- 1Department of Urology, Herlev and Gentofte Hospital, Herlev, Denmark
- 2Department of Clinical Medicine, University of Copenhagen, Copenhagen, Denmark
- 3Laboratory of Reproductive Biology, University Hospital of Copenhagen, Rigshospitalet, Copenhagen, Denmark
- 4Department of Translational Medicine and Reproductive Medicine Centre, Lunds University and Skane University Hospital, Malmö, Sweden
- 5Department of Growth and Reproduction, University Hospital of Copenhagen, Rigshospitalet, Copenhagen, Denmark
- 6Department of Urology, University of Michigan, Ann Arbor, MI, United States
- 7Centre of Andrology & Fertility Clinic, Department D, Odense University Hospital, Odense C, Denmark
Background: Infertile men with non-obstructive azoospermia (NOA) have impaired spermatogenesis. Dilated and un-dilated atrophic seminiferous tubules are often present in the testes of these patients, with the highest likelihood of active spermatogenesis in the dilated tubules. Little is known about the un-dilated tubules, which in NOA patients constitute the majority. To advance therapeutic strategies for men with NOA who fail surgical sperm retrieval we aimed to characterize the spermatogonial stem cell microenvironment in atrophic un-dilated tubules.
Methods: Testis biopsies approximately 3x3x3 mm3 were obtained from un-dilated areas from 34 patients. They were classified as hypospermatogenesis (HS) (n=5), maturation arrest (MA) (n=14), and Sertoli cell only (SCO) (n= 15). Testis samples from five fertile men were included as controls. Biopsies were used for histological analysis, RT-PCR analysis and immunofluorescence of germ and Sertoli cell markers.
Results: Anti-Müllerian hormone mRNA and protein expression was increased in un-dilated tubules in all three NOA subtypes, compared to the control, showing an immature state of Sertoli cells (p<0.05). The GDNF mRNA expression was significantly increased in MA (P=0.0003). The BMP4 mRNA expression showed a significant increase in HS, MA, and SCO (P=0.02, P=0.0005, P=0.02, respectively). The thickness of the tubule wall was increased 2.2-fold in the SCO-NOA compared to the control (p<0.05). In germ cells, we found the DEAD-box helicase 4 (DDX4) and melanoma-associated antigen A4 (MAGE-A4) mRNA and protein expression reduced in NOA (MAGE-A: 46% decrease in HS, 53% decrease in MA, absent in SCO). In HS-NOA, the number of androgen receptor positive Sertoli cells was reduced 30% with a similar pattern in mRNA expression. The γH2AX expression was increased in SCO as compared to HS and MA. However, none of these differences reached statistical significance probably due to low number of samples.
Conclusions: Sertoli cells were shown to be immature in un-dilated tubules of three NOA subtypes. The increased DNA damage in Sertoli cells and thicker tubule wall in SCO suggested a different mechanism for the absence of spermatogenesis from SCO to HS and MA. These results expand insight into the differences in un-dilated tubules from the different types of NOA patients.
Introduction
Azoospermia is identified in 1% of all men and up to 20% of infertile men, and is subdivided as obstructive and non-obstructive azoospermia (NOA) (1, 2). NOA, the most severe form of impaired male fertility, is the absence of spermatozoa in the ejaculate caused by reduced or missing spermatogenesis (3). Several potential causes of NOA are known, including varicocele, previous gonadotoxic therapy, Y chromosome microdeletions and Klinefelter’s Syndrome, but in up to half of the cases a clear etiology is not identified (4). Based on the histological assessment of testis biopsies, men with NOA, independent of cause, can be classified into one of the three categories: Hypospermatogenesis (HS) - decreased spermatogenesis with all types of germ cells present, maturation arrest (MA) - premature arrest of spermatogenesis, and Sertoli cell-only (SCO) - absence of germ cells (5, 6).
In men diagnosed with NOA, surgical retrieval of spermatozoa, which can be used for intracytoplasmic sperm injection (ICSI), is performed. However, sperm retrieval rates are approximately 50% (7), leaving half of all NOA patients to rely on donor sperm. Novel therapeutic techniques, such as spermatogonial stem cell-based transplantation or autologous tissue transplantation, might represent alternatives to restore fertility in the future (8, 9). In order to advance these strategies more detailed information on the microenvironment of the seminiferous tubules including germ cells and Sertoli cells from different types of NOA patients is required.
Sertoli cells in the seminiferous tubules support the niche in which the spermatogonial stem cells (SSCs) differentiate into spermatozoa (10, 11). A delicate and intricate hormonal balance between Sertoli cell function and SSCs is required to support full spermatogenesis, which also involves Leydig cells providing sufficient amounts of androgens. Testosterone from Leydig cells acts via the androgen receptor (AR) which is expressed in mature Sertoli cells but not in the germ cells. Moreover, Anti-Müllerian hormone (AMH) is secreted by immature Sertoli cells and is downregulated by increased intratesticular testosterone during puberty (12). Sertoli cells produce glial cell-derived neurotrophic factor (GDNF) and bone morphogenetic protein 4 (BMP4) that influence undifferentiated spermatogonia in rodent but their role remains unclear in human (13–16). Peritubular myoid cells (PTMCs) constitute the tubular wall and act to contract seminiferous tubules leading to the transfer of immotile sperm. PTMCs exerts paracrine functions on Sertoli cells and Leydig cells (17, 18). Activations of Leydig cell expressed luteinizing hormone(LH) receptor lead to production of testosterone which signal through AR receptors expressed on Sertoli cells (19). Collectively, successful spermatogenesis is dependent on proper function of several cell types in the testis and hormonal stimulation, which in NOA patients are aberrant in one or more, currently unknown, steps.
Men with NOA occasionally have pockets of “dilated” seminiferous tubules. These tubules are likely to have a normal diameter but appear dilated in relation to the surrounding atrophic “un-dilated” tubules. For practical reasons the terms “dilated” and “un-dilated” will be used in the remainder of the text. Dilated tubules often manage, for unknown reasons, to sustain active spermatogenesis while the un-dilated areas lack this ability. Extraction of sperm cells from the dilated tubules by microdissection testicular sperm extraction (mTESE) is used clinically to obtain spermatozoa for ICSI. In an attempt to advance understanding of reasons for the spermatogenic impairment in the un-dilated seminiferous tubules in NOA patients, we explored different markers of germ and Sertoli cells using mRNA expression and immunofluorescence. Thus, in order to understand how to advance spermatogenesis in testis tissue from NOA patients, we aimed at understanding the difference in molecular characteristics of germ cells and Sertoli cells between testes from men with normal spermatogenesis and atrophic un-dilated tubules in testis biopsies from NOA men with HS, MA and SCO.
Materials and Methods
Human Testis Materials
Testis tissue was obtained from 34 NOA patients who underwent mTESE as a part of treatment for infertility and from five adult men with normal sperm production and proven fertility, who provided a biopsy in connection with vasectomy. In patients with NOA, testis biopsies approximately 3x3x3 mm3 were obtained anteriorly right under the tunica albuginea in connection with the mTESE procedure and used for histopathological diagnosis. Additional testis biopsies that did not show dilated seminiferous tubules (as observed under the operating microscope) were taken from the same area. Consequently, in NOA men, only biopsies from un-dilated tubules were used in this study. All testicular biopsies for research purposes were placed immediately in McCoy 5A medium (modified 22330-021, Gibco, UK) for transportation to the Laboratory of Reproductive Biology for the cryopreservation. Then they were equilibrated for 20 min in media consisting of 1.5 M ethylene glycol, 0.1 M sucrose, 10 mg/ml human serum albumin (HSA) (CSL Behring, Germany), frozen and cryopreserved in -196°C liquid nitrogen according to previous published methods (20).
Clinical Workup
All men with NOA were diagnosed after a complete medical history and physical examination including scrotal ultrasound. Azoospermia was diagnosed according to the 5th edition of World Health Organization (WHO) laboratory manual for the “Examination and processing of human semen” (21). A full hormonal evaluation including serum levels of follicle-stimulating hormone (FSH), LH, inhibin. B and testosterone was performed. All men were assessed for the presence of Y chromosome microdeletions and a karyotype was obtained. Fasting morning blood samples were drawn. Serum testosterone levels were analyzed by a chemiluminescence immunoassay (Access 2, Beckman Coulter, Brea, CA, USA), follicle-stimulating hormone FSH and LH by a time-resolved immunofluorometric assay (Delfia, Wallac, Turku, Finland), and inhibin B by a specific two-sided enzyme-immunometric assay (Inhibin B gen II, Beckman Coulter Ltd, High Wycombe, UK). Culture of peripheral blood lymphocytes was used for karyotype analysis. The diagnosis of NOA was made after a complete assessment by an experienced andrologist using all the above information. Men with testis size larger than 15ml, indication of obstructive causes of azoospermia and Klinefelter’s Syndrome were not included.
Tissue Processing and Histology
Thawing was done by progressively using the following three thawing media. Thawing medium I: 0.75 M ethylene glycol, 0.25 M sucrose in PBS, and 10 mg/ml HSA; thawing medium II: 0.25 M sucrose in PBS, and 10 mg/ml HSA; thawing medium III: PBS and 10 mg/ml HSA, each medium for 10 min (20). After thawing, one testis biopsy from each patient was divided into three parts. One part for immunostaining, one for qPCR, and one was re-frozen for future use. Tissues for immunostaining were fixed in 4% paraformaldehyde (PFA) at room temperature overnight, embedded in paraffin and cut in 5-μm sections. Sections were deparaffinized in xylene, rehydrated with series of graded ethanol. Sections for histological evaluation were stained with periodic acid-Schiff reagent (PAS). Due to the heterogeneity of testis tissues in men with NOA, the spermatogenetic status of all 34 samples was histologically re-analyzed on sections stained with PAS in addition to the original histopathological diagnosis made as part of clinical care using a 3x3x3 mm3 biopsy taken anteriorly under the tunica albuginea. If different, the histopathological diagnosis from un-dilated tubules was used.
Immunofluorescence Staining
After deparaffinization and rehydration of the section, antigens were retrieved by boiling in TEG buffer (10 mM Tris, 0.5 mM ethylene glycol-bis (2-aminoethylehter)-N, N, N’, N’-tetraacetic acid (EGTA), pH 9) for 30 min. After non-specific binding was blocked with 1% bovine serum albumin (BSA) in Tris-buffered saline (TBS) buffer (50 mM Tris, 150 mM NaCl, pH7.6) for 30 min, the sections were incubated with primary antibodies at +4°C overnight. All antibodies were diluted in TBS with 1% BSA. The primary antibodies included (Supplementary Table 1): a monoclonal mouse anti-melanoma antigen genes-A (MAGE-A) (1:100) for detection of MAGE-A1, MAGE-A2, MAGE-A3, MAGE-A4, MAGE-A6, MAGE-A10, MAGE-A12 which were previously shown to be present in spermatogonia and some spermatocytes (22, 23), a monoclonal mouse anti-ubiquitin carboxyl-terminal hydrolase L1 (UCHL1, also known as protein gene product 9.5, PGP 9.5) (1:100) present in human spermatogonia (24), a polyclonal rabbit anti- phosphorylation of histone H2AX in Serine 139 (γH2AX) (meiotic marker in germ cells and a marker of DNA double-strand breaks in somatic cells) (1:1500) (25, 26), a monoclonal mouse anti-Vimentin (1:200) for somatic cells (27), a polyclonal rabbit anti-SOX9 (1:100) for Sertoli cells (28), a polyclonal goat anti-Müllerian hormone (AMH) (1:100) for immature Sertoli cells (28), a monoclonal rabbit anti-androgen receptor (AR) (1:100) for mature Sertoli cells (29), a rabbit polyclonal anti-alpha-smooth muscle actin (ACTA) (1:150) for peritubular myoid cells (30), a goat polyclonal anti-cytochrome P450 17A1 (CYP17A1) (1:200) for Leydig cells (23, 31). And universal negative control serum (NC498H, Biocare Medical) was used for negative control. After washing 3 times in TBS with Tween 20®, the slides were incubated with the following secondary antibodies at room temperature for 1h: FITC-conjugated donkey anti-mouse IgG antibody/Alexa Fluor 594 donkey anti-rabbit IgG antibody/Alexa Fluor 568 donkey anti-goat IgG antibody (1:500, Jackson ImmunoResearch). After washing, the slides were stained with 4’,6 – diamidino-2-phenylindole (DAPI) for nuclear staining. Pictures were taken on a Zeiss Axiophot microscope, operated with a Leica DFC420C digital microscope camera and LAS V4.9 software (Leica).
Five seminiferous tubules per section were randomly chosen (two tubules from upper panel, two tubules from lower panel, one tubule from the center) and two histological sections at different depths of the biopsy were evaluated per sample. The number of SOX9/AR-positive Sertoli cells and MAGE-A-positive germ cells per square millimeter (mm2) was calculated. Firstly, we measured the diameter of the tubule to calculate the area (mm2) of the tubule. Then, we counted the number of SOX9/AR/MAGE-A-positive cells within each tubule. We got the number of SOX9/AR/MAGE-A-positive cells/tubule area (mm2). Finally, we calculated the mean number of SOX9/AR/MAGE-A-positive cells/mm2 based on 10 tubules per testis biopsy. For the MAGE-A-positive germ cell, we only counted positive cells located on the basement membrane to exclude counting of spermatocytes. We evaluated AMH expression based on its staining intensity from “strong” “moderate” “mild” to “absent”. The thickness of tubule wall was measured at four points around the circumference of each tubule, with ACTA-positive signal thickness measured at the ends of two perpendicular axes.
RNA Extraction and Quantitative RT-PCR
RNA was extracted from testis biopsies from all 34 NOA patients and five normal control testis tissues using Trizol reagent (Invitrogen) and 1-bromo-3-chloropropane (Sigma). Then the following steps were performed using the RNeasy Kit (Qiagen) according to the manufacturer’s protocol. The average RNA obtained was 22ug with a range from 5ug to 80ug. The 260/280 ratio was found to be 2.1 ± 0.04 (range: 2.00 to 2.14). The total RNA from each sample used to make the cDNA was 1ug. cDNA was synthesized by using of High-Capacity cDNA Reverse Transcription Kit (Applied Biosystems). The dilution of the cDNA template used to do qPCR was 1:5.
Quantitative RT-PCR was performed by using TaqMan Fast Advanced Master Mix (Applied Biosystems) and the LightCycler 480 Instrument II (Roche Diagnostics). The cDNA from each sample was used as a RT-PCR template to be detected of the expression of those genes from TaqMan primer assays (Supplementary Table 2). GAPDH was used as an internal control and the normal adult testis tissue as a positive control.
Statistics
Individual values were presented as mean ± standard deviation (sd). GraphPad Prism version 8.0 was used for statistical analyses. Kruskal-Wallis test with Dunn’s multiple comparisons test was used to analyze the difference of mRNA expression among HS, MA, SCO to the normal control group, respectively. For the histological results, each subtype of NOA was compared to the normal control. The statistical difference of the number of MAGE-A/SOX9/AR-positive cells and the thickness of tubule wall among HS, MA, SCO to the normal control group was analyzed by Kruskal-Wallis test with Dunn’s multiple comparisons test. Chi-square test was used to analyze the percentage of AMH-positive tubules in NOA subtypes compared to normal control group. Correlations between mRNA expression and hormone levels were tested by Spearman rank correlation coefficient. P values < 0.05 were considered statistically significant.
Results
PAS Staining for Histopathological Diagnosis
Based on histological evaluation of biopsies from the un-dilated areas of seminiferous tubules and the clinical diagnosis, our material consisted of 5 HS, 14 MA, and 15 SCO samples (Table 1).
Characterization of Niche Related Cells in the Testis From Different Types of NOA Patients Using Immunofluorescence Staining
Somatic Cells
SOX9-positive Sertoli cells appeared disorganized with a scattered distribution in HS while organized with a more circle like location close to the basal membrane in MA compared to normal group, contrasting the SCO samples which resembled the normal tissue with cells tidily located close to the basement membrane and near one another to each other (Figures 1A, B). The total number of SOX9-positive cells was counted in ten tubules and the average number per tubule was 20 in HS, 19 in MA, 24 in SCO, and 18 in normal control group. The average number of AR-positive cells per tubule was 21 in HS, 22 in MA, 24 in SCO, and 28 in normal control group. Combining the different size of tubules, the number of SOX9/AR-positive Sertoli cells per mm2 was not significantly different in NOA subtypes compared to the normal group (Figures 1A-D). The percentage of AMH-positive tubules showed significant increase in HS, MA, and SCO compared to normal control group (HS, MA, SCO: P<0.001). The percentage of tubules with “strong” AMH expression in each NOA subtypes was significantly increased to normal control (HS: P<0.001, MA: P=0.001, SCO: P<0.001). The same significant increase was observed in tubules with “moderate” AMH expression (HS, MA, SCO: P<0.001). The percentage of tubules with “mild” AMH expression significantly increased only in SCO (P=0.006) (Figures 1E, F).
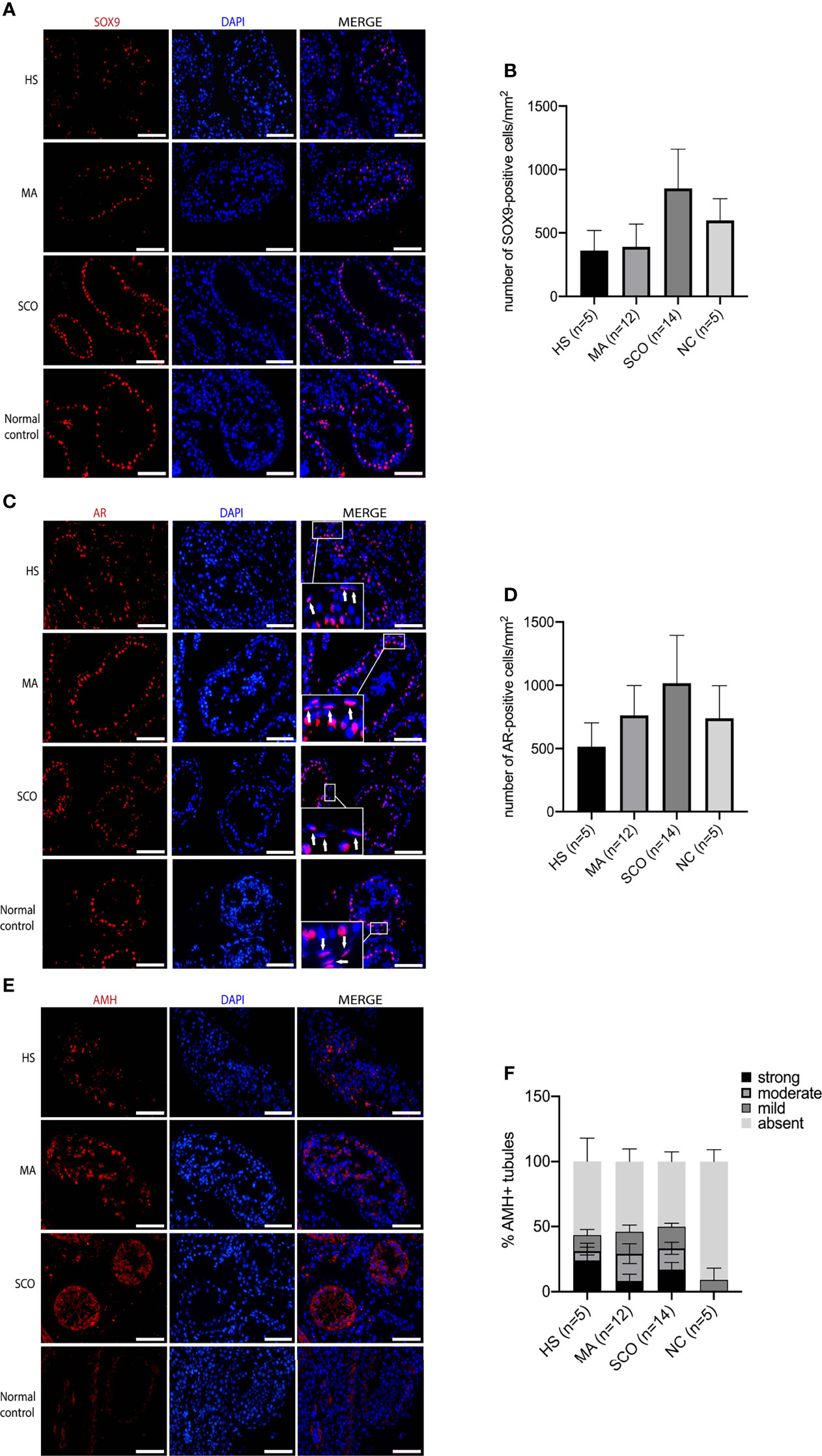
Figure 1 Immunofluorescence staining of Sertoli cell markers in un-dilated seminiferous tubules from hypospermatogenesis (HS), maturation arrest (MA), Sertoli cell only (SCO), and normal control (NC) samples. (A) Sox9 (red) for Sertoli cells. (B) The number of Sox9-positive cells per mm2 was counted from ten tubules of each biopsy. (C) AR (red) for mature Sertoli cells, white arrow indicated that AR also expressed in PTMCs. (D) AR-positive cells per mm2 was counted from ten tubules of each biopsy. (E) AMH (red) for immature Sertoli cells. (F) AMH staining was categorized into “strong” “moderate” “mild” “absent” based on staining intensity. The percentage of AMH-positive tubules (including strong, moderate, mild expression of AMH) showed significant increase in HS, MA, and SCO comparing to normal control group. The percentage of tubules with “strong” AMH expression in each NOA subtypes was significantly increased to normal control (HS: P < 0.001, MA: P = 0.001, SCO: P < 0.001). The percentage of tubules with “moderate” AMH expression in each NOA subtypes was also significantly increased (HS, MA, SCO: P< 0.001). The percentage of “mild” AMH expression only significantly increased in SCO (P = 0.006). DAPI (blue) for nuclear staining. Scale bar: 100 μm. n represent the number of individuals included.
Visualizing the peritubular myoid cells (PTMCs) via ACTA-positive staining, the distribution of cells and the thickness of tubule wall appeared to be normal in both HS and MA patients, while the tubule wall in the SCO patient was 2.2-fold thicker than in the normal testis tissues (P = 0.001) (Figures 2A, B).
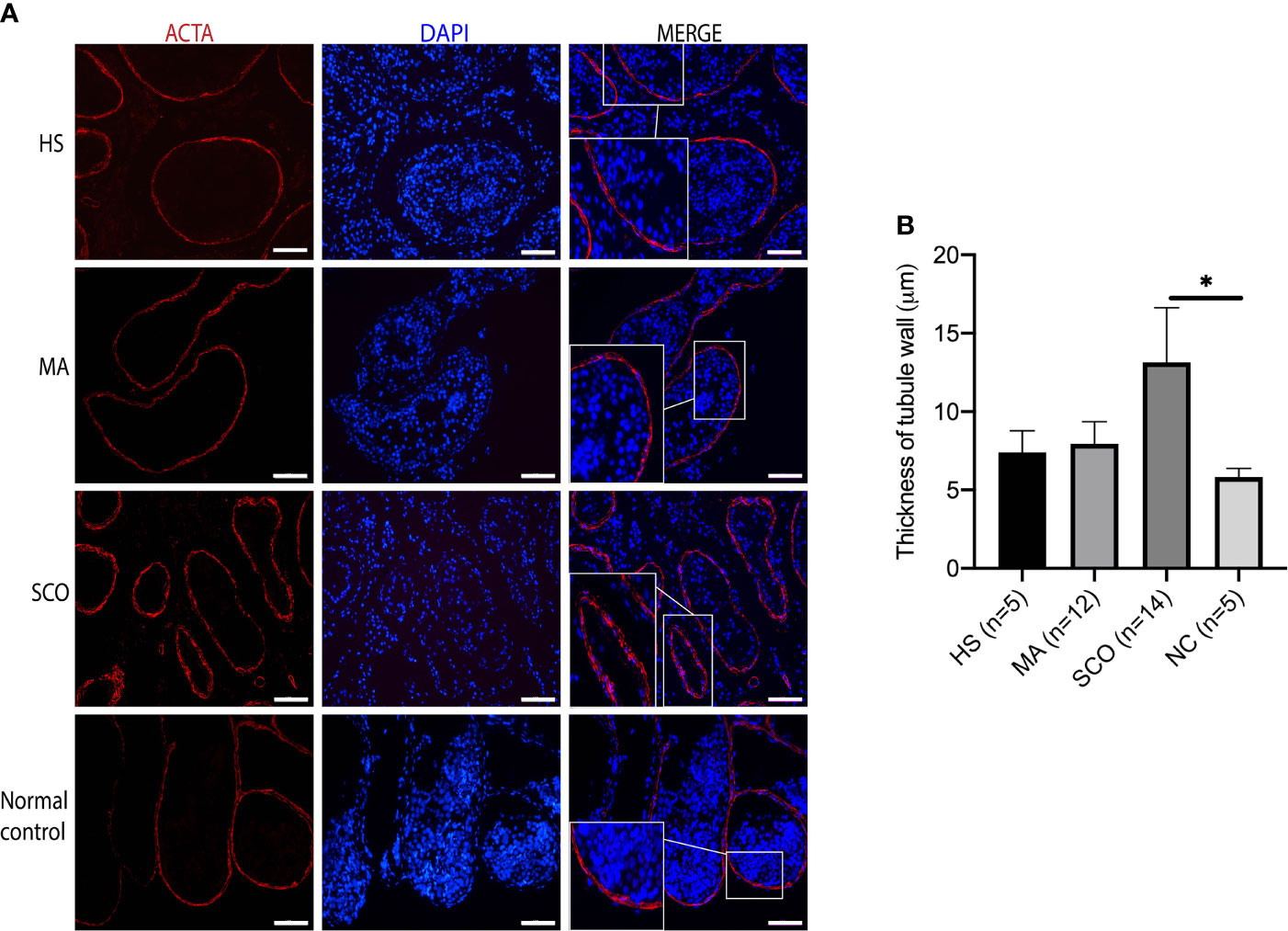
Figure 2 Immunofluorescence staining of peritubular myoid cell (PTMC) marker in un-dilated seminiferous tubules from HS, MA, SCO, and NC samples. (A) Alpha-smooth muscle actin (ACTA) (red) for PTMC, DAPI (blue) for nuclear staining, Scale bar: 100 μm. (B) Thickness analysis of tubule wall. Asterisk indicated significant difference between SCO and normal control group (*p < 0.05).
Leydig cells visualized via CYP17A1 expression showed no difference in distribution between any of the NOA samples and the normal controls (Figure 3). Due to the tiny testis biopsies the interstitial tissues were not fixed well and quantification of the CYP17A1-positive Leydig cells was not done in this study.
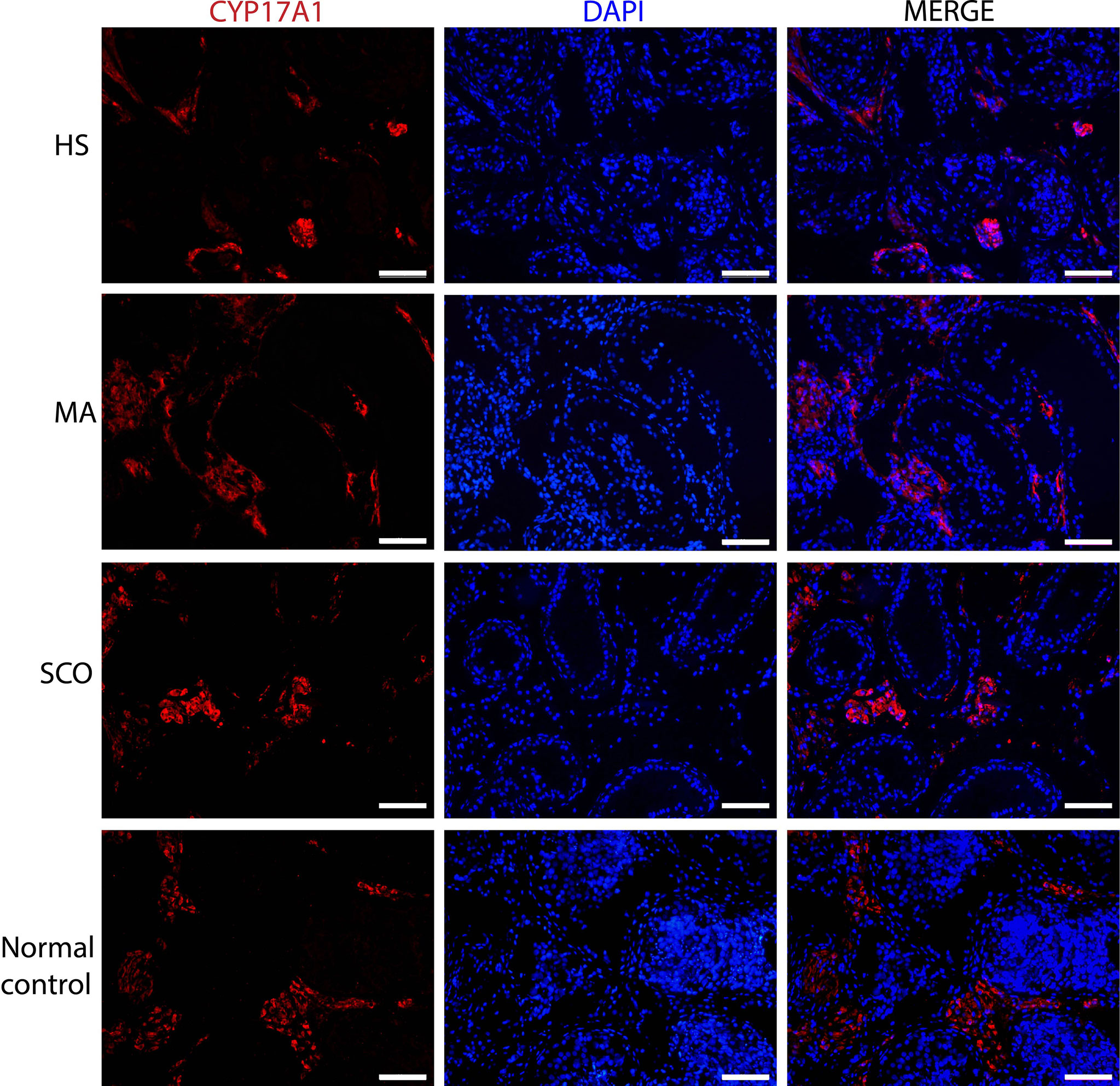
Figure 3 Immunofluorescence staining of Leydig cell marker in un-dilated seminiferous tubules from HS, MA, SCO, and NC samples. CYP17A1 (red) for Leydig cells, DAPI (blue) for nuclear staining. Scale bar: 100 μm.
γH2AX-Positive Cells
In HS, there was a strong homogenous nuclear staining and prominent γH2AX foci in most of the germ cells (Figure 4). Some nuclear staining was weak and dispersed, but most were dotted and strong. Almost no γH2AX expression was detected in Sertoli cells stained with Vimentin. In MA, γH2AX was strongly expressed in the nuclei of germ cells in a dotted and dispersed pattern. There were a few Sertoli cells with γH2AX staining (Figure 4). In SCO, almost all Sertoli cells showed a pronounced expression γH2AX in the nuclei (Figure 4).
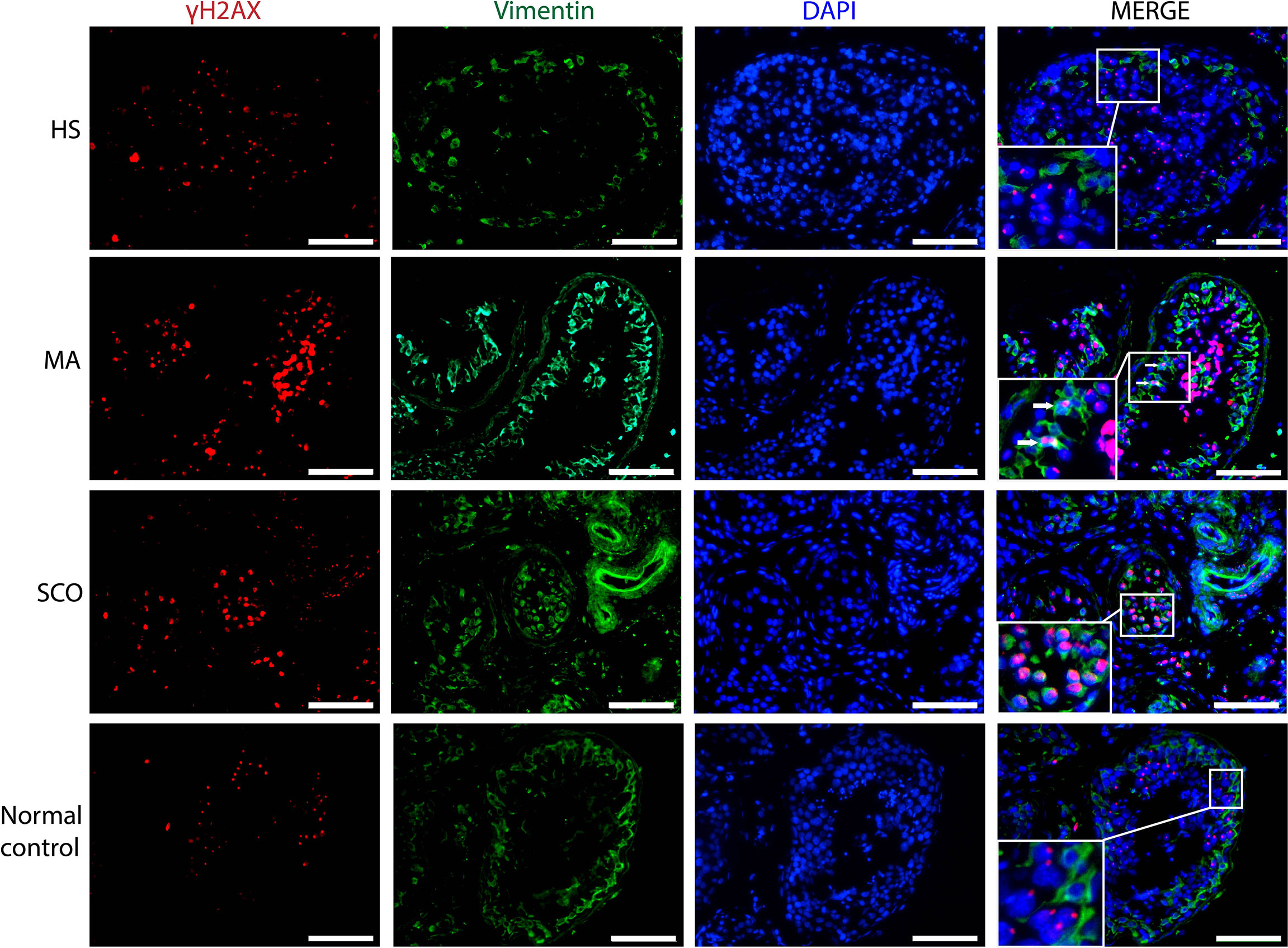
Figure 4 Immunofluorescence staining of γH2AX (red), somatic cell marker Vimentin (green), nuclear marker DAPI (blue) in un-dilated seminiferous tubules in samples from HS, MA, and SCO samples. White arrow indicated γH2AX-positive Sertoli cells in MA. As almost all Sertoli cells expressed γH2AX in SCO, we did not add arrow to indicate them. There were no γH2AX-positive Sertoli cells in HS and normal control group. Scale bar: 100 μm.
Germ Cells
Both germ cell markers, MAGE-A and UCHL1, were positively expressed in the un-dilated seminiferous tubules of HS and MA patients indicating the presence of germ cells (Figures 5A, B). The average number of MAGE-A-positive cells per tubule was 17 in HS, 15 in MA, 0 in SCO, and 26 in normal control group. The number of MAGE-A-positive cells per mm2 was not significantly different in HS and MA compared to the normal group (Figure 5C). In contrast, no expression of MAGE-A and UCHL1 was present in SCO patients (Figures 5A-C). The staining patterns in these three types were mainly cytoplasmic and the location of positive cells was near the basement membrane of the seminiferous tubules as observed in the normal control.
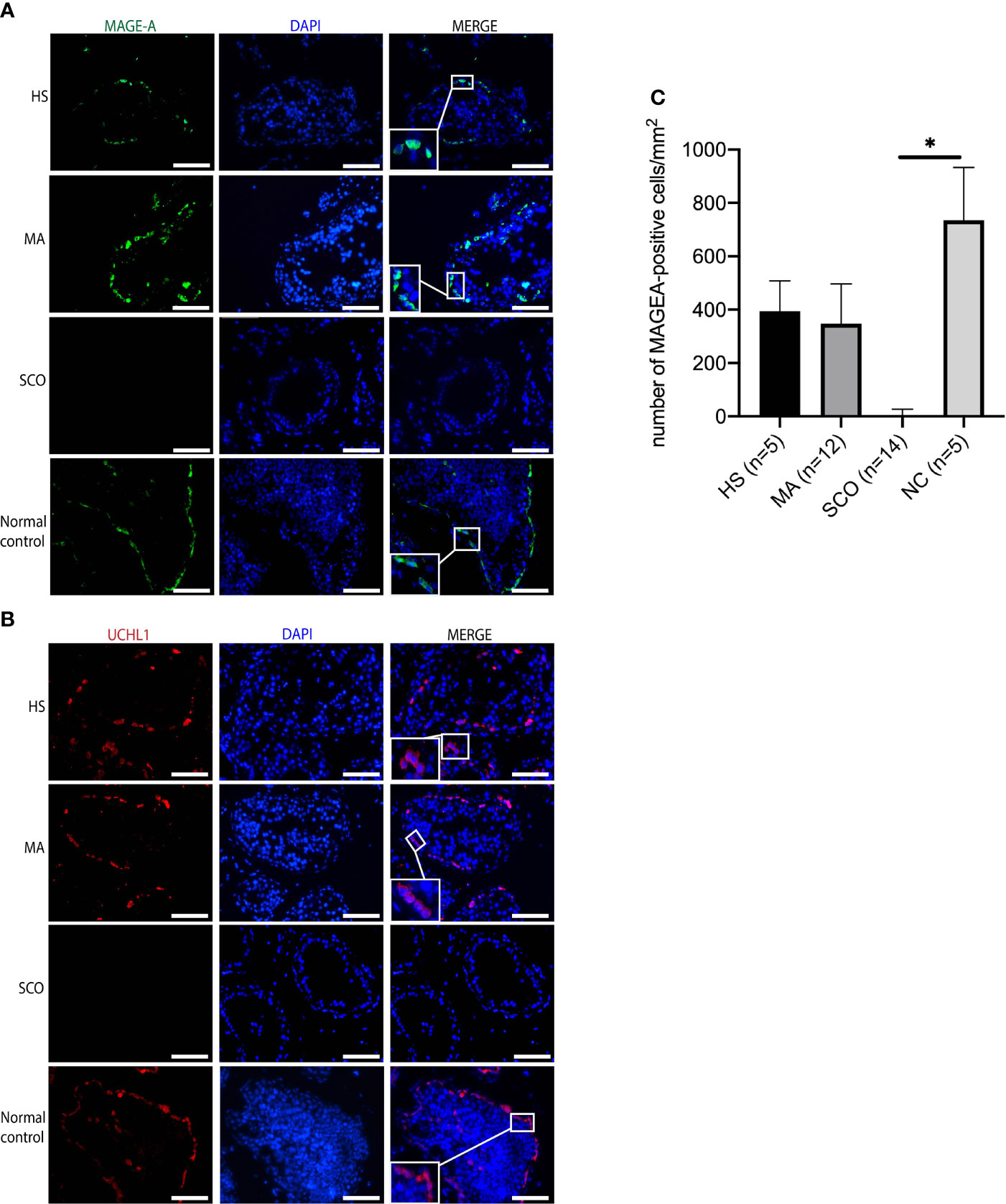
Figure 5 Immunofluorescence staining of germ cell markers in un-dilated seminiferous tubules from HS, MA, SCO, and NC samples. (A) MAGE-A (green) for germ cells, (B) UCHL1 (red) for germ cells, DAPI (blue) for nuclear staining, Scale bar: 100 μm. (C) The number of MAGE-A-positive cells per mm2 was counted based on ten tubules of each biopsy. Asterisk indicated significant difference between SCO and normal control group (*p < 0.05).
qPCR Analysis of Niche Related Cells
The mRNA expression of DEAD-box helicase 4 (DDX4, also named VASA) germ cell-specific gene was significantly decreased in HS, MA, SCO compared to normal control group (P=0.006, P=0.004, P=0.004, respectively) (Figure 6). In SCO, the mRNA expression of MAGE-A4 was significantly downregulated (P=0.004) (Figure 6). The mRNA expression of AMH (indicating immature Sertoli cells) was significantly increased in MA and SCO compared to normal control group (P=0.001, P =0.0002, respectively) (Figure 6). Contrary to the AMH, the mRNA expression of androgen receptor (AR) (indicating mature Sertoli cell) showed no difference in the three types of NOA samples compared to the normal group (Figure 6). The mRNA expression of GDNF was significantly increased in MA (P=0.0003) (Figure 6). The mRNA expression of BMP4 showed a significant increase in HS, MA, and SCO (P=0.02, P=0.0005, P=0.02, respectively) (Figure 6).
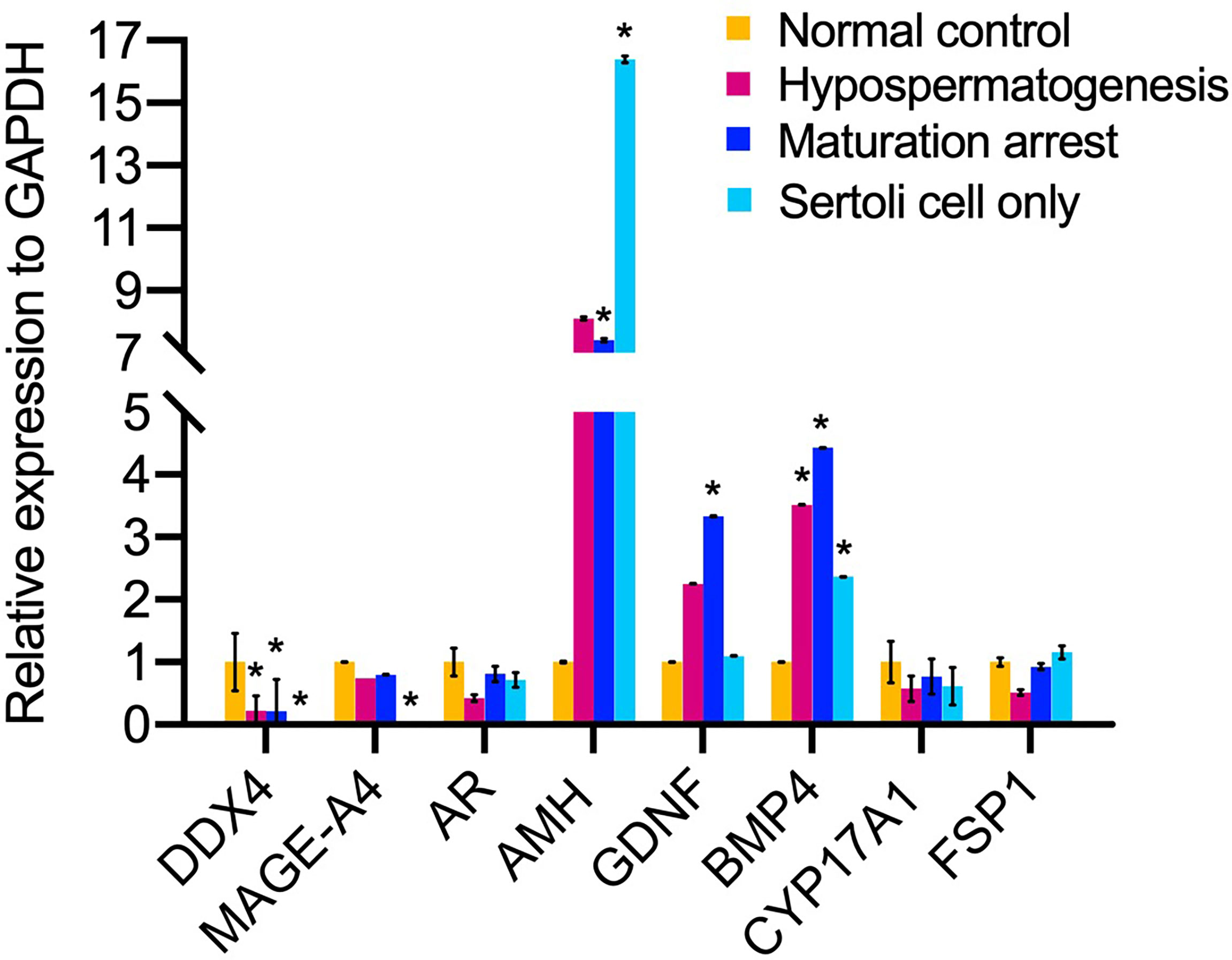
Figure 6 The mRNA expression analysis of niche related cells in un-dilated seminiferous tubules from non-obstructive azoospermia patients (NOA) and healthy adult men. The relative mRNA expression of germ cell DDX4, MAGE-A4, and somatic cell AMH, AR, GDNF, BMP4, CYP17A1, fibroblast-specific protein 1 (FSP1) to internal control GAPDH. Kruskal-Wallis test, *p < 0.05.
Correlation Between mRNA Expression and Serum FSH
In MA, serum FSH showed a significant negative correlation with mRNA expression of CYP17A1 (r=-0.55, P=0.04) (Table 2). No other correlations were found between the remaining genes and hormone values.
Discussion
This study demonstrated pronounced testicular differences within the un-dilated seminiferous tubules between different types of NOA patients and normal control group. The maturation state of Sertoli cells, the number of germ cells, and the thickness of tubule wall were distinct in NOA subtypes. Collectively, the microenvironments within the un-dilated tubules are different in different subtypes of NOA patients.
The maturation state of Sertoli cells was evaluated by AMH and AR expression on both mRNA and protein level. The higher AMH mRNA expression and more tubules with AMH expression in all three NOA subtypes compared to that in the normal group suggests that there were more immature Sertoli cells within the un-dilated tubules from all three NOA subtypes. Earlier studies also showed that immature Sertoli cells were observed in the testis from infertile adult men (32–34). After a higher dilution of AMH antibody employed, we found that there was a threshold level of detection and variable AMH expression in the normal control group. The results are consistent with a previous report that showed both AMH positive staining within the seminiferous tubules of patients with Sertoli-cell-only syndrome (SCOS) and in men with normal spermatogenesis, but staining intensity was stronger in SCOS than in normal group (28). Furthermore, in adult men, AMH is secreted in both serum and seminal plasma (35). It was reported that the seminal AMH concentration was variable ranging from undetectable to a high level (36) suggesting a Sertoli cell secretion. Collectively, this argues for a mild AMH expression in tubules from normal fertile men. In HS and MA, the number of AR-positive mature Sertoli cells appeared to be reduced. This tendency was also shown in AR mRNA expression level. In SCO, the number of SOX9- and AR-positive cells was slightly higher than the normal group, but no significant differences were found, and the AR mRNA expression showed a decreased tendency. Thus, there are both mature and immature Sertoli cells in SCO patients, to what extend both were increased needs to be addressed in a future study. The increased expression of AMH in Sertoli cells may reflect a maturation failure of Sertoli cells in connection with puberty (37, 38) or alternatively de-differentiation of mature Sertoli cells to acquire a more immature state (34). However, the current study is unable to distinguish between maturation failure and de-differentiation of the Sertoli cells.
It has been reported in mice that the overexpression of GDNF showed accumulation of undifferentiated spermatogonia (14) and inhibiting GDNF signaling could promote differentiation of SSC (39). We found GDNF mRNA overexpressing in MA suggesting GDNF could contribute to the maturation failure in MA. It has been reported that FSH induces the GDNF expression (40) and the higher FSH values (i.e. 20.6 IU/L in MA NOA subgroup as compared to 1.5-12.4 IU/L in the normal group) could explain the observed higher expression of GDNF. All three subtypes of NOA showed overexpression of BMP4 mRNA confirming a previous study that showed BMP4 overexpression in MA-NOA and SCO-NOA at the protein level in relation to control group (41). In contrast, mRNA expression of BMP4 was reported lower in SCO (42). This discrepancy may be due to mixing the control group with both tissues from men with hypospermatogenesis and normal spermatogenesis.
The germ cell status was evaluated by using qPCR and immunofluorescence. The mRNA expression of DDX4 and MAGE-A4 was significantly reduced in SCO and exhibited a decreased tendency in HS and MA. This is consistent with other studies that reported a reduced germ-cell niche in HS and MA from infertile men (43–45). The number of MAGE-A-positive germ cells was slightly reduced in HS and MA while absent in SCO. Similarly, the germ cell specific UCHL1 expression was absent in SCO. The attenuated germ cell numbers may either be related to meiotic defects (46, 47) and/or impairment and immaturity of Sertoli cells being unable to support full germ cell maturation.
The presence of histone H2AX phosphorylation (γH2AX) was used to identify germ cells in the prophase of the first meiotic division (48), but Sertoli cells expressing DNA damage response also become stained (25). H2AX is a histone variant that belongs to the H2A family and prevent genome instability and cancer (49–51), while the phosphorylated form γH2AX is regarded as a robust marker of DNA double-strand breaks (DSBs) (52, 53). We found that the γH2AX expression was different between three types of NOA patients. There were more Sertoli cells expressing γH2AX in SCO than in HS and MA biopsies. The accumulation of γH2AX positive staining in Sertoli cells demonstrated that the Sertoli cells may be undergoing accelerated degradation.
The present study suggested that Sertoli cells in un-dilated seminiferous tubules of NOA patients were immature and expressed an increased DNA damage compared to normal controls. In MA, few Sertoli cells appeared to have DNA damages response. In SCO, many γH2AX-positive Sertoli cells may undergo DNA damage response.
Collectively, the present study suggests that un-dilated seminiferous tubules from three subtypes of NOA patients show a different expression of cell specific markers that most likely reflect their compromised ability to sustain spermatogenesis or alternatively that compromised germ cells influence Sertoli cell function. However, both HS and MA subtype of NOA patients demonstrate, in un-dilated seminiferous tubules, the quantitative presence of germ cell numbers approaches that of normal testis in some instances. It may therefore be envisioned that these germ cells could be matured to haploid germ cells and used in connection with ART. This will require the development of an in vitro culture system providing a proper environment, for instance by co-culture with spent media from cultures of mature normal Sertoli cells. Alternatively, testis tissue from men with NOA could be cultured together with mature Sertoli cells from a normal testis without direct cell contact between the NOA tissue and the supplied normal Sertoli cells. Alternatively, or in combination, growth factors and hormones known to advance meiosis may be used to advance meiosis in cultures of testis tissue from NOA patients in whom sperm retrieval was unsuccessful (54, 55). The fact that some of these men with NOA actually present with a few dilated areas of seminiferous tubules with spermatogenesis suggests that it is possible to define conditions of sufficient quality to advance meiosis to the haploid state.
The thicker tubule wall of seminiferous tubules in NOA patients with SCO may affect the contractility of the tubules and the propulsion of the tubular contents to the rete testis.
A limitation of our study is the relatively small sample size. More samples are necessary in the future for further exploration of mechanisms behind NOA.
In conclusion, this study provides insights into understanding the un-dilated (atrophic) tubules which constitute a major part of seminiferous tubules of NOA patients. Improvement of Sertoli cell function either during in vitro culture or by co-culture with Sertoli cells from fertile men may constitute strategies for fertility restoration in patients with different types of NOA that fail surgical sperm retrieval. The impairment and immaturity of Sertoli cells and germ-cell loss are likely to contribute to the impaired spermatogenesis.
Data Availability Statement
The original contributions presented in the study are included in the article/Supplementary Material. Further inquiries can be directed to the corresponding author.
Ethics Statement
The studies involving human participants were reviewed and approved by the Regional Ethical committee of the Capital Region of Denmark (H-16033784) and the Region of Southern Denmark (S-20200088). The patients/participants provided their written informed consent to participate in this study.
Author Contributions
CJ, DW, LM, AG, NJ, MF, DO, LD, JF, CA, JS conceived and designed the experiments. CJ collected testis biopsies for experimental use. DW performed the experiments. CJ, DW, LM, SH, SP, EN, CA performed data analysis and interpretation. DW wrote the manuscript. All authors contributed to the article and approved the submitted version.
Funding
This article is part of the ReproUnion collaborative study, co-financed by EU Interreg ÖKS, Capital Region of Denmark, Region Skåne and Ferring Pharmaceuticals. Further, the support from Vissing Fonden (519140 AHO/PPT) and the Danish Child Cancer Foundation (2021-7395) is greatly acknowledged.
Conflict of Interest
The authors declare that the research was conducted in the absence of any commercial or financial relationships that could be construed as a potential conflict of interest.
Publisher’s Note
All claims expressed in this article are solely those of the authors and do not necessarily represent those of their affiliated organizations, or those of the publisher, the editors and the reviewers. Any product that may be evaluated in this article, or claim that may be made by its manufacturer, is not guaranteed or endorsed by the publisher.
Supplementary Material
The Supplementary Material for this article can be found online at: https://www.frontiersin.org/articles/10.3389/fendo.2022.825904/full#supplementary-material
References
1. Kasak L, Laan M. Monogenic Causes of non-Obstructive Azoospermia: Challenges, Established Knowledge, Limitations and Perspectives. Hum Genet (2021) 140(1):135–54. doi: 10.1007/s00439-020-02112-y
2. Lee JY, Dada R, Sabanegh E, Carpi A, Agarwal A. Role of Genetics in Azoospermia. Urology (2011) 77(3):598–601. doi: 10.1016/j.urology.2010.10.001
3. Su LM, Palermo GD, Goldstein M, Veeck LL, Rosenwaks Z, Schlegel PN. Testicular Sperm Extraction With Intracytoplasmic Sperm Injection for Nonobstructive Azoospermia: Testicular Histology can Predict Success of Sperm Retrieval. J Urol (1999) 161(1):112–6. doi: 10.1097/00005392-199901000-00038
4. Salas-Huetos A, Tuttelmann F, Wyrwoll MJ, Kliesch S, Lopes AM, Goncalves J, et al. Disruption of Human Meiotic Telomere Complex Genes TERB1, TERB2 and MAJIN in Men With Non-Obstructive Azoospermia. Hum Genet (2021) 140(1):217–27. doi: 10.1007/s00439-020-02236-1
5. Caroppo E, Colpi EM, D’Amato G, Gazzano G, Colpi GM. Prediction Model for Testis Histology in Men With Non-Obstructive Azoospermia: Evidence for a Limited Predictive Role of Serum Follicle-Stimulating Hormone. J Assist Reprod Genet (2019) 36(12):2575–82. doi: 10.1007/s10815-019-01613-8
6. Ezeh UI, Moore HD, Cooke ID. A Prospective Study of Multiple Needle Biopsies Versus a Single Open Biopsy for Testicular Sperm Extraction in Men With non-Obstructive Azoospermia. Hum Reprod (1998) 13(11):3075–80. doi: 10.1093/humrep/13.11.3075
7. Tournaye H, Krausz C, Oates RD. Concepts in Diagnosis and Therapy for Male Reproductive Impairment. Lancet Diabetes Endocrinol (2017) 5(7):554–64. doi: 10.1016/S2213-8587(16)30043-2
8. Gassei K, Orwig KE. Experimental Methods to Preserve Male Fertility and Treat Male Factor Infertility. Fertil Steril (2016) 105(2):256–66. doi: 10.1016/j.fertnstert.2015.12.020
9. Gul M, Hildorf S, Dong L, Thorup J, Hoffmann ER, Jensen CFS, et al. Review of Injection Techniques for Spermatogonial Stem Cell Transplantation. Hum Reprod Update (2020) 26(3):368–91. doi: 10.1093/humupd/dmaa003
10. Griswold MD. The Central Role of Sertoli Cells in Spermatogenesis. Semin Cell Dev Biol (1998) 9(4):411–6. doi: 10.1006/scdb.1998.0203
11. de Rooij DG. The Spermatogonial Stem Cell Niche. Microsc Res Tech (2009) 72(8):580–5. doi: 10.1002/jemt.20699
12. Sansone A, Kliesch S, Isidori AM, Schlatt S. AMH and INSL3 in Testicular and Extragonadal Pathophysiology: What Do We Know? Andrology (2019) 7(2):131–8. doi: 10.1111/andr.12597
13. Singh D, Paduch DA, Schlegel PN, Orwig KE, Mielnik A, Bolyakov A, et al. The Production of Glial Cell Line-Derived Neurotrophic Factor by Human Sertoli Cells Is Substantially Reduced in Sertoli Cell-Only Testes. Hum Reprod (2017) 32(5):1108–17. doi: 10.1093/humrep/dex061
14. Meng X, Lindahl M, Hyvonen ME, Parvinen M, de Rooij DG, Hess MW, et al. Regulation of Cell Fate Decision of Undifferentiated Spermatogonia by GDNF. Science (2000) 287(5457):1489–93. doi: 10.1126/science.287.5457.1489
15. Nagano M, Ryu BY, Brinster CJ, Avarbock MR, Brinster RL. Maintenance of Mouse Male Germ Line Stem Cells In Vitro. Biol Reprod (2003) 68(6):2207–14. doi: 10.1095/biolreprod.102.014050
16. Ma M, Yang S, Zhang Z, Li P, Gong Y, Liu L, et al. Sertoli Cells From Non-Obstructive Azoospermia and Obstructive Azoospermia Patients Show Distinct Morphology, Raman Spectrum and Biochemical Phenotype. Hum Reprod (2013) 28(7):1863–73. doi: 10.1093/humrep/det068
17. Welsh M, Moffat L, Belling K, de Franca LR, Segatelli TM, Saunders PT, et al. Androgen Receptor Signalling in Peritubular Myoid Cells Is Essential for Normal Differentiation and Function of Adult Leydig Cells. Int J Androl (2012) 35(1):25–40. doi: 10.1111/j.1365-2605.2011.01150.x
18. Konrad L, Albrecht M, Renneberg H, Aumuller G. Transforming Growth Factor-Beta2 Mediates Mesenchymal-Epithelial Interactions of Testicular Somatic Cells. Endocrinology (2000) 141(10):3679–86. doi: 10.1210/endo.141.10.7728
19. Zirkin BR, Papadopoulos V. Leydig Cells: Formation, Function, and Regulation. Biol Reprod (2018) 99(1):101–11. doi: 10.1093/biolre/ioy059
20. Kvist K, Thorup J, Byskov AG, Hoyer PE, Mollgard K, Yding Andersen C. Cryopreservation of Intact Testicular Tissue From Boys With Cryptorchidism. Hum Reprod (2006) 21(2):484–91. doi: 10.1093/humrep/dei331
21. World Health Organization. WHO Laboratory Manual for the Examination and Processing of Human Semen. 5th ed. Geneva: World Health Organization (2010).
22. Jungbluth AA, Busam KJ, Kolb D, Iversen K, Coplan K, Chen YT, et al. Expression of MAGE-Antigens in Normal Tissues and Cancer. Int J Cancer (2000) 85(4):460–5. doi: 10.1002/(SICI)1097-0215(20000215)85:4<460::AID-IJC3>3.0.CO;2-N
23. Wang D, Hildorf SE, Ntemou E, Dong L, Pors SE, Mamsen LS, et al. Characterization and Survival of Human Infant Testicular Cells After Direct Xenotransplantation. Front Endocrinol (2022) 13:853482. doi: 10.3389/fendo.2022.853482
24. Dong L, Kristensen SG, Hildorf S, Gul M, Clasen-Linde E, Fedder J, et al. Propagation of Spermatogonial Stem Cell-Like Cells From Infant Boys. Front Physiol (2019) 10:1155. doi: 10.3389/fphys.2019.01155
25. Wang M, Liu X, Chang G, Chen Y, An G, Yan L, et al. Single-Cell RNA Sequencing Analysis Reveals Sequential Cell Fate Transition During Human Spermatogenesis. Cell Stem Cell (2018) 23(4):599–614.e4. doi: 10.1016/j.stem.2018.08.007
26. Wang Y, Wang H, Zhang Y, Du Z, Si W, Fan S, et al. Reprogramming of Meiotic Chromatin Architecture During Spermatogenesis. Mol Cell (2019) 73(3):547–61.e6. doi: 10.1016/j.molcel.2018.11.019
27. Aslani F, Sebastian T, Keidel M, Frohlich S, Elsasser HP, Schuppe HC, et al. Resistance to Apoptosis and Autophagy Leads to Enhanced Survival in Sertoli Cells. Mol Hum Reprod (2017) 23(6):370–80. doi: 10.1093/molehr/gax022
28. Lan KC, Chen YT, Chang C, Chang YC, Lin HJ, Huang KE, et al. Up-Regulation of SOX9 in Sertoli Cells From Testiculopathic Patients Accounts for Increasing Anti-Mullerian Hormone Expression via Impaired Androgen Receptor Signaling. PloS One (2013) 8(10):e76303. doi: 10.1371/journal.pone.0076303
29. Hau RK, Miller SR, Wright SH, Cherrington NJ. Generation of a hTERT-Immortalized Human Sertoli Cell Model to Study Transporter Dynamics at the Blood-Testis Barrier. Pharmaceutics (2020) 12(11):1005. doi: 10.3390/pharmaceutics12111005
30. Marini M, Rosa I, Guasti D, Gacci M, Sgambati E, Ibba-Manneschi L, et al. Reappraising the Microscopic Anatomy of Human Testis: Identification of Telocyte Networks in the Peritubular and Intertubular Stromal Space. Sci Rep (2018) 8(1):14780. doi: 10.1038/s41598-018-33126-2
31. Yuan Y, Li L, Cheng Q, Diao F, Zeng Q, Yang X, et al. In Vitro Testicular Organogenesis From Human Fetal Gonads Produces Fertilization-Competent Spermatids. Cell Res (2020) 30(3):244–55. doi: 10.1038/s41422-020-0283-z
32. Bar-Shira Maymon B, Paz G, Elliott DJ, Hammel I, Kleiman SE, Yogev L, et al. Maturation Phenotype of Sertoli Cells in Testicular Biopsies of Azoospermic Men. Hum Reprod (2000) 15(7):1537–42. doi: 10.1093/humrep/15.7.1537
33. Bar-Shira Maymon B, Yavetz H, Yogev L, Kleiman SE, Lifschitz-Mercer B, Schreiber L, et al. Detection of Calretinin Expression in Abnormal Immature Sertoli Cells in non-Obstructive Azoospermia. Acta Histochem (2005) 107(2):105–12. doi: 10.1016/j.acthis.2005.02.002
34. Steger K, Rey R, Louis F, Kliesch S, Behre HM, Nieschlag E, et al. Reversion of the Differentiated Phenotype and Maturation Block in Sertoli Cells in Pathological Human Testis. Hum Reprod (1999) 14(1):136–43. doi: 10.1093/humrep/14.1.136
35. Andersen JM, Herning H, Witczak O, Haugen TB. Anti-Mullerian Hormone in Seminal Plasma and Serum: Association With Sperm Count and Sperm Motility. Hum Reprod (2016) 31(8):1662–7. doi: 10.1093/humrep/dew121
36. Fenichel P, Rey R, Poggioli S, Donzeau M, Chevallier D, Pointis G. Anti-Mullerian Hormone as a Seminal Marker for Spermatogenesis in non-Obstructive Azoospermia. Hum Reprod (1999) 14(8):2020–4. doi: 10.1093/humrep/14.8.2020
37. Rajpert-De Meyts E, Jorgensen N, Graem N, Muller J, Cate RL, Skakkebaek NE. Expression of Anti-Mullerian Hormone During Normal and Pathological Gonadal Development: Association With Differentiation of Sertoli and Granulosa Cells. J Clin Endocrinol Metab (1999) 84(10):3836–44. doi: 10.1210/jcem.84.10.6047
38. Sharpe RM, McKinnell C, Kivlin C, Fisher JS. Proliferation and Functional Maturation of Sertoli Cells, and Their Relevance to Disorders of Testis Function in Adulthood. Reproduction (2003) 125(6):769–84. doi: 10.1530/rep.0.1250769
39. Parker N, Falk H, Singh D, Fidaleo A, Smith B, Lopez MS, et al. Responses to Glial Cell Line-Derived Neurotrophic Factor Change in Mice as Spermatogonial Stem Cells Form Progenitor Spermatogonia Which Replicate and Give Rise to More Differentiated Progeny. Biol Reprod (2014) 91(4):92. doi: 10.1095/biolreprod.114.119099
40. Chen H, Mruk D, Xiao X, Cheng CY. Human Spermatogenesis and its Regulation. In: Male hypogonadism. Cham: Humana Press, (2017). p. 49–72.
41. Hai Y, Sun M, Niu M, Yuan Q, Guo Y, Li Z, et al. BMP4 Promotes Human Sertoli Cell Proliferation via Smad1/5 and ID2/3 Pathway and its Abnormality is Associated With Azoospermia. Discov Med (2015) 19(105):311–25.
42. Paduch DA, Hilz S, Grimson A, Schlegel PN, Jedlicka AE, Wright WW. Aberrant Gene Expression by Sertoli Cells in Infertile Men With Sertoli Cell-Only Syndrome. PloS One (2019) 14(5):e0216586. doi: 10.1371/journal.pone.0216586
43. Guarch R, Pesce C, Puras A, Lazaro J. A Quantitative Approach to the Classification of Hypospermatogenesis in Testicular Biopsies for Infertility. Hum Pathol (1992) 23(9):1032–7. doi: 10.1016/0046-8177(92)90265-5
44. Soderstrom KO, Suominen J. Human Hypospermatogenesis. Histopathology and Ultrastructure. Arch Pathol Lab Med (1982) 106(5):231–4.
45. Hentrich A, Wolter M, Szardening-Kirchner C, Luers GH, Bergmann M, Kliesch S, et al. Reduced Numbers of Sertoli, Germ, and Spermatogonial Stem Cells in Impaired Spermatogenesis. Mod Pathol (2011) 24(10):1380–9. doi: 10.1038/modpathol.2011.97
46. Takagi S, Itoh N, Kimura M, Sasao T, Tsukamoto T. Spermatogonial Proliferation and Apoptosis in Hypospermatogenesis Associated With Nonobstructive Azoospermia. Fertil Steril (2001) 76(5):901–7. doi: 10.1016/s0015-0282(01)02732-7
47. Johnson L, Thompson DL Jr., Varner DD. Role of Sertoli Cell Number and Function on Regulation of Spermatogenesis. Anim Reprod Sci (2008) 105(1-2):23–51. doi: 10.1016/j.anireprosci.2007.11.029
48. Mahadevaiah SK, Turner JM, Baudat F, Rogakou EP, de Boer P, Blanco-Rodriguez J, et al. Recombinational DNA Double-Strand Breaks in Mice Precede Synapsis. Nat Genet (2001) 27(3):271–6. doi: 10.1038/85830
49. Georgoulis A, Vorgias CE, Chrousos GP, Rogakou EP. Genome Instability and Gammah2ax. Int J Mol Sci (2017) 18(9):1979. doi: 10.3390/ijms18091979
50. Bassing CH, Suh H, Ferguson DO, Chua KF, Manis J, Eckersdorff M, et al. Histone H2AX: A Dosage-Dependent Suppressor of Oncogenic Translocations and Tumors. Cell (2003) 114(3):359–70. doi: 10.1016/s0092-8674(03)00566-x
51. Gorgoulis VG, Vassiliou LV, Karakaidos P, Zacharatos P, Kotsinas A, Liloglou T, et al. Activation of the DNA Damage Checkpoint and Genomic Instability in Human Precancerous Lesions. Nature (2005) 434(7035):907–13. doi: 10.1038/nature03485
52. Rogakou EP, Nieves-Neira W, Boon C, Pommier Y, Bonner WM. Initiation of DNA Fragmentation During Apoptosis Induces Phosphorylation of H2AX Histone at Serine 139. J Biol Chem (2000) 275(13):9390–5. doi: 10.1074/jbc.275.13.9390
53. Pilch DR, Sedelnikova OA, Redon C, Celeste A, Nussenzweig A, Bonner WM. Characteristics of Gamma-H2AX Foci at DNA Double-Strand Breaks Sites. Biochem Cell Biol (2003) 81(3):123–9. doi: 10.1139/o03-042
54. Lee DR, Kim KS, Yang YH, Oh HS, Lee SH, Chung TG, et al. Isolation of Male Germ Stem Cell-Like Cells From Testicular Tissue of Non-Obstructive Azoospermic Patients and Differentiation Into Haploid Male Germ Cells In Vitro. Hum Reprod (2006) 21(2):471–6. doi: 10.1093/humrep/dei319
Keywords: non-obstructive azoospermia, un-dilated tubules, hypospermatogenesis, maturation arrest, Sertoli cell only, male infertility
Citation: Jensen CFS, Wang D, Mamsen LS, Giwercman A, Jørgensen N, Fode M, Ohl D, Dong L, Hildorf SE, Pors SE, Fedder J, Ntemou E, Andersen CY and Sønksen J (2022) Sertoli and Germ Cells Within Atrophic Seminiferous Tubules of Men With Non-Obstructive Azoospermia. Front. Endocrinol. 13:825904. doi: 10.3389/fendo.2022.825904
Received: 30 November 2021; Accepted: 06 April 2022;
Published: 02 June 2022.
Edited by:
Jan-Bernd Stukenborg, Karolinska Institutet (KI), SwedenReviewed by:
Jingtao Guo, The University of Utah, United StatesArtur Mayerhofer, Ludwig Maximilian University of Munich, Germany
Copyright © 2022 Jensen, Wang, Mamsen, Giwercman, Jørgensen, Fode, Ohl, Dong, Hildorf, Pors, Fedder, Ntemou, Andersen and Sønksen. This is an open-access article distributed under the terms of the Creative Commons Attribution License (CC BY). The use, distribution or reproduction in other forums is permitted, provided the original author(s) and the copyright owner(s) are credited and that the original publication in this journal is cited, in accordance with accepted academic practice. No use, distribution or reproduction is permitted which does not comply with these terms.
*Correspondence: Elissavet Ntemou, ZWxpcy5udGVtb3VAZ21haWwuY29t
†These authors have contributed equally to this work and share first authorship