- 1Ministry of Education and Shanghai Key Laboratory of Children’s Environmental Health, Xinhua Hospital, Shanghai Jiao Tong University School of Medicine, Shanghai, China
- 2Guangxi Key Laboratory of Tumor Immunology and Microenvironmental Regulation, Guilin Medical University, Guilin, China
Triclosan (TCS) is an antimicrobial chemical widely used in personal care products. Most of the TCS component is discharged and enters the aquatic ecosystem after usage. TCS has a similar structure as thyroid hormones that are synthesized by thyroid follicular epithelial cells, thus TCS has a potential endocrine disrupting effect. It is still not clear how the different levels of the environmental TCS would affect early development in vivo. This study examines the effects of TCS on thyroid hormone secretion and the early development of zebrafish. The fertilized zebrafish eggs were exposed to TCS at 0 (control), 3, 30, 100, 300, and 900 ng/mL, and the hatching rate and the larvae mortality were inspected within the first 14 days. The total triiodothyronine (TT3), total thyroxine (TT4), free triiodothyronine (FT3), and free thyroxine (FT4) were measured at 7, 14, and 120 days post-fertilization (dpf). The histopathological examinations of thyroid follicles were conducted at 120 dpf. TCS exposure at 30-300 ng/mL reduced the hatching rate of larvae to 34.5% to 28.2 % in the first 48 hours and 93.8 .7 % to 86.8 % at 72 h. Extremely high TCS exposure (900 ng/mL) strongly inhibited the hatching rate, and all the larvae died within 1 day. Exposure to TCS from 3 to 300 ng/mL reduced the thyroid hormones production. The mean TT3 and FT3 levels of zebrafish decreased in 300 ng/mL TCS at 14 dpf (300 ng/mL TCS vs. control : TT3 , 0.19 ± 0.08 vs. 0.39 ± 0.06; FT3, 19.21 ± 3.13 vs. 28.53 ± 1.98 pg/mg), and the FT4 decreased at 120 dpf ( 0.09 ± 0.04 vs. 0.20 ± 0.14 pg/mg). At 120 dpf , in the 300 ng/mL TCS exposure group, the nuclear area and the height of thyroid follicular epithelial cells became greater, and the follicle cell layer got thicker. This happened along with follicle hyperplasia, nuclear hypertrophy, and angiogenesis in the thyroid. Our study demonstrated that early life exposure to high TCS levels reduces the rate and speed of embryos hatching, and induces the histopathological change of thyroid follicle, and decreases the TT3, FT3, and FT4 production in zebrafish.
Introduction
Thyroid hormones are synthesized and secreted by the thyroid follicular epithelial cells, and are critical for fetal growth and neuro-development in early life (1, 2). The thyroid gland is sensitive to environmental endocrine disruptor chemicals (EDCs) during early life (3). Triclosan (TCS) is a widely used antimicrobial agent in personal care products with a similar molecular structure as the thyroid hormone, thus it is a potential EDC. TCS has been widely used in personal care products, such as soaps, toothpastes, shampoos, and cosmetics for more than 40 years (4, 5). Most of TCS is discharged into residential drains after usage, and contaminates the aquatic ecosystem (6, 7). TCS can be detected in rivers, lakes (8), and drinking water (9). The highest TCS concentrations can reach up to 5370 ng/L in effluent water of wastewater treatment plants (10) and 1023 ng/L in rivers (11). TCS is bio-accumulated in fish and other aquatic species (12–14), and can also be detected in human urine, blood, fetal cord blood, and breast milk (15–17), suggesting there is a wide exposure to TCS of the general population in different regions of the world. For example, TCS has been detected in pregnant women with the median urinary concentration ranging from 0.4 μg/L to 26.5 μg/L (18–22).
Concerns have been raised on the potential risk for TCS to perturb thyroid endocrine functioning, in part, due to the structural similarities of TCS and thyroid hormones (23). Several animal studies had examined the potential thyroid-disrupting effect of TCS in rodents (24, 25). Most of the rodent studies found that TCS exposure decreased the serum total thyroxine (T4) and triiodothyronine (T3) levels (26, 27) at the doses ranging from 1 to 300 mg/kg/d. Considering TCS is a persistent and ubiquitous pollutant in the aquatic environment, the thyroid disturbing effect of TCS at different aquatic relevant concentrations needs to be studied. But few studies have directly evaluated the effect of TCS early exposure in fish (28, 29). Moreover, the histopathologic examination is considered the gold standard for evaluating the pathological changes in tissues and organs (30), but few studies have explored the impact of TCS exposure on thyroid follicular pathological changes in fish.
Zebrafish has been a good model for studies of thyroid function, since the development of its thyroid system is comparable to human (31). None has examined the effect of early exposure to TCS from the embryo stage at the environment-related level or the bio-sample concentrations, on the thyroid gland and the related hormone levels. In this study, by using a zebrafish model, we examined the impacts of early exposure to TCS at environmentally relevant concentrations on the histopathology of thyroid follicles and the thyroid hormones levels.
Materials and Methods
TCS
TCS (Irgasan, 5-chloro-2-(2, 4-dichlorophenoxy) phenol, ≥ 97.0 % purity (HPLC), CAS no. 3380-34-5) and dimethyl sulfoxide (DMSO, 99 % purity) were purchased from Sigma-Aldrich (St. Louis, MO).
Zebrafish Maintenance and TCS Exposure
Zebrafish strains of AB wild-type line were used in the study. The male and female zebrafish at 5-months-old were acclimated in tanks containing dechlorinated tap water for 4 weeks before mating under a photoperiod of 14:10 h light/dark cycle. The fish were fed with brine shrimp twice per day.
Fertilized eggs were collected within 30 min after natural mating and unfertilized eggs were discarded. Fertilized eggs were randomly assigned to 0 (0.01 % DMSO as solvent control), 3, 30, 100, 300, and 900 ng/mL TCS (600 eggs per group) and raised up to 7 and 14 days at 28.0 ± 0.5°C under 14:10 h light/dark photoperiod cycle (32). These TCS concentrations were set based on the environmental exposure levels of both humans and wildlife (17, 33). There was 0.01% DMSO used as a solvent to enhance TCS solubility (34), as the control group . The zebrafish embryos were tolerant to low concentrations (0.01 %) of DMSO (35).
There were 200 fertilized eggs with a density of one embryo per 2 mL medium put into a culture dish until 72 h post-fertilization (hpf). The larvae were transferred into glass tanks with a density of one larva per 60 mL medium until 14 days post-fertilization (dpf) (36). After 14 dpf, the zebrafish were transferred into larger glass tanks with a density of one fish per 200 mL medium (36). Half of the medium was changed twice per day to ensure TCS concentration stable. The number of larvae hatched were inspected and recorded twice per day in the first 3 dpf (i.e., 72 hpf). The larvae were fed with paramecium twice per day until 14 dpf. Starting from 15 dpf, larvae were fed brine shrimp. The pH of raising water was maintained at 7.5 ± 0.5, conductivity was maintained at 550 ± 50 μS, temperature was maintained at 28 ± 0.5°C , and the dissolved oxygen of the raising water was monitored (32).
Hatching Rate and Mortality
The hatching rate was calculated as the number of larvae hatched during the first 3 days divided by the total number of fertilized eggs. The death of larvae was inspected and recorded twice per day for first 14 days, and the dead larvae were removed from dishes/tanks. The mortality was calculated as the number of dead larvae divided by the total number of hatched larvae.
Thyroid Hormone Measurements
At 7, 14, and 120 dpf, the zebrafish were euthanized by immersing in ice-cold water for 40 min (37, 38), and thyroid hormones (THs) were measured by ELISA (enzyme-linked immunosorbent assay) kits. Specifically, at 7 dpf, 50 larvae were homogenated and put together for one measurement. Three replicates (50 larvae each replicate) were conducted. At 14 dpf, 100 larvae were gathered for each replicate for THs measurements. For the zebrafish at 120 dpf, the heads were removed for thyroid histological analysis, and the rest of fish body was used for thyroid hormones measurement.
The free triiodothyronine (FT3), free thyroxine (FT4), total triiodothyronine (TT3), and total thyroxine (TT4) levels were measured by ELISA (Labor Diagnostika Nord commercial kit, Nordhorn, Germany) (39). The larvae samples (at 7 and 14 dpf) and the body of the fish (with head, viscera, and gut removed, at 120 dpf) were sonicated in cold 0.01 M phosphate buffer saline (PBS) at w/v of 1mg/5μL (w: wet weight of samples, v: volume of 0.01 M PBS, mg/μL), put on intermittent sonic oscillation for 5 min, vortexed vigorously for 10 min, then centrifuged at 12,000 × g at 4°C for 5 min. The supernatant was collected to measure thyroid hormones and total protein concentration. Total protein concentration was measured with the Pierce© BCA Protein Assay Kit (Thermo Fisher Scientific Inc., Rockford, IL) to normalize thyroid hormone concentration (40). The limit of detection (LOD) was 0.1 ng/mL for TT3, 8 nmol/L for TT4, 0.3 pg/mL for FT3, and 1 pg/mL for FT4. Values below the limit of detection were replaced with values equal to the LOD divided to 2.
Hematoxylin and Eosin (H&E) Staining of Thyroid Gland and Histological Analysis
The histological analysis of the thyroid gland was conducted in zebrafish at 120 dpf. The heads of zebrafish were fixed in 10% neutral formalin (Zhongshan Beijing Biotechnology Co., Ltd., Beijing, China) for at least 12 h, and transferred to 70% ethanol, all at room temperature. Each zebrafish head was placed in processing cassettes, dehydrated through a serial alcohol gradient, and embedded in the wax blocks.
Serial transverse cross-sections were cut using a microtome 5 μm and dewaxed in xylene, rehydrated through decreasing concentrations of ethanol, and washed in PBS. The sections were then stained with hematoxylin for 4−5 min and with eosin for 1−2 min (Zhongshan Beijing Biotechnology Co., Ltd.). Thyroid follicles were located according to their being dispersed among the afferent branchial arterioles (for example, ventral aorta) in the subpharyngeal region (41). Stained thyroid follicle sections were photographed under light microscopy (BX53−p; Olympus Corporation, Tokyo, Japan).
The Nuclear Size and Height of Follicular Epithelial Cells
Photographs of each follicle were taken at the largest follicle diameter (determined by observing serial sections) with an Olympus digital camera (BX53−p; Olympus Corporation). The height of follicular epithelial cells and the long and short diameters of their cell nuclei were quantitatively analyzed. The nuclear size was calculated as the long diameter × short diameter × π/4 (41). At least 3-5 histological tissue sections per one fish sample and three different follicles (or three separate areas) per one section were selected for measuring in cases where the follicular structure was unclear or absent. The nuclear size was calculated in at least 50 thyroid follicular cell nuclei per fish at a magnification of ×1000. The height of a follicular epithelial cell was calculated by the mean of five measures along the follicle perimeter at equal intervals, and 40-80 follicular epithelial cells were measured for each fish (42). Image Pro−Plus 6.0 software (Media Cybernetics, Inc., Rockville, MD) was used to analyze the photos.
Statistical Analysis
The ANOVA F-test was used to compare the differences in thyroid hormone levels, the height of thyroid follicle epithelial cells and nuclear size, and chi square test was used to compare the difference in hatching rate and mortality of larvae among TCS exposure groups. We used linear regression models to evaluate the associations of TCS exposures with thyroid hormones and thyroid follicle histopathological changes. The level of significance was two-sided P value < 0.05. All analyses were performed using the SAS 9.3 software (SAS Institute, Inc., Cary, NC).
Results
TCS Exposure and Hatching Rate of Larva
All the hatched larvae presented a well-developed head, body, and tail within 72 h. At 48 hpf, the hatching rate was 42% in the control group, and 47.8 % in 3 ng/ml TCS exposure group . But with the increase of TCS exposure concentrations , the hatching rate significantly dropped from 34.5 % to 4.3 % in 30 - 900 ng/mL TCS exposure (P < 0.01. Table 1). At 72 hpf, the hatching rate was 95.8 % in the control group, while the hatching rates in 3-300 ng/mL TCS exposure groups slightly reduced as TCS dose increased, but the differences were not statistically significant compared to the control. However, 900 ng/mL of TCS exposure strongly inhibited the hatching rate: only 4.3 % of fertilized eggs hatched at 48 hpf, and 18.3 % at 72 hpf (Table 1).
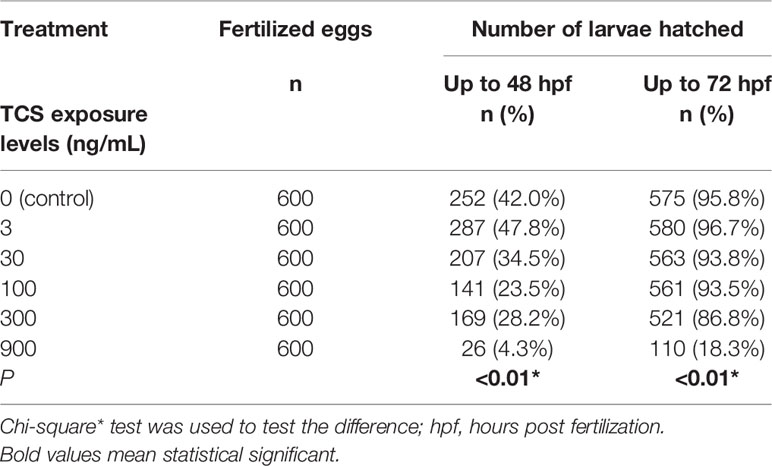
Table 1 The association of TCS exposure concentration and the hatching rate of zebrafish embryos within 48 and 72 h.
TCS Exposure and Mortality of Zebrafish Larvae
All the larvae died shortly after hatching in 900 ng/mL TCS exposure group, and the mortality reached 100 % at 3 dpf. But in the TCS exposure 3 to 300 ng/mL groups, the mortalities of larvae were comparable to the control group at 7 dpf and 14 dpf, respectively (Table 2).
TCS Exposure and Thyroid Hormone Levels in Larvae and Zebrafish
We further measured the thyroid hormone levels in larvae after exposure to different concentrations of TCS at different time points. At 7 dpf, the TT3 and FT3 levels were comparable as control in 0 to 300 ng/mL TCS exposure groups; while the TT4 and FT4 levels were reduced with TCS exposure doses from 0 to 300 ng/mL, but the difference was not statistically significant (Supplementary Table S1).
At 14 dpf, the TT3 and FT3 levels in larvae were significantly lower in all the TCS exposure groups when compared to the control group. TT3 levels were on average 0.20 to 0.21 ng/mg lower in 3-300 ng/mL TCS exposure groups than that of the control group (all P < 0.05, Table 3), and the FT3 levels were 9.49, 10.74, and 9.32 pg/mg lower in TCS 30, 100, and 300 ng/mL groups, respectively (P < 0.01 , Table 3). The FT4 level tended to decrease with the increase of TCS exposure doses (P trend < 0.05, Table 3); while TT4 levels had no statistically significant changes in 0-300 ng/mL TCS exposure groups (Table 3).

Table 3 The influence of TCS exposure on the thyroid hormone level of zebrafish larvae at 14 days post fertilization.
At 120 dpf, the FT4 levels were 0.14, 0.12, and 0.11 pg/mg lower in 30, 100, and 300 ng/mL TCS groups when compared to the control group, respectively (Figure 1 and Supplementary Table S2, all P < 0.01). The TT3, TT4, and FT3 levels were comparable among the five TCS exposure groups. After adjusted for sex, the FT4 levels were still lower in TCS 30, 100, and 300 ng/mL groups than that in the control group (all P < 0.01, Supplementary Table S3).
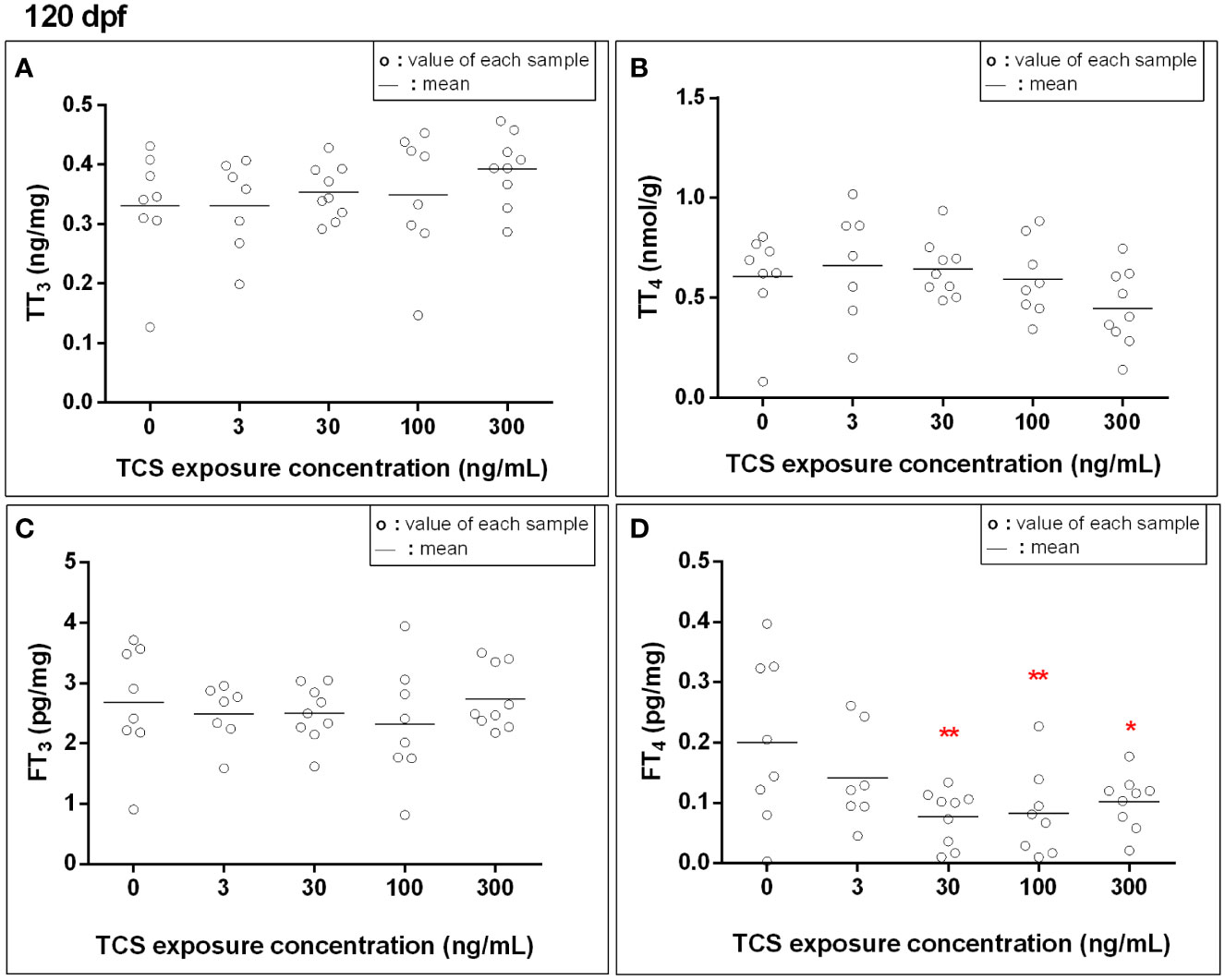
Figure 1 The thyroid hormone levels [(A): total triiodothyronine, (B): total thyroxine, (C): free triiodothyronine, (D): free thyroxine] in zebrafish exposed to TCS for 120 days after fertilizing. Thyroid hormone concentrations in fish at 120 dpf were measured for each exposure group, with each sample homogenized from every single fish at 120 dpf. P trend for FT4 = 0.01. *P < 0.05, **P < 0.01.
TCS Exposure and Thyroid Histopathological Change in Zebrafish
We further examined the histological change of the thyroid gland in 120 dpf zebrafish. Ventral aorta was used to locate the dispersed thyroid follicles. In 3-300 ng/mL TCS exposure groups, the thyroid follicular epithelial cells became hyperplasia (increased number of follicular cell) and hypertrophy (enlarged follicular cell size and nuclear size), shown as increased nuclear area and cell height of thyroid follicle epithelial cells (Figures 2 and 3, Supplementary Table S4). At the same time, fish had oval thyroid follicles composed of a uniform monolayer of cuboidal thyrocytes and lumen filled with pink-stained colloid in most of the follicles in the control group (Figures 3A, F). But after exposure to TCS for 120 days, colloid depletion of follicles and angiogenesis (the development of new blood vessels from an existing vasculature) were observed (Figures 3B–E at 400×; G, H, I, and J at 1000×). TCS exposure induced follicle hyperplasia (Figures 3C–E), and nuclear hypertrophy ( Figures 3G–J), and the thickening of the layer of follicle epithelial cell in those follicles (Figures 3C–E). In the zebrafish exposed to high TCS levels at 100 ng/mL and 300 ng/mL, the thyroid follicle cells had obvious morphological alterations, for example, the follicle cells became significantly larger and follicular interstitial hyperplasia (Figures 3C–E, G).
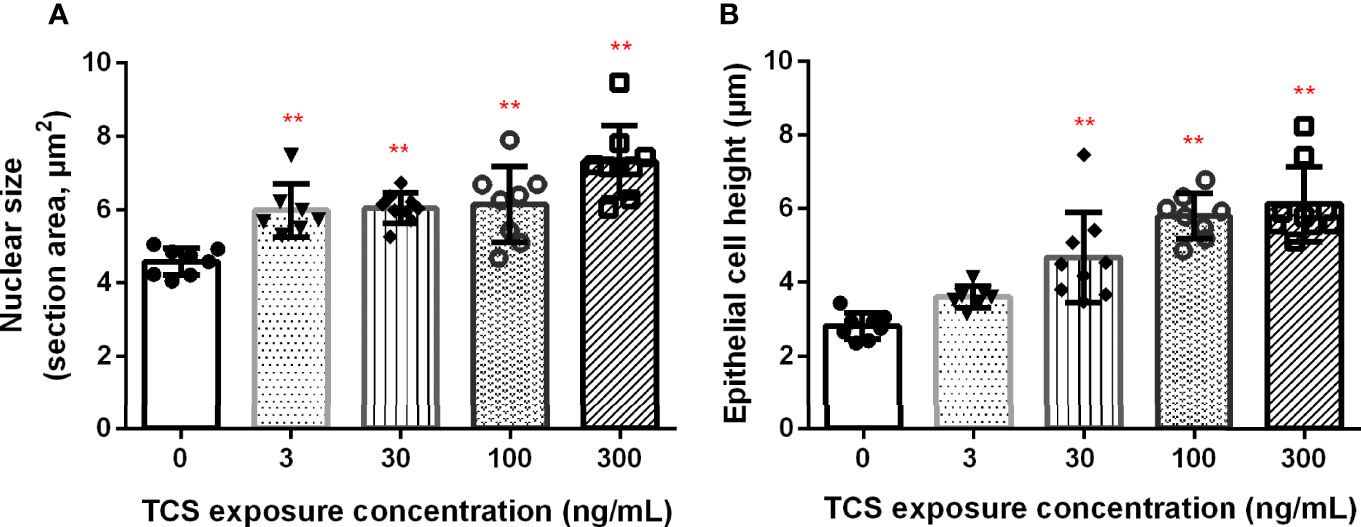
Figure 2 The association between TCS exposure concentrations and nuclear area (A) and the height (B) of thyroid follicle cells. P trend < 0.0001 both for nuclear size and epithelial height of the thyroid. ** P< 0.01 in the TCS exposure group vs. the control group.
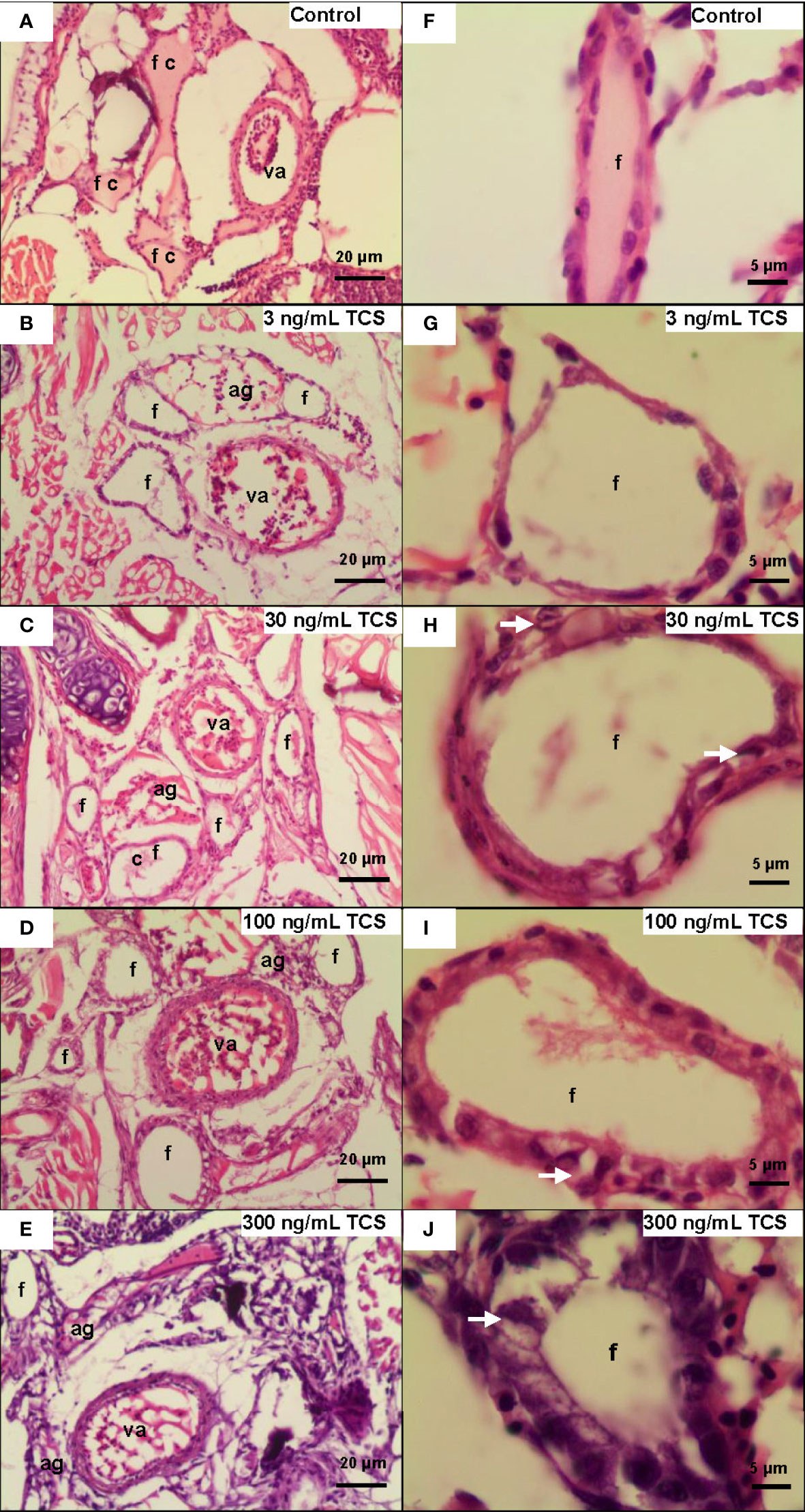
Figure 3 The morphological changes of thyroid follicles in different TCS concentration groups. (A) In the control group, the zebrafish follicle squamous to cuboidal follicular epithelium, with colloid in follicle at 400× and 1000× (F). (B–E) Follicle hyperplasia with decreased colloid in the lumen and angiogenesis in TCS 3, 30, 100, and 300 ng/mL exposure at 400×. (G–J) Hypertrophy and hyperplasia of follicle cells in TCS 3, 30, 100, and 300 ng/mL exposure at 1000×. (va = ventral aorta; c = colloid; f = thyroid follicle; ag = angiogenesis; white arrows = hypertrophy).
Discussion
In this study, we examined the disrupting effects of early exposure to TCS on the thyroid hormones in larvae and adult zebrafish, and the pathological changes of thyroid follicles. We found that the hatching rate of zebrafish embryos reduced whin 48 hpf with high TCS exposure, while the hatching rate became similar as the control when exposing to TCS at 0 - 300 ng/mL. TCS exposure did not change the mortality of larvae at 0 - 300 ng/mL, either. But in the extremely high TCS exposure (900 ng/mL), both the hatching rate and the survival of larvae were severely reduced. As to the effects of TCS on thyroid hormones, the levels of TT3 and FT3 were lower in larvae at 14 dpf in 30, 100, and 300 ng/mL TCS exposure, and the FT4 level was lower at 120 dpf in 30, 100, and 300 ng/mL TCS exposure. Moreover, with increasing concentration of TCS exposure, the epithelial height and nuclear size (area) of thyroid follicular cell turned larger in the thyroid gland of adult zebrafish at 120 dpf.
Zebrafish is an ideal animal model for study of chemical pollutants in water and thyroid hormone-disruption, partly due to its high (71 %) genetic similarity to humans (43). We set 2, 3, 7, and 14 days for larvae phase TCS exposure, 120 days (long-time) for adult phase TCS exposure (36). In this study, TCS exposure duration time was chosen according to the physiological development characteristics of zebrafish. The thyroid hormone level of zebrafish became stable after 7 dpf (44), and zebrafish reach sexual maturity at 120 dpf (45). Series of comparable TCS concentrations with natural and human exposure concentration were set in this study. Similar to our results, a previous study also found that significantly delayed hatchability of fertilized eggs and increased mortality of larvae in TCS 500 ng/mL exposure for 6 days (33). The influence of EDCs on zebrafish’s thyroid histopathology has been explored in many other related EDCs, such as perchlorate (46), arsenate (47), fluoride (48), and microcystin-LR (49). To date, there was only one study focused on the TCS exposure to adult zebrafish and thyroid histopathology (29). Consistent with our results, it reported that the thyroid follicle epithelium was changed in the sub-chronic (21 days) TCS treated fish (29). The reduction or increase in height of thyroid follicle cells are both the evidence of pathological diagnosis in thyroid dysfunction.
In previous studies, Schnitzler et al. (28) found that 20, 50, and 100 ng/mL TCS exposure to embryos decreased T4 and T3 levels at 9 days post-hatching (dph) and 12 dph, and increased T4 levels at 15 dph in Cyprinodon variegatus. Pinto et al. (29) assessed the effect and possible mechanism of TCS on adult zebrafish. They found that TCS exposure to adult zebrafish (about 100 μg/g per day for 21 days via diet) induced a reduction in circulating thyroid hormones, hyperplasia of follicles and the thyrocyte height. In our experiments, the TT4 and FT4 levels tended to be decreased with higher TCS exposure after 7 days, although they were not significant. The obvious thyroid disrupting effect by TCS shown at 14 dpf, and the FT4 decreased significantly with the increasing TCS exposure concentration both at 14 and 120 dpf. The impact of TCS exposure on thyroid hormone levels (TT3 and FT3) at 14 dpf provides the causal support for the findings in the human population (17). In the fish models, only three studies have examined the influence of TCS exposure on thyroid disruption (28, 29). Similar to our results, one study found that TCS exposure delayed the hatching of 6-13 h in medaka fish (28). A recent study found that the offspring of zebrafish exposed to TCS decreased the survival rate and delayed maturation (50), which might be partially explained by decreased thyroid hormone resulting from TCS exposure (50). The adverse effect of long-term exposure to TCS on thyroid hormone levels might be due to the cumulative effect of TCS on the development of zebrafish thyroid glands.
Most of the TT4 and a few of the TT3 are synthesized by thyroid follicular cells directly and then are transported to blood and be functioned in tissues (51). FT3 is the most active free form which comes from FT4 deiodination. FT3 and FT4, TT3 and TT4 can be transformed into each other and maintain dynamic balance in the blood (51). TT4 and TT3 are usually used as an indicator of the reserve capacity of the thyroid gland. In this study, under the high TCS exposure levels, TT3 and FT3, rather than TT4 or FT4, decreased statistically significantly in larvae at 14 dpf. Continuous exposing to 120 dpf, only the FT4 level decreased significantly. More studies about the mechanism still need to be explored.
In our study, since the larvae all died by day 3 after they were hatched, thyroid hormone levels were not measured in the 900 ng/mL TCS exposure group. We used the whole body of larvae fish at 7 and 14 dpf and the body without head, viscera, and gut of adult fish at 120 dpf to measure the thyroid hormone levels as most previous studies. In zebrafish, thyroid hormone is expressed in both hepatic and muscle (52). If adult zebrafish were used as models, plasma or body are generally used as biological samples (53). Thyroid hormone levels in muscle can reflect the growth and development of the body (52), and in our study, the thyroid hormone concentration in the muscle was found related to the TCS exposure level and therefore presumably affects the body growth of the zebrafish.
In this study, we selected the TCS exposure (3, 30, 100, 300, and 900 ng/mL) based on the environmental TCS levels, including wildlife and general population TCS exposure levels in a natural environment. The 3-100 ng/mL TCS is the range of urinary TCS concentrations in pregnant women (17), and 3-30 ng/mL is the TCS range in waste water (10). TCS was found in ranges from 5370 ng/L to 86,161 ng/L in wastewater treatment plants (10), and 1.85 ng/L to 9650 ng/L in rivers and surface water worldwide (9–11, 54, 55). The median urinary TCS concentration is 2.52 ng/mL (17, 19), while the highest urinary TCS concentrations in pregnant women was close to 100 ng/mL (17). It is about 25% of the lethal concentration (LC50) for zebrafish (33). In zebrafish, LC50 values of TCS were 420 μg/L (95% CI, 380 - 450 ng/mL) for embryos at 96 hpf, and 340 μg/L for adult zebrafish within 96 h (33).
The toxic effects of TCS were well recognized on bacterial resistance, reproductive toxicity, and cytotoxicity in aquatic organisms (56–58), but the results on thyroid hormone disruption were inconsistent (59, 60). Most of the animal studies mainly focused on rodents (24, 61). In the adult rat, TCS treatment reduced the levels of blood TT4 and FT4, but no change was found in the levels of blood thyroid stimulating hormone (TSH) (25, 62–64). Another study found that maternal TCS (300 mg/kg/day) exposure during pregnancy decreased approximately 30% of TT4 in dams at the postpartum day 22 in mice (64). Previous studies found that TCS exposure decreased thyroid hormone levels and inhibited metamorphosis (59, 65), or neither changed the thyroid hormone level nor altered the metamorphosis in Xenopus laevis (60).
We speculate that TCS may inhibit thyroid hormones secretion by acting as a disruptor of the hypothalamic-pituitary-thyroid (HPT) axis or due to the increasing thyroid hormones clearance. Some mechanisms have been studied but the data were controversial. TCS exposure may promote (29) or inhibit (66) the sodium-iodide symporter (NIS)-mediated iodide uptake in animals’ thyroid, then increase or decrease the thyroid hormone levels. TCS decreased the thyroid hormones in the circulation of zebrafish but induced TSH gene transcription by a negative feedback regulation (29). TCS was reported to inhibit the activity of thyroid peroxidase (TPO) and thyroid hormone synthesis at concentrations of 50 ng/mL in rat (66) , and increased T4 metabolism and decreased T4 bioavailability by increasing the hepatic enzymes activity of glucuronyltransferase and pentoxyresorufin-O-deethylase (PROD) in the liver (67–69), or by activating the nuclear receptor, pregnane X receptor (PXR) (24, 61). It was also found that TCS caused hypothyroidism in rats through p38/TRHr-dependent pathway (57). Therefore, the mechanism about TCS inhibiting the thyroid hormones is still unclear and needs further investigation.
The result in larvae of this study is consistent with our previous study in humans, in which we observed an inverse association between maternal urinary TCS and cord blood FT3 level in Chinese newborns (17). It is established that thyroid hormone is critical for intrauterine neurodevelopment, due to the regulation of migration, proliferation, and differentiation of fetal neuronal cells, as well as synaptogenesis and myelination (1, 2). The nervous system is highly thyroid hormone sensitive in prenatal life, especially in the early weeks of embryonic development (70).
Our study found that zebrafish exposed with TCS for 120 days (from embryo to adult phase) caused thyroid histopathology changes in 3, 30, 100, and 300 ng/mL TCS exposure, and disturbed the thyroid hormone level (FT4) in 30, 100, and 300 ng/mL TCS exposure. This is the first study to explore the thyroid disturbed effect by long-term TCS exposure starting from the early life in zebrafish. It has been reported that the formation, growth, and differentiation of thyroid follicles in zebrafish appear to be independent of TSH (71), although the TSH receptor is responsible for thyroid gland differentiation in zebrafish (72). Future study with measurements on TSH level can lead to exploring the potential disruptive effect of TCS on the feedback mechanism of HPT axis. Considering an environmental TCS exposure, we set various TCS concentrations in water from very low to high levels in our experiment but did not measure the direct TCS concentration in the fish.
We found that exposure to TCS delayed the hatching of zebrafish embryos, decreased the thyroid hormone (FT3 and TT3) levels in the zebrafish larvae and the FT4 level in adult zebrafish (even adjusted for age). Moreover, TCS exposure affected the structure of thyroid follicles by increasing nuclear area and epithelial cell height of zebrafish thyroid follicles. Considering the reduced level of thyroid hormones which are crucial for neurodevelopment (73), our study raises the concern that early TCS exposure (from conception) may profoundly influence child neurobehavioral development at such a highly sensitive window.
Data Availability Statement
The raw data supporting the conclusions of this article will be made available by the authors, without undue reservation.
Ethics Statement
The studies involving animals were reviewed and approved by the Ethics Committee of Xinhua Hospital Affiliated to Shanghai Jiao Tong University School of Medicine.
Author Contributions
FO conceptualized the study. FO, NT, and PF implemented the study, FO and NT interpretated data and drafted the manuscript. FO, NT, PF, WYW, WJW, and XY contributed to acquisition and analysis of research data. LC had intensively revised the manuscript. All authors have reviewed and approved the manuscript as submitted.
Funding
This study was supported by grants from the National Natural Science Foundation of China [grant number No. 81961128023; 81673178], Shanghai Municipal Education Commission - Gaofeng Clinical Medicine Grant [grant number 20152518], and in part by the Collaborative Innovation Program of Shanghai Municipal Health Commission [grant number 2020CXJQ01].
Conflict of Interest
The authors declare that the research was conducted in the absence of any commercial or financial relationships that could be construed as a potential conflict of interest.
Publisher’s Note
All claims expressed in this article are solely those of the authors and do not necessarily represent those of their affiliated organizations, or those of the publisher, the editors and the reviewers. Any product that may be evaluated in this article, or claim that may be made by its manufacturer, is not guaranteed or endorsed by the publisher.
Acknowledgments
We thank Mr. Yangou Du (McGill University, Canada) for the reviewing and editing of our manuscript.
Supplementary Material
The Supplementary Material for this article can be found online at: https://www.frontiersin.org/articles/10.3389/fendo.2022.850231/full#supplementary-material
References
1. Lavado-Autric R, Auso E, Garcia-Velasco JV, Arufe Mdel C, Escobar del Rey F, Berbel P, et al. Early Maternal Hypothyroxinemia Alters Histogenesis and Cerebral Cortex Cytoarchitecture of the Progeny. J Clin Invest (2003) 111:1073–82. doi: 10.1172/JCI16262
2. Bernal J. Thyroid Hormones and Brain Development. Vitam Horm (2005) 71:95–122. doi: 10.1016/S0083-6729(05)71004-9
3. Brouwer A, Morse DC, Lans MC, Schuur AG, Murk AJ, Klasson-Wehler E, et al. Interactions of Persistent Environmental Organohalogens With the Thyroid Hormone System: Mechanisms and Possible Consequences for Animal and Human Health. Toxicol Ind Health (1998) 14:59–84. doi: 10.1177/074823379801400107
4. Witorsch RJ. Critical Analysis of Endocrine Disruptive Activity of Triclosan and its Relevance to Human Exposure Through the Use of Personal Care Products. Crit Rev Toxicol (2014) 44:535–55. doi: 10.3109/10408444.2014.910754
5. Jones RD, Jampani HB, Newman JL, Lee AS. Triclosan: A Review of Effectiveness and Safety in Health Care Settings. Am J Infect Control (2000) 28:184–96. doi: 10.1067/mic.2000.102378
6. Reiss R, Mackay N, Habig C, Griffin J. An Ecological Risk Assessment for Triclosan in Lotic Systems Following Discharge From Wastewater Treatment Plants in the United States. Environ Toxicol Chem (2002) 21:2483–92. doi: 10.1002/etc.5620211130
7. Dann AB, Hontela A. Triclosan: Environmental Exposure, Toxicity and Mechanisms of Action. J Appl Toxicol (2011) 31:285–311. doi: 10.1002/jat.1660
8. Kolpin DW, Furlong ET, Meyer MT, Thurman EM, Zaugg SD, Barber LB, et al. Pharmaceuticals, Hormones, and Other Organic Wastewater Contaminants in U.S. Streams, 1999-2000: A National Reconnaissance. Environ Sci Technol (2002) 36:1202–11. doi: 10.1021/es011055j
9. Li X, Ying GG, Su HC, Yang XB, Wang L. Simultaneous Determination and Assessment of 4-Nonylphenol, Bisphenol A and Triclosan in Tap Water, Bottled Water and Baby Bottles. Environ Int (2010) 36:557–62. doi: 10.1016/j.envint.2010.04.009
10. Kumar KS, Priya SM, Peck AM, Sajwan KS. Mass Loadings of Triclosan and Triclocarbon From Four Wastewater Treatment Plants to Three Rivers and Landfill in Savannah, Georgia, USA. Arch Environ Contam Toxicol (2010) 58:275–85. doi: 10.1007/s00244-009-9383-y
11. Peng X, Yu Y, Tang C, Tan J, Huang Q, Wang Z. Occurrence of Steroid Estrogens, Endocrine-Disrupting Phenols, and Acid Pharmaceutical Residues in Urban Riverine Water of the Pearl River Delta, South China. Sci Total Environ (2008) 397:158–66. doi: 10.1016/j.scitotenv.2008.02.059
12. Coogan MA, Edziyie RE, La Point TW, Venables BJ. Algal Bioaccumulation of Triclocarban, Triclosan, and Methyl-Triclosan in a North Texas Wastewater Treatment Plant Receiving Stream. Chemosphere (2007) 67:1911–8. doi: 10.1016/j.chemosphere.2006.12.027
13. Arnot JA, Pawlowski S, Champ S. A Weight-of-Evidence Approach for the Bioaccumulation Assessment of Triclosan in Aquatic Species. Sci Total Environ (2018) 618:1506–18. doi: 10.1016/j.scitotenv.2017.09.322
14. Lyndall J, Fuchsman P, Bock M, Barber T, Lauren D, Leigh K, et al. Probabilistic Risk Evaluation for Triclosan in Surface Water, Sediments, and Aquatic Biota Tissues. Integr Environ Assess Manag (2010) 6:419–40. doi: 10.1897/IEAM_2009-072.1
15. Hovander L, Malmberg T, Athanasiadou M, Athanassiadis I, Rahm S, Bergman A, et al. Identification of Hydroxylated PCB Metabolites and Other Phenolic Halogenated Pollutants in Human Blood Plasma. Arch Environ Contam Toxicol (2002) 42:105–17. doi: 10.1007/s002440010298
16. Adolfsson-Erici M, Pettersson M, Parkkonen J, Sturve J. Triclosan, a Commonly Used Bactericide Found in Human Milk and in the Aquatic Environment in Sweden. Chemosphere (2002) 46:1485–9. doi: 10.1016/s0045-6535(01)00255-7
17. Wang X, Ouyang F, Feng L, Wang X, Liu Z, Zhang J. Maternal Urinary Triclosan Concentration in Relation to Maternal and Neonatal Thyroid Hormone Levels: A Prospective Study. Environ Health Perspect (2017) 125:067017. doi: 10.1289/EHP500
18. Liu H, Li J, Xia W, Zhang B, Peng Y, Li Y, et al. Blood Pressure Changes During Pregnancy in Relation to Urinary Paraben, Triclosan and Benzophenone Concentrations: A Repeated Measures Study. Environ Int (2019) 122:185–92. doi: 10.1016/j.envint.2018.11.003
19. Philippat C, Mortamais M, Chevrier C, Petit C, Calafat AM, Ye X, et al. Exposure to Phthalates and Phenols During Pregnancy and Offspring Size at Birth. Environ Health Perspect (2012) 120:464–70. doi: 10.1289/ehp.1103634
20. Arbuckle TE, Marro L, Davis K, Fisher M, Ayotte P, Belanger P, et al. Exposure to Free and Conjugated Forms of Bisphenol A and Triclosan Among Pregnant Women in the MIREC Cohort. Environ Health Perspect (2015) 123:277–84. doi: 10.1289/ehp.1408187
21. Watkins DJ, Ferguson KK, Anzalota Del Toro LV, Alshawabkeh AN, Cordero JF, Meeker JD. Associations Between Urinary Phenol and Paraben Concentrations and Markers of Oxidative Stress and Inflammation Among Pregnant Women in Puerto Rico. Int J Hyg Environ Health (2015) 218:212–9. doi: 10.1016/j.ijheh.2014.11.001
22. Lassen TH, Frederiksen H, Kyhl HB, Swan SH, Main KM, Andersson AM, et al. Prenatal Triclosan Exposure and Anthropometric Measures Including Anogenital Distance in Danish Infants. Environ Health Perspect (2016) 124:1261–8. doi: 10.1289/ehp.1409637
23. Haggard DE, Noyes PD, Waters KM, Tanguay RL. Phenotypically Anchored Transcriptome Profiling of Developmental Exposure to the Antimicrobial Agent, Triclosan, Reveals Hepatotoxicity in Embryonic Zebrafish. Toxicol Appl Pharmacol (2016) 308:32–45. doi: 10.1016/j.taap.2016.08.013
24. Paul KB, Hedge JM, DeVito MJ, Crofton KM. Short-Term Exposure to Triclosan Decreases Thyroxine In Vivo via Upregulation of Hepatic Catabolism in Young Long-Evans Rats. Toxicol Sci (2010) 113:367–79. doi: 10.1093/toxsci/kfp271
25. Crofton KM, Paul KB, Devito MJ, Hedge JM. Short-Term In Vivo Exposure to the Water Contaminant Triclosan: Evidence for Disruption of Thyroxine. Environ Toxicol Pharmacol (2007) 24:194–7. doi: 10.1016/j.etap.2007.04.008
26. Axelstad M, Boberg J, Vinggaard AM, Christiansen S, Hass U. Triclosan Exposure Reduces Thyroxine Levels in Pregnant and Lactating Rat Dams and in Directly Exposed Offspring. Food Chem Toxicol (2013) 59:534–40. doi: 10.1016/j.fct.2013.06.050
27. Rodriguez PE, Sanchez MS. Maternal Exposure to Triclosan Impairs Thyroid Homeostasis and Female Pubertal Development in Wistar Rat Offspring. J Toxicol Environ Health A (2010) 73:1678–88. doi: 10.1080/15287394.2010.516241
28. Schnitzler JG, Frederich B, Dussenne M, Klaren PH, Silvestre F, Das K. Triclosan Exposure Results in Alterations of Thyroid Hormone Status and Retarded Early Development and Metamorphosis in Cyprinodon Variegatus. Aquat Toxicol (2016) 181:1–10. doi: 10.1016/j.aquatox.2016.10.019
29. Pinto PIS, Guerreiro EM and Power DM. Triclosan Interferes With the Thyroid Axis in the Zebrafish (Danio Rerio). Toxicol Res (2013) 2:60–9. doi: 10.1039/c2tx20005h
30. Watermann A, Gieringer R, Bauer AM, Kurch S, Kiesslich R, Tremel W, et al. Fluorescein- and Egfr-Antibody Conjugated Silica Nanoparticles for Enhancement of Real-Time Tumor Border Definition Using Confocal Laser Endomicroscopy in Squamous Cell Carcinoma of the Head and Neck. Nanomater (Basel) (2019) 9:1378. doi: 10.3390/nano9101378
31. Wendl T, Lun K, Mione M, Favor J, Brand M, Wilson SW, et al. Pax2.1 is Required for the Development of Thyroid Follicles in Zebrafish. Development (2002) 129:3751–60. doi: 10.1242/dev.129.15.3751
32. Avdesh A, Chen M, Martin-Iverson MT, Mondal A, Ong D, Rainey-Smith S, et al. Regular Care and Maintenance of a Zebrafish (Danio Rerio) Laboratory: An Introduction. J Vis Exp (2012) 69:e4196. doi: 10.3791/4196
33. Oliveira R, Domingues I, Koppe Grisolia C, Soares AM. Effects of Triclosan on Zebrafish Early-Life Stages and Adults. Environ Sci Pollut Res Int (2009) 16:679–88. doi: 10.1007/s11356-009-0119-3
34. Goldsmith P. Zebrafish as a Pharmacological Tool: The How, Why and When. Curr Opin Pharmacol (2004) 4:504–12. doi: 10.1016/j.coph.2004.04.005
35. Lantz-McPeak S, Guo X, Cuevas E, Dumas M, Newport GD, Ali SF, et al. Developmental Toxicity Assay Using High Content Screening of Zebrafish Embryos. J Appl Toxicol (2015) 35:261–72. doi: 10.1002/jat.3029
36. Altenhofen S, Nabinger DD, Bitencourt PER, Bonan CD. Dichlorvos. alters morphology and behavior in zebrafish (Danio rerio) larvae. Environ Pollut (2019) 245:1117–23. doi: 10.1016/j.envpol.2018.11.095
37. Leary S, Underwood W, Anthony R, Cartner S, Corey D, Grandin T, et al. American Veterinary Medical Association for the Euthanasia of Animals. In: AVMA Guidelines 2013 Edition. Schaumburg, Illinois, USA: American Veterinary Medical Association.
38. Strykowski JL, Schech JM. Effectiveness of Recommended Euthanasia Methods in Larval Zebrafish (Danio Rerio). J Am Assoc Lab Anim Sci (2015) 54:81–4.
39. Tang T, Yang Y, Chen Y, Tang W, Wang F, Diao X. Thyroid Disruption in Zebrafish Larvae by Short-Term Exposure to Bisphenol AF. Int J Environ Res Public Health (2015) 12:13069–84. doi: 10.3390/ijerph121013069
40. Campion EM, Loughran ST, Walls D. Protein Quantitation and Analysis of Purity. Methods Mol Biol (2017) 1485:225–55. doi: 10.1007/978-1-4939-6412-3_12
41. Patino R, Wainscott MR, Cruz-Li EI, Balakrishnan S, McMurry C, Blazer VS, et al. Effects of Ammonium Perchlorate on the Reproductive Performance and Thyroid Follicle Histology of Zebrafish. Environ Toxicol Chem (2003) 22:1115–21. doi: 10.1002/etc.5620220520
42. Liu FJ, Wang JS, Theodorakis CW. Thyrotoxicity of Sodium Arsenate, Sodium Perchlorate, and Their Mixture in Zebrafish. Danio Rerio Environ Sci Technol (2006) 40:3429–36. doi: 10.1021/es052538g
43. Howe K, Clark MD, Torroja CF, Torrance J, Berthelot C, Muffato M, et al. The Zebrafish Reference Genome Sequence and its Relationship to the Human Genome. Nature (2013) 496:498–503. doi: 10.1038/nature12111
44. Chang J, Wang M, Gui W, Zhao Y, Yu L, Zhu G. Changes in Thyroid Hormone Levels During Zebrafish Development. Zoolog Sci (2012) 29:181–4. doi: 10.2108/zsj.29.181
45. Liu Y, Wang J, Fang X, Zhang H, Dai J. The Thyroid-Disrupting Effects of Long-Term Perfluorononanoate Exposure on Zebrafish (Danio Rerio). Ecotoxicology (2011) 20:47–55. doi: 10.1007/s10646-010-0555-3
46. Schmidt F, Schnurr S, Wolf R, Braunbeck T. Effects of the Anti-Thyroidal Compound Potassium-Perchlorate on the Thyroid System of the Zebrafish. Aquat Toxicol (2012) 109:47–58. doi: 10.1016/j.aquatox.2011.11.004
47. Liu F, Gentles A, Theodorakis CW. Arsenate and Perchlorate Toxicity, Growth Effects, and Thyroid Histopathology in Hypothyroid Zebrafish. Danio Rerio Chemosphere (2008) 71:1369–76. doi: 10.1016/j.chemosphere.2007.11.036
48. Jianjie C, Wenjuan X, Jinling C, Jie S, Ruhui J, Meiyan L. Fluoride Caused Thyroid Endocrine Disruption in Male Zebrafish (Danio Rerio). Aquat Toxicol (2016) 171:48–58. doi: 10.1016/j.aquatox.2015.12.010
49. Liu Z, Tang R, Li D, Hu Q, Wang Y. Subacute Microcystin-LR Exposure Alters the Metabolism of Thyroid Hormones in Juvenile Zebrafish (Danio Rerio). Toxins (Basel) (2015) 7:337–52. doi: 10.3390/toxins7020337
50. Stenzel A, Wirt H, Patten A, Theodore B, King-Heiden T. Larval Exposure to Environmentally Relevant Concentrations of Triclosan Impairs Metamorphosis and Reproductive Fitness in Zebrafish. Reprod Toxicol (2019) 87:79–86. doi: 10.1016/j.reprotox.2019.05.055
51. Fujita H. Functional Morphology of the Thyroid. Int Rev Cytol (1988) 113:145–85. doi: 10.1016/s0074-7696(08)60848-7
52. Yang X, Xie J, Wu T, Yue G, Chen J, Zhao R. Hepatic and Muscle Expression of Thyroid Hormone Receptors in Association With Body and Muscle Growth in Large Yellow Croaker, Pseudosciaena Crocea (Richardson). Gen Comp Endocrinol (2007) 151:163–71. doi: 10.1016/j.ygcen.2007.01.005
53. Yamano K. The Role of Thyroid Hormone in Fish Development With Reference to Aquaculture. JARQ (2005) 39:161–68. doi: 10.6090/jarq.39.161
54. Ma X, Wan Y, Wu M, Xu Y, Xu Q, He Z, et al. Occurrence of Benzophenones, Parabens and Triclosan in the Yangtze River of China, and the Implications for Human Exposure. Chemosphere (2018) 213:517–25. doi: 10.1016/j.chemosphere.2018.09.084
55. Nag SK, Das Sarkar S, Manna SK. Triclosan - an Antibacterial Compound in Water, Sediment and Fish of River Gomti, India. Int J Environ Health Res (2018) 28:461–70. doi: 10.1080/09603123.2018.1487044
56. James MO, Li W, Summerlot DP, Rowland-Faux L, Wood CE. Triclosan is a Potent Inhibitor of Estradiol and Estrone Sulfonation in Sheep Placenta. Environ Int (2010) 36:942–9. doi: 10.1016/j.envint.2009.02.004
57. Zhang P, Yang M, Zeng L, Liu C. P38/TRHr-Dependent Regulation of TPO in Thyroid Cells Contributes to the Hypothyroidism of Triclosan-Treated Rats. Cell Physiol Biochem (2019) 45:1303–15. doi: 10.1159/000487558
58. Hirota R, Ohya Y, Yamamoto-Hanada K, Fukutomi IY, Muto G, Ngatu-Nlandu R, et al. Triclosan-Induced Alteration of Gut Microbiome and Aggravation of Asthmatic Airway Response in Aeroallergen-Sensitized Mice. Allergy (2018) 74:996–9. doi: 10.1111/all.13639
59. Veldhoen N, Skirrow RC, Osachoff H, Wigmore H, Clapson DJ, Gunderson MP, et al. The Bactericidal Agent Triclosan Modulates Thyroid Hormone-Associated Gene Expression and Disrupts Postembryonic Anuran Development. Aquat Toxicol (2006) 80:217–27. doi: 10.1016/j.aquatox.2006.08.010
60. Fort DJ, Rogers RL, Gorsuch JW, Navarro LT, Peter R, Plautz JR. Triclosan and Anuran Metamorphosis: No Effect on Thyroid-Mediated Metamorphosis in Xenopus Laevis. Toxicol Sci (2010) 113:392–400. doi: 10.1093/toxsci/kfp280
61. Zorrilla LM, Gibson EK, Jeffay SC, Crofton KM, Setzer WR, Cooper RL, et al. The Effects of Triclosan on Puberty and Thyroid Hormones in Male Wistar Rats. Toxicol Sci (2009) 107:56–64. doi: 10.1093/toxsci/kfn225
62. Stoker TE, Gibson EK, Zorrilla LM. Triclosan Exposure Modulates Estrogen-Dependent Responses in the Female Wistar Rat. Toxicol Sci (2010) 117:45–53. doi: 10.1093/toxsci/kfq180
63. Paul KB, Hedge JM, Bansal R, Zoeller RT, Peter R, DeVito MJ, et al. Developmental Triclosan Exposure Decreases Maternal, Fetal, and Early Neonatal Thyroxine: A Dynamic and Kinetic Evaluation of a Putative Mode-of-Action. Toxicology (2012) 300:31–45. doi: 10.1016/j.tox.2012.05.023
64. Paul KB, Hedge JM, Devito MJ, Crofton KM. Developmental Triclosan Exposure Decreases Maternal and Neonatal Thyroxine in Rats. Environ Toxicol Chem (2010) 29:2840–4. doi: 10.1002/etc.339
65. Hinther A, Bromba CM, Wulff JE, Helbing CC. Effects of Triclocarban, Triclosan, and Methyl Triclosan on Thyroid Hormone Action and Stress in Frog and Mammalian Culture Systems. Environ Sci Technol (2011) 45:5395–402. doi: 10.1021/es1041942
66. Wu Y, Beland FA, Fang JL. Effect of Triclosan, Triclocarban, 2,2’,4,4’-Tetrabromodiphenyl Ether, and Bisphenol A on the Iodide Uptake, Thyroid Peroxidase Activity, and Expression of Genes Involved in Thyroid Hormone Synthesis. Toxicol In Vitro (2016) 32:310–9. doi: 10.1016/j.tiv.2016.01.014
67. Zhou Z, Yang J, Chan KM. Toxic Effects of Triclosan on a Zebrafish (Danio Rerio) Liver Cell Line, ZFL. Aquat Toxicol (2017) 191:175–88. doi: 10.1016/j.aquatox.2017.08.009
68. Schuur AG, Legger FF, van Meeteren ME, Moonen MJ, van Leeuwen-Bol I, Bergman A, et al. In Vitro Inhibition of Thyroid Hormone Sulfation by Hydroxylated Metabolites of Halogenated Aromatic Hydrocarbons. Chem Res Toxicol (1998) 11:1075–81. doi: 10.1021/tx9800046
69. Wang LQ, Falany CN, James MO. Triclosan as a Substrate and Inhibitor of 3’-Phosphoadenosine 5’-Phosphosulfate-Sulfotransferase and UDP-Glucuronosyl Transferase in Human Liver Fractions. Drug Metab Dispos (2004) 32:1162–9. doi: 10.1124/dmd.104.000273
70. Nicholson JL, Altman J. The Effects of Early Hypo- and Hyperthyroidism on the Development of Rat Cerebellar Cortex. I. Cell Proliferation and Differentiation. Brain Res (1972) 44:13–23. doi: 10.1016/0006-8993(72)90362-9
71. Alt B, Reibe S, Feitosa NM, Elsalini OA, Wendl T, Rohr KB. Analysis of Origin and Growth of the Thyroid Gland in Zebrafish. Dev Dyn (2006) 235:1872–83. doi: 10.1002/dvdy.20831
72. Opitz R, Maquet E, Zoenen M, Dadhich R, Costagliola S. TSH Receptor Function is Required for Normal Thyroid Differentiation in Zebrafish. Mol Endocrinol (2011) 25:1579–99. doi: 10.1210/me.2011-0046
Keywords: triclosan, early exposure, thyroid hormone, thyroid follicles, zebrafish
Citation: Tang N, Fan P, Chen L, Yu X, Wang W, Wang W and Ouyang F (2022) The Effect of Early Life Exposure to Triclosan on Thyroid Follicles and Hormone Levels in Zebrafish. Front. Endocrinol. 13:850231. doi: 10.3389/fendo.2022.850231
Received: 07 January 2022; Accepted: 02 May 2022;
Published: 03 June 2022.
Edited by:
Maaike Van Gerwen, Icahn School of Medicine at Mount Sinai, United StatesReviewed by:
Monia Perugini, University of Teramo, ItalyCaroline Serrano-Nascimento, Federal University of São Paulo, Brazil
Copyright © 2022 Tang, Fan, Chen, Yu, Wang, Wang and Ouyang. This is an open-access article distributed under the terms of the Creative Commons Attribution License (CC BY). The use, distribution or reproduction in other forums is permitted, provided the original author(s) and the copyright owner(s) are credited and that the original publication in this journal is cited, in accordance with accepted academic practice. No use, distribution or reproduction is permitted which does not comply with these terms.
*Correspondence: Fengxiu Ouyang, b3V5YW5nZmVuZ3hpdUB4aW5odWFtZWQuY29tLmNu; b3V5YW5nZmVuZ3hpdUAxMjYuY29t