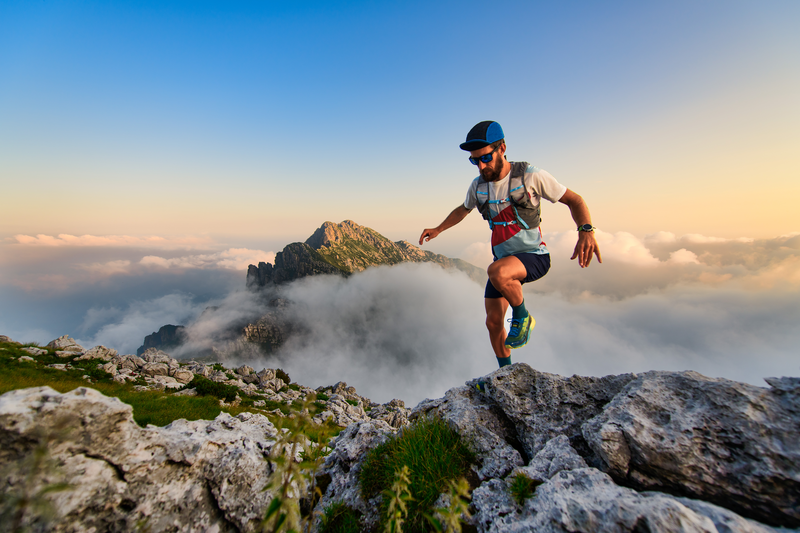
94% of researchers rate our articles as excellent or good
Learn more about the work of our research integrity team to safeguard the quality of each article we publish.
Find out more
ORIGINAL RESEARCH article
Front. Endocrinol. , 28 March 2022
Sec. Obesity
Volume 13 - 2022 | https://doi.org/10.3389/fendo.2022.790414
A correction has been applied to this article in:
Corrigendum: Exercise and/or Cold Exposure Alters the Gene Expression Profile in the Fat Body and Changes the Heart Function in Drosophila
The major reason of human morbidity and mortality is obesity and related diseases. Brown adipose tissue (BAT) is associated with low total adipose tissue content and a lower risk of type 2 diabetes mellitus. Studies have shown that exercise and cold expose may induce browning. In this study, we verified (1) whether exercise and/or cold exposure can improve the expression level of ucp4c, serca, ampkα, camkII, sirt1, octβ3r, and hamlet; (2) if these interventions can save cardiac dysfunction induced by a high-fat diet (HFD) in Drosophila. w1118 (wild-type) virgin female flies collected within 8 h after eclosion were divided into eight groups: the normal feed control group (NFD-C), the normal feed exercise group (NFD-E), the normal feed cold exposure group (NFD-CA), the normal feed exercise/cold exposure group (NFD-EC), the HFD control group (HFD-C), the HFD exercise group (HFD-E), the HFD cold exposure group (HFD-CA), and the HFD exercise/cold exposure group (HFD-EC). After exercise and/or cold exposure for 7 days, the mRNA expression levels of ucp4c, serca, ampkα, camk II, sirt1, octβ3r, and hamlet were tested by qRT-PCR, and m-mode was used to assess cardiac function. In addition, we assessed the triacylglycerol (TAG) levels, motor ability, fat mass (by Oil Red O [ORO] staining), and morphological features. The results of TAG, ORO staining, and morphological features all indicate that after interventions, body size of Drosophila was smaller compared with the control group, irrespective of the feeding patterns. The mRNA expression levels of ucp4c, serca, octβ3r, hamlet, ampkα, camkII, and sirt1 were changed to varying degrees under different intervention states (exercise and/or cold exposure). Cold exposure and exercise/cold exposure partly improved cardiac function and the normal fruit flies’ cardiac function and exercise ability. However, after exercise intervention, exercise ability and heart function were improved in both HFD and normal-fat diet (NFD) fruit flies. In conclusion, different intervention states (exercise and/or cold exposure) can change the mRNA expression levels of ucp4c, serca, octβ3r, hamlet, ampkα, camkII, and sirt1. Exercise is the most effective way to restore HFD-induced cardiac dysfunction.
Obesity increases the risk of metabolic diseases and cardiovascular disease and impairs exercise capacity (1). Brown adipose tissue (BAT) is emerging as a promising and interesting target for therapeutic intervention in metabolic disease and obesity. A large number of studies have shown that BAT thermogenesis is efficiently activated upon repeated cold exposure or exercise and is important in the regulation of body weight and energy expenditure (2–4).
UCP1-mediated mitochondrial thermogenesis is a hallmark of both brown and beige adipocytes and is commonly used as a molecular marker to identify brown and beige adipocytes (5–7). For beige fat thermogenesis, SERCA2b is required. SERCA2b is important in both the presence and absence of UCP1. When UCP1 is absent, beige fat enhances tricarboxylic acid metabolism, glycolysis, and pyruvate dehydrogenase activity to dynamically expend glucose for ATP-dependent thermogenesis by the SERCA2b pathway (8). Moreover, the PRDM16 and β3-adrenergic receptors, as beige markers, also play an indispensable role in browning (9, 10). Exercise and cold exposure are two factors that induce browning, and during the process, the genes that control energy expenditure also play an integral role. A number of studies have mentioned that AMPK, CAMKII, and SIRT1 play a potentially important role in regulating the thermogenic program that allows for brown and beige adipocytes to take up more glucose and burn more lipid through non-shivering thermogenesis (11, 12).
Drosophila and human genomes have shown that ~80% of human diseases in which the disease-related gene has been identified have an orthologue in Drosophila according to the analyses from Homophila database (13). Moreover, because of its relatively short lifespan, low economic cost, and easy to be fed, Drosophila is considered an excellent model for studying cardiovascular disease, metabolism, obesity, and exercise (14–18). However, little research has reported the relationship of cold exposure/exercise, obesity, and cardiovascular disease in Drosophila. In the present study, we explored the effects of expression levels of ucp4 c (the homologous gene of ucp1), serca (the homologous gene of serca2b), octβ3r (the homologous gene of β3-adrenergic receptor), hamlet (the homologous gene of prdm16), ampkα (the homologous gene of ampk), camk II (the homologous gene of camk), and sirt1 (the homologous gene of sirt1) genes in Drosophila induced by exercise and/or cold exposure on exercise capacity and cardiac function. This is of great significance for the study of cardiovascular diseases caused by a high-fat diet.
Triacylglycerol (TAG) is the main lipid storage form in both humans and flies; therefore, quantification of TAG content has always been used to define obesity in flies. According to the multi-factor analysis of variance (ANOVA) of triacylglycerol (TAG) levels (Table 1), feeding patterns and interventions exert major effects on TAG levels and have no interaction. In the present study, fruit flies’ morphological characteristics were imaged using a Leica stereomicroscope. As shown in Figure 1, high-fat diet (HFD) fruit flies have a larger body size, proving that HFD can induce an obese Drosophila model. Moreover, fruit flies had a smaller body size after exercise and/or cold exposure under two different feeding regimes. In addition, as shown in Figure 2, the results of our Oil Red O (ORO) staining assay revealed that fruit flies in the control group without exercise and/or cold exposure possessed more adipose tissue. Similarly, as shown in Figure 3, in both HFD and normal-fat diet (NFD) Drosophila, exercise and/or cold exposure reduced whole-body triacylglycerol (TAG) levels. In summary, all data with respect to the flies’ morphological characteristics, TAG levels, or ORO staining indicate that exercise and/or cold exposure decreased adipose tissue levels.
Figure 1 Drosophila morphological characteristics. The wings and legs of fruit flies were removed for observation.
As shown in Figure 4, in NFD fruit flies, (1) after exercise, the relative mRNA expression levels of ucp4c, serca, ampkα, and sirt1 were significantly higher, while camk II, hamlet, and octβ3r showed no significant change; (2) after cold exposure, the relative mRNA expression levels of ucp4c, serca, and sirt1 were significantly higher, while ampkα, camk II, hamlet, and octβ3r showed no significant change; (3) after exercise and cold exposure, the relative mRNA levels of ucp4c, octβ3r, and sirt1 were significantly higher, while serca, ampkα, camk II, and hamlet showed no significant change. In HFD fruit flies, (1) after exercise, the relative mRNA expression levels of ucp4c, serca, and ampkα were significantly higher, while camk II, hamlet, octβ3r, and sirt1 showed no significant change; (2) after cold exposure, the relative mRNA expression levels of ucp4c, serca, camk II, and octβ3r were significantly higher, while ampkα, hamlet, and sirt1 showed no significant change; (3) after exercise and cold exposure, the relative mRNA levels of serca, ampkα, camk II, hamlet, and sirt1were significantly higher, while ucp4c and octβ3r showed no significant change.
Figure 4 mRNA expression levels of ucp4c, serca, octβ3r, hamlet, ampkα, camk II, and sirt1 under different interventions. *P < 0.05, **P < 0.01, ***P < 0.001.
The climbing index of fruit flies was tested using a climbing device that measures the negative geotaxis behavior (RING) of flies (19). As shown in Table 2, feeding patterns and intervention methods have strong effects on locomotor capacity and have interactive effects. According to Figure 5, in both HFD and NFD fruit flies, exercise had a positive effect on the climbing index. Interestingly, after cold exposure for 7 days, in HFD fruit flies, the climbing index was significantly increased, while in NFD fruit flies, the climbing index was significantly decreased. As regards fruit flies under exercise/cold exposure conditions, in NFD fruit flies, no significant change in climbing index was observed, whereas in HFD fruit flies, the climbing index was significantly increased (Figure 5).
Figure 5 Flies’ locomotor ability under different interventions. *P < 0.05, **P < 0.01, ***P < 0.001.
Indicators to evaluate cardiac function are abundant. In Drosophila, M-mode is a common method. Table 3 shows the main effect and interaction of cardiac function indices. As shown in Figure 6, in HFD fruit flies, the levels of HR, AI, and FL were significantly increased while FS was significantly decreased compared with NFD fruit flies, confirming that HFD reduces heart function. (1) After exercise intervention, in the NFD group, HR, AI, and FL were significantly decreased, while DI, SD, and FS were significantly increased and HP, SI, and DD were not significantly different. In the HFD group, HR, AI, and FS also decreased, and HP, DI, DD, and FS significantly increased. (2) After cold exposure, in the NFD group, HR significantly decreased, and HP, SI, DI, DD, SD, and FS significantly increased. In the HFD group, HR significantly decreased, HP, SI, DD, FS, and FL significantly increased, and others had no significant changes. (3) After exercise/cold exposure, in NFD fruit flies, DD and SD significantly increased, and others had no significant change. In the HFD group, HR significantly decreased and FS and FL significantly decreased.
Figure 6 Heart function in HFD and NFD fruit flies. *P < 0.05, **P < 0.01, ***P < 0.001, ns P > 0.05.
Comparing these results, we see that HR was significantly decreased irrespective of the intervention, which means that all interventions can decelerate the heartbeat of fruit flies. Moreover, the results shown in Figures 7, 8 indicate that regular exercise can restore HFD-induced reductions in cardiac function, strengthen the heart pumping function, and reduce the incidence of AI and FL. However, among fruit flies in the cold exposure and exercise/cold exposure groups, different results were observed between the NFD and HFD groups. In HFD fruit flies, cold exposure or exercise/cold exposure seems not a good way to save heart function as AI and FL significantly increased, which means the risk of cardiovascular disease increased. In NFD fruit flies, after cold exposure or exercise/cold exposure, AI and FL were not significantly changed, but FS was significantly increased after cold intervention, which indicates that cold exposure strengthens the heart pumping function.
Adipose tissue is composed of a complex network that participates in the regulation of different biological functions, such as vascular tone control, glucose and lipid metabolism, and body weight homeostasis. Imbalance of these adaptive homeostatic mechanisms leads to adipose tissue dysfunctionality, which will cause obesity and metabolic disease-associated inflammation or metaflammation (1). For example, adipose tissue expansion, adipocyte hyperplasia, and hypertrophy (increase in cell number and cell size) always lead to obesity (20), which represents a major risk factor for the development of several of the most common medical conditions, such as type 2 diabetes mellitus, dyslipidemia, non-alcoholic fatty liver, and cardiovascular disease. Recently, BAT has emerged as a promising and interesting target for therapeutic intervention in metabolic disease and obesity. UCP1, SERCA2B, AMPK, PRDM16, β3-adrenergic receptor, CAMK, and SIRT1 have been considered key mediators of energy expenditure and heat production in brown and beige adipocytes (8–12). Animal studies and human studies have found that both cold exposure and exercise can promote browning of adipose tissue (21, 22). However, there are no reports on fruit flies. In this study, we discussed the possibility of improving the expression level of ucp4c, serca, octβ3r, hamlet, ampkα, camk II, and sirt1 in Drosophila and restoring cardiac insufficiency and low exercise capacity caused by HFD through exercise and/or cold exposure. Our main findings are as follows: (1) Under different intervention states, the mRNA expression levels of ucp4c, serca, octβ3r, hamlet, ampkα, camk II, and sirt1 were changed (Figure 4). (2) In the NFD group, only exercise can improve the climbing index, which decreased significantly under cold exposure or exercise/cold exposure conditions. In the HFD group, exercise and/or cold exposure significantly increased the climbing index. (3) In the NFD group, exercise can effectively improve cardiac function, including lowering HR, decreasing the risk of AI and FB, and enhancing the pumping capacity. Cold exposure and exercise/cold exposure only partially improved cardiac function. In the HFD group, although all interventions decreased HR and improved FS, cold exposure or exercise/cold exposure increased the risk of arrhythmia and fibrillation to different degrees; only exercise significantly reduced the risk of AI and FL.
In Drosophila, as shown in Figure 9, fat is distributed throughout the body in six parts: deep fat tissue, peripheral fat tissue, pericardial fat tissue, ventral fat tissue, dorsal fat tissue, and breast-specific fat tissue. We can easily observe the dorsal vessel surrounded by fat, and fat is concentrated around the ventral and dorsal sides; the body structure is relatively simple. Drosophila’s relatively short lifespan, its organ systems that perform essentially the same metabolic functions as their vertebrate counterparts, and the sophisticated genetic tools available for studying this species (23) lay a firm foundation for Drosophila to be an excellent model for studying obesity, diabetes, cardiomyopathy, and exercise. In addition, the reasons choosing Drosophila as the animal model for this research are as follows: (1) Most genes and gene families known to function in metabolic disease and adipose proteins’ structure and function are highly conserved between flies and humans (24). (2) Fruit flies develop obesity, and their associated complications during caloric overload are similar to those of humans (25). (3) The Drosophila cardiac system shares many similarities and provides many advantages as a model of cardiovascular diseases (16), the Drosophila dorsal vessel is an organ for hemolymph circulation that resembles the vertebrate heart at its transient linear tube stage, and dorsal vessel morphogenesis shares several similarities with early events of vertebrate heart development. In particular, cardiac progenitors are derived from a laterally positioned mesoderm, being specified through the use of conserved cellular induction pathways and downstream transcriptional effectors (14, 15). (4) The Drosophila blood system contains cells that are functionally similar to myeloid cells in mammals. As in mammalian systems, multiple sites of hematopoiesis are evident in Drosophila, and the mechanisms involved in this process employ many of the same molecular strategies that exemplify blood development in humans (26). (5) Drosophila researchers have been able to investigate the impacts of exercise on the animals’ physical fitness, cardiac health, ageing, and more (27). Meanwhile, in fruit flies, previous studies have demonstrated that translational results can be obtained and that can serve to complement mammalian studies (14), and numerous studies have used Drosophila as a model for studying exercise (28, 29). Therefore, we decided to explore the expression levels of ucp4c, serca octβ3r, hamlet, ampkα, camk II, and sirt1 in Drosophila under exercise and/or cold exposure conditions and the effects on HFD-induced cardiac insufficiency and low exercise capacity.
Figure 9 Fat tissue distribution in Drosophila. The original image collected from flybase.org was modified. DFt, Deep fat tissue; PFt, Peripheral fat tissue; PcFt, Pericardial fat tissue. VFt, Ventral fat tissue; DSFt, Dorsal fat tissue; 1-9, Breast-specific fat tissue.
Adipose tissue is categorized into white, brown, and beige. WATs store excess energy as TAGs in one huge single lipid droplet. In total opposition to the specialized role of white adipocytes in energy storage, brown adipocytes have high mitochondrial content and vast numbers of small lipid droplets, and they occupy an important position in uncoupled respiration (30). Particularly, adipose tissue has prominent plasticity properties, and white adipocytes can differentiate into brown-like adipocytes (beige adipocytes) in a process called browning (31). Beige adipose tissue resembles BAT by morphology and function and also has the ability to produce heat.
When the rate of food combustion (decreased metabolic efficiency) or the rate of heat production increases, individual adipocytes receive a signal from the sympathetic nervous system. In brown adipocytes, the hormone and neurotransmitter norepinephrine (NE) activates β3-adrenergic receptors, and then the signal is transmitted via cAMP and protein kinase A, causing the release of free fatty acids (FFAs) from TAGs, initiating TAG breakdown. FFAs are involved in the physiological activation of UCP1 and/or the transport mechanism and UCP1 thus allows for mitochondrial combustion of substrates, uncoupled from the production of ATP (10) In beige adipocytes, calcium cycling is promoted by adrenergic activation and Ca2+ released from the sarco(endo)plasmic reticulum (SER) is recycled by the SER Ca2+-ATPase 2b (SERCA2b) pump (8). Many research studies indicate that Ca2+ release also plays a fundamental role in a range of signaling processes in muscles, and RYR1, CASQ, SERCA, and SLN jointly regulate calcium homeostasis (28, 29, 32–35). In Drosophila, ucp4c is the homologous gene of UCP1, serca is the homologous gene of SERCA2B, RyR is the homologous gene of RYR1/RYR2, and no homologous genes of CASQ and SLN were found. In our research, we focus on heart function, and so have little discussion about muscles, which is also a limitation of this study. Therefore, we decided to observe the expression levels of ucp4c, serca, octβ3, hamlet, ampkα, camk II, and sirt1 through the intervention of exercise and/or cold expose to provide a basis for further research.
During exercise, fruit flies need a large amount of energy for muscular activity. Living at low temperatures, fruit flies also need energy to resist the cold environment. As shown in Figures 2–4, upon loss of fat mass, the fly’s lean size and TAG levels significantly decreased upon energy consumption. TAGs stored in adipose tissue are mobilized to provide fatty acids for energy conversion. These may be the reasons why mRNA expression levels of ucp4c, serca, octβ3r, hamlet, camk II, ampkα, and sirt1 were changed to varying degrees under different intervention states. However, this is not enough to prove whether browning occurs in the flies, and so more experimental studies are needed.
As is well known, obesity is correlated with cardiovascular risk factors. Abdominal obesity is a major risk factor for the development of type 2 diabetes and atherogenic dyslipidemia, which increases the risk of premature coronary heart disease. This increased risk can be largely attributed to high accumulation of abdominal adipose tissue, especially of visceral adipose tissue (36). Studies showed that weight reduction is significantly associated with a lower risk of AF recurrence; a reduction in AF symptoms affects both CVD risk factors and cardiac structure and function (37). So, can the risk of obesity-induced cardiovascular disease be reduced by decreasing abdominal fat levels through exercise and/or cold exposure?
Many studies have verified that regular exercise positively affects the lipid profile and blood pressure, reducing the rate of cardiovascular events and associated mortality (37, 38). This has also been demonstrated in fruit flies by Wen et al. (39). After exercise intervention, a series of molecular changes occur in the vascular system, such as increases in absolute vascular nitric oxide (NO) levels, vascular regeneration, endothelial repair, and cardiac expression of miR-126 (40). All of these changes can effectively prove that exercise significantly helps the prevention of in cardiovascular disease. Our experiment also proves that exercise can restore HFD-induced reductions in cardiac function and strengthen the heart pumping function (Figures 7, 8). In addition, the locomotor ability of fruit flies also significantly improved (Figure 5). What about the effect of cold exposure or exercise/cold exposure? Although high lipid consumption relieves abdominal fat accumulation, improves locomotor capacity, slows down the heart rate, and strengthens the heart pumping function, FL significantly increased and AI did not significantly change, which means cold exposure may not be very effective in restoring HFD-induced cardiac insufficiency. Interventions affect cardiovascular responses, which may be altered by underlying cardiovascular disease. Upon cold exposure, HFD fruit flies need to burn fat to fight the cold, which creates a greater pressure load on their heart and may increase the risk of FL and AL. A number of studies have pointed out that cold environments can cause myocardial ischemia, arrhythmia, myocardial hypertrophy, and other cardiac disorders (41, 42). From our findings, we can speculate that, for humans, a cold environment may not be suitable for patients with obesity and cardiovascular disease, which may increase the risk of FL, and that regardless of whether the individuals are healthy or have cardiovascular disease or obesity, exercise seems to be the best approach.
It can be seen from Figure 5 that cold environments seem to exert no effects on HFD fruit flies’ locomotor capacity. The HFD flies had improved locomotor capacity after losing weight. However, their locomotor capacity was significantly lower in the NFD group. Upon cold exposure, we observed that the fruit flies were more likely to be in a motionless state compared with the control group, in agreement with a previous study which reported that 50% of fruit flies could not maintain flight for 1 s at 15°C (43). We speculate that the decline in locomotor capacity results from a decrease in daily activity, due to which muscular strength is lower than that of normal fruit flies. Kellys (44) indicated that physical inactivity may be particularly deleterious in T2D or in the elderly, and even a short-term reduction in physical activity has significant impact on skeletal muscle protein and carbohydrate metabolism. In addition, lower temperatures can reduce metabolic rates, change muscle contraction activity, and impair muscle physiology (45). Moreover, the CM Williams’ research has shown that low temperatures induce a reversible loss of neuromuscular coordination in insects (46). In conclusion, compared with cold exposure or exercise/cold exposure conditions, only regular exercise can truly help restore HFD-induced cardiac insufficiency and low exercise capacity.
We used wild-type Drosophila melanogaster strain w1118 virgin female flies (collected within 8 h after eclosion). NFD fruit flies were housed in an incubator at 25°C while HFD flies were housed in an incubator at 22–24°C and 50% relative humidity under a 12/12-h light/dark cycle. Fresh food was provided once every 2 days without anesthesia. High-fat medium was made by mixing 30% coconut oil and 70% standard medium.
Flies were randomly divided into eight groups: the NFD control group (NFD-C), the NFD exercise group (NFD-E), the NFD cold exposure group (NFD-CA), the NFD exercise/cold exposure group (NFD-EC), the HFD control group (HFD-C), the HFD exercise group (HFD-E), the HFD cold exposure group (HFD-CA), and the HFD exercise/cold exposure group (HFD-EC). According their natural negative geotaxis behavior, we designed an exercise device (19). Vials were horizontally loaded (with feed and housing 20 flies each) into a steel tube that was rotated about its horizontal axis at a gear control shafting speed. To stimulate most flies to climb, each vial was rotated along its long axis. Few flies that failed to climb were actively walking on the inner wall of the vial. Vials rotated at 0.16 rev/s, and flies exercised for 2.5 hours per day.
For D. melanogaster, 18–25°C is an acclimation temperature. When the temperature falls below 10°C, the development of D. melanogaster will halt (34). Fat content significantly reduces when temperatures fall below 13°C. Therefore, at 1–7 days after emergence, vials were kept at 15°C for 2.5 hours.
Ten abdominal subcutaneous adipose tissue samples were collected from each group and total RNA was isolated from tissue using TRIzol (Invitrogen, CA) according to the manufacturer’s protocol. Adipose tissues were extracted from the abdominal sternite and the abdominal tergite (Figure 10). cDNA was generated using Superscript III reverse transcriptase (Invitrogen, CA) and used as the templates for quantitative real-time PCR. Real-time PCR was performed using SYBR green using an ABI7300 Real-time PCR Instrument (Applied Biosystems). The tested genes’ relative abundance was calculated by the 2−ΔΔCt method. Primers used for expression analysis were as follows:
Figure 10 Adipose tissue was extracted from the bright yellow areas. *The original image was collected from flybase.org and modified.
Primer sequences of ucp4c: F: 5′-CGGCTTCTCGGCAATGGTGAC-3′; R: 5′-CTTCCTCGTTCCGCTCGTTCTG-3′.
Primer sequences of serca: F: 5′-TGCTGGTGAAGATTCTGCTGTTGG-3′; R: 5′-CACGGCGTTGGCTATCAGGATAAG-3′.
Primer sequences of Octβ3R: F: 5′- TGTGGTCAACAAGGCCTACG-3′; R: 5′- GTGTTCGGCGCTGTTAAGGA-3′.
Primer sequences of hamlet: F: 5′- ATAGATCCTTTGGCCAGCAGAC-3′; R: 5′- AGTACTCCTCCCTTTCGGCAAT-3′.
Primer sequences of AMPKα: F: 5′- GGCCATCGCTTACCATCTGA -3′; R: 5′- ATCGATGATTGGGGAACGGG-3′.
Primer sequences of Camk II: F: 5′- ACAACCATTTTAAATCCTCATGTGC-3′; R: 5′- TGTGCATGTCCTTGTTATCA-3′.
Primer sequences of Sirt1: F: 5′- TAGAGCCTCACATGCAAGCTCTA-3′; R: 5′- GCCAATCATAAGATGTTGCTGAAC-3′.
TAG levels were measured by ELISA (TAG ELISA Kits, MLBIO) as follows: (1) Samples (n = 15, three groups) were homogenized with grinders and centrifuged for 15 min (4000 rpm, 4°C). (2) Standard wells were set and 50 μl standard solution was added to the standard wells. (3) To the sample wells, 40 μl sample solution and 10 μl of the sample were added and mixed gently. (4) Except for the blank wells, 100 μl of HRP-conjugate reagent II was added to each well. (5) Plates were incubated for 1 h at 37°C. (6) After centrifugation, wells were washed five times with washing buffer for 30 s. (7) Stop solution (50 μl) was added to each well to stop the reactions. (8) The blank values were subtracted from all others.
ORO staining was used to quantify lipids. (1) Fruit flies were dissected and the adipose tissue was exposed in phosphate-buffered saline (PBS). (2) PBS was removed and samples were fixed with 4% paraformaldehyde for 20 min. (3) Samples were washed three times with PBS for 10 min. (4) Oil Red O reagent was dropped on the samples, which were incubated for 1 h at room temperature. (5) The samples were washed three times with PBS for 10 min. (6) The samples were transferred to a slide with mounting solution. (7) Photos were taken with a Leica stereo microscope.
First, 30 flies were fixed back down on a Petri dish after applying anesthesia with FlyNap for 2–3 min. Second, the head was rapidly removed, artificial hemolymph was added (visualizing the beating heart in Drosophila), and then all internal organs except the heart and any abdominal fat were removed. Oxygen was pumped for 15 min at room temperature. Fruit flies’ heartbeats were recorded by an EM-CCD high-speed camera (video at 130 fps for 30 s), and the cardiogram data were recorded by HC Image software. Semi-Automated Optical Heartbeat Analysis (SOHA, kindly gifted by Ocorr and Bodmer), a software program that accurately quantifies heart rate (HR), heart period (HP), diastolic diameter (DD), systolic diameter (SD), systolic interval (SI), diastolic interval (DI), arrhythmicity index (AI), fibrillations (FL), and the percentage of fractional shortening (FS), was used to assess functional cardiac parameters of fruit flies.
Flies from each group were transformed into the climbing apparatus, which consisted of an 18-cm-long glass tube with an inner diameter of 2.8 cm (sponges were placed in the ends of the tube to prevent escape yet allow air exchange), 20 pieces per tube, 5 tubes with in total 20 fruit flies per tube, 5 tubes in total (47). Fruit flies were gently shaken to the bottom and climbed up the wall of the tube because of the negative geotaxis instinct. The first three times were considered adaptive climbing, and the fourth, fifth, and sixth climbing heights reached by the fruit flies at the end of 5 seconds were recorded by a video camera. The climbing index is the average climbing height of the flies in each vial.
SPSS version 25.0 software was used to analyze all data. Data are presented as the mean ± standard error of the mean (SEM). The statistical techniques performed to identify differences between the groups were multi-way ANOVA and least significant difference (LSD) tests. Statistical significance was set at P < 0.05.
The mRNA expression levels of ucp4c, serca, octβ3r, hamlet, ampkα, camk II, and sirt1 were changed to varying degrees under different intervention states (exercise and/or cold exposure), which laid the foundation for future research. Compared with cold exposure or exercise/cold exposure, exercise is the best way to restore HFD-induced cardiac dysfunction, which facilitates the study of the mechanisms underlying brown and beige adipose tissue activity and generation in obesity and other metabolic diseases.
The original contributions presented in the study are included in the article/supplementary material. Further inquiries can be directed to the corresponding author.
TH and LZ conceived and designed the experiments and wrote the manuscript. TH, XJ, JL, MD, SZ, TW and QL collected the samples. TH, XJ, JL, JFYL, and GZ performed the experiments. TH, XJ, JL, analyzed the data. All authors have read and approved the final manuscript.
This work was supported by the National Natural Science Foundation of China (No. 31671243 and 32071175), the Hunan Province Graduate Education Innovation Project and Professional Ability Enhancement Project Fund (Project Number: CX20200533) and Key scientific research project of Hunan Education Department (Project Number: 19A328).
The authors declare that the research was conducted in the absence of any commercial or financial relationships that could be construed as a potential conflict of interest.
All claims expressed in this article are solely those of the authors and do not necessarily represent those of their affiliated organizations, or those of the publisher, the editors and the reviewers. Any product that may be evaluated in this article, or claim that may be made by its manufacturer, is not guaranteed or endorsed by the publisher.
We thank Karen Ocorr and Rolf Bodmer (American Burnham medical institute of neurology and aging center) for supporting semi-automatic optical echocardiography analysis software.
1. Unamuno X, Gomez-Ambrosi J, Rodriguez A, Becerril S, Fruhbeck G, Catalan V. Adipokine Dysregulation and Adipose Tissue Inflammation in Human Obesity. Eur J Clin Invest (2018) 48(9):e12997. doi: 10.1111/eci.12997
2. Saito M, Okamatsu-Ogura Y, Matsushita M, Watanabe K, Yoneshiro T, Nio-Kobayashi J, et al. High Incidence of Metabolically Active Brown Adipose Tissue in Healthy Adult Humans: Effects of Cold Exposure and Adiposity. Diabetes (2009) 58(7):1526–31. doi: 10.2337/db09-0530
3. van Marken Lichtenbelt WD, Vanhommerig JW, Smulders NM, Drossaerts JM, Kemerink GJ, Bouvy ND, et al. Cold-Activated Brown Adipose Tissue in Healthy Men. N Engl J Med (2009) 360(15):1500–8. doi: 10.1056/NEJMoa0808718
4. Cypess AM, Lehman S, Williams G, Tal I, Rodman D, Goldfine AB, et al. Identification and Importance of Brown Adipose Tissue in Adult Humans. N Engl J Med (2009) 360(15):1509–17. doi: 10.1056/NEJMoa0810780
5. Ricquier D, Casteilla L, Bouillaud F. Molecular Studies of the Uncoupling Protein. FASEB J (1991) 5(9):2237–42. doi: 10.1096/fasebj.5.9.1860614
6. Nicholls DG, Locke RM. Thermogenic Mechanisms in Brown Fat. Physiol Rev (1984) 64(1):1–64. doi: 10.1152/physrev.1984.64.1.1
7. Mulya A, Kirwan JP. Brown and Beige Adipose Tissue: Therapy for Obesity and Its Comorbidities? Endocrinol Metab Clin North Am (2016) 45(3):605–21. doi: 10.1016/j.ecl.2016.04.010
8. Ikeda K, Kang Q, Yoneshiro T, Camporez JP, Maki H, Homma M, et al. UCP1-Independent Signaling Involving SERCA2b-Mediated Calcium Cycling Regulates Beige Fat Thermogenesis and Systemic Glucose Homeostasis. Nat Med (2017) 23(12):1454–65. doi: 10.1038/nm.4429
9. Seale P, Kajimura S, Yang W, Chin S, Rohas LM, Uldry M, et al. Transcriptional Control of Brown Fat Determination by PRDM16. Cell Metab (2007) 6(1):38–54. doi: 10.1016/j.cmet.2007.06.001
10. Cannon B, Nedergaard J. Brown Adipose Tissue: Function and Physiological Significance. Physiol Rev (2004) 84(1):277–359. doi: 10.1152/physrev.00015.2003
11. Chen CC, Kuo CH, Leu YL, Wang SH. Corylin Reduces Obesity and Insulin Resistance and Promotes Adipose Tissue Browning Through SIRT-1 and Beta3-AR Activation. Pharmacol Res (2021) 164:105291. doi: 10.1016/j.phrs.2020.105291
12. Desjardins EM, Steinberg GR. Emerging Role of AMPK in Brown and Beige Adipose Tissue (BAT): Implications for Obesity, Insulin Resistance, and Type 2 Diabetes. Curr Diabetes Rep (2018) 18(10):80. doi: 10.1007/s11892-018-1049-6
13. Chien S, Reiter LT, Bier E, Gribskov M. Homophila: Human Disease Gene Cognates in Drosophila. Nucleic Acids Res (2002) 30(1):149–51. doi: 10.1093/nar/30.1.149
14. Tao Y, Schulz RA. Heart Development in Drosophila. Semin Cell Dev Biol (2007) 18(1):3–15. doi: 10.1016/j.semcdb.2006.12.001
15. Gould RA, Aboulmouna LM, Varner JD, Butcher JT. Hierarchical Approaches for Systems Modeling in Cardiac Development. Wiley Interdiscip Rev Syst Biol Med (2013) 5(3):289–305. doi: 10.1002/wsbm.1217
16. Wolf MJ, Rockman HA. Drosophila, Genetic Screens, and Cardiac Function. Circ Res (2011) 109(7):794–806. doi: 10.1161/CIRCRESAHA.111.244897
17. Watanabe LP, Riddle NC. New Opportunities: Drosophila as a Model System for Exercise Research. J Appl Physiol (1985) (2019) 127(2):482–90. doi: 10.1152/japplphysiol.00394.2019
18. Sujkowski A, Wessells R. Using Drosophila to Understand Biochemical and Behavioral Responses to Exercise. Exerc Sport Sci Rev (2018) 46(2):112–20. doi: 10.1249/JES.0000000000000139
19. Zheng L, Feng Y, Wen DT, Wang H, Wu XS. Fatiguing Exercise Initiated Later in Life Reduces Incidence of Fibrillation and Improves Sleep Quality in Drosophila. Age (Dordr) (2015) 37(4):9816. doi: 10.1007/s11357-015-9816-7
20. Wang QA, Tao C, Gupta RK, Scherer PE. Tracking Adipogenesis During White Adipose Tissue Development, Expansion and Regeneration. Nat Med (2013) 19(10):1338–44. doi: 10.1038/nm.3324
21. Nascimento EBM, van Marken Lichtenbelt WD. In Vivo Detection of Human Brown Adipose Tissue During Cold and Exercise by PET/CT. Handb Exp Pharmacol (2019) 251:283–98. doi: 10.1007/164_2018_121
22. Sullo A, Brizzi G, Maffulli N. Deiodinating Activity in the Brown Adipose Tissue of Rats Following Short Cold Exposure After Strenuous Exercise. Physiol Behav (2003) 80(2-3):399–403. doi: 10.1016/j.physbeh.2003.09.005
23. Gáliková M, Klepsatel P. Obesity and Aging in the Drosophila Model. Int J Mol Sci (2018) 19(7):1896. doi: 10.3390/ijms19071896
24. Reiter LT, Potocki L, Chien S, Gribskov M, Bier E. A Systematic Analysis of Human Disease-Associated Gene Sequences in Drosophila Melanogaster. Genome Res (2001) 11(6):1114–25. doi: 10.1101/gr.169101
25. Musselman LP, Kuhnlein RP. Drosophila as a Model to Study Obesity and Metabolic Disease. J Exp Biol (2018) 221(Pt Suppl 1):jeb163881. doi: 10.1242/jeb.163881
26. Banerjee U, Girard JR, Goins LM, Spratford CM. Drosophila as a Genetic Model for Hematopoiesis. Genetics (2019) 211(2):367–417. doi: 10.1534/genetics.118.300223
27. Riddle NC. Drosophila Melanogaster, a New Model for Exercise Research. Acta Physiol (Oxf) (2019) 227(3):e13352. doi: 10.1111/apha.13352
28. Clarke OB, Hendrickson WA. Structures of the Colossal RyR1 Calcium Release Channel. Curr Opin Struct Biol (2016) 39:144–52. doi: 10.1016/j.sbi.2016.09.002
29. Woo JS, Jeong SY, Park JH, Choi JH, Lee EH. Calsequestrin: A Well-Known But Curious Protein in Skeletal Muscle. Exp Mol Med (2020) 52(12):1908–25. doi: 10.1038/s12276-020-00535-1
30. Correa LH, Heyn GS, Magalhaes KG. The Impact of the Adipose Organ Plasticity on Inflammation and Cancer Progression. Cells (2019) 8(7):662. doi: 10.3390/cells8070662
31. Chu DT, Gawronska-Kozak B. Brown and Brite Adipocytes: Same Function, But Different Origin and Response. Biochimie (2017) 138:102–5. doi: 10.1016/j.biochi.2017.04.017
32. Furlan S, Mosole S, Murgia M, Nagaraj N, Argenton F, Volpe P, et al. Calsequestrins in Skeletal and Cardiac Muscle From Adult Danio Rerio. J Muscle Res Cell Motil (2016) 37(1-2):27–39. doi: 10.1007/s10974-015-9432-2
33. Stammers AN, Susser SE, Hamm NC, Hlynsky MW, Kimber DE, Kehler DS, et al. The Regulation of Sarco(Endo)Plasmic Reticulum Calcium-ATPases (SERCA). Can J Physiol Pharmacol (2015) 93(10):843–54. doi: 10.1139/cjpp-2014-0463
34. Pant M, Bal NC, Periasamy M. Sarcolipin: A Key Thermogenic and Metabolic Regulator in Skeletal Muscle. Trends Endocrinol Metab (2016) 27(12):881–92. doi: 10.1016/j.tem.2016.08.006
35. Bal NC, Maurya SK, Sopariwala DH, Sahoo SK, Gupta SC, Shaikh SA, et al. Sarcolipin is a Newly Identified Regulator of Muscle-Based Thermogenesis in Mammals. Nat Med (2012) 18(10):1575–9. doi: 10.1038/nm.2897
36. Despres JP, Lemieux I, Prud'homme D. Treatment of Obesity: Need to Focus on High Risk Abdominally Obese Patients. BMJ (2001) 322(7288):716–20. doi: 10.1136/bmj.322.7288.716
37. Lavie CJ, Pandey A, Lau DH, Alpert MA, Sanders P. Obesity and Atrial Fibrillation Prevalence, Pathogenesis, and Prognosis: Effects of Weight Loss and Exercise. J Am Coll Cardiol (2017) 70(16):2022–35. doi: 10.1016/j.jacc.2017.09.002
38. Lavie CJ, Arena R, Swift DL, Johannsen NM, Sui X, Lee DC, et al. Exercise and the Cardiovascular System: Clinical Science and Cardiovascular Outcomes. Circ Res (2015) 117(2):207–19. doi: 10.1161/CIRCRESAHA.117.305205
39. Wen DT, Zheng L, Yang F, Li HZ, Hou WQ. Endurance Exercise Prevents High-Fat-Diet Induced Heart and Mobility Premature Aging and Dsir2 Expression Decline in Aging Drosophila. Oncotarget (2018) 9(7):7298–311. doi: 10.18632/oncotarget.23292
40. Schuler G, Adams V, Goto Y. Role of Exercise in the Prevention of Cardiovascular Disease: Results, Mechanisms, and New Perspectives. Eur Heart J (2013) 34(24):1790–9. doi: 10.1093/eurheartj/eht111
41. Hauton D, May S, Sabharwal R, Deveci D, Egginton S. Cold-Impaired Cardiac Performance in Rats is Only Partially Overcome by Cold Acclimation. J Of Exp Biol (2011) 214(18):3021–31. doi: 10.1242/jeb.053587
42. Liang J, Yin K, Cao X, Han Z, Huang Q, Zhang L, et al. Attenuation of Low Ambient Temperature-Induced Myocardial Hypertrophy by Atorvastatin via Promoting Bcl-2 Expression. Cell Physiol Biochem (2017) 41(1):286–95. doi: 10.1159/000456111
43. Lehmann FO. Ambient Temperature Affects Free-Flight Performance in the Fruit Fly Drosophila Melanogaster. J Comp Physiol B (1999) 169(3):165–71. doi: 10.1007/s003600050207
44. Bowden Davies KA, Pickles S, Sprung VS, Kemp GJ, Alam U, Moore DR, et al. Reduced Physical Activity in Young and Older Adults: Metabolic and Musculoskeletal Implications. Ther Adv Endocrinol Metab (2019) 10:2042018819888824. doi: 10.1177/2042018819888824
45. Scaraffia PY, Gerez de Burgos NM. Effects of Temperature and pH on Hexokinase From the Flight Muscles of Dipetalogaster Maximus (Hemiptera: Reduviidae). J Med Entomol (2000) 37(5):689–94. doi: 10.1603/0022-2585-37.5.689
46. Williams CM, Watanabe M, Guarracino MR, Ferraro MB, Edison AS, Morgan TJ, et al. Cold Adaptation Shapes the Robustness of Metabolic Networks in Drosophila Melanogaster. Evolution (2014) 68(12):3505–23. doi: 10.1111/evo.12541
Keywords: exercise, cold expose, cardia function, ucp4c, SIRT1
Citation: Huang T, Jian X, Liu J, Zheng L, Li FQ, Meng D, Wang T, Zhang S, Liu Y, Guan Z and Feng J (2022) Exercise and/or Cold Exposure Alters the Gene Expression Profile in the Fat Body and Changes the Heart Function in Drosophila. Front. Endocrinol. 13:790414. doi: 10.3389/fendo.2022.790414
Received: 06 October 2021; Accepted: 25 February 2022;
Published: 28 March 2022.
Edited by:
Yanchuan (Yan) Shi, Garvan Institute of Medical Research, AustraliaReviewed by:
Naresh Chandra Bal, KIIT University, IndiaCopyright © 2022 Huang, Jian, Liu, Zheng, Li, Meng, Wang, Zhang, Liu, Guan and Feng. This is an open-access article distributed under the terms of the Creative Commons Attribution License (CC BY). The use, distribution or reproduction in other forums is permitted, provided the original author(s) and the copyright owner(s) are credited and that the original publication in this journal is cited, in accordance with accepted academic practice. No use, distribution or reproduction is permitted which does not comply with these terms.
*Correspondence: Lan Zheng, TGFuemhlbmdAaHVubnUuZWR1LmNu
†These authors contributed equally to the work and share first authorship
Disclaimer: All claims expressed in this article are solely those of the authors and do not necessarily represent those of their affiliated organizations, or those of the publisher, the editors and the reviewers. Any product that may be evaluated in this article or claim that may be made by its manufacturer is not guaranteed or endorsed by the publisher.
Research integrity at Frontiers
Learn more about the work of our research integrity team to safeguard the quality of each article we publish.