- 1Department of Pediatrics, West China Second University Hospital, Sichuan University, Chengdu, China
- 2Key Laboratory of Birth Defects and Related Diseases of Women and Children, Ministry of Education, Sichuan University, Chengdu, China
- 3Reproductive Endocrinology and Regulation Laboratory, West China Second University Hospital, Sichuan University, Chengdu, China
- 4Department of Andrology/Sichuan Human Sperm Bank, West China Second University Hospital, Sichuan University, Chengdu, China
Brain and muscle aryl-hydrocarbon receptor nuclear translocator like protein1 (BMAL1), a core component of circadian oscillation, is involved in many physiological activities. Increasing evidence has demonstrated the essential role of BMAL1 in reproductive physiology. For instance, BMAL1-knockout (KO) mice were infertile, with impaired reproductive organs and gametes. Additionally, in BMAL1-KO mice, hormone secretion and signaling of hypothalamus-pituitary-gonadal (H-P-G) hormones were also disrupted, indicating that H-P-G axis was impaired in BMAL1-KO mice. Moreover, both BMAL1-KO mice and BMAL1-knockdown by small interfering RNA (siRNA) in vitro cultured steroidogenic cells showed that BMAL1 was associated with gonadal steroidogenesis and expression of related genes. Importantly, BMAL1 also participates in pathogenesis of human reproductive diseases. In this review, we elaborate on the impaired reproduction of BMAL1-KO mice including the reproductive organs, reproductive endocrine hormones, and reproductive processes, highlighting the vital role of BMAL1 in fertility and reproductive endocrinology.
Introduction
Circadian rhythms play essential roles in various physiological processes as well as the development of organisms, which are now known to be largely controlled by a group of transcription factor genes. In mammals, such molecular clock is expressed and operational in most cells of the body (1). The central clock in the SCN (suprachiasmatic nucleus) is the master circadian oscillator that synchronizes the peripheral clocks (2, 3). The circadian clock transcription factor, BMAL1 (brain and muscle aryl hydrocarbon receptor nuclear translocator like protein 1), also known as ARNTL1 or MOP3, which is expressed in most tissues especially in endocrine tissues, is a member of the bHLH-PAS (basic helix loop helix-period aryl hydrocarbon receptor nuclear translocator single-minded) family of transcription factors (4–7). BMAL1 and its partner, CLOCK (circadian locomotor output cycles kaput), heterodimerize and bind to E-box elements in the promoter regions of clock-controlled genes to initiate their transcription, thereby driving the circadian rhythm (7).
A variety of physiological functions of BMAL1 have been identified. Studies have revealed that BMAL1-knockout (KO) mice have multiple defects including premature aging (8, 9), skeletal dysplasia (10–12), abnormal hair growth (13, 14), and especially poor reproduction (15–17). BMAL1-KO mice were infertile (15–17), with defective gonads and gametes (15, 18–20). Moreover, the hypothalamus–pituitary–gonad (H-P-G) axis was found dysfunctional in these animals, including the abnormal secretion and signaling of gonadal steroids (19, 21, 22), gonadotropins (15, 23), and gonadotropin-releasing hormones (GnRH) (24). Also, BMAL1-KO mice exhibited problematic mating behaviors (25), uterine decidualization (26, 27) and embryo implantation (22). Moreover, accumulating clinical evidence has suggested that BMAL1 also participates in human reproductive diseases (26–29), suggesting BMAL1 as a potential theraputic target for related reproductive diseases.
BMAL1 in Reproductive Organ Development and Fertility
Various clues indicate that the development of the reproductive organs in BMAL1-KO mice is impaired (9, 16, 17, 22, 23, 30). Among such studies, these mice were reported to be arrhythmic under free-running conditions (constant darkness, constant temperature), with delayed puberty, irregular estrus cycles, and infertility (15, 16, 30). These fertility-impaired mice usually presented with structural and functional abnormalities in reproductive organs and gametes.
Effect of BMAL1 Knockout on Female Reproduction in Mice
Female BMAL1-KO mice displayed undersized and underdeveloped ovaries with a significant reduction in the number of corpora lutea and a higher proportion of atretic follicles (15). Despite these ovarian abnormalities, BMAL-KO female mice can ovulate; however, the quantity and quality of the oocytes are reduced (15, 16, 22, 31). A couple of studies reported that female BMAL1-KO mice had fewer ovulated oocytes and more abnormal oocytes after superovulation (15, 22, 32), which resembles the phenotypes of elder wild-type (WT) mice (15, 33), consistent with the premature aging feature of BMAL1-KO mice (9, 34). In addition, the BMAL1-KO mice exhibited abnormal follicle development, lower fertilization rate and retarded early embryo/blastocyst development (15, 32). Of note, although both in vivo fertilization in female BMAL1-KO mice and in vitro fertilization using BMAL1-KO oocytes were found to be impaired, the success rate of the in vitro fertilization was higher compared to that of in vivo fertilization in female BMAL1-KO mice (32), suggesting that the environment for fertilization and/or early embryo development might be affected by BMAL deletion. Further studies revealed high reactive oxygen species (ROS) levels in ovaries and oviducts of the female BMAL1-KO mice (32). Consistently, BMAL1 was found as a direct regulator for ROS homeostasis (9, 34). Although ROS are important regulators of various physiological processes (35), excessive ROS could be detrimental for normal reproductive activities (36–38). Therefore, the excessive ROS in ovaries and oviducts may account for the defective follicular development, fertilization and early embryo development in female BMAL1-KO mice.
In addition, female BMAL1-KO mice had undersized uteri (15). Recent studies have revealed that BMAL1 is associated with trophoblast invasion of the uterus decidualization (26, 27), which is a prerequisite for embryo implantation (39, 40). Furthermore, female BMAL1-KO mice were reported to experience an unsuccessful implantation (22, 32), since well-fertilized oocytes and well-developed preimplantation embryos could be observed in the reproductive tract on the implantation day (22). Therefore, the compromised implantation process of female BMAL1-KO mice can contribute to the impaired fertility of female BMAL1-KO mice (22). These structural abnormalities of the uterus and ovaries correlated with functional defects (Figure 1).
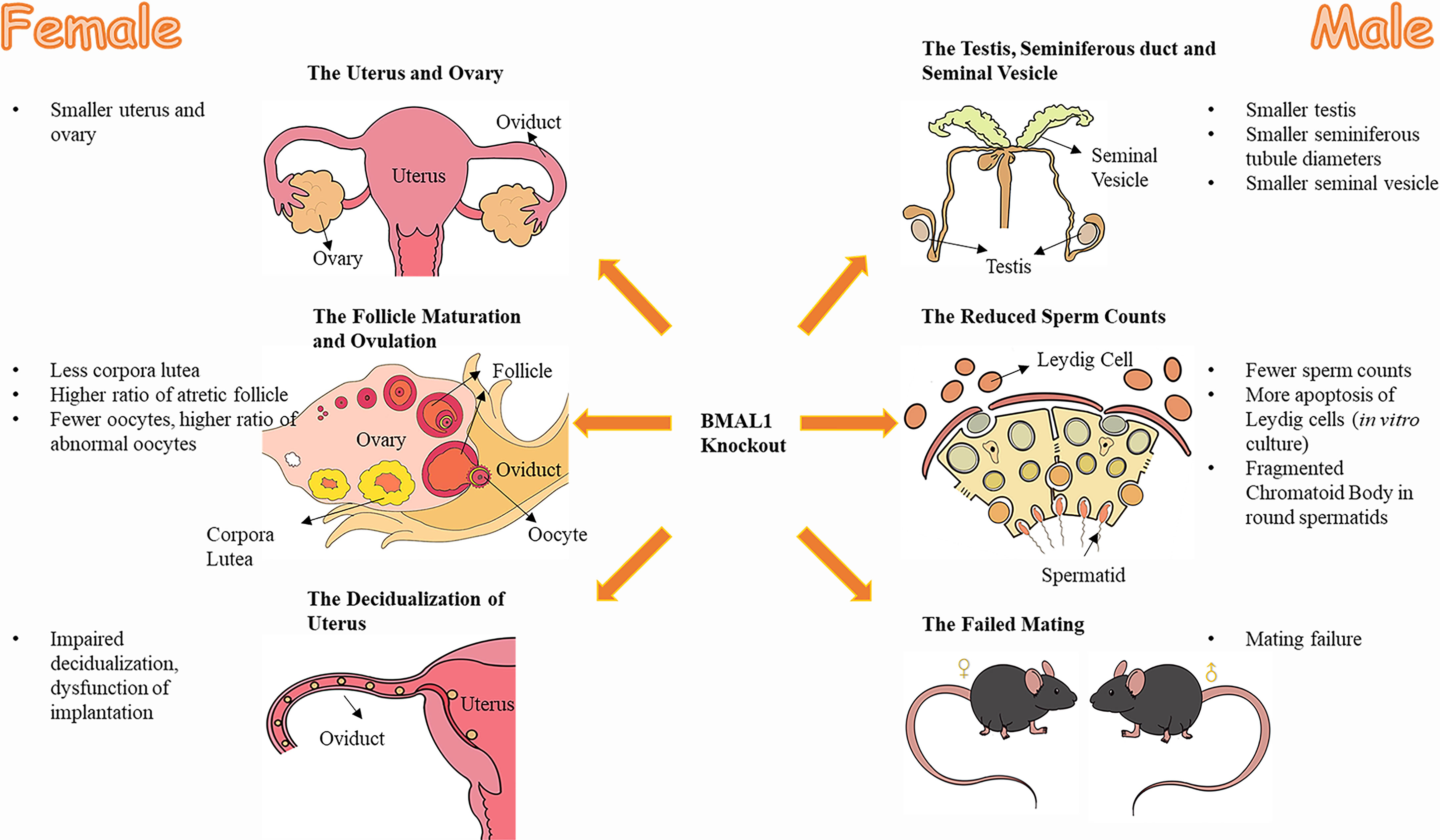
Figure 1 The effect of BMAL1 knockout on mice reproduction. Both male and female BMAL1-KO mice suffered from varying degrees of dysplasia and dysfunction in their reproductive tissues. The female BMAL1-KO mice have smaller uteri and ovaries, with less corpora lutea, higher ratio of atretic follicles, fewer oocytes, higher ratio of abnormal oocytes, impaired decidualization procedures and thereby the impaired implantation function of the uterus. Male BMAL1-KO mice possess smaller testis, smaller seminiferous tubules, smaller seminal vesicles, with fewer sperms, more impaired Leydig cells, fragmented Chromatoid Body in round spermatids and their impaired behaviors of mating.
Effect of BMAL1 Knockout on Male Reproduction in Mice
Similar to the findings noted in females, male BMAL1-KO mice contained abnormal gonad and gametes. Male BMAL1-KO mice possessed slightly smaller testes, markedly smaller seminal vesicles, and generally reduced seminiferous tubule diameters (9, 23). The in vitro culture of TM3 Leydig cells have shown that silencing the BMAL1 gene might contribute to the apoptosis of Leydig cells which produce testosterone (41).
Male BMAL1-KO mice exhibited a nearly 70% reduction in sperm count (23). However, other characteristics, including sperm motility, sperm capacitation, and the outcome of in vitro fertilization were normal, although there was a trend of slightly decreased successful fertilization (23). Consequently, the aforementioned findings, such as the decreased sperm counts and quality, could not serve as solid evidence to explain the infertility of male BMAL1-KO mice. Apart from that, BMAL1 might also directly affect the structure of sperm. The chromatoid body (CB) is a special structure in pachytene spermatocytes and spermatids, which plays a pivotal role in gametogenesis during meiosis and spermatogenesis (42–44). Of interest, BMAL1 depletion converted the CB into fragments in round spermatids (18), and BMAL1 expression declined with abnormal CB structures and functions with age (45). As CB functions in mRNA silencing and translational regulation, which are important for spermatogenesis, BMAL1 might interfere with such procedures in spermatogenesis (18). The abnormalities of the sperm might also contribute to the reduced fertility of BMAL1-KO mice. Furthermore, male BMAL1-KO mice failed to mate with receptive WT female mice, showing no mating behaviours (23, 25). The underlying mechanism will be outlined in the following section.
In conclusion, male BMAL1-KO mice exhibit defective gonads and abnormal mating behavior, factors that might account for male infertility (Figure 1).
BMAL1 in Hypothalamus-Pituitary-Gonad Regulatory Axis
Poor gonadal development and dysfunction usually indicate abnormal gonadotropins and GnRH levels. The secretion of gonadotropins, including luteinizing hormone (LH) and follicle-stimulating hormone (FSH), is controlled by upstream signals derived from the hypothalamus. There, GnRH is consistently recognized as the master regulator for the secretion of downstream gonadotropin, including FSH and LH derived from the pituitary (46–51). Additionally, another stimulator of gonadotropins, kisspeptin derived from Kiss1 neurons, especially in the anteroventral periventricular nucleus of the hypothalamus, is significantly associated with a GnRH surge and induces gonadotropins secretion; thus it could directly affect pituitary gonadotrophs to release LH and FSH (47, 49, 52–56).
Effect of BMAL1 Knockout on Hypothalamus/Pituitary Hormones in Mice
BMAL1-KO mice exhibited abnormal pituitary gonadotropin levels (15, 31, 57). In female mammals, ovulation occurs after a pro-estrous LH surge triggered by estradiol peaks (58). However, female BMAL1-KO mice did not present with an LH surge (57). Meanwhile, male BMAL1-KO mice exhibited significantly altered FSH levels both in serum and in the whole pituitary, with reduced mRNA expressions of FSHβ in the pituitary (23, 25). Thus, BMAL1 absence could affect the secretion of gonadotropin from the pituitary.
To determine the reasons for abnormal gonadotropin levels, more studies have been conducted by focusing on the signaling mechanisms in the hypothalamus. GnRH secretion in BMAL1-KO mice was in normal range (57). In the meantime, Kiss1 neurons show normal c-Fos expression in Female BMAL1-KO mice at the time of the LH surge, suggesting a normal responding state of these neurons (57). Furthermore, female BMAL1-KO mice showed an enhanced LH response to exogenous kisspeptin and a normally induced LH response to exogenous GnRH, suggesting unimpaired reactivity in the pituitary of these female mice and an even higher sensitivity to exogenous kisspeptin (57). Similarly, male BMAL1-KO mice also exhibited higher sensitivity to kisspeptin and GnRH, with normal expression of GnRH and Kisspeptin in the hypothalamus (25). Notably, the GnRH receptor (GnRHR) gene was established to contain an E-box in the promoter region, and was under the regulation of BMAL1 (59). In Female BMAL1-KO mice, the expression of GnRHR in the pituitary remained unchanged, but that in Male BMAL1-KO mice was reduced (25). Therefore, BMAL1 participates in signaling from the hypothalamus to the pituitary. However, in terms of the lost LH surge and the abnormal reactivity of the pituitary to the hypothalamus in BMAL1-KO mice, there might be some additional mechanisms underlying the abnormal signaling from the hypothalamus to the pituitary.
Deleting BMAL1 specifically in important cells associated with the GnRH/LH pathways also resulted in alterations in gonadotropin release and response patterns (60). Conditional BMAL1 knockout in arginine vasopressin (AVP) neurons, which were demonstrated to play a specific role in the LH surge, resulted in a low and delayed LH surge; In KISS1 neuron conditional BMAL1-KO mice, the secretion pattern with double peaks and an altered surge time of induced LH were noted. Furthermore, in GnRH-neuron conditional BMAL1-KO mice, the LH surge was noted disappeared at a specific time, and the amplitude was found to be low (60). Such cells played important roles in hypothalamus endocrine signal transmission and were also proved to participate in mechanisms involving in GnRH/LH signaling (60). Therefore, the altered timing and pattern of the LH surge in these mice indicated that BMAL1 in these cells was involved in the transmission and feedback of hormones from the hypothalamus to the pituitary.
Altogether, BMAL1 participates in hormone secretion and signaling of hypothalamus and pituitary.
Effect of BMAL1 Knockout on Hypothalamus-Pituitary Regulation of Reproduction in Mice
LH and FSH are considered pivotal signals for gonadal development, follicle development, spermatogenesis, gonadal steroidogenesis, constitute the key steps for reproductive outcomes (61–67).
Female BMAL1-KO mice did not have an LH surge, an important stimulus for periodic ovulation. It is possible that the impaired quantity and quality of the superovulated oocytes of female BMAL1-KO mice were associated with the phenomenon (15). Apart from ovulation, LH also plays a significant role in the maintenance of corpora lutea (68, 69). This was consistent with the reduced number of corpora lutea and the disordered structure of the ovaries (15). Moreover, the delayed puberty and the disordered estrous cycle might also be attributed to the disrupted production of hormone signals (15). Such abnormal hypothalamus and pituitary hormone secretion and signaling in female BMAL1-KO mice might be associated with the impaired fertility.
Male BMAL1-KO mice had higher levels of LH, a hormone that primarily functions through Leydig cells to produce testosterone for male reproduction. Thus, the higher LH level in male BMAL1-KO mice was in line with the comparatively low testosterone levels. FSH facilitates male reproduction by promoting the proliferation of Sertoli cells and spermatogonia, thereby increasing sperm counts in synergy with testosterone (70–72). Therefore, it is possible that the lower levels of FSH and testosterone in male BMAL1-KO mice were responsible for the decreased sperm counts. Moreover, male BMAL1-KO mice showed reduced hypothalamic expressions of vasoactive intestinal peptide (Vip) and tyrosine hydroxylase (Th) (25), both of which are associated with the mating behaviors of male mice (73–75). The abnormal hypothalamus and pituitary hormone secretion and signaling of male BMAL1-KO mice were possibly associated with low sperm counts and impaired mating behaviors in these mice.
Altogether, BMAL1 may affect hormone secretion and signaling of hypothalamus and pituitary, thus affecting the fertility of BMAL1-KO mice.
BMAL1 in Gonadal Hormone Production
Reproductive activities are usually associated with gonadal hormones. Indeed, abnormal gonadal hormone levels might directly result in severe defects in the reproduction of BMAL1-KO mice.
Effect of BMAL1 Deficiency on Gonadal Hormone Secretion and Actions
In females, progesterone and estradiol are considered crucial hormones for embryo implantation and maintenance (39). However, BMAL1 depletion impaired female gonadal hormone secretion. Indeed, female BMAL1-KO mice showed decreased progesterone levels during the estrus cycle and early gestation (15, 22, 76). BMAL1 knockdown by small interfering RNA (siRNA) in in vitro cultured porcine granulosa cells and luteinizing granulosa cells of rat and human, also led to a decrease in progesterone and estradiol synthesis (21, 77, 78). Additionally, evidence suggests that leptin and leptin receptor (Lepr) exerted positive effects on estradiol synthesis in murine granulosa cells, whereas BMAL1 knockdown reduced estradiol synthesis and suppressed the stimulating effect of leptin and lepr on estradiol synthesis and associated gene expressions (20). Therefore, BMAL1 plays a role in the process by which leptin signaling induces estradiol synthesis (20). Another hormone, prostaglandin E2 (PGE2), was also found to be reduced in the reproductive tissues of female BMAL1-KO mice (21, 79). Regulated by rate-limiting enzymes encoded by the gene prostaglandin-endoperoxide synthase 2/cyclooxygenase 2 (ptgs2 or cox2), PGE2 has been deemed vital for ovulatory cascade, sperm penetration, and fertilization, and decidual preparation for implantation (80–84). Moreover, in vitro cultured BMAL1 rat luteinizing granulosa cells and BMAL1-KO uterus cells exhibited considerably downregulated ptgs2 expression, and PGE2 secretion (21, 79). Therefore, BMAL1 is important for female gonadal hormone secretion, which could directly account for the impaired fertility of female BMAL1-KO mice. Female BMAL1-KO mice were reported to undergo unsuccessful implantation. Exogenous supplementation with progesterone substantially rescued the implantation failure, although the recovered implantation sites were smaller and fewer compared to those noted in the WT group (22). Similarly, in female steroidogenic factor-1 (SF1) conditional BMAL1-KO mice, also characterized by poor steroidogenesis as well as failed implantation, exogenous progesterone supplement could greatly improve the implantation rate (76). Furthermore, progesterone was found to participate in the uterus decidualization pathways (39, 84–86), which are influenced by BMAL1 depletion (27). Thus, the impaired steroidogenesis due to BMAL1 loss might underlie the failed implantation of BMAL1-KO mice.
In males, testosterone also plays a crucial role in testis development and spermatogenesis (87–89). In line with the morphological abnormalities of the testis and seminal vesicles, male BMAL1-KO mice showed reduced testosterone secretion (23, 25, 90). In fact, BMAL1 was reported to be only expressed and oscillate in Leydig cells which account for testosterone production (19, 23), whereas BMAL1 oscillation in the whole testis was demonstrated to be arrhythmic (91, 92). In goat Leydig cells and in vitro cultured TM3 Leydig cells, BMAL1 knockdown led to a reduction in testosterone levels (41, 93). Thus, BMAL1 loss could be responsible for the poor gonadal hormone secretion in male BMAL1-KO mice.
Overall, the existing evidence for implantation related infertility in BMAL1-KO mice can be considered closely associated with the abnormal secretion of reproductive hormones.
Effect of BMAL1 Deficiency on Gonadal Hormone Genes Expression
In line with the impaired steroidogenesis, BMAL1-KO and interference altered the expression of most steroidogenic genes, including steroidogenic acute regulatory protein (StAR), cytochrome P450 family 11 subfamily A member 1(Cyp11a1), 3β-hydroxysteroid dehydrogenase 2 (Hsd3b2), 17-β-hydroxysteroid dehydrogenase 3 (Hsd17B3), cytochrome P450 family 17 subfamily A member 1(Cyp17a1), and cytochrome P450 aromatase (Cyp19a1). BMAL1-KO mice also altered the expression of related hormone receptor genes, including follicle-stimulating hormone receptor (FSHr), luteinizing hormone receptor (LHr, Lhcgr), and estrogen receptor β (ERβ) (15, 20–23, 41, 77, 94, 95) (Table 1). For example, BMAL1 knockdownin goat Leydig cells reduced testosterone production and the expression of StAR and HSD3B2, whereas BMAL1 overexpression enhanced testosterone production and the expression of StAR and Hsd17b2 (93). In rat luteinizing granulosa cells, BMAL1 knockdown suppressed the expression of a series of ovarian genes, such as StAR, Cyp19a1, Cyp11a1, Ptgs2, Lhcgr, and Hsd3b2, with reduced progesterone and PGE2.
Among steroidogenic genes, StAR is necessary for cholesterol transfer into the mitochondrial membrane (101). Both male and female BMAL1-KO mice reduced StAR protein and mRNA expression both in vivo and in vitro (15, 21–23, 41). Moreover, the StAR gene contains an E-box element near its promoter region, and BMAL1 can directly induce its transcription (23, 96, 97). Similarly, the expression of HSD17B3 was also demonstrated to be directly regulated by BMAL1 (93). Erβ, a major estradiol receptor type in the SCN, plays a role in reproduction, especially in follicle maturation (102–105). With an E-box in its promoter, BMAL1 was demonstrated to directly regulate Erβ expression (99). Lepr also contains an E-box in its promoter and is subjected to regulation by BMAL1 (20). In summary, BMAL1 can affect steroidogenesis and hormone signaling by directly affecting certain steroidogenesis related genes.
Effect of BMAL1 Deficiency on Signaling Pathways Associated With Steroidogenesis
Silencing information regulator 2 related enzyme 1 (sirtuin1, SIRT1) is an NAD+-dependent deacetylase; it collaborates with BMAL1 in the circadian regulation (106–109). It has already been established that SIRT1 participates in the steroidogenic function of estrogen-producing cells. Further evidence confirmed that SIRT1-BMAL1 signaling is involved in estradiol production in steroidogenic cells, by regulating the c-Jun N-terminal kinase (JNK) pathway (78). Moreover, BMAL1, SIRT1, and the JNK pathways seem to mutually affect each other, forming a BMAL1-SIRT1-JNK loop that functions in gonadal steroidogenesis (78).
Studies have revealed that the PI3K/AKT/mTOR pathway participates in the biological processes of apoptosis and steroidogenesis in granulosa and Leydig cells (110–114). Coincidently, in the in vitro cell culture of porcine granulosa cells and the TM3 Leydig cell line, the PI3K/AKT/mTOR pathway was suppressed after intervention of BMAL1,with reduced steroidogenesis and increased apoptosis of steroidogenic cells (41, 77). Notably, this pathway was also reported to be involved in leptin-lepr signaling in the regulation of steroidogenesis (20). Therefore, BMAL1 might have functions in the PI3K/AKT/mTOR pathway and thus play a role in the apoptosis of gonadal steroid hormone-producing cells and steroidogenesis.
The PI3K/NF-κB pathway is also involved in steroidogenesis. NF-κB was demonstrated to participate in circadian rhythm generation and was also associated with the PI3K/AKT/mTORC1 pathway, which functions in steroid hormone production (110–114). Female BMAL1-KO mice exhibited impaired steroidogenesis and activated phosphorylation of the PI3K/NF-κB pathway. Moreover, data from the in vitro cultured mice theca and granulosa cells confirmed that the BMAL1 knockdown increased phosphorylation of the PI3K/NF-κB pathway, along with severely impaired luteal steroidogenesis (95). Further evidence indicated that BMAL1 might directly establish negative interactions with NF-κB p (RelA), a subunit of NF-κB (95). Therefore, BMAL1 is also involved in the regulation of PI3K/NF-κB pathway, inducing steroidogenesis.
BMAL1 in the Etiology of Human Reproductive Pathogenesis
Accumulating clinical evidence has demonstrated the role of BMAL1 in human reproductive pathologies. Single nucleotide polymorphisms (SNPs) of circadian genes revealed that BMAL1 is associated with all epithelial ovarian cancers (EOCs) and their histopathologic subtypes (28). Moreover, BMAL1 was obviously downregulated in an early stage transformed model of EOCs (28). One variant of BMAL1 was previously linked to a higher number of pregnancies and a higher number of miscarriages (29). In addition, BMAL1 expression was downregulated in recurrent miscarriage patients (26), and BMAL1 was revealed to affect trophoblast invasion during decidualization by regulating TIMP3, a protein that controls the invasiveness of trophoblast cells (26). Another mechanistic study revealed that BMAL1 facilitated the migration and invasion of the extra-villous trophoblast (EVT) via the SP1-DNMT1/DAB2IP pathway, which could be the underlying mechanism through which progesterone treatment prevents spontaneous abortion (27).
Conclusions and Prospects
As current review has indicated, BMAL1 knockout leads to impaired structures and functions of reproductive organs and gametes, signaling from the hypothalamus to pituitary impaired gonadal hormone secretion and impaired hormone secretion. Furthermore, dysfunctional BMAL1 was demonstrated to participate in human reproductive diseases. These findings revealed the critical role of BMAL1 in reproductive endocrinology and fertility. Still, the exact underlying mechanisms of how BMAL1 impacts on reproduction need to be further explored. The disparate phenotypes in different BMAL1-KO models (Table 2) have offered us deep insights into the role of BMAL1 in reproduction. Among these mice, global BMAL1-KO mice and steroidogenesis cells conditional BMAL1-KO (SF1- KO) mice were infertile, while other conditional BMAL1-KO mouse models were fertile such as the induced BMAL1-KO (iKO) mice (115). The iKO mice were treated with tamoxifen (TAM) at 8-weeks of age, thus losing BMAL1 in adulthood, and in these animals, reproductive tissues and reproductive endocrine systems are well-developed while devoid of BMAL1. However, BMAL1-KO mice had impaired reproductive tissues and gametes, as well as impaired reproductive endocrine systems. Thus, BMAL1 has a critical role in reproductive endocrinology and fertility.
As the core transcription factor of the circadian rhythm, BMAL1 also serves its circadian function in regulating the rhythm of reproductive endocrine hormones. Indeed, reproductive endocrine hormones like estradiol (20), and testosterone (118, 119), have been demonstrated to be rhythmically released. And the associated steroidogenic genes showed robust circadian rhythm in corresponding steroidogenic cells (20, 90, 93). Moreover, the disruption of BMAL1 by siRNA in mice steroidogenic cells led to the disrupted rhythm of associated genes including StAR, Cyp11a1, Hsd3b2, and Hsd17b3 (20, 90, 93). Additionally, another gene family crucial for cholesterol transportation to produce testosterone in the testis, namely apolipoprotein (Apo) genes (including Apoa1, Apoa2 and Apoc3), was reported to be expressed in a robust circadian manner in WT mice (90). In BMAL1-KO mice, the rhythmicity of the expressions of Apo genes is lost, along with impaired metabolism of cholesterol, which might be associated with the reduced testosterone in Male BMAL1-KO mice (90). Accordingly, the circadian gene BMAL1 can also influence reproductive endocrinology by regulating the rhythmic expression of corresponding genes.
In conclusion, the established mouse model and the mechanistic studies have provided insights into the role of BMAL1 in reproduction. This could have clinical significance, as more reproductive pathologies can be attributed to defects at the gene level, such as the key role of BMAL1 outlined in this review. It is anticipated that a deeper understanding of the role of BMAL1 in reproduction could be applied to clinical practices in reproductive disease pathology and target therapies in the future.
Author Contributions
DM and JL devised topic. YJ and SL wrote the draft. JY and YJ devised tables. AZ, YY, RZ, and TR devised figures. WX, YQ, and XJ revised for accuracy. DM financially support this work. All authors contributed to the article and approved the submitted version.
Funding
This study was partially supported by National Natural Science Foundation of China (81971433, 81701500); the grants from the Science and Technology Bureau of Sichuan Province (2021YJ0017, 2020YFS0041).
Conflict of Interest
The authors declare that the research was conducted in the absence of any commercial or financial relationships that could be construed as a potential conflict of interest.
Publisher’s Note
All claims expressed in this article are solely those of the authors and do not necessarily represent those of their affiliated organizations, or those of the publisher, the editors and the reviewers. Any product that may be evaluated in this article, or claim that may be made by its manufacturer, is not guaranteed or endorsed by the publisher.
References
1. Yoo SH, Yamazaki S, Lowrey PL, Shimomura K, Ko CH, Buhr ED, et al. PERIOD2::LUCIFERASE Real-Time Reporting of Circadian Dynamics Reveals Persistent Circadian Oscillations in Mouse Peripheral Tissues. Proc Natl Acad Sci USA (2004) 101(15):5339–46. doi: 10.1073/pnas.0308709101
2. Stetson MH, Watson-Whitmyre M. Nucleus Suprachiasmaticus: The Biological Clock in the Hamster? Sci (New York NY) (1976) 191(4223):197–9. doi: 10.1126/science.942799
3. Inouye ST, Kawamura H. Persistence of Circadian Rhythmicity in a Mammalian Hypothalamic “Island” Containing the Suprachiasmatic Nucleus. Proc Natl Acad Sci USA (1979) 76(11):5962–6. doi: 10.1073/pnas.76.11.5962
4. Hogenesch JB, Gu YZ, Jain S, Bradfield CA. The Basic-Helix-Loop-Helix-PAS Orphan MOP3 Forms Transcriptionally Active Complexes With Circadian and Hypoxia Factors. Proc Natl Acad Sci USA (1998) 95(10):5474–9. doi: 10.1073/pnas.95.10.5474
5. Gekakis N, Staknis D, Nguyen HB, Davis FC, Wilsbacher LD, King DP, et al. Role of the CLOCK Protein in the Mammalian Circadian Mechanism. Sci (New York NY) (1998) 280(5369):1564–9. doi: 10.1126/science.280.5369.1564
6. Kewley RJ, Whitelaw ML, Chapman-Smith A. The Mammalian Basic Helix-Loop-Helix/PAS Family of Transcriptional Regulators. Int J Biochem Cell Biol (2004) 36(2):189–204. doi: 10.1016/s1357-2725(03)00211-5
7. Bunger MK, Wilsbacher LD, Moran SM, Clendenin C, Radcliffe LA, Hogenesch JB, et al. Mop3 Is an Essential Component of the Master Circadian Pacemaker in Mammals. Cell (2000) 103(7):1009–17. doi: 10.1016/s0092-8674(00)00205-1
8. Kondratov RV. A Role of the Circadian System and Circadian Proteins in Aging. Ageing Res Rev (2007) 6(1):12–27. doi: 10.1016/j.arr.2007.02.003
9. Kondratov RV, Kondratova AA, Gorbacheva VY, Vykhovanets OV, Antoch MP. Early Aging and Age-Related Pathologies in Mice Deficient in BMAL1, the Core Componentof the Circadian Clock. Genes Dev (2006) 20(14):1868–73. doi: 10.1101/gad.1432206
10. Li X, Liu N, Gu B, Hu W, Li Y, Guo B, et al. BMAL1 Regulates Balance of Osteogenic-Osteoclastic Function of Bone Marrow Mesenchymal Stem Cells in Type 2 Diabetes Mellitus Through the NF-κb Pathway. Mol Biol Rep (2018) 45(6):1691–704. doi: 10.1007/s11033-018-4312-7
11. Samsa WE, Vasanji A, Midura RJ, Kondratov RV. Deficiency of Circadian Clock Protein BMAL1 in Mice Results in a Low Bone Mass Phenotype. Bone (2016) 84:194–203. doi: 10.1016/j.bone.2016.01.006
12. Chen G, Tang Q, Yu S, Xie Y, Sun J, Li S, et al. The Biological Function of BMAL1 in Skeleton Development and Disorders. Life Sci (2020) 253:117636. doi: 10.1016/j.lfs.2020.117636
13. Watabe Y, Tomioka M, Watabe A, Aihara M, Shimba S, Inoue H. The Clock Gene Brain and Muscle Arnt-Like Protein-1 (BMAL1) Is Involved in Hair Growth. Arch Dermatol Res (2013) 305(8):755–61. doi: 10.1007/s00403-013-1403-0
14. Geyfman M, Andersen B. Clock Genes, Hair Growth and Aging. Aging (2010) 2(3):122–8. doi: 10.18632/aging.100130
15. Boden MJ, Varcoe TJ, Voultsios A, Kennaway DJ. Reproductive Biology of Female Bmal1 Null Mice. Reproduction (2010) 139(6):1077–90. doi: 10.1530/rep-09-0523
16. Boden MJ, Kennaway DJ. Circadian Rhythms and Reproduction. Reproduction (2006) 132(3):379–92. doi: 10.1530/rep.1.00614
17. Kennaway DJ, Boden MJ, Varcoe TJ. Circadian Rhythms and Fertility. Mol Cell Endocrinol (2012) 349(1):56–61. doi: 10.1016/j.mce.2011.08.013
18. Peruquetti RL, de Mateo S, Sassone-Corsi P. Circadian Proteins CLOCK and BMAL1 in the Chromatoid Body, a RNA Processing Granule of Male Germ Cells. PloS One (2012) 7(8):e42695. doi: 10.1371/journal.pone.0042695
19. Chen H, Gao L, Xiong Y, Yang D, Li C, Wang A, et al. Circadian Clock and Steroidogenic-Related Gene Expression Profiles in Mouse Leydig Cells Following Dexamethasone Stimulation. Biochem Biophys Res Commun (2017) 483(1):294–300. doi: 10.1016/j.bbrc.2016.12.149
20. Chu G, Ma G, Sun J, Zhu Y, Xiang A, Yang G, et al. Leptin Receptor Mediates Bmal1 Regulation of Estrogen Synthesis in Granulosa Cells. Animals: Open Access J MDP (2019) 9(11):899. doi: 10.3390/ani9110899
21. Chen H, Zhao L, Kumazawa M, Yamauchi N, Shigeyoshi Y, Hashimoto S, et al. Downregulation of Core Clock Gene Bmal1 Attenuates Expression of Progesterone and Prostaglandin Biosynthesis-Related Genes in Rat Luteinizing Granulosa Cells. Am J Physiol Cell Physiol (2013) 304(12):C1131–40. doi: 10.1152/ajpcell.00008.2013
22. Ratajczak CK, Boehle KL, Muglia LJ. Impaired Steroidogenesis and Implantation Failure in Bmal1-/- Mice. Endocrinology (2009) 150(4):1879–85. doi: 10.1210/en.2008-1021
23. Alvarez JD, Hansen A, Ord T, Bebas P, Chappell PE, Giebultowicz JM, et al. The Circadian Clock Protein BMAL1 Is Necessary for Fertility and Proper Testosterone Production in Mice. J Biol Rhythms (2008) 23(1):26–36. doi: 10.1177/0748730407311254
24. Choe HK, Kim HD, Park SH, Lee HW, Park JY, Seong JY, et al. Synchronous Activation of Gonadotropin-Releasing Hormone Gene Transcription and Secretion by Pulsatile Kisspeptin Stimulation. Proc Natl Acad Sci USA (2013) 110(14):5677–82. doi: 10.1073/pnas.1213594110
25. Schoeller EL, Clark DD, Dey S, Cao NV, Semaan SJ, Chao LW, et al. Bmal1 Is Required for Normal Reproductive Behaviors in Male Mice. Endocrinology (2016) 157(12):4914–29. doi: 10.1210/en.2016-1620
26. Lv S, Wang N, Ma J, Li WP, Chen ZJ, Zhang C. Impaired Decidualization Caused by Downregulation of Circadian Clock Gene BMAL1 Contributes to Human Recurrent Miscarriage†. Biol Reprod (2019) 101(1):138–47. doi: 10.1093/biolre/ioz063
27. Li S, Zhai J, Liu J, Hong Y, Zhao W, Zhao A, et al. BMAL1 Facilitates Trophoblast Migration and Invasion via SP1-DNMT1/DAB2IP Pathway in Recurrent Spontaneous Abortion. Oncotarget (2017) 8(52):89451–64. doi: 10.18632/oncotarget.20702
28. Jim HS, Lin HY, Tyrer JP, Lawrenson K, Dennis J, Chornokur G, et al. Common Genetic Variation in Circadian Rhythm Genes and Risk of Epithelial Ovarian Cancer (EOC). J Genet Genome Res (2015) 2(2):017. doi: 10.23937/2378-3648/1410017
29. Kovanen L, Saarikoski ST, Aromaa A, Lönnqvist J, Partonen T. ARNTL (BMAL1) and NPAS2 Gene Variants Contribute to Fertility and Seasonality. PloS One (2010) 5(4):e10007. doi: 10.1371/journal.pone.0010007
30. Kennaway DJ. The Role of Circadian Rhythmicity in Reproduction. Hum Reprod Update (2005) 11(1):91–101. doi: 10.1093/humupd/dmh054
31. Chu A, Zhu L, Blum ID, Mai O, Leliavski A, Fahrenkrug J, et al. Global But Not Gonadotrope-Specific Disruption of Bmal1 Abolishes the Luteinizing Hormone Surge Without Affecting Ovulation. Endocrinology (2013) 154(8):2924–35. doi: 10.1210/en.2013-1080
32. Xu J, Li Y, Wang Y, Xu Y, Zhou C. Loss of Bmal1 Decreases Oocyte Fertilization, Early Embryo Development and Implantation Potential in Female Mice. Zygote (Cambridge Engl) (2016) 24(5):760–7. doi: 10.1017/s0967199416000083
33. Miller BH, Takahashi JS. Central Circadian Control of Female Reproductive Function. Front Endocrinol (2013) 4:195. doi: 10.3389/fendo.2013.00195
34. Khapre RV, Kondratova AA, Susova O, Kondratov RV. Circadian Clock Protein Bmal1 Regulates Cellular Senescence In Vivo. Cell Cycle (Georgetown Tex) (2011) 10(23):4162–9. doi: 10.4161/cc.10.23.18381
35. Balaban RS, Nemoto S, Finkel T. Mitochondria, Oxidants, and Aging. Cell (2005) 120(4):483–95. doi: 10.1016/j.cell.2005.02.001
36. Shkolnik K, Tadmor A, Ben-Dor S, Nevo N, Galiani D, Dekel N. Reactive Oxygen Species Are Indispensable in Ovulation. Proc Natl Acad Sci USA (2011) 108(4):1462–7. doi: 10.1073/pnas.1017213108
37. Ruder EH, Hartman TJ, Goldman MB. Impact of Oxidative Stress on Female Fertility. Curr Opin Obstet Gynecol (2009) 21(3):219–22. doi: 10.1097/gco.0b013e32832924ba
38. Tamura H, Takasaki A, Miwa I, Taniguchi K, Maekawa R, Asada H, et al. Oxidative Stress Impairs Oocyte Quality and Melatonin Protects Oocytes From Free Radical Damage and Improves Fertilization Rate. J Pineal Res (2008) 44(3):280–7. doi: 10.1111/j.1600-079X.2007.00524.x
39. Ramathal CY, Bagchi IC, Taylor RN, Bagchi MK. Endometrial Decidualization: Of Mice and Men. Semin Reprod Med (2010) 28(1):17–26. doi: 10.1055/s-0029-1242989
40. Kim SM, Kim JS. A Review of Mechanisms of Implantation. Dev Reprod (2017) 21(4):351–9. doi: 10.12717/dr.2017.21.4.351
41. Ding H, Zhao J, Liu H, Wang J, Lu W. BMAL1 Knockdown Promoted Apoptosis and Reduced Testosterone Secretion in TM3 Leydig Cell Line. Gene (2020) 747:144672. doi: 10.1016/j.gene.2020.144672
42. Fujii Y, Fujita H, Yokota S. Synthesis of β-Tubulin Occurs Within Chromatoid Body of Round Spermatids. Cytoskeleton (Hoboken NJ) (2017) 74(5):197–204. doi: 10.1002/cm.21363
43. Peruquetti RL. Perspectives on Mammalian Chromatoid Body Research. Anim Reprod Sci (2015) 159:8–16. doi: 10.1016/j.anireprosci.2015.05.018
44. Onohara Y, Fujiwara T, Yasukochi T, Himeno M, Yokota S. Localization of Mouse Vasa Homolog Protein in Chromatoid Body and Related Nuage Structures of Mammalian Spermatogenic Cells During Spermatogenesis. Histochem Cell Biol (2010) 133(6):627–39. doi: 10.1007/s00418-010-0699-5
45. Santos EG, Silva MA, Amorim RP, Giordano LS, Silva DS, Rasmussen LT, et al. Aging and Chromatoid Body Assembly: Are These Two Physiological Events Linked? Exp Biol Med (Maywood NJ) (2018) 243(11):917–25. doi: 10.1177/1535370218784871
46. Clarke IJ, Pompolo S. Synthesis and Secretion of Gnrh. Anim Reprod Sci (2005) 88(1-2):29–55. doi: 10.1016/j.anireprosci.2005.05.003
47. Karsch FJ, Bowen JM, Caraty A, Evans NP, Moenter SM. Gonadotropin-Releasing Hormone Requirements for Ovulation. Biol Reprod (1997) 56(2):303–9. doi: 10.1095/biolreprod56.2.303
48. Moenter SM, Caraty A, Locatelli A, Karsch FJ. Pattern of Gonadotropin-Releasing Hormone (Gnrh) Secretion Leading Up to Ovulation in the Ewe: Existence of a Preovulatory Gnrh Surge. Endocrinology (1991) 129(3):1175–82. doi: 10.1210/endo-129-3-1175
49. Barbacka-Surowiak G, Surowiak J, Stokłosowa S. The Involvement of Suprachiasmatic Nuclei in the Regulation of Estrous Cycles in Rodents. Reprod Biol (2003) 3(2):99–129.
50. Legan SJ, Karsch FJ. A Daily Signal for the LH Surge in the Rat. Endocrinology (1975) 96(1):57–62. doi: 10.1210/endo-96-1-57
51. Pawson AJ, McNeilly AS. The Pituitary Effects of Gnrh. Anim Reprod Sci (2005) 88(1-2):75–94. doi: 10.1016/j.anireprosci.2005.05.010
52. Li XF, Kinsey-Jones JS, Cheng Y, Knox AM, Lin Y, Petrou NA, et al. Kisspeptin Signalling in the Hypothalamic Arcuate Nucleus Regulates Gnrh Pulse Generator Frequency in the Rat. PloS One (2009) 4(12):e8334. doi: 10.1371/journal.pone.0008334
53. Keen KL, Wegner FH, Bloom SR, Ghatei MA, Terasawa E. An Increase in Kisspeptin-54 Release Occurs With the Pubertal Increase in Luteinizing Hormone-Releasing Hormone-1 Release in the Stalk-Median Eminence of Female Rhesus Monkeys In Vivo. Endocrinology (2008) 149(8):4151–7. doi: 10.1210/en.2008-0231
54. Messager S, Chatzidaki EE, Ma D, Hendrick AG, Zahn D, Dixon J, et al. Kisspeptin Directly Stimulates Gonadotropin-Releasing Hormone Release via G Protein-Coupled Receptor 54. Proc Natl Acad Sci USA (2005) 102(5):1761–6. doi: 10.1073/pnas.0409330102
55. Roa J, Castellano JM, Navarro VM, Handelsman DJ, Pinilla L, Tena-Sempere M. Kisspeptins and the Control of Gonadotropin Secretion in Male and Female Rodents. Peptides (2009) 30(1):57–66. doi: 10.1016/j.peptides.2008.08.009
56. Clayton RN. Mechanism of Gnrh Action in Gonadotrophs. Hum Reprod (Oxf Engl) (1988) 3(4):479–83. doi: 10.1093/oxfordjournals.humrep.a136732
57. Tonsfeldt KJ, Schoeller EL, Brusman LE, Cui LJ, Lee J, Mellon PL. The Contribution of the Circadian Gene Bmal1 to Female Fertility and the Generation of the Preovulatory Luteinizing Hormone Surge. J Endocrine Soc (2019) 3(4):716–33. doi: 10.1210/js.2018-00228
58. Smarr BL, Gile JJ, de la Iglesia HO. Oestrogen-Independent Circadian Clock Gene Expression in the Anteroventral Periventricular Nucleus in Female Rats: Possible Role as an Integrator for Circadian and Ovarian Signals Timing the Luteinising Hormone Surge. J Neuroendocrinol (2013) 25(12):1273–9. doi: 10.1111/jne.12104
59. Resuehr D, Wildemann U, Sikes H. Olcese J. E-Box Regulation of Gonadotropin-Releasing Hormone (Gnrh) Receptor Expression in Immortalized Gonadotrope Cells. Mol Cell Endocrinol (2007) 278(1-2):36–43. doi: 10.1016/j.mce.2007.08.008
60. Bittman EL. Circadian Function in Multiple Cell Types Is Necessary for Proper Timing of the Preovulatory LH Surge. J Biol Rhythms (2019) 34(6):622–33. doi: 10.1177/0748730419873511
61. McGee EA, Hsueh AJ. Initial and Cyclic Recruitment of Ovarian Follicles. Endocrine Rev (2000) 21(2):200–14. doi: 10.1210/edrv.21.2.0394
62. Hansson V, Djoseland O, Torgersen O, Ritzen EM, French FS, Nayfeh SN. Hormones and Hormonal Target Cells in the Testis. Andrologia (1976) 8(3):195–202. doi: 10.1111/j.1439-0272.1976.tb02135.x
63. Koskenniemi JJ, Virtanen HE, Toppari J. Testicular Growth and Development in Puberty. Curr Opin Endocrinol Diabetes Obes (2017) 24(3):215–24. doi: 10.1097/med.0000000000000339
64. de Kretser DM. Endocrinology of Male Infertility. Br Med Bull (1979) 35(2):187–92. doi: 10.1093/oxfordjournals.bmb.a071568
65. Wong WJ, Khan YS. Histology, Sertoli Cell. Statpearls. Treasure Island, FL: StatPearls Publishing Copyright © 2021, StatPearls Publishing LLC (2021).
66. Robertson JL, Clifton DK, de la Iglesia HO, Steiner RA, Kauffman AS. Circadian Regulation of Kiss1 Neurons: Implications for Timing the Preovulatory Gonadotropin-Releasing Hormone/Luteinizing Hormone Surge. Endocrinology (2009) 150(8):3664–71. doi: 10.1210/en.2009-0247
67. Russell DL, Robker RL. Molecular Mechanisms of Ovulation: Co-Ordination Through the Cumulus Complex. Hum Reprod Update (2007) 13(3):289–312. doi: 10.1093/humupd/dml062
68. Cooke ID. The Corpus Luteum. Hum Reprod (Oxf Engl) (1988) 3(2):153–6. doi: 10.1093/oxfordjournals.humrep.a136665
69. Keyes PL, Wiltbank MC. Endocrine Regulation of the Corpus Luteum. Annu Rev Physiol (1988) 50:465–82. doi: 10.1146/annurev.ph.50.030188.002341
70. Huhtaniemi I. A Short Evolutionary History of FSH-Stimulated Spermatogenesis. Hormones (Athens Greece) (2015) 14(4):468–78. doi: 10.14310/horm.2002.1632
71. Shiraishi K, Matsuyama H. Gonadotoropin Actions on Spermatogenesis and Hormonal Therapies for Spermatogenic Disorders [Review]. Endocrine J (2017) 64(2):123–31. doi: 10.1507/endocrj.EJ17-0001
72. Zirkin BR. Spermatogenesis: Its Regulation by Testosterone and FSH. Semin Cell Dev Biol (1998) 9(4):417–21. doi: 10.1006/scdb.1998.0253
73. Gozes I, Meltzer E, Rubinrout S, Brenneman DE, Fridkin M. Vasoactive Intestinal Peptide Potentiates Sexual Behavior: Inhibition by Novel Antagonist. Endocrinology (1989) 125(6):2945–9. doi: 10.1210/endo-125-6-2945
74. Argiolas A. Neuropeptides and Sexual Behaviour. Neurosci Biobehav Rev (1999) 23(8):1127–42. doi: 10.1016/s0149-7634(99)00068-8
75. Argiolas A, Melis MR. Neuropeptides and Central Control of Sexual Behaviour From the Past to the Present: A Review. Prog Neurobiol (2013) 108:80–107. doi: 10.1016/j.pneurobio.2013.06.006
76. Liu Y, Johnson BP, Shen AL, Wallisser JA, Krentz KJ, Moran SM, et al. Loss of BMAL1 in Ovarian Steroidogenic Cells Results in Implantation Failure in Female Mice. Proc Natl Acad Sci USA (2014) 111(39):14295–300. doi: 10.1073/pnas.1209249111
77. Wang W, Yin L, Bai L, Ma G, Zhao C, Xiang A, et al. Bmal1 Interference Impairs Hormone Synthesis and Promotes Apoptosis in Porcine Granulosa Cells. Theriogenology (2017) 99:63–8. doi: 10.1016/j.theriogenology.2017.05.010
78. Zhang J, Liu J, Zhu K, Hong Y, Sun Y, Zhao X, et al. Effects of BMAL1-SIRT1-Positive Cycle on Estrogen Synthesis in Human Ovarian Granulosa Cells: An Implicative Role of BMAL1 in PCOS. Endocrine (2016) 53(2):574–84. doi: 10.1007/s12020-016-0961-2
79. Zhao L, Yang L, Zhang J, Xiao Y, Wu M, Ma T, et al. Bmal1 Promotes Prostaglandin E(2) Synthesis by Upregulating Ptgs2 Transcription in Response to Increasing Estradiol Levels in Day 4 Pregnant Mice. Am J Physiol Endocrinol Metab (2021) 320(4):E747–e59. doi: 10.1152/ajpendo.00466.2020
80. Gnecco JS, Ding T, Smith C, Lu J, Bruner-Tran KL, Osteen KG. Hemodynamic Forces Enhance Decidualization via Endothelial-Derived Prostaglandin E2 and Prostacyclin in a Microfluidic Model of the Human Endometrium. Hum Reprod (Oxf Engl) (2019) 34(4):702–14. doi: 10.1093/humrep/dez003
81. Kennedy TG, Gillio-Meina C, Phang SH. Prostaglandins and the Initiation of Blastocyst Implantation and Decidualization. Reproduction (2007) 134(5):635–43. doi: 10.1530/rep-07-0328
82. Sugimoto Y, Inazumi T, Tsuchiya S. Roles of Prostaglandin Receptors in Female Reproduction. J Biochem (2015) 157(2):73–80. doi: 10.1093/jb/mvu081
83. Boruszewska D, Kowalczyk-Zieba I, Suwik K, Staszkiewicz-Chodor J, Jaworska J, Lukaszuk K, et al. Prostaglandin E(2) Affects In Vitro Maturation of Bovine Oocytes. Reprod Biol Endocrinol: RB&E (2020) 18(1):40. doi: 10.1186/s12958-020-00598-9
84. Norwitz ER, Schust DJ, Fisher SJ. Implantation and the Survival of Early Pregnancy. N Engl J Med (2001) 345(19):1400–8. doi: 10.1056/NEJMra000763
85. Gellersen B, Brosens JJ. Cyclic Decidualization of the Human Endometrium in Reproductive Health and Failure. Endocrine Rev (2014) 35(6):851–905. doi: 10.1210/er.2014-1045
86. Bhurke AS, Bagchi IC, Bagchi MK. Progesterone-Regulated Endometrial Factors Controlling Implantation. Am J Reprod Immunol (New York NY: 1989) (2016) 75(3):237–45. doi: 10.1111/aji.12473
87. Zirkin BR, Papadopoulos V. Leydig Cells: Formation, Function, and Regulation. Biol Reprod (2018) 99(1):101–11. doi: 10.1093/biolre/ioy059
88. Walker WH. Non-Classical Actions of Testosterone and Spermatogenesis. Philos Trans R Soc Lond Ser B Biol Sci (2010) 365(1546):1557–69. doi: 10.1098/rstb.2009.0258
89. Smith LB, Walker WH. The Regulation of Spermatogenesis by Androgens. Semin Cell Dev Biol (2014) 30:2–13. doi: 10.1016/j.semcdb.2014.02.012
90. Yang L, Ma T, Zhao L, Jiang H, Zhang J, Liu D, et al. Circadian Regulation of Apolipoprotein Gene Expression Affects Testosterone Production in Mouse Testis. Theriogenology (2021) 174:9–19. doi: 10.1016/j.theriogenology.2021.06.023
91. Morse D, Cermakian N, Brancorsini S, Parvinen M, Sassone-Corsi P. No Circadian Rhythms in Testis: Period1 Expression Is Clock Independent and Developmentally Regulated in the Mouse. Mol Endocrinol (Baltimore Md) (2003) 17(1):141–51. doi: 10.1210/me.2002-0184
92. Alvarez JD, Chen D, Storer E, Sehgal A. Non-Cyclic and Developmental Stage-Specific Expression of Circadian Clock Proteins During Murine Spermatogenesis. Biol Reprod (2003) 69(1):81–91. doi: 10.1095/biolreprod.102.011833
93. Xiao Y, Zhao L, Li W, Wang X, Ma T, Yang L, et al. Circadian Clock Gene BMAL1 Controls Testosterone Production by Regulating Steroidogenesis-Related Gene Transcription in Goat Leydig Cells. J Cell Physiol (2021) 236(9):6706–25. doi: 10.1002/jcp.30334
94. Hasegawa T, Zhao L, Caron KM, Majdic G, Suzuki T, Shizawa S, et al. Developmental Roles of the Steroidogenic Acute Regulatory Protein (Star) as Revealed by Star Knockout Mice. Mol Endocrinol (Baltimore Md) (2000) 14(9):1462–71. doi: 10.1210/mend.14.9.0515
95. Wang Y, Chen M, Xu J, Liu X, Duan Y, Zhou C, et al. Core Clock Gene Bmal1 Deprivation Impairs Steroidogenesis in Mice Luteinized Follicle Cells. Reproduction (2020) 160(6):955–67. doi: 10.1530/rep-20-0340
96. Son GH, Chung S, Choe HK, Kim HD, Baik SM, Lee H, et al. Adrenal Peripheral Clock Controls the Autonomous Circadian Rhythm of Glucocorticoid by Causing Rhythmic Steroid Production. Proc Natl Acad Sci USA (2008) 105(52):20970–5. doi: 10.1073/pnas.0806962106
97. Nakao N, Yasuo S, Nishimura A, Yamamura T, Watanabe T, Anraku T, et al. Circadian Clock Gene Regulation of Steroidogenic Acute Regulatory Protein Gene Expression in Preovulatory Ovarian Follicles. Endocrinology (2007) 148(7):3031–8. doi: 10.1210/en.2007-0044
98. Morris JK, Richards JS. An E-Box Region Within the Prostaglandin Endoperoxide Synthase-2 (PGS-2) Promoter Is Required for Transcription in Rat Ovarian Granulosa Cells. J Biol Chem (1996) 271(28):16633–43. doi: 10.1074/jbc.271.28.16633
99. Cai W, Rambaud J, Teboul M, Masse I, Benoit G, Gustafsson JA, et al. Expression Levels of Estrogen Receptor Beta Are Modulated by Components of the Molecular Clock. Mol Cell Biol (2008) 28(2):784–93. doi: 10.1128/mcb.00233-07
100. Fu L, Lee CC. The Circadian Clock: Pacemaker and Tumour Suppressor. Nat Rev Cancer (2003) 3(5):350–61. doi: 10.1038/nrc1072
101. Lin D, Sugawara T, Strauss JF 3rd, Clark BJ, Stocco DM, Saenger P, et al. Role of Steroidogenic Acute Regulatory Protein in Adrenal and Gonadal Steroidogenesis. Sci (New York NY) (1995) 267(5205):1828–31. doi: 10.1126/science.7892608
102. Krege JH, Hodgin JB, Couse JF, Enmark E, Warner M, Mahler JF, et al. Generation and Reproductive Phenotypes of Mice Lacking Estrogen Receptor Beta. Proc Natl Acad Sci USA (1998) 95(26):15677–82. doi: 10.1073/pnas.95.26.15677
103. Emmen JM, Couse JF, Elmore SA, Yates MM, Kissling GE, Korach KS. In Vitro Growth and Ovulation of Follicles From Ovaries of Estrogen Receptor (ER){Alpha} and ER{Beta} Null Mice Indicate a Role for ER{Beta} in Follicular Maturation. Endocrinology (2005) 146(6):2817–26. doi: 10.1210/en.2004-1108
104. Couse JF, Yates MM, Deroo BJ, Korach KS. Estrogen Receptor-Beta Is Critical to Granulosa Cell Differentiation and the Ovulatory Response to Gonadotropins. Endocrinology (2005) 146(8):3247–62. doi: 10.1210/en.2005-0213
105. Vida B, Hrabovszky E, Kalamatianos T, Coen CW, Liposits Z, Kalló I. Oestrogen Receptor Alpha and Beta Immunoreactive Cells in the Suprachiasmatic Nucleus of Mice: Distribution, Sex Differences and Regulation by Gonadal Hormones. J Neuroendocrinol (2008) 20(11):1270–7. doi: 10.1111/j.1365-2826.2008.01787.x
106. Nakahata Y, Kaluzova M, Grimaldi B, Sahar S, Hirayama J, Chen D, et al. The NAD+-Dependent Deacetylase SIRT1 Modulates CLOCK-Mediated Chromatin Remodeling and Circadian Control. Cell (2008) 134(2):329–40. doi: 10.1016/j.cell.2008.07.002
107. Asher G, Gatfield D, Stratmann M, Reinke H, Dibner C, Kreppel F, et al. Sirt1 Regulates Circadian Clock Gene Expression Through Per2 Deacetylation. Cell (2008) 134(2):317–28. doi: 10.1016/j.cell.2008.06.050
108. Chang HC, Guarente L. SIRT1 Mediates Central Circadian Control in the SCN by a Mechanism That Decays With Aging. Cell (2013) 153(7):1448–60. doi: 10.1016/j.cell.2013.05.027
109. Levine DC, Hong H, Weidemann BJ, Ramsey KM, Affinati AH, Schmidt MS, et al. NAD(+) Controls Circadian Reprogramming Through PER2 Nuclear Translocation to Counter Aging. Mol Cell (2020) 78(5):835–49.e7. doi: 10.1016/j.molcel.2020.04.010
110. Zhu S, Zhou J, Sun X, Zhou Z, Zhu Q. ROS Accumulation Contributes to Abamectin-Induced Apoptosis and Autophagy via the Inactivation of PI3K/AKT/Mtor Pathway in TM3 Leydig Cells. J Biochem Mol Toxicol (2020) 34(8):e22505. doi: 10.1002/jbt.22505
111. Chen F, Wang Y, Liu Q, Hu J, Jin J, Ma Z, et al. Ero1α Promotes Testosterone Secretion in Hcg-Stimulated Mouse Leydig Cells via Activation of the PI3K/AKT/Mtor Signaling Pathway. J Cell Physiol (2020) 235(7-8):5666–78. doi: 10.1002/jcp.29498
112. Cui Y, Chen R, Ma L, Yang W, Chen M, Zhang Y, et al. Mir-205 Expression Elevated With EDS Treatment and Induced Leydig Cell Apoptosis by Targeting RAP2B via the PI3K/AKT Signaling Pathway. Front Cell Dev Biol (2020) 8:448. doi: 10.3389/fcell.2020.00448
113. Wen R, Hu S, Xiao Q, Han C, Gan C, Gou H, et al. Leptin Exerts Proliferative and Anti-Apoptotic Effects on Goose Granulosa Cells Through the PI3K/Akt/Mtor Signaling Pathway. J Steroid Biochem Mol Biol (2015) 149:70–9. doi: 10.1016/j.jsbmb.2015.01.001
114. Cai Z, He S, Li T, Zhao L, Zhang K. Plumbagin Inhibits Proliferation and Promotes Apoptosis of Ovarian Granulosa Cells in Polycystic Ovary Syndrome by Inactivating PI3K/Akt/Mtor Pathway. Anim Cells Syst (2020) 24(4):197–204. doi: 10.1080/19768354.2020.1790416
115. Yang G, Chen L, Grant GR, Paschos G, Song WL, Musiek ES, et al. Timing of Expression of the Core Clock Gene Bmal1 Influences Its Effects on Aging and Survival. Sci Trans Med (2016) 8(324):324ra16. doi: 10.1126/scitranslmed.aad3305
116. Ratajczak CK, Asada M, Allen GC, McMahon DG, Muglia LM, Smith D, et al. Generation of Myometrium-Specific Bmal1 Knockout Mice for Parturition Analysis. Reprod Fertil Dev (2012) 24(5):759–67. doi: 10.1071/rd11164
117. Mereness AL, Murphy ZC, Forrestel AC, Butler S, Ko C, Richards JS, et al. Conditional Deletion of Bmal1 in Ovarian Theca Cells Disrupts Ovulation in Female Mice. Endocrinology (2016) 157(2):913–27. doi: 10.1210/en.2015-1645
118. Reinberg A, Lagoguey M, Cesselin F, Touitou Y, Legrand JC, Delassalle A, et al. Circadian and Circannual Rhythms in Plasma Hormones and Other Variables of Five Healthy Young Human Males. Acta Endocrinol (1978) 88(3):417–27. doi: 10.1530/acta.0.0880417
Keywords: circadian gene BMAL1, reproductive endocrine disorder, fertility, circadian rhythm, reproduction
Citation: Jiang Y, Li S, Xu W, Ying J, Qu Y, Jiang X, Zhang A, Yue Y, Zhou R, Ruan T, Li J and Mu D (2022) Critical Roles of the Circadian Transcription Factor BMAL1 in Reproductive Endocrinology and Fertility. Front. Endocrinol. 13:818272. doi: 10.3389/fendo.2022.818272
Received: 19 November 2021; Accepted: 09 February 2022;
Published: 02 March 2022.
Edited by:
Ye Chun Ruan, Hong Kong Polytechnic University, Hong Kong SAR, ChinaReviewed by:
Ueli Schibler, Université de Genève, SwitzerlandGuangrui Yang, Dalian University of Technology, China
Copyright © 2022 Jiang, Li, Xu, Ying, Qu, Jiang, Zhang, Yue, Zhou, Ruan, Li and Mu. This is an open-access article distributed under the terms of the Creative Commons Attribution License (CC BY). The use, distribution or reproduction in other forums is permitted, provided the original author(s) and the copyright owner(s) are credited and that the original publication in this journal is cited, in accordance with accepted academic practice. No use, distribution or reproduction is permitted which does not comply with these terms.
*Correspondence: Jinhui Li, eW95b2xlZTgyNEAxNjMuY29t; Dezhi Mu, bXVkekBzY3UuZWR1LmNu
†These authors have contributed equally to this work and share first authorship