- Université Paris Cité, Unité de Biologie Fonctionnelle et Adaptative, CNRS UMR 8251, Team “Biologie et Pathologie du Pancréas Endocrine”, Paris, France
Diabetes Mellitus is a metabolic disorder characterized by a chronic hyperglycemia due to an impaired insulin secretion and a decreased in peripheral insulin sensitivity. This disease is a major public health problem due to it sharp prevalence. Therefore, it is crucial to readapt therapeutic approaches for the treatment of this pathology. One of the strategies would be through P2-type purinergic receptors pathway via ATP binding. In addition to its well-known role as an intracellular energy intermediary in numerous biochemical and physiological processes, ATP is also an important extracellular signaling molecule. ATP mediates its effects by binding and activating two classes of P2 purinoreceptors: P2X receptors that are ligand-gated ion channel receptors, existing in seven isoforms (P2X 1 to 7) and P2Y receptors that are G-protein coupled receptors, existing in eight isoforms (P2Y 1/2/4/6/11/12/13/14). These receptors are ubiquitously distributed and involved in numerous physiological processes in several tissues. The concept of purinergic signaling, originally formulated by Geoffrey Burnstock (1929-2020), was also found to mediate various responses in the pancreas. Several studies have shown that P2 receptors are expressed in the endocrine pancreas, notably in β cells, where ATP could modulate their function but also their plasticity and thus play a physiological role in stimulating insulin secretion to face some metabolic demands. In this review, we provide a historical perspective and summarize current knowledge on P2-type purinergic signaling in the regulation of pancreatic β-cell functional plasticity, which would be a promising novel therapeutic approach for the treatment of type 2 diabetes.
1 Introduction on purinergic signaling
The intracellular adenosine triphosphate (ATP) was first known for its role as an energy molecule involved in the storage, transport and energy regulation of cellular metabolism. In pancreatic β-cells, intracellular ATP plays a major role in stimulating insulin secretion and maintaining glucose homeostasis. ATP, generated by glycolysis and oxidative phosphorylation, causes the closure of ATP-dependent potassium channels (K+-ATP), leading to an accumulation of intracellular potassium (1, 2) and to membrane depolarization. This will induce the opening of voltage-gated calcium channels (VDCC) and an influx of Ca2+, resulting in an increase in the concentration of intracellular calcium (3). The important influx of calcium is the main trigger of insulin granule exocytosis (4–8).
In addition to its intracellular role, ATP with ADP and adenosine has also been recognized as an extracellular signaling molecule that can stimulate signaling pathways by binding to purinergic receptors. This concept was originally proposed and formulated by Geoffrey Burnstock in 1972 (9, 10). Extracellular ATP can be released by various systems (exocytosis of secretory granules, vesicular transports and membrane channels such as connexin, pannexin hemi-channels and voltage-gated anion channels) and stimulate purinergic receptors. Nevertheless, ATP can also be released from damaged cells (11). The increase in ATP levels in the extracellular space activates ectonucleotidases (ectonucleoside triphosphate diphosphohydrolases type I or NTPDases) which will rapidly degrade ATP into ADP and adenosine which can also activate some purinergic receptors (12).
Purinergic signaling is the result from the binding of extracellular ATP and its metabolites to purinergic receptors: P1 receptors which have a high affinity for adenosine, a degradation product of ATP by ectonucleotidases and P2 receptors that are activated mainly by ATP, but also by ADP, UTP and UDP and divided into two subgroups P2X and P2Y receptors.
P2X receptors are ionotropic, ligand-gated ion channel receptors which are permeable to Na+, K+ and Ca2+ ions after activation by ATP (Figure 1). To date, seven subunits (P2X 1→7) has been identified in many cells of various species, including humans (hP2XR), rats (rP2XR), mice (mP2XR) and zebrafish (zP2X4.1R), where they contribute to the control of many physiological functions (13–19). The structural properties and pharmacology on this class of receptors have been well described in a recent review in 2021 (20).
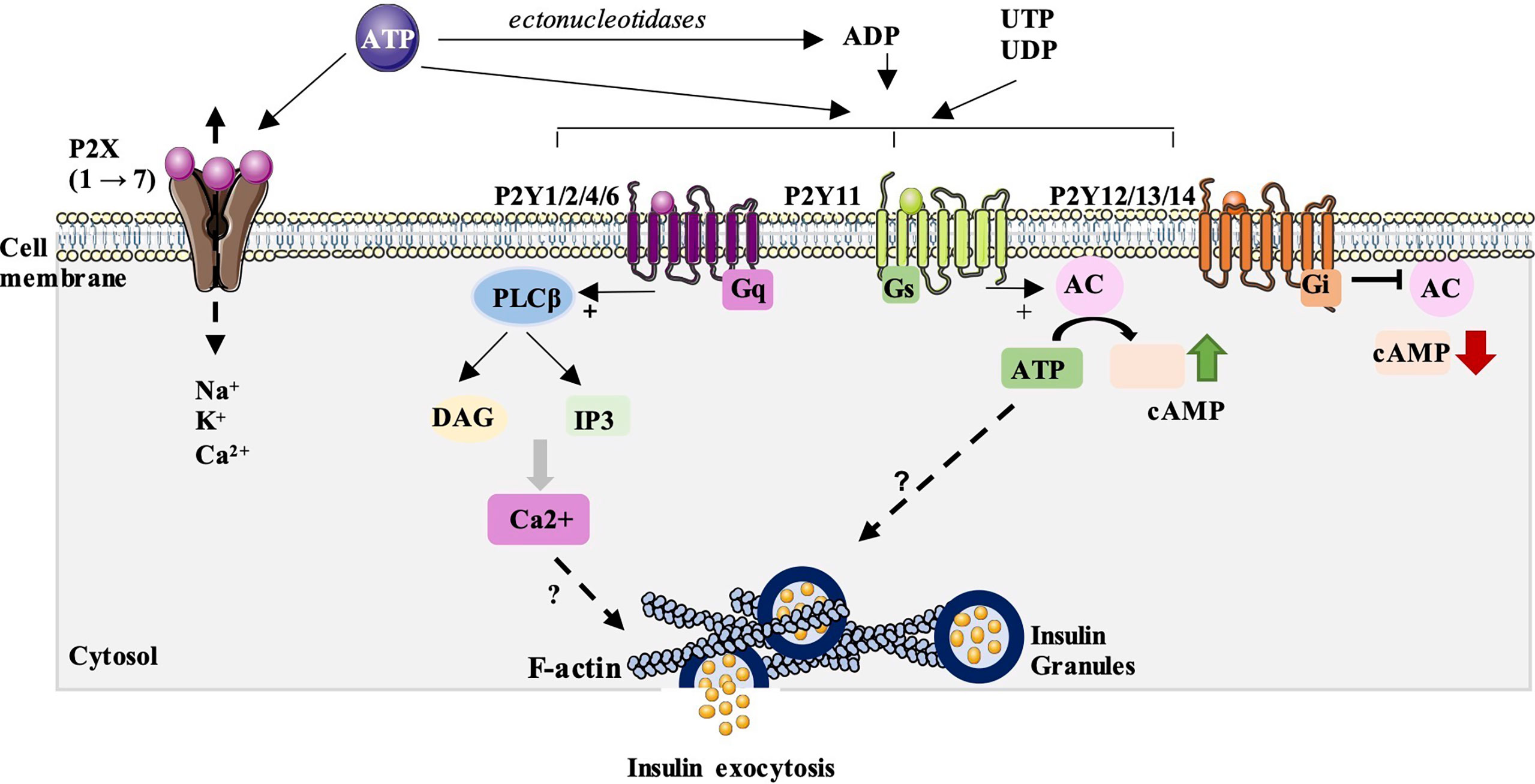
Figure 1 P2 purinergic receptors signaling and their possible involvement in insulin secretion. P2X receptors are ionotropic, ligand-gated ion channel receptors which are permeable to Na+, K+ and Ca2+ ions after activation by ATP. To date, seven subunits (P2X1→7) has been identified. Their impact on insulin secretion is quite controversial, with stimulatory and/or inhibitory effects described. P2Y receptors are metabotropic receptors from the large family of G protein-coupled receptors. They can activated by ATP but also by ADP, UTP and UDP. Eight receptor subtypes have been identified and subdivided into two P2Y receptor subfamilies: P2Y1, 2, 4 and 6 which are mainly coupled to Gq proteins and activate phospholipase C β pathway (PLCβ). P2Y11 can also be coupled to Gs proteins and thus stimulate adenylate cyclase (AC) and the synthesis of the second messenger, cAMP. The second group includes the P2Y12, 13 and 14 isoforms and are mainly coupled to Gi proteins and inhibit adenylate cyclase and cAMP synthesis. A study done by our team has showed that P2Y activation can stimulate glucose-induced insulin secretion by acting on the distal steps of insulin exocytosis including the subcortical actin network and insulin granules exocytosis. However, the mechanisms involved in this stimulation remains unknown.
P2Y receptors are metabotropic receptors from the large family of G protein-coupled receptors. To date, eight receptor subtypes have been identified and functionally defined (P2Y1, 2, 4,6, 11, 12, 13, 14) (21–24). These eight subtypes have been subdivided into two P2Y receptor subfamilies based on their associated signaling pathways (23, 25) (Figure 1). The first group consists of isoforms P2Y1, 2, 4, 6 and 11. These subtypes are mainly coupled to Gq/G11 proteins which activate phospholipase C (PLC), thus inducing the synthesis of two second messengers: IP3, which mobilizes calcium from the endoplasmic reticulum, and diacylglycerol (DAG) which stimulates the protein kinase C pathway. P2Y11 can also be coupled to Gs proteins and thus stimulate adenylate cyclase and the synthesis of the second messenger, cAMP. The second group includes the P2Y12, 13 and 14 isoforms and are mainly coupled to Gi/o proteins and inhibit adenylate cyclase and cAMP synthesis. The structural properties and pharmacology of these receptors have been well described in the recent review of Jacobson et al. (26).
P2-Purinergic receptors are widely distributed in all tissues and involved in many biological activities such as exocrine and endocrine secretions, platelet aggregation, vascular tone, nociception, neuromodulation but also in cell proliferation, migration, differentiation, inflammation and cell death (16, 27, 28). Their ubiquitous expression in metabolic tissues, notably in the pancreas, have also suggested quite early a role for these receptors in the regulation of the metabolic state and glucose metabolism. In exocrine pancreas, several studies have shown the expression of P2X and P2Y subtypes in acinar and ductal cells in many species (rat, mouse and human) and their roles in modulating cell proliferation and exocrine secretion (29, 30). In α-cells, there is little and controversial information on the role of purinergic signalling in these cells. Some reports have shown that ATP, by activating P2Y receptors, can stimulate glucagon secretion in mouse islets (31) while others have shown an inhibitory effect of P2Y-signaling (32). Thus, the role of purinergic signaling in glucagon secretion needs to be further investigated in order to better understand its involvement in α-cells.
The aim of this review is to gather, at best, the studies carried out to better understand the involvement of P2 purinergic signaling in the endocrine pancreas, most notably in pancreatic β-cells and the possible therapeutic potential that these receptors may present for the treatment of diabetes pathogenesis.
2 Extracellular ATP in regulation of pancreatic ß-cell function
2.1 Modulation of insulin release by P2R signaling
The first study on the role of purinergic signaling in insulin secretion was first carried out by Rodrigue-Candela et al. in 1963. They have shown that ATP stimulates insulin secretion from β-cells of rabbit pancreas (33). These observations were later confirmed on primate pancreas (34). The stimulatory effect of ATP on insulin secretion was then demonstrated in isolated and perfused rat pancreases (35–37) and on hamster pancreases (38). The use of more potent agonists, in particular, ADP and its analogues (α-β-methylene, ADPβS or ADPγS) have made it possible to show greater stimulation of insulin secretion but which requires stimulating concentrations of glucose (8.3mM) (39–42) while the use of a P2 receptor antagonist abolishes the stimulatory effect of ATP on insulin secretion (37). Stimulation by glucose or by potassium channel inhibitors such as glibenclamide has made it possible to demonstrate the presence of ATP in the secretory granules and in particular in the insulin granules (43–45). ATP was also shown to be co-secreted with insulin during granule exocytosis and amplified glucose-induced insulin secretion by stimulating P2 receptors (46–48) and modulating the biphasic response insulin secretion in a dose-dependent manner (49, 50). Furthermore, the study by Fernandez-Alvarez et al. showed that P2Y receptor agonists (α, β-methylene ATP or ADPβS) amplify insulin secretion from human islets (51).
Interestingly, it has been reported that ATP can be released during the process of “kiss and run” exocytosis without insulin being secreted (48) suggesting that ATP can act as an autocrine regulator of insulin secretion. ATP can also be released from nerve endings and play a role in the neuronal control of insulin secretion (52). Studies have shown that ATP can be stored and co-secreted with acetylcholine and act synergistically on insulin secretion, particularly in the cephalic phase (pre-prandial period) (53). It was shown that ATP, by binding to P2X or P2Y receptors activates various intracellular pathways, including the K+/ATP-dependent pathway or the Ca2+ pathway. Consequently, it increases β-cell insulin secretion from many models including human β-cells (54, 55).
At the opposite, it has been shown that ATP can also exert an inhibitory effect on insulin secretion. Contrary to what has been observed on the stimulatory effect of ATP in rats, a decrease in insulin secretion in response to ATP has been shown in mice (39). This inhibition was later confirmed by two studies which showed, using membrane capacitance and calcium flux measurement techniques, on MIN6 β-cells, that ATP reduces by 60% the exocytosis induced by depolarization, by interfering with the exocytosis machinery (56). The ATP inhibitory effect observed in mice does not seem to be due to the differences in the expression profile of these receptors between the different species since a study reported that the use of P2 receptor antagonist amplifies the insulin secretion of rat islets in response to stimulating concentrations of glucose (57).
2.2 P2X signaling in pancreatic β-cells
Using various approaches, numerous studies, including ours, have identified the presence of the seven subtypes of P2X receptors (1 to 7) in rat and mouse islet β-cells and the INS1 β-cell line but their role remains so contradictory (42, 58, 59). Electrophysiological, RT-PCR and immunocytochemical studies showed that P2X1, P2X3 and P2X4 are expressed in adult rats and mice β-cells (60–62) and that P2X1 and P2X3 can be rapidly desensitized, suggesting that the paracrine or neuronal activation of these receptors would contribute to maintain insulin secretion induced by high-glucose or acetylcholine (62). The expression of P2X3 and P2X5 has also been reported in human β-cells and it was shown that P2X3 isoform could have an amplifying effect on insulin secretion (55). However, in the rat β-cell line, P2X3 receptors appear to negatively regulate insulin secretion independently of extracellular glucose concentrations (59). As for the P2X7 receptors, several studies have shown that P2X7 receptors are expressed in mouse islets and β-cell lines (MIN6). These same studies have reported that P2X7 is downregulated in β-cells from type 2 diabetic patients but highly expressed in obese patients and may be involved in the secretion of pro-inflammatory cytokines such as interleukin-1β (63, 64). In INS-1E β-cell line, the activation of P2X7 receptors regulates ATP secretion and intracellular calcium signaling and consequently regulates insulin secretion (29). However, it was reported that activation of P2X7 inhibits insulin secretion in response to glucose (65). Studies carried out on knockout mice for the P2X7 receptor showed the impact of these receptors on glucose homeostasis (63). P2X7-/- mice exhibit hyperglycemia, glucose intolerance, and impaired β-cell function when fed a high-fat diet and are unable to increase β-cell mass in response to increased nutrient load. The islets from these mice also show increased β-cell apoptosis (63). Several studies have also linked the P2X7 isoform to the pathogenesis of type 1 diabetes (T1D). Indeed, knockout of the P2X7 receptor prevents streptozotocin-induced T1D in mice (66) and inhibition of this receptor by oxidized ATP delays islet graft rejection (67).
2.3 P2Y signaling in pancreatic β-cells
Numerous studies have reported the impact of P2Y receptors in modulating insulin secretion in the pancreatic β-cells. Bertrand et al. showed for the first time that P2Y receptors can modulate the biphasic response of insulin secretion in rat pancreas (49). This same team later reported that ADPβS, a preferential agonist of P2Y1 receptors, activates insulin secretion from perfused pancreas and isolated rat islets (41, 50, 68). Fischer et al. also showed that the use of a potent P2Y1 agonist significantly amplifies the insulin secretion in response to glucose from isolated islets and perfused rat pancreases (69). More recently, the activation of P2Ys, with more specific agonists than previously used, including 2Me(S)ATP-α-β, has been shown to potentiate insulin secretion in response to glucose from rat islets (70). A study by Léon et al. on P2Y1-/- mice shows that these mice develop hyperglycemia as well as a tendency towards glucose intolerance and increased insulin secretion compared to WT mice. These results indicate that P2Y1 receptor play an important role in maintaining glucose homeostasis (71). Nevertheless, the study by Ohtani et al. shows that activation of P2Y1 and P2Y6 decreases glucose-induced insulin secretion from mouse islets (72). Later, this same team showed that extracellular ATP increased insulin secretion from mouse islets, but that exposure to high concentrations of ATP counteracted this increase, probably via the P2Y1 and P2X4 receptors (73).
We and others have subsequently shown, using RT-PCR and western blot approaches, that the β-cell line derived from rat insulinoma, INS-1, expresses the six isoforms of P2Y (P2Y1, 2, 4, 6, 12 and 13) (59, 74, 75). P2Y1, P2Y6 and P2Y13 are also expressed in mouse β-TC6 cells and in mouse β cells and islets (31, 72). In humans, the P2Y2, 4, 11 and 12 isoforms have been cloned and characterized in the human pancreas (76, 77). In fact, our study carried out in type 2 diabetic rat model, the Goto-Kakizaki rat and in Wistar healthy rats showed that the level of expression of P2Y receptors varies significantly according to glucose environment. We found that P2Y1 was significantly downregulated compared to Wistar rats but the level of expression of P2Y4 was not modified. However, the level of expression of P2Y12 and P2Y13 was significantly increased in Goto-Kakizaki diabetic islets (75). Interestingly, in that same study, we showed that the activation of P2Y receptors in rat β-cell line, INS-1 832/13 β-cells, with impaired insulin secretion following exposure to elevated glucose levels, restores glucose-stimulated insulin secretion (GSIS) competence through the distal steps of insulin exocytosis, by increasing insulin granules exocytosis and the reorganization of the subcortical actin network (Figure 1). The activation of P2Y receptors with 2Me(S)ATP also amplified GSIS of healthy Wistar rat islets and, more interesting, partially restored the altered GSIS of diabetic islets from the type 2 diabetic Goto-Kakizaki (GK) rats. Our results emphasize the beneficial effects of the activation of P2Y receptors in insulin secretion on pancreatic β-cells especially in a diabetic context (75). In addition, we showed that the injection of glucose with 2Me(S)ATP decreases significantly the blood glucose levels in the type 2 diabetic GK rats compared to untreated GK rats and this by increasing the glucose-induced insulin secretion and blood glucose clearance by peripheral tissues. Thus, our results show that acute injection of 2Me(S)ATP restores partially the diabetic state of this diabetic rats (75). Our study, along with those cited above, suggests that P2-purinergic signaling may be a good candidate for the treatment of metabolic diseases such as obesity and diabetes.
3 P2 receptors in regulation of ß-cell survival
In addition to all these studies showing the important role of purinergic P2 signaling on the insulin secretory function of β-cells, the presence of numerous isoforms of P2 receptors in pancreatic islets has also raised interest in their role in other processes such as their possible involvement in inflammatory, proliferative and apoptotic pathways (Figure 2).
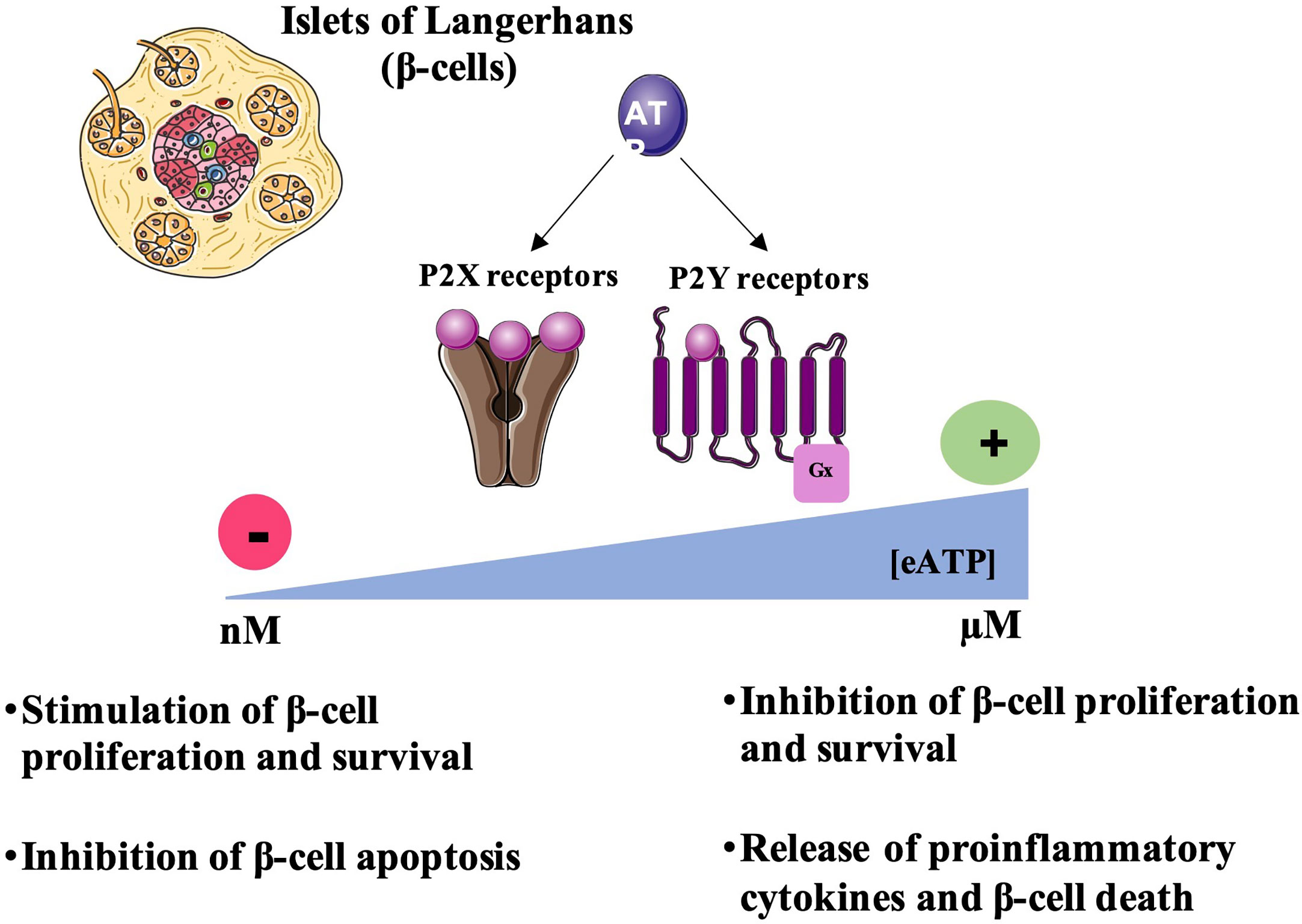
Figure 2 Regulation of β-cell survival by P2R signaling. Under physiological conditions, extracellular ATP concentration [eATP] is in the nanomolar range. Thus, eATP by activating P2 receptors can stimulate β-cell proliferation and survival and inhibit the release of proinflammatory cytokines preventing β-cell death. However, at the inflammation sites or tissue damage, eATP concentrations increases and reaches micromolar ranges activating P2 receptors thus inhibiting β-cell proliferation and inducing the release of proinflammatory cytokines leading to β-cell death.
Numerous studies have shown that ATP and its analogues could be involved in tissue remodeling, in particular in the regulation and regeneration of tissues such as the nervous system or the liver (78). A study in the brain have shown that the stimulation of purinergic receptors induces the proliferation of astrocytes indicating the role of these receptors in the remodeling and in the proliferation of neuronal cells (79, 80). Another study carried out on P2X4-/- mice showed that the P2X4 receptor contributes to hepatic regeneration after hepatectomy, suggesting the role of this isoform in the protection and proliferation of hepatocytes (81). In the pancreas, it was shown that the activation of P2Y6 isoform protects β-cells from cell death induced by the pro-inflammatory cytokine, TNF-α (Tumor Necrosis Factor-α) while the activation of P2Y13 in mouse β-cell line, MIN6C4, stimulates caspase-3 activity and reduces cell proliferation (82). These effects were abolished using P2Y13 antagonist, MRS2211 (82). ATP-induced apoptosis was also shown in the HIT-T15 hamster β cell line (83). Moreover, in β-TC6 cells, proliferation was inhibited by micromolar concentrations of ATP, probably via the activation of P2X4 receptor. A reduction in the viability of these cells has also been noted, but the results do not support the involvement of a specific P2 receptor subtype (73). Moreover, hyperglycemia in type 2 diabetes pathogenesis promotes extracellular ATP release activating P2X7 receptors and leading to the release of proinflammatory cytokines and β-cell apoptosis (19).
The low-grade chronic inflammation observed in type 2 diabetic patients constitutes a stimulus inducing migration and infiltration of macrophages and immune cells in many tissues including the endocrine pancreas (84, 85). The consequence of this inflammation is an increased dysfunction of the islets of Langerhans which aggravates the decrease in insulin secretion and induces apoptosis of the β cells thus reducing the β-cell mass. Therefore, the reconstitution of a new pool of functional β-cells constitutes a promising therapeutic approach for the treatment of this pathology. Thus, based on the data cited previously, it would be interesting to deepen our knowledge and study the impact of P2-purinergic receptors in the induction of inflammatory and pro-apoptotic processes as well as their potential trophic effect in pancreatic β-cells, notably in diabetic models.
4 Conclusions
Since the first cloning of the P2X and P2Y receptors in the 1990s, many subtypes have been identified and their roles in large systems, tissues and cells are better characterized. The therapeutic hopes raised in the field of diabetic pathologies are an encouragement to continue efforts in this direction and to extend the field of investigation to the field of functional plasticity of pancreatic β-cells in which ATP and its purinergic receptors seem to have a physiological and/or physiopathological action.
Author contributions
NM wrote the manuscript and produced the figures. JM reviewed the manuscript. CT-C designed and critically reviewed the manuscript. All authors contributed to the article and approved the submitted version.
Funding
This work is supported by a PhD fellowship for Nour Mesto, from the Ministère de l’Enseignement Supérieur et de la Recherche.
Conflict of interests
The authors declare that the research was conducted in the absence of any commercial or financial relationships that could be construed as a potential conflict of interest.
Publisher’s note
All claims expressed in this article are solely those of the authors and do not necessarily represent those of their affiliated organizations, or those of the publisher, the editors and the reviewers. Any product that may be evaluated in this article, or claim that may be made by its manufacturer, is not guaranteed or endorsed by the publisher.
References
1. Detimary P, Van den Berghe G, Henquin JC. Concentration dependence and time course of the effects of glucose on adenine and guanine nucleotides in mouse pancreatic islets. J Biol Chem (1996) 271:20559–65. doi: 10.1074/jbc.271.34.20559
2. Tarasov A, Dusonchet J, Ashcroft F. Metabolic regulation of the pancreatic beta-cell ATP-sensitive k+ channel: a pas de deux. Diabetes (2004) 53(Suppl 3):S113–122. doi: 10.2337/diabetes.53.suppl_3.s113
3. Rorsman P, Ashcroft FM. Pancreatic β-cell electrical activity and insulin secretion: Of mice and men. Physiol Rev (2018) 98:117–214. doi: 10.1152/physrev.00008.2017
4. Henquin JC. Triggering and amplifying pathways of regulation of insulin secretion by glucose. Diabetes (2000) 49:1751–60. doi: 10.2337/diabetes.49.11.1751
5. Rorsman P, Renström E. Insulin granule dynamics in pancreatic beta cells. Diabetologia (2003) 46:1029–45. doi: 10.1007/s00125-003-1153-1
6. Henquin JC. Regulation of insulin secretion: a matter of phase control and amplitude modulation. Diabetologia (2009) 52:739–51. doi: 10.1007/s00125-009-1314-y
7. Vakilian M, Tahamtani Y, Ghaedi K. A review on insulin trafficking and exocytosis. Gene (2019) 706:52–61. doi: 10.1016/j.gene.2019.04.063
8. Kalwat MA, Scheuner D, Rodrigues-Dos-Santos K, Eizirik DL, Cobb MH. The pancreatic ß-cell response to secretory demands and adaption to stress. Endocrinology (2021) 162:bqab173. doi: 10.1210/endocr/bqab173
10. Burnstock G. Purinergic signalling: From discovery to current developments. Exp Physiol (2014) 99:16–34. doi: 10.1113/expphysiol.2013.071951
11. Burnstock G, Boeynaems J-M. Purinergic signalling and immune cells. Purinergic Signal (2014) 10:529–64. doi: 10.1007/s11302-014-9427-2
12. Zimmermann H. History of ectonucleotidases and their role in purinergic signaling. Biochem Pharmacol (2021) 187:114322. doi: 10.1016/j.bcp.2020.114322
14. Vassort G. Adenosine 5’-triphosphate: a P2-purinergic agonist in the myocardium. Physiol Rev (2001) 81:767–806. doi: 10.1152/physrev.2001.81.2.767
15. North RA. Molecular physiology of P2X receptors. Physiol Rev (2002) 82:1013–67. doi: 10.1152/physrev.00015.2002
16. Burnstock G, Knight GE. Cellular distribution and functions of P2 receptor subtypes in different systems. Int Rev Cytol (2004) 240:31–304. doi: 10.1016/S0074-7696(04)40002-3
17. Stojilkovic SS. Purinergic regulation of hypothalamopituitary functions. Trends Endocrinol Metab (2009) 20:460–8. doi: 10.1016/j.tem.2009.05.005
18. Khakh BS, North RA. Neuromodulation by extracellular ATP and P2X receptors in the CNS. Neuron (2012) 76:51–69. doi: 10.1016/j.neuron.2012.09.024
19. Garcia-Jacobo RE, Bergamin LS, Vultaggio-Poma V, Thorstenberg ML, Tarantini M, García-Hernández MH, et al. The purinergic landscape of type 2 diabetes mellitus. Molecules (2022) 27:1838. doi: 10.3390/molecules27061838
20. Illes P, Müller CE, Jacobson KA, Grutter T, Nicke A, Fountain SJ, et al. Update of P2X receptor properties and their pharmacology: IUPHAR review 30. Br J Pharmacol (2021) 178:489–514. doi: 10.1111/bph.15299
22. von Kügelgen I, Wetter A. Molecular pharmacology of P2Y-receptors. Naunyn Schmiedebergs Arch Pharmacol (2000) 362:310–23. doi: 10.1007/s002100000310
23. Abbracchio MP, Burnstock G, Boeynaems J-M, Barnard EA, Boyer JL, Kennedy C, et al. International union of pharmacology LVIII: update on the P2Y G protein-coupled nucleotide receptors: from molecular mechanisms and pathophysiology to therapy. Pharmacol Rev (2006) 58:281–341. doi: 10.1124/pr.58.3.3
24. von Kügelgen I. Molecular pharmacology of P2Y receptor subtypes. Biochem Pharmacol (2021) 187:114361. doi: 10.1016/j.bcp.2020.114361
25. von Kügelgen I. Pharmacological profiles of cloned mammalian P2Y-receptor subtypes. Pharmacol Ther (2006) 110:415–32. doi: 10.1016/j.pharmthera.2005.08.014
26. Jacobson KA, Delicado EG, Gachet C, Kennedy C, von Kügelgen I, Li B, et al. Update of P2Y receptor pharmacology: IUPHAR review 27. Br J Pharmacol (2020) 177:2413–33. doi: 10.1111/bph.15005
27. Burnstock G. Purinergic signalling. Br J Pharmacol (2006) 147(Suppl 1):S172–181. doi: 10.1038/sj.bjp.0706429
28. Burnstock G, Verkhratsky A. Long-term (trophic) purinergic signalling: Purinoceptors control cell proliferation, differentiation and death. Cell Death Dis (2010) 1:e9. doi: 10.1038/cddis.2009.11
29. Novak I. Purinergic receptors in the endocrine and exocrine pancreas. Purinergic Signal (2008) 4:237–53. doi: 10.1007/s11302-007-9087-6
30. Ko T, An HJ, Ji YG, Kim OJ, Lee DH. P2Y receptors regulate proliferation of human pancreatic duct epithelial cells. Pancreas (2012) 41:797–803. doi: 10.1097/MPA.0b013e31823ba3b3
31. Parandeh F, Abaraviciene SM, Amisten S, Erlinge D, Salehi A. Uridine diphosphate (UDP) stimulates insulin secretion by activation of P2Y6 receptors. Biochem Biophys Res Commun (2008) 370:499–503. doi: 10.1016/j.bbrc.2008.03.119
32. Tudurí E, Filiputti E, Carneiro EM, Quesada I. Inhibition of Ca2+ signaling and glucagon secretion in mouse pancreatic alpha-cells by extracellular ATP and purinergic receptors. Am J Physiol Endocrinol Metab (2008) 294:E952–960. doi: 10.1152/ajpendo.00641.2007
33. Rodrigue-Candela JL, Martin-Hernandez D, Castilla-Cortazar T. Stimulation of insulin secretion in vitro by adenosine triphosphate. Nature (1963) 197:1304. doi: 10.1038/1971304a0
34. Levine RA, Oyama S, Kagan A, Glick SM. Stimulation of insulin and growth hormone secretion by adenine nucleotides in primates. J Lab Clin Med (1970) 75:30–6.
35. Loubatières-Mariani MM, Loubatières AL, Chapal J, Valette G. [Adenosine triphosphate (ATP) and glucose. action on insulin and glucagon secretion]. C R Seances Soc Biol Fil (1976) 170:833–6.
36. Loubatieres-Mariani M-M, Chapal J, Lignon F, Valette G. Structural specificity of nucleotides for insulin secretory action from the isolated perfused rat pancreas. Eur J Pharmacol (1979) 59:277–86. doi: 10.1016/0014-2999(79)90291-7
37. Chapal J, Loubatieres-Mariani MM. Effects of phosphate-modified adenine nucleotide analogues on insulin secretion from perfused rat pancreas. Br J Pharmacol (1981) 73:105–10. doi: 10.1111/j.1476-5381.1981.tb16778.x
38. Feldman JM, Jackson TB. Specificity of nucleotide-induced insulin secretion. Endocrinology (1974) 94:388–94. doi: 10.1210/endo-94-2-388
39. Petit P, Bertrand G, Schmeer W, Henquin JC. Effects of extracellular adenine nucleotides on the electrical, ionic and secretory events in mouse pancreatic beta-cells. Br J Pharmacol (1989) 98:875–82. doi: 10.1111/j.1476-5381.1989.tb14616.x
40. Chapal J, Hillaire-Buys D, Bertrand G, Pujalte D, Petit P, Loubatières-Mariani MM. Comparative effects of adenosine-5’-triphosphate and related analogues on insulin secretion from the rat pancreas. Fundam Clin Pharmacol (1997) 11:537–45. doi: 10.1111/j.1472-8206.1997.tb00858.x
41. Petit P, Hillaire-Buys D, Manteghetti M, Debrus S, Chapal J, Loubatières-Mariani MM. Evidence for two different types of P2 receptors stimulating insulin secretion from pancreatic b cell. Br J Pharmacol (1998) 125:1368–74. doi: 10.1038/sj.bjp.0702214
42. Petit P, Lajoix A-D, Gross R. P2 purinergic signalling in the pancreatic beta-cell: control of insulin secretion and pharmacology. Eur J Pharm sciences: Off J Eur Fed Pharm Sci (2009) 37:67–75. doi: 10.1016/j.ejps.2009.01.007
43. Hazama A, Hayashi S, Okada Y. Cell surface measurements of ATP release from single pancreatic beta cells using a novel biosensor technique. Pflugers Arch (1998) 437:31–5. doi: 10.1007/s004240050742
44. Karanauskaite J, Hoppa MB, Braun M, Galvanovskis J, Rorsman P. Quantal ATP release in rat beta-cells by exocytosis of insulin-containing LDCVs. Pflugers Arch (2009) 458:389–401. doi: 10.1007/s00424-008-0610-6
45. Galvanovskis J, Braun M, Rorsman P. Exocytosis from pancreatic β-cells: mathematical modelling of the exit of low-molecular-weight granule content. Interface Focus (2011) 1:143–52. doi: 10.1098/rsfs.2010.0006
46. Leitner JW, Sussman KE, Vatter AE, Schneider FH. Adenine nucleotides in the secretory granule fraction of rat islets. Endocrinology (1975) 96:662–77. doi: 10.1210/endo-96-3-662
47. Bertrand G, Gross R, Chapal J, Loubatières-Mariani MM. Difference in the potentiating effect of adenosine triphosphate and alpha, beta-methylene ATP on the biphasic insulin response to glucose. Br J Pharmacol (1989) 98:998–1004. doi: 10.1111/j.1476-5381.1989.tb14631.x
48. Obermüller S, Lindqvist A, Karanauskaite J, Galvanovskis J, Rorsman P, Barg S. Selective nucleotide-release from dense-core granules in insulin-secreting cells. J Cell Sci (2005) 118:4271–82. doi: 10.1242/jcs.02549
49. Bertrand G, Chapal J, Loubatières-Mariani MM, Roye M. Evidence for two different P2-purinoceptors on beta cell and pancreatic vascular bed. Br J Pharmacol (1987) 91:783–7. doi: 10.1111/j.1476-5381.1987.tb11276
50. Li GD, Milani D, Dunne MJ, Pralong WF, Theler JM, Petersen OH, et al. Extracellular ATP causes Ca2(+)-dependent and -independent insulin secretion in RINm5F cells. phospholipase c mediates Ca2+ mobilization but not Ca2+ influx and membrane depolarization. J Biol Chem (1991) 266:3449–57.
51. Fernandez-Alvarez J, Hillaire-Buys D, Loubatières-Mariani MM, Gomis R, Petit P. P2 receptor agonists stimulate insulin release from human pancreatic islets. Pancreas (2001) 22:69–71. doi: 10.1097/00006676-200101000-00012
52. Tahani HM. The purinergic nerve hypothesis and insulin secretion. Z Ernahrungswiss (1979) 18:128–38. doi: 10.1007/BF02023727
53. Bertrand G, Chapal J, Loubatieres-Mariani MM. Potentiating synergism between adenosine diphosphate or triphosphate and acetylcholine on insulin secretion. Am J Physiol (1986) 251:E416–421. doi: 10.1152/ajpendo.1986.251.4.E416
54. Squires PE, James RF, London NJ, Dunne MJ. ATP-induced intracellular Ca2+ signals in isolated human insulin-secreting cells. Pflugers Arch (1994) 427:181–3. doi: 10.1007/BF00585959
55. Jacques-Silva MC, Correa-Medina M, Cabrera O, Rodriguez-Diaz R, Makeeva N, Fachado A, et al. ATP-gated P2X3 receptors constitute a positive autocrine signal for insulin release in the human pancreatic beta cell. Proc Natl Acad Sci USA (2010) 107:6465–70. doi: 10.1073/pnas.0908935107
56. Hauge-Evans AC, Squires PE, Belin VD, Roderigo-Milne H, Ramracheya RD, Persaud SJ, et al. Role of adenine nucleotides in insulin secretion from MIN6 pseudoislets. Mol Cell Endocrinol (2002) 191:167–76. doi: 10.1016/s0303-7207(02)00051-5
57. Salehi A, Qader SS, Quader SS, Grapengiesser E, Hellman B. Inhibition of purinoceptors amplifies glucose-stimulated insulin release with removal of its pulsatility. Diabetes (2005) 54:2126–31. doi: 10.2337/diabetes.54.7.2126
58. Richards-Williams C, Contreras JL, Berecek KH, Schwiebert EM. Extracellular ATP and zinc are co-secreted with insulin and activate multiple P2X purinergic receptor channels expressed by islet beta-cells to potentiate insulin secretion. Purinergic signalling (2008) 4:393–405. doi: 10.1007/s11302-008-9126-y
59. Santini E, Cuccato S, Madec S, Chimenti D, Ferrannini E, Solini A. Extracellular adenosine 5’-triphosphate modulates insulin secretion via functionally active purinergic receptors of X and y subtype. Endocrinology (2009) 150:2596–602. doi: 10.1210/en.2008-1486
60. Coutinho-Silva R, Parsons M, Robson T, Burnstock G. Changes in expression of P2 receptors in rat and mouse pancreas during development and ageing. Cell Tissue Res (2001) 306:373–83. doi: 10.1007/s004410100458
61. Coutinho-Silva R, Parsons M, Robson T, Lincoln J, Burnstock G. P2X and P2Y purinoceptor expression in pancreas from streptozotocin-diabetic rats. Mol Cell Endocrinol (2003) 204:141–54. doi: 10.1016/s0303-7207(03)00003-0
62. Silva AM, Rodrigues RJ, Tomé AR, Cunha RA, Misler S, Rosário LM, et al. Electrophysiological and immunocytochemical evidence for P2X purinergic receptors in pancreatic beta cells. Pancreas (2008) 36:279–83. doi: 10.1097/MPA.0b013e31815a8473
63. Glas R, Sauter NS, Schulthess FT, Shu L, Oberholzer J, Maedler K. Purinergic P2X7 receptors regulate secretion of interleukin-1 receptor antagonist and beta cell function and survival. Diabetologia (2009) 52:1579–88. doi: 10.1007/s00125-009-1349-0
64. Pandolfi J, Ferraro A, Lerner M, Serrano JR, Dueck A, Fainboim L, et al. Purinergic signaling modulates human visceral adipose inflammatory responses: implications in metabolically unhealthy obesity. J Leukoc Biol (2015) 97:941–9. doi: 10.1189/jlb.3A1214-626R
65. Lee DH, Park K-S, Kim D-R, Lee J-W, Kong ID. Dual effect of ATP on glucose-induced insulin secretion in HIT-T15 cells. Pancreas (2008) 37:302–8. doi: 10.1097/MPA.0b013e318168daaa
66. Vieira FS, Nanini HF, Takiya CM, Coutinho-Silva R. P2X7 receptor knockout prevents streptozotocin-induced type 1 diabetes in mice. Mol Cell Endocrinol (2016) 419:148–57. doi: 10.1016/j.mce.2015.10.008
67. Vergani A, Fotino C, D’Addio F, Tezza S, Podetta M, Gatti F, et al. Effect of the purinergic inhibitor oxidized ATP in a model of islet allograft rejection. Diabetes (2013) 62:1665–75. doi: 10.2337/db12-0242
68. Bertrand G, Chapal J, Puech R, Loubatières-Mariani MM. Adenosine-5’-O-(2-thiodiphosphate) is a potent agonist at P2 purinoceptors mediating insulin secretion from perfused rat pancreas. Br J Pharmacol (1991) 102:627–30. doi: 10.1111/j.1476-5381.1991.tb12223.x
69. Fischer B, Chulkin A, Boyer JL, Harden KT, Gendron FP, Beaudoin AR, et al. 2-thioether 5’-O-(1-thiotriphosphate)adenosine derivatives as new insulin secretagogues acting through P2Y-receptors. J Med Chem (1999) 42:3636–46. doi: 10.1021/jm990158y
70. Farret A, Filhol R, Linck N, Manteghetti M, Vignon J, Gross R, et al. P2Y receptor mediated modulation of insulin release by a novel generation of 2-substituted-5’-O-(1-boranotriphosphate)-adenosine analogues. Pharm Res (2006) 23:2665–71. doi: 10.1007/s11095-006-9112-4
71. Léon C, Freund M, Latchoumanin O, Farret A, Petit P, Cazenave J-P, et al. The P2Y(1) receptor is involved in the maintenance of glucose homeostasis and in insulin secretion in mice. Purinergic Signal (2005) 1:145–51. doi: 10.1007/s11302-005-6209-x
72. Ohtani M, Suzuki J-I, Jacobson KA, Oka T. Evidence for the possible involvement of the P2Y(6) receptor in Ca (2+) mobilization and insulin secretion in mouse pancreatic islets. Purinergic Signal (2008) 4:365–75. doi: 10.1007/s11302-008-9122-2
73. Ohtani M, Ohura K, Oka T. Involvement of P2X receptors in the regulation of insulin secretion, proliferation and survival in mouse pancreatic β-cells. Cell Physiol biochemistry: Int J Exp Cell physiology biochemistry Pharmacol (2011) 28:355–66. doi: 10.1159/000331752
74. Lugo-Garcia L, Filhol R, Lajoix A-D, Gross R, Petit P, Vignon J. Expression of purinergic P2Y receptor subtypes by INS-1 insulinoma beta-cells: A molecular and binding characterization. Eur J Pharmacol (2007) 568:54–60. doi: 10.1016/j.ejphar.2007.04.012
75. Mesto N, Bailbe D, Eskandar M, Pommier G, Gil S, Tolu S, et al. Involvement of P2Y signaling in the restoration of glucose-induced insulin exocytosis in pancreatic β cells exposed to glucotoxicity. J Cell Physiol (2022) 237:881–96. doi: 10.1002/jcp.30564
76. Stam NJ, Klomp J, Van de Heuvel N, Olijve W. Molecular cloning and characterization of a novel orphan receptor (P2P) expressed in human pancreas that shows high structural homology to the P2U purinoceptor. FEBS Lett (1996) 384:260–4. doi: 10.1016/0014-5793(96)00321-3
77. Burnstock G. Purinergic signalling: pathophysiology and therapeutic potential. Keio J Med (2013) 62:63–73. doi: 10.2302/kjm.2013-0003-re
78. Burnstock G. Short- and long-term (trophic) purinergic signalling. Philos Trans R Soc Lond B Biol Sci (2016) 371:20150422. doi: 10.1098/rstb.2015.0422
79. Burnstock G. Introduction to purinergic signalling in the brain. Adv Exp Med Biol (2013) 986:1–12. doi: 10.1007/978-94-007-4719-7_1
80. Lecca D, Ceruti S, Fumagalli M, Abbracchio MP. Purinergic trophic signalling in glial cells: functional effects and modulation of cell proliferation, differentiation, and death. Purinergic Signal (2012) 8:539–57. doi: 10.1007/s11302-012-9310-y
81. Besnard A, Gautherot J, Julien B, Tebbi A, Garcin I, Doignon I, et al. The P2X4 purinergic receptor impacts liver regeneration after partial hepatectomy in mice through the regulation of biliary homeostasis. Hepatology (2016) 64:941–53. doi: 10.1002/hep.28675
82. Tan C, Salehi A, Svensson S, Olde B, Erlinge D. ADP receptor P2Y(13) induce apoptosis in pancreatic beta-cells. Cell Mol Life Sci (2010) 67:445–53. doi: 10.1007/s00018-009-0191-3
83. Lee DH, Kim EG, Park K-S, Jeong S-W, Kong ID, Lee J-W. Characteristics of P2X7-like receptor activated by adenosine triphosphate in HIT-T15 cells. Pancreas (2007) 35:53–62. doi: 10.1097/01.mpa.0000278676.58491.ef
84. Akash MSH, Rehman K, Chen S. Role of inflammatory mechanisms in pathogenesis of type 2 diabetes mellitus. J Cell Biochem (2013) 114:525–31. doi: 10.1002/jcb.24402
Keywords: P2 purinergic receptors, P2X signaling, P2Y signaling, pancreatic ß-cell function, pancreatic ß-cell plasticity, diabetes
Citation: Mesto N, Movassat J and Tourrel-Cuzin C (2022) P2-type purinergic signaling in the regulation of pancreatic β-cell functional plasticity as a promising novel therapeutic approach for the treatment of type 2 diabetes? Front. Endocrinol. 13:1099152. doi: 10.3389/fendo.2022.1099152
Received: 15 November 2022; Accepted: 29 November 2022;
Published: 09 December 2022.
Edited by:
Teresa Pereira, Uppsala University, SwedenReviewed by:
Mikio Hayashi, Kansai Medical University, JapanCopyright © 2022 Mesto, Movassat and Tourrel-Cuzin. This is an open-access article distributed under the terms of the Creative Commons Attribution License (CC BY). The use, distribution or reproduction in other forums is permitted, provided the original author(s) and the copyright owner(s) are credited and that the original publication in this journal is cited, in accordance with accepted academic practice. No use, distribution or reproduction is permitted which does not comply with these terms.
*Correspondence: Cécile Tourrel-Cuzin, cecile.tourrel-cuzin@u-paris.fr