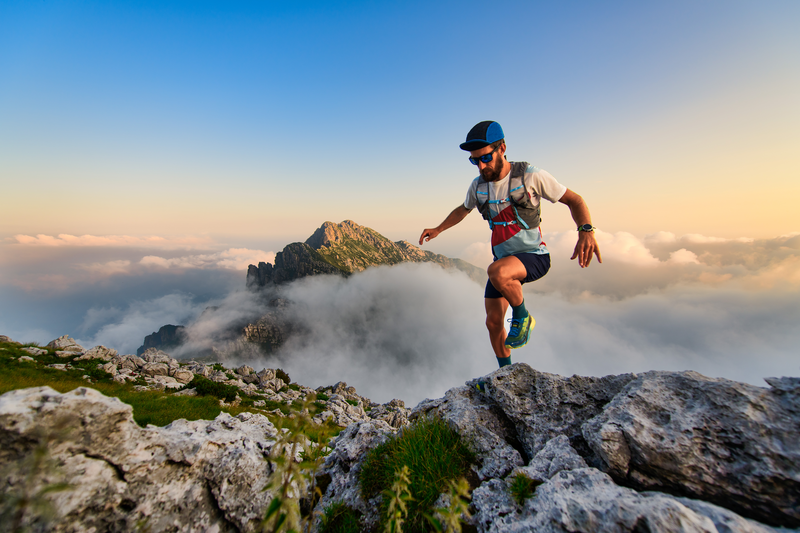
94% of researchers rate our articles as excellent or good
Learn more about the work of our research integrity team to safeguard the quality of each article we publish.
Find out more
ORIGINAL RESEARCH article
Front. Endocrinol. , 16 March 2022
Sec. Cellular Endocrinology
Volume 13 - 2022 | https://doi.org/10.3389/fendo.2022.861452
This article is part of the Research Topic Advances of Endocrine and Metabolic Cardiovascular Outcomes: From Basic to Clinical Science View all 15 articles
Diabetic retinopathy (DR), a microvascular complication of diabetes mellitus, is the leading cause of vision loss in the working-age population worldwide. Unfortunately, current clinical treatments cannot completely prevent the occurrence and development of DR. Salidroside (Sal) is a medicinal supplement that has antioxidative and cytoprotective properties. This study aimed to investigate the therapeutic effect of Sal on DR. Briefly, Sal treatment was applied to wide-type mice and db/db mice (a widely used diabetic mice) at 25 mg/kg by oral gavage once daily from 8 weeks to 20 weeks. Mice’s bodyweight, blood glucose, total cholesterol, triglyceride, high density lipoprotein and low density lipoprotein were recorded and analyzed. Retinal trypsin digestion and evans blue dye assay were used to detect retinal microvessel changes and function. Retinal glutathione and malondialdehyde content measurements were applied to assess retinal oxidative stress. Full-length transcriptome analysis was performed to explore the underlying mechanisms of Sal protection. Our results found that Sal treatment could successfully relieve blood glucose and blood lipid abnormalities, and reduce retinal oxidative stress level in diabetic mice. Also, Sal treatment repaired the abnormal transcriptome caused by diabetes, alleviated the microvascular lesion of the fundus in diabetic mice, and protected retinal normal barrier function. This study enriches the indications of Sal in the treatment of diabetic diseases, providing practical research ideas for the comprehensive preventions and treatments of DR.
Diabetes mellitus (DM) is a group of metabolic diseases characterized by hyperglycemia, insulin resistance, and impaired insulin secretion. According to the International Diabetes Federation (IDF) statistics, the number of diabetic patients globally is around 463 million people, and it is expected to achieve 700 million in 2045 (1). As one of the most common microvascular complications of DM, diabetic retinopathy (DR) has affected 50% of type 1 diabetes and 30% of type 2 diabetes worldwide (2), and it is the leading cause of vision loss in the working-age population (3). Especially in the last 30 years, due to the aging population and the changes in lifestyles, the number of patients with type 2 diabetes has increased sharply, and the corresponding incidence rate has also shown a significantly increasing trend. The global age-standardized prevalence for blindness caused by DR has increased from 14.9% in 1990 to 18.5% in 2020 (4), indicating an urgent and severe need for DR prevention and treatment.
Retina is the only part of the body where the arteries, veins and capillaries can be directly observed with the naked eye. Retinal vascular system can effectively reflect the systemic blood circulation and microvascular changes under DM condition (5, 6). Damage to the retinal vascular system is an essential feature of the pathogenesis of DR. The typical morphological characteristics include loss of pericytes, thickening of the basement membrane, increased vascular permeability, vascular occlusion, and microaneurysms (7, 8). Retinal inflammation, oxidative stress, and glial cell dysfunction are the main pathogenic factors of DR injury (9, 10). These factors can induce damages such as destructive retinal vascular barrier, abnormal angiogenesis, and impaired retinal ganglion cell, ultimately leading to decreased vision and blindness in patients (8). So far, the clinical treatments for DR mainly include retinal laser photocoagulation, intravitreal anti-VEGF injection, sustained released dexamethasone (Ozurdex) implant, and vitreoretinal surgery (11–13). However, these treatments cannot completely prevent the occurrence and development of DR. Many patients still experience deteriorations and eventually develop blindness after clinical treatments (14). Therefore, in-depth studying the pathogenesis of DR, discovering new strategies and targets for DR treatment have crucial clinical and social significance for the prognosis of DR patients and the alleviation of medical burden (15).
Rhodiola Rosea is a precious functional medicinal plant. In China and other Asian countries, it is often used as a commercial dietary supplement to treat stress, fatigue, and altitude sickness (16–18). Salidroside (Sal) is a biologically active ingredient extracted from the Rhodiola plant, with potent antioxidant, anti-inflammatory and neuroprotective effects (19–21). This pharmacological feature can effectively target the retinal oxidative stress, retinal inflammation and nerve cell damage caused by DR. In addition, recent studies have shown that Sal can reduce the blood glucose level in diabetic mice by increasing insulin sensitivity and reducing ß-cell loss, effectively lowering diabetic deteriorations (22–24). However, the role of Sal in DR is still unclear.
In this study, we revealed that long-term application of Sal could both reduce the blood glucose levels and blood lipid levels in diabetic mice and significantly alleviate DR-induced vascular leakage and microangiopathy. Moreover, Sal treatment greatly reversed the changes in the retinal transcriptome caused by DM. All these results highlight the potential value of Sal as a dietary supplement for DR control.
Male wide-type C57BLKS/J mice (WT, 20-30 g, 8 weeks) and C57BLKS/J db/db mice (40-60 g, 8 weeks, genetically diabetic leptin receptor-mutated mice charactered by spontaneous obesity and hyperglycemia) were purchased from Nanjing Biomedical Research Institute of Nanjing University (Nanjing, China) and housed in a comfortable environment at 22 ± 2°C with 12 hours light/dark cycle. All the experimental procedures were approved by the Animal Ethical Committee of Xiangya hospital, Central South University (Changsha, China).
In total, 21 WT mice and 24 db/db mice were used in the study. Based on previous study (25), Sal treatment was administered at 25 mg/kg by oral gavage once daily, while the control group received water. Before the experiment, the mice were kept for 3 days to acclimate. Then WT mice and db/db mice were divided into four groups: WT group (WT mice treated with water; n = 12), WT + Sal group (WT mice treated with 25 mg/kg Sal; n = 9); db/db group (db/db mice treated with water; n = 12), db/db + Sal group (db/db mice treated with 25 mg/kg Sal; n = 12).
Before blood samples measurement, mice were deprived of food and water for 6 hours to maintain a fasting state. Blood glucose levels in the caudal veins were measured every 4 weeks using an automatic blood glucose mete (Accu-Chek; Roche, Mannheim, Germany). Blood lipid levels, including total cholesterol (TC), triglyceride (TG), high density lipoprotein (HDL) and low density lipoprotein (LDL), were determined using an automatic blood lipid mete (CCM-111; On·call, Hangzhou, China) 12 weeks after Sal treatment. All measurements were performed and calculated according to the instructions.
Fresh dissected retinas were fixed in 4% paraformaldehyde for 1 hour and divided into four quadrants at the optic disk. Then retinas were digested in 3% Trypsin, 0.1 M Tris (pH 7.8) for 3 hours at 37°C until the medium became cloudy. After the incubation, the internal limiting membrane and disintegrated neuronal tissue were carefully removed, and then the remaining retinal vasculature was flattened on a glass slide for air dry. At last, air-dried retinal vasculature was treated with periodic acid solution and stained with Schiff’s reagent and hematoxylin (C0142S; Solarbio, Beijing, China). A digital imaging system was used to observe the histological change of retinal vasculature. All measurements were conducted in a masked manner and observed under the bright field.
Retinal vascular barrier function was determined by evans blue dye assay. Briefly, 30 mg/kg evans blue was injected through the caudal vein. Two hours later, mouse was anesthetized and perfused with 10 ml PBS to flush blood out of circulation. The retinas were collected and fixed in 4% paraformaldehyde for 1 hours. The left retina was flattened on a glass slide and observed under 652 nm excitation using a fluorescence microscope (DM5000 B; Leica, Wetzlar, Germany), the right retina was incubated with 0.3 ml formamide overnight at 70°C and centrifuged at 12,000 g for 15 minutes to extract evans blue from the retina. The absorbance of extract was determined with a spectrophotometer at 620 nm, the concentration of evans blue dye in retinal extract was calculated from the standard curve of evans blue in formamide and normalized to the dry retinal weight.
Malondialdehyde (MDA) and glutathione (GSH) are crucial biochemical indicators reflecting oxidative stress levels. The retinal MDA content was measured using a Lipid Peroxidation MDA Assay Kit (S0131S; Beyotime, Shanghai, China). The retinal GSH content was measured using a Micro Reduced GSH Assay Kit (BC1175; Solarbio, Beijing, China). The measured contents were calibrated using the samples’ protein concentration.
Mouse retinas were collected 12 weeks after Sal intervention. Two individual retinas were pooled and treated as one sample; each group contained 3 samples. TRIzol (Invitrogen, Carlsbad, CA, USA) was used to isolate total RNA, cDNA-PCR Sequencing Kit (SQK-PCS109, Oxford Nanopore Technologies Ltd, Oxford, UK) was applied to convert total RNA to cDNA. The final cDNA was then run on the PromethION platform at the Biomarker Technology Company (Beijing, China). Genes with a fold-change ≥ 1.5 identified by edgeR and a false discovery rate (FDR) < 0.05 were considered differentially expressed (BMKCloud, http://www.biocloud.net/). Gene functional annotation were based on the following databases: KEGG (Kyoto Encyclopedia of Genes and Genomes, https://www.genome.jp/kegg/) and GO (Gene Ontology, http://www.geneontology.org/).
SPSS version 22.0 (IBM, Armonk, NY, USA) was used for the statistical analyses. Data are expressed as the mean ± standard deviation (SD). One-way analysis of variance (ANOVA) followed by Tukey’s post hoc test were used for comparisons between two sets. Statistical significance was set at p < 0.05.
Overweight and hyperglycemia are typical features in db/db mice. As shown in Figures 1A, B and Supplementary Table 1, db/db mice showed a significant increase in bodyweight and blood glucose level compared with those in WT mice, and these pathological changes were more obvious along with aging. At week 16 and week 20, the blood glucose levels of db/db mice were nearly five times higher than that of WT mice, but such an alteration was effectively alleviated by Sal intervention. In addition, dyslipidemia is also an important feature that distinguishes db/db mice from WT mice. At 20 weeks, the contents of TC, TG, HDL and LDL in the blood of db/db mice were all greatly higher than those of WT mice. However, Sal treatment effectively improved these lipid profiles (Figures 1C–F and Supplementary Table 1). Despite Sal’s powerful function in regulating blood glucose and lipids in db/db mice, its therapeutic effect on normal WT mice was not found (Figure 1 and Supplementary Table 1). Taken together, these data suggested that the long-term oral administration of Sal improved blood glucose and lipid profiles in db/db mice, indicating that the Sal is an effective and safe drug in treating dysglycemia and dyslipidemia caused by diabetes.
Figure 1 Sal improved blood glucose and lipid profiles in db/db mice. (A) The bodyweight of WT and db/db mice after Sal intervention. (B) The blood glucose of WT and db/db mice after Sal intervention. (C-F) The blood levels of total cholesterol (TC), high density lipoprotein (HDL), triglyceride (TG) and low density lipoprotein (LDL) in WT and db/db mice after Sal intervention. Data are the mean ± SD; ##p < 0.01 (db/db mice compared with WT mice), *p < 0.05, **p < 0.01 (db/db + Sal mice compared with db/db mice).
Retinal microvascular changes are the early feature DR (26). To investigate the role of Sal on DR, we quantified the number of retinal pericyte ghosts and acellular capillaries in db/db mice after Sal administration. The results showed that retinal pericyte ghosts and acellular capillaries were greatly increased in db/db mice when compared with WT mice (Figure 2 and Supplementary Table 1). However, Sal treatment significantly blunted the pericyte dropout and retinal acellular capillary formation in db/db mice without obvious influence on WT mice (Figure 2 and Supplementary Table 1). These results demonstrated that Sal intervention alleviated retinal microvascular changes, suggesting a therapeutic effect of Sal on early DR.
Figure 2 Sal alleviated retinal microvascular changes in db/db mice. (A) The representative photomicrographs of normal and diabetic microvessel stained by Schiff’s reagent and hematoxylin; The green arrows pointed is acellular capillaries, and the red arrows pointed is pericyte ghost. (B) The number of pericyte ghost in WT and db/db mice after Sal intervention. (C) The number of acellular capillaries in WT and db/db mice after Sal intervention. Data are the mean ± SD. Scale bar = 50 μm.
Pericyte loss and acellular capillaries formation could alter retinal vascular permeability and lead to vascular leakage (27). To further determine the therapeutic effect of Sal on early DR, evans blue assays were applied to assess retinal vascular barrier function in db/db mice. As shown in Figure 3A, db/db mice exhibited more evans blue leakage area in the retina compared with WT mice, and Sal treatment markedly alleviated this leakage extent. Evans blue quantitative results also revealed that Sal treatment greatly decreased retinal evans blue leakage levels in diabetic conditions (Figure 3B and Supplementary Table 1). All these results suggested that Sal protects retinal vascular barrier function in db/db mice, indicating a therapeutic effect on diabetes-induced retinal vascular dysfunction.
Figure 3 Sal protected retinal vascular barrier function in db/db mice. (A) The representative photomicrographs of retinal vessels stained by evans blue in WT mice and db/db mice with or without Sal intervention. (B) Retinal normalized evans blue contents in WT and db/db mice after Sal intervention. Data are the mean ± SD. Scale bar = 500 μm.
Previous studies have demonstrated that oxidative stress plays a crucial role in DR pathogenesis (28). Our results found that retinal GSH levels (an important biochemical indicator reflecting cellular anti-oxidative stress ability) were decreased in db/db mice, while Sal intervention restored retinal GSH levels in diabetic conditions (Figures 4A and Supplementary Table 1). Moreover, retinal MDA contents, a metabolite of oxidative stress, were increased in diabetic mice and were recovered to normal levels after Sal treatment (Figures 4B and Supplementary Table 1). It was noteworthy that Sal had no effect on GSH and MDA contents in WT mice. All these results suggested that Sal relieves retinal oxidative stress in db/db mice, showing an excellent antioxidant property in DR.
Figure 4 Sal relieved retinal oxidative stress in db/db mice. (A) Retinal GSH contents in WT and db/db mice after Sal intervention. (B) Retinal MDA contents in WT and db/db mice after Sal intervention. Data are the mean ± SD.
To determine the underlying mechanism of Sal treatment on early DR, full-length transcriptome analysis was performed on the retina of WT, db/db and Sal-treated db/db mice at 12 weeks after intervention. As shown in Figure 5A, there were 208 up-regulated differentially expressed genes (DEGs) and 182 down-regulated DEGs between WT mice and db/db mice, and 144 up-regulated DEGs and 133 down-regulated DEGs between db/db mice and Sal-treated db/db mice. Among these diabetes-induced DEGs, 14 up-regulated DEGs and 21 down-regulated DEGs were completely restored by Sal treatment, and only one DEGs (Tmem252) was aggravated after Sal intervention (Figures 5A, B). Except for the completely restored DEGs, Sal treatment mitigated the expression of approximately 73.08% up-regulated DEGs and 66.35% down-regulated DEGs induced by diabetes, including 8 oxidation-related genes (Aldh4a1, Acad12, Ado, Kdm4d, Acacb, Mical1, Th and Htatip2) (Supplementary Figure 1). These results suggested that Sal exhibits its powerful DR therapeutic effect largely depending on ameliorating retinal transcriptome abnormalities.
Figure 5 Sal ameliorates retinal transcriptome abnormalities in db/db mice. (A) The Venn diagram showing the differentially expressed genes (DEGs) among WT mice, db/db mice and Sal-treated db/db mice. (B) The heat map showing the Sal-restored DEGs (35 genes) and Sal-aggravated DEGs (1 gene) between WT and db/db mice.
To systematically identify the retinal biological process and pathways associated with Sal treatment, we performed GO and KEGG analysis on the up-regulated DEGs and down-regulated DEGs between db/db mice and Sal-treated db/db mice (Figure 6). KEGG analysis indicated that the AMPK signaling pathway and the PI3K-Akt signaling pathway were two crucial pathways after Sal treatment, as DEGs were mainly concentrated in these two pathways (Figure 6A).GO analysis results showed that the retinal biological process, cellular component, and molecular function were all altered by Sal intervention. The most DEGs were enriched in biological process, and the less DEGs were enriched in molecular function (Figure 6B).
Figure 6 The analysis of DEGs between db/db mice and Sal-treated db/db mice. (A) The DEGs between db/db mice and Sal-treated db/db mice were analysed by KEGG databases. (B) The DEGs between db/db mice and Sal-treated db/db mice were analysed by GO databases.
DR is one of the most destructive microvascular complications of diabetes, which can lead to irreversible retinal damage (1). Although DR is not a fatal disease, it significantly influences the patient’s vision, inconveniences the patient’s daily life, and affects people’s quality of life. In addition, the cost of DR care and treatment has become an important source of medical burden for families and society. The prevention and treatment of DR has been unsatisfactory for many years. Existing clinical treatment methods have not benefited all patients, and there is yet a need to discover new therapeutic targets for DR (14, 15).
So far, DR is divided into two stages based on the degree of microvascular disease: early non-proliferative diabetic retinopathy (NPDR) and late proliferative diabetic retinopathy (PDR) (29). Patients with NPDR have no obvious symptoms in the early stage. Microaneurysm, capillary leakage and local bleeding points can be seen by fundus examination. These microvascular abnormalities increase the permeability of retinal blood vessels, causing retinal edema and the symptoms of blurred vision in patients. This stage is the best time to prevent and treat DR clinically (30). With the progression of DR, patients with NPDR may have abnormal neovascularization in the fundus while entering the PDR stage. Because of the greater fragility of neovascularization, vitreous hemorrhage is likely to be caused by easy rupture and leakage. In addition, secondary formation of retinal fibroproliferative membrane and tractional retinal detachment will induce permanent damage to patients’ vision, leading to blindness (31). At this stage, the treatment cost is higher, while the treatment outcome is far inferior to the NPDR stage (32). Therefore, early intervention, prevention and control of DR is important.
In this study, we started by giving 25 mg/kg of Sal oral treatment for diabetic mice at the early stage of abnormal blood glucose to realize the effective prevention and the control of DR through the early intervention of drugs. The results demonstrated that Sal treatment can effectively reduce the blood glucose and the blood lipid levels of diabetic mice, which is consistent with the results of some previous studies (22, 23, 25). The mechanism of its occurrence may be related to Sal improving the insulin resistance of mice and increasing the survival of pancreatic islet b cells (22). Our results also found that Sal treatment can effectively improve the fundus microvascular disease in diabetic mice, protect the blood-retinal barrier function and reduce microvascular leakage. This may benefit from regulating blood glucose and blood lipids by Sal. Many studies have shown that hyperglycemia and hyperlipidemia are substantial causes of DR through various pathways, such as protein kinase C (PKC), polyol, and hexosamine (33–35). Also, the advanced glycation end products (AGEs) induce apoptosis of vascular endothelial cells, pericytes and nerve tissues, leading to the occurrence of DR (36). Thus, the application of hypoglycemic drugs and hypolipidemic drugs can reduce the incidence of DR.
Although hyperglycemia is an important cause of DR, the impact of simply lowering blood glucose in controlling the progression of DR is limited. A large sample of clinical studies has shown that the active control of blood glucose has not significantly affected the progression of DR in patients with type 2 diabetes (37). Further, intensive blood glucose control has no remarkable benefit in improving the prognosis of DR (38). In fact, abnormal glucose and lipid metabolism in diabetic patients can interfere with the normal redox reaction in the cell, leading to an increment in ROS and oxidative stress (26). Increased ROS further induces epigenetic changes in mitochondrial enzymes, forming a metabolic memory that is unable to alleviate the symptoms of DR even when blood glucose is controlled to a normal level (39, 40). Thus, in the early stage of DR, reducing the level of oxidative stress in the retina is the key to preventing the progression of DR. As a natural antioxidant, Sal has been widely concerned about its antioxidant function (19, 41) In the retina, Sal has been proved to prevent the pigment epithelial cell damage induced by hydrogen peroxide and the vascular endothelial cell damage caused by hypoxia through reducing oxidative stress (42–45). This statement is consistent with the result of the excellent antioxidant properties of Sal in DR in this study, illustrating that the improvement of fundus microvascular disease by Sal in diabetic mice is partially due to its antioxidant activity.
The sequencing results of this study found that Sal can significantly repair the abnormal transcriptome expression caused by diabetes. Among the 390 differential genes in db/db mice and normal wild-type mice, about 70% were improved to varying degrees after the Sal treatment, 9% of which completely repaired, and only less than 0.3% of which deteriorated. This result indicated the powerful role of Sal treating DR from another perspective. Previous studies have confirmed that the P13K-AKT and AMPK signalling pathways are crucial for Sal to exert therapeutic effects by activating the above pathways to reduce diabetic-induced damages in the heart, liver, and kidney (24, 25, 46, 47). In our study, the sequencing results displayed that the retina after Sal treatment was also accompanied by the enrichment of genes related to the P13K-AKT and AMPK signalling pathways, suggesting the DR protective effect of Sal is likely to depend on the above pathways. However, the specific mechanism of action needs further studies in the future.
This study explored the effects of oral Sal administration on the prevention and treatment of early diabetes and DR. Our results depicted that a long-term and low-dose oral Sal treatment can successfully relieve blood glucose and blood lipid abnormalities and reduce the oxidative stress level in the retina in diabetic mice. Also, Sal treatment repairs the abnormal transcriptome caused by diabetes, alleviates the microvascular disease of the fundus in diabetic mice, and protects the normal retinal barrier function. This study enriches the indications of Sal in the treatment of diabetic diseases, providing practical research ideas for the comprehensive preventions and treatments of DR.
The datasets presented in this study can be found in online repositories. The names of the repository/repositories and accession number(s) can be found below: SRA, PRJNA800617.
The animal study was reviewed and approved by Animal Ethical Committee of Xiangya Hospital, Central South University.
FY wrote the first draft of the paper. XJ and WZ edited the paper. FY, XX, and LD designed research. FY, WZ, LQ, and ZP performed research. FY analyzed data. All authors contributed to the article and approved the submitted version.
This work was supported by the National Natural Science Foundation of China (grant numbers 81974134 and 82171058 to XX; 82070966 to LD), the National key research and development program of China (grant numbers 2020YFC2008205 to XX), the Key R & D plan of Hunan Province of China (grant numbers 2020SK2076 to XX), the Natural Science Foundation of Hunan Province (grant numbers 2019JJ40474 to XX) and the Science and Technology Innovation Program of Hunan Province (grant numbers 2021RC3026 to LD).
The authors declare that the research was conducted in the absence of any commercial or financial relationships that could be construed as a potential conflict of interest.
All claims expressed in this article are solely those of the authors and do not necessarily represent those of their affiliated organizations, or those of the publisher, the editors and the reviewers. Any product that may be evaluated in this article, or claim that may be made by its manufacturer, is not guaranteed or endorsed by the publisher.
We thank Mingfang Xia, Manjuan Peng, Jingning Long, Zhou Zeng, Yangzhou Zhang, Rong Rong, Mengling You and Zhaolin Gao for technical assistance.
The Supplementary Material for this article can be found online at: https://www.frontiersin.org/articles/10.3389/fendo.2022.861452/full#supplementary-material
1. Saeedi P, Petersohn I, Salpea P, Malanda B, Karuranga S, Unwin N, et al. Global and Regional Diabetes Prevalence Estimates for 2019 and Projections for 2030 and 2045: Results From the International Diabetes Federation Diabetes Atlas, 9(Th) Edition. Diabetes Res Clin Pract (2019) 157:107843. doi: 10.1016/j.diabres.2019.107843
2. Yau JW, Rogers SL, Kawasaki R, Lamoureux EL, Kowalski JW, Bek T, et al. Global Prevalence and Major Risk Factors of Diabetic Retinopathy. Diabetes Care (2012) 35(3):556–64. doi: 10.2337/dc11-1909
3. Teo ZL, Tham YC, Yu M, Chee ML, Rim TH, Cheung N, et al. Global Prevalence of Diabetic Retinopathy and Projection of Burden Through 2045: Systematic Review and Meta-Analysis. Ophthalmology (2021) 128(11):1580–91. doi: 10.1016/j.ophtha.2021.04.027
4. The Vision Loss Expert Group of the Global Burden of Disease Study. Causes of Blindness and Vision Impairment in 2020 and Trends Over 30 Years, and Prevalence of Avoidable Blindness in Relation to VISION 2020: The Right to Sight: An Analysis for the Global Burden of Disease Study. Lancet Global Health (2021) 9(2):e144–60. doi: 10.1016/s2214-109x(20)30489-7
5. Sun Y, Smith LEH. Retinal Vasculature in Development and Diseases. Annu Rev Vision Sci (2018) 4:101–22. doi: 10.1146/annurev-vision-091517-034018
6. Heitmar R, Lip GYH, Ryder RE, Blann AD. Retinal Vessel Diameters and Reactivity in Diabetes Mellitus and/or Cardiovascular Disease. Cardiovasc Diabetol (2017) 16(1):56. doi: 10.1186/s12933-017-0534-6
7. Roy S, Kim D. Retinal Capillary Basement Membrane Thickening: Role in the Pathogenesis of Diabetic Retinopathy. Prog Retinal Eye Res (2021) 82:100903. doi: 10.1016/j.preteyeres.2020.100903
8. Stitt AW, Curtis TM, Chen M, Medina RJ, McKay GJ, Jenkins A, et al. The Progress in Understanding and Treatment of Diabetic Retinopathy. Prog Retinal Eye Res (2016) 51:156–86. doi: 10.1016/j.preteyeres.2015.08.001
9. Ortega ÁL. Oxidative Stress in Diabetic Retinopathy. Antioxidants (Basel Switzerland) (2021) 10(1):50. doi: 10.3390/antiox10010050
10. Coughlin BA, Feenstra DJ, Mohr S. Müller Cells and Diabetic Retinopathy. Vision Res (2017) 139:93–100. doi: 10.1016/j.visres.2017.03.013
11. Cheung N, Wong IY, Wong TY. Ocular Anti-VEGF Therapy for Diabetic Retinopathy: Overview of Clinical Efficacy and Evolving Applications. Diabetes Care (2014) 37(4):900–5. doi: 10.2337/dc13-1990
12. Campochiaro PA, Hafiz G, Mir TA, Scott AW, Zimmer-Galler I, Shah SM, et al. Pro-Permeability Factors in Diabetic Macular Edema; the Diabetic Macular Edema Treated With Ozurdex Trial. Am J Ophthalmol (2016) 168:13–23. doi: 10.1016/j.ajo.2016.04.017
13. Oellers P, Mahmoud TH. Surgery for Proliferative Diabetic Retinopathy: New Tips and Tricks. J Ophthalmic Vision Res (2016) 11(1):93–9. doi: 10.4103/2008-322x.180697
14. Wykoff CC, Khurana RN, Nguyen QD, Kelly SP, Lum F, Hall R, et al. Risk of Blindness Among Patients With Diabetes and Newly Diagnosed Diabetic Retinopathy. Diabetes Care (2021) 44(3):748–56. doi: 10.2337/dc20-0413
15. Wong TY, Sabanayagam C. Strategies to Tackle the Global Burden of Diabetic Retinopathy: From Epidemiology to Artificial Intelligence. Ophthalmologica J Int d’ophtalmologie Int J Ophthalmol Z fur Augenheilkunde (2020) 243(1):9–20. doi: 10.1159/000502387
16. Chen C, Song J, Chen M, Li Z, Tong X, Hu H, et al. Rhodiola Rosea Extends Lifespan and Improves Stress Tolerance in Silkworm, Bombyx Mori. Biogerontology (2016) 17(2):373–81. doi: 10.1007/s10522-015-9622-8
17. Ishaque S, Shamseer L, Bukutu C, Vohra S. Rhodiola Rosea for Physical and Mental Fatigue: A Systematic Review. BMC Complementary Altern Med (2012) 12:70. doi: 10.1186/1472-6882-12-70
18. Pu WL, Zhang MY, Bai RY, Sun LK, Li WH, Yu YL, et al. Anti-Inflammatory Effects of Rhodiola Rosea L.: A Review. Biomedicine Pharmacother = Biomed Pharmacotherapie (2020) 121:109552. doi: 10.1016/j.biopha.2019.109552
19. Sun S, Tuo Q, Li D, Wang X, Li X, Zhang Y, et al. Antioxidant Effects of Salidroside in the Cardiovascular System. Evidence-Based Complementary Altern Medicine: eCAM (2020) 2020:9568647. doi: 10.1155/2020/9568647
20. Song D, Zhao M, Feng L, Wang P, Li Y, Li W. Salidroside Attenuates Acute Lung Injury via Inhibition of Inflammatory Cytokine Production. Biomedicine Pharmacother = Biomed Pharmacotherapie (2021) 142:111949. doi: 10.1016/j.biopha.2021.111949
21. Su Y, Zong S, Wei C, Song F, Feng H, Qin A, et al. Salidroside Promotes Rat Spinal Cord Injury Recovery by Inhibiting Inflammatory Cytokine Expression and NF-κb and MAPK Signaling Pathways. J Cell Physiol (2019) 234(8):14259–69. doi: 10.1002/jcp.28124
22. Ju L, Wen X, Wang C, Wei Y, Peng Y, Ding Y, et al. Salidroside, a Natural Antioxidant, Improves β-Cell Survival and Function via Activating AMPK Pathway. Front Pharmacol (2017) 8:749. doi: 10.3389/fphar.2017.00749
23. Yang L, Zhou L, Wang X, Wang W, Wang J. Inhibition of HMGB1 Involved in the Protective of Salidroside on Liver Injury in Diabetes Mice. Int Immunopharmacol (2020) 89(Pt A):106987. doi: 10.1016/j.intimp.2020.106987
24. Ni J, Li Y, Xu Y, Guo R. Salidroside Protects Against Cardiomyocyte Apoptosis and Ventricular Remodeling by AKT/HO-1 Signaling Pathways in a Diabetic Cardiomyopathy Mouse Model. Phytomed: Int J Phytother Phytopharmacol (2021) 82:153406. doi: 10.1016/j.phymed.2020.153406
25. Wu D, Yang X, Zheng T, Xing S, Wang J, Chi J, et al. A Novel Mechanism of Action for Salidroside to Alleviate Diabetic Albuminuria: Effects on Albumin Transcytosis Across Glomerular Endothelial Cells. Am J Physiol Endocrinol Metab (2016) 310(3):E225–37. doi: 10.1152/ajpendo.00391.2015
26. Rodríguez ML, Pérez S, Mena-Mollá S, Desco MC, Ortega ÁL. Oxidative Stress and Microvascular Alterations in Diabetic Retinopathy: Future Therapies. Oxid Med Cell Longevity (2019) 2019:4940825. doi: 10.1155/2019/4940825
27. Beltramo E, Porta M. Pericyte Loss in Diabetic Retinopathy: Mechanisms and Consequences. Curr Med Chem (2013) 20(26):3218–25. doi: 10.2174/09298673113209990022
28. Hammes HP. Diabetic Retinopathy: Hyperglycaemia, Oxidative Stress and Beyond. Diabetologia (2018) 61(1):29–38. doi: 10.1007/s00125-017-4435-8
29. Wong TY, Cheung CM, Larsen M, Sharma S, Simó R. Diabetic Retinopathy. Nat Rev Dis Primers (2016) 2:16012. doi: 10.1038/nrdp.2016.12
30. Al-Jarrah MA, Shatnawi H. Non-Proliferative Diabetic Retinopathy Symptoms Detection and Classification Using Neural Network. J Med Eng Technol (2017) 41(6):498–505. doi: 10.1080/03091902.2017.1358772
31. Besirli CG, Johnson MW. Proliferative Diabetic Retinopathy. Mayo Clinic Proc (2009) 84(12):1054. doi: 10.4065/mcp.2009.0131
32. Ting DSW, Wong TY. Proliferative Diabetic Retinopathy: Laser or Eye Injection? Lancet (London England) (2017) 389(10085):2165–6. doi: 10.1016/s0140-6736(17)31194-7
33. Kusuhara S, Fukushima Y, Ogura S, Inoue N, Uemura A. Pathophysiology of Diabetic Retinopathy: The Old and the New. Diabetes Metab J (2018) 42(5):364–76. doi: 10.4093/dmj.2018.0182
34. Dagher Z, Park YS, Asnaghi V, Hoehn T, Gerhardinger C, Lorenzi M. Studies of Rat and Human Retinas Predict a Role for the Polyol Pathway in Human Diabetic Retinopathy. Diabetes (2004) 53(9):2404–11. doi: 10.2337/diabetes.53.9.2404
35. Sahajpal NS, Goel RK, Chaubey A, Aurora R, Jain SK. Pathological Perturbations in Diabetic Retinopathy: Hyperglycemia, Ages, Oxidative Stress and Inflammatory Pathways. Curr Protein Pept Sci (2019) 20(1):92–110. doi: 10.2174/1389203719666180928123449
36. Ying L, Shen Y, Zhang Y, Wang Y, Liu Y, Yin J, et al. Association of Advanced Glycation End Products With Diabetic Retinopathy in Type 2 Diabetes Mellitus. Diabetes Res Clin Pract (2021) 177:108880. doi: 10.1016/j.diabres.2021.108880
37. Patel A, MacMahon S, Chalmers J, Neal B, Billot L, Woodward M, et al. Intensive Blood Glucose Control and Vascular Outcomes in Patients With Type 2 Diabetes. New Engl J Med (2008) 358(24):2560–72. doi: 10.1056/NEJMoa0802987
38. Duckworth W, Abraira C, Moritz T, Reda D, Emanuele N, Reaven PD, et al. Glucose Control and Vascular Complications in Veterans With Type 2 Diabetes. New Engl J Med (2009) 360(2):129–39. doi: 10.1056/NEJMoa0808431
39. Kowluru RA, Mohammad G. Epigenetics and Mitochondrial Stability in the Metabolic Memory Phenomenon Associated With Continued Progression of Diabetic Retinopathy. Sci Rep (2020) 10(1):6655. doi: 10.1038/s41598-020-63527-1
40. Mahajan N, Arora P, Sandhir R. Perturbed Biochemical Pathways and Associated Oxidative Stress Lead to Vascular Dysfunctions in Diabetic Retinopathy. Oxid Med Cell Longevity (2019) 2019:8458472. doi: 10.1155/2019/8458472
41. Yuan Y, Wu SJ, Liu X, Zhang LL. Antioxidant Effect of Salidroside and its Protective Effect Against Furan-Induced Hepatocyte Damage in Mice. Food Funct (2013) 4(5):763–9. doi: 10.1039/c3fo00013c
42. Shi X, Dong N, Qiu Q, Li S, Zhang J. Salidroside Prevents Hypoxia-Induced Human Retinal Microvascular Endothelial Cell Damage via Mir-138/ROBO4 Axis. Invest Ophthalmol Visual Sci (2021) 62(9):25. doi: 10.1167/iovs.62.9.25
43. Qian C, Liang S, Wan G, Dong Y, Lu T, Yan P. Salidroside Alleviates High-Glucose-Induced Injury in Retinal Pigment Epithelial Cell Line ARPE-19 by Down-Regulation of Mir-138. RNA Biol (2019) 16(10):1461–70. doi: 10.1080/15476286.2019.1637696
44. Shi K, Wang X, Zhu J, Cao G, Zhang K, Su Z. Salidroside Protects Retinal Endothelial Cells Against Hydrogen Peroxide-Induced Injury via Modulating Oxidative Status and Apoptosis. Biosci Biotech Biochem (2015) 79(9):1406–13. doi: 10.1080/09168451.2015.1038212
45. Yin Y, Liu D, Tian D. Salidroside Prevents Hydroperoxide-Induced Oxidative Stress and Apoptosis in Retinal Pigment Epithelium Cells. Exp Ther Med (2018) 16(3):2363–8. doi: 10.3892/etm.2018.6494
46. Zheng T, Yang X, Li W, Wang Q, Chen L, Wu D, et al. Salidroside Attenuates High-Fat Diet-Induced Nonalcoholic Fatty Liver Disease via AMPK-Dependent TXNIP/NLRP3 Pathway. Oxid Med Cell Longevity (2018) 2018:8597897. doi: 10.1155/2018/8597897
Keywords: salidroside, diabetes, retinopathy, oxidative stress, vascular barrier, transcriptome
Citation: Yao F, Jiang X, Qiu L, Peng Z, Zheng W, Ding L and Xia X (2022) Long-Term Oral Administration of Salidroside Alleviates Diabetic Retinopathy in db/db Mice. Front. Endocrinol. 13:861452. doi: 10.3389/fendo.2022.861452
Received: 24 January 2022; Accepted: 21 February 2022;
Published: 16 March 2022.
Edited by:
Ye Ding, Georgia State University, United StatesReviewed by:
Mohamed El-Sherbiny, Almaarefa University, Saudi ArabiaCopyright © 2022 Yao, Jiang, Qiu, Peng, Zheng, Ding and Xia. This is an open-access article distributed under the terms of the Creative Commons Attribution License (CC BY). The use, distribution or reproduction in other forums is permitted, provided the original author(s) and the copyright owner(s) are credited and that the original publication in this journal is cited, in accordance with accepted academic practice. No use, distribution or reproduction is permitted which does not comply with these terms.
*Correspondence: Xiaobo Xia, eGJ4aWEyMUBjc3UuZWR1LmNu; Lexi Ding, bGV4aWRpbmdAY3N1LmVkdS5jbg==; Wei Zheng, emhlbmd3ZWlpbnh5QGNzdS5lZHUuY24=
Disclaimer: All claims expressed in this article are solely those of the authors and do not necessarily represent those of their affiliated organizations, or those of the publisher, the editors and the reviewers. Any product that may be evaluated in this article or claim that may be made by its manufacturer is not guaranteed or endorsed by the publisher.
Research integrity at Frontiers
Learn more about the work of our research integrity team to safeguard the quality of each article we publish.