- 1Affiliated Hospital of Qingdao University, Qingdao, China
- 2Huashan Hospital, Fudan University, Shanghai, China
Background: The impact of diabetes on reproductive function is still not clearly defined. This study aimed to evaluate accelerated ovarian aging in women with type 2 diabetes mellitus (T2DM) and its association with adverse lipid profile.
Methods: Female patients with T2DM (n=964) and non-T2DM controls (n=263) aging from 18-80 years were included. Levels of circulating sex hormones were measured at the follicular phase in menstruating women. We analyzed the age-specific trends in the levels of sex hormones between T2DM and controls. The correlations of sex hormones with the lipid profile, including low-density lipoprotein cholesterol (LDL-C), high-density lipoprotein cholesterol (HDL-C), total cholesterol (TC) and triglycerides (TG) were also evaluated.
Results: In the temporal trends analysis, LH and FSH both started to increase obviously approximately from the age of 45 years among patients with T2DM, and displayed peaks of LH and FSH among patients with T2DM aged between 61 and 65, both of which were obviously earlier than that in non-T2DM controls and proved the accelerated ovarian aging among patients with T2DM. E2 of patients with T2DM was continuous lower than that of non-T2DM controls from approximately 45 years old, and LH and FSH of patients with T2DM were higher than those of non-T2DM controls between the age of 55 to 65 years. Multiple linear regression analyses revealed that LH was positively correlated with LDL-C (Coefficient=0.156, P=0.001) and TC (Coefficient=0.134, P=0.025), and was negatively correlated with HDL-C (Coefficient =-0.065, P=0.001) in patients with T2DM aged between 51 and 60, which was independent of age, T2DM duration, body mass index (BMI), glycosylated hemoglobin (HbA1c), FSH, E2 and other potential confounders. Higher E2 level was significantly and independently correlated with lower LDL-C (Coefficient= -0.064, P=0.033) in patients with T2DM aged between 51 and 60.
Conclusions: This study suggests that patients with T2DM have accelerated ovarian aging, and it is correlated with the occurrence of disturbed lipid profile in patients with T2DM. With an ever increasing number of female patients with T2DM diagnosed at younger ages, the accelerated ovarian aging and its adverse impacts in T2DM need to be carefully managed.
Introduction
Type 2 diabetes mellitus (T2DM) is a common disease with a serious global pandemic, and could cause substantial adverse impacts on human health (1). The dramatically rising global prevalence of T2DM especially in young adults further increases its global burden (2). Apart from those common diabetic complications such as diabetic neuropathy and diabetic retinopathy, reproductive dysfunction or complications such as subfertility are also prevalent in diabetic patients (3). Ovarian aging commonly refers to the decline of female ovarian functions with age, accompanied by the deterioration in the quantity and quality of ovarian follicles, and dysfunction of granulosa cells and theca cells. (4, 5) Some previous studies have found an association between type 1 diabetes mellitus and accelerated ovarian aging (6–8), and a few studies based on animal models demonstrate that diabetes-obesity syndrome may be associated with impaired oocyte quality (9, 10). However, clinical research on the association between T2DM and accelerated ovarian aging is limited, and the results have been inconsistent. Some studies reported accelerated ovarian aging in patients with T2DM (11–13) while others found no relationships between T2DM and reproductive aging (8, 14).
Cardiovascular complications and microvascular complications are common in patients with T2DM (15, 16). Cardiovascular disease (CVD) is the leading cause of mortality in postmenopausal women (17, 18), and the morbidity rate of CVDs in DM patients is more than twice of that of non-diabetic patients (19). As one of the major causes of atherosclerotic cardiovascular diseases, the dysregulation of lipid metabolism serves as a common feature of T2DM, traditionally characterized by elevated fasting and postprandial triglycerides (TC), low high density lipoprotein-cholesterol (HDL-C), high low density lipoprotein-cholesterol (LDL-C) (20–22). As one of the major characteristics of dyslipdemia, increased LDL-C is an independent risk factor for the development of CVDs, which in women usually occurs in the postmenopausal state (23–25). Among sex hormones, negative associations of E2 with TC and LDL-C have been demonstrated in estrogen receptor (ERα) -deficient models, postmenopausal women with E2 deficiency and women on E2 treatment (26–28). However, studies on the relations between gonadotropins– luteinizing hormone (LH) and follicle-stimulating hormone (FSH)– and lipid levels are still limited and have reported inconsistent findings (27, 29–31). For instance, emerging evidence indicates that FSH level may relate to CVD risks through its association with lipid levels, and some observational studies on women in the menopausal transition demonstrates the association positive (32–35), while other studies on postmenopausal women and older postmenopausal women show it negative (18, 30, 31). Furthermore, clinical investigations show that adoption of hormone replacement therapy (HRT) based on E2 level alone only partially reversed dyslipidemia or lipid oxidation in postmenopausal women. These lead us to hypothesize that gonadotropins may have independent impacts on the lipid profile in perimenopausal or postmenopausal women with T2DM.
The primary purpose of this study is to assess the differences in the age-specific trends of sex hormones in female patients with T2DM and non-T2DM controls, thereby evaluating accelerated ovarian aging in women with T2DM; we also aim to study the correlations of sex hormones with the lipid profile among patients with T2DM.
Methods
Study Population
Participants were selected from the database of inpatients at Affiliated Hospital of Medical College Qingdao University (Shandong, China). The inclusion criteria were: female patients with or without T2DM aging between 18 and 80, hospitalized between 2013 and 2020. The exclusion criteria were: patients with space occupying lesion, history of polycystic ovarian syndrome or pituitary tumor, acute complications of T2DM, autoimmune diease and who reported taking hormone therapy, as is shown in Figure 1. Thus, we included 964 female patients diagnosed with T2DM and 263 non-T2DM controls in the final study. The protocol was designed according to the Declaration of Helsinki and was approved by the ethics committee of the Affiliated Hospital of Qingdao University. The study was registered on http://www.chictr.org.cn/under number ChiCTR2100045896.
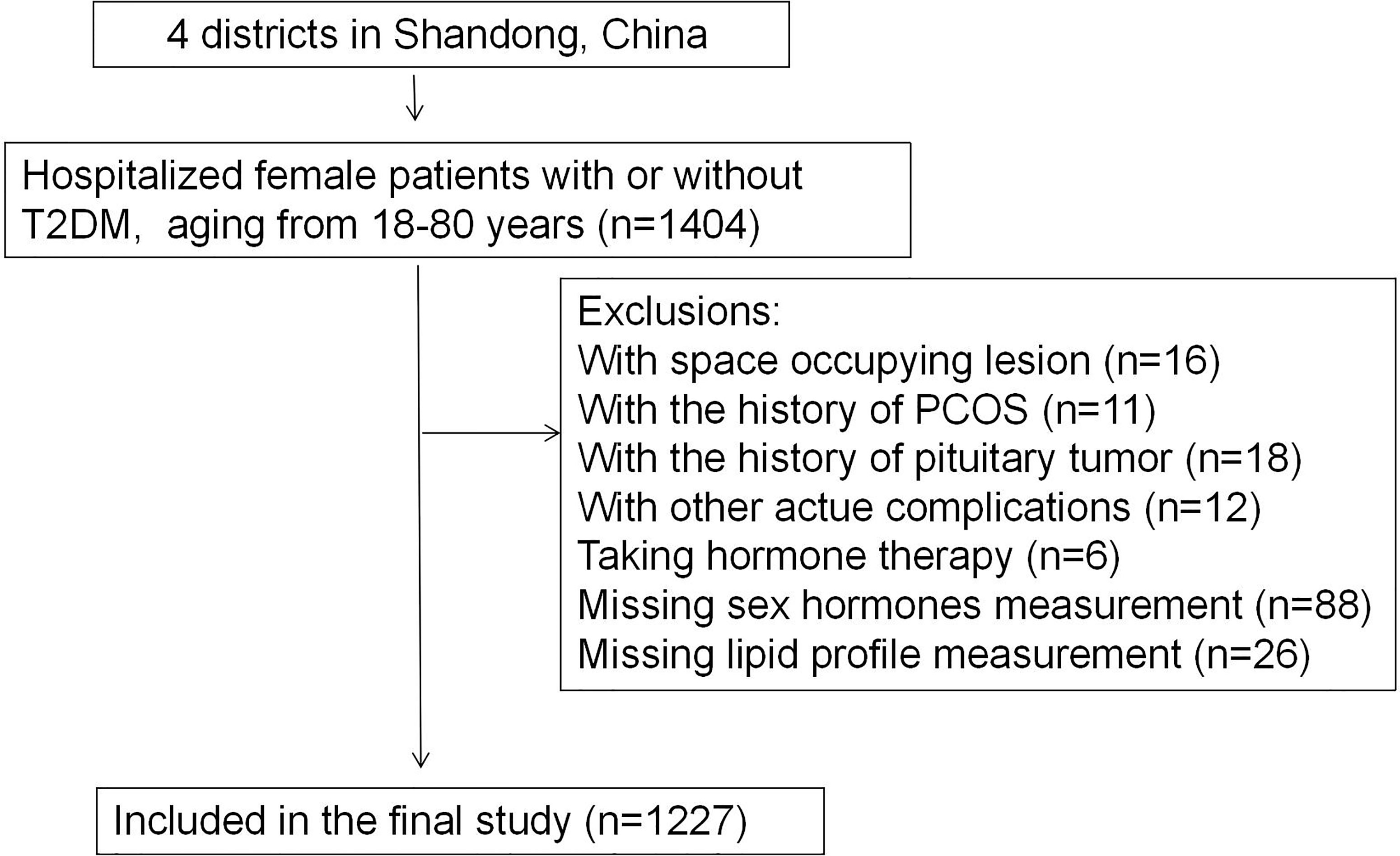
Figure 1 Flowchart of the inclusion and exclusion of participants (T2DM, type 2 diabetes mellitus; PCOS, polycystic ovarian syndrome).
Anthropometric and Laboratory Data
The characteristics of the study population include age, sex, height, weight and hip circumference, smoking and drinking status, diabetes duration, blood glucose, blood pressure (BP), lipid profiles including LDL-C, HDL-C, TC, triglycerides (TG), free fatty acid (FFA), liver and renal function, and personal medical history. Clinical examinations were conducted by a trained staff group according to a standard guideline. In particular, height and weight were measured with patients standing without shoes and with lightweight clothing. Waist circumference was measured at the midpoint of the lowest rib and the iliac crest. BP was reported as the means of three consecutive measurements with an interval of 5 minutes.
Blood samples were obtained between 6:00 and 9:00 AM after fasting for at least 8 hours. Venipuncture was performed in the median cubital vein, and centrifugation and dispensing of serum separated from blood samples were completed within 1 hour. Levels of circulating sex hormones were measured at the follicular phase in menstruating women. All samples were transported under cold chain to a central laboratory for testing within 2 to 4 hours. Sex hormones including total testosterone (TT), estradiol (E2), FSH, and LH were measured by electrochemiluminescence immunoassay (Roche E602, Roche, Basel, Switzerland). The minimal detectable limits for each hormone were as follows: 0.09 nmol/L (TT), 18.35 pmol/L (E2), and 0.1 IU/L (LH and FSH). Glycosylated hemoglobin (HbA1c) was assessed by high-performance liquid chromatography (MQ-2000PT, Medconn, Shanghai, China). Serum glucose and lipid profiles were measured by Beckman Coulter AU 680 (Beckman Coulter, Krefeld, Germany). Body mass index (BMI) was calculated as weight divided by height squared (kg/m2).
Statistical Analysis
The SPSS version 22.0 software and R were used to perform statistical analyses. Continuous variables are presented as median (interquartile range); categorical variables are presented as frequency (%). Mann-Whitney test was used for testing differences between T2DM group and non-T2DM control group for continuous variables, Kruskal-Wallis H test for testing differences between age groups and chi-square test for categorical variables. To assess the temporal trends in circulating sex hormones across age groups, curves were fitted using three models (linear model, Locally Weighted regression (LOESS) model and binary linear regression model), and R2 representing the coefficient of determination in the linear model was calculated. The correlations between the levels of sex hormones and lipid profile variables were further assessed by multiple linear regression and results were expressed as standardized coefficients, in which potential confounders such as age, T2DM duration, BMI, HbA1c and blood pressures were adjusted. LH, FSH and E2 levels were divided into four quartiles, with the first quartile (Q1) representing the lowest quartile and the fourth quartile (Q4) being the highest quartile. All statistical analyses were two sided; and P values less than 0.05 were considered to be statistically significant.
Results
Patients’ Characteristics and the Changes of Circulating Sex Hormones Across Age
General demographic and laboratory characteristics of the participants were summarized in Supplemental Table 1 and Supplemental Table 2. This study recruited 964 female patients with T2DM and 263 non-T2DM controls. The median age of patients with T2DM and non-T2DM controls were respectively 62 and 59 years, and percentage of menopause were respectively 87.1% and 73.04%; patients with T2DM were more likely to experience higher BMI compared with controls (Table 1). Compared with non-T2DM controls, patients with T2DM had significantly lower levels of LH (P<0.001), FSH (P<0.001) and E2 (P<0.001); no difference in the testosterone level between patients with T2DM and non-T2DM controls was observed (P=0.421; Table 1). The decreased levels of LH, FSH and E2 in patients with T2DM are consistent with findings from previous literatures (36–39), which may be partially attributed to the impairment of hyperglycemia on hypothalamic function (40–42).
In general, patients with T2DM in older age groups were more likely to experience longer diabetes duration, higher systolic blood pressure and lower diastolic blood pressure (P<0.001); patients in the eldest group were likely to experience lower LDL-C and TC levels, where the increased percentage of statins as medication may play a role; differences of TG and FFA between age groups didn’t reach statistical significance (Supplemental Table 2). LH and FSH levels were significantly higher in groups more than 51 years old, while the T and E2 showed an opposite trend.
Patients With T2DM Had Accelerated Ovarian Aging
In the temporal trends analysis,we got the following results: (1) LH and FSH both started to increase obviously approximately from the age of 45 years among patients with T2DM, and displayed peaks of LH and FSH among patients with T2DM aged between 61 and 65, both of which were obviously earlier than that in non-T2DM controls (Figures 2A, B). E2 started to decrease obviously from earlier than 40 years old among patients with T2DM, while it started to decrease obviously approximately from the age of 45 years among non-T2DM controls (Figure 2C). The earlier changes in sex hormones suggests accelerated ovarian aging in patients with T2DM. (2) E2 of patients with T2DM was continuous lower than that of non-T2DM controls from approximately 45 years old, thus proving accelerated ovarian aging in patients with T2DM. On the other hand, LH and FSH of patients with T2DM were higher than those of non-T2DM controls between the age of 55 and 65 years. The rise in levels of LH and FSH after the decrease in E2 level could be due to the slightly delayed feedback of aggravated E2 deficiency in the secretion of gonadotropins.
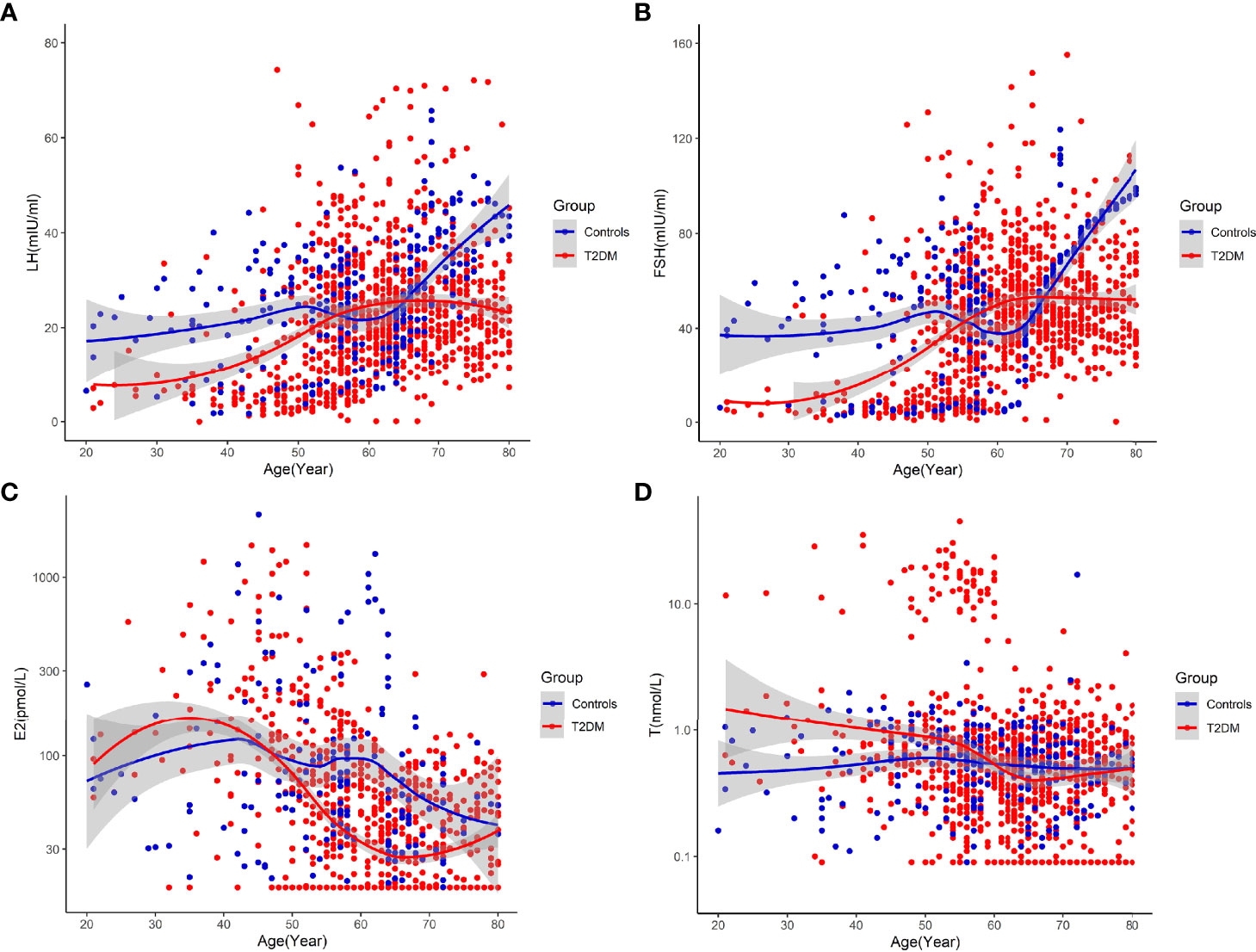
Figure 2 Temporal trends in circulating sex hormones across age groups in patients with T2DM and non-T2DM controls [(A) Temporal trends in circulating LH across age groups. (B) Temporal trends in circulating FSH across age groups. (C) Temporal trends in circulating E2 across age groups. (D) Temporal trends in circulating testosterone (T) across age groups].
We further analyzed the age-specific trends in sex hormones by clarifying differences in the circulating sex hormones between patients with T2DM and non-T2DM controls stratified by age. Patients with T2DM had significantly lower LH and FSH than non-T2DM controls before 50 years old, but the difference disappeared in the age groups of 51-60 years (Supplemental Figure 1 and Figure 3). Both LH and FSH were significantly higher in patients with T2DM compared with non-T2DM controls in the age groups of 61-65 years (p < 0.001). Moreover, patients with T2DM appeared to keep having significantly lower E2 level than non-T2DM controls from the age of 51 years (Figure 4). The findings above confirmed the age-specific differences in sex hormones between women with and without T2DM indicated by Figure 2.
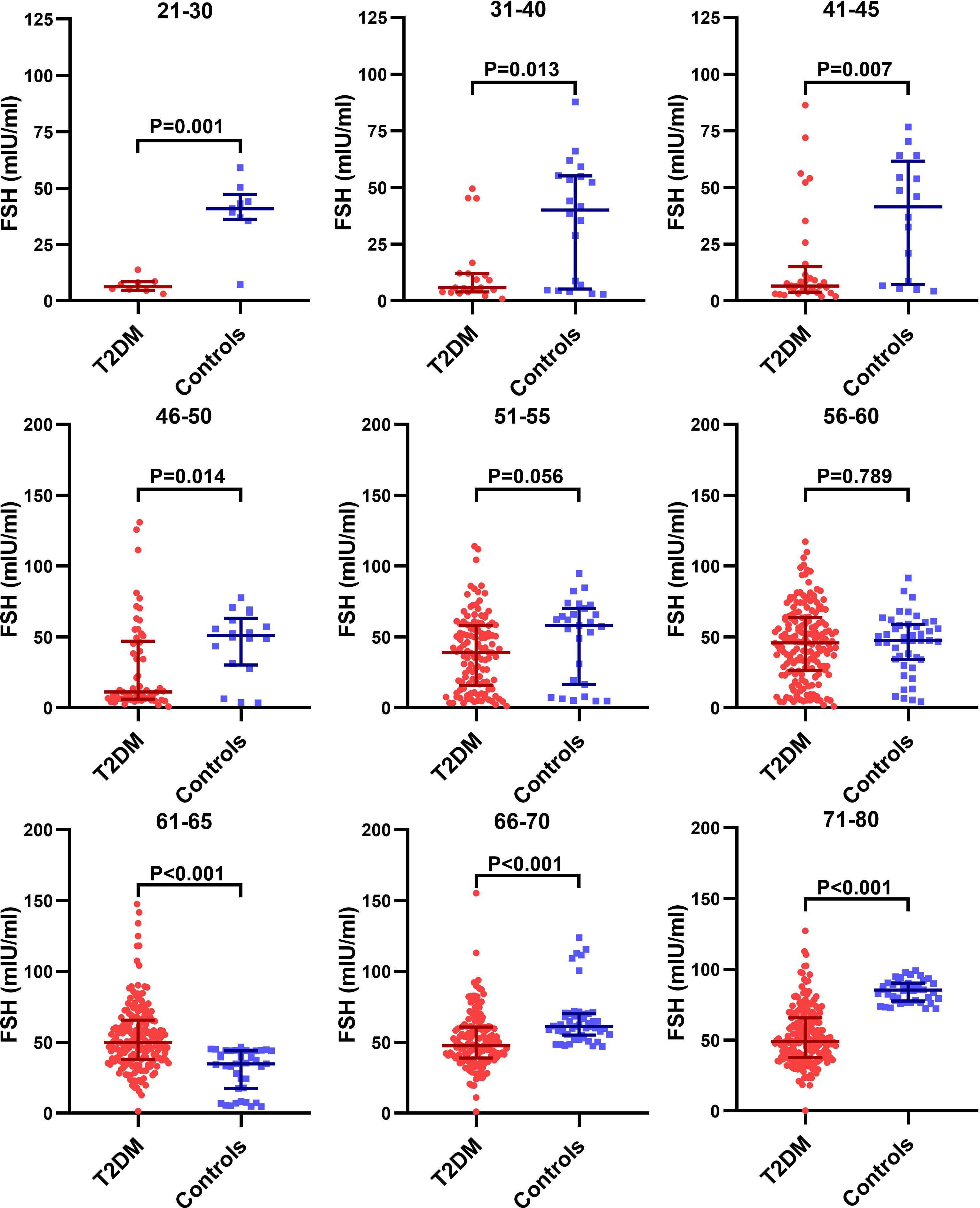
Figure 3 Difference in the circulating FSH level between patients with T2DM and non-T2DM controls stratified by age.
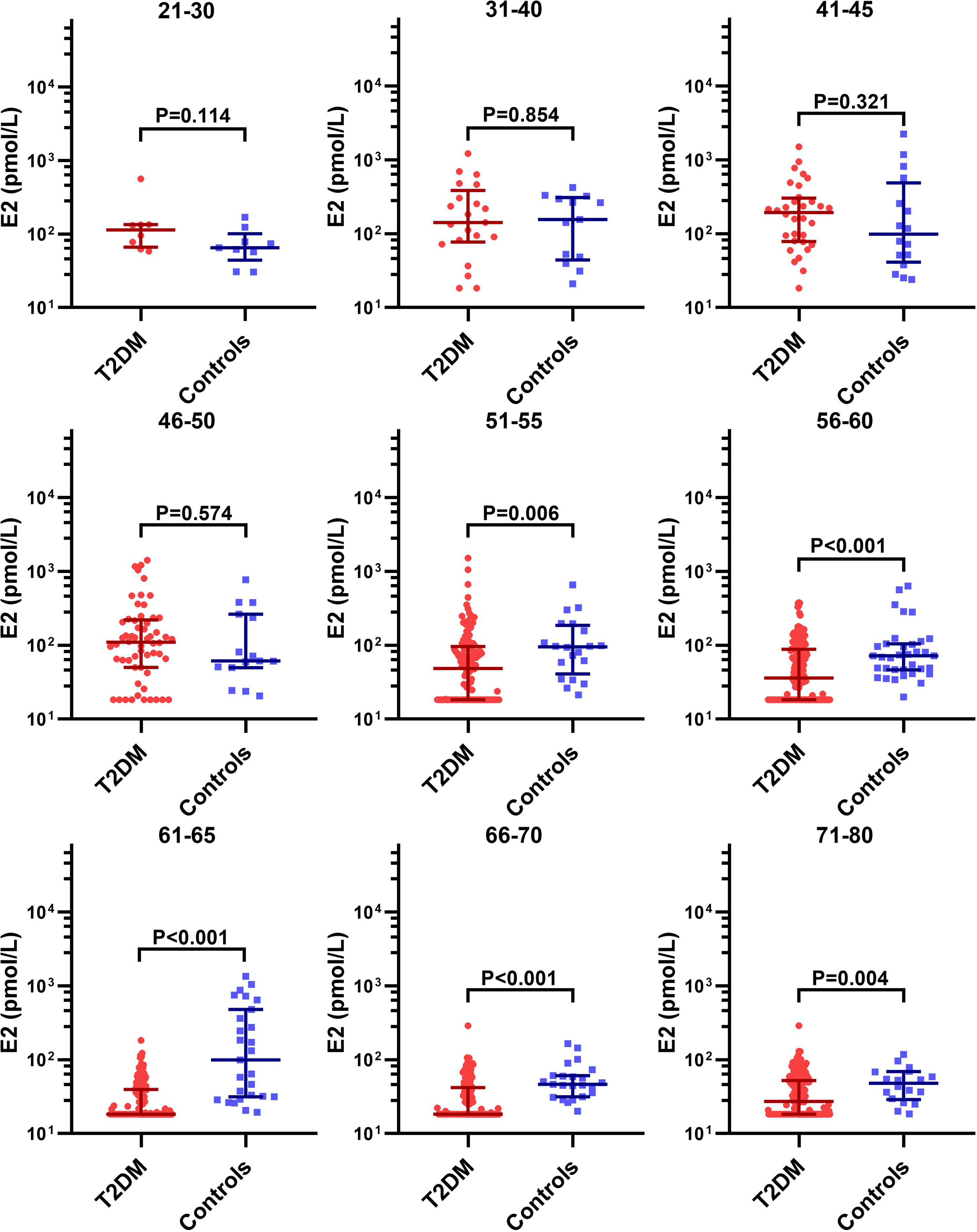
Figure 4 Difference in the circulating E2 level between patients with T2DM and non-T2DM controls stratified by age.
Correlations of Sex Hormones With Lipid Profile in Patients With T2DM Aged Between 51 and 60
As Figure 3, Supplemental Figure 1 and Figure 4 suggests, the aging-related changes in sex hormones of patients with T2DM were much more obvious in the age group of 51-60 years than the earlier age groups. Given that our second aim is to evaluate the association of accelerated ovarian aging with adverse lipid profile including LDL-C, HDL-C, TC, TG and FFA in patients with T2DM, we chose the age group of 51-60 years to assess the relationship of sex hormones with lipid profile considering that: (1) levels of sex hormones in this age group not only show drastic changes, but also were approximate to perimenopausal levels, thus worth investigating; (2) this age group was also characterized by disturbed lipid profile (Supplemental Table 2).
In the analyses of clinical characteristics of patients with T2DM aged between 51 and 60 by the quartiles of LH and FSH (Supplemental Tables 3, 4), patients with the higher level of LH and FSH were more likely to experience significantly higher TC and HDL-C compared with those with the bottom quartile of LH and FSH.
The correlations of lipid profile with LH, FSH and E2 in patients with T2DM aged 51-60 years were then analyzed (Figure 5). LH had significantly positive correlations with LDL-C and TC levels (P<0.001; Figure 5), suggesting a driving role of LH in dyslipidemia. FSH had significant positive correlations with LDL-C and TC levels (P<0.001), and had a modest positive correlation with HDL-C (P=0.008; Figure 5). E2 had significantly negative correlations with LDL-C and TC levels (P<0.01; Figure 5), suggesting a protective role of E2 against dyslipidemia.
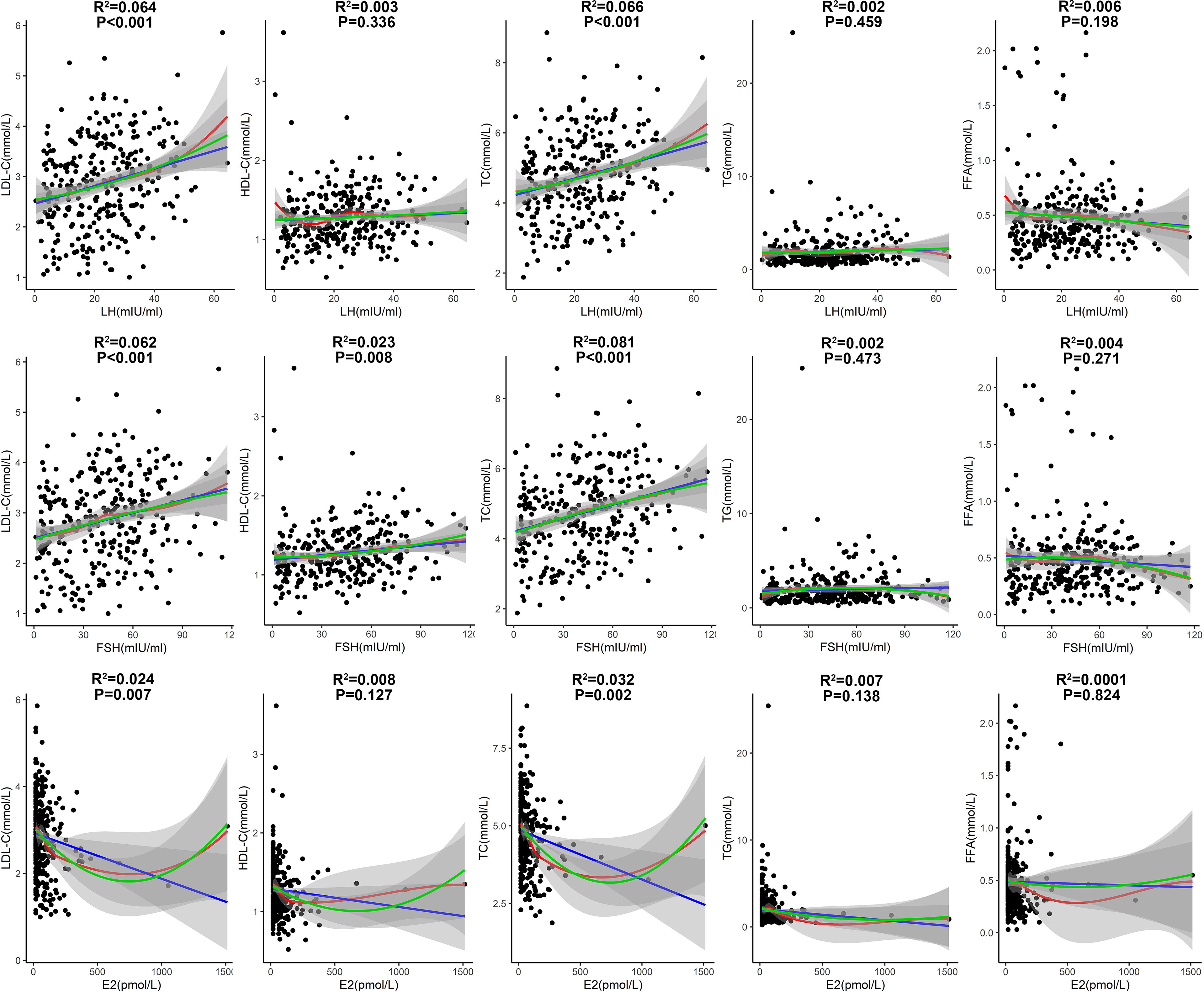
Figure 5 Correlations of lipid profile with LH, FSH and E2 in patients with T2DM aged 51-60 years. (Fitted curves of linear model are shown in blue; smoothing fitted curves of LOESS model are in red; fitted curves of binary linear regression model are in green. Adjusted R2 and P values from the linear model are shown in the figure).
In the multiple linear regression analyses by adjusting for age, T2DM duration, BMI, blood pressures, HbA1c, sex hormones and other potential confounders (Table 2), LH was positively correlated with LDL-C (Coefficient=0.156, P=0.001) and TC (Coefficient=0.134, P=0.025), and was negatively correlated with HDL-C (Coefficient =-0.065, P=0.001) in patients with T2DM aged between 51 and 60. Higher E2 level was significantly and independently correlated with lower LDL-C (Coefficient= -0.064, P=0.033) in patients with T2DM aged between 51 and 60.
Discussion
A major finding in this study is the accelerated ovarian aging among patients with T2DM. Reproductive aging such as premature ovarian failure can promote the development of cardiovascular diseases and has been reported among patients with T1DM (6–8). However, the reproductive aging of patients with T2DM is less well understood, and evidence from clinical studies is still limited. In the present study, we found that LH and FSH both started to increase obviously approximately from the age of 45 years among patients with T2DM, and displayed peaks of LH and FSH among patients with T2DM aged between 61 and 65, both of which were obviously earlier than that in non-T2DM controls; meanwhile, E2 started to decrease obviously approximately from the age of 40 years among patients with T2DM, while it started to decrease obviously approximately from the age of 45 years among non-T2DM controls (Figure 2). The findings above prove that aging-related changes in sex hormones occur earlier in patients with T2DM and patients with T2DM have accelerated ovarian aging than controls.
The age-specific trends of LH, FSH and E2 in patients with T2DM and non-diabetic controls are consistent with findings from several studies (11–13). Previous clinical research found a decline in ovarian volume, total antral follicle count, inhibin B secretion and anti-Miller hormone level in patients with T2DM compared with healthy controls, all of which were also markers for ovarian aging (9, 43). And a few studies based on animal models demonstrated that diabetes-obesity syndrome was associated with suppression of cyclic ovarian follicular recruitment patterns, anovulation, acyclicity, depressed ovarian steroid hormone synthesis and release, hypovascularization and tissue ischemia, enhanced follicular atresia, premature tissue atrophy and involution (9, 10). Despite such supporting evidence for accelerated ovarian aging in patients with T2DM, the underlying mechanisms are far from being clarified. Here, we deduced several possible mechanisms as follows: 1) It’s well established that low-grade chronic inflammation is involved in the pathophysiology T2DM (44), due mainly to the activation of the myeloid cell lineage (e.g., macrophages and neutrophils) (45). Inflammation of the whole body can affect oocytes via the follicle microenvironment and oxidative stress (46), thus impairing oocyte meiosis and oocyte quality (47). Indeed, chronic inflammation is found in aging ovaries, which is proved by the increase of multiple proinflammatory transcription factors, cytokines and chemokines (4, 48). For example, TNF-α binds to its death receptors (such as Fas) and then activates apoptosis of granular cells and oocytes through Fas-associated death domain-dependent activation of caspase-8; while TNFα knockout mice proved higher levels of granular cell proliferation (49). In addition, macrophage infiltration could induce the accumulation of collagen fibers in extracellular matrix, thereby promoting ovarian fibrosis (50). 2) Oxidative stress plays a key role in the pathophysiology of T2DM. During the progression of diabetes, hyperglycemia promotes mitochondrial dysfunction and induces the formation of oxygen species (ROS) that causes oxidative stress in several tissues including ovaries (45). And several studies have shown that the accumulation of ROS in the ovaries deteriorates oocyte quality, induces granulosa cell apoptosis, and accelerates degeneration of the corpus luteum (51). Excessive ROS may change the mitochondrial membrane potential by regulating the ratio of pro-/anti-apoptosis factors (49, 52), which eventually leads to the activation of caspase-3 which cleaves various structural and regulatory proteins in ovarian cells and causes apoptosis (46, 49). Moreover, oxidative stress and the accumulation of acquired mtDNA mutations could cause mtDNA damage (53). The effect of mtDNA alterations in ovarian follicle cells on qualitative ovarian reserve depletion and ovarian aging is also supported by the robust evidence (46, 53), though the mechanisms require further probe. 3) Vascular damage is one of the most common complications of diabetes, characterized by endothelium cell dysfunction, impaired angiogenesis, platelet activation and subsequent microembolisms (45). According to previous research, vascular impairment could lead to early menopause (54), in which the pathophysiological features of diabetic vascular damage may play an important part. And it’s worth noticing that dyslipidemia also could accelerate the impairment of oocyte quality, as it promotes endothelial dysfunction and platelet hyperactivation, potentially causing vascular damage and imposing cytolipotoxic effects (9, 45). 4) Diabetes is known to be associated with an accelerated rate of the accumulation of advanced glycation end products (AGEs) (55). The accumulation of AGEs has been reported to be closely related to declining ovarian function (56). The glycation process causes protein damage, and the interaction of AGEs and their receptors could lead to the activation of a number of proinflammatory pathways, increased production of ROS in oocytes, and endothelial dysfunction via inactivation of endothelial nitric oxide synthase and prostacyclin synthase, thereby inducing a premature decline in ovarian function (48, 56). Moreover, AGEs were reported to accelerate the progression of nuclear maturation, though the mechanisms still unknown (57).
We also found that LDL-C and TC levels were positively correlated with LH and FSH, and negatively correlated E2 with LDL-C and TC levels in patients with T2DM aged between 51 and 60, suggesting perimenopausal changes of gonadotropins and E2 are correlated with dyslipidemia. According to previous studies, consistent cross-sectional and longitudinal associations of menopause with worsening lipid profile have been observed (58), and for decades the associations have been mainly attributed to estrogen deficiency. Negative associations of E2 with TC and LDL-C has been demonstrated in ERα-deficient models, women with E2 deficiency and postmenopausal women on E2 treatment (59–65), results consistent with our study findings. These protective effects of E2 can be largely attributed to its upregulation of low-density lipoprotein receptors (LDLR) on hepatic cells: 1) through nuclear receptor ER, E2 stimulates the expression of transcription factors related to cholesterol metabolism, which upregulates LDLR expression as a downstream target (66); 2) through G protein-coupled estrogen receptor, E2 prevents PCSK9-dependent LDLR degradation, thus reducing the levels of TC and LDL-C as well (67). In terms of gonadotropins, although studies regarding the role of LH in dyslipidemia are scarce, several previous studies addressing the links between FSH and lipid levels in postmenopausal women have demonstrated that higher FSH in postmenopausal women was related to higher levels of both TC and LDL-C, with stronger relations seen in younger compared to older postmenopausal women, which were close to the results of our results (29, 68). In the animal model of ovariectomized mice which simulated the postmenopausal period, an increase in both TC and LDL-C was also found when FSH was elevated independent of E2 (68); in postmenopausal women, adjusting the estrogen dose in HRT to attain a target FSH decrease might lead to beneficial alterations in TC and LDL-C (68). Further, studies on women in the menopausal transition suggests that an increase in FSH accompanying the menopause transition may contribute to the higher risk of CVDs including subclinical atherosclerosis after menopause (32–34). The biological mechanisms identified so far come down to the downregulation of LDLR expression (68). LDLRs in the liver determine the turnover rate of LDL-C and thus regulate the plasma LDL concentrations. FSH may interact with its receptors (FSHR) in hepatocytes and reduce LDLR levels, which subsequently attenuates the endocytosis of LDL-C, resulting in an elevated circulating LDL-C level (68). All in all, FSH might reduce the uptake and metabolism, especially degradation of LDL-C in liver (68).
According to our study, the LH and FSH levels began to increase obviously approximately from 45 years old, indicating the beginning of perimenopausal stage, which commonly lasts 2 to 10 years and is characterized by less regular menstruation (69); the relations between gonadotropins and LDL-C and TC were found in female patients with T2DM aged between 51 and 60, generally a perimenopausal or early postmenopausal state. Correspondingly, the indication from 2019 ACC/AHA Guideline on the Primary Prevention of Cardiovascular Disease directly recommends patients 40 to 75 years of age with LDL-C>70 mg/dL (1.8 mmol/L) to start moderate-intensity statin therapy for primary prevention once diagnosed with DM, indicating that the cholesterol management in women with T2DM should start from a relative early age to prevent from CVDs (70). Interestingly, randomized controlled trials suggest that hormone treatment achieves most cardiovascular benefits if initiated soon after menopause, and is potentially harmful if initiated late (>10 years) after menopause (26). However, the early prevention is not fully implemented clinical work, and diabetic women are less likely to be on optimal lipid-lowering therapy compared to men (71), though the British Heart Foundation states that the number of women living with CVDs is now roughly the same as the number of men (26). Combined with our finding of T2DM accelerating ovarian aging, more research is needed on metabolic changes in women with T2DM of an age range linked with drastic changes of sex hormone levels.
This study had some limitations. The retrospective study cannot infer cause and effect; the restricted sample size may not be generalized to the larger population; there is insufficient experimental evidence to explain the relationships we found. Further, the varied amount of subjects taking statins between age groups and groups divided by LH and FSH quartiles could cause bias in results; but the correlations we found in subjects aged between 51 and 60 were more likely to be underestimated since the percentage of statins taking was higher in groups of higher LH and FSH levels compared with groups with the bottom quartile of LH and FSH. Finally, this study emphasizes the necessity of an early management of ovarian aging in patients with T2DM, and lends credibility to the suggestion to adopt the cholesterol management as early as the age of 40 in women with T2DM; while the mechanisms of the association between T2DM and accelerated ovarian aging, and the correlations of gonadotropins with the lipid profile and other cardiovascular risks still require investigation.
Overall, the study indicates that patients with T2DM have accelerated ovarian aging, and the elevated LH and FSH levels together with the decreased E2 level caused by the accelerated ovarian aging are correlated with the occurrence of disturbed lipid profile in patients with T2DM. With an ever increasing number of female patients with T2DM diagnosed at younger ages, the accelerated ovarian aging and its adverse impacts in T2DM need to be carefully managed. Besides, further evaluation of these relations and the mechanisms underlying them is warranted, especially via prospective studies that can provide a clearer understanding for accelerated ovarian aging in patients with T2DM.
Data Availability Statement
The original contributions presented in the study are included in the article/Supplementary Material. Further inquiries can be directed to the corresponding author.
Ethics Statement
The studies involving human participants were reviewed and approved by medical ethics committee of the Affiliated Hospital of Qingdao University. The patients/participants provided their written informed consent to participate in this study. Written informed consent was obtained from the individual(s) for the publication of any potentially identifiable images or data included in this article.
Author Contributions
YHW: designing and registering the work; the acquisition, analysis or interpretation of data for the work; drafting the work. YGW: agree to be accountable for all aspects of the work in ensuring that questions related to the accuracy or integrity of any part of the work are appropriately investigated and resolved. All authors contributed to the article and approved the submitted version.
Conflict of Interest
The authors declare that the research was conducted in the absence of any commercial or financial relationships that could be construed as a potential conflict of interest.
Publisher’s Note
All claims expressed in this article are solely those of the authors and do not necessarily represent those of their affiliated organizations, or those of the publisher, the editors and the reviewers. Any product that may be evaluated in this article, or claim that may be made by its manufacturer, is not guaranteed or endorsed by the publisher.
Supplementary Material
The Supplementary Material for this article can be found online at: https://www.frontiersin.org/articles/10.3389/fendo.2022.780979/full#supplementary-material
Supplementary Figure 1 | Difference in the circulating LH level between patients with T2DM and non-T2DM controls stratified by age.
References
1. Chatterjee S, Khunti K, Davies MJ. Type 2 Diabetes. Lancet (2017) 389:2239–51. doi: 10.1016/S0140-6736(17)30058-2
2. Lascar N, Brown J, Pattison H, Barnett AH, Bailey CJ, Bellary S. Type 2 Diabetes in Adolescents and Young Adults. Lancet Diabetes Endocrinol (2018) 6:69–80. doi: 10.1016/S2213-8587(17)30186-9
3. Thong EP, Codner E, Laven JSE, Teede H. Diabetes: A Metabolic and Reproductive Disorder in Women. Lancet Diabetes Endocrinol (2020) 8:134–49. doi: 10.1016/S2213-8587(19)30345-6
4. Park SU, Walsh L, Berkowitz KM. Mechanisms of Ovarian Aging. Reproduction (2021) 162(2):R19–33. doi: 10.1530/REP-21-0022
5. Das M, Sauceda C, Webster NJG. Mitochondrial Dysfunction in Obesity and Reproduction. Endocrinology (2021) 162(1):bqaa158. doi: 10.1210/endocr/bqaa158
6. Wellons MF, Matthews JJ, Kim C. Ovarian Aging in Women With Diabetes: An Overview. Maturitas (2017) 96:109–13. doi: 10.1016/j.maturitas.2016.11.019
7. Soto N, Iñiguez G, López P, Larenas G, Mujica V, Rey RA, et al. Anti-Mullerian Hormone and Inhibin B Levels as Markers of Premature Ovarian Aging and Transition to Menopause in Type 1 Diabetes Mellitus. Hum Reprod (2009) 24(11):2838–44. doi: 10.1093/humrep/dep276
8. Brand JS, Onland-Moret NC, Eijkemans MJ, Tjønneland A, Roswall N, Overvad K, et al. Diabetes and Onset of Natural Menopause: Results From the European Prospective Investigation Into Cancer and Nutrition. Hum Reprod (2015) 30(6):1491–8. doi: 10.1093/humrep/dev054
9. Isik S, Ozcan HN, Ozuguz U, Tutuncu YA, Berker D, Alimli AG, et al. Evaluation of Ovarian Reserve Based on Hormonal Parameters, Ovarian Volume, and Antral Follicle Count in Women With Type 2 Diabetes Mellitus. J Clin Endocrinol Metab (2012) 97(1):261–9. doi: 10.1210/jc.2011-1923
10. Garris DR, Garris BL. Diabetes (Db/Db) Mutation-Induced Ovarian Involution: Progressive Hypercytolipidemia. Exp Biol Med (Maywood) (2003) 228(9):1040–50. doi: 10.1177/153537020322800909
11. Monterrosa-Castro A, Portela-Buelvas K, Blumel-Mendez JE. Early and Premature Menopause in Women With Diabetes Mellitus Type 2. Expert Rev Endocrinol Metab (2014) 9:297–9. doi: 10.1586/17446651.2014.922863
12. Monterrosa-Castro A, Blumel JE, Portela-Buelvas K, Mezones-Holguin E, Baron G, Bencosme A, et al. Type II Diabetes Mellitus and Menopause: A Multinational Study. Climacteric (2013) 16:663–72. doi: 10.3109/13697137.2013.798272
13. Tabesh M, Shaw JE, Zimmet PZ, Soderberg S, Koye DN, Kowlessur S, et al. Association Between Type 2 Diabetes Mellitus and Disability: What Is the Contribution of Diabetes Risk Factors and Diabetes Complications? J Diabetes (2018) 10:744–52. doi: 10.1111/1753-0407.12659
14. López-López R, Huerta R, Malacara JM. Age at Menopause in Women With Type 2 Diabetes Mellitus. Menopause (1999) 6(2):174–8. doi: 10.1097/00042192-199906020-00016
15. Bhupathiraju SN, Hu FB. Epidemiology of Obesity and Diabetes and Their Cardiovascular Complications. Circ Res (2016) 118:1723–35. doi: 10.1161/CIRCRESAHA.115.306825
16. George SM, Ballard-Barbash R, Manson JE, Reedy J, Shikany JM, Subar AF, et al. Comparing Indices of Diet Quality With Chronic Disease Mortality Risk in Postmenopausal Women in the Women's Health Initiative Observational Study: Evidence to Inform National Dietary Guidance. Am J Epidemiol (2014) 180:616–25. doi: 10.1093/aje/kwu173
17. Tang O, Matsushita K, Coresh J, Sharrett AR, McEvoy JW, Windham BG, et al. Mortality Implications of Prediabetes and Diabetes in Older Adults. Diabetes Care (2020) 43:382–8. doi: 10.2337/dc19-1221
18. Wang N, Shao H, Chen Y, Xia F, Chi C, Li Q, et al. Follicle-Stimulating Hormone, Its Association With Cardiometabolic Risk Factors, and 10-Year Risk of Cardiovascular Disease in Postmenopausal Women. J Am Heart Assoc (2017) 6:2. doi: 10.1161/JAHA.117.005918
19. Peters SA, Huxley RR, Woodward M. Diabetes as Risk Factor for Incident Coronary Heart Disease in Women Compared With Men: A Systematic Review and Meta-Analysis of 64 Cohorts Including 858,507 Individuals and 28,203 Coronary Events. Diabetes Tologia (2014) 57:1542–51. doi: 10.1007/s00125-014-3260-6
20. Wu L, Parhofer KG. Diabetic Dyslipidemia. Metabol: Clin Exp (2014) 63:1469–79. doi: 10.1016/j.metabol.2014.08.010
21. Itoh H, Komuro I, Takeuchi M, Akasaka T, Daida H, Egashira Y, et al. Achieving LDL Cholesterol Target Levels <1.81 Mmol/L may Provide Extra Cardiovascular Protection in Patients at High Risk: Exploratory Analysis of the Standard Versus Intensive Statin Therapy for Patients With Hypercholesterolaemia and Diabetic Retinopathy Study. Diabetes Obes Metab (2019) 21:791–800. doi: 10.1111/dom.13575
22. Farkouh ME, Godoy LC, Brooks MM, Mancini GBJ, Vlachos H, Bittner VA, et al. Influence of LDL-Cholesterol Lowering on Cardiovascular Outcomes in Patients With Diabetes Mellitus Undergoing Coronary Revascularization. J Am Coll Cardiol (2020) 76:2197–207. doi: 10.1016/j.jacc.2020.09.536
23. Cho KI, Yu J, Hayashi T, Han SH, Koh KK. Strategies to Overcome Residual Risk During Statins Era. Circ J (2019) 83:1973–9. doi: 10.1253/circj.CJ-19-0624
24. Berman AN, Blankstein R. Optimizing Dyslipidemia Management for the Prevention of Cardiovascular Disease: A Focus on Risk Assessment and Therapeutic Options. Curr Cardiol Rep (2019) 21:110. doi: 10.1007/s11886-019-1175-z
25. Wadhera RK, Steen DL, Khan I, Giugliano RP, Foody JM. A Review of Low-Density Lipoprotein Cholesterol, Treatment Strategies, and Its Impact on Cardiovascular Disease Morbidity and Mortality. J Clin Lipidol (2016) 10:472–89. doi: 10.1016/j.jacl.2015.11.010
26. Palmisano BT, Zhu L, Eckel RH, Stafford JM. Sex Differences in Lipid and Lipoprotein Metabolism. Mol Metab (2018) 15:45–55. doi: 10.1016/j.molmet.2018.05.008
27. Palmisano BT, Zhu L, Stafford JM. Role of Estrogens in the Regulation of Liver Lipid Metabolism. Adv Exp Med Biol (2017) 1043:227–56. doi: 10.1007/978-3-319-70178-3_12
28. Wang X, Magkos F, Mittendorfer B. Sex Differences in Lipid and Lipoprotein Metabolism: It's Not Just About Sex Hormones. J Clin Endocrinol Metab (2011) 96:885–93. doi: 10.1210/jc.2010-2061
29. Serviente C, Tuomainen TP, Virtanen J, Witkowski S, Niskanen L, Bertone-Johnson E. Follicle-Stimulating Hormone Isassociated With Lipids in Postmenopausal Women. Menopause (2019) 26:540–5. doi: 10.1097/GME.0000000000001273
30. Bertone-Johnson ER, Virtanen JK, Nurmi T, Niskanen L, Mursu J, Voutilainen S, et al. Follicle-Stimulating Hormone Levels and Subclinical Atherosclerosis in Older Postmenopausal Women. Am J Epidemiol (2018) 187:16–26. doi: 10.1093/aje/kwx174
31. Bertone-Johnson ER, Virtanen JK, Niskanen L, Nurmi T, Ronkainen K, Voutilainen S, et al. Association of Follicle-Stimulating Hormone Levels and Risk of Type 2 Diabetes in Older Postmenopausal Women. Menopause (2017) 24:796–802. doi: 10.1097/GME.0000000000000834
32. El Khoudary SR, Wildman RP, Matthews K, Thurston RC, Bromberger JT, Sutton-Tyrrell K. Endogenous Sex Hormones Impact the Progression of Subclinical Atherosclerosis in Women During the Menopausal Transition. Atherosclerosis (2012) 225:180–6. doi: 10.1016/j.atherosclerosis.2012.07.025
33. El Khoudary SR, Santoro N, Chen HY, Tepper PG, Brooks MM, Thurston RC, et al. Trajectories of Estradiol and Follicle-Stimulating Hormone Over the Menopause Transition and Early Markers of Atherosclerosis After Menopause. Eur J Prev Cardiol (2016) 23:694–703. doi: 10.1177/2047487315607044
34. Munir JA, Wu H, Bauer K, Bindeman J, Byrd C, Feuerstein IM, et al. The Perimenopausal Atherosclerosis Transition: Relationships Between Calcified and Noncalcified Coronary, Aortic, and Carotid Atherosclerosis and Risk Factors and Hormone Levels. Menopause (2012) 19:10–5. doi: 10.1097/gme.0b013e318221bc8d
35. Celestino Catao Da Silva D, Nogueira De Almeida Vasconcelos A, Cleto Maria Cerqueira J, De Oliveira Cipriano Torres D, Oliveira Dos Santos AC, De Lima Ferreira Fernandes Costa H, et al. Endogenous Sex Hormones Are Not Associated With Subclinical Atherosclerosis in Menopausal Women. Minerva Ginecol (2013) 65:297–302. doi: 10.1210/jcem.76.4.8473380
36. Wang N, Kuang L, Han B, Li Q, Chen Y, Zhu C, et al. Follicle-Stimulating Hormone Associates With Prediabetes and Diabetes in Postmenopausal Women. Acta Diabetol (2016) 53:227–36. doi: 10.1007/s00592-015-0769-1
37. Quinn MA, Ruffe H, Brown JB, Ennis G. Circulating Gonadotrophins and Urinary Oestrogens in Postmenopausal Diabetic Women. Aust New Z J Obstet Gynaecol (1981) 21:234–6. doi: 10.1111/j.1479-828X.1981.tb00138.x
38. Wang X, Xian T, Jia X, Zhang L, Liu L, Man F, et al. A Cross-Sectional Study on the Associations of Insulin Resistance With Sex Hormone, Abnormal Lipid Metabolism in T2DM and IGT Patients. Medicine (2017) 96:e7378. doi: 10.1097/MD.0000000000007378
39. South SA, Asplin CM, Carlsen EC, Booth RA Jr., Weltman JY, Johnson ML, et al. Alterations in Luteinizing Hormone Secretory Activity in Women With Insulin-Dependent Diabetes Mellitus and Secondary Amenorrhea. J Clin Endocrinol Metab (1993) 76:1048–53.
40. Blades RA, Bryant KR, Whitehead SA. Feedback Effects of Steroids and Gonadotrophin Control in Adult Rats With Streptozotocin-Induced Diabetes Mellitus. Diabetes Tologia (1985) 28:348–54. doi: 10.1007/BF00283142
41. Distiller LA, Sagel J, Morley JE, Seftel HC. Pituitary Responsiveness to Luteinizing Hormone-Releasing Hormone in Insulin-Dependent Diabetes Mellitus. Diabetes (1975) 24:378–80. doi: 10.2337/diab.24.4.378
42. Katayama S, Brownscheidle CM, Wootten V, Lee JB, Shimaoka K. Absent or Delayed Preovulatory Luteinizing Hormone Surge in Experimental Diabetes Mellitus. Diabetes (1984) 33:324–7. doi: 10.2337/diab.33.4.324
43. Broekmans FJ, Soules MR, Fauser BC. Ovarian Aging: Mechanisms and Clinical Consequences. Endocr Rev (2009) 30(5):465–93. doi: 10.1210/er.2009-0006
44. Yaribeygi H, Sathyapalan T, Atkin SL, Sahebkar A. Molecular Mechanisms Linking Oxidative Stress and Diabetes Mellitus. Oxid Med Cell Longev (2020) 2020:8609213. doi: 10.1155/2020/8609213
45. Daryabor G, Atashzar MR, Kabelitz D, Meri S, Kalantar K. The Effects of Type 2 Diabetes Mellitus on Organ Metabolism and the Immune System. Front Immunol (2020) 11:1582:1582. doi: 10.3389/fimmu.2020.01582
46. Yang Z, Tang Z, Cao X, Xie Q, Hu C, Zhong Z, et al. Controlling Chronic Low-Grade Inflammation to Improve Follicle Development and Survival. Am J Reprod Immunol (2020) 84(2):e13265. doi: 10.1111/aji.13265
47. Snider AP, Wood JR. Obesity Induces Ovarian Inflammation and Reduces Oocyte Quality. Reproduction (2019) 158(3):R79–90. doi: 10.1530/REP-18-0583
48. Huang Y, Hu C, Ye H, Luo R, Fu X, Li X, et al. Inflamm-Aging: A New Mechanism Affecting Premature Ovarian Insufficiency. J Immunol Res (2019) 2019:8069898. doi: 10.1155/2019/8069898
49. Yang L, Chen Y, Liu Y, Xing Y, Miao C, Zhao Y, et al. The Role of Oxidative Stress and Natural Antioxidants in Ovarian Aging. Front Pharmacol (2021) 11:617843:617843. doi: 10.3389/fphar.2020.617843
50. Briley SM, Jasti S, McCracken JM, Hornick JE, Fegley B, Pritchard MT, et al. Reproductive Age-Associated Fibrosis in the Stroma of the Mammalian Ovary. Reproduction (2016) 152(3):245–60. doi: 10.1530/REP-16-0129
51. Sohel MMH, Akyuz B, Konca Y, Arslan K, Sariozkan S, Cinar MU. Oxidative Stress Modulates the Expression of Apoptosis-Associated microRNAs in Bovine Granulosa Cells In Vitro. Cell Tissue Res (2019) 376(2):295–308. doi: 10.1007/s00441-019-02990-3
52. Yang H, Xie Y, Yang D, Ren D. Oxidative Stress-Induced Apoptosis in Granulosa Cells Involves JNK, P53 and Puma. Oncotarget (2017) 8(15):25310–22. doi: 10.18632/oncotarget.15813
53. Busnelli A, Navarra A, Levi-Setti PE. Qualitative and Quantitative Ovarian and Peripheral Blood Mitochondrial DNA (mtDNA) Alterations: Mechanisms and Implications for Female Fertility. Antioxid (Basel) (2021) 10(1):55. doi: 10.3390/antiox10010055
54. Yi Y, El Khoudary SR, Buchanich JM, Miller RG, Rubinstein D, Orchard TJ, et al. Association of Age at Diabetes Complication Diagnosis With Age at Natural Menopause in Women With Type 1 Diabetes: The Pittsburgh Epidemiology of Diabetes Complications (EDC) Study. J Diabetes Complications (2021) 35(3):107832. doi: 10.1016/j.jdiacomp.2020.107832
55. Pertynska-Marczewska M, Diamanti-Kandarakis E. Aging Ovary and the Role for Advanced Glycation End Products. Menopause (2017) 24(3):345–51. doi: 10.1097/GME.0000000000000755
56. Li CJ, Lin LT, Tsai HW, Chern CU, Wen ZH, Wang PH, et al. The Molecular Regulation in the Pathophysiology in Ovarian Aging. Aging Dis (2021) 12(3):934–49. doi: 10.14336/AD.2020.1113
57. Takeo S, Kimura K, Shirasuna K, Kuwayama T, Iwata H. Age-Associated Deterioration in Follicular Fluid Induces a Decline in Bovine Oocyte Quality. Reprod Fertil Dev (2017) 29(4):759–67. doi: 10.1071/RD15228
58. Wang Q, Ferreira DLS, Nelson SM, Sattar N, Ala-Korpela M, Lawlor DA. Metabolic Characterization of Menopause: Cross-Sectional and Longitudinal Evidence. BMC Med (2018) 16(1):17. doi: 10.1186/s12916-018-1008-8
59. Ribas V, Nguyen MT, Henstridge DC, Nguyen AK, Beaven SW, Watt MJ, et al. Impaired Oxidative Metabolism and Inflammation Are Associated With Insulin Resistance in ERalpha-Deficient Mice. Am J Physiol Endocrinol Metab (2010) 298(2):E304–19. doi: 10.1152/ajpendo.00504.2009
60. Roelfsema F, Yang RJ, Veldhuis JD. Differential Effects of Estradiol and Progesterone on Cardiovascular Risk Factors in Postmenopausal Women. J Endocr Soc (2018) 2(7):794–805. doi: 10.1210/js.2018-00073
61. Schnatz PF, Jiang X, Aragaki AK, Nudy M, O'Sullivan DM, Williams M, et al. Effects of Calcium, Vitamin D, and Hormone Therapy on Cardiovascular Disease Risk Factors in the Women's Health Initiative: A Randomized Controlled Trial. Obstet Gynecol (2017) 129(1):121–9. doi: 10.1097/AOG.0000000000001774
62. Costa GBC, Carneiro G, Umeda L, Pardini D, Zanella MT. Influence of Menopausal Hormone Therapy on Body Composition and Metabolic Parameters. Biores Open Access (2020) 9(1):80–5. doi: 10.1089/biores.2019.0050
63. Podfigurna A, Stellmach A, Szeliga A, Czyzyk A, Meczekalski B. Metabolic Profile of Patients With Premature Ovarian Insufficiency. J Clin Med (2018) 7(10):374. doi: 10.3390/jcm7100374
64. Gulhan I, Bozkaya G, Uyar I, Oztekin D, Pamuk BO, Dogan E. Serum Lipid Levels in Women With Premature Ovarian Failure. Menopause (2012) 19(11):1231–4. doi: 10.1097/gme.0b013e318254102b
65. Sharma G, Hu C, Staquicini DI, Brigman JL, Liu M, Mauvais-Jarvis F, et al. Preclinical Efficacy of the GPER-Selective Agonist G-1 in Mouse Models of Obesity and Diabetes. Sci Transl Med (2020) 12(528):eaau5956. doi: 10.1126/scitranslmed.aau5956
66. Meng Y, Zong L. Estrogen Stimulates SREBP2 Expression in Hepatic Cell Lines via an Estrogen Response Element in the SREBP2 Promoter. Cell Mol Biol Lett (2019) 24:65. doi: 10.1186/s11658-019-0194-5
67. Fu W, Gao XP, Zhang S, Dai YP, Zou WJ, Yue LM. 17β-Estradiol Inhibits PCSK9-Mediated LDLR Degradation Through GPER/PLC Activation in HepG2 Cells. Front Endocrinol (Lausanne) (2020) 10:930. doi: 10.3389/fendo.2019.00930
68. Song Y, Wang ES, Xing LL, Shi S, Qu F, Zhang D, et al. Follicle-Stimulating Hormone Induces Postmenopausal Dyslipidemia Through Inhibiting Hepatic Cholesterol Metabolism. J Clin Endocrinol Metab (2016) 101:254–63. doi: 10.1210/jc.2015-2724
69. Honour JW. Biochemistry of the Menopause. Ann Clin Biochem (2018) 55:18–33. doi: 10.1177/0004563217739930
70. Arnett DK, Blumenthal RS, Albert MA, Buroker AB, Goldberger ZD, Hahn EJ, et al. ACC/AHA Guideline on the Primary Prevention of Cardiovascular Disease: A Report of the American College of Cardiology/American Heart Association Task Force on Clinical Practice Guidelines. Circulation (2019) 2019:140 e596–e646. doi: 10.1161/CIR.0000000000000678
Keywords: ovarian aging, type 2 diabetes mellitus, luteinizing hormone, follicle stimulating hormone, lipids
Citation: Wang Y and Wang Y (2022) Accelerated Ovarian Aging Among Type 2 Diabetes Patients and Its Association With Adverse Lipid Profile. Front. Endocrinol. 13:780979. doi: 10.3389/fendo.2022.780979
Received: 22 September 2021; Accepted: 24 February 2022;
Published: 30 March 2022.
Edited by:
Marc R. Blackman, Washington DC VA Medical Center, United StatesReviewed by:
Wei Wang, Second Hospital of Hebei Medical University, ChinaXiaodong Sun, Affiliated Hospital of Weifang Medical University, China
An Jin Zhang, Shanghai University of Medicine and Health Sciences, China
Qing He, Tianjin Medical University General Hospital, China
Copyright © 2022 Wang and Wang. This is an open-access article distributed under the terms of the Creative Commons Attribution License (CC BY). The use, distribution or reproduction in other forums is permitted, provided the original author(s) and the copyright owner(s) are credited and that the original publication in this journal is cited, in accordance with accepted academic practice. No use, distribution or reproduction is permitted which does not comply with these terms.
*Correspondence: Yangang Wang, d2FuZ3lnMTk2NkAxMjYuY29t