression Patterns, Different Ligand Selectivity, and a Novel Nuclear Isoform With Transactivating Activity. FASEB J (2012) 26:2941–50. doi: 10.1096/fj.11-201095
70. Lareau LF, Brenner SE. Regulation of Splicing Factors by Alternative Splicing and NMD is Conserved Between Kingdoms Yet Evolutionarily Flexible. Mol Biol Evol (2015) 32(4):1072–9. doi: 10.1093/molbev/msv002
71. Yang B, Jiang Q, Chan T, Ko WK, Wong AO. Goldfish Kisspeptin: Molecular Cloning, Tissue Distribution of Transcript Expression, and Stimulatory Effects on Prolactin, Growth Hormone and Luteinizing Hormone Secretion and Gene Expression via Direct Actions at the Pituitary Level. Gen Comp Endocrinol (2010) 165:60–71. doi: 10.1016/j.ygcen.2009.06.001
72. Pasquier J, Lafont AG, Denis F, Lefranc B, Dubessy C, Moreno-Herrera A, et al. Eel Kisspeptins: Identification, Functional Activity, and Inhibition on Both Pituitary LH and GnRH Receptor Expression. Front Endocrinol (Lausanne) (2018) 8:353. doi: 10.3389/fendo.2017.00353
73. Trudeau VL. Kiss and Tell: Deletion of Kisspeptins and Receptors Reveal Surprising Results. Endocrinology (2015) 156:769–71. doi: 10.1210/en.2015-1019
74. Trudeau VL. Facing the Challenges of Neuropeptide Gene Knockouts: Why do They Not Inhibit Reproduction in Adult Teleost Fish? Front Neurosci (2018) 12:302. doi: 10.3389/fnins.2018.00302
75. Grone BP, Maruska KP, Korzan WJ, Fernald RD. Social Status Regulates Kisspeptin Receptor mRNA in the Brain of Astatotilapia Burtoni. Gen Comp Endocrinol (2010) 169:98–107. doi: 10.1016/j.ygcen.2010.07.018
76. Song Y, Duan X, Chen J, Huang W, Zhu Z, Hu W. The Distribution of Kisspeptin (Kiss)1- and Kiss2-Positive Neurons and Their Connections With Gonadotrophin-Releasing Hormone-3 Neurons in the Zebrafish Brain. J Neuroendocrinol (2015) 27:198–211. doi: 10.1111/jne.12251
77. Song Y, Chen J, Tao B, Luo D, Zhu Z, Hu W. Kisspeptin2 Regulates Hormone Expression in Female Zebrafish (Danio Rerio) Pituitary. Mol Cell Endocrinol (2020) 513:110858. doi: 10.1016/j.mce.2020.110858
78. Jiang Q, He M, Ko WK, Wong AO. Kisspeptin Induction of Somatolactin-Alpha Release in Goldfish Pituitary Cells: Functional Role of cAMP/PKA-, PLC/PKC-, and Ca(2+)/calmodulin-Dependent Cascades. Am J Physiol Endocrinol Metab (2014) 307:E872–884. doi: 10.1152/ajpendo.00321.2014
79. Valipour A, Heidari B, Asghari SM, Balalaie S, Rabouti H, Omidian N. The Effect of Different Exogenous Kisspeptins on Sex Hormones and Reproductive Indices of the Goldfish (Carassius Auratus) Broodstock. J Fish Biol (2021) 98(4):1137–43. doi: 10.1111/jfb.14645
80. Valipour A, Heidari B, Vaziri H, Asghari SM. Expression of Reproductive-Related Genes and Changes in Oocyte Maturation of Goldfish Broodstock (Carassius Auratus) Following Injection of Different Exogenous Kisspeptins. Reprod Domest Anim (2021) 56(10):1349–57. doi: 10.1111/rda.13998
81. Zmora N, Stubblefield J, Golan M, Servili A, Levavi-Sivan B, Zohar Y. The Medio-Basal Hypothalamus as a Dynamic and Plastic Reproduction-Related Kisspeptin-Gnrh-Pituitary Center in Fish. Endocrinology (2014) 155:1874–86. doi: 10.1210/en.2013-1894
82. Zmora N, Stubblefield JD, Wong TT, Levavi-Sivan B, Millar RP, Zohar Y. Kisspeptin Antagonists Reveal Kisspeptin 1 and Kisspeptin 2 Differential Regulation of Reproduction in the Teleost, Morone Saxatilis. Biol Reprod (2015) 93:76. doi: 10.1095/biolreprod.115.131870
83. Espigares F, Carrillo M, Gomez A, Zanuy S. The Forebrain-Midbrain Acts as Functional Endocrine Signaling Pathway of Kiss2/Gnrh1 System Controlling the Gonadotroph Activity in the Teleost Fish European Sea Bass (Dicentrarchus Labrax). Biol Reprod (2015) 92:70. doi: 10.1095/biolreprod.114.125138
84. Espigares F, Zanuy S, Gomez A. Kiss2 as a Regulator of Lh and Fsh Secretion via Paracrine/Autocrine Signaling in the Teleost Fish European Sea Bass (Dicentrarchus Labrax). Biol Reprod (2015) 93:114. doi: 10.1095/biolreprod.115.131029
85. Selvaraj S, Ohga H, Kitano H, Nyuji M, Yamaguchi A, Matsuyama M. Peripheral Administration of Kiss1 Pentadecapeptide Induces Gonadal Development in Sexually Immature Adult Scombroid Fish. Zool Sci (2013) 30:446–54. doi: 10.2108/zsj.30.446
86. Selvaraj S, Ohga H, Nyuji M, Kitano H, Nagano N, Yamaguchi A, et al. Subcutaneous Administration of Kiss1 Pentadecapeptide Accelerates Spermatogenesis in Prepubertal Male Chub Mackerel (Scomber Japonicus). Comp Biochem Physiol A (2013) 166:228–36. doi: 10.1016/j.cbpa.2013.06.007
87. Ohga H, Selvaraj S, Adachi H, Imanaga Y, Nyuji M, Yamaguchi A, et al. Functional Analysis of Kisspeptin Peptides in Adult Immature Chub Mackerel (Scomber Japonicus) Using an Intracerebroventricular Administration Method. Neurosci Lett (2014) 561:203–7. doi: 10.1016/j.neulet.2013.12.072
88. Nocillado JN, Zohar Y, Biran J, Levavi-Sivan B, Elizur A. Chronic Kisspeptin Administration Stimulated Gonadal Development in Pre-Pubertal Male Yellowtail Kingfish (Seriola Lalandi; Perciformes) During the Breeding and non-Breeding Season. Gen Comp Endocrinol (2013) 191:168–76. doi: 10.1016/j.ygcen.2013.06.005
89. Wang B, Liu Q, Liu X, Xu Y, Shi B. Molecular Characterization of Kiss2 Receptor and In Vitro Effects of Kiss2 on Reproduction-Related Gene Expression in the Hypothalamus of Half-Smooth Tongue Sole (Cynoglossus Semilaevis). Gen Comp Endocrinol (2017) 249:55–63. doi: 10.1016/j.ygcen.2017.04.006
90. Wang B, Yang G, Xu Y, Zhang Y, Liu X. In Vitro Effects of Tongue Sole LPXRFa and Kisspeptin on Relative Abundance of Pituitary Hormone mRNA and Inhibitory Action of LPXRFa on Kisspeptin Activation in the PKC Pathway. Anim Reprod Sci (2019) 203:1–9. doi: 10.1016/j.anireprosci.2019.01.009
91. Shi Y, Zhang Y, Li S, Liu Q, Lu D, Liu M, et al. Molecular Identification of the Kiss2/Kiss1ra System and its Potential Function During 17alpha-Methyltestosterone-Induced Sex Reversal in the Orange-Spotted Grouper, Epinephelus Coioides. Biol Reprod (2010) 83:63–74. doi: 10.1095/biolreprod.109.080044
92. Park JW, Jin YH, Oh SY, Kwon JY. Kisspeptin2 Stimulates the HPG Axis in Immature Nile Tilapia (Oreochromis Niloticus). Comp Biochem Physiol B (2016) 202:31–8. doi: 10.1016/j.cbpb.2016.07.009
93. Zhang H, Zhang B, Qin G, Li S, Lin Q. The Roles of the Kisspeptin System in the Reproductive Physiology of the Lined Seahorse (Hippocampus Erectus), an Ovoviviparous Fish With Male Pregnancy. Front Neurosci (2018) 12:940. doi: 10.3389/fnins.2018.00940
94. Oliveira CCV, Fatsini E, Fernández I, Anjos C, Chauvigné F, Cerdà J, et al. Kisspeptin Influences the Reproductive Axis and Circulating Levels of microRNAs in Senegalese Sole. Int J Mol Sci (2020) 21:9051. doi: 10.3390/ijms21239051
95. Chaube R, Sharma S, Senthilkumaran B, Bhat SG, Joy KP. Kisspeptins Stimulate the Hypothalamus - Pituitary - Ovarian Axis and Induce Final Oocyte Maturation and Ovulation in Female Stinging Catfish (Heteropneustes Fossilis): Evidence From In Vivo and In Vitro Studies. Aquaculture (2022) 548:737734. doi: 10.1016/j.aquaculture.2021.737734
96. Li W, Hu J, Sun C, Dong J, Liu Z, Yuan J, et al. Characterization of Kiss2/Kissr2 System in Largemouth Bass (Micropterus Salmoides) and Kiss2–10 Peptide Regulation of the Hypothalamic–Pituitary–Gonadal Axis. Comp Biochem Physiol B (2022) 257:110671. doi: 10.1016/j.cbpb.2021.110671
97. Zhao Y, Wayne NL. Effects of Kisspeptin1 on Electrical Activity of an Extrahypothalamic Population of Gonadotropin-Releasing Hormone Neurons in Medaka (Oryzias Latipes). PloS One (2012) 7:e37909. doi: 10.1371/journal.pone.0037909
98. Zhao Y, Lin MC, Mock A, Yang M, Wayne NL. Kisspeptins Modulate the Biology of Multiple Populations of Gonadotropin-Releasing Hormone Neurons During Embryogenesis and Adulthood in Zebrafish (Danio Rerio). PloS One (2014) 9:e104330. doi: 10.1371/journal.pone.0104330
99. Ma XL, Yuan BL, Zhou LB. The Kiss2/GPR54 System Stimulates the Reproductive Axis in Male Black Porgy, Acanthopagrus Schlegelii. Gen Comp Endocrinol (2019) 280:158–67. doi: 10.1016/j.ygcen.2019.04.024
100. Song H, Wang M, Qi J, Wang Z, Zhang Q. Native Recombinant Kisspeptin can Induce Gnrh1 and Kissr2 Expression in Paralichthys Olivaceus In Vitro. Comp Biochem Physiol B (2016) 200:36–43. doi: 10.1016/j.cbpb.2016.05.002
101. Chang JP, Mar A, Wlasichuk M, Wong AO. Kisspeptin-1 Directly Stimulates LH and GH Secretion From Goldfish Pituitary Cells in a Ca(2+)-Dependent Manner. Gen Comp Endocrinol (2012) 179:38–46. doi: 10.1016/j.ygcen.2012.07.028
102. Pasquier J, Lafont AG, Leprince J, Vaudry H, Rousseau K, Dufour S. First Evidence for a Direct Inhibitory Effect of Kisspeptins on LH Expression in the Eel, Anguilla Anguilla. Gen Comp Endocrinol (2011) 173:216–25. doi: 10.1016/j.ygcen.2011.05.019
103. Elizur A, Nocillado J, Biran J, Sivan B, Zohar Y. Advancement of the Onset of Puberty in Seriola Lalandi by Chronic Treatment With Kiss Peptides. Indian J Sci Technol (2011) 4:274–5. doi: 10.17485/ijst/2011/v4is.167
104. Beck BH, Fuller SA, Peatman E, McEntire ME, Darwish A, Freeman DW. Chronic Exogenous Kisspeptin Administration Accelerates Gonadal Development in Basses of the Genus Morone. Comp Biochem Physiol A (2012) 162:265–73. doi: 10.1016/j.cbpa.2012.03.019
105. Chaube R, Sharma S, Senthilkumaran B, Bhat SG, Joy KP. Identification of Kisspeptin2 cDNA in the Catfish Heteropneustes Fossilis: Expression Profile, in Situ Localization and Steroid Modulation. Gen Comp Endocrinol (2020) 294:113472. doi: 10.1016/j.ygcen.2020.113472
106. Marín-Juez R, Viñas J, Mechaly AS, Planas JV, Piferrer F. Stage-Specific Gene Expression During Spermatogenesis in the Senegalese Sole (Solea Senegalensis), a Fish With Semi-Cystic Type of Spermatogenesis, as Assessed by Laser Capture Microdissection and Absolute Quantitative PCR. Gen Comp Endocrinol (2013) 188:242–50. doi: 10.1016/j.ygcen.2013.04.015
107. Mechaly AS, Viñas J, Piferrer F. Sex-Specific Changes in the Expression of Kisspeptin, Kisspeptin Receptor, Gonadotropins and Gonadotropins Receptors in the Senegalese Sole (Solea Senegalensis) During a Full Reproductive Cycle. Comp Biochem Physiol A (2012) 162:364–71. doi: 10.1016/j.cbpa.2012.04.003
108. Selvaraj S, Kitano H, Fujinaga Y, Ohga H, Yoneda M, Yamaguchi A, et al. Molecular Characterization, Tissue Distribution, and mRNA Expression Profiles of Two Kiss Genes in the Adult Male and Female Chub Mackerel (Scomber Japonicus) During Different Gonadal Stages. Gen Comp Endocrinol (2010) 169:28–38. doi: 10.1016/j.ygcen.2010.07.011
109. Shahi N, Singh AK, Sahoo M, Mallik SK, Thakuria D. Molecular Cloning, Characterization and Expression Profile of Kisspeptin1 and Kisspeptin1 Receptor at Brain-Pituitary-Gonad (BPG) Axis of Golden Mahseer, Tor Putitora (Hamilto) During Gonadal Development. Comp Biochem Physiol B (2017) 205:13–29. doi: 10.1016/j.cbpb.2016.11.010
110. Tovar-Bohórquez MO, Mechaly AS, Elisio M, Chalde T, Canosa LF, Miranda LA, et al. Kisspeptins and Their Receptors in the Brain-Pituitary-Gonadal Axis of Odonthestes Bonariensis. Their Relation With Gametogenesis Along the Reproductive Cycle. Gen Comp Endocrinol (2017) 252:209–18. doi: 10.1016/j.ygcen.2017.06.028
111. Singh A, Lal B, Parhar IS, Millar RP. Seasonal Expression and Distribution of Kisspeptin1 (Kiss1) in the Ovary and Testis of Freshwater Catfish, Clarias Batrachus: A Putative Role in Steroidogenesis. Acta Histochem (2021) 123:151766. doi: 10.1016/j.acthis.2021.151766
112. Singh A, Lal B, Parkash J, Millar RP. Gametogenic and Steroidogenic Action of Kisspeptin-10 in the Asian Catfish, Clarias Batrachus: Putative Underlying Mechanistic Cascade. Comp Biochem Physiol B (2021) 256:110642. doi: 10.1016/j.cbpb.2021.110642
113. Kim NN, Choi YU, Park HS, Choi CY. Kisspeptin Regulates the Somatic Growth-Related Factors of the Cinnamon Clownfish Amphiprion Melanopus. Comp Biochem Physiol A (2015) 179:17–24. doi: 10.1016/j.cbpa.2014.08.020
114. Ogawa S, Nathan FM, Parhar IS. Habenular Kisspeptin Modulates Fear in the Zebrafish. Proc Natl Acad Sci USA (2014) 111:3841–6. doi: 10.1073/pnas.1314184111
115. Nathan FM, Ogawa S, Parhar IS. Kisspeptin1 Modulates Odorant-Evoked Fear Response via Two Serotonin Receptor Subtypes (5-HT1A and 5-HT2) in Zebrafish. J Neurochem (2015) 133:870–8. doi: 10.1111/jnc.13105
116. Escobar S, Felip A, Zanuy S, Carrillo M. Is the Kisspeptin System Involved in Responses to Food Restriction in Order to Preserve Reproduction in Pubertal Male Sea Bass (Dicentrarchus Labrax)? Comp Biochem Physiol A (2016) 199:38–46. doi: 10.1016/j.cbpa.2016.05.005
117. Gopurappilly R, Ogawa S, Parhar IS. Functional Significance of GnRH and Kisspeptin, and Their Cognate Receptors in Teleost Reproduction. Front Endocrinol (Lausanne) (2013) 4:24:24. doi: 10.3389/fendo.2013.00024
118. Cheng Z, Garvin D, Paguio A, Stecha P, Wood K, Fan F. Luciferase Reporter Assay System for Deciphering GPCR Pathways. Curr Chem Genomics (2010) 4:84–91. doi: 10.2174/1875397301004010084
119. Son YL, Ubuka T, Soga T, Yamamoto K, Bentley GE, Tsutsui K. Inhibitory Action of Gonadotropin-Inhibitory Hormone on the Signaling Pathways Induced by Kisspeptin and Vasoactive Intestinal Polypeptide in GnRH Neuronal Cell Line, GT1-7. FASEB J (2016) 30:2198–210. doi: 10.1096/fj.201500055
120. Ohga H, Fujinaga Y, Selvaraj S, Kitano H, Nyuji M, Yamaguchi A, et al. Identification, Characterization, and Expression Profiles of Two Subtypes of Kisspeptin Receptors in a Scombroid Fish (Chub Mackerel). Gen Comp Endocrinol (2013) 193:130–40. doi: 10.1016/j.ygcen.2013.07.016
121. Ohga H, Akase F, Sakanoue R, Matsushima A, Ohta K, Matsuyama M. Alanine Scanning and Characterization of Core Peptides in Scombridae Fish Family for Construction of Kiss1 Super Analog. Gen Comp Endocrinol (2020) 288:113356. doi: 10.1016/j.ygcen.2019.113356
122. Felip A, Espigares F, Zanuy S, Gomez A. Differential Activation of Kiss Receptors by Kiss1 and Kiss2 Peptides in the Sea Bass. Reproduction (2015) 150:227–43. doi: 10.1530/REP-15-0204
123. Wang B, Yang G, Liu Q, Qin J, Xu Y, Li W, et al. Inhibitory Action of Tongue Sole LPXRFa, the Piscine Ortholog of Gonadotropin-Inhibitory Hormone, on the Signaling Pathway Induced by Tongue Sole Kisspeptin in COS-7 Cells Transfected With Their Cognate Receptors. Peptides (2017) 95:62–7. doi: 10.1016/j.peptides.2017.07.014
124. Wang B, Yang G, Xu Y, Li W, Liu X. Recent Studies of LPXRFa Receptor Signaling in Fish and Other Vertebrates. Gen Comp Endocrinol (2019) 277:3–8. doi: 10.1016/j.ygcen.2018.11.011
125. Spicer OS, Zmora N, Wong TT, Golan M, Levavi-Sivan B, Gothilf Y, et al. The Gonadotropin-Inhibitory Hormone (Lpxrfa) System’s Regulation of Reproduction in the Brain-Pituitary Axis of the Zebrafish (Danio Rerio). Biol Reprod (2017) 96:1031–42. doi: 10.1093/biolre/iox032
126. Wang B, Yang G, Liu Q, Qin J, Xu Y, Li W, et al. Characterization of LPXRFa Receptor in the Half-Smooth Tongue Sole (Cynoglossus Semilaevis): Molecular Cloning, Expression Profiles, and Differential Activation of Signaling Pathways by LPXRFa Peptides. Comp Biochem Physiol A (2018) 223:23–32. doi: 10.1016/j.cbpa.2018.05.008
127. Castaño JP, Martinez-Fuentes AJ, Gutierrez-Pascual E, Vaudry H, Tena-Sempere M, Malagon MM. Intracellular Signaling Pathways Activated by Kisspeptins Through GPR54: Do Multiple Signals Underlie Function Diversity? Peptides (2009) 30:10–5. doi: 10.1016/j.peptides.2008.07.025
128. Ciani E, Haug TM, Maugars G, Weltzien FA, Falcón J, Fontaine R. Effects of Melatonin on Anterior Pituitary Plasticity: A Comparison Between Mammals and Teleosts. Front Endocrinol (Lausanne) (2021) 11:605111. doi: 10.3389/fendo.2020.605111
129. Kitahashi T, Parhar IS. Comparative Aspects of Kisspeptin Gene Regulation. Gen Comp Endocrinol (2013) 181:197–202. doi: 10.1016/j.ygcen.2012.10.003
130. Martinez-Chavez CC, Minghetti M, Migaud H. GPR54 and Rgnrh I Gene Expression During the Onset of Puberty in Nile Tilapia. Gen Comp Endocrinol (2008) 156:224–33. doi: 10.1016/j.ygcen.2008.01.019
131. Cowan M, Davie A, Migaud H. Photoperiod Effects on the Expression of Kisspeptin and Gonadotropin Genes in Atlantic Cod, Gadus Morhua, During First Maturation. Comp Biochem Physiol A (2012) 163:82–94. doi: 10.1016/j.cbpa.2012.05.191
132. Mitani Y, Kanda S, Akazome Y, Zempo B, Oka Y. Hypothalamic Kiss1 But Not Kiss2 Neurons are Involved in Estrogen Feedback in Medaka (Oryzias Latipes). Endocrinology (2010) 151:1751–9. doi: 10.1210/en.2009-1174
133. Zmora N, Stubblefield J, Zulperi Z, Klenke U, Zohar Y. Kisspeptin-Photoperiod/Gonadal Steroid Relationships in the Brain of Two Perciforms, the Striped Bass and Hybrid Bass. Indian J Sci Technol (2011) 4:10–1. doi: 10.17485/ijst%2F2011%2Fv4iS8%2F30854
134. Carnevali O, Gioacchini F, Maradonna F, Olivotto I, Migliarini B. Melatonin Induces Follicle Maturation in Danio Rerio. PloS One (2011) 6:e19978. doi: 10.1371/journal.pone.0019978
135. Loganathan K, Moriya S, Parhar IS. High Melatonin Conditions by Constant Darkness and High Temperature Differently Affect Melatonin Receptor Mt1 and TREK Channel Trek2a in the Brain of Zebrafish. Zebrafish (2018) 15:473–83. doi: 10.1089/zeb.2018.1594
136. Alvarado MV, Carrillo M, Felip A. Melatonin-Induced Changes in Kiss/Gnrh Gene Expression Patterns in the Brain of Male Sea Bass During Spermatogenesis. Comp Biochem Physiol A (2015) 185:69–79. doi: 10.1016/j.cbpa.2015.03.010
137. Imamura S, Hur SP, Takeuchi Y, Badruzzaman M, Mahardini A, Rizky D, et al. Effect of Short- and Long-Term Melatonin Treatments on the Reproductive Activity of the Tropical Damselfish Chrysiptera Cyanea. Fish Physiol Biochem (2022) 48:253–62. doi: 10.1007/s10695-022-01051-x
138. Espigares F, Rocha A, Gomez A, Carrillo M, Zanuy S. Photoperiod Modulates the Reproductive Axis of European Sea Bass Through Regulation of Kiss1 and Gnrh2 Neuronal Expression. Gen Comp Endocrinol (2017) 240:35–45. doi: 10.1016/j.ygcen.2016.09.007
139. Shahjahan M, Kitahashi T, Ogawa S, Parhar IS. Temperature Differentially Regulates the Two Kisspeptin Systems in the Brain of Zebrafish. Gen Comp Endocrinol (2013) 193:79–85. doi: 10.1016/j.ygcen.2013.07.015
140. Shahjahan M, Kitahashi T, Ando H. Temperature Affects Sexual Maturation Through the Control of Kisspeptin, Kisspeptin Receptor, GnRH and GTH Subunit Gene Expression in the Grass Puffer During the Spawning Season. Gen Comp Endocrinol (2017) 243:138–45. doi: 10.1016/j.ygcen.2016.11.012
141. Navarro VM. Metabolic Regulation of Kisspeptin - the Link Between Energy Balance and Reproduction. Nat Rev Endocrinol (2020) 16(8):407–20. doi: 10.1038/s41574-020-0363-7
142. Luque RM, Kineman RD, Tena-Sempere M. Regulation of Hypothalamic Expression of KiSS-1 and GPR54 Genes by Metabolic Factors: Analyses Using Mouse Models and a Cell Line. Endocrinology (2007) 148:4601–11. doi: 10.1210/en.2007-0500
143. Wahab F, Aziz F, Irfan S, Zaman WU, Shahab M. Short-Term Fasting Attenuates the Response of the HPG Axis to Kisspeptin Challenge in the Adult Male Rhesus Monkey (Macaca Mulatta). Life Sci (2008) 83:633–7. doi: 10.1016/j.lfs.2008.09.001
144. Castellano JM, Navarro VM, Fernández-Fernández R, Nogueiras R, Tovar S, Roa J, et al. Changes in Hypothalamic KiSS-1 System and Restoration of Pubertal Activation of the Reproductive Axis by Kisspeptin in Undernutrition. Endocrinology (2005) 146:3917–25. doi: 10.1210/en.2005-0337
145. Dong TS, Vu JP, Oh S, Sanford D, Pisegna JR, Germano P. Intraperitoneal Treatment of Kisspeptin Suppresses Appetite and Energy Expenditure and Alters Gastrointestinal Hormones in Mice. Dig Dis Sci (2019) 65:2254–63. doi: 10.1007/s10620-019-05950-7
146. Saito R, Tanaka K, Nishimura H, Nishimura K, Sonoda S, Ueno H. Centrally Administered Kisspeptin Suppresses Feeding via Nesfatin-1 and Oxytocin in Male Rats. Peptides (2019) 112:114–24. doi: 10.1016/j.peptides.2018.12.003
147. Stengel A, Wang L, Goebel-Stengel M, Tache Y. Centrally Injected Kisspeptin Reduces Food Intake by Increasing Meal Intervals in Mice. Neuroreport (2011) 22:253–7. doi: 10.1097/WNR.0b013e32834558df
148. Talbi R, Laran-Chich MP, Magoul R, El Ouezzani S, Simonneaux V. Kisspeptin and RFRP-3 Differentially Regulate Food Intake and Metabolic Neuropeptides in the Female Desert Jerboa. Sci Rep (2016) 6:36057. doi: 10.1038/srep36057
149. Kanda S, Karigo T, Oka Y. Steroid Sensitive Kiss2 Neurones in the Goldfish: Evolutionary Insights Into the Duplicate Kisspeptin Gene-Expressing Neurones. J Neuroendocrinol (2012) 24:897–906. doi: 10.1111/j.1365-2826.2012.02296.x
150. Guo Y, Wang Q, Li G, He M, Tang H, Zhang H. Molecular Mechanism of Feedback Regulation of 17beta-Estradiol on Two Kiss Genes in the Protogynous Orange-Spotted Grouper (Epinephelus Coioides). Mol Reprod Dev (2017) 84:495–507. doi: 10.1002/mrd.22800
151. Nocillado JN, Mechaly AS, Elizur A. In Silico Analysis of the Regulatory Region of the Yellowtail Kingfish and Zebrafish Kiss and Kiss Receptor Genes. Fish Physiol Biochem (2013) 39:59–63. doi: 10.1007/s10695-012-9642-0
152. Wang Q, Sham KW, Ogawa S, Li S, Parhar IS, Cheng CH. Regulation of the Two Kiss Promoters in Goldfish (Carassius Auratus) by Estrogen via Different ERalpha Pathways. Mol Cell Endocrinol (2013) 375:130–9. doi: 10.1016/j.mce.2013.04.023
153. Imamura S, Hur SP, Takeuchi Y, Badruzzaman M, Mahardini A, Rizky D, et al. The mRNA Expression Patterns of Kisspeptins, GnRHs, and Gonadotropins in the Brain and Pituitary Gland of a Tropical Damselfish, Chrysiptera Cyanea, During the Reproductive Cycle. Fish Physiol Biochem (2020) 46:277–91. doi: 10.1007/s10695-019-00715-5
154. Yue HM, Ye H, Ruan R, Du H, Li CJ, Wei Q. Feedback Regulation of 17beta-Estradiol on Two Kisspeptin Genes in the Dabry’s Sturgeon (Acipenser Dabryanus). Comp Biochem Physiol B (2019) 230:1–9. doi: 10.1016/j.cbpb.2019.01.006
155. Imamura S, Hur SP, Takeuchi Y, Bouchekioua S, Takemura A. Molecular Cloning of Kisspeptin Receptor Genes (Gpr54-1 and Gpr54-2) and Their Expression Profiles in the Brain of a Tropical Damselfish During Different Gonadal Stages. Comp Biochem Physiol A (2017) 203:9–16. doi: 10.1016/j.cbpa.2016.07.015
156. Wang B, Liu Q, Liu X, Xu Y, Song X, Shi B. Molecular Characterization of Kiss2 and Differential Regulation of Reproduction-Related Genes by Sex Steroids in the Hypothalamus of Half-Smooth Tongue Sole (Cynoglossus Semilaevis). Comp Biochem Physiol A (2017) 213:46–55. doi: 10.1016/j.cbpa.2017.08.003
157. Alvarado MV, Servili A, Moles G, Gueguen MM, Carrillo M, Kah O, et al. Actions of Sex Steroids on Kisspeptin Expression and Other Reproduction-Related Genes in the Brain of the Teleost Fish European Sea Bass. J Exp Biol (2016) 219:3353–65. doi: 10.1242/jeb.137364
158. Klenke U, Zmora N, Stubblefield J, Zohar Y. Expression Patterns of the Kisspeptin System and GnRH1 Correlate in Their Response to Gonadal Feedback in Female Striped Bass. Indian J Sci Technol (2011) 4:33–4. doi: 10.17485/ijst/2011/v4is.29
159. Golshan M, Habibi HR, Alavi SM. Transcripts of Genes Encoding Reproductive Neuroendocrine Hormones and Androgen Receptor in the Brain and Testis of Goldfish Exposed to Vinclozolin, Flutamide, Testosterone, and Their Combinations. Fish Physiol Biochem (2016) 42:1157–65. doi: 10.1007/s10695-016-0205-7
160. Ogawa S, Ng KW, Ramadasan PN, Nathan FM, Parhar IS. Habenular Kiss1 Neurons Modulate the Serotonergic System in the Brain of Zebrafish. Endocrinology (2012) 153:2398–407. doi: 10.1210/en.2012-1062
161. Paullada-Salmeron JA, Cowan M, Aliaga-Guerrero M, Morano F, Zanuy S, Munoz-Cueto JA. Gonadotropin Inhibitory Hormone Down-Regulates the Brain-Pituitary Reproductive Axis of Male European Sea Bass (Dicentrarchus Labrax). Biol Reprod (2016) 94:121. doi: 10.1095/biolreprod.116.139022
162. Paullada-Salmeron JA, Cowan M, Aliaga-Guerrero M, Lopez-Olmeda JF, Mananos EL, Zanuy S, et al. Testicular Steroidogenesis and Locomotor Activity are Regulated by Gonadotropin-Inhibitory Hormone in Male European Sea Bass. PloS One (2016) 11:e0165494. doi: 10.1371/journal.pone.0165494
163. Liu Q, Wang B, Liu X, Xu Y, Shi B, Liu Z. Effects of Gonadotropin-Inhibitory Hormone Peptides on the Reproduction-Related Gene Expression in the Hypothalamus of Half-Smooth Tongue Sole (Cynoglossus Semilaevis). Prog Fish Sci (2017) 38:56–62. doi: 10.11758/yykxjz.20160805001
164. Aliaga-Guerrero M, Paullada-Salmeron JA, Piquer V, Mananos EL, Munoz-Cueto JA. Gonadotropin-Inhibitory Hormone in the Flatfish, Solea Senegalensis: Molecular Cloning, Brain Localization and Physiological Effects. J Comp Neurol (2018) 526:349–70. doi: 10.1002/cne.24339
165. Wang Q, Qi X, Guo Y, Li S, Zhang Y, Liu X, et al. Molecular Identification of GnIH/GnIHR Signal and its Reproductive Function in Protogynous Hermaphroditic Orange-Spotted Grouper (Epinephelus Coioides). Gen Comp Endocrinol (2015) 216:9–23. doi: 10.1016/j.ygcen.2015.04.016
166. Habibi HR, Nelson ER, Allan ER. New Insights Into Thyroid Hormone Function and Modulation of Reproduction in Goldfish. Gen Comp Endocrinol (2012) 175:19–26. doi: 10.1016/j.ygcen.2011.11.003
167. Tovo-Neto A, da Silva Rodrigues M, Habibi HR, Nobrega RH. Thyroid Hormone Actions on Male Reproductive System of Teleost Fish. Gen Comp Endocrinol (2018) 265:230–6. doi: 10.1016/j.ygcen.2018.04.023
168. Ogawa S, Ng KW, Xue X, Ramadasan PN, Sivalingam M, Li S, et al. Thyroid Hormone Upregulates Hypothalamic Kiss2 Gene in the Male Nile Tilapia, Oreochromis Niloticus. Front Endocrinol (Lausanne) (2013) 4:184. doi: 10.3389/fendo.2013.00184
169. Waye A, Trudeau VL. Neuroendocrine Disruption: More Than Hormones are Upset. J Toxicol Environ Health B (2011) 14:270–91. doi: 10.1080/10937404.2011.578273
170. Faheem M, Jahan N, Khaliq S, Lone KP. Modulation of Brain Kisspeptin Expression After Bisphenol-A Exposure in a Teleost Fish, K. Fish Physiol Biochem (2019) 45:33–42. doi: 10.1007/s10695-018-0532-y
171. Qiu W, Fang M, Liu J, Fu C, Zheng C, Chen B, et al. In Vivo Actions of Bisphenol F on the Reproductive Neuroendocrine System After Long-Term Exposure in Zebrafish. Sci Total Environ (2019) 665:995–1002. doi: 10.1016/j.scitotenv.2019.02.154
172. Yue Z, Yu M, Zhao H, Wang J, Zhang X, Tian H, et al. The Anti-Estrogenicity of Chronic Exposure to Semicarbazide in Female Japanese Flounders (Paralichthys Olivaceus), and its Potential Mechanisms. Mar Pollut Bull (2018) 129:806–12. doi: 10.1016/j.marpolbul.2017.10.081
173. Yue Z, Yu M, Zhang X, Wang J, Ru S. The Anti-Androgenic Effect of Chronic Exposure to Semicarbazide on Male Japanese Flounder (Paralichthys Olivaceus) and its Potential Mechanisms. Comp Biochem Physiol C (2018) 210:30–4. doi: 10.1016/j.cbpc.2018.04.005
174. Smith CM, Vera MKM, Bhandari RK. Developmental and Epigenetic Effects of Roundup and Glyphosate Exposure on Japanese Medaka (Oryzias Latipes). Aquat Toxicol (2019) 210:215–26. doi: 10.1016/j.aquatox.2019.03.005
175. Mitchell K, Zhang WS, Lu C, Tao B, Chen L, Hu W, et al. Targeted Mutation of Secretogranin-2 Disrupts Sexual Behavior and Reproduction in Zebrafish. Proc Natl Acad Sci USA (2020) 117:12772–83. doi: 10.1073/pnas.2002004117
176. Tanaka S, Zmora N, Levavi-Sivan B, Zohar Y. Vasoactive Intestinal Peptide Indirectly Elicits Pituitary Lh Secretion Independent of GnRH in Female Zebrafish. Endocrinology (2022) 163:113. doi: 10.1210/endocr/bqab264
177. Trudeau VL. Neuroendocrine Control of Reproduction in Teleost Fish: Concepts and Controversies. Annu Rev Anim Biosci (2022) 10:18.11–24. doi: 10.1146/annurev-animal-020420-042015
Keywords: fish, kisspeptin, kisspeptin receptor, reproduction, signaling pathway, gene regulation
Citation: Wang B, Mechaly AS and Somoza GM (2022) Overview and New Insights Into the Diversity, Evolution, Role, and Regulation of Kisspeptins and Their Receptors in Teleost Fish. Front. Endocrinol. 13:862614. doi: 10.3389/fendo.2022.862614
Received: 26 January 2022; Accepted: 21 February 2022;
Published: 22 March 2022.
Edited by:
Chad D. Foradori, Auburn University, United StatesReviewed by:
Hélène Volkoff, Memorial University of Newfoundland, CanadaKataaki Okubo, The University of Tokyo, Japan
Ya-Xiong Tao, Auburn University, United States
Copyright © 2022 Wang, Mechaly and Somoza. This is an open-access article distributed under the terms of the Creative Commons Attribution License (CC BY). The use, distribution or reproduction in other forums is permitted, provided the original author(s) and the copyright owner(s) are credited and that the original publication in this journal is cited, in accordance with accepted academic practice. No use, distribution or reproduction is permitted which does not comply with these terms.
*Correspondence: Bin Wang, wangbin@ysfri.ac.cn; Alejandro S. Mechaly, amechaly@inbiotec-conicet.gob.ar; Gustavo M. Somoza, somoza@intech.gov.ar
†These authors have contributed equally to this work