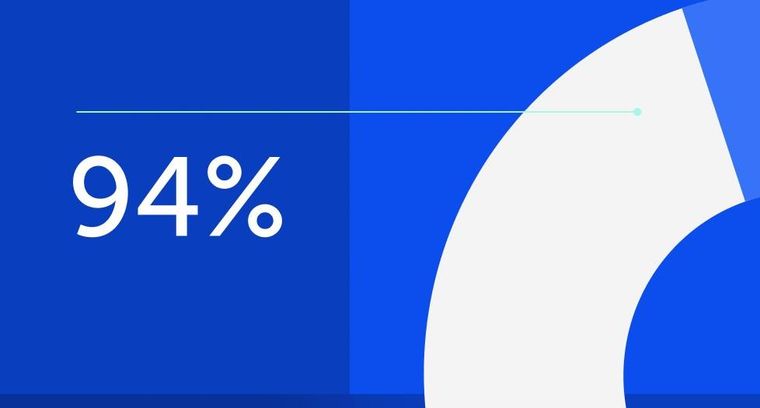
94% of researchers rate our articles as excellent or good
Learn more about the work of our research integrity team to safeguard the quality of each article we publish.
Find out more
ORIGINAL RESEARCH article
Front. Endocrinol., 21 April 2022
Sec. Obesity
Volume 13 - 2022 | https://doi.org/10.3389/fendo.2022.856667
This article is part of the Research TopicRising Stars: Obesity 2021View all 5 articles
Objective: To investigate the effect of BMI in male and/or female partners on embryo development and clinical pregnancy outcome during ART.
Methods: Data of 11,130 cycles between January 2018 and December 2020 were retrospectively analyzed. They were divided into Group A, B, C, and D based on couples’ BMI values, also were divided into Group I, II, III and IV in IVF cycles and Group i, ii, iii, and iv in ICSI cycles. After grouping, inter-group indicators comparisons and logistic regression analysis were performed.
Results: In IVF cycles, CPR in Group I and Group III were higher than Group IV. In Group III, it was higher than Group II. The AR in Group IV was higher, but the LBR was lower than Group I, Group II, and Group III. Logistic regression analysis results suggested that AR in Group IV was higher than that in Group I in IVF cycles, whereas LBR was lower.
In ICSI cycles, high-quality embryo rate in Group i and Group ii were both higher than that in Group iii and Group iv. The CPR in Group i was higher than Group ii and Group iv, and in Group iii was higher than Group ii and Group iv. The AR in Group i was lower than Group iii and Group iv, and AR in Group ii was lower than Group iv. LBR, in Group I it was higher than Group ii, Group iii, and Group iv. Logistic regression analysis results suggested CPR in Group ii was significantly lower than that in Group i. AR in Group iii was considerably higher than that in Group i. LBR in Group ii and Group iv were significantly lower than that in Group i.
Conclusion: Female higher BMI was not conducive to the formation of high-quality embryos in ICSI cycle. Female and/or male BMI affected AR and LBR more than CPR not only in IVF cycles, but also in ICSI cycles.
Overweight and obesity, which have already reached epidemic levels in some countries, have become topics of urgent importance. The World Health Organization (WHO) defined overweight as a body mass index (BMI) of over 25 kg/m²; a BMI over 30 kg/m² is considered to indicate obesity (1). WHO data for 2016 showed that 13% of the world’s adults were obese, with obesity prevalence of 15% in women and 11% in men. Obesity is an important risk factor for a number of non-communicable diseases, such as hypertension, cardiovascular disease, type 2 diabetes and other metabolic diseases (2), osteoarthritis (3), gallstone disease (4), asthma, and other chronic respiratory diseases (5–7), and multiple malignancies (8).
Obesity is often accompanied by changes in the levels of endocrine and reproductive hormones. Overweight women are more likely to suffer from polycystic ovary syndrome, menstrual disorders, infertility, miscarriage, poor pregnancy outcomes, and multiple pregnancy complications (including gestational diabetes, pre-eclampsia, and fetal macrosomia) (9–12). Studies have shown that high female BMI is associated with reduced blastocyst formation rate during in vitro fertilization (IVF) (13), abnormal oocyte morphology (14), low clinical pregnancy rate (CPR), high abortion rate (AR), and low live birth rate (LBR) (15–23). However, other research findings also suggested that female BMI had no influence on the assisted reproduction technology (ART) outcomes (24–28). This may be due to differences in the distribution of people selected in their study, as well as in the BMI grouping.
Meanwhile, male BMI has been related to sperm parameters. In men, obesity was found to cause low sperm concentration, low percentage of forward-moving sperm, low rate of normal sperm morphology, and high DNA fragmentation index (DFI%) (29, 30). Nevertheless, Anifandis et al. suggested that male BMI was not related to sperm parameters (31, 32), but it did affect embryo quality, and led to bad pregnancy outcomes (20, 27, 32–35). Conversely, the results of other studies suggested that BMI did not affect in vitro fertilization, embryo development and quality, pregnancy outcomes, and obstetric outcomes (24, 30, 31, 33, 36–38).
However, these studies have rarely considered the effect of BMI on oocytes in women and sperm quality men. Meanwhile, comprehensive and holistic assessments of ART pregnancy outcomes have been rarely conducted. Therefore, the aim of this study was to evaluate the effects of female and male BMI on the process of ART. In addition, most of the previous investigations have been focused on determining the impact of BMI and ART on pregnancy outcomes in women, but little attention has been paid to that influence on ART in men. Therefore, here, our purpose was to assess the overall impact of BMI of female and male subjects on in vitro fertilization, embryo development, and clinical outcome during the first cycle of ovulation induction to provide improved guidance that would increase clinical ART pregnancy success rates.
We collected the data of 24,081 cycles of patients that had undergone in vitro fertilization treatment in the First Affiliated Hospital of Zhengzhou University, the Reproductive and Genetic Hospital between January 2018 and December 2020. Based on the inclusion and exclusion criteria, we eventually analyzed the clinical data of 11,130 cycles.
Based on the BMI guidelines for the Chinese population (17, 39), the couples were divided into four groups based on the couple’s BMI. They were divided into the following groups: Group A (female and male BMI < 24 kg/m², N = 2526); Group B (female BMI < 24 kg/m² and male BMI ≥ 24 kg/m², N = 4349); Group C (female BMI ≥ 24 kg/m² and male BMI < 24 kg/m², N = 1455); and Group D (female and male BMI ≥ 24 kg/m², N = 2800). Then, the baseline data of each group were compared with those of the other groups and analyzed. Next, the data of these groups were divided into IVF and ICSI groups depending on the method of insemination. Further, BMI = 24 kg/m² was set as the threshold value, based on the results of the previous analysis. IVF cycles were divided into Group I (female and male BMI < 24 kg/m², N = 2007); Group II (female BMI < 24 kg/m² and male BMI≥ 24 kg/m², N = 3483); Group III (female BMI ≥ 24 kg/m² and male BMI< 24 kg/m², N = 1179); and Group IV (female and male BMI ≥ 24 kg/m², N = 2275). Meanwhile, ICSI cycles data were divided into Group i (female and male BMI < 24 kg/m², N = 519); Group ii (female BMI < 24 kg/m² and male BMI ≥ 24 kg/m², N = 866); Group iii (female BMI ≥ 24 kg/m² and male BMI< 24 kg/m², N = 276); and Group iv (female and male BMI ≥ 24 kg/m², N = 525). After grouping, the indicators of embryo development and pregnancy outcomes were compared among the groups, including oocyte maturation rate, normal fertilization rate, cleavage rate, high-quality embryo rate, blastocyst formation rate, high score blastocyst rate, CPR (number of pregnancy cycles/number of transplant cycles), AR (number of abortion cycles/number of clinical pregnancy cycles) and LBR (number of live birth cycles/number of transplant cycles).
AR, and LBR. A diagrammatic representation of our study is depicted in Figure 1A; the percentages of the different groups are displayed in Figure 1B).
Figure 1 (A) Flow charts representing the populations included in the current study. (B) Pie charts representing the percentages of different groups.
The following inclusion criteria were applied: (1) Couples undergoing in vitro fertilization-embryo transfer (IVF-ET) or intracytoplasmic sperm injection-embryo transfer (ICSI-ET) in the first cycle; (2) Couples with successful egg retrieval, embryo formation, and fresh transplantation after controlled ovarian stimulation (COS); (3) Men and women with complete BMI and follow-up records; (4) Female’s and male’s BMI ≥18.5 kg/m².
The exclusion criteria were as follows: (1) Donor and frozen semen cycles; (2) Preimplantation genetic diagnosis/screening (PGD/PGS) cycles; (3) Intrauterine insemination cycles; (4) Cycles of incomplete follow-up data; (5) Women and/or men with chromosome abnormality or (and) genetic disorder; (6) Men with diseases that affect semen quality or lead to infertility, such as urinary tract and genital infections, and medications.
Semen was routinely analyzed by marker plate count and hematoxylin-eosin (HE) staining. The volume of the semen obtained, sperm concentration, percentage of progressive motility (PR) sperm, percentage of non-progressive motility (NP) sperm, percentage of in-motility (IM) sperm, and DFI% were measured. DFI% was detected by sperm chromatin structure analysis (SCSA). The criteria to evaluate the sperm parameters based on the 5th World Health Organization guidelines (40). The male spouse ejaculated once 2–7 days before oocyte retrieval and again on the day of oocyte retrieval. At room temperature, the semen was treated with density gradient centrifugation and upstream method to remove impurities and abnormal sperm from seminal plasma and semen and capacitate the sperm. The oocytes were collected 37 hours after HCG injection, the granulosa cells were removed after short-term fertilization, and the prokaryocyte formation was observed 16–18 hours after fertilization. Vitrolife (Västra Frölunda, Sweden) or COOK medium was used for in vitro culture.
High-quality D3 cleavage-stage or D5 blastocyst-stage embryos were transplanted on the 3rd or 5th day after oocyte retrieval, based on the morphological evaluation of the embryos and the physical condition of the woman patients. The grading criteria of high-quality embryos were based on previous of our publications center (41). We used 1–2 cleavage-stage embryos or 1 blastocyst-stage embryos for transplantation. Surplus embryos or blastocysts were selected according to certain quality standards, and the surplus embryos or blastocysts were vitrified. Luteal phase support was given on the day of egg retrieval.
The serum chorionic gonadotropin (β-HCG) levels were measured 14/18 days after the embryo transfer. A threshold of β-HCG > 50 IU/L was set for biochemical pregnancy. Abdominal color Doppler ultrasound was performed 35 days after the embryo transfer to determine whether a gestational sac had been formed. An ultrasound finding of a gestational sac was considered to indicate a clinical pregnancy (42). Then, the patients were followed up regularly by telephone to obtain information on their periodic coverage, pregnancy, and obstetric outcomes (such as live births and third-trimester abortions), which were recorded in the central archives.
SPSS 25.0 software was employed for data analysis. Continuous numerical variables were represented by mean ± SD. One-way ANOVA and Mann-Whitney U-test were used to analyze the differences among groups. Categorical variables were represented by frequency and percentage (%), and the differences between groups were assessed by chi-square test (χ2-test). Logistic regression was used to analyze the data and explore the factors affecting the CPR, AR and LBR. The binary outcome of this model was whether clinical pregnancy, abortion and live birth or not. P < 0.05 was considered to indicate statistically significant differences.
We collected clinical data from 11,130 infertile couples who received assisted reproductive technology treatment at our center between January 2018 and December 2020. They received fresh transplants, and were followed up. Initially, the couples were divided into four groups (Group A, B, C, and D) based on the couple’s BMI. Then, we analyzed their baseline data and conducted comparison among the four groups. The demographic characteristics, baseline endocrine levels, and other data of the female patients in the different subgroups are presented as mean ± SD in Table 1.
As can be seen in Table 1A, there were significant differences in female age, basal hormone level, antral Follicle Count (AFC), DFI%, No. of oocytes retrieved and MII among the groups. And male age, semen volume, progressive motility (PR), non-progressive motility (NR), in-motility (IM) and DFI% among the groups also were significantly different. Differences among the four groups, were also established in the age of females (P < 0.01), but there was no difference except for that between Group C and group D (P > 0.05). Group A was constituted by the youngest participants, whereas Group D included the oldest. There was a similar pattern of male age distribution among the four groups (P < 0.001), but there was also no difference except between Group C and Group D (P > 0.05). No difference was found in the basal FSH levels between Group A and Group B and between Group C and Group D (P > 0.05). However, the basal FSH levels in Group A and Group B were significantly higher than those in Group C and Group B (P < 0.001). As visible in Table 1, the basal LH level of Group A and Group B were higher than that of Group D (P < 0.05). Nevertheless, there was no significant difference between the other groups (P > 0.05). Except for Group A and Group D (P > 0.05), there were differences in basal AMH levels among groups (P < 0.05). Group C had the highest basal AMH level, whereas Group B had the lowest. Significant differences in AFC were detected among the four groups (P < 0.01). The No. of oocytes retrieved in Group B was significantly lower than those in Group A, Group C, and Group D (P < 0.05). No significant differences were observed among the other groups (P > 0.05). Except for Group A and Group B, Group B and Group C, there was no difference in No. of MII oocytes between the other groups (P > 0.05). The No. of MII oocytes in Group B was significantly lower than those in Group A and Group C (P < 0.05). Compared with Group A, semen volume and PR in Group B and Group D were obviously lower (P < 0.05). While NP, IM and DFI% in Group B and Group D were significantly higher than that in Group A (P < 0.05). PR in Group C was higher than that in Group B, while DFI% in Group C was lower (P < 0.05). The same as DFI% in Group C was lower than that in Group D (P < 0.05).
Table 1B showed that the distribution of causes of infertility was different among groups, but the method of fertilization was the different. There were differences in the causes of infertility among the four groups (P < 0.001). Among the four groups, the proportions of PCOS in Group C (15.60%) and Group D (14.50%) were significantly higher than those in Group A (6.50%) and Group B (6.25%).
Table 2 presents the effects of female’s BMI on oocytes maturation, female’s and (or) male’s BMI oocytes fertilization, and embryo development in IVF cycles (Table 2A) and ICSI cycles (Table 2B). As seen in Table 2A, Group II had a higher 2PN fertilization rate than Group III (0.66 ± 0.19 vs 0.64 ± 0.18, p = 0.006). However, no differences were found in the mature oocyte rate, 2PN fertilization rate, normal fertilization cleavage rate, high-quality embryo rate, blastocyst formation, and high-scoring blastocyst rate (P > 0.05). As can be observed in Table 2B, there was no difference in the mature oocyte rate, 2PN fertilization rate, normal fertilization cleavage rate, blastocyst formation, and high-scoring blastocyst rate (P > 0.05). The high-quality embryo rates of Group i (0.69 ± 0.25) and Group ii (0.69 ± 0.25) were higher than those of Group iii (0.64 ± 0.28) and Group iv (0.62 ± 0.22) (P < 0.05).
Table 2a Effect of female or (and) male BMI on oocytes maturation, fertilization, embryo development, and embryo quality in IVF cycles.
Table 2b Effect of female and Male BMI on oocytes maturation, fertilization, embryo development, and embryo quality in ICSI cycles.
Further, we analyzed the clinical pregnancy outcomes of each group (Tables 3A, 3B).
The results displayed in Table 3A showed that in the IVF cycles, the CPR in Group I and Group III were significantly higher (62.13% vs 59.12%, P = 0.045; 64.03% vs 59.12%, P = 0.005) than that in Group IV. The CPR in Group III was significantly higher than that in Group II (64.03% vs 60.24%, P = 0.021). However, no differences in CPR among the other groups (P > 0.05) were established. The AR in Group IV was higher than those in Group I, Group II, and Group III (20.00% vs 13.95%, P = 0.000; 20.00% vs 15.82%, P = 0.002; 20.00% vs 15.63%, P = 0.014). Additionally, the LBR in Group IV was lower than those in Group I, Group II, and Group III (47.25% vs 53.46%, P = 0.000; 47.25% vs 50.70%, P = 0.011; 47.25% vs 54.03%, P = 0.000). No differences in AR and LBR were detected among the other groups (P > 0.05).
The data obtained for the ICSI cycles showed that the CPR of Group i was significantly higher than those of Group ii and Group iv (68.40% vs 59.12%, P = 0.000; 68.40% vs 60.38%, P = 0.005); that of Group iii was significantly higher than those of Group ii and Group iv (68.12% vs 59.12%, P = 0.009; 68.12% vs 60.38%, P = 0.031). Nevertheless, there was no difference in CPR between Group i and Group iii and between Group ii and Group iv (Table 3B). At the same time, we analyzed the differences in AR and LBR in the ICSI cycles among the four groups. Group iii had significantly higher AR values than those in Group i and Group ii (19.15% vs 8.68%, P = 0.001; 19.15% vs 11.72%, P = 0.016). The LBR in Group i was significantly higher than those in Group ii, Group iii, and Group iv (62.62% vs 52.19%, P = 0.000; 62.62% vs 55.07%, P = 0.040, 62.62% vs 51.62%, P = 0.000). Nonetheless, the values of AR and LBR in the other groups were similar, with no difference among the groups (P > 0.05) (Table 3B).
To further investigate the effect of female and male BMI on the pregnancy outcomes, we excluded the effects of female’s and male’s age, infertility cause, basal FSH level, basal LH level, basal AMH level, AFC, No. of oocytes retrieved, No. of MII oocytes, and DFI% by logistic regression analysis; the results are shown in Tables 4A, B.
Table 4a Logistic regression analysis on the effect of female and male BMI on pregnancy outcomes in IVF cycles.
Table 4b Logistic regression analysis on the effect of female and male BMI on pregnancy outcomes in ICSI cycles.
As visible in Table 3A, female and/or male BMI had influence on CRP, AR, and LBR not only in the IVF cycles, but also in the ICSI cycles. After the confounding factors were excluded by logistic regression analysis, we found that female and male BMI affected mainly AR and LBR, but not CPR in the IVF cycles. Compared with Group I (the reference), the AR in Group IV was higher (aOR: 1.46, 95% CI: 1.17–1.81, P = 0.000), whereas the LBR was lower (aOR: 0.86, 95% CI: 0.75–0.97, P = 0.017) (Table 4A). However, a difference was present between the ICSI cycles and IVF cycles. Specifically, in the ICSI cycles, CPR in Group ii was significantly lower than that in Group i after excluding the confounding factors (aOR: 0.73, 95% CI: 0.58–0.93, P = 0.012) (Table 4B). The value of AR in Group iii was considerably higher than that in Group I (aOR: 2.23, 95% CI: 1.30–3.83, P = 0.004). LBR in Group ii and Group iv were significantly lower (aOR: 0.72, 95% CI: 0.57–0.91, P = 0.006; aOR: 0.74, 95% CI: 0.57–0.96, P = 0.022) than that in Group i (Table 4B).
Following social development, the living standard of human beings has been substantially improved, which changed lifestyle. The proportion of obese people worldwide has been steadily increasing. Over recent years, the effect of obesity on egg and sperm quality and the effect of obesity on embryo development, pregnancy, and obstetric outcomes have attracted significant research attention in the field of assisted reproduction. Although many studies have been published, the findings on the effects of BMI on oocytes and sperms quality, embryo development, and the ART process outcomes remain controversial.
In our study, we found differences in female’s and male’s age in different BMI subgroups. This was largely due to personal habits and lifestyle variety. We also found differences in the levels of FSH, LH, AMH, and other hormones in the women in different BMI groups. These variations might have been caused by pulses of progonadoliberin-1 produced by the hypothalamus, which stimulates the secretion of follicle-stimulating hormone (FSH) and luteinizing hormone (LH) by the pituitary. Then, FSH and LH induce the secretion of steroid hormone by the ovarian and testicular tissue, leading to excessive androgen production in women and estrogen production in men. The elevated concentrations of androgen and estrogen exert a negative feedback effect on the hypothalamus-pituitary-ovary/testis axis. This could affect gonadotropin production, causing fluctuations and even imbalances in the reproductive hormones (43, 44). The results of our baseline data analysis showed that the AFC, No. of oocytes retrieved, and No. of MII oocytes in overweight and obese women were higher than those in normal weight women, which may be related to the high proportion of overweight and obese women with PCOS. We found that men with higher BMI had lower semen volume and PR, but had higher NP, IM and DFI%. Sperm movement is produced by adenosine triphosphate (ATP) continuously produced by mitochondria located in the middle of the sperm. A higher BMI leaded to overproduction of reactive oxygen species (ROS). An excess of ROS alters the phospholipid membrane, thereby destroying its selectivity, and also inhibits oxidative phosphorylation, ultimately resulting in reduced ATP production. And the ROS overproduction resulting in the oxidation of DNA bases (mainly guanosine) by producing by-products of lipid degradation that bind to DNA or come in direct interaction with DNA strands, resulting in nonspecific C single and double-strand breaks and increased DNA damage (45–49).
The accumulation of adipose tissue was found that could to lead to oxidative stress and DNA damage (e.g., DNA methylation), leading to defective oocytes and embryo development in offspring mice (50–52). Early embryonic development is known to be driven mainly by oocytes. Han et al. found that the absence of Stella protein (also known as DPPA3 or PGC7) in the oocytes of obese female mice could mediate epigenetic effects such as hydroxymethylcytosine modification and DNA damage (53). Triglyceride was established to be lipotoxic to embryos, and that palmitic acid, the most common fatty acid in human serum, could cause low proliferation of embryonic trophoblast stem cells and dose-dependent apoptosis (54). Our results showed that compared with normal female, the high-quality embryo rate in the female subgroups with BMI ≥ 24 kg/m² were lower in the ICSI cycles but not in the IVF cycles, which was consistent with the results of Depalo et al. (14). Another study showed that obesity in women resulted in poor IVF outcomes but did not affect embryo quality (55). However, this study was retrospective and did not eliminate enough confounding factors. Meanwhile, male BMI had no effect on high-quality embryo rate when the female BMI was normal. At female BMI ≥ 24 kg/m², the high-quality embryo rate in the male BMI ≥ 24 kg/m² subgroup was lower than that in the male BMI ≤ 24 kg/m² subgroup, but the difference was not statistically significant. This result was consistent with the findings of Anifandis et al. (32).
A systematic review conducted in 2021 suggested that a statistical association was found between female higher BMI and lower CPR, higher AR and lower LBR (15). In our study, we found that only a high female BMI did not negatively affect CPR not only in IVF cycles but also in ICSI cycles. We found low CPR in male with high BMI regardless of the female BMI in the ICSI cycles. However, CPR was affected only if the female had a high BMI and the male had an abnormal BMI in the IVF cycle. After adjustments for confounding factors, we also revealed that neither female nor male BMI exerted any effect on CPR in the IVF cycles. In our study, we found no effect of female BMI on CRP, which was consistent with the conclusions of Haghighi et al. (26). After adjustments for confounding factors, we found that only high female and male BMI resulted in high AR and low LBR in IVF. Meanwhile, high female BMI individually led to high AR in ICSI when the male’s BMI was normal. In the ICSI cycles, LBR in Group ii and Group iv were lower than that in the reference group. The male’s BMI had a greater impact on LBR than the female’s BMI. This might because of female and male with higher BMI might affect the quality of oocytes and sperms, and thus the development and quality of the embryo, which in turn affects CPR, LBR, and AR.
In our study, we conducted multiple stratified analyses to explore the effects of BMI in both men and women on the embryo development and ART outcomes. IVF and ICSI cycle analyses were conducted. We analyzed the effects of BMI of men and women on oocyte, semen quality, embryo development, embryo quality, and ART outcomes, but we lacked basic research on the mechanisms underlying these effects. This is something we need to look at further.
In conclusion, the effects of female and male BMI on embryo development and ART outcomes remain controversial. Thus, to improve the quality of assisted reproduction, more prospective and basic research is needed to explore the impact of BMI on human fertility, which was the purpose of this investigation. In the ICSI cycle, an abnormally higher female BMI was not conducive to the formation of high-quality embryos. Female and/or male BMI affected AR and LBR more than CPR not only in the IVF cycles but also in the ICSI cycles. However, our study had limitations as it was retrospective. Therefore, further research is needed to confirm our findings.
The original contributions presented in the study are included in the article/supplementary material. Further inquiries can be directed to the corresponding author.
Written informed consent was obtained from the individual(s) for the publication of any potentially identifiable images or data included in this article.
LQ developed the original concept of this study. LQ, Y-PL and Y-CS participated in the study design. Y-PL, X-LC, S-MW and N-NW participated in data collection, Y-PL conducted data analysis and interpretation and the writing of the original version of the manuscript. HS guided the data analysis and gave guidance. All authors participated in the manuscript revision. All authors have contributed to critical discussion and reviewed the final version.
This work was supported by the Chinese Natural Science Funds Youth Funds (81701505).
The authors declare that the research was conducted in the absence of any commercial or financial relationships that could be construed as a potential conflict of interest.
All claims expressed in this article are solely those of the authors and do not necessarily represent those of their affiliated organizations, or those of the publisher, the editors and the reviewers. Any product that may be evaluated in this article, or claim that may be made by its manufacturer, is not guaranteed or endorsed by the publisher.
The authors would like to thank to the Chinese Natural Science Funds Youth Funds for providing financial support.
1. Consultation Who Expert. Appropriate Body-Mass Index for Asian Populations and Its Implications for Policy and Intervention Strategies. Lancet (2004) 363(9403):157–63. doi: 10.1016/S0140-6736(03)15268-3
2. Organ World Health. Obesity: Preventing and Managing the Global Epidemic. Report of a WHO Consultation. World Health Organ Tech Rep Ser (2000) 894(i-xii):1–253.
3. Kulkarni K, Karssiens T, Kumar V, Pandit H. Obesity and Osteoarthritis. Maturitas (2016) 89:22–8. doi: 10.1016/j.maturitas.2016.04.006
4. Shaffer EA. Gallstone Disease: Epidemiology of Gallbladder Stone Disease. Best Pract Res Clin Gastroenterol (2006) 20(6):981–96. doi: 10.1016/j.bpg.2006.05.004
5. Eneli IU, Skybo T, . Camargo JRCA. Weight Loss and Asthma: A Systematic Review. Thorax (2008) 63(8):671–6. doi: 10.1136/thx.2007.086470
6. Mcclean KM, Kee F, Young IS, Elborn JS. Obesity and the Lung: 1. Epidemiology. Thorax (2008) 63(7):649–54. doi: 10.1136/thx.2007.086801
7. Crummy F, Piper AJ, Naughton MT. Obesity and the Lung: 2. Obesity and Sleep-Disordered Breathing. Thorax (2008) 63(8):738–46. doi: 10.1136/thx.2007.086843
8. Cancer International Agency for Research On. IARC Handbooks of Cancer Prevention Volume 6 Weight Control and Physical Activity. Lyon: IARC Press. (2001).
9. Chavarro JE, Rich-Edwards JW, Rosner BA, Willett WC. Diet and Lifestyle in the Prevention of Ovulatory Disorder Infertility. Obstet Gynecol (2007) 110(5):1101050–8. doi: 10.1097/01.AOG.0000287293.25465.e1
10. Group The Eshre Capri Workshop. Nutrition and Reproduction in Women. Hum Reprod Update (2006) 12(3):193–207. doi: 10.1093/humupd/dmk003
11. Villamor E, Cnattingius S. Interpregnancy Weight Change and Risk of Adverse Pregnancy Outcomes: A Population-Based Study. Lancet (2006) 368(9542):1164–70. doi: 10.1016/S0140-6736(06)69473-7
12. Metwally M, Ong KJ, Ledger WL, Li TC. Does High Body Mass Index Increase the Risk of Miscarriage After Spontaneous and Assisted Conception? A Meta-Analysis of the Evidence. Fertil Steril (2008) 90(3):714–26. doi: 10.1016/j.fertnstert.2007.07.1290
13. Umul M, Kose SA, Bilen E, Altuncu AG, Oksay T, Guney M. Effect of Increasing Paternal Body Mass Index on Pregnancy and Live Birth Rates in Couples Undergoing Intracytoplasmic Sperm Injection. Andrologia (2015) 47(3):360–4. doi: 10.1111/and.12272
14. Depalo R, Garruti G, Totaro I, Panzarino M, Vacca MP, Giorgino F, et al. Oocyte Morphological Abnormalities in Overweight Women Undergoing In Vitro Fertilization Cycles. Gynecol Endocrinol (2011) 27(11):880–4. doi: 10.3109/09513590.2011.569600
15. Xiong Y, Wang J, Huang S, Liu C, Liu Y, Qi Y, et al. Association Between Maternal Prepregnancy Body Mass Index and Pregnancy Outcomes Following Assisted Reproductive Technology: A Systematic Review and Dose-Response Meta-Analysis. Obes Rev (2021) 22(6):e13219. doi: 10.1111/obr.13219
16. Wang X, Hao J, Zhang F, Li J, Kong H, Guo Y. Effects of Female and Male Body Mass Indices on the Treatment Outcomes and Neonatal Birth Weights Associated With In Vitro Fertilization/Intracytoplasmic Sperm Injection Treatment in China. Fertil Steril (2016) 106(2):460–6. doi: 10.1016/j.fertnstert.2016.04.021
17. Ding W, Zhang F-L, Liu X-C, Hu L-L, Dai S-J, Li G, et al. Impact of Female Obesity on Cumulative Live Birth Rates in the First Complete Ovarian Stimulation Cycle. Front Endocrinol (Lausanne) (2019) 10:516. doi: 10.3389/fendo.2019.00516
18. Imterat M, Agarwal A, Esteves SC, Meyer J, Harlev A. Impact of Body Mass Index on Female Fertility and ART Outcomes. Panminerva Med (2019) 61(1):58–67. doi: 10.23736/S0031-0808.18.03490-0
19. Cozzolino M, García-Velasco JA, Meseguer M, Pellicer A, Bellver J. Female Obesity Increases the Risk of Miscarriage of Euploid Embryos. Fertil Steril (2020) 115(6):1495–502. doi: 10.1016/j.fertnstert.2020.09.139
20. Zhao Z, Jiang X, Li J, Zhang M, Liu J, Dai S, et al. The Combined Impact of Female and Male Body Mass Index on Cumulative Pregnancy Outcomes After the First Ovarian Stimulation. Front Endocrinol (Lausanne) (2021) 12:735783. doi: 10.3389/fendo.2021.735783
21. Provost Meredith P, Acharya Kelly S, Acharya Chaitanya R, Yeh Jason S, Steward Ryan G, Eaton Jennifer L, et al. Pregnancy Outcomes Decline With Increasing Body Mass Index Analysis of 239,127 Fresh Autologous In Vitro Fertilization Cycles From the 2008–2010 Society for Assisted Reproductive Technology Registry. Fertil Steril (2016) 105(3):663–9. doi: 10.1016/j.fertnstert.2015.11.008
22. Prost E, Reignier A, Leperlier F, Caillet P, Barrière P, Fréour T, et al. Female Obesity Does Not Impact Live Birth Rate After Frozen-Thawed Blastocyst Transfer. Fertil Steril (2020) 107(4):840–7. doi: 10.1093/humrep/deaa010
23. Fabozzi G, Cimadomo D, Allori M, Vaiarelli A, Colamaria S, Argento C, et al. Maternal Body Mass Index Associates With Blastocyst Euploidy and Live Birth Rates the Tip of an Iceberg. Reprod BioMed Online (2021) 43(4):645–54. doi: 10.1016/j.rbmo.2021.07.006
24. Kim J, Juneau C, Patounakis G, Morin S, Neal S, Seli E, et al. The Appraisal of Body Content (ABC) Trial Obesity Does Not Significantly Impact Gamete Production in Infertile Men and Women. J Assist Reprod Genet (2020) 37(11):2733–42. doi: 10.1007/s10815-020-01930-3
25. Bartolacci A, Buratini J, Moutier C, Guglielmo MC, Brambillasca F, Renzini MM, et al. Maternal Body Mass Index Affects Embryo Morphokinetics a Time-Lapse Study. J Assist Reprod Genet (2019) 36(6):1109–16. doi: 10.1007/s10815-019-01456-3
26. Haghighi Z, Rezaei Z, Es-Haghi Ashtiani S. Effects of Women’s Body Mass Index on In Vitro Fertilization Success a Retrospective Cohort Study. Gynecol Endocrinol (2012) 28(7):536–9. doi: 10.3109/09513590.2011.650657
27. Wu Z, Lu X, Wang M, Cheng H. Correlation Between Body Mass Index of Chinese Males and Assisted Reproductive Technology Outcome. Int J Clin Exp Med (2015) 15(8):21472–6.
28. Banker M, Sorathiya D, Shah S. Effect of Body Mass Index on the Outcome of In-Vitro Fertilization Intracytoplasmic Sperm Injection in Women. J Hum Reprod Sci (2017) 10(1):37–43. doi: 10.4103/jhrs.JHRS_75_16
29. Fariello RM, Pariz JR, Spaine DM, Cedenho AP, Bertolla RP, Fraietta R. Association Between Obesity and Alteration of Sperm DNA Integrity and Mitochondrial Activity. BJU Int (2012) 110(6):863–7. doi: 10.1111/j.1464-410X.2011.10813.x
30. Sarah MC, Zsolt PN, Daniel BS, Sydney RA, Deandrea PE, Alicia KS, et al. Impact of Male Partner Characteristics and Semen Parameters on In Vitro Fertilization and Obstetric Outcomes in a Frozen Oocyte Donor Model. Fertil Steril (2018) 110(5):859–69. doi: 10.1016/j.fertnstert.2018.06.003
31. Li FY, Yang QL, Shi H, Xin H, Luo XY, Sun YP. Effects of Obesity on Sperm Retrieval, Early Embryo Quality and Clinical Outcomes in Men With Nonobstructive Azoospermia Undergoing Testicular Sperm Aspiration-Intracytoplasmic Sperm Injection Cycles. Andrologia (2019) 51(6):e13265. doi: 10.1111/and.13265
32. Anifandis G, Dafopoulos K, Messini CI, Polyzos N, Messinis IE. The BMI of Men and Not Sperm Parameters Impact on Embryo Quality and the IVF Outcome. Andrology (2013) 1(1):85–9. doi: 10.1111/j.2047-2927.2012.00012.x
33. Hoek J, Schoenmakers S, Van Duijn L, Willemsen SP, Van Marion ES, Laven JSE, et al. A Higher Preconceptional Paternal Body Mass Index Influences Fertilization Rate and Preimplantation Embryo Development. Andrology (2021) 10(3):486–94. doi: 10.1111/andr.13128
34. Rabia M, Jyotsna P, Chiara A, Osama N, Yacoub K, Tarekel T. Effect of Male Body Mass Index on Assisted Reproduction Treatment Outcome: An Updated Systematic Review and Meta-Analysis. Reprod BioMed Online (2018) 4):459–71. doi: 10.1016/j.rbmo.2018.01.002
35. Yang QL, Zhao FF, Hu LL, Bai R, Zhang N, Yao GD, et al. Effect of Paternal Overweight or Obesity on IVF Treatment Outcomes and the Possible Mechanisms Involved. Sci Rep (2016) 6:29787. doi: 10.1038/srep29787
36. Julia K, George P, Caroline J, Scott M, Shelby N, Paul B, et al. The Appraisal of Body Content (ABC) Trial: Increased Male or Female Adiposity Does Not Significantly Impact In Vitro Fertilization Laboratory or Clinical Outcomes. Fertil Steril (2021) 116(2):444–52. doi: 10.1016/j.fertnstert.2020.12.037
37. Tola EN, Oral HB. The Role of Paternal Obesity on the Success of Intracytoplasmic Sperm Injection Cycle a Tertiary IVF Center in Turkey. J Pak Med Assoc (2019) 69(5):640–6.
38. Arezoo A, Mahnaz A, Mandana H, Zahra Z. The Effects of Maternal and Paternal Body Mass Index on Live Birth Rate After Intracytoplasmic Sperm Injection Cycles. Int J Fertil Steril (2019) 13(1):24–31. doi: 10.22074/ijfs.2019.5433
39. Chen C, Lu FC, Health Department of Disease Control Ministry Of, China Pr. The Guidelines for Prevention and Control of Overweight and Obesity in Chinese Adults. BioMed Environ Sci (2004) 1–36.
40. Who. WHO Laboratory Manual for the Examination of Human Semen and Sperm Cervical Mucus Interaction. 5th Edn. Cambridge: Cambridge Press (2009).
41. Jin HX, Dai SJ, Song WY, Yao GD, Shi SL, Sun YP. Embryo Developmental Potential of Microsurgically Corrected Human Three-Pronuclear Zygotes. Syst Biol Reprod Med (2015) 61(2):96–102. doi: 10.3109/19396368.2014.986693
42. Zeadna A, Son WY, Moon JH, Dahan MH. A Comparison of Biochemical Pregnancy Rates Between Women Who Underwent IVF and Fertile Controls Who Conceived Spontaneously. Hum Reprod (2015) 30(4):783–8. doi: 10.1093/humrep/dev024
43. Hammoud AO, Gibson M, Peterson CM, Hamilton BD, Carrell DT. Obesity and Male Reproductive Potential. J Androl (2006) 27(5):619–26. doi: 10.2164/jandrol.106.000125
44. Jungheim Emily S, Moley Kelle H. Current Knowledge of Obesity’s Effects in the Pre- and Periconceptional Periods and Avenues for Future Research. Am J Obstet Gynecol (2010) 203(6):525–30. doi: 10.1016/j.ajog.2010.06.043
45. Mir J, Franken D, Andrabi SW, Ashraf M, Rao K. Impact of Weight Loss on Sperm DNA Integrity in Obese Men. Andrologia (2018). doi: 10.1111/and.12957
46. Omrani AB, Eisa AN, Javed M, Ghedan AM, Matrafi AH, Sufyan AH. Associations of Sperm DNA Fragmentation With Lifestyle Factors and Semen Parameters of Saudi Men and Its Impact on ICSI Outcome. Reprod Biol Endocrinol (2018) 16(1):49. doi: 10.1186/s12958-018-0369-3
47. Le MT, Nguyen DN, Le DD, Tran NQT. Impact of Body Mass Index and Metabolic Syndrome on Sperm DNA Fragmentation in Males From Infertile Couples: A Cross-Sectional Study From Vietnam. Metabol Open (2020) 7:100054. doi: 10.1016/j.metop.2020.100054
48. La Vignera S, Condorelli RA, Vicari E, Calogero AE. Negative Effect of Increased Body Weight on Sperm Conventional and Nonconventional Flow Cytometric Sperm Parameters. J Androl (2012) 33(1):53–8. doi: 10.2164/jandrol.110.012120
49. Barbagallo F, Condorelli Rosita A, Mongioì Laura M, Cannarella R, Cimino L, Magagnini MC, et al. Molecular Mechanisms Underlying the Relationship Between Obesity and Male Infertility. Metabolites (2021) 11(12). doi: 10.3390/metabo11120840
50. Mateescu B, Batista L, Cardon M, Gruosso T, De Feraudy Y, Mariani O, et al. miR-141 and miR-200a Act on Ovarian Tumorigenesis by Controlling Oxidative Stress Response. Nat Med (2011) 17(12):1627–35. doi: 10.1038/nm.2512
51. Pembrey M, Saffery R, Bygren LO, Network in Epigenetic Epidemiology. Human Transgenerational Responses to Early-Life Experience: Potential Impact on Development, Health and Biomedical Research. J Med Genet (2014) 51(9):563–72. doi: 10.1136/jmedgenet-2014-102577
52. Ge Z-J, Luo S-M, Lin F, Liang Q-X, Huang L, Wei Y-C, et al. DNA Methylation in Oocytes and Liver of Female Mice and Their Offspring Effects of High-Fat-Diet-Induced Obesity. Environ Health Perspect (2014) 122(2):159–64. doi: 10.1289/ehp.1307047
53. Han L, Ren C, Li L, Li X, Ge J, Wang H, et al. Embryonic Defects Induced by Maternal Obesity in Mice Derive From Stella Insufficiency in Oocytes. Nat Genet (2018) 50(3):432–42. doi: 10.1038/s41588-018-0055-6
54. Jungheim Emily S, Macones George A, Odem Randall R, Patterson Bruce W, Moley Kelle H. Elevated Serum α-Linolenic Acid Levels are Associated With Decreased Chance of Pregnancy After In Vitro Fertilization. Fertil Steril (2011) 96(4):880–3. doi: 10.1016/j.fertnstert.2011.07.1115
Keywords: BMI, high-quality embryo rate, clinical pregnancy rate, abortion rate, live birth rate
Citation: Qi L, Liu Y-p, Wang S-m, Shi H, Chen X-l, Wang N-n and Su Y-c (2022) Abnormal BMI in Male and/or Female Partners Are Deleterious for Embryonic Development and Pregnancy Outcome During ART Process: A Retrospective Study. Front. Endocrinol. 13:856667. doi: 10.3389/fendo.2022.856667
Received: 17 January 2022; Accepted: 04 March 2022;
Published: 21 April 2022.
Edited by:
Negar Naderpoor, Monash University, AustraliaReviewed by:
Laura Maria Mongioì, University of Catania, ItalyCopyright © 2022 Qi, Liu, Wang, Shi, Chen, Wang and Su. This is an open-access article distributed under the terms of the Creative Commons Attribution License (CC BY). The use, distribution or reproduction in other forums is permitted, provided the original author(s) and the copyright owner(s) are credited and that the original publication in this journal is cited, in accordance with accepted academic practice. No use, distribution or reproduction is permitted which does not comply with these terms.
*Correspondence: Ying-chun Su, c3V5aW5nY2h1bjFAMTI2LmNvbQ==
†These authors have contributed equally to this work and share first authorship
Disclaimer: All claims expressed in this article are solely those of the authors and do not necessarily represent those of their affiliated organizations, or those of the publisher, the editors and the reviewers. Any product that may be evaluated in this article or claim that may be made by its manufacturer is not guaranteed or endorsed by the publisher.
Research integrity at Frontiers
Learn more about the work of our research integrity team to safeguard the quality of each article we publish.