- 1Department of Endocrinology, The First Medical Center, Chinese PLA General Hospital, Beijing, China
- 2Department of Radiology, Peking University Cancer Hospital, Beijing, China
- 3Department of Endocrinology, Beijing Tongren Hospital, Capital Medical University, Beijing, China
- 4Department of Endocrinology, The Sixth Medical Center of PLA General Hospital, Beijing, China
- 5Department of Endocrinology Guangdong Provincial People’s Hospital, Guangdong Academy of Medical Sciences, Guangzhou, China
Background: The changes of endothelial function in type 2 diabetes mellitus (T2DM) patients are closely associated with the development of cardiovascular disease (CVD). However, it is still unclear whether commonly used antidiabetic drugs can improve endothelial function. Flow-mediated dilation (FMD) is a noninvasive tool for evaluating endothelial function, which typically examines changes in the brachial artery diameter in response to ischemia using ultrasound. We performed a network meta-analysis (NMA) to explore the associations between changes in endothelial function and antidiabetic drugs by evaluating FMD in T2DM patients.
Methods: We systematically searched several electronic databases for randomized controlled trials (RCTs) published from inception until January 25, 2022 with no language restriction. The primary outcome was FMD change in all studies, and we performed subgroup analysis in T2DM patients without CVD. NMA was performed to calculate the mean differences (MDs) with 95% confidence intervals (CIs).
Results: From the 1,987 candidate articles identified in the initial search, 30 RCTs were eventually included in the analysis. In all studies, glucagon-like peptide-1 receptor (GLP-1R) agonists [MD = 3.70 (1.39–5.97)], TZD [MD = 1.96 (0.006–3.89)] produced improvement of FMD change compared to lifestyle intervention. GLP-1R agonists [MD = 3.33 (1.36–5.34) and MD = 3.30 (1.21–5.43)] showed significantly greater improvements in FMD change in pairwise comparisons with sulfonylureas and placebo. SGLT-2i also showed efficacy compared to sulfonylureas (MD = 1.89, 95% CI, 0.10, 3.75). In studies of T2DM patients without CVD, GLP-1R agonists [MD = 3.53 (1.24–5.76)], and TZD [MD = 2.30 (0.27–3.24)] produced improvements in FMD change compared to lifestyle treatment. GLP-1R agonists [MD = 3.25 (1.13–5.40), and MD = 3.85 (1.68–6.13)] showed significantly greater improvements in pairwise comparisons with sulfonylureas, and placebo.
Conclusion: In T2DM patients, both GLP-1R agonists, SGLT-2i and TZD have favorable effects to improve endothelial function in T2DM patients. In T2DM patients without CVD, GLP-1R agonists had a greater effect to improve endothelial function than sulfonylureas. These suggested that GLP-1R agonists are associated with significantly improved endothelial function in T2DM patients.
Introduction
Cardiovascular diseases (CVDs) remain a leading cause of death and disability in patients with type 2 diabetes mellitus (T2DM) (1). In addition to their glucose-lowering effects, some antidiabetic drugs may improve cardiovascular outcomes. For example, the Liraglutide Effect and Action in Diabetes: Evaluation of Cardiovascular Outcome Results (LEADER) trial showed that glucagon-like peptide-1 receptor (GLP-1R) agonists significantly reduce major adverse cardiovascular events, such as cardiovascular death, nonfatal stroke, and nonfatal myocardial infarction (2). Other large clinical trials, such as the Empagliflozin Outcome Trial in Patients With Chronic Heart Failure With Reduced Ejection Fraction (EMPEROR-Reduced) (3) and the Dapaglifozin And Prevention of Adverse-outcomes in Heart Failure trial (DAPA-HF) (4), also showed that a newer class of antidiabetic drug, sodium glucose co-transporter-2 (SGLT-2) inhibitors, reduce the risk of hospitalization for heart failure or cardiovascular death. These two classes of antihyperglycemic agents are already recommended by the American Diabetes Association for the management of diabetes in patients with CVD or kidney disease (5).
However, the cardiovascular effects of different antidiabetic drugs are still controversial. Dipeptidyl peptidase-4 (DPP-4) inhibitors are another class of new oral antidiabetic drug that have been shown to have positive cardiac and vascular effects in preliminary studies. But the Cardiovascular and Renal Microvascular Outcome Study With Linagliptin (CARMELINA) trial, a placebo-controlled trial of the DPP-4 inhibitor, linagliptin, demonstrated non-inferiority and failed to prove cardiovascular benefits in T2DM patients with high cardiovascular risk over several years of observation (6). Similarly, the Acarbose Cardiovascular Evaluation (ACE) trial, which included 6,522 individuals with coronary heart disease and impaired glucose tolerance, showed that the α-glucosidase inhibitor acarbose was neutral with regard to major adverse cardiovascular events (7). Furthermore, a meta-analysis that included 1,325,446 diabetes patients suggested that another class of widely used insulin sensitizing drug, sulfonylureas, was associated with a significantly increased risk for cardiovascular death compared to other oral drugs for diabetes (8). In view of the inconsistent cardiovascular effects of antidiabetic drugs, we speculated that the effects of common antidiabetic drugs on vascular endothelial function in T2DM patients remained differences.
Endothelial dysfunction is closely associated with the development of CVDs involving inflammatory reactions and atherosclerotic progression in T2DM patients (9). The endothelium is highly responsive to various hemodynamic stimuli, namely, shear stress, circumferential stain, and wall strain (10). Endothelial function can be assessed non-invasively using the flow-mediated dilation (FMD) technique. FMD represents an endothelium-dependent, noninvasive tool for evaluating endothelial function, which typically examines changes in the brachial artery diameter in response to ischemia using ultrasound. A higher FMD reflects a better state of vascular elasticity (11). Several studies have demonstrated the prognostic value of brachial artery FMD for cardiovascular events: In the Multi-Ethnic Study of Atherosclerosis (MESA) trial, FMD was an independent predictor of cardiovascular events, and this inverse association remained significant after adjusting for multiple CVD risk factors (12). The Flow-Mediated Dilation Japan (FMD-J) study, a multicenter study that included 462 individuals from 22 university hospitals and affiliated clinics in Japan, also suggested that the decrease of FMD is closely associated with coronary events in patients with coronary artery disease after 3-year follow-up (13). The expert consensus statement of the European Society of Cardiology recommended FMD for examining the pathophysiology of CVD and possibly identifying subjects at risk for future cardiovascular events (14). However, it remains unclear how antidiabetic drugs affect FMD, as different studies have yielded conflicting results.
Several systematic reviews and meta-analyses have reported the impacts of specific classes of antidiabetic drugs on vascular function (15–17). However, there is still a lack of comprehensive evidence regarding the effects of traditional and newly developed antidiabetic drugs on endothelial function in T2DM patients based on FMD assessment. The utility of traditional pairwise meta-analysis is limited because it cannot evaluate the effects of interventions in head-to-head trials. The network meta-analysis (NMA) has overcome this limitation as it allows the comparison of the effects of two or more interventions through direct and indirect evidence (18). Therefore, we implemented NMA of randomized controlled trials (RCTs) to explore the effects of antidiabetic drugs on endothelial function in T2DM patients by FMD and to summarize the performance of these different drug treatments.
Methods
Data Sources and Searches
Our study adhered to the Network Meta-analysis of the Preferred Reporting Items for Systematic Reviews and Meta Analyses (PRISMA-NMA) and the Cochrane Handbook for Systematic Reviews of Interventions. Two reviewers (JW, HL) initially screened titles and abstracts independently and the full texts of studies were perused to examine the suitability of potentially eligible articles. Any disagreements were resolved by two reviewers through discussion and an experienced professor was invited to judge the final set of standards if necessary. As all analyses were based on previous published studies, no ethical approval or patient consent was required. We searched the PubMed, the Embase, the Cochrane Central Register of Controlled Trials, the ClinicalTrials, the Wan Fang Database, the China National Knowledge Infrastructure Database, the Chinese Biomedical Literature Database, and the Chinese Scientific Journal Database from inception to January 25, 2022 for RCTs. The following Medical Subject Headings (MESH) terms and free text terms combined with Boolean operators were used in the search strategy with no language restriction: “metformin,” “sulphonylureas,” “glinides,” “Thiazolidinedione”, “α-glucosidase inhibitors,” “dipeptidyl-peptidase IV inhibitors,” “GLP-1RAs,” “SGLT-2 inhibitors,” “flow-mediated dilation,” “FMD”, “endothelial function,” “endothelium”, “endothelial dysfunction”, “Hypoglycemic Agents” “type 2 diabetes,” and “randomized controlled trials.” In addition, we conducted a recursive manual search to retrieve full texts of studies from the bibliographies of relevant reports or similar systematic reviews to check for potentially eligible studies that may have been missed in the initial screen. The details of the search strategy are presented in the Supplementary Material. All citations were managed using Endnote X9 software (Thompson ISI Research Soft, Philadelphia, PA).
Study Selection
We included eligible RCTs based on the PICOS criteria, summarized below. Population: T2DM patients were diagnosed using appropriate clinical criteria, such as the American Diabetes Association Guidelines or the WHO-1999 criteria. The patients included in the studies were not limited to those with or without CVD. Intervention: Eight drug classes were included: metformin, sulfonylureas, glinides, thiazolidinedione, α-glucosidase inhibitors, DPP-4 inhibitors, GLP-1R agonists, and SGLT-2 inhibitors. Studies discussing agents that have been withdrawn, such as rosiglitazone, or those that are no longer available in clinical practice were excluded. Comparison: Interventions with eight classes of drug were compared to each other or with placebo and lifestyle intervention. Several studies designed control treatment as diet and (or) exercise without using drugs, we defined them as one class of intervention, lifestyle treatment to analysis (Table 1). Insulin treatment differs in type and dosage in T2DM patients; therefore, insulin intervention studies were also excluded (Figure 1). Outcome: The outcome was endothelial function assessed by FMD change from baseline to post-treatment with percentage (ΔFMD%) as standard of measurement in all studies. Study design: We confined our analysis to RCTs published without year and language restriction. Other studies, such as single-arm studies, were excluded.
Data Extraction and Quality Assessment
Two authors (YW, JW) independently extracted data to collect the relevant data from the included studies by following the Cochrane Consumers and Communication Review Group’s data extraction template, namely, the name of the first author, the publication year, baseline characteristics (intervention, sample size, baseline age, baseline BMI, and baseline HbA1c level) and quality of the RCT. We extracted the mean, standard deviation (mean ± SD) and number of patients of experimental group and control group at baseline and at the last observation to calculate the change of FMD from baseline to post-treatment in each comparison. For effect sizes of FMD change, we present mean differences and 95% confidence intervals. The risk of bias was evaluated using the Cochrane Risk of Bias tool (Supplementary Figure 1). Any discrepancies of data extraction or quality assessment were resolved through discussion with the third author (HL).
Data Synthesis and Analysis
We first implemented a conventional pairwise meta-analysis to analyze the direct evidence from the included studies. Heterogeneity of treatment effects across trials was assessed by I2 statistics. When the P-value was ≥0.1, and I2 was ≤50%, it suggested that there was mild statistical heterogeneity. When the P-value was <0.1, and I2 was >50%, we explored sources of heterogeneity by using subgroup analysis (Supplementary Figure 5). Comparison-adjusted funnel plots were drawn to determine the presence of publication bias. A network plot of each treatment was produced as a summary description to provide all of the available evidence for each treatment (Figure 2).
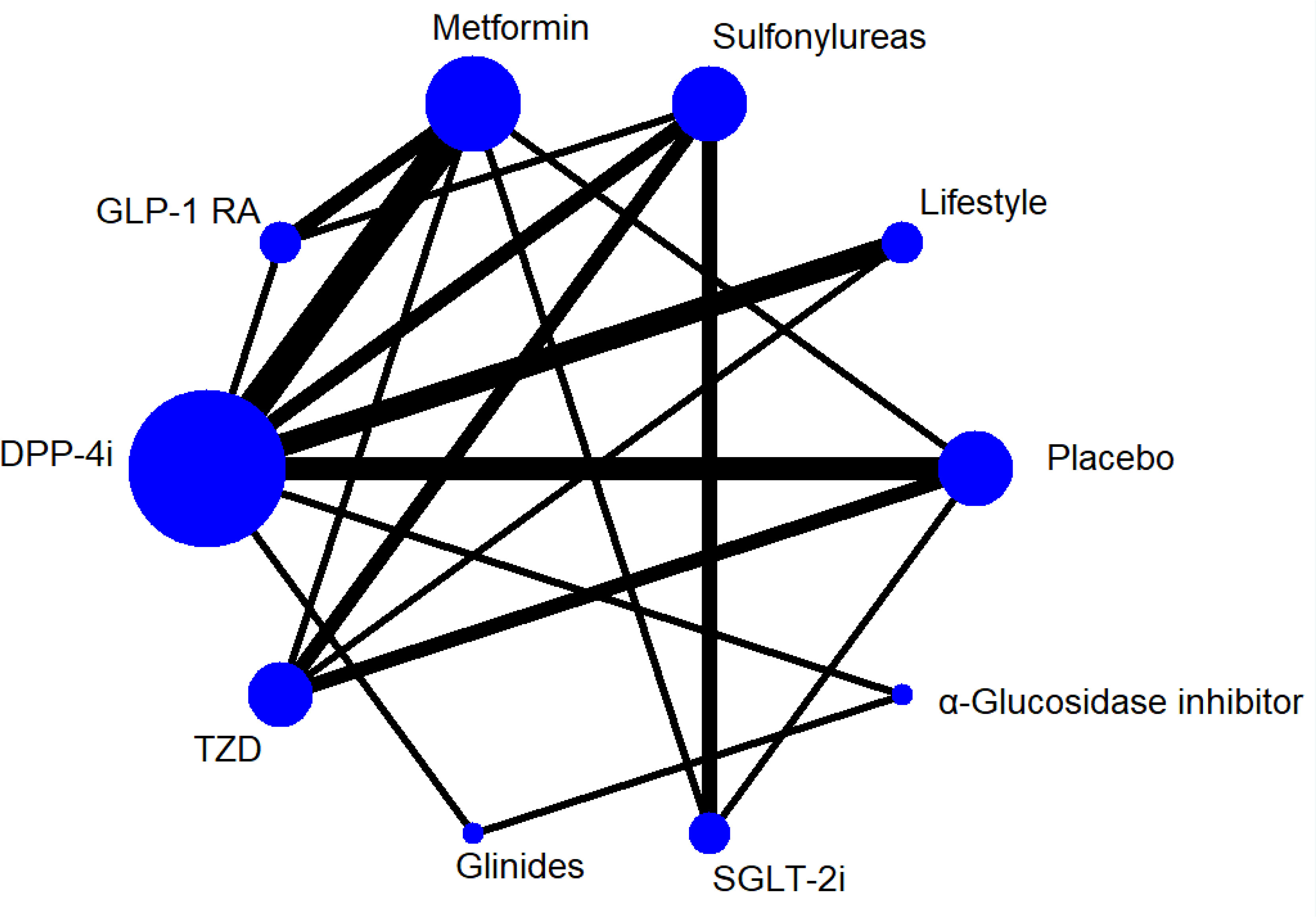
Figure 2 Network Plot for all studies. GLP-1 RA, glucagon-like peptide-1 receptor agonist. SGLT-2i, sodium-glucose co-transporter 2 inhibitor. DPP-4i, dipeptidyl peptidase-4 inhibitor. TZD, Thiazolidinedione. Lifestyle,exercise and/or diet.
As the presence of effect sizes refers to continuous outcome, FMD change from baseline to post-treatment was calculated for each comparison. The 95% confidence interval (CI) and pooled mean difference (MDs) were calculated as measures of estimated uncertainty and pooled effect size, respectively. If the study for which SD of changes from baseline is not available, we use the formula “SD change = [baselineSD2 + finalSD2 − (2 ∗ Corr ∗ baselineSD ∗ final SD)]1/2” to calculate SD change from baseline for experimental intervention and comparator intervention (19). If the mean and SD data to extract were presented in another form by calculating other available values, such as range or interquartile ranges (IQRs) to clarify SD, we use median to be a substitute for mean (19), Range/6 to estimate SD (20), and for estimating SD from IQR, we use formula SD ≈ (Q3−Q1)/1.35 (21).
To ensure sufficient similarity of various treatment comparisons and thus provide valid indirect inferences, two authors (JW, YW) independently evaluated the transitivity assumption by comparing and examining the clinical and methodological characteristics, such as the characteristics of participants, experimental design, and measurement of time frame, to ensure the clinical and methodological characteristics as comparable as possible for each included study before statistical analyses (22). If the study clinical characteristics is uncertain to meet the include criterion, consult three clinical experts to reach a consensus.
Based on the maximum likelihood and Bayesian estimation, the Markov chain Monte Carlo (MCMC) method with prior non-informative distributions was used in our NMA. We used the random-effect model with vague priors for multi-arm trials. Normal prior distributions with a mean of zero and a variance of 10−4 were used for all trial baselines and treatment effects, and uniform prior distributions with a mean of zero and a variance of 5 were used to calculate between-trial standard deviation. The estimation of posterior distribution is via Markov chain Monte Carlo method by using Gibbs Sampling. The model convergence was assessed by trace plots and Brooks–Gelman–Rubin plots. Three parallel Markov chains were used to check for convergence by starting analysis from different initial states, through stimulation to obtain the target distributions (23). A burn-in of 20,000 iterations was conducted to ensure the three chains had converged and the subsequent 80,000 iterations were sampled for the analysis.
The surface under the cumulative ranking curve (SUCRA) was presented as a simple numerical statistic cumulative ranking probability plot summarized to evaluate the change of FMD in each intervention. A higher SUCRA value (up to 1) indicates greater likelihood of the treatment being in the top rank or being highly effective, while lower values (down to zero) indicate that the treatment is worse (24, 25). The global inconsistency was used to explore the consistency of direct and indirect evidence in the network (Supplementary Figure 3), P <0.05 indicated the probability of inconsistency. All analyses were conducted in STATA, version 14.0 (Stata, Corp, College Station, TX, USA) and OpenBUGS, Version 3.2.3 (MRC Biostatistics Unit, Cambridge, UK).
Results
Study Selection
Figure 1 shows the literature selection procedure. The initial search initially identified 1,987 candidate articles including sixteen additional articles that were found from relevant systematic reviews or meta-analyses by hand searching. A total of 1,168 were discarded due to duplication, 1,738 were removed after checking the title and abstract, and 219 were excluded at full-text stage according to our exclude criterion. Thirty articles were included after checking the full text. All records were discarded for various reasons as shown in Figure 1.
The 30 studies finally included in the analysis included 10 interventions; 24 studies were written in English (26–49) and six were written in Chinese (50–55). The studies were divided into the CVD group and non-CVD group according to CVD status of the participants. The CVD group consisted of five studies (31, 32, 37, 42, 45) in which participants were all diagnosed with CVD (hypertension, coronary atherosclerosis, or carotid atherosclerotic disease). The non-CVD group consisted of 25 studies (27–30, 33–36, 38–41, 43, 44, 46–49), none of whom were diagnosed with CVD. The participant characteristics and interventions in the studies are summarized in Table 1.
ROB Quality Assessment
We evaluated the quality of the included studies based on the Cochrane Collaboration tool for assessing the risk of bias (ROB). Ten studies were designed as open-label (28, 30, 31, 37, 39–43) or non-blind (29). Two studies (31, 42) were judged to have high risk of selective reporting bias because their data were extracted by transforming the original data manually. The details of ROB quality assessment for each RCT are shown in Supplementary Figure 1.
Primary Outcome
The primary outcome was FMD of all treatments in all 30 trials. Figure 2 shows the network plot of interventions for all 30 trials. In terms of the outcome of FMD, two classes of drug showed significant benefits with regard to improving FMD in all T2DM patients compared to lifestyle treatment: GLP-1R agonists (MD = 3.70, 95% CI, 1.39, 5.97), TZD (MD = 1.96, 95% CI, 0.006, 3.89). In addition, GLP-1R agonists showed efficacy compared to sulfonylureas (MD = 3.33, 95% CI, 1.36, 5.34) and placebo (MD = 3.30, 95% CI, 1.21, 5.43), and SGLT-2i also showed efficacy compared to sulfonylureas (MD = 1.89, 95% CI, 0.10, 3.75) (Table 2).
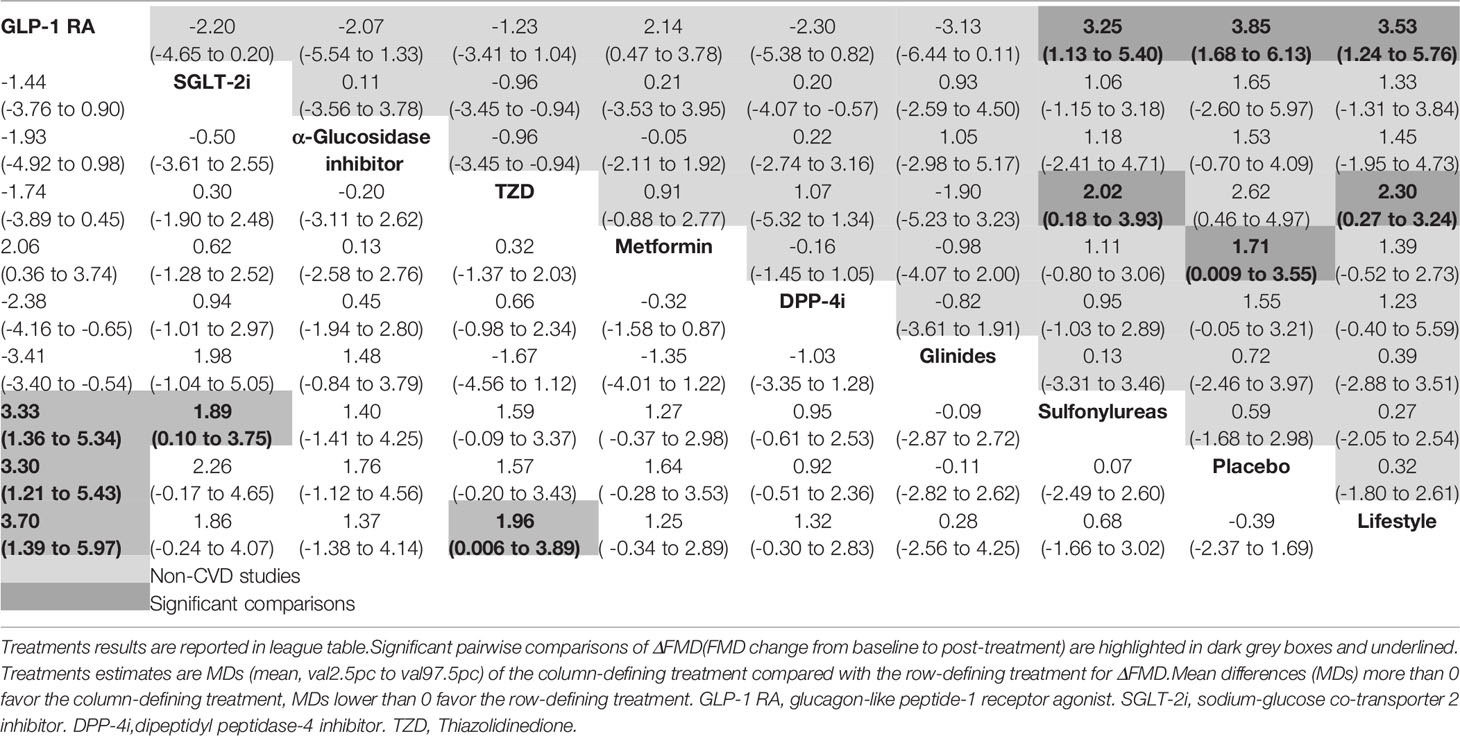
Table 2 Network meta-analysis results for ΔFMD in all studies (30 trials, left lower half) and non-CVD studies (25 trials, right upper half).
The SUCRA curves showed the detailed ranking of each treatment (Supplementary Figure 2). Based on the SUCRA values, GLP-1R agonists (SUCRA 96.8%) showed the leading effect with regard to improvement of FMD, followed by SGLT-2 inhibitors (SUCRA 74.9%), α-glucosidase inhibitors (SUCRA 62.1%), metformin (SUCRA 60.6%) and thiazolidinedione (SUCRA 69.1%). The DPP-4 inhibitors (SUCRA 51.0%) are better than glinides (SUCRA 23.9%). The efficacy of sulfonylureas (SUCRA 22.7%) with regard to FMD improvement was lower than any other antidiabetic drug and nearly equivalent to placebo (SUCRA 23.3%). Lifestyle was the least effective treatment with SUCRA 15.1% (Supplementary Table 1).
Subgroup Outcomes
The non-CVD group included 25 studies, GLP-1R agonists (MD = 3.53, 95% CI, 1.24,5.76), and TZD (MD = 2.30, 95% CI, 0.27,3.24) produced improvements in FMD change compared to lifestyle intervention. GLP-1R agonists (MD = 3.25, 95% CI, 1.13, 5.40), and MD = 3.85, 95% CI, 1.68, 6.13) showed significantly greater improvements in FMD in pairwise comparisons with sulfonylureas, and placebo. Metformin also showed efficacy compared to placebo (MD = 1.71, 95% CI, 0.009, 3.55), and thiazolidinedione showed significantly greater improvements in FMD in pairwise comparisons with sulfonylureas (MD = 2.02, 95% CI, 0.18, 3.93) (Table 2).
The SUCRA value of non-CVD studies indicates that GLP-1R agonists (SUCRA 96.4%) had the highest probability of FMD improvement compared to seven other classes of antidiabetic drugs and lifestyle intervention. Thiazolidinedione (SUCRA 79.8%) also ranked highly among the 10 interventions, followed by metformin, α-glucosidase inhibitors, and DPP-4i with SUCRA 58.7, 57.4, and 54.6%. SGLT-2 inhibitors showed almost equal efficacy with these three drugs, with SUCRA 56.3% in non-CVD participants. Glinides and sulfonylureas (SUCRA 34.1 and 27.4%) showed the lowest efficacy among all included antidiabetic drugs (Supplementary Table 1).
Bias and Assessment of Inconsistency
No significant inconsistencies were identified as determined using the global inconsistency between direct and indirect estimates compared to all studies (P = 0.894, Supplementary Figure 3). Several factors may contribute to this inconsistency, for example, the sample size, study area, and study design.
The publication bias was investigated by visual examination of the funnel plot and several scatter plots were not symmetrical in the inverted funnel (Supplementary Figure 4).
Discussion
A total of 30 RCTs were included in the NMA. Both GLP-1R agonists, SGLT-2i, and thiazolidinedione (TZD) significantly improved FMD change from baseline to post-treatment in specific comparisons, suggesting that these three drugs may have positive effects on endothelial function of T2DM patients. Furthermore, GLP-1R agonists had a greater effect than sulfonylureas in all T2DM patients. In non-CVD T2DM patients, GLP-1R agonists also had a favorable effect than sulfonylureas, placebo and lifestyle treatment on improvement of FMD change. These may suggest that GLP-1R agonists are the most beneficial drugs for improving endothelial function.
Endothelial dysfunction is characterized by increased vascular tone and increased production of procoagulant and proinflammatory factors, which are associated with progression of atherosclerosis (56). Patients with T2DM are more susceptible to endothelial function impairment due to insulin resistance, accumulation of advanced glycation end products, and a vascular inflammatory state (10), resulting in a significantly higher risk for CVD. Therefore, the improvement of endothelial function is important for T2DM patients. FMD is a potential indicator of vascular endothelial function, which is also a predictor of long-term cardiovascular events (14). Previous studies have explored the effects of several newer antidiabetic drugs on endothelial function, but the conclusions have been inconsistent. Therefore, we performed a systematic review to comprehensively explore the effects of different antidiabetic drugs on endothelial function by evaluating FMD in T2DM patients.
The Liraglutide Effect and Action in Diabetes: Evaluation of Cardiovascular Outcome Results (LEADER) (2) and the Trial to Evaluate Cardiovascular and Other Long-term Outcomes with Semaglutide in Subjects with Type 2 Diabetes (SUSTAIN-6) (57) showed a reduced risk for major adverse cardiovascular events (MACE), supporting the possible beneficial cardiovascular effects of GLP-1R agonist-based treatments. With regard to endothelial function, GLP-1R agonists have been shown to significantly reduce arterial stiffness based on assessment of pulse wave velocity (16). Similar to these active outcomes of vascular protection, in our meta-analysis, GLP-1R agonists were the highest-ranking antidiabetic drugs with regard to improvement of FMD. This drug also significantly improved FMD in the non-CVD subgroup compared with metformin and sulfonylureas. This is the first study to indicate that GLP-1R agonists may be effective for improving vascular endothelial function in T2DM patients. The effects of GLP-1R agonists on vascular endothelium could be divided into direct and indirect effects based on in vitro studies and animal experiments. First, the AMPK-eNOS pathway was activated by GLP-1R agonists acting directly on endothelial cells, promoting NO production, and mediating endothelial vasorelaxation (58). Second, GLP-1R agonists may protect the endothelium indirectly by having anti-inflammatory effects and improving lipid metabolism, slowing down the progression of atherosclerosis (59, 60). In addition, GLP-1R agonists are involved in the inhibition of platelet aggregation and thrombosis. The mechanism remains unclear whether this process is mediated by endothelial cells (61), but this effect on vascular endothelial function is positive in our analysis. On the other hand, the effects of GLP-1R agonists on FMD were more prominent in subgroup analysis of T2DM patients without comorbid CVD (i.e., the non-CVD group). We speculated that patients without comorbid CVD may be more sensitive to the effects of GLP-1R agonists because they have better vascular functional status than patients with CVD or at least the endothelial cell function is not severely impaired in this population.
DPP-4 inhibitors and SGLT-2 inhibitors are two newer classes of hypoglycemic agents, and DPP-4 inhibitors have been in use for longer time. The Saxagliptin Assessment of Vascular Outcomes Recorded in Patients with Diabetes Mellitus-Thrombolysis in Myocardial Infarction (SAVOR-TIMI) 53 trial is one of the earliest completed trials of a DPP-4 inhibitor, which suggested that saxagliptin (a DPP-4 inhibitor antidiabetic drug) was related to increased hospitalization rates in patients with heart failure, raising concerns about the cardiovascular safety of this class of drug (62). In several subsequent clinical RCTs with MACE events as major endpoints, such as the Trial Evaluating Cardiovascular Outcomes with Sitagliptin (TECOS) and CARMELINA trials, there were no significant differences in the incidence of MACE events between DPP-4 inhibitor treatment and placebo (63). Reaven and his colleague reported the SAVOR-TIMI, TECOS and CARMELINA was primarily designed as non-inferiority trials, so they concluded these trials may have less sufficient power to assess the cardiovascular benefits of DPP-4 inhibitors (64). However, in our analysis, DPP-4 inhibitors did not show significant improvement on FMD better than the two new antidiabetic drugs, GLP-R against and SGLT-2 inhibitors. Moreover, two studies (42, 43) observed FMD from baseline to a medium-long time frame, the results indicated that sitagliptin, one class drug of DPP-4 inhibitor, did not significantly influenced endothelial function during the final observation. As the result of TECOS and CARMELINA trials indicated, DPP-4 inhibitors also did not perform significant effect to reduce the incidence of MACE events (63), this suggested that the protective effect of DPP-4 inhibitor on endothelial function remains limited. DPP-4 inhibitors are known to effect endothelial cells through GLP-1-dependent and GLP-1-independent pathways in animal models. The activation of the GLP-1R in endothelial cells could increase the phosphorylation of e-NOS and nitric oxide production (65), leading to vasodilation and endothelial repair. SDF-1α is a natural substrate of DPP-4 inhibitors, and is a major regulator of endothelial progenitor cells (EPCs) (66). SDF-1α can repair blood vessels by inducing EPC homing and migration (67). However, increased EPCs may have dual effects, because a sustained increase in number of EPCs is closely associated with the development of vascular stenosis and CVD, and this may have a detrimental effect on vascular endothelial function (68). Thus, the potential effects of the GLP-1-independent pathway may partially explain the differences between the effects of GLP-1R agonists and DPP-4 inhibitors on endothelial function. Therefore, the effects of DPP-4 inhibitors on FMD may represent the combined result of GLP-1-dependent and GLP-1-independent mechanisms, and this combined effect may not as effective as GLP-R against in terms of endothelial function.
Previous RCTs have confirmed the protective effects of SGLT-2 inhibitors on cardiovascular function. However, the specific mechanisms remain poorly understood. Recent meta-analyses based on several small RCTs have reported an ameliorative effect of SGLT-2 inhibitors on endothelial function by assessing FMD (16). In the present study, SGLT-2 inhibitors ranked better than traditional antidiabetic agents, such as metformin, glinides and sulfonylureas with regard to improvement of FMD, but as a new class of antidiabetic drug with certain cardiovascular benefits, SGLT-2 inhibitors still ranked behind the most effective drugs, GLP-1R agonists. It should be noted that SGLT-2 inhibitors, such as canagliflozin, may be associated with increased risk for limb amputation in patients with T2DM and show a close association with inadequate peripheral vascular perfusion (69). SGLT-2 inhibitors is proved to reduce blood volume in T2DM patients by increasing osmotic diuresis and reducing peripheral perfusion (70), which may significantly affect the change of FMD (71). In addition, previous clinical studies have suggested that SGLT-2 inhibitors may have cardiovascular protective effects by regulating renal blood flow and improving myocardial metabolism (72, 73). We speculated that the cardiovascular protective effects of SGLT-2 inhibitors may depend on their direct effects on cardiac and renal target organs. The hypoglycemic effects of SGLT-2 inhibitors may also improve endothelial function by reducing the hyperglycemic state of the vascular endothelium, but this effect seems to be limited. Further studies are required to confirm these speculations.
In the present study, sulfonylureas showed the lowest ranking among the classes of antidiabetic drug in terms of improving FMD. This suggests that the association between sulfonylureas and vascular endothelial function may not be strong. As a traditional hypoglycemic agent, the cardiovascular effects of sulfonylureas on patients with T2DM remain controversial (74). A network meta-analysis that included 18 studies using sulfonylureas showed that neither gliclazide nor glimepiride was associated with an increased risk for cardiovascular mortality, whereas glibenclamide showed a correlation with increased cardiovascular mortality risk (75). First-generation sulfonylureas are associated with cardiovascular mortality, which may be related to their blocking effect on ATP-sensitive K+ channels (KATP channels) of cardiovascular smooth muscle cells. This effect impairs myocardial ischemic preadaptation, a physiological mechanism of protecting the myocardium against ischemic insult, which leads to reduction of coronary blood flow and increased peripheral vascular resistance (76). However, it is unclear whether this effect could affect FMD. Furthermore, different sulfonylurea drugs show variable abilities to interfere with KATP channels, e.g., unlike glibenclamide, glimepiride has been shown to have no significant effect on vascular KATP channels (77), which may explain the inconsistencies of cardiovascular outcomes among previous meta-analysis. In the present study, the sulfonylureas mainly included two drugs, glibenclamide and glimepiride. As the effects of these two drugs on endothelial function were taken into account simultaneously, the ranking of sulfonylureas on FMD may need to be interpreted carefully.
The ranking of α-glycosidase inhibitors in the SUCRA table was prominent, and this effect should not be overlooked. A meta-analysis of five RCTs involving α-glucosidase inhibitor treatment showed that these drugs delayed the increase in CIMT in T2DM patients (78), suggesting that α-glycosidase inhibitors may have a positive effect on the vascular endothelium. Our network analysis of α-glycosidase inhibitors supports this conclusion. The postprandial peak in glycemic status induces oxidative stress, which directly impairs endothelial cell function (79). Therefore, α-glycosidase inhibitors may protect the vascular endothelium by effectively reducing postprandial hyperglycemia to attenuate injury due to oxidative stress. Although the completed RCT of α-glycosidase inhibitors, the Acarbose Cardiovascular Evaluation trial (ACE), did not find that these drugs reduced heart failure or cardiovascular death in patients with T2DM or impaired glucose tolerance, this study had several limitations, i.e., the study was performed only in China and the participants all had impaired glucose tolerance and coronary heart disease (80). Further relevant clinical studies are required to elucidate the effects of α-glucosidase inhibitors on vascular function in T2DM patients.
As traditional insulinotropic agents, the effects of thiazolidinediones (TZDs) on cardiovascular events are highly controversial and previous clinical trials have yielded inconsistent results. As rosiglitazone and troglitazone are no longer widely used in clinical practice, we only discuss the effects of one TZD drug, pioglitazone, which is still used in the clinic. In the SUCRA table, pioglitazone ranked better than the new class of drug, DPP-4 inhibitors, and metformin in improving FMD, suggesting that pioglitazone may have a positive effect on vascular function. In a study that used a hypertensive rat model, pioglitazone was shown to activate peroxisome-activated receptors (PPARs) by regulating endothelin-1 (ET-1) expression to attenuate the effects of oxidative stress on the vasculature. In addition, pioglitazone may improve vasodilatory function by increasing ET-1 receptor B (ETB) expression to release endothelial cell relaxing factors (81). The PROspective pioglitAzone Clinical Trial In macroVascular Events (PROactive trial) was the first large RCT to evaluate the effects of pioglitazone monotherapy on cardiovascular outcomes, and the results showed that pioglitazone reduced the risks of all-cause mortality, nonfatal heart attack, and stroke in patients with T2DM with macrovascular disease (82). The results of a meta-analysis showed a beneficial effect of pioglitazone on the risk for recurrent cardiovascular events in patients with established CVD (83). However, it is not clear whether the effects of pioglitazone on improving vascular endothelial function could have a beneficial effect on future CVD events, particularly as side effects, such as edema and fluid retention, are associated with increased risk for heart failure in T2DM patients (84). Therefore, the effects of pioglitazone on endothelial function must be interpreted carefully in the context of other complications.
In our network, metformin also had a significant positive effect on FMD change in non-CVD subgroup analysis. As an insulin sensitizer, metformin is still the drug of first-line choice for T2DM treatment which shown multiple beneficial effects against CVD. A long-term clinical trial compared to placebo shown that the metformin added treatment significantly reduced levels of several endothelial function biomarker which are associated with the risk of CV morbidity in T2DM patients (85). In vitro evidence also shown that metformin may represent the result of multiple mechanisms involving AMPK activation, endothelium-dependent vascular response, and oxidative stress on endothelial protection (86). These suggest that metformin may improve endothelial function through different pathways, our analysis also supported the potential beneficial effect of metformin in endothelial function especially on T2DM patients without CVD.
This meta-analysis analyzed the effects of several common antidiabetic drugs on FMD. We found that several antidiabetic drugs have positive effects on endothelial function while simultaneously contributing to blood glucose control, and these effects may explain their specific benefits for the risk of future CVD outcomes in T2DM patients. Though from the analysis we didn’t find lifestyle change to be an effective treatment to benefit the endothelial function compared to other antidiabetics drugs, however, the effect of lifestyle on vascular function needs more evidence to prove. Therefore, the potential effects on endothelial function should be taken into consideration when choosing suitable treatments for patients with T2DM.
As far as we know, this is the first study to indicate that GLP-1R agonists may be effective for improving vascular endothelial function in T2DM patients. As FMD is recommended as a reproducible and practical technique to be used for different term pharmacological interventions (87), several factors such as the vascular condition of the patient, measure time frame, and laboratory experience should be noticed to evaluate the effect of FMD (88). Patient under different vascular condition may reflect the response of FMD measurement, our subgroup analysis result indicated that more than two antidiabetic drugs may have positive effect on endothelial function in T2DM patients who under better vascular condition. For studies under different measure time frame, we mainly analyzed studies measured FMD at medium to long time frames to focus on its stable effect on CVD prediction value. Studies should perform FMD according to guidelines which are crucial to ensure valid conclusions and clinical evaluation.
Limitations
This study was a comprehensive assessment of representative antidiabetic drugs for treatment of T2DM, and we found that their effects on vascular function as measured via FMD varied considerably. The novel antidiabetic drugs GLP-1R agonists may have unique advantages in improving vascular function in T2DM patients. However, this study had some limitations that should be taken into account when interpreting our findings. First, in our network, we extracted the mean, SD, and sample size at baseline and post-treatment to calculate the change of FMD. The data extraction and transformation process of two studies (31, 42) may lead to follow-up bias. Second, eight classes of antidiabetic drug were analyzed. However, there may have been discrepancies due to different drugs within a single class. In addition, several factors may have contributed to the inconsistencies observed in this study. The duration of diabetes ranged from newly diagnosed to more than 3 years, there were ethnic and regional differences in participants (20 study populations were from Asia, 3were from Europe, 5 were from North America, 1 was from South America, and 1 was from Oceania) included in the analysis. In addition, even if twelve of the included studies clearly stated that assessing FMD was performed by professional ultrasound physicians in a blinded manner, there may have been measurement error.
Some potential confounders affecting FMD were not controlled in our analysis. There were several factors that may have affected FMD assessment in studies, namely, age, sex, BMI, HbA1c and measure timeframe. The influence of these factors should be considered when interpreting the results of this meta-analysis. Many studies were not designed as RCTs, and so the evidence that could be used for network analysis was limited. In addition, we only included published studies, and so cannot exclude the possibility of publication bias.
Conclusions
Three classes of antidiabetic drug, GLP-1R agonists, TZD, and SGLT-2i inhibitors, may have positive effects on endothelial function in T2DM patients. Among antidiabetic drugs of the present network meta-analysis, GLP-1R agonists were superior to other antidiabetic drugs in endothelial function improvement. Thus, GLP-1R agonists have potential as novel therapeutics to protect endothelial function and reduce CVD outcomes in T2DM patients.
Data Availability Statement
The original contributions presented in the study are included in the article/Supplementary Material. Further inquiries can be directed to the corresponding authors.
Author Contributions
YHW designed the study, performed statistical analysis, and interpreted the data for analysis. MYY wrote the first draft. JCW and HZL conducted the database search, screened and extracted data. XLZ, LZ, and XDH contributed to the discussion and editing. ZHL designed the study and critically revised the draft manuscript. HXG had full access to the data and had final responsibility for the decision to submit for publication. All authors listed have made a substantial, direct, and intellectual contribution to the work and approved it for publication.
Conflict of Interest
The authors declare that the research was conducted in the absence of any commercial or financial relationships that could be construed as a potential conflict of interest.
Publisher’s Note
All claims expressed in this article are solely those of the authors and do not necessarily represent those of their affiliated organizations, or those of the publisher, the editors and the reviewers. Any product that may be evaluated in this article, or claim that may be made by its manufacturer, is not guaranteed or endorsed by the publisher.
Supplementary Material
The Supplementary Material for this article can be found online at: https://www.frontiersin.org/articles/10.3389/fendo.2022.818537/full#supplementary-material
References
1. Timmis A, Townsend N, Gale C, Grobbee R, Maniadakis N, Flather M, et al. European Society of Cardiology: Cardiovascular Disease Statistics 2017. Eur Heart J (2018) 39:508–79. doi: 10.1093/eurheartj/ehx628
2. Marso SP, Daniels GH, Brown-Frandsen K, Kristensen P, Mann JF, Nauck MA, et al. Liraglutide and Cardiovascular Outcomes in Type 2 Diabetes. N Engl J Med (2016) 375:311–22. doi: 10.1056/NEJMoa1603827
3. McMurray JJV, Solomon SD, Inzucchi SE, Køber L, Kosiborod MN, Martinez FA, et al. Dapagliflozin in Patients With Heart Failure and Reduced Ejection Fraction. N Engl J Med (2019) 381:1995–2008. doi: 10.1056/NEJMoa1911303
4. Petrie MC, Verma S, Docherty KF, Inzucchi SE, Anand I, Belohlávek J, et al. Effect of Dapagliflozin on Worsening Heart Failure and Cardiovascular Death in Patients With Heart Failure With and Without Diabetes. JAMA (2020) 323(14):1353–68. doi: 10.1001/jama.2020.1906
5. Marx N, Davies MJ, Grant PJ, Mathieu C, Petrie JR, Cosentino F, et al. Guideline Recommendations and the Positioning of Newer Drugs in Type 2 Diabetes Care. Lancet Diabetes Endocrinol (2021) 9(1):46–52. doi: 10.1016/S2213-8587(20)30343-0
6. Rosenstock J, Perkovic V, Johansen OE, Cooper ME, Kahn SE, Marx N, et al. Effect of Linagliptin vs Placebo on Major Cardiovascular Events in Adults With Type 2 Diabetes and High Cardiovascular and Renal Risk: The CARMELINA Randomized Clinical Trial. JAMA (2019) 321:69–79. doi: 10.1001/jama.2018.18269
7. Holman RR, Coleman RL, Chan JCN, Chiasson JL, Feng H, Ge J, et al. Effects of Acarbose on Cardiovascular and Diabetes Outcomes in Patients With Coronary Heart Disease and Impaired Glucose Tolerance (ACE): A Randomized, Double-Blind, Placebo-Controlled Trial. Lancet Diabetes Endocrinol (2017) 5:877–86. doi: 10.1016/S2213-8587(17)30309-1
8. Phung OJ, Schwartzman E, Allen RW, Engel SS, Rajpathak SN. Sulphonylureas and Risk of Cardiovascular Disease: Systematic Review and Meta-Analysis. Diabetes Med (2013) 30(10):1160–71. doi: 10.1111/dme.12232
9. Green DJ, Hopman MT, Padilla J, Laughlin MH, Thijssen DH. Vascular Adaptation to Exercise in Humans: Role of Hemodynamic Stimuli. Physiol Rev (2017) 97:495–528. doi: 10.1152/physrev.00014.2016
10. Kaur R, Kaur M, Singh J. Endothelial Dysfunction and Platelet Hyperactivity in Type 2 Diabetes Mellitus: Molecular Insights and Therapeutic Strategies. Cardiovasc Diabetol (2018) 17(1):121. doi: 10.1186/s12933-018-0763-3
11. Thijssen DH, Black MA, Pyke KE, Padilla J, Atkinson G, Harris RA, et al. Assessment of Flow-Mediated Dilation in Humans: A Methodological and Physiological Guideline. Am J Physiol Heart Circ Physiol (2011) 300(1):H2–12. doi: 10.1152/ajpheart.00471.2010
12. Yeboah J, Folsom AR, Burke GL, Johnson C, Polak JF, Post W, et al. Predictive Value of Brachial Flow-Mediated Dilation for Incident Cardiovascular Events in a Population-Based Study: The Multi-Ethnic Study of Atherosclerosis. Circulation (2009) 120(6):502–9. doi: 10.1161/CIRCULATIONAHA.109.864801
13. Maruhashi T, Soga J, Fujimura N, Idei N, Mikami S, Iwamoto Y, et al. Endothelial Dysfunction, Increased Arterial Stiffness, and Cardiovascular Risk Prediction in Patients With Coronary Artery Disease: FMD-J (Flow-Mediated Dilation Japan) Study a. J Am Heart Assoc (2018) 7(14):e008588. doi: 10.1161/JAHA.118.008588
14. Thijssen DHJ, Bruno RM, van Mil ACCM, Holder SM, Faita F, Greyling A, et al. Expert Consensus and Evidence-Based Recommendations for the Assessment of Flow-Mediated Dilation in Humans. Eur Heart J (2019) 40(30):2534–47. doi: 10.1093/eurheartj/ehz350
15. Stojanović M, Prostran M, Radenković M. Thiazolidinediones Improve Flow-Mediated Dilation: A Meta-Analysis of Randomized Clinical Trials. Eur J Clin Pharmacol (2016) 72(4):385–98. doi: 10.1007/s00228-015-1999-4
16. Batzias K, Antonopoulos AS, Oikonomou E, Siasos G, Bletsa E, Stampouloglou PK, et al. Effects of Newer Antidiabetic Drugs on Endothelial Function and Arterial Stiffness:A Systematic Review and Meta-Analysis. J Diabetes Res (2018) 2018:1232583. doi: 10.1155/2018/1232583
17. Fei Y, Tsoi MF, Cheung BMY. Cardiovascular Outcomes in Trials of New Antidiabetic Drug Classes: A Network Meta-Analysis. Cardiovasc Diabetol (2021) 18(1):112. doi: 10.1186/s12933-019-0916-z
18. Salanti G. Indirect and Mixed-Treatment Comparison, Network, or Multiple-Treatments Meta-Analysis: Many Names, Many Benefits, Many Concerns for the Next Generation Evidence Synthesis Tool. Res Synth Methods (2012) 3(2):80–97. doi: 10.1002/jrsm.1037
19. Higgins JP, Li T, Deeks JJ. Choosing Effect Measures and Computing Estimates of Effect. In: Cochrane Handbook for Systematic Reviews of Interventions. Chichester (UK): John Wiley & Sons (2019).
20. Hozo SP, Djulbegovic B, Hozo I. Estimating the Mean and Variance From the Median, Range, and the Size of a Sample. BMC Med Res Methodol (2005) 5:13. doi: 10.1186/1471-2288-5-13
21. Wan X, Wang W, Liu J, Tong T. Estimating the Sample Mean and Standard Deviation From the Sample Size, Median, Range and/or Interquartile Range. BMC Med Res Methodol (2014) 14:135. doi: 10.1186/1471-2288-14-135
22. Caldwell DM, Ades AE, Higgins JP. Simultaneous Comparison of Multiple Treatments: Combining Direct and Indirect Evidence. BMJ (2005) 331(7521):897–900. doi: 10.1136/bmj.331.7521.897
23. van Valkenhoef G, Kuiper J. Gemtc: Network Meta-Analysis Using Bayesian Methods. R package version 0.8. Available at: http://cran.r-project.org/web/packages/gemtc/index.html
24. Page MJ, Shamseer L, Altman DG, Tetzlaff J, Sampson M, Tricco AC. Epidemiology and Reporting Characteristics of Systematic Reviews of Biomedical Research: A Cross-Sectional Study. PloS Med (2016) 13(5):e1002028. doi: 10.1371/journal.pmed.1002028
25. Salanti G, Ades AE, Ioannidis JP. Graphical Methods and Numerical Summaries for Presenting Results From Multiple-Treatment Meta-Analysis: An Overview and Tutorial. J Clin Epidemiol (2011) 64:163–71. doi: 10.1016/j.jclinepi.2010.03.016
26. Asnani S, Kunhiraman B, Jawa A, Akers D, Lumpkin D, Fonseca V. Pioglitazone Restores Endothelial Function in Patients With Type 2 Diabetes Treated With Insulin. Metab Syndr Relat Disord (2006) 4(3):179–84. doi: 10.1089/met.2006.4.179
27. Lambadiari V, Pavlidis G, Kousathana F, Varoudi M, Vlastos D, Maratou E, et al. Effects of 6-Month Treatment With the Glucagon Like Peptide-1 Analogue Liraglutide on Arterial Stiffness, Left Ventricular Myocardial Deformation and Oxidative Stress in Subjects With Newly Diagnosed Type 2 Diabetes. Cardiovasc Diabetol (2018) 17(1):8. doi: 10.1186/s12933-017-0646-z
28. Suzuki K, Watanabe K, Suzuki K, Ouchi M, Futami-Suda S, Igari Y, et al. Sitagliptin Improves Vascular Endothelial Function in Japanese Type 2 Diabetes Patients Without Cardiovascular Disease. J Diabetes Mellitus (2012) 2(3):338–45. doi: 10.4236/jdm.2012.23053
29. Suzuki K, Tanaka S, Aoki C, Kato K, Jojima K, Aso Y. Greater Efficacy and Improved Endothelial Dysfunction in Untreated Type 2 Diabetes With Liraglutide Versus Sitagliptin. Dokkyo J Med Sci (2014) 41(3):211–20.
30. Tamura H, Kondo Y, Ito K, Hasebe M, Satoh S, Terauchi Y. Comparison of the Effect of Empagliflozin and Glimepiride on the Endothelial Function in Patients With Type 2 Diabetes-a Randomized Study. Diabetes (2018) 67:305. doi: 10.2337/db18-1138-P
31. Sposito AC, Breder I, Soares AAS, Kimura-Medorima ST, Munhoz DB, Cintra RMR, et al. Dapagliflozin Effect on Endothelial Dysfunction in Diabetic Patients With Atherosclerotic Disease: A Randomized Active-Controlled Trial. Cardiovasc Diabetol (2021) 20(1):74. doi: 10.1186/s12933-021-01264-z
32. Kelly AS, Thelen AM, Kaiser DR, Gonzalez-Campoy JM, Bank AJ. Rosiglitazone Improves Endothelial Function and Inflammation But Not Asymmetric Dimethylarginine or Oxidative Stress in Patients With Type 2 Diabetes Mellitus. Vasc Med (2007) 12(4):311–8. doi: 10.1177/1358863X07084200
33. Zainordin NA, Hatta SFWM, Mohamed Shah FZ, Rahman TA, Ismail N, Ismail Z, et al. Effects of Dapagliflozin on Endothelial Dysfunction in Type 2 Diabetes With Established Ischemic Heart Disease (EDIFIED). J Endocr Soc (2019) 4(1):bvz017.
34. Tsuchiya K, Akaza I, Yoshimoto T, Hirata Y. Pioglitazone Improves Endothelial Function With Increased Adiponectin and High-Density Lipoprotein Cholesterol Levels in Type 2 Diabetes. Endocr J (2009) 56(5):691–8. doi: 10.1507/endocrj.K08E-308
35. Papathanassiou K, Naka KK, Kazakos N, Kanioglou C, Makriyiannis D, Pappas K, et al. Pioglitazone vs Glimepiride: Differential Effects on Vascular Endothelial Function in Patients With Type 2 Diabetes. Atherosclerosis (2009) 205(1):221–6. doi: 10.1016/j.atherosclerosis.2008.11.027
36. Naka KK, Papathanassiou K, Bechlioulis A, Pappas K, Kazakos N, Kanioglou C, et al. Effects of Pioglitazone and Metformin on Vascular Endothelial Function in Patients With Type 2 Diabetes Treated With Sulfonylureas. Diabetes Vasc Dis Res (2012) 9(1):52–8. doi: 10.1177/1479164111424515
37. Shigiyama F, Kumashiro N, Miyagi M, Iga R, Kobayashi Y, Kanda E, et al. Linagliptin Improves Endothelial Function in Patients With Type 2 Diabetes: A Randomized Study of Linagliptin Effectiveness on Endothelial Function. J Diabetes Investig (2017) 8(3):330–40. doi: 10.1111/jdi.12587
38. Tripolt NJ, Aberer F, Riedl R, Url J, Dimsity G, Meinitzer A, et al. Effects of Linagliptin on Endothelial Function and Postprandial Lipids in Coronary Artery Disease Patients With Early Diabetes: A Randomized, Placebo-Controlled, Double-Blind Trial. Cardiovasc Diabetol (2018) 17(1):71. doi: 10.1186/s12933-018-0716-x
39. Li F, Chen J, Leng F, Lu Z, Ling Y. Effect of Saxagliptin on Circulating Endothelial Progenitor Cells and Endothelial Function in Newly Diagnosed Type 2 Diabetic Patients. Exp Clin Endocrinol Diabetes (2017) 125(6):400–7. doi: 10.1055/s-0042-124421
40. Kitao N, Miyoshi H, Furumoto T, Ono K, Nomoto H, Miya A, et al. The Effects of Vildagliptin Compared With Metformin on Vascular Endothelial Function and Metabolic Parameters: A Randomized, Controlled Trial (Sapporo Athero-Incretin Study 3). Cardiovasc Diabetol (2017) 16(1):125. doi: 10.1186/s12933-017-0607-6
41. Kim G, Oh S, Jin SM, Hur KY, Kim JH, Lee MK. The Efficacy and Safety of Adding Either Vildagliptin or Glimepiride to Ongoing Metformin Therapy in Patients With Type 2 Diabetes Mellitus. Expert Opin Pharmacother (2017) 18(12):1179–86. doi: 10.1080/14656566.2017.1353080
42. Nomoto H, Miyoshi H, Fukumoto T, Oba K, Tsutsui H, Inoue A, et al. A Randomized Controlled Trial Comparing The Effects Of Sitagliptin And Glimepiride On Endothelial Function And Metabolic Parameters: Sapporo Athero-Incretin Study 1 (Sais1). PloS One (2016) 11(10):e0164255. doi: 10.1371/journal.pone.0164255
43. Maruhashi T, Higashi Y, Kihara Y, Yamada H, Sata M, Ueda S, et al. Long-Term Effect of Sitagliptin on Endothelial Function in Type 2 Diabetes: A Sub-Analysis of the PROLOGUE Study. Cardiovasc Diabetol (2016) 15(1):134. doi: 10.1186/s12933-016-0438-x
44. Nakamura K, Oe H, Kihara H, Shimada K, Fukuda S, Watanabe K, et al. DPP-4 Inhibitor and Alpha-Glucosidase Inhibitor Equally Improve Endothelial Function in Patients With Type 2 Diabetes: EDGE Study. Cardiovasc Diabetol (2014) 13:110. doi: 10.1186/s12933-014-0110-2
45. Sawada T, Shiotani H, Terashita D, Nagasawa Y, Kim SS, Koide M, et al. Comparison of Effects of α-Glucosidase Inhibitors and Glinide Drugs on Endothelial Dysfunction in Diabetic Patients With Coronary Artery Disease. Circ J (2014) 78(1):248–55. doi: 10.1253/circj.CJ-13-0918
46. Irace C, De Luca S, Shehaj E, Carallo C, Loprete A, Scavelli F, et al. Exenatide Improves Endothelial Function Assessed by Flow Mediated Dilation Technique in Subjects With Type 2 Diabetes: Results From an Observational Research. Diabetes Vasc Dis Res (2013) 10(1):72–7. doi: 10.1177/1479164112449562
47. Baltzis D, Dushay JR, Loader J, Wu J, Greenman RL, Roustit M, et al. Effect of Linagliptin on Vascular Function: A Randomized, Placebo-Controlled Study. J Clin Endocrinol Metab (2016) 101(11):4205–13. doi: 10.1210/jc.2016-2655
48. Caballero AE, Saouaf R, Lim SC, Hamdy O, Abou-Elenin K, O'Connor C, et al. The Effects of Troglitazone, an Insulin-Sensitizing Agent, on the Endothelial Function in Early and Late Type 2 Diabetes: A Placebo-Controlled Randomized Clinical Trial. Metabolism (2003) 52(2):173–80. doi: 10.1053/meta.2003.50023
49. Widlansky ME, Puppala VK, Suboc TM, Malik M, Branum A, Signorelli K, et al. Impact of DPP-4 Inhibition on Acute and Chronic Endothelial Function in Humans With Type 2 Diabetes on Background Metformin Therapy. Vasc Med (2017) 22(3):189–96. doi: 10.1177/1358863X16681486
50. Li JP, Zhou P. Observation on Effect of Liraglutide on Endothelial Function, Immune Function and Related Factors in Patients With Type 2 Diabetes Mellitus. China Pract Med (2019) 14(21):6–8. doi: 10.14163/j.cnki.11-5547/r.2019.21.003
51. Shi X, Wei Z. Effect of SGLT-2 Inhibitor Compared With Conventional Hypoglycemic Therapy on Vascular Endothelial Function in Patients With Type 2 Diabetes. Anhui Med J (2020) 41(1):42–6. doi: 10.3969/j.issn.1000-0399.2020.01.013
52. Zhang ZG, Liu GF. Metformin Effects on Endothelial Function in Type 2 Diabetes. J Shanxi Med Univ (2002) 033(005):428–9. doi: 10.3969/j.issn.1007-6611.2002.05.016
53. Deng ZL. Effect of Vildagliptin on Vascular Endothelial Cell Function in Patients With Newly Diagnosed Type 2 Diabetes. Acad J Guangzhou Med Univ (2015) 1):28–31. doi: 10.3969/j.issn.2095-9664.2015.01.008
54. Tang F, Li Y, Jiang TJ. Sitagliptin Ameliorates Vascular Endothelial Function in Newly Diagnosed Type 2 Diabetes Patients. J Chin Phys (2017) 9:1330–7. doi: 10.3760/cma.j.issn.1008-1372.2017.09.013
55. Han BY, Lu LM, Zhang LP, Fan ZY. Effect of DPP-4 Inhibitor Saxagliptin on Endothelium-Dependent Vasodilation in Patients With Type 2 Diabetes. J Shanxi Med Univ (2014) 45(004):291–4. doi: 10.13753/j.issn.1007-6611.2014.04.011
56. Gimbrone MA Jr, García-Cardeña G. Endothelial Cell Dysfunction and the Pathobiology of Atherosclerosis. Circ Res (2016) 118(4):620–36. doi: 10.1161/CIRCRESAHA.115.306301
57. Marso SP, Bain SC, Consoli A, Eliaschewitz FG, Jódar E, Leiter LA, et al. Semaglutide and Cardiovascular Outcomes in Patients With Type 2 Diabetes. N Engl J Med (2016) 375(19):1834–44. doi: 10.1056/NEJMoa1607141
58. Han L, Yu Y, Sun X, Wang B. Exendin-4 Directly Improves Endothelial Dysfunction in Isolated Aortas From Obese Rats Through the cAMP or AMPK-eNOS Pathways. Diabetes Res Clin Pract (2012) 97(3):453–60. doi: 10.1016/j.diabres.2012.04.001
59. Drucker DJ. Mechanisms of Action and Therapeutic Application of Glucagon-Like Peptide-1. Cell Metab (2018) 27(4):740–56. doi: 10.1016/j.cmet.2018.03.001
60. Sposito AC, Berwanger O, de Carvalho LSF, Saraiva JFK. GLP-1ras in Type 2 Diabetes: Mechanisms That Underlie Cardiovascular Effects and Overview of Cardiovascular Outcome Data. Cardiovasc Diabetol (2018) 17(1):157. doi: 10.1186/s12933-018-0800-2
61. Chien CT, Fan SC, Lin SC, Kuo CC, Yang CH, Yu TY, et al. Glucagon-Like Peptide-1 Receptor Agonist Activation Ameliorates Venous Thrombosis-Induced Arteriovenous Fistula Failure in Chronic Kidney Disease. Thromb Haemost (2014) 112(5):1051–64. doi: 10.1160/th14-03-0258
62. Scirica BM, Bhatt DL, Braunwald E, Steg PG, Davidson J, Hirshberg B, et al. Saxagliptin and Cardiovascular Outcomes in Patients With Type 2 Diabetes Mellitus. N Engl J Med (2013) 369(14):1317–26. doi: 10.1056/NEJMoa1307684
63. Scheen AJ. Cardiovascular Effects of New Oral Glucose-Lowering Agents: DPP-4 and SGLT-2 Inhibitors. Circ Res (2018) 122(10):1439–59. doi: 10.1161/CIRCRESAHA.117.311588
64. Koska J, Sands M, Burciu C, Reaven P. Cardiovascular Effects of Dipeptidyl Peptidase-4 Inhibitors in Patients With Type 2 Diabetes. Diabetes Vasc Dis Res (2015) 12(3):154–63. doi: 10.1177/1479164114562411
65. Matsubara J, Sugiyama S, Sugamura K, Nakamura T, Fujiwara Y, Akiyama E, et al. A Dipeptidyl Peptidase-4 Inhibitor, Des-Fluoro-Sitagliptin, Improves Endothelial Function and Reduces Atherosclerotic Lesion Formation in Apolipoprotein E-Deficient Mice. J Am Coll Cardiol (2012) 59(3):265–76. doi: 10.1016/j.jacc.2011.07.053
66. Fadini GP, Avogaro A. Dipeptidyl Peptidase-4 Inhibition and Vascular Repair by Mobilization of Endogenous Stem Cells in Diabetes and Beyond. Atherosclerosis (2013) 229:23–9. doi: 10.1016/j.atherosclerosis.2013.04.007
67. Kamel NM, Abd El Fattah MA, El-Abhar HS, Abdallah DM. Novel Repair Mechanisms in a Renal Ischaemia/Reperfusion Model: Subsequent Saxagliptin Treatment Modulates the Pro-Angiogenic GLP-1/cAMP/VEGF, ANP/eNOS/NO, SDF-1α/CXCR4, and Kim-1/STAT3/HIF-1α/VEGF/eNOS Pathways. Eur J Pharmacol (2019) 861:172620. doi: 10.1016/j.ejphar.2019.172620
68. Rigato M, Avogaro A, Fadini GP. Levels of Circulating Progenitor Cells, Cardiovascular Outcomes and Death: A Meta-Analysis of Prospective Observational Studies. Circ Res (2016) 118(12):1930–9. doi: 10.1161/CIRCRESAHA.116.308366
69. Neal B, Perkovic V, Mahaffey KW, de Zeeuw D, Fulcher G, Erondu N, et al. Canagliflozin and Cardiovascular and Renal Events in Type 2 Diabetes. N Engl J Med (2017) 377(7):644–57. doi: 10.1056/NEJMoa1611925
70. Tanaka A, Node K. Increased Amputation Risk With Canagliflozin Treatment: Behind the Large Cardiovascular Benefit? Cardiovasc Diabetol (2017) 16(1):129. doi: 10.1186/s12933-017-0611-x
71. Frech TM, Murtaugh MA. Non-Invasive Digital Thermal Monitoring and Flow-Mediated Dilation in Systemic Sclerosis. Clin Exp Rheumatol (2019) 37 Suppl 119(4):97–101.
72. Cherney DZ, Perkins BA, Soleymanlou N, Maione M, Lai V, Lee A, et al. Renal Hemodynamic Effect of Sodium-Glucose Cotransporter 2 Inhibition in Patients With Type 1 Diabetes Mellitus. Circulation (2014) 129(5):587–97. doi: 10.1161/CIRCULATIONAHA.113.005081
73. Ferrannini E, Mark M, Mayoux E. CV Protection in the EMPA-REG OUTCOME Trial: A "Thrifty Substrate" Hypothesis. Diabetes Care (2016) 39(7):1108–14. doi: 10.2337/dc16-0330
74. Webb DR, Davies MJ, Jarvis J, Seidu S, Khunti K. The Right Place for Sulphonylureas Today. Diabetes Res Clin Pract (2019) 157:107836. doi: 10.1016/j.diabres.2019.107836
75. Simpson SH, Lee J, Choi S, Vandermeer B, Abdelmoneim AS, Featherstone TR. Mortality Risk Among Sulfonylureas: A Systematic Review and Network Meta-Analysis. Lancet Diabetes Endocrinol (2015) 3(1):43–51. doi: 10.1016/S2213-8587(14)70213-X
76. Abdelmoneim AS, Eurich DT, Light PE, Senior PA, Seubert JM, Makowsky MJ, et al. Cardiovascular Safety of Sulphonylureas: Over 40 Years of Continuous Controversy Without an Answer. Diabetes Obes Metab (2015) 17(6):523–32. doi: 10.1111/dom.12456
77. Sato T, Nishida H, Miyazaki M, Nakaya H. Effects of Sulfonylureas on Mitochondrial ATP-Sensitive K+ Channels in Cardiac Myocytes: Implications for Sulfonylurea Controversy. Diabetes Metab Res Rev (2006) 22(5):341–7. doi: 10.1002/dmrr.621
78. Geng DF, Jin DM, Wu W, Fang C, Wang JF. Effect of Alpha-Glucosidase Inhibitors on the Progression of Carotid Intima-Media Thickness: A Meta-Analysis of Randomized Controlled Trials. Atherosclerosis (2011) 218(1):214–9. doi: 10.1016/j.atherosclerosis.2011.05.004
79. Ceriello A, Esposito K, Piconi L, Ihnat MA, Thorpe JE, Testa R, et al. Oscillating Glucose is More Deleterious to Endothelial Function and Oxidative Stress Than Mean Glucose in Normal and Type 2 Diabetic Patients. Diabetes (2008) 57(5):1349–54. doi: 10.2337/db08-0063
80. Wamil M, McMurray JJV, Scott CAB, Coleman RL, Sun Y, Standl E, et al. Predicting Heart Failure Events in Patients With Coronary Heart Disease and Impaired Glucose Tolerance: Insights From the Acarbose Cardiovascular Evaluation (ACE) Trial. Diabetes Res Clin Pract (2020) 170:108488. doi: 10.1016/j.diabres.2020.108488
81. Palacios-Ramírez R, Hernanz R, Martín A, Pérez-Girón JV, Barrús MT, González-Carnicero Z, et al. Pioglitazone Modulates the Vascular Contractility in Hypertension by Interference With ET-1 Pathway. Sci Rep (2019) 9(1):16461. doi: 10.1038/s41598-019-52839-6
82. Dormandy JA, Charbonnel B DJAE, Erdmann E, Massi-Benedetti M, Moules IK, Skene AM, et al. Secondary Prevention on Macrovascular Events in Patients With Type 2 Diabetes in the PROactive Study (PROspective Pioglitazone Clinical Trial In Macrovascular Events): A Randomized, Controlled Trial. Lancet (2005) 366:1279–89. doi: 10.1016/S0140-6736(05)67528-9
83. de Jong M, van der Worp HB, van der Graaf Y, Visseren FLJ, Westerink J. Pioglitazone and the Secondary Prevention of Cardiovascular Disease. A Meta-Analysis of Randomized-Controlled Trials. Cardiovasc Diabetol (2017) 16(1):134. doi: 10.1186/s12933-017-0617-4
84. Lebovitz HE. Thiazolidinediones: The Forgotten Diabetes Medications. Curr Diabetes Rep (2019) 19(12):151. doi: 10.1007/s11892-019-1270-y
85. de Jager J, Kooy A, Schalkwijk C, van der Kolk J, Lehert P, Bets D, et al. Long-Term Effects of Metformin on Endothelial Function in Type 2 Diabetes: A Randomized Controlled Trial. J Intern Med (2014) 275(1):59–70. doi: 10.1111/joim.12128
86. Salvatore T, Pafundi PC, Galiero R, Rinaldi L, Caturano A, Vetrano E, et al. Can Metformin Exert as an Active Drug on Endothelial Dysfunction in Diabetic Subjects? Biomedicines (2020) 9(1):3. doi: 10.3390/biomedicines9010003
87. Charakida M, de Groot E, Loukogeorgakis SP, Khan T, Lüscher T, Kastelein JJ, et al. Variability and Reproducibility of Flow-Mediated Dilatation in a Multicentre Clinical Trial. Eur Heart J (2013) 34(45):3501–7. doi: 10.1093/eurheartj/eht223
Keywords: antidiabetic drugs, endothelial function, flow-mediated dilation, type 2, diabetes, meta-analysis
Citation: Wang Y, Yao M, Wang J, Liu H, Zhang X, Zhao L, Hu X, Guan H and Lyu Z (2022) Effects of Antidiabetic Drugs on Endothelial Function in Patients With Type 2 Diabetes Mellitus: A Bayesian Network Meta-Analysis. Front. Endocrinol. 13:818537. doi: 10.3389/fendo.2022.818537
Received: 19 November 2021; Accepted: 14 February 2022;
Published: 17 March 2022.
Edited by:
Lu Cai, University of Louisville, United StatesReviewed by:
Michelle Elaine Orme, Independent researcher, United KingdomZhen Qiu, Renmin Hospital of Wuhan University, China
Copyright © 2022 Wang, Yao, Wang, Liu, Zhang, Zhao, Hu, Guan and Lyu. This is an open-access article distributed under the terms of the Creative Commons Attribution License (CC BY). The use, distribution or reproduction in other forums is permitted, provided the original author(s) and the copyright owner(s) are credited and that the original publication in this journal is cited, in accordance with accepted academic practice. No use, distribution or reproduction is permitted which does not comply with these terms.
*Correspondence: Haixia Guan, aHhndWFuQHZpcC4xMjYuY29t; Zhaohui Lyu, YTE3NTMxMDczMTA2QDE2My5jb20=
†These authors have contributed equally to this work