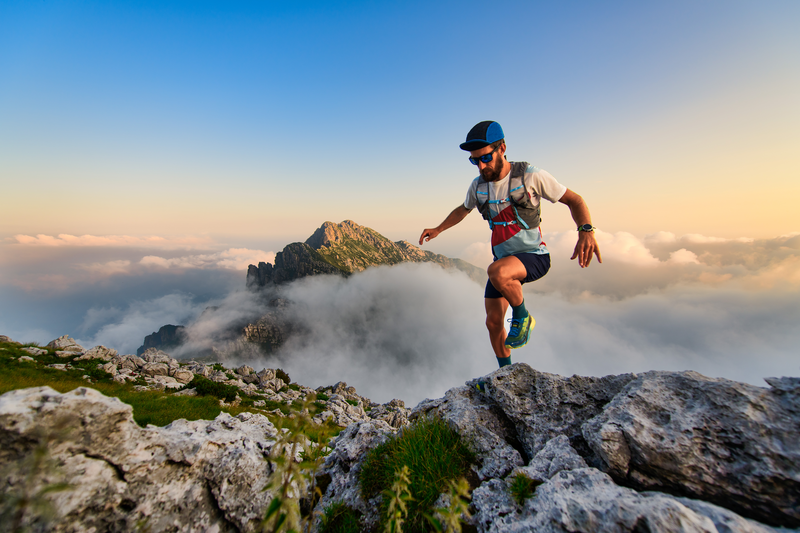
94% of researchers rate our articles as excellent or good
Learn more about the work of our research integrity team to safeguard the quality of each article we publish.
Find out more
MINI REVIEW article
Front. Endocrinol. , 08 March 2022
Sec. Cellular Endocrinology
Volume 13 - 2022 | https://doi.org/10.3389/fendo.2022.854718
This article is part of the Research Topic Physiology and Pathophysiology of GPCR Signal Transduction View all 8 articles
Over thousands of years of evolution, animals have developed many ways to protect themselves. One of the most protective ways to avoid disease is to prevent the absorption of harmful components. This protective function is a basic role of bitter taste receptors (TAS2Rs), a G protein-coupled receptor family, whose presence in extraoral tissues has intrigued many researchers. In humans, there are 25 TAS2Rs, and although we know a great deal about some of them, others are still shrouded in mystery. One in this latter category is bitter taste receptor 39 (TAS2R39). Besides the oral cavity, it has also been found in the gastrointestinal tract and the respiratory, nervous and reproductive systems. TAS2R39 is a relatively non-selective receptor, which means that it can be activated by a range of mostly plant-derived compounds such as theaflavins, catechins and isoflavones. On the other hand, few antagonists for this receptor are available, since only some flavones have antagonistic properties (all of them detailed in the document). The primary role of TAS2R39 is to sense the bitter components of food and protect the organism from harmful compounds. There is also some indication that this bitter taste receptor regulates enterohormones and in turn, regulates food intake. In the respiratory system, it may be involved in the congestion process of allergic rhinitis and may stimulate inflammatory cytokines. However, more thorough research is needed to determine the precise role of TAS2R39 in these and other tissues.
Humans have five primary tastes: bitter, sweet, sour, salty, and umami (1), which are identified as membrane receptors. These receptors enable animals to analyze the chemical structure of food as soon as it hits the taste buds located on their tongue before any harmful components are digested. Although on the surface the role of these receptors may look the same, they are anything but alike. Though ubiquitous to us all, sensing salty taste remained a mystery until recently when researchers identified specialized cells that recognize this taste through the sodium ion channel (2). Other types of ion channels are used to detect the sour components of food (3), while the other three tastes are mediated differently, i.e. through specialized G protein-coupled receptors (GPCRs) known as taste receptors (TASRs) (4). Although these receptors sense different compounds, they share several structural and functional attributes. These glycoprotein receptors consist of seven transmembrane α-helices. Upon binding of a ligand, interaction with heterotrimeric G proteins occurs, which activates the propagation of signaling cascade in the cell (5). To identify umami, sweet and bitter tastes, two families of GPCRs have been identified: TAS1R and TAS2R. The first family of receptors contains three members: TAS1R1, TAS1R2 and TAS1R3 (6). This family is responsible for detecting both sweet and umami tastes. This is done by dimerizing different members into one receptor: the TAS1R1-TAS1R3 heterodimer is responsible for umami, while TAS1R2-TAS1R3 complex is responsible for sweet (7). On the other hand, the TAS2R family is made up of a much more diverse group of receptors, which in humans consists of 25 functional genes, as well as 8 pseudogenes, though the number varies greatly from species to species (8, 9). Researchers have also discovered that although we possess a relatively low number of bitter taste receptors, we are able to detect as bitter thousands of compounds from a wide range of families. We can categorize bitter receptors into four groups based on their specificity to ligands: receptors that detect a broad spectrum of ligands; more selective receptors with just a few ligands; receptors somewhere between these two groups; and receptors that recognize specific chemical motifs (10). One member of the group that detects a moderate number of bitter compounds is TAS2R39, which is the focal point of this review.
TAS2R39 is encoded by the TAS2R39 gene, which is located on chromosome 7 (7q34). Interestingly, this gene sequence contains no intron, so it belongs to a small group of genes that are known as intron-less or single-exon genes (11). This bitter taste receptor is still relatively unknown, since the first ligands for it were discovered just over a decade ago (4). Another area where more concrete information is lacking are the locations where it is expressed. As with other members of the bitter-taste receptor family, TAS2R39 is expressed in the oral tissue. The little information available about its expression indicates that it is one of the TAS2Rs that are expressed in the lowest quantities (12). The extraoral presence of bitter taste receptors has been well documented. These can be found in several types of tissues, including the brain, the respiratory system, the cardiac system, and even the reproductive system (6, 13–15). However, discrepancies exist among these receptors since some are more well-known, while others remain unexplored. Studies exploring the expression of the TAS2R39 gene have identified TAS2R39 in the colon (16), bronchi (17), nasal mucosa (14), arteries (18), and skin (19). Additionally, cell lines used in research have been identified as expressing this gene, including the HuTu-80 and NCI-H716 intestinal cells (16), the hTERT-HM myometrial cells (20), and lung macrophages (21), while the protein has been detected in the cells of choroid plexus of the nervous system (22). Further data are constantly being added to databases such as the Human Protein Atlas, while new locations for TAS2R39 expression are being discovered, including the pancreas and spleen, and even the brain, testes and ovaries (11, 23). Although TAS2R39 appears to be widely present in human tissues, it is important to note that in all these tissues its gene expression is low and, that, as is reflected by the relatively little knowledge we have about this bitter taste receptor, detecting it is a challenge.
TAS2R39 is a 338 amino acid long protein that, as we mentioned earlier, has seven transmembrane domains (numbered from I to VII). Based on computer analysis using modelling software, a structure-based pharmacophore model was created (24). This model suggests that the binding pocket of a TAS2R39 ligand is located extracellularly between transmembrane helices III, V, VI and VII, with a hydrophobic interaction between the ligand and receptor that covers most of the interaction area. Additionally, hydrogen bond acceptors and donors contribute to the binding and, potentially, π-π aromatic influences may also occur in the binding pocket. The structural differences between agonists and antagonists have also been inspected. Although at first sight they are quite similar, two key differences have been identified: as well as lacking a hydrogen donor, antagonists display stereochemical flexibility, which fills the binding pocket and, in this way, prevent a conformational change in the receptor upon its activation (24). Another study compared ligands that activate TAS2R14 and TAS2R39 by analyzing the structure-activity relationship. In that study it was observed that glycosylation had an inhibiting effect on TAS2R14, while activation of the TAS2R39 was preserved (though a higher concentration of agonists was needed to activate the receptor). The compounds tested were members of the isoflavonoid group. Alteration of the C-ring skeletal structure of these compounds did not hinder the activation of these two receptors, though their potency and efficacy may be affected. Also inspected was the effect of different substituents on the binding properties. It was found that, for a compound to be a TAS2R39 ligand, substitutes, preferably a hydroxy group, are obligatory (25).
All elements of the signaling cascade of the TAS2R signaling pathway remain unknown. However, researchers have discovered the importance of gustducin as a member of the G-coupled protein mechanism (26, 27). This is even more evident from the fact that TAS2Rs are expressed in cells containing gustducin (28, 29). The perception of taste starts on the tongue in the taste organ, i.e. the taste bud (30). Located throughout the oral cavity, these buds contain between 50 and 100 taste cells (31). Each of the five basic tastes is sensed by specific cells. Cells that detect bitter taste are called type-II cells, which can also sense sweet and umami tastes. Which taste they detect depends on the receptor that is expressed (32). Although it has not been confirmed, we can postulate that the signaling pathway in the oral cavity is similar in the extraoral locations. When a bitter ligand binds to the receptor, a conformational change is induced. This causes the dissociation of α-gustducin from the β and γ subunits of the G protein-coupled unit. The β and γ subunits of this protein then activate the β2 isoform of phospholipase C (PLCβ2), which in turn leads to the synthesis of inositol 1,4,5-triphosphate (IP3) and diacylglycerol (DAG). IP3 binds to IP3 receptor, resulting in the release of Ca2+ from the endoplasmic reticulum. This change in the intracellular concentration of ions induces the activation of membrane ion channels, which transport Na+ into the cells, thereby depolarizing the membrane. Once action potential is reached, neurotransmitter ATP is released from the cells, with the signal propagating forwards through afferent nerves (33, 34). However, this is not the only way the signaling pathway is activated. Upon ligand binding, another pathway is activated: the α-gustducin subunit stimulates taste phosphodiesterase (PDE), which hydrolyses cAMP. What happens then is not known but some theories suggest that this may disinhibit cyclic nucleotide-inhibited channels and in turn elevate the intracellular levels of Ca2+, which again results in the exocytosis of neurotransmitters (Figure 1) (33, 35).
Figure 1 The Bitter Taste Receptor Signaling Pathway: when the ligand binds to the TAS2R, a conformational change is induced. This in turn triggers the dissociation of the α-subunit of gustducin from the β- and γ-subunits. This dissociation of subunits signifies two divergent pathways of signal transduction. In the first of these, β- and γ-subunits activate the β2 isoform of phospholipase C (PLCβ2), which cleaves phosphatidylinositol 4,5-bisphosphate (PIP2) into diacyl glycerol (DAG) and inositol 1,4,5-trisphosphate (IP3). IP3 then travels to Endoplasmic Reticulum (ER), where it binds to its receptor (IP3R), leading to the secretion of Ca2+ from the ER into the cytoplasm. This increase in intracellular Ca2+ levels leads to the activation of sodium-selective transmembrane transporters TRPM4 and 5, thereby depolarizing the cellular membrane. Depolarization activates voltage-gated sodium channels (VGNC), thus hastening depolarization. When the action potential (AP) is reached, the calcium homeostasis modulator 1 and 3 (CALHM1/3) channel and pannexin 1 channels are activated, which leads to the transportation of ATP from cytoplasm to the intercellular space. Through P2X ionotropic purinergic receptors 2 and 3 (P2X2/P2X3), ATP is taken into the afferent nerve, thereby propagating the signal further down. The other pathway occurs through the α-subunit of gustducin, but the exact mechanism of signal propagation is unknown. It is postulated that α-gustducin lowers the level of cAMP by activating its hydrolysation though phosphodiesterase (PDE), but the next steps are still unknown. The lowering of cAMP may lead to a decrease in the levels of cNMPs intracellularly, which may regulate protein kinases and in turn regulate the ion activity in the cell. It is also possible that cNMP directly regulates cNMP-gated ion channels, thereby depolarizing the membrane and eliciting the release of the neurotransmitter.
For a long time, TAS2R39 was considered an orphan receptor, i.e. there were no known ligands that activated it. Recently, however, interest in this receptor has increased and, in 2009, its first ligands were discovered (10). These first ligands discovered were agonists, and most compounds were extracted or derived from plants. Various groups of TAS2R39 ligands also appeared, some of which were more specific, while others also bound to other bitter taste receptors. More studies exploring possible ligands, the most prevalent source of which were again plants, were then conducted. With theaflavins extracted from black tea, TAS2R39 shows a preference for theaflavin and theaflavin-3,3-O’-digalatte, while theaflavin-3-O’-galatte also activated TAS2R14 (36). Catechins derived from green tea are another group of ligands for this bitter taste receptor, with epicatechin gallate and epigallocatechin gallate demonstrating relatively strong affinity to TAS2R39, and epicatechin and epigallocatechin displaying lower affinity. These data show that the galloyl group creates important bonds for the ligand-receptor interaction. Note that these catechins also bind to other bitter receptors, and the only one that seems to be selective for the TAS2R39 is epigallocatechin (37). Soybean is another plant from which such compounds can be extracted. Genistein, a predominant isoflavone in soybeans, activated both TAS2R39 and TAS2R14, which is becoming TAS2R39’s closest relative in terms of the ligands they both bind to. However, glucosylated forms of genistein do not activate TAS2R14, though they did activate TAS2R39. Further experiments with similar isoflavones were conducted and 15 other TAS2R39 agonists were discovered, with acetylgenistin, genistin, glycitin and malonyl genistin being specific to TAS2R39 (25). A more comprehensive study was conducted in which 67 flavonoid and isoflavonoid compounds were found to be ligands for this receptor, with new specific ligands being acacetin, 5,2′-dihydroxyflavone, gardenin A, genkwanin gossypetin, 6-methoxyflavonol and 4′-hydroxyflavanone (38). A recent study also noted the activation of this bitter taste receptor by vanillin, a major component of vanilla widely used as a flavouring agent (39).
Small molecules are not the only ones that can activate this bitter taste receptor, as some peptides have been also identified as TAS2R39 agonists. Amino acids, especially those exhibiting more hydrophobic properties, show greater affinity to the receptor, since hydrophobicity is one of the major factors of ligand-bitter taste receptor interaction (40). A broad study testing all proteinogenic amino acids on all bitter taste receptors uncovered more details about the amino acid activation of bitter taste receptors. It was discovered that the most sensitive bitter taste receptors for amino acids are TAS2R1, TAS2R4, and TAS2R39, since these were activated by phenylalanine (Phe) and tryptophan (Trp). Curiously, it was observed that TAS2R39 was activated by the D-conformation amino acids, such as D-Trp. Note that these agonists expressed low levels of receptor activation. In the next stage of the study, dipeptides and tripeptides from the previously mentioned amino acids were examined. Interestingly, TAS2R39 was activated by Trp-Trp and Leu-Trp dipeptides but not by other Trp-containing combinations (like Trp-Leu), which indicates that the interaction between these kinds of ligands and receptors cannot be described solely by the presence or position of the activating amino acid in a dipeptide. Of the tested tripeptides, Trp-Trp-Trp and Leu-Leu-Leu both activated TAS2R39 (41). Finally, larger peptides found in the cheese-maturation process also activated TAS2R39 as well as TAS2R1.
Although most ligands now discovered act as agonists of the TAS2R39, some studies also identified antagonists for this receptor (42). Like many agonists, specific blockers for these bitter taste receptors come from the flavanone group of compounds. Two of the 14 screened flavanones 6,3’-dimethoxyflavanone and 4’-fluoro-6-methoxyflavanone showed clear inhibiting tendencies against TAS2R39, while 6-methoxyflavanone displayed a less inhibiting tendency. Researchers also tested the specificity of these ligands to TAS2R39 by comparing this bitter taste receptor with the other receptor that binds many of the flavonoids, i.e. TAS2R14. Here an inhibition was also observed but to a lesser extent than with TAS2R39. Structural analysis of these inhibitors showed that for a ligand to act as an antagonist on this bitter taste receptor, a methoxy group on position 6 of the A ring is mandatory, as is the absence of a double bond in the C ring of the structure (42). All currently known TAS2R39 exclusive receptor ligands can be found in the Table 1, while all known TAS2R39 ligands are listed in the Supplementary Table S1.
What is evident from the current data is that since studies of these bitter taste receptors are still ongoing and the first specific ligands were discovered only a decade ago, we still have much to learn about the interactions between the receptor and its ligands and about how to define more ligands, specific agonists, and antagonists. More specific ligands on TAS2R39 could help us discover more specific properties of this bitter taste receptor and clarify its physiological and potential pathophysiological roles.
Animal models, especially rat and mouse, are key to understanding protein function in humans when there are orthologues for the protein under study. It is not surprising, therefore, that, apart from in humans, our knowledge of bitter taste receptors in these two species is the broadest. Roughly 35 functional genes have been discovered in mice (45) and roughly the same number have been discovered in rats (46). However, the mystery surrounding bitter taste receptors in these animals remains. Although some have been deorphanized (47), there is much more that we do not know. We can see that – like in humans – rats and mice possess different groups of TAS2Rs, with some responding to a broad range of ligands and others being tuned more specifically. Also, just as we observed the expression of taste receptors in a myriad of human tissues, the same is true for these rodents: bitter receptors were detected, for example, in the nasal mucosa (14), vascular system (48), and intestinal system (49) of rats, and probably also appear in many other tissues.
Although their name appears to suggest that they have a simple assignment, bitter taste receptors are involved in numerous biological processes and are therefore an important component of homeostasis. As stated earlier, their primary function is, in conjunction with other taste receptors, to sense the nutritional constituents of ingested food, but they also have a specific role in sensing the presence of toxic components such as plant alkaloids to prevent their ingestion before they harm the organism (15). However, not all bitter compounds are dangerous and some are beneficial to our health (50). Moreover, many common foods and beverages, such as cocoa beans, coffee and beer, are bitter, and we still enjoy their taste. As well as taste sensing, numerous studies have analyzed the function of this receptor in other tissues and systems. There is reliable evidence that bitter taste receptors are involved in glucose homeostasis (51). A possible role of various bitter taste receptors (including the ortholog to human TAS2R39, TAS2R139) in the control of food intake has been studied in the gastrointestinal system of rats (52). Bitter compounds and, in turn, TAS2Rs, have been linked with controlling the secretion of ghrelin, a hormone known as the hunger hormone, which, when released, increases food intake (52). Protein YY (PYY), glucagon-like peptide 1 (GLP-1) and cholecystokinin (CCK) have also been identified as enterohormones that control the appetite (53) and can be influenced by bitter compounds. It was discovered that by activating certain TAS2Rs, such as TAS2R5, GLP-1 secretion can be increased, thus leading to a decrease in food intake in rats. However, when TAS2R39 was targeted specifically, PYY secretion increased with no influence on food intake. Also, when a combination of ligands that bind to other bitter taste receptors but are preferential to TAS2R39 was tested, an increase in food intake was noted (54).
Another area where bitter taste receptors are important is the respiratory system. Their presence in this system has been well documented (55), while it is suggested that taste receptors there are important components of the innate immune system. TAS2R39 has been detected in the human bronchi but it is unlikely to be involved in bronchial relaxation since the ligands that bind to it produced no effects (17). However, once non-specific agonists were used, bronchial relaxation was observed, so the role of other bitter taste receptors cannot be ignored. The importance of TAS2Rs in the respiratory system came out of a study that inspected nasal mucosa in patients suffering from allergies. Both healthy individuals and allergic rhinitis patients were included in the study, which showed that bitter taste receptors, including TAS2R39, are ubiquitously present in nasal tissue and that their expression is increased in allergic patients. Further experiments revealed that TAS2R39 expression in this type of tissue increases when stimulated by certain cytokines, namely IL-3, IL-5, IL-10 and TGF-β. Whereas interleukins induced the expression of several bitter taste receptors, TGF-β up-regulated only TAS2R39 (14). Moreover, stimulation of the nasal mucosa by various bitter compounds triggered constriction of the mucosa in patients, while in rats, vascular constriction of the mucosa was observed. This suggests that bitter taste receptors may have an important role in constriction of the nasal mucosa, thus improving congestion and controlling allergic rhinitis. However, as some bitter taste receptors (including TAS2R39) were up-regulated by inflammatory cytokines, it may be that these receptors also participate in the pathogenesis of inflammatory diseases. These conflicting results reinforce the need for more thorough studies to decipher the role of these receptors in the homeostasis of organisms.
As this is a fairly novel topic of research, the significance of TAS2R39 is yet to be determined. Though present in extraoral locations, it is yet to be fully determined in which specific tissues and at what levels it is present. A more detailed future analysis of the signaling pathway could enable us to better understand its role in extraoral tissues and in turn make it easier to modulate it. Primary research exploring its role in the control of food intake is promising, though more concrete studies are needed to decipher its role in the control of satiety. Its role in the respiratory system also remains unclear since a possible preventive role in congestion seems to be at odds with its possible pro-inflammatory effect. These discrepancies with regard to TAS2R39 show us that without further research, this receptor will remain the hidden one.
AA and MP conceived the idea and drafted a proposal. FJ reviewed the literature and scripted the basis of the manuscript. ER-G and RB-D elaborated the figures and tables. MB and XT critically reviewed the manuscript. All the authors reviewed the manuscript and approved the final version.
This work was supported the Proyecto AGL2017-83477-R financiado por MCIN/AEI/10.13039/501100011033/FEDER “Una manera de hacer Europa” This project has received funding from the European Union’s Horizon 2020 research and innovation programme under the Marie Skłodowska Curie grant agreement No 945413 and from the Universitat Rovira i Virgili (URV). MP and XT are Serra Húnter fellows. The funding providers had no role in the design, analysis or writing of this article.
The authors declare that this work reflects only their view and that the European Research Executive Agency is not responsible for any use that may be made of the information it contains.
The authors declare that the research was conducted in the absence of any commercial or financial relationships that could be construed as a potential conflict of interest.
All claims expressed in this article are solely those of the authors and do not necessarily represent those of their affiliated organizations, or those of the publisher, the editors and the reviewers. Any product that may be evaluated in this article, or claim that may be made by its manufacturer, is not guaranteed or endorsed by the publisher.
The Supplementary Material for this article can be found online at: https://www.frontiersin.org/articles/10.3389/fendo.2022.854718/full#supplementary-material
1. Chandrashekar J, Hoon MA, Ryba NJP, Zuker CS. The Receptors and Cells for Mammalian Taste. Nature (2006) 444:288–94. doi: 10.1038/nature05401
2. Nomura K, Nakanishi M, Ishidate F, Iwata K, Taruno A. All-Electrical Ca2+-Independent Signal Transduction Mediates Attractive Sodium Taste in Taste Buds. Neuron (2020) 106:816–29.e6. doi: 10.1016/j.neuron.2020.03.006
3. Zhang J, Jin H, Zhang W, Ding C, O’Keeffe S, Ye M, et al. Sour Sensing From the Tongue to the Brain. Cell (2019) 179:392–402.e15. doi: 10.1016/j.cell.2019.08.031
4. Kikut-Ligaj D, Trzcielinska-Lorych J. How Taste Works: Cells, Receptors and Gustatory Perception. Cell Mol Biol Lett (2015) 20:699–716. doi: 10.1515/cmble-2015-0042
5. Weis WI, Kobilka BK. The Molecular Basis of G Protein-Coupled Receptor Activation. Annu Rev Biochem (2018) 87:897–919. doi: 10.1146/annurev-biochem-060614-033910
6. Li F. Taste Perception: From the Tongue to the Testis. Mol Hum Reprod (2013) 19:349–60. doi: 10.1093/molehr/gat009
7. Bachmanov A, Bosak N, Lin C, Matsumoto I, Ohmoto M, Reed D, et al. Genetics of Taste Receptors. Curr Pharm Des (2014) 20(16):2669–83. doi: 10.2174/13816128113199990566
8. Behrens M, Ziegler F. Structure-Function Analyses of Human Bitter Taste Receptors—Where do We Stand? Molecules (2020) 25:4423. doi: 10.3390/molecules25194423
9. Yarmolinsky DA, Zuker CS, Ryba NJP. Common Sense About Taste: From Mammals to Insects. Cell (2009) 139:234–44. doi: 10.1016/j.cell.2009.10.001
10. Meyerhof W, Batram C, Kuhn C, Brockhoff A, Chudoba E, Bufe B, et al. The Molecular Receptive Ranges of Human TAS2R Bitter Taste Receptors. Chem Senses (2009) 35:157–70. doi: 10.1093/chemse/bjp092
11. TAS2R39 Gene - GeneCards | T2R39 Protein | T2R39 Antibody. Available at: https://www.genecards.org/cgi-bin/carddisp.pl?gene=TAS2R39#expression [Accessed May 5, 2021].
12. Behrens M, Foerster S, Staehler F, Raguse JD, Meyerhof W. Gustatory Expression Pattern of the Human TAS2R Bitter Receptor Gene Family Reveals a Heterogenous Population of Bitter Responsive Taste Receptor Cells. J Neurosci (2007) 27:12630–40. doi: 10.1523/JNEUROSCI.1168-07.2007
13. Clark AA, Liggett SB, Munger SD. Extraoral Bitter Taste Receptors as Mediators of Off-Target Drug Effects. FASEB J (2012) 26:4827–31. doi: 10.1096/fj.12-215087
14. Kook JH, Kim HK, Kim HJ, Kim KW, Kim TH, Kang KR, et al. Increased Expression of Bitter Taste Receptors in Human Allergic Nasal Mucosa and Their Contribution to the Shrinkage of Human Nasal Mucosa. Clin Exp Allergy (2016) 46:584–601. doi: 10.1111/cea.12727
15. Behrens M, Meyerhof W. Gustatory and Extragustatory Functions of Mammalian Taste Receptors. Physiol Behav (2011) 105:4–13. doi: 10.1016/j.physbeh.2011.02.010
16. Rozengurt N, Wu SV, Chen MC, Huang C, Sternini C, Rozengurt E. Colocalization of the α-Subunit of Gustducin With PYY and GLP-1 in L Cells of Human Colon. Am J Physiol - Gastrointest Liver Physiol (2006) 291:792–802. doi: 10.1152/ajpgi.00074.2006
17. Grassin-Delyle S, Abrial C, Fayad-Kobeissi S, Brollo M, Faisy C, Alvarez J-C, et al. The Expression and Relaxant Effect of Bitter Taste Receptors in Human Bronchi. Respir Res (2013) 14:134. doi: 10.1186/1465-9921-14-134
18. Chen JG, Ping NN, Liang D, Li MY, Mi YN, Li S, et al. The Expression of Bitter Taste Receptors in Mesenteric, Cerebral and Omental Arteries. Life Sci (2017) 170:16–24. doi: 10.1016/j.lfs.2016.11.010
19. Shaw L, Mansfield C, Colquitt L, Lin C, Ferreira J, Emmetsberger J, et al. Personalized Expression of Bitter “Taste” Receptors in Human Skin. PloS One (2018) 13:e0205322. doi: 10.1371/journal.pone.0205322
20. Zheng K, Lu P, Delpapa E, Bellve K, Deng R, Condon JC, et al. Bitter Taste Receptors as Targets for Tocolytics in Preterm Labor Therapy. FASEB J (2017) 31:4037–52. doi: 10.1096/fj.201601323RR
21. Grassin-Delyle S, Salvator H, Mantov N, Abrial C, Brollo M, Faisy C, et al. Bitter Taste Receptors (TAS2Rs) in Human Lung Macrophages: Receptor Expression and Inhibitory Effects of TAS2R Agonists. Front Physiol (2019) 10:1267. doi: 10.3389/fphys.2019.01267
22. Duarte AC, Rosado T, Costa AR, Santos J, Gallardo E, Quintela T, et al. The Bitter Taste Receptor TAS2R14 Regulates Resveratrol Transport Across the Human Blood-Cerebrospinal Fluid Barrier. Biochem Pharmacol (2020) 177:113953. doi: 10.1016/j.bcp.2020.113953
23. Tissue Expression of TAS2R39 - Summary - The Human Protein Atlas. Available at: https://www.genecards.org/cgi-bin/carddisp.pl?gene=TAS2R39 #expression [Accessed May 5, 2021].
24. Roland WSU, Sanders MPA, Van Buren L, Gouka RJ, Gruppen H, Vincken JP, et al. Snooker Structure-Based Pharmacophore Model Explains Differences in Agonist and Blocker Binding to Bitter Receptor Htas2r39. PloS One (2015) 10:1–14. doi: 10.1371/journal.pone.0118200
25. Roland WSU, Vincken JP, Gouka RJ, Van Buren L, Gruppen H, Smit G. Soy Isoflavones and Other Isoflavonoids Activate the Human Bitter Taste Receptors Htas2r14 and Htas2r39. J Agric Food Chem (2011) 59:11764–71. doi: 10.1021/jf202816u
26. Wong GT, Gannon KS, Margolskee RF. Transduction of Bitter and Sweet Taste by Gustducin. Nature (1996) 381:796–800. doi: 10.1038/381796a0
27. Spielman AI. Gustducin and its Role in Taste. J Dent Res (1998) 77:539–44. doi: 10.1177/00220345980770040601
28. Abe K, Kusakabe Y, Tanemura K, Emori Y, Arai S. Primary Structure and Cell-Type Specific Expression of a Gustatory G Protein-Coupled Receptor Related to Olfactory Receptors. J Biol Chem (1993) 268:12033–9. doi: 10.1016/s0021-9258(19)50304-0
29. Boughter JD, Pumplin DW, Yu C, Christy RC, Smith DV. Differential Expression of-Gustducin in Taste Bud Populations of the Rat and Hamster. J Neurosci (1997) 17(8):2852–8. doi: 10.1523/JNEUROSCI.17-08-02852.1997
30. Roper SD, Chaudhari N. Taste Buds: Cells, Signals and Synapses. Nat Rev Neurosci (2017) 18:485–97. doi: 10.1038/nrn.2017.68
31. Witt M. Anatomy and Development of the Human Taste System. In: Handbook of Clinical Neurology. Amsterdam: Elsevier B.V. (2019) p. 147–71. doi: 10.1016/B978-0-444-63855-7.00010-1
32. Taruno A, Nomura K, Kusakizako T, Ma Z, Nureki O, Foskett JK. Taste Transduction and Channel Synapses in Taste Buds. Pflugers Arch (2021) 473:3–13. doi: 10.1007/s00424-020-02464-4
33. Jeruzal-Świątecka J, Fendler W, Pietruszewska W. Clinical Role of Extraoral Bitter Taste Receptors. Int J Mol Sci (2020) 21:1–23. doi: 10.3390/ijms21145156
34. Ahmad R, Dalziel JE. G Protein-Coupled Receptors in Taste Physiology and Pharmacology. Front Pharmacol (2020) 11:587664. doi: 10.3389/fphar.2020.587664
35. Margolskee RF. Molecular Mechanisms of Bitter and Sweet Taste Transduction. J Biol Chem (2002) 277:1–4. doi: 10.1074/jbc.R100054200
36. Yamazaki T, Sagisaka M, Ikeda R, Nakamura T, Matsuda N, Ishii T, et al. The Human Bitter Taste Receptor Htas2r39 is the Primary Receptor for the Bitterness of Theaflavins. Biosci Biotechnol Biochem (2014) 78:1753–6. doi: 10.1080/09168451.2014.930326
37. Narukawa M, Noga C, Ueno Y, Sato T, Misaka T, Watanabe T. Evaluation of the Bitterness of Green Tea Catechins by a Cell-Based Assay With the Human Bitter Taste Receptor Htas2r39. Biochem Biophys Res Commun (2011) 405:620–5. doi: 10.1016/j.bbrc.2011.01.079
38. Roland WSU, van Buren L, Gruppen H, Driesse M, Gouka RJ, Smit G, et al. Bitter Taste Receptor Activation by Flavonoids and Isoflavonoids: Modeled Structural Requirements for Activation of Htas2r14 and Htas2r39. J Agric Food Chem (2013) 61:10454–66. doi: 10.1021/jf403387p
39. Morini G, Winnig M, Vennegeerts T, Borgonovo G, Bassoli A. Vanillin Activates Human Bitter Taste Receptors TAS2R14, TAS2R20, and TAS2R39. Front Nutr (2021) 8:683627. doi: 10.3389/fnut.2021.683627
40. Maehashi K, Huang L. Bitter Peptides and Bitter Taste Receptors. Cell Mol Life Sci (2009) 66:1661–71. doi: 10.1007/s00018-009-8755-9
41. Kohl S, Behrens M, Dunkel A, Hofmann T, Meyerhof W. Amino Acids and Peptides Activate at Least Five Members of the Human Bitter Taste Receptor Family. J Agric Food Chem (2013) 61:53–60. doi: 10.1021/jf303146h
42. Roland WSU, Gouka RJ, Gruppen H, Driesse M, van Buren L, Smit G, et al. 6-Methoxyflavanones as Bitter Taste Receptor Blockers for Htas2r39. PloS One (2014) 9:1–10. doi: 10.1371/journal.pone.0094451
43. Yamazaki T, Narukawa M, Mochizuki M, Misaka T, Watanabe T. Activation of the Htas2r14 Human Bitter-Taste Receptor by (-)-Epigallocatechin Gallate and (-)-Epicatechin Gallate. Biosci Biotechnol Biochem (2013) 77:1981–3. doi: 10.1271/BBB.130329
44. Ueno Y, Sakurai T, Okada S, Abe K, Misaka T. Human Bitter Taste Receptors Htas2r8 and Htas2r39 With Differential Functions to Recognize Bitter Peptides. Biosci Biotechnol Biochem (2011) 75:1188–90. doi: 10.1271/bbb.100893
45. Masamoto M, Mitoh Y, Kobashi M, Shigemura N, Yoshida R. Effects of Bitter Receptor Antagonists on Behavioral Lick Responses of Mice. Neurosci Lett (2020) 730:135041. doi: 10.1016/j.neulet.2020.135041
46. Wu SV, Chen MC, Rozengurt E. Genomic Organization, Expression, and Function of Bitter Taste Receptors (T2R) in Mouse and Rat. Physiol Genomics (2005) 22:139–49. doi: 10.1152/physiolgenomics.00030.2005
47. Lossow K, Hübner S, Roudnitzky N, Slack JP, Pollastro F, Behrens M, et al. Comprehensive Analysis of Mouse Bitter Taste Receptors Reveals Different Molecular Receptive Ranges for Orthologous Receptors in Mice and Humans. J Biol Chem (2016) 291:15358–77. doi: 10.1074/jbc.M116.718544
48. Alshehri S. Study on the Effects of Bitter Taste Receptor Agonists on the Rat Pulmonary Artery. [Dissertation]. [Glasgow (UK)]: University of Strathclyde (2020). doi: 10.13140/RG.2.2.11735.91045
49. Kaji I, Karaki SI, Fukami Y, Terasaki M, Kuwahara A. Secretory Effects of a Luminal Bitter Tastant and Expressions of Bitter Taste Receptors, T2Rs, in the Human and Rat Large Intestine. Am J Physiol - Gastrointest Liver Physiol (2009) 296:971–81. doi: 10.1152/ajpgi.90514.2008
50. Nissim I, Dagan-Wiener A, Niv MY. The Taste of Toxicity: A Quantitative Analysis of Bitter and Toxic Molecules. IUBMB Life (2017) 69:938–46. doi: 10.1002/iub.1694
51. Dotson CD, Zhang L, Xu H, Shin YK, Vigues S, Ott SH, et al. Bitter Taste Receptors Influence Glucose Homeostasis. PloS One (2008) 3:e3974. doi: 10.1371/journal.pone.0003974
52. Serrano J, Casanova-Martí À, Depoortere I, Blay MTMT, Terra X, Pinent M, et al. Subchronic Treatment With Grape-Seed Phenolics Inhibits Ghrelin Production Despite a Short-Term Stimulation of Ghrelin Secretion Produced by Bitter-Sensing Flavanols. Mol Nutr Food Res (2016) 60:2554–64. doi: 10.1002/mnfr.201600242
53. Steinert RE, Feinle-Bisset C, Asarian L, Horowitz M, Beglinger C, Geary N. Ghrelin, CCK, GLP-1, and PYY(3-36): Secretory Controls and Physiological Roles in Eating and Glycemia in Health, Obesity, and After RYGB. Physiol Rev (2017) 97:411–63. doi: 10.1152/physrev.00031.2014
54. Grau-Bové C, Miguéns A, González C, Rodríguez E, Blay MT. Modulation of Food Intake by Differential TAS2R Stimulation in Rat. Nutrients (2020) 12:3784. doi: 10.3390/nu12123784
Keywords: TAS2R39, bitter taste, catechin, GPCR, food intake, respiratory system, TAS2R39 agonist, TAS2R39 antagonist
Citation: Jalševac F, Terra X, Rodríguez-Gallego E, Beltran-Debón R, Blay MT, Pinent M and Ardévol A (2022) The Hidden One: What We Know About Bitter Taste Receptor 39. Front. Endocrinol. 13:854718. doi: 10.3389/fendo.2022.854718
Received: 14 January 2022; Accepted: 04 February 2022;
Published: 08 March 2022.
Edited by:
Nina Vardjan, University of Ljubljana, SloveniaReviewed by:
Milan Sencanski, University of Belgrade, SerbiaCopyright © 2022 Jalševac, Terra, Rodríguez-Gallego, Beltran-Debón, Blay, Pinent and Ardévol. This is an open-access article distributed under the terms of the Creative Commons Attribution License (CC BY). The use, distribution or reproduction in other forums is permitted, provided the original author(s) and the copyright owner(s) are credited and that the original publication in this journal is cited, in accordance with accepted academic practice. No use, distribution or reproduction is permitted which does not comply with these terms.
*Correspondence: Montserrat Pinent, bW9udHNlcnJhdC5waW5lbnRAdXJ2LmNhdA==
Disclaimer: All claims expressed in this article are solely those of the authors and do not necessarily represent those of their affiliated organizations, or those of the publisher, the editors and the reviewers. Any product that may be evaluated in this article or claim that may be made by its manufacturer is not guaranteed or endorsed by the publisher.
Research integrity at Frontiers
Learn more about the work of our research integrity team to safeguard the quality of each article we publish.