- 1Key Laboratory of Mariculture, Ministry of Education, Ocean University of China, Qingdao, China
- 2College of Ocean, Key Laboratory of Tropical Biological Resources of Ministry of Education, Hainan University, Haikou, China
Neurokinin B (NKB), a member of the tachykinin (TAC) family, plays important roles in mammalian neuropeptide secretion in related to reproduction. However, its potential role in spawning migration teleost is less clear. In the present study, Japanese eel (Anguilla japonica) was employed to study the performance of NKB in regulating reproduction. Results showed that two tac3 and one tacr3 genes were identified in Japanese eel. Sequence analysis showed that two tac3 transcripts, tac3a and tac3b, encode four NKBs: NKBa-13, NKBa-10, NKBb-13, and NKBb-10. However, compared with other species, a mutation caused early termination of TACR3 protein was confirmed, leading to the loss of the 35 amino acid (aa) C-terminal of the receptor. Expression analysis in different tissues showed that both tac3a and tac3b mRNAs were highly expressed in the brain. In situ hybridization localized both tac3a and tac3b mRNAs to several brain regions, mainly in the telencephalon and hypothalamus. Because of the mutation in TACR3 of Japanese eel, we further analyzed whether it could activate the downstream signaling pathway. Luciferase assay results showed the negative regulation of cAMP Response Element (CRE) and Sterol Response Element (SRE) signal pathways by Japanese eel NKBs. Intraperitoneal injection of four different NKB mature peptides at 100 ng/g had negative effect on either gnrh or gth gene expression. However, the high concentration of NKBa-10 and NKBb-13 (1,000 ng/g) upregulated mgnrh and fshb or lhb expression level significantly, which may be mediated by other receptors. In general, the NKBs/NK3Rs system has important functions in regulating eel puberty onset.
Introduction
Puberty refers to the developmental transition to a mature, reproductive state in mammals (1). In rodents, the first external sign of pubertal development is vaginal opening, although hormonal changes precede this (2). In fish, puberty is the developmental period during which an individual becomes capable of reproducing sexually for the first time and implies a functional competence of the brain–pituitary–gonad axis (3–5). It starts sometimes after sex differentiation and is associated with the initiation of germ cell maturation and full functional differentiation of the germ cell–supporting somatic cells of the gonads and culminates in the first spermiation and sperm hydration or ovulation (6).
Japanese eel, Anguilla japonica, is one of the most important species of the aquaculture industry of East Asia. However, because of the catadromous migration reproductive character, the aquaculture industry is limited by the natural resources of glass eels from the ocean. Artificial reproduction studies of elopomorphes started in early 1930s, while until now, although great process has been made (7), the maturation rate of parental eels, survival rate of fertilized eggs, growth speed of hatched larva are still far beyond industrial application, especially the extremely high cost in raring hatched larva, limited this industry to the new horizon. The elopomorphes have a complex migratory life cycle with the occurrence of two metamorphoses (8). The first one is when leptocephali larvae metamorphose into glass eels at the time of their arrival over the continental shelf, at the end of a long oceanic journey, after which, glass eels become “yellow” eels; the second metamorphosis is termed “silvering (transition from yellow to silver eel)”, after years of growing in freshwater, the yellow eels stop growth and start the downstream migration toward the area for breeding in the ocean (9). The silvering of eels was suggested to be considered as a pubertal development step, according to hormonal profiles and experimental results on the gonadotropic axis (10). It is believed that, at the silver stage, eels are blocked at a prepubertal stage (11), but chronic administration of carp or salmon pituitary extract (gonadotropic treatment) is able to induce sexual maturation (12, 13).
The neurokinin B (NKB), coded by the tac3 gene (tac2 gene in rodents), is an important member of tachykinin family. NKB, kisspeptin, and dynorphin are co-expressed in the arcuate nucleus and play an important role in the regulation of GnRH secretion (14–17). The NKB signal was important in reproduction especially puberty of human. Mutations in the genes encoding NKB (tac3) or its receptor NK3R (tacr3) lead to hypogonadotropic hypogonadism—a disease characterized by the failure of sexual maturation, impaired gametogenesis, and infertility, indicating that the NKB/NK3R system is indispensable for human reproduction (18, 19). In monkeys (Macaca mulatta), transient activation of NK3R stimulates GnRH release, but repeated stimulation of this pathway does not induce GnRH pulsatile release (20). In addition, injecting antagonists of NK3R could inhibit LH secretion levels in ovariectomized rats (Rattus norvegicus) (21). Taken together, in addition to its role in the downstream part of the pubertal mechanism (GnRH pulse generator), NKB signal may also be involved in the upstream part of the central pubertal system.
In teleost, NKB/NK3R signaling pathway has been characterized in zebrafish (Danio rerio) (22), goldfish (Carassius auratus) (23), tilapia (Oreochromis mossambicus) (24), spotted sea bass (Lateolabrax maculatus) (25) and carp (Ctenopharyngodon idella) (26). Previously, using goldfish as a modal, we have confirmed the NKB function in regulating GnRH and GtH expression both in vivo and in vitro (23). Furthermore, we have also demonstrated that NKB could act directly on the ovary to enhance the 17β-estradiol (E2) synthesis and release and, therefore, the growth of oocytes (27). In the present study, we used Japanese eel, whose ovary development is blocked at the prepubertal stage, as a model to further investigate the role of NKBs in fish puberty onset. cDNA for tac3a, tac3b, and tacr3s were characterized and cloned, and the distribution of tac3s in different tissues was determined. Because tac3s mRNA was highly expressed in the brain, we further detected the location of both genes in brain regions of the eel. Interestingly, mutation caused early termination of NK3R protein translation was found in all tested samples. To confirm whether the mutated NK3R could active the downstream signaling, using luciferase assay, we confirmed that NKB of Japanese eel could not activate neither CRE nor SRE signaling pathway. Finally, to evaluate whether NKB can still regulate the reproductive functions by receptors of other genes, we measured two Japanese eel GnRHs that were homologous to mammalian or chicken GnRH (namely, mgnrh and cgnrh, respectively), fshb and lhb gene expression by real-time PCR in eels injected with NKBs. These novel findings demonstrated that the NKBs/NK3Rs system has important functions in regulating eel puberty onset.
Materials and methods
Animals
Female Japanese eels with body weight (BW) of 1.0 ± 0.2kg were obtained from Guangzhou, Zhuhai (Guangdong), and Qingdao (Shandong), China, and allowed to acclimatize for 7 days in tanks with a photoperiod of 14L:10D and temperature of 25°C. Zebrafish were obtained from an ornamental fish market in Qingdao, China. The animals were fed with commercial diet without any supplemental hormones. All animal experiments were conducted in accordance with the guidelines and approval of the Animal Research and Ethics Committees of Yat-Sen University and the Ocean University of China.
Cloning and Sequence Analysis of tac3s/tacr3s Genes
Total RNA from more than 80 Japanese eel brains and ovaries and three zebrafish brains were prepared by using TRIzol (Invitrogen, USA). One microgram of isolated RNA was used to synthesize cDNA using the ReverTra Ace-a First-Strand cDNA Synthesis Kit (TOYOBO, Japan). To amplify the cDNA fragments of the eel tac3a, tac3b, and tacr3, PCR primers were designed on the basis of the nucleotide sequences of searched from the genome data. Full-length cDNA sequences encoding these genes were obtained by the 5′ and 3′ rapid amplification of cDNA ends (RACE) with several gene-specific primers (Table 1). The tacr3a1 sequence of zebrafish was obtained from National Center for Biotechnology Information (NCBI) and cloned. The primers used were listed in Table 1. For all PCR reactions, amplifications were performed as follows: denaturation at 94°C for 5 min, followed by 40 cycles at 94°C for 20 s, 55°C–60°C for 20 s, and 72°C for 2 min. The reaction ended with a final extension at 72°C for 5 min. The amplification products were purified using the E.Z.N.A. Gel Extraction Kit (Omega BioTek, USA) and subcloned into the pGEM-T vector (Promega, USA). Three different positive clones were sequenced on an ABI 3700 sequencer (Applied Biosystems, USA). The putative amino acid (aa) sequences were predicted using the BioEdit software, and the putative seven-transmembrane domains were predicted using the TMHMM Server v. 2.0 (http://www.cbs.dtu.dk/services/TMHMM-2.0/). The NKBs aa sequences were aligned with Clustal W 1.81 (28). The protein phylogenetic analysis was conducted with MEGA 6.0 using the neighbor-joining method.
RNA Extraction, Reverse Transcription, and qPCR
Total RNA was extracted using TRIzol reagent (Life Technologies China, Inc.). The amount and purity of the RNA were determined on a NanoDrop 2000 spectrophotometer (ThermoFisher Scientific). One microgram of isolated RNA was used to synthesize first-strand cDNAs using the ReverTra Ace-a First-strand cDNA Synthesis Kit (TOYOBO, Japan). Real-time PCR was performed on a Roche LightCycler 480 using the SYBR Green I Kit (TOYOBO, Japan) according to the manufacturer’s instructions. Elongation factor-1α, β-actin, and 18s were used as internal controls. Relative mRNA levels were determined using the standard ΔΔcycle threshold method.
In Situ Hybridization
The in situ hybridization was performed as we previously reported (23, 29). The brains of five female Japanese eels were removed and fixed in buffered 4% paraformaldehyde for 24 h and then embedded in paraffin. Seven-micron-thick sections were cut for ISH. The sections were pasted onto aminopropylsilane-treated glass slides and dried in an oven at 37°C. Sense and antisense digoxigenin (DIG)–labeled riboprobes about 300–400 bp in length were synthesized from the open reading frames (ORFs) of Japanese eels tac3s genes using a DIG RNA Labeling Kit (Roche Diagnostics, Mannheim, Germany). The sections were briefly rehydrated by a graded series of ethanol solutions (100% to 80%) after being cleared in xylene, permeabilized with 0.8 M HCl for 10 min followed by proteinase K (10 µg/ml) digestion for 2 min, washed in 1× Phosphate Buffered Saline (PBS) for 10 min, then washed in 2×Sodium Citrate Buffer (SSC) for 10 min, prehybridized at 55◦C for 1 h, and hybridized with DIG-labeled riboprobes diluted in hybridization buffer at 55°C overnight in a wet box (25). After hybridization, the sections were washed in grade series of Sodium Citrate Buffer (SSC) and Phosphate Buffered Saline (PBS) solution and blocked with blocking reagent (Roche Diagnostics). DIG was detected with an alkaline phosphatase-conjugated anti-DIG antibody (Roche Diagnostics; diluted 1:3,000), and chromogenic development was conducted with an Nitro-Blue-Tetrazolium/5-bromo-4-chloro-3-inodlyl-phosphate (NBT/BCIP) stock solution (Roche Diagnostics).
Peptide Synthesis and Preparation
On the basis of the result that we got of the eel TAC3A and TAC3B, peptides corresponding to the eel NKB peptides (SGTGLSATLPQRF-NH2) were synthesized by GL Biochem (Shanghai, China). The purity of the synthesized peptides was >95% as determined by analytical HPLC. Eel peptides were dissolved into DMSO and diluted to the desired concentration in saline (0.7% NaCl) for in vivo injection experiments.
In vivo Injection of TAC in Japanese Eel
A total of 45 healthy Japanese eels with similar BW were selected and divided into nine groups. Five eels in each group were kept in different aquariums, and the aquariums were numbered. The control group was injected with 0.2 ml of saline with 0.1% Dimethyl sulfoxide (DMSO), whereas the treatment groups were injected intraperitoneally with high concentration (1,000 ng/g BW) and low concentration (100 ng/g BW).
Cell Culture and Co-transfection
The tacr3 cDNAs of Japanese eel and zebrafish were subcloned into the pcDNA3.1 expression vector (Invitrogen, USA). The COS-7 cells and 293T cells were maintained at 37°C in Dulbecco's Modified Eagle Medium (DMEM) containing 10% fetal bovine serum (Gibco, USA). Twenty hours before transfection, 1 × 105 cells per well were seeded into 24-well tissue culture plates. A total of 500 ng of SRE-Luc or CRE-Luc reporter plasmid (30), 300 ng of pcDNA3.1-tacr3 of Japanese eel, and 50 ng of pRL-CMV containing the Renilla luciferase reporter gene (31) were co-transfected into the COS-7 cells in 500 ml of serum-free medium using Lipofectamine reagent (Invitrogen, USA). Six hours after transfection, cells were incubated with vehicle or various (from 10−10 to 10−6 M) concentrations of NKBa-10, NKBa-13, NKBb-10, and NKBb-13 for a further 24 h (stimulated with 1 × 10−5 M FSK (Sigma, USA) for CRE promoter). A total of 250 ng of SRE-Luc or CRE-Luc reporter plasmid, 200 ng of pcDNA3.1-tacr3a1 of zebrafish, and 50 ng of TK containing the Renilla luciferase reporter gene (31) were co-transfected into the 293T cells in 500 ml of serum-free medium using Lipofectamine 3000 reagent (Invitrogen, USA). Six hours after transfection, cells were incubated with vehicle or various (from 10−9 to 10−7 M) concentrations of NKBa-10 for a further 36 h. Cells were harvested, and luminescence was measured by the Dual Luciferase Kit (Promega, USA).
Statistical Analysis
All data were expressed as the mean ± S.E.M, and the number of samples is indicated in the figure legends. Statistical significance was determined using a one-way ANOVA followed by Dunnett’s test for multiple rage comparison. A Student’s t-test was used for comparison between two groups. Statistical significance was defined as P < 0.05.
Results
Identification and Synteny Analysis of the tac3 and tacr3 Genes in Japanese Eel
Two tac3 genes and one tacr3 gene from the Japanese eel were cloned, named tac3a (OL804257), tac3b (OL804258), and tacr3 (ON402757). The ORF of tac3a is 375 bp, coding a 125-aa precursor with a predicted signal peptide of 21 aa (Figure 1A). The ORF of tac3b is 297 bp, coding a 99-aa precursor with a predicted signal peptide of 21 aa (Figure 1B). In addition, the ORF of tac3r is 909 bp coding a 302-aa G protein–coupled receptor (GPCR) with seven transmembrane domains (Figure 1C). Sequence analysis showed that each precursor contains two putative peptides. The four Japanese eel tachykinin peptides were designated NKBa-13, NKBa-10, NKBb-13, and NKBb-10 according to their length. Sequence analysis showed that a common C-terminal sequence (FVGLM) was conversed in all NKB-10 and NKB-13 in teleost. Sequence alignment of TAC3 precursor in teleost showed that NKBa-10 and NKBa-13 were better conserved, whereas NKBb-10 and NKBb-13 had lower similarity in teleost (Figures 2A, B). Sequence comparison analysis revealed the TACR3 had a mutation of adenine at the 908th position, resulting an early termination of the GPCR translation (Figure 2C).
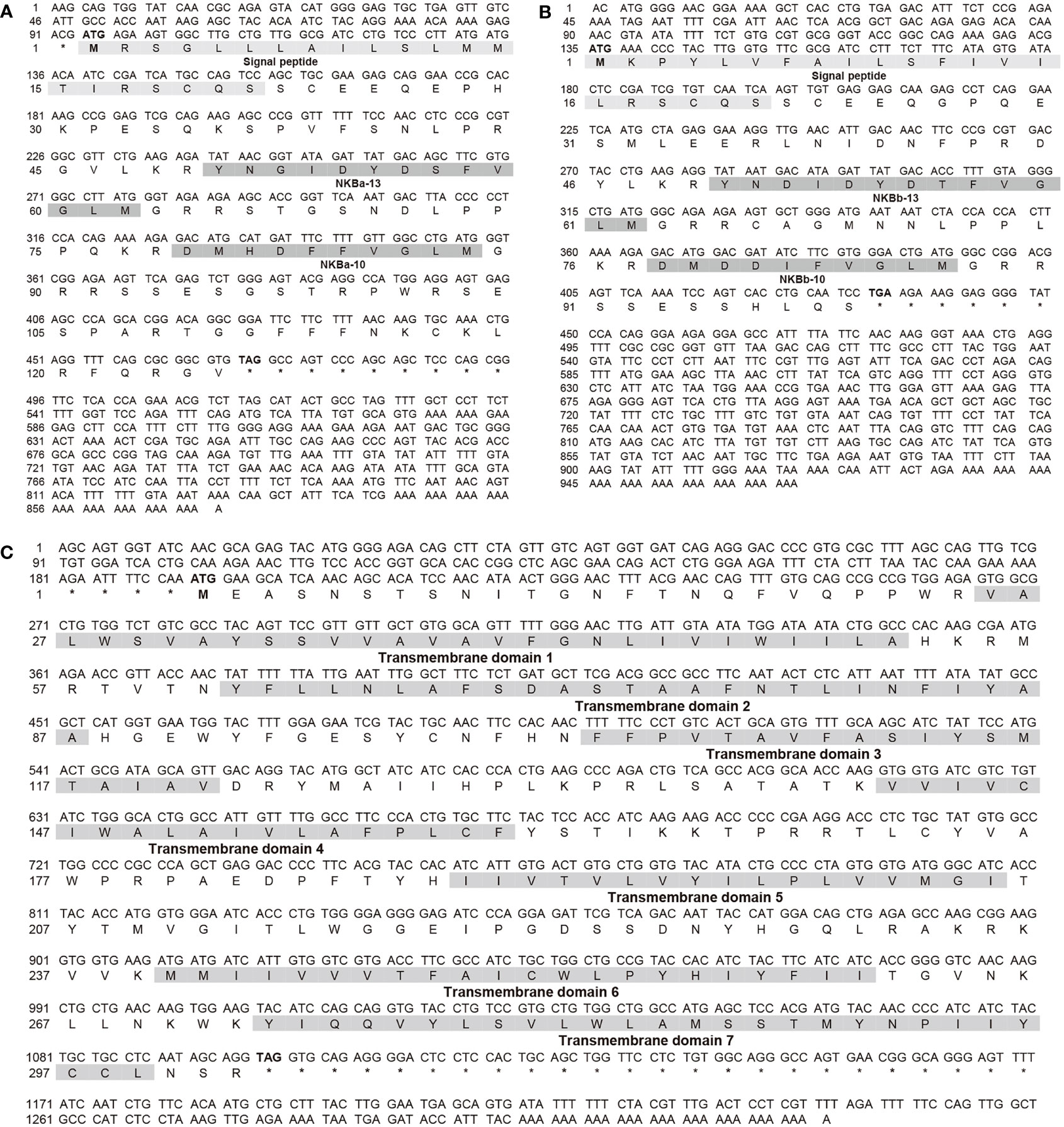
Figure 1 Nucleotide sequences and deduced amino acid sequences of tac3a (A) and tac3b (B) in Japanese eel. The signal peptides and NKB peptides were shaded, and their names under the corresponding sequence. Nucleotide sequences and deduced amino acid sequences of tacr3 (C) in Japanese eel. The transmembrane domains were numbered under the corresponding sequence, and their sequence compositions are shaded.
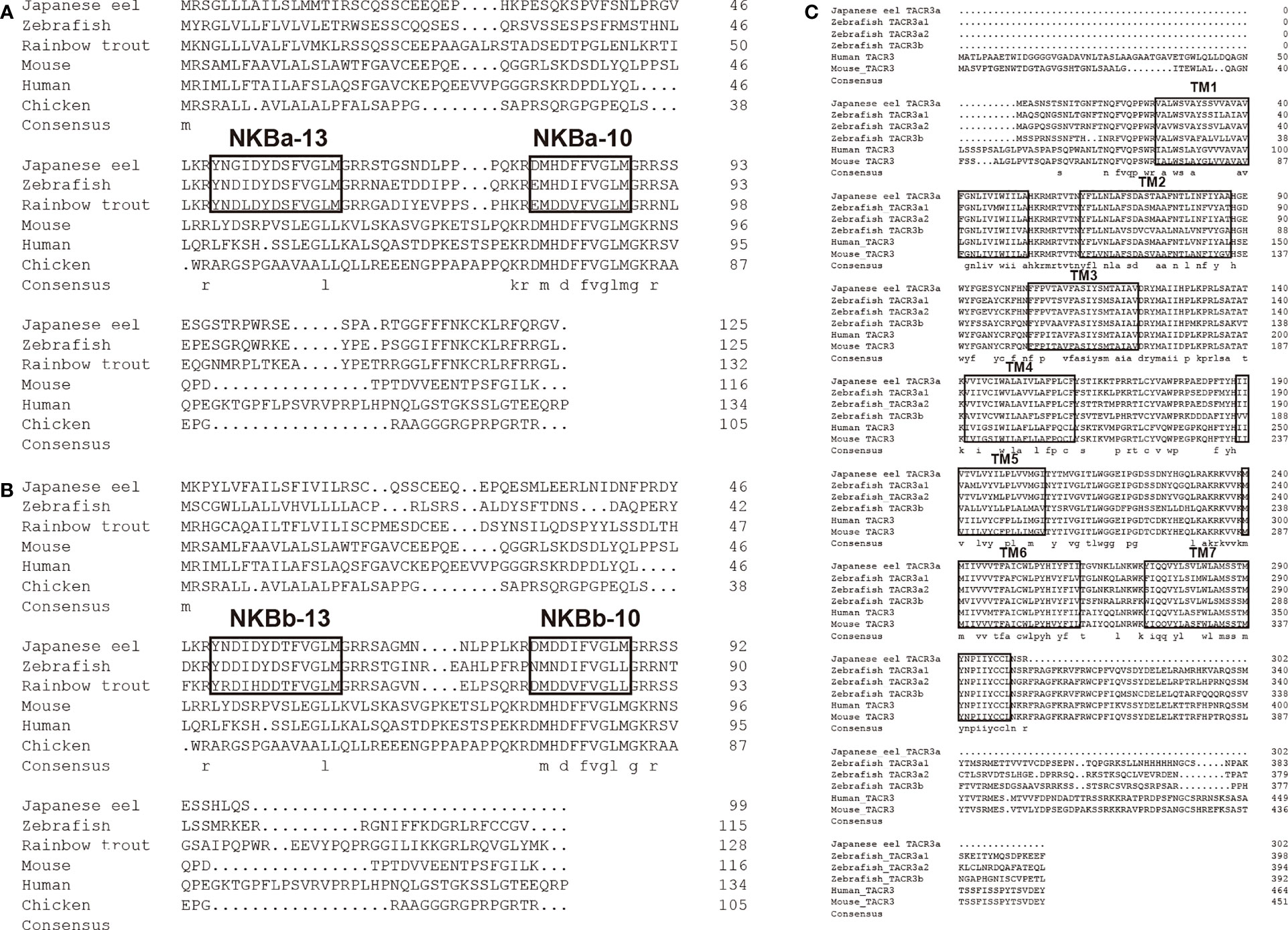
Figure 2 Comparison of the amino acid sequences of Japanese eel tac3a (A) and tac3b (B) precursors from different species. The boxed letters indicated the sequence of mature NKB peptides in the detected species. Comparison of the amino acid sequences of Japanese eel tacr3 (C) precursors from different species. The boxed letters indicated the sequence of transmembrane domains in the detected species.
Phylogenetic trees of the TAC3s and TACRs were constructed. As shown in Figure 3A, TAC3s from teleost and other vertebrates formed two large clades, and the teleost clade was subdivided into TAC3a and TAC3b clades. The two TAC3 of the Japanese eel are clustered in the TAC3b clade, which is clustered together with the TAC3b of Atlantic salmon (Salmo salar), rainbow trout (Oncorhynchus mykiss), and European sea bass (Dicentrarchus labrax) TAC3b. Phylogenetic tree of TACRs showed three TACR clades, which Japanese eel TACR3a was clustered into TACR3 clade, indicating the evolutionary conservation (Figure 3B).
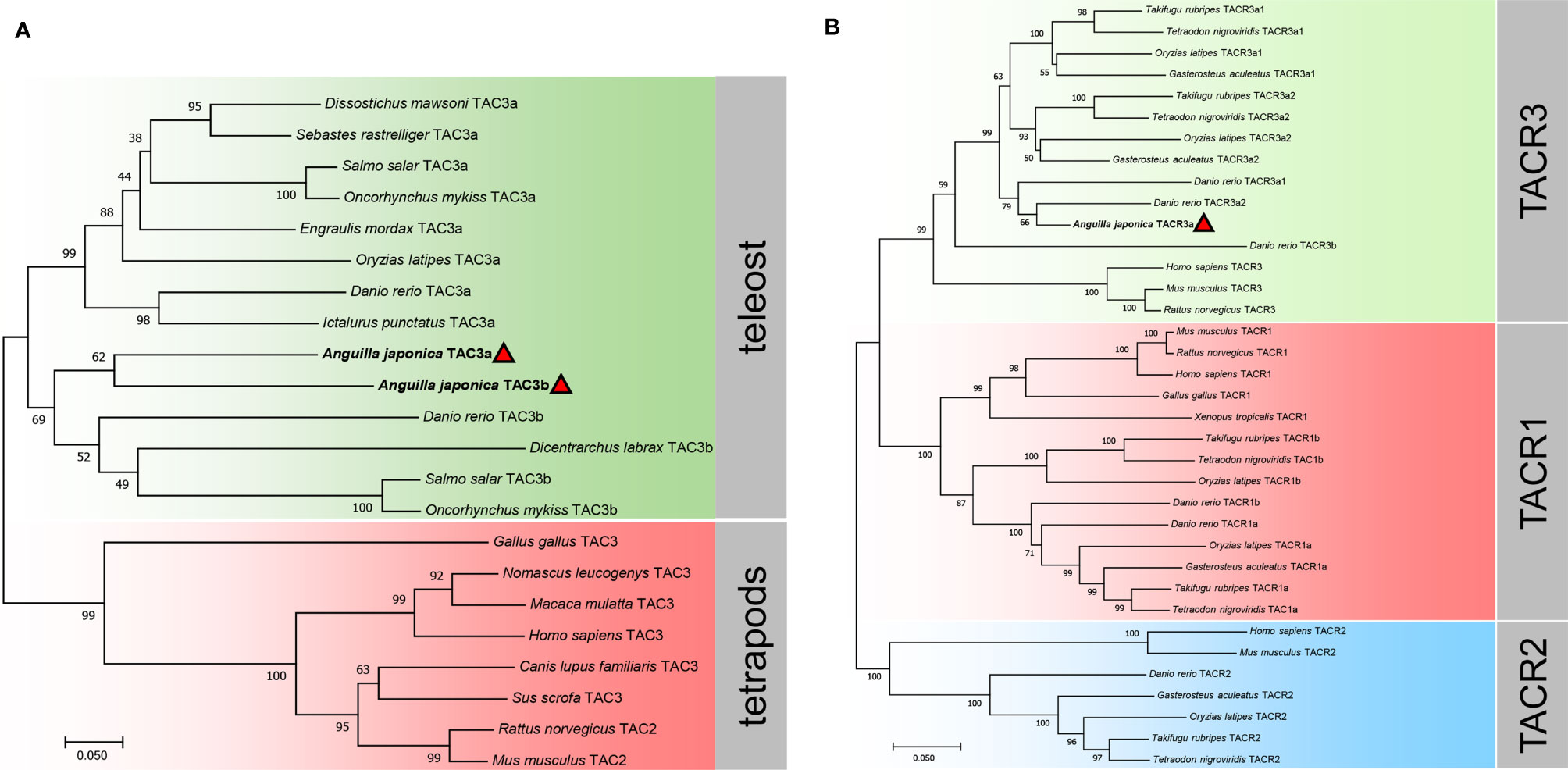
Figure 3 Phylogenetic analysis of the tac3 (A) and tacr (B) gene family in vertebrates. The phylogenetic trees were constructed by MEGA 7 using the neighbor-joining method. Data were resampled with 1,000 bootstrap replicates. The accession numbers of each sequence are Dissostichus mawsoni tac3a (DAA35156.1), Sebastes rastrelliger tac3a (XP_037630002.1), Salmo salar tac3a (XP_013991334.1), Oncorhynchus mykiss tac3a (XP_021421533.1), Engraulis mordax tac3a (DAA35163.1), Oryzias latipes tac3a (NP_001265832.1), Danio rerio tac3a (NP_001243318.1), Ictalurus punctatus tac3a (XP_017343311.1), Danio rerio tac3b (NP_001243319.1), Dicentrarchus labrax tac3b (QYB17058.1), Salmo salar tac3b (XP_014022643.1), Oncorhynchus mykiss tac3b (CDQ91399.1), Gallus gallus tac3 (XP_015155892.2), Nomascus leucogenys tac3 (XP_003252831.1), Macaca mulatta tac3 (XP_001115535.2), Homo sapiens tac3 (AAQ10783.1), Canis lupus familiaris tac3 (NP_001279988.1), Sus scrofa tac3 (NP_001007197.1), Rattus norvegicus tac2 (NP_062035.1), Mus musculus tac2 (BAA03316.1), Takifugu rubripes tacr3a1 (NP_001267026.1), Tetraodon nigroviridis tacr3a1 (DAA35148.1), Oryzias latipes tacr3a1 (NP_001265839.1), Gasterosteus aculeatus tacr3a1 (XP_040043470.1), Takifugu rubripes tacr3a2 (NP_001267041.1), Tetraodon nigroviridis tacr3a2 (DAA35149.1), Oryzias latipes tacr3a2 (NP_001265803.1), Gasterosteus aculeatus tacr3a2 (XP_040042202.1), Danio rerio tacr3a1 (NP_001243567.1), Danio rerio tacr3a2 (NP_001243564.1), Danio rerio tacr3b (XP_002666594.1), Homo sapiens tacr3 (NP_001050.1), Mus musculus tacr3 (AAH66845.1), Rattus norvegicus tacr3 (NP_058749.1), Mus musculus tacr1 (NP_033339.2), Rattus norvegicus tacr1 (NP_036799.1), Homo sapiens tacr1 (NP_001049.1), Gallus gallus tacr1 (NP_990199.1),Xenopus tropicalis tacr1 (NP_001106489.1), Takifugu rubripes tacr1b (XP_029693280.1), Tetraodon nigroviridis tacr1b (CAG05392.1), Oryzias latipes tacr1b (XP_011479687.1), Danio rerio tacr1b (NP_001268728.1), Danio rerio tacr1a (NP_001257407.1), Oryzias latipes tacr1a (NP_001265826.1), Gasterosteus aculeatus tacr1a (XP_040052094.1), Takifugu rubripes tacr1a (NP_001267036.1), Tetraodon nigroviridis tacr1a (CAG12579.1), Homo sapiens tacr2 (NP_001048.2), Mus musculus tacr2 (NP_033340.3), Danio rerio tacr2 (NP_001314788.1), Gasterosteus aculeatus tacr2 (XP_040059362.1), Oryzias latipes tacr2 (XP_011489426.1), Takifugu rubripes tacr2 (NP_001267009.1), and Tetraodon nigroviridis tacr2 (DAA35150.1).
Tissue Expression of Japanese Eel tac3s
The expression patterns of the tac3s genes in different tissues and brain regions were shown in Figure 4. Tac3a mRNA was mainly expressed in the pituitary, followed by the hypothalamus and telencephalon, with low level expressed in the optic tectum, medulla, liver, intestine, and gonad. However, tac3b mRNA was only expressed in telencephalon, optic tectum, medulla, and hypothalamus, and the expression was relatively high in the telencephalon.
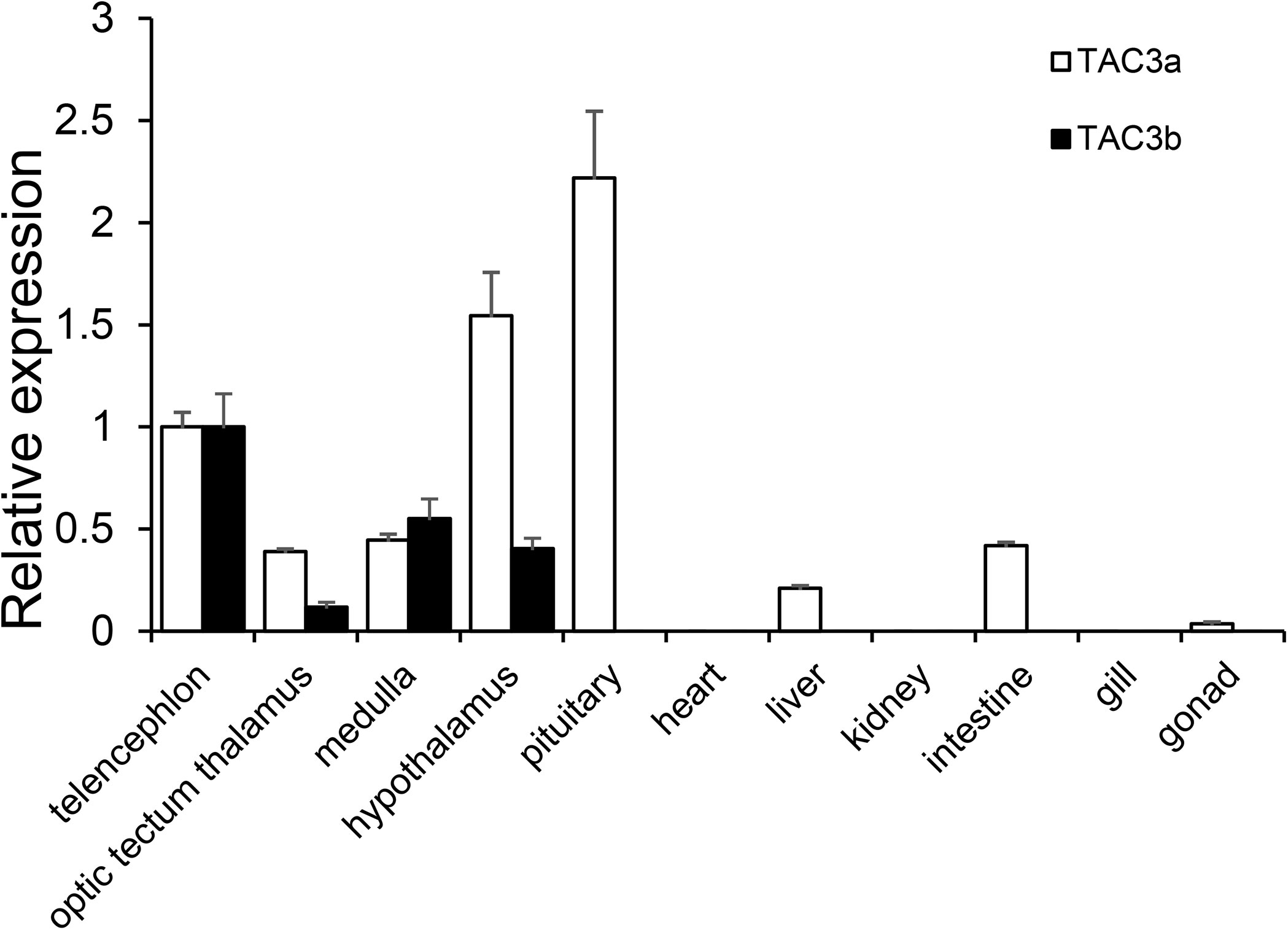
Figure 4 Expression of tac3s mRNAs in various tissues (telencephalon, optic tectum thalamus, medulla, hypothalamus, pituitary, heart, liver, kidney, intestine, gill, and gonad) of Japanese eel. The X-axis indicates different tissues. The results are normalized to 18s rRNA. The data are shown as the mean ± S.E.M (n = 3), and the mRNA levels of tac3a and tac3b were calculated as fold change relative to the mRNA levels of the heart. The different lowercased letters indicated significant differences (P < 0.05).
Localization of the mRNA of tac3s in the Brain
Sections of brain tissue of Japanese eel were taken to detect the mRNA distribution of tac3a and tac3b by in situ hybridization. Localization of both tac3a and tac3b in the brain is shown in Figures 5, 6. Tac3a positive signals were detected in the postcommissural nucleus of the ventral telencephalon (VP), the ventral hypothalamus (Hv), the nucleus of the posterior recess (NRP), the torus longitudinalis (TL), the reticular formation (RF), the molecular layer of corpus cerebelli (CCeM) (Figures 5A–D). The tac3b positive signals were detected in the lateral part of the dorsal telencephalon (Dl), the supracommissural nucleus of the ventral telencephalon (Vs), the Hv, the NRP, the TL, the RF, and the CCeM (Figures 6A–D).
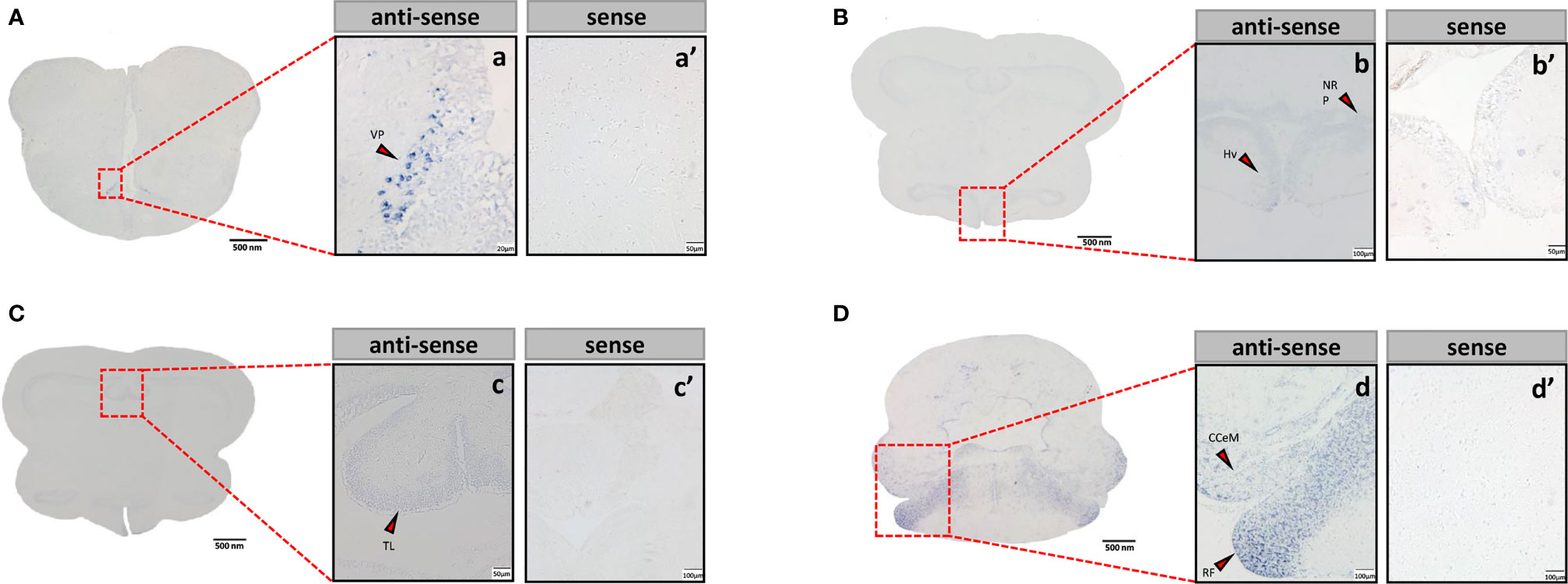
Figure 5 Tac3a mRNA in the brain of Japanese eel was detected by in situ hybridization (ISH). Tac3a localization in the telencephalon (A), thalamus (B), midbrain (C), and cerebellum (D). Scale bar = 500 μm. Positive signal of tac3a in the telencephalon (a), thalamus (b), midbrain (c), and cerebellum (d). Negative signals of tac3a with sense probe results in the telencephalon (a’), thalamus (b’), midbrain (c’), and cerebellum (d’). b, c’, d, d’: Scale bar = 100 μm. a’, b’, c: scale bar = 50 μm. a: scale bar = 20 μm. VP, the postcommissural nucleus of the ventral telencephalon; Hv, the ventral hypothalamus; NRP, the nucleus of the posterior recess; TL, the torus longitudinalis; RF, the reticular formation; CCeM, the molecular layer of corpus cerebelli.
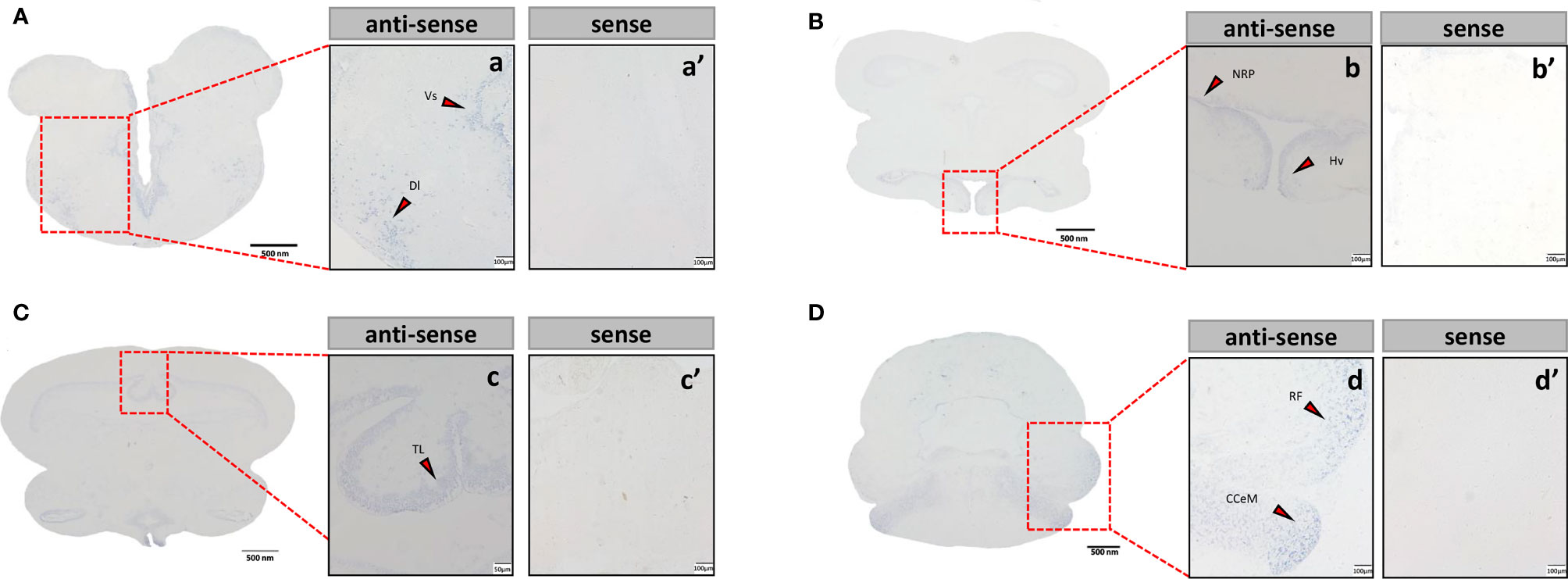
Figure 6 Tac3b mRNA in the brain of Japanese eel was detected by in situ hybridization (ISH). Tac3b localization in the telencephalon. Tac3b localization in the telencephalon (A), thalamus (B), midbrain (C), and cerebellum (D). Scale bar = 500 μm. Positive signal of tac3b in the telencephalon (a), thalamus (b), midbrain (c), and cerebellum (d). Negative signals of tac3a with sense probe results in the telencephalon (a’), thalamus (b’), midbrain (c’), and cerebellum (d’). a, a’, b, b’, c’, d, d’: Scale bar = 100 μm. c: scale bar = 50 μm. Dl, the lateral part of the dorsal telencephalon; Vs, the supracommissural nucleus of the ventral telencephalon; Hv, the ventral hypothalamus; NRP, the nucleus of the posterior recess; TL, the torus longitudinalis; RF, the reticular formation; CCeM, the molecular layer of corpus cerebelli.
Binding Analysis of NKB and TACR3 in Japanese Eel
As shown in Figure 7, NKBa-10 of Japanese eel could weakly activate the CRE pathway via TACR3, whereas NKBa-13, NKBb-10, and NKBb-13 had no effect under the same conditions. Moreover, none of the four peptides binding with the receptor TACR3 could activate the SRE pathway, which indicated that they could not cause the increase of transcriptional activity of SRE pathway. In Figure 8, NKBa-10 of Japanese eel binding with TACR3A1 receptor of zebrafish could effectively activate CRE and SRE signaling pathways, especially significantly increasing the transcriptional activity of SRE signaling pathway.
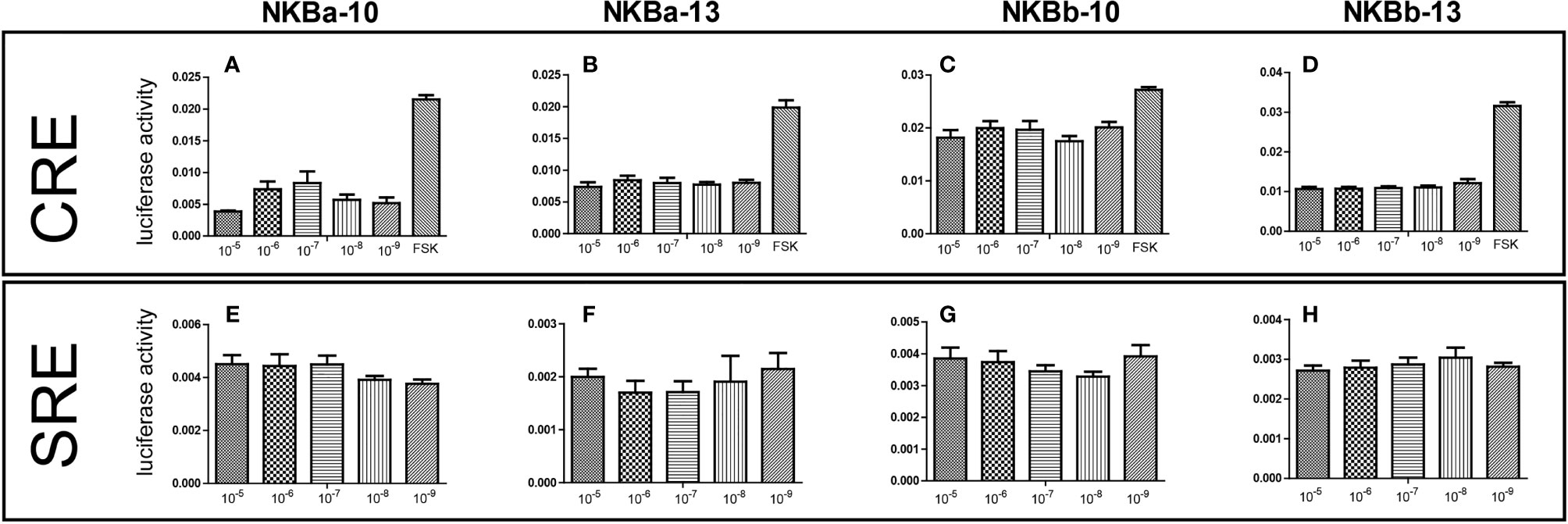
Figure 7 (A–D) NKB peptides induced CRE driven promoter activities co-transfected with Japanese eel tacr3. (E–H) NKB peptides induced SRE driven promoter activities co-transfected with Japanese eel tacr3.
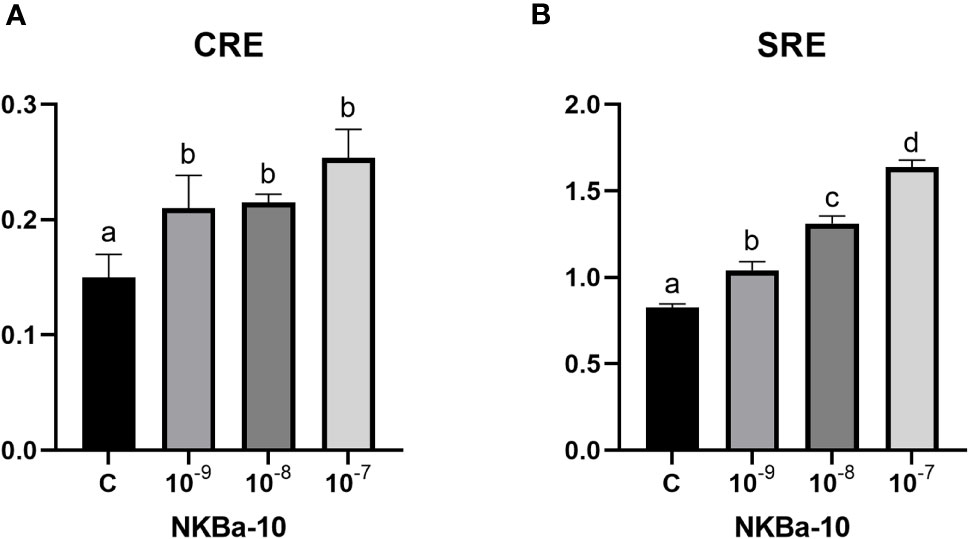
Figure 8 (A) NKBa-10 peptides induced CRE driven promoter activities co-transfected with zebrafish tacr3a1. (B) NKBa-10 peptides induced SRE driven promoter activities co-transfected with zebrafish tacr3a1. The different lowercased letters indicated significant differences (P < 0.05).
Regulation of mRNA Levels of mgnrh, cgnrh, lhb, and fshb genes in the Brain by NKB in vivo
The results showed that high concentration (1,000 ng/g BW) of NKBa-10 and NKBb-13 could increase the expression level of mgnrh significantly (P < 0.05), whereas NKBa-13 and NKBb-10 had no effect on the expression of mgnrh. However, neither of the four peptides in low concentration (100 ng/g BW) had effect on the expression of mgnrh. In addition, both high and low concentration of NKBa-10, NKBa-13, NKBb-10, and NKBb-13 were unable to activate cgnrh expression compared with control group (Figure 9A).
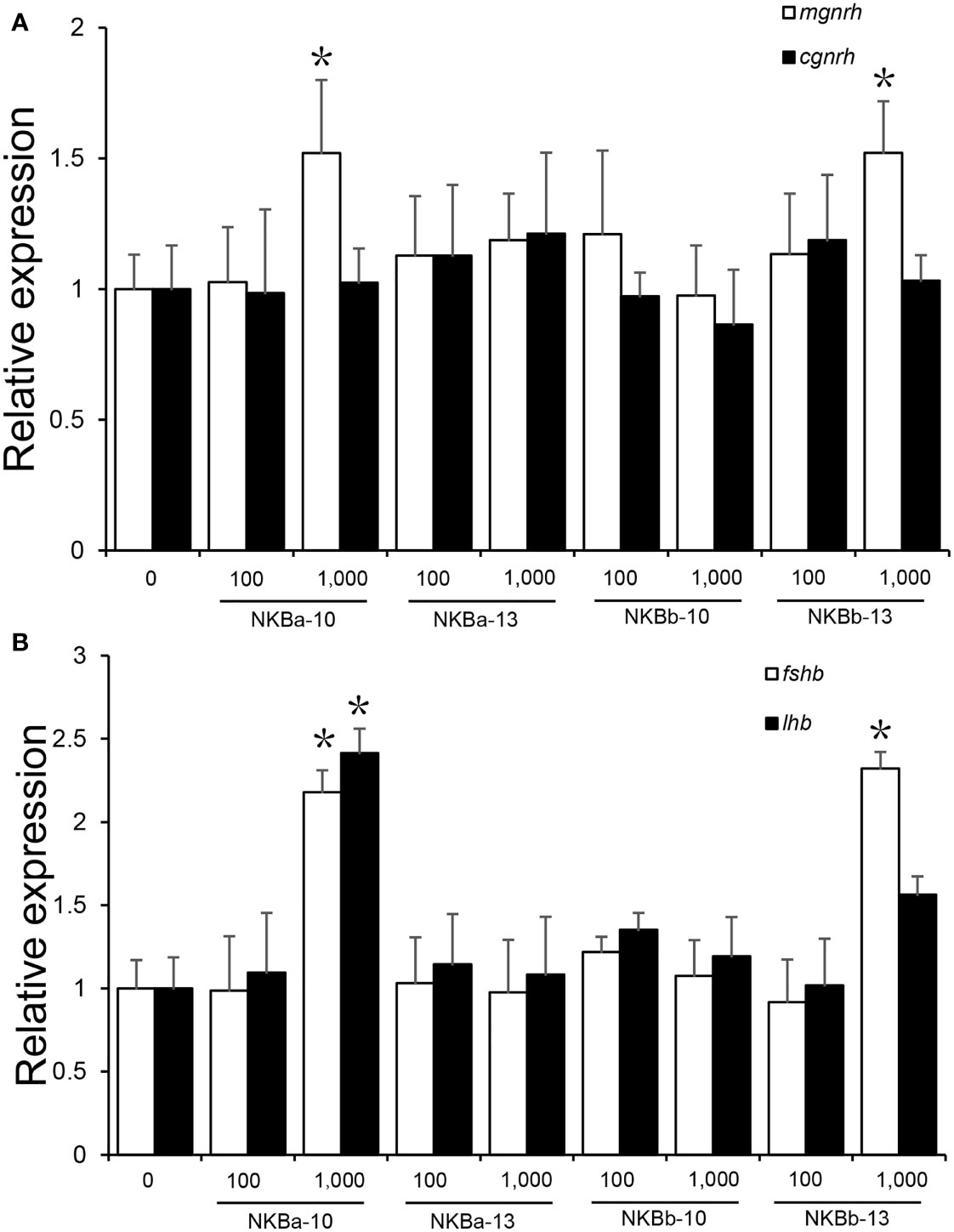
Figure 9 (A) Effects of NKBa-10, NKBa-13, NKBb-10, and NKBb-13 on mgnrh and cgnrh expression of Japanese eel after intraperitoneal injection for 6 h. The X-axis indicates the different NKB peptides and their corresponding concentrations. (B) Effects of NKBa-10, NKBa-13, NKBb-10, and NKBb-13 on fshb and lhb of Japanese eel after intraperitoneal injection for 6 h. The X-axis indicates the different NKB peptides and their corresponding concentrations. The asterisk indicated significant difference of one gene among different stimulate concentration (P < 0.05).
As shown in Figure 9B, high concentration (1,000 ng/g BW) of NKBa-10 and NKBb-13 could significantly upregulate the expression level of fshb (P < 0.05), whereas NKBa-13 and NKBb-10 had no effect on fshb in both peptide concentrations. In the regulation of lhr, only high concentration of NKBa-10 could increase lhr level significantly (P < 0.05). The other three peptides—NKBa-13, NKBb-10, and NKBb-13—could not regulate lhr level in both concentrations compared with control group.
Discussion
Tachykinin, encoded by tac1, tac3, and tac4, is considered as one of the largest families of neuropeptides. It is named due to the ability to rapidly induce intestine contraction (32). More and more evidence showed that tac1 gene produces substance P (SP) and neurokinin A (NKA), tac3 encodes NKB and NKF, and tac4 encodes hemokinin-1 (HK-1) and endokinins (33). Among them, NKB showed its essential role in the onset of puberty and gonadotropin secretion (22, 34). Different from mammals, with only one tac gene to code NKBs, teleosts undergo a teleost-specific genome duplication event (35), which, in the present, causes two tac3 genes. In recent years, an increasing number of studies have reported that the NKB/NKR system plays a critical role in teleost, especially in reproduction (23, 36, 37). Previous studies also have reported two tac3 genes (tac3a and tac3b) in zebrafish, goldfish, salmon, and spotted sea bass (22, 29, 36, 37). In the present study, we have identified two tac3 and one tacr3 genes in Japanese eel. There were two tachykinin peptides in each of Japanese eel TAC3 precursor including NKB-13 (also known as NKB-related peptide) and NKB-10, which suggested conserved functions in teleost.
Studies in mammals and other vertebrates showed that tachykinin shared conservative carboxyl-terminal sequence, -FXGLM-NH2 (38, 39). The four NKBs of Japanese eel generated from two tac3 genes presented the common C-terminal motif (-FVGLM-NH2), which is also found in zebrafish (22), goldfish (23), and spotted sea bass (29). From a chemical point of view, two distinct moieties were presented in tachykinins: a N-terminal sequence with variability in all tachykinins and a C-terminal sequence, which is responsible for receptor activation (38). The terminal Met is regard as an essential factor for tachykinin function because the tachykinin-like peptides from invertebrates with a C-terminal Arg are unable to activate mammalian tachykinin receptors (40, 41). In the present study, three out of four NKBs in Japanese eel—NKBa-13, NKBa-10, and NKBb-13—had identical C-terminal Met, which is supported by the results in zebrafish (22), goldfish (23), spotted sea bass (29), and European eel (Anguilla anguilla) (42).
In the present study, we found mutation in tacr3. To confirm whether the mutation is ubiquitous or from an individual difference, we have collected more than 80 Japanese eels both cultured and wild caught from different locations, including Guangzhou (113°E, 23.5°N), Zhuhai (Guangdong) (113.5°E, 22°N), and Qingdao (Shandong) (120.4°E, 36°N), China. The DNA was purified for PCR with specific primers to amplified the mutation site. The PCR products were then subcloned into E. coli, and three clones were sent for Sanger’s sequencing to confirm the accuracy. As a result, all the sequences confirmed the mutation. In addition, we have noticed the European eel (Anguilla Anguilla) TAC3R sequence (XM_035417067.1), when the mutation in Japanese eel was confirmed. However, the sequence is predicted on the basis of the whole genome sequence. Hence, we have further checked the whole genome sequences in both European eel and American eel (Anguilla rostrata). Interestingly, both genome sequences showed the same results with the Japanese eel, which ended at the mutation site. Unfortunately, we are not able to get the sample or DNA of these eels, so we can not 100% sure about the mutation in other eel species. However, we can be sure about the mutation in the Japanese eel.
Tissue distribution results showed that both tac3a and tac3b were highly expressed in the brain of Japanese eel, consistent with the results in other fishes including zebrafish, goldfish, orange-spotted grouper (Epinephelus coioides), and spotted sea bass (22, 23, 26, 29, 37, 43). Furthermore, tac3a was found mainly expressed in the hypothalamus and pituitary. It is proverbial that the hypothalamus plays an important role in reproductive regulation, which suggests that tac3s genes may be involved in reproductive regulation of Japanese eel as well. In zebrafish and spotted sea bass, tac3a was mainly expressed in the hypothalamus but not detected in the pituitary (22, 29, 37). Whereas, in goldfish, tac3a was strongly expressed in the pituitary (23), consistent with the result in Japanese eel. These results indicated that the expression pattern may be variable in different species. The expression of tac3b in Japanese eel was detected only in the brain but not in pituitary, which is coincident with the result in goldfish (13). In gonad, tac3b was not detected in Japanese eel, which is different from zebrafish or spotted sea bass (29, 37), which may be caused by the different developing stages of gonad.
In situ hybridization results showed that tac3s positive signals were observed in multiple brain regions including telencephalon, mesencephalon, and hypothalamus, which were similar to the results in spotted sea bass (29) and orange-spotted grouper (36). Although it has been reported that tac3s expression is different in brain regions among different species, which is speculated to be caused by interspecies difference or physiological state difference (29). However, tac3s expression was still highest in the brains of teleosts. In addition, it has been established that NKBs is a key regulator of GnRH, regulating the onset of puberty and reproductive physiology in mammals (44), and is involved in the regulation of the reproductive axis in some teleosts, which suggests that NKBs may be involved in the reproductive process and have regulatory effect.
To test whether the mutation in tacr3 cause intracellular structural incompletion, we synthesized four NKBs and used luciferase assay to detect the activation of CRE and SRE signaling pathways. Our results showed that none of the NKBs could activate neither CRE nor SRE signaling pathway via Japanese eel TACR3. In addition, we conducted experiments using NKBa-10 through zebrafish receptor and found that it could activate both two signaling pathways effectively. As a result, the Japanese eel receptor is dysfunctional in further signal transduction (45, 46). By blasting the genome sequence, we figured out another potential tac3r; however, limited to the yellow eel samples, the cloning of the gene failed. These results indicate a possibility that the transcription of the other tac3r was started when the yellow eel turned to the silver eel. A large number of studies proved that mutations in the tac3 or tacr3 genes lead function decline at the hypothalamic level (47). In different species, NKB has different regulatory effects on the release of LH, and the similar condition occurs in different developmental stages of the same species. For example, NKB has no effect on the release of LH in luteinized ewes, but it can promote the release of LH in follicular phase (48). We investigated the reproductive regulation of NKB in Japanese eel. Quantitative Real-time (qPCR) results showed that NKBa-10 and NKBb-13 in high concentration can upregulate the mRNA levels of mgnrh and fshb of Japanese eel, whereas only high concentration of NKBa-10 can increase the mRNA levels of lhb; taking the luciferase assay and the in vivo injection results together, the NKBs may bind to other receptors to increase the gnrh or gth expression level.
In conclusion, our present study provides the novel insights into the reproductive function of NKB in Japanese eel. We identified and characterized the NKB/NK3R system in Japanese eel. In addition, we demonstrated dysfunction of the TAC3R and the stimulation of gnrh and gth by high dose of NKBs. These results present the first demonstrates of NKB/NK3R system in reproduction of Japanese eel.
Data Availability Statement
The datasets presented in this study can be found in online repositories. The names of the repository/repositories and accession number(s) can be found below: NCBI; OL804257.1, OL804258.1.
Ethics Statement
The animal study was reviewed and approved by Animal Research and Ethics Committees of Sun Yat-sen University and Ocean University of China.
Author Contributions
XQ, HW, and YL designed the study. WZ performed the RNA extraction and cDNA preparation. WZ performed the sequence analysis and the tissue expression analysis. CZ performed the in situ hybridization (ISH) experiment. WZ performed cell culture and co-transfection. WZ performed the in vivo injection. CZ and LL wrote the manuscript. XQ provided manuscript editing and feedback. All authors read and approved the final manuscript.
Funding
This study was supported by grants from the National Natural Science Foundation of China (41976089).
Conflict of Interest
The authors declare that the research was conducted in the absence of any commercial or financial relationships that could be construed as a potential conflict of interest.
Publisher’s Note
All claims expressed in this article are solely those of the authors and do not necessarily represent those of their affiliated organizations, or those of the publisher, the editors and the reviewers. Any product that may be evaluated in this article, or claim that may be made by its manufacturer, is not guaranteed or endorsed by the publisher.
References
1. Waylen A, Wolke D. Sex 'N' Drugs 'N' Rock 'N' Roll: The Meaning and Social Consequences of Pubertal Timing. Eur J Endocrinol (2004) 151 Suppl 3:U151–9. doi: 10.1530/eje.0.151u151
2. Vandenbergh JG. Acceleration and Inhibition of Puberty in Female Mice by Pheromones. J Reprod Fertil Suppl (1973) 19:411–9.
3. Schulz RW, Henk J. Puberty in Male Fish: Concepts and Recent Developments With Special Reference to the African Catfish (Clarias Gariepinus). Aquaculture (1999) 177(1-4):5–12. doi: 10.1016/S0044-8486(99)00064-2
4. Weltzien FA, Andersson E, Andersen Ø, Shalchian-Tabrizi K, Norberg B. The Brain-Pituitary-Gonad Axis in Male Teleosts, With Special Emphasis on Flatfish (Pleuronectiformes). Comp Biochem Physiol A Mol Integr Physiol (2004) 137(3):447–77. doi: 10.1016/j.cbpb.2003.11.007
5. Jalabert B. Particularities of Reproduction and Oogenesis in Teleost Fish Compared to Mammals. Reprod Nutr Dev (2005) 45(3):261–79. doi: 10.1051/rnd:2005019
6. Okuzawa K, Kumakura N, Mori A, Gen K, Yamaguchi S, Kagawa H. Regulation of GnRH and its Receptor in a Teleost, Red Seabream. Prog Brain Res (2002) 141:95–110. doi: 10.1016/S0079-6123(02)41087-4
7. IjIRI S, Tsukamoto K, Chow S, Kurogi H, Adachi S, Tanaka H. Controlled Reproduction in the Japanese Eel (Anguilla Japonica), Past and Present. Aquac Eur (2011) 36(2):13–7.
8. Wald G. Metamorphosis: An Overview. In: Metamorphosis. Boston, MA:Springer (1981). p. 1–39. doi: 10.1007/978-1-4613-3246-6_1
9. Waters JM, McDowall RM. Phylogenetics of the Australasian Mudfishes: Evolution of an Eel-Like Body Plan. Mol Phylogenet Evol (2005) 37(2):417–25. doi: 10.1016/j.ympev.2005.07.003
10. Aroua S, Schmitz M, Baloche S, Vidal B, Rousseau K, Dufour S. Endocrine Evidence That Silvering, a Secondary Metamorphosis in the Eel, is a Pubertal Rather Than a Metamorphic Event. Neuroendocrinology (2005) 82(3-4):221–32. doi: 10.1159/000092642
11. Dufour S, Weltzien FA, Sebert ME, Le Belle N, Vidal B, Vernier P, et al. Dopaminergic Inhibition of Reproduction in Teleost Fishes: Ecophysiological and Evolutionary Implications. Ann N Y Acad Sci (2005) 1040:9–21. doi: 10.1196/annals.1327.002
12. Fontaine M, Bertrand E, Lopez E, Callamand O. On the Maturation of Genital Organs in the Female Eel (Anguilla Anguilla L.) and Spontaneous Emmision of Eggs in the Aquarium. C R Hebd Seances Acad Sci (1964) 259:2907–10. doi: 10.1002/pssb.2220690209
13. Laurent P, Kirsch R, Fontaine MM. Structural Modifications of the Esophagus Linked to Osmoregulation in Eels. C R Hebd Seances Acad Sci Ser D: Sci Nat (1975) 280(19):2227–9.
14. Dahl SK, Amstalden M, Coolen L, Fitzgerald M, Lehman M. Dynorphin Immunoreactive Fibers Contact GnRH Neurons in the Human Hypothalamus. Reprod Sci (Thousand Oaks Calif) (2009) 16(8):781–7. doi: 10.1177/1933719109336619
15. Ciofi P, Leroy D, Tramu G. Sexual Dimorphism in the Organization of the Rat Hypothalamic Infundibular Area. Neuroscience (2006) 141(4):1731–45. doi: 10.1016/j.neuroscience.2006.05.041
16. Kinoshita M, Tsukamura H, Adachi S, Matsui H, Uenoyama Y, Iwata K, et al. Involvement of Central Metastin in the Regulation of Preovulatory Luteinizing Hormone Surge and Estrous Cyclicity in Female Rats. Endocrinology (2005) 146(10):4431–6. doi: 10.1210/en.2005-0195
17. Clarkson J, Herbison AE. Postnatal Development of Kisspeptin Neurons in Mouse Hypothalamus; Sexual Dimorphism and Projections to Gonadotropin-Releasing Hormone Neurons. Endocrinology (2006) 147(12):5817–25. doi: 10.1210/en.2006-0787
18. Topaloglu AK, Reimann F, Guclu M, Yalin AS, Kotan LD, Porter KM, et al. TAC3 and TACR3 Mutations in Familial Hypogonadotropic Hypogonadism Reveal a Key Role for Neurokinin B in the Central Control of Reproduction. Nat Genet (2009) 41(3):354–8. doi: 10.1038/ng.306
19. Bhangoo A, Jacobson-Dickman E. The Genetics of Idiopathic Hypogonadotropic Hypogonadism:Unraveling the Biology of Human Sexual Development. Pediatr Endocrinol Rev (2009) 6(3):395–404.
20. Ramaswamy S, Seminara SB, Ali B, Ciofi P, Amin NA, Plant TM. Neurokinin B Stimulates GnRH Release in the Male Monkey (Macaca Mulatta) and is Colocalized With Kisspeptin in the Arcuate Nucleus. Endocrinology (2010) 151(9):4494–503. doi: 10.1210/en.2010-0223
21. Corander MP, Challis BG, Thompson EL, Jovanovic Z, Loraine Tung YC, Rimmington D, et al. The Effects of Neurokinin B Upon Gonadotrophin Release in Male Rodents. J Neuroendocrinol (2010) 22(3):181–7. doi: 10.1111/j.1365-2826.2009.01951.x
22. Biran J, Palevitch O, Ben-Dor S, Levavi-Sivan B. Neurokinin Bs and Neurokinin B Receptors in Zebrafish-Potential Role in Controlling Fish Reproduction. Proc Natl Acad Sci USA (2012) 109(26):10269–74. doi: 10.1073/pnas.1119165109
23. Qi X, Zhou W, Li S, Liu Y, Ye G, Liu X, et al. Goldfish Neurokinin B: Cloning, Tissue Distribution, and Potential Role in Regulating Reproduction. Gen Comp Endocrinol (2015) 221:267–77. doi: 10.1016/j.ygcen.2014.10.017
24. Biran J, Golan M, Mizrahi N, Ogawa S, Parhar IS, Levavi-Sivan B. Direct Regulation of Gonadotropin Release by Neurokinin B in Tilapia (Oreochromis Niloticus). Endocrinology (2014) 155(12):4831–42. doi: 10.1210/en.2013-2114
25. Zhou Y, Qi X, Wen H, Zhang K, Zhang X, Li J, et al. Identification, Expression Analysis, and Functional Characterization of Motilin and Its Receptor in Spotted Sea Bass (Lateolabrax Maculatus). Gen Comp Endocrinol (2019) 277:38–48. doi: 10.1016/j.ygcen.2019.02.013
26. Hu G, He M, Ko WK, Lin C, Wong AO. Novel Pituitary Actions of TAC3 Gene Products in Fish Model: Receptor Specificity and Signal Transduction for Prolactin and Somatolactin α Regulation by Neurokinin B (NKB) and NKB-Related Peptide in Carp Pituitary Cells. Endocrinology (2014) 155(9):3582–96. doi: 10.1210/en.2014-1105
27. Qi X, Salem M, Zhou W, Sato-Shimizu M, Ye G, Smitz J, et al. Neurokinin B Exerts Direct Effects on the Ovary to Stimulate Estradiol Production. Endocrinology (2016) 157(9):3355–65. doi: 10.1210/en.2016-1354
28. Thompson JD, Higgins DG, Gibson TJ. CLUSTAL W: Improving the Sensitivity of Progressive Multiple Sequence Alignment Through Sequence Weighting, Position-Specific Gap Penalties and Weight Matrix Choice. Nucleic Acids Res (1994) 22(22):4673–80. doi: 10.1093/nar/22.22.4673
29. Zhang Z, Wen H, Li Y, Li Q, Li W, Zhou Y, et al. TAC3 Gene Products Regulate Brain and Digestive System Gene Expression in the Spotted Sea Bass (Lateolabrax Maculatus). Front Endocrinol (2019) 10. doi: 10.3389/fendo.2019.00556
30. Liu J, Jones KL, Sumer H, Verma PJ. Stable Transgene Expression in Human Embryonic Stem Cells After Simple Chemical Transfection. Mol Reprod Dev (2009) 76(6):580–6. doi: 10.1002/mrd.20983
31. Huang YS, Richter JD. Analysis of mRNA Translation in Cultured Hippocampal Neurons. Methods Enzymol (2007) 431:143–62. doi: 10.1016/S0076-6879(07)31008-2
32. Carter MS, Krause JE. Structure, Expression, and Some Regulatory Mechanisms of the Rat Preprotachykinin Gene Encoding Substance P, Neurokinin A, Neuropeptide K, and Neuropeptide Gamma. J Neurosci (1990) 10(7):2203–14. doi: 10.1523/JNEUROSCI.10-07-02203.1990
33. Zhang Y, Lu L, Furlonger C, Wu GE, Paige CJ. Hemokinin is a Hematopoietic-Specific Tachykinin That Regulates B Lymphopoiesis. Nat Immunol (2000) 1(5):392–7. doi: 10.1038/80826
34. Rance NE, Krajewski SJ, Smith MA, Cholanian M, Dacks PA. Neurokinin B and the Hypothalamic Regulation of Reproduction. Brain Res (2010) 1364:116–28. doi: 10.1016/j.brainres.2010.08.059
35. Christoffels A, Koh EG, Chia JM, Brenner S, Aparicio S, Venkatesh B. Fugu Genome Analysis Provides Evidence for a Whole-Genome Duplication Early During the Evolution of Ray-Finned Fishes. Mol Biol Evol (2004) 21(6):1146–51. doi: 10.1093/molbev/msh114
36. Chen H, Xiao L, Liu Y, Li S, Li G, Zhang Y, et al. Neurokinin B Signaling in Hermaphroditic Species, a Study of the Orange-Spotted Grouper (Epinephelus Coioides). Gen Comp Endocrinol (2018) 260:125–35. doi: 10.1016/j.ygcen.2018.01.009
37. Zhou W, Li S, Liu Y, Qi X, Chen H, Cheng CH, et al. The Evolution of Tachykinin/Tachykinin Receptor (TAC/TACR) in Vertebrates and Molecular Identification of the TAC3/TACR3 System in Zebrafish (Danio Rerio). Mol Cell Endocrinol (2012) 361(1-2):202–12. doi: 10.1016/j.mce.2012.04.007
38. Almeida TA, Rojo J, Nieto PM, Pinto FM, Hernandez M, Martín JD, et al. Tachykinins and Tachykinin Receptors: Structure and Activity Relationships. Curr Med Chem (2004) 11(15):2045–81. doi: 10.2174/0929867043364748
39. Hashemian P, Javid H, Tadayyon Tabrizi A, Hashemy SI. The Role of Tachykinins in the Initiation and Progression of Gastrointestinal Cancers: A Review. Int J Cancer Manag (2020) 13(5):e100717. doi: 10.5812/ijcm.100717
40. Siviter RJ, Coast GM, Winther AM, Nachman RJ, Taylor CA, Shirras AD, et al. Expression and Functional Characterization of a Drosophila Neuropeptide Precursor With Homology to Mammalian Preprotachykinin a. J Biol Chem (2000) 275(30):23273–80. doi: 10.1074/jbc.M002875200
41. Nässel DR. Tachykinin-Related Peptides in Invertebrates: A Review. Peptides (1999) 20(1):141–58. doi: 10.1016/S0196-9781(98)00142-9
42. Campo A, Lafont AG, Lefranc B, Leprince J, Tostivint H, Kamech N, et al. Tachykinin-3 Genes and Peptides Characterized in a Basal Teleost, the European Eel: Evolutionary Perspective and Pituitary Role. Front Endocrinol (2018) 9. doi: 10.3389/fendo.2018.00304
43. Ogawa S, Ramadasan PN, Goschorska M, Anantharajah A, Ng KW, Parhar IS. Cloning and Expression of Tachykinins and Their Association With Kisspeptins in the Brains of Zebrafish. J Comp Neurol (2012) 520(13):2991–3012. doi: 10.1002/cne.23103
44. Lehman MN, Coolen LM, Goodman RL. Minireview: Kisspeptin/Neurokinin B/dynorphin (KNDy) Cells of the Arcuate Nucleus: A Central Node in the Control of Gonadotropin-Releasing Hormone Secretion. Endocrinology (2010) 151(8):3479–89. doi: 10.1210/en.2010-0022
45. DeFea KA, Zalevsky J, Thoma MS, Déry O, Mullins RD, Bunnett NW. Beta-Arrestin-Dependent Endocytosis of Proteinase-Activated Receptor 2 is Required for Intracellular Targeting of Activated ERK1/2. J Cell Biol (2000) 148(6):1267–81. doi: 10.1083/jcb.148.6.1267
46. Li H, Leeman SE, Slack BE, Hauser G, Saltsman WS, Krause JE, et al. A Substance P (Neurokinin-1) Receptor Mutant Carboxyl-Terminally Truncated to Resemble a Naturally Occurring Receptor Isoform Displays Enhanced Responsiveness and Resistance to Desensitization. Proc Natl Acad Sci USA (1997) 94(17):9475–80. doi: 10.1073/pnas.94.17.9475
47. Young J, Bouligand J, Francou B, Raffin-Sanson ML, Gaillez S, Jeanpierre M, et al. TAC3 and TACR3 Defects Cause Hypothalamic Congenital Hypogonadotropic Hypogonadism in Humans. J Clin Endocrinol Metab (2010) 95(5):2287–95. doi: 10.1210/jc.2009-2600
Keywords: tachykinins, neurokinin B, reproductive regulation, ovary development, Japanese eel
Citation: Zuo C, Lyu L, Zou W, Wen H, Li Y and Qi X (2022) TAC3/TACR3 System Function in the Catadromous Migration Teleost, Anguilla japonica. Front. Endocrinol. 13:848808. doi: 10.3389/fendo.2022.848808
Received: 05 January 2022; Accepted: 23 June 2022;
Published: 22 July 2022.
Edited by:
Bin Wang, Yellow Sea Fisheries Research Institute (CAFS), ChinaReviewed by:
Satoshi Ogawa, Monash University Malaysia, MalaysiaGuangfu Hu, Huazhong Agricultural University, China
Copyright © 2022 Zuo, Lyu, Zou, Wen, Li and Qi. This is an open-access article distributed under the terms of the Creative Commons Attribution License (CC BY). The use, distribution or reproduction in other forums is permitted, provided the original author(s) and the copyright owner(s) are credited and that the original publication in this journal is cited, in accordance with accepted academic practice. No use, distribution or reproduction is permitted which does not comply with these terms.
*Correspondence: Xin Qi, cXhAb3VjLmVkdS5jbg==
†These authors have contributed equally to this work