- 1Department of Gynecological Endocrinology, Beijing Obstetrics and Gynecology Hospital, Capital Medical University, Beijing Maternal and Child Health Care Hospital, Beijing, China
- 2Department for Women’s Health, University Women’s Hospital and Research Center for Women’s Health, University of Tuebingen, Tuebingen, Germany
Objective: This study aims to evaluate the effect of Kuntai capsule on ovarian function in cisplatin-induced premature ovarian insufficiency rats and to explore the mechanism of Kuntai capsule on the ovarian function of rats.
Methods: Seventy-four female Sprague-Dawley rats were used for this study. Eight of the rats were randomly assigned to the Control group. The remaining sixty-six rats were utilized to establish the POI model via Cisplatin and then randomly divided into four groups: the model Control group, the Estradiol group, and groups treated with low and high doses of Kuntai capsule. For the 28-day administration, the Control and model Control groups were intragastrically administered with 2.0 mL of 0.9% sodium chloride daily, the Estradiol group with 2.0 mL of Estradiol suspension (0.2mg/kg/d), and the low dose Kuntai capsule group and the high dose Kuntai capsule group with 2.0 mL of Kuntai capsule suspension (0.6g/kg/d, 1.8g/kg/d, respectively). Sex hormone levels, estrous cycle, and ovarian coefficient of the five groups were compared, histological sections analyzed follicle counts, and the protein expressions of growth differentiation factor 9, light chain 3 A-II, and Beclin 1 in the ovarian tissue were detected by Western blotting.
Results: After the 28-day administration, the serum Estradiol and Follicle-Stimulating Hormone levels of the group treated with low dose of Kuntai capsule were not significantly different from the Control group, the serum anti-Müllerian Hormone level of the group treated with high dose of Kuntai capsule was significantly higher than the Estradiol group. The estrous cycle of the group treated with low dose of Kuntai capsule was significantly lower than the model Control group. Regarding ovarian coefficient, resting and growing follicles, growth differentiation factor 9, light chain 3 A-II, and Beclin 1 expression, both Kuntai capsule groups outperformed the model Control group with the statistical difference (P<0.05).
Conclusion: Kuntai capsule can improve the estrous cycle and ovarian coefficient of rats with premature ovarian insufficiency, maintain the number of resting and growing follicles, and up-regulate the protein expression of growth differentiation factor 9, light chain 3 A-II, and Beclin 1 of rats’ ovaries.
1 Introduction
Premature ovarian insufficiency (POI) is defined as a cessation of ovarian function before the age of 40 years. European Society of Human Reproduction and Embryology (ESHRE) recommends the following diagnostic criteria: amenorrhea or oligomenorrhoea for at least four months and elevated follicle-stimulating hormone (FSH) levels (> 25 IU/L) on two occasions (> 4 weeks apart) (1).
Over the past 20 years, the development of spontaneous POI has been relatively stable, but induced POI is on the rise. Worldwide, over 6.6 million women are diagnosed with cancer each year, and about 10% of them are diagnosed during reproductive age (age < 40); they usually receive aggressive chemotherapy and radiotherapy, which may cause POI and subsequent fertility loss in more than 80% of cases (2–6).
According to the National Cancer Institute, up to 10% to 20% of all cancer patients have been prescribed with Cisplatin and other platinum-based drugs. Cisplatin is a cell cycle non-specific drug that destroys the primordial follicle, induces follicle apoptosis, and reduces the follicle’s pool. Treatment with Cisplatin exceeding 400mg could lead to irreversible ovarian damage (7, 8).
Can Kuntai capsule (a traditional Chinese medicine) preserve or improve patients’ ovarian function after they are treated with Cisplatin? Kuntai capsule is derived from Huanglian Ejiao Decoction, a classic prescription from Treatise on Febrile, written by Chinese sage Zhang Zhongjing in the early 3rd century. It consists of six herbs for treating ovarian insufficiency: Rehmannia glutinosa, Coptis, Paeonia, Scutellaria, Equus asinus, and Poria cocos.
In this study, Kuntai capsule was used to treat Cisplatin-induced POI rats, and its effect and possible mechanisms on these rats’ ovarian function were preliminarily explored.
2 Materials and methods
2.1 Experimental design
After ten days of adaptive feeding, seventy-four specific-pathogen-free grade healthy female Sprague-Dawley rats, aged 8-week-old and weighing 180-200g (Animal License: SCXK (Beijing) 2011-0012), were involved in this study. These rats were housed in groups of 3 per cage and maintained under standard laboratory conditions (20-24°C; relative humidity: 50%-70%, light cycles: 10h light, 14h dark; bedding material kept dry).
These rats were then randomly divided into the Control group (n=8) and the POI model group (n=66), the latter intraperitoneally administered with 2ml of Cisplatin Injection (Jiangsu Hansoh Pharmaceutical Co. Ltd., lot number: H20040812, strength: 6ml:30mg) at a dose of 6mg/kg for once a week for two weeks to establish the model (9–13). The POI model group was then randomly divided into four groups: the model Control group (mo Control), the group treated with E2 (E2), and the groups treated with low and high doses of Kuntai capsule (KTC-L, KTC-H).
For the 28-day administration, the Control and mo Control groups were intragastrically administered with 2.0 mL of 0.9% sodium chloride daily; the E2 group with 2.0 mL of Estradiol suspension at a dose of 0.2mg/kg/d (Abbott Biologicals BV lot number:356933, Strength: 2mg/tablet); and the KTC-L and KTC-H groups with 2.0 mL of Kuntai capsule suspension (Guiyang Xintian Pharmaceutical Co. Ltd. lot number: 180405, Strength: 0.5g; 0.6g/kg/d, 1.8g/kg/d, respectively). Animal drug dose conversion was calculated based on the coefficient of equivalent dose transferring from rats to humans using body surface area (14) (Figure 1 and Table 1).
2.2 Indicators and material
2.2.1 Estrous cycle
During administration, the vaginal smears of five rats in each group were evaluated between 09:00 and 10:00 using Wright’s-Giemsa stain to assess the estrous cycles.
2.2.2 Blood collection and evaluation of AMH, FSH, and E2
After the POI model was established, five rats were randomly selected from the Control and POI model groups. Blood samples of 1.0 mL/rat were collected from the retro-orbital sinus. Twenty-four hours after the last drug administration, blood samples of 1.0 mL of all rats were collected from the abdominal aorta. Serum AMH, FSH, and E2 levels of the blood samples were measured by enzyme-linked immunosorbent assay (ELISA).
2.2.3 Ovarian sample collection
After the final drug administration, rats were sacrificed by overdose of sodium pentobarbital (100mg/kg). Ovaries were removed immediately for further analysis, and ovarian fat pads were gently withdrawn. Bilateral ovaries were weighted with analytical balance, and ovarian coefficients were recorded.
The left ovarian tissue was fixed in 4% paraformaldehyde, dehydrated with gradient alcohol, embedded with paraffin, and sectioned for hematoxylin and eosin (HE) staining to evaluate the number of resting follicles (primordial follicles + primary follicles) and growing follicles (secondary follicles + antral follicles). The right ovarian tissue was frozen in liquid nitrogen and stored at a -80°C freezer for protein analysis of Growth differentiation factor-9 (GDF9), light chain 3 (LC3) A-II, and Beclin 1, which are relative to follicle development or autophagy.
2.3 Statistical analysis
The Shapiro-Wilk test was used to check if the continuous variables follow a normal distribution, and Levene’s test was used to assess the equality of variances. Variables normally distributed were shown as ‘mean ± SD’. One-way analysis of variance (ANOVA)-LSD was used for comparing group means. P < 0.05 was considered statistically significant.
3 Results
3.1 Estrous cycle
The typical pictures of smears of vaginal cells at different stages of the estrous cycle in rats are shown in Figure 2A. The estrous cycles of the Control (5.18 ± 1.54) and KTC-L groups (5.46 ± 1.48) were significantly lower than the mo Control (8.35 ± 0.60) and KTC-H groups (7.2 ± 3.83) (P=0.000, P=0.006; P=0.000, P=0.02; respectively). No statistical significance was observed when comparing the KTC-L group with the Control and E2 groups (P=0.676; 5.30 ± 1.83, P=0.809, respectively) (Figure 2B).
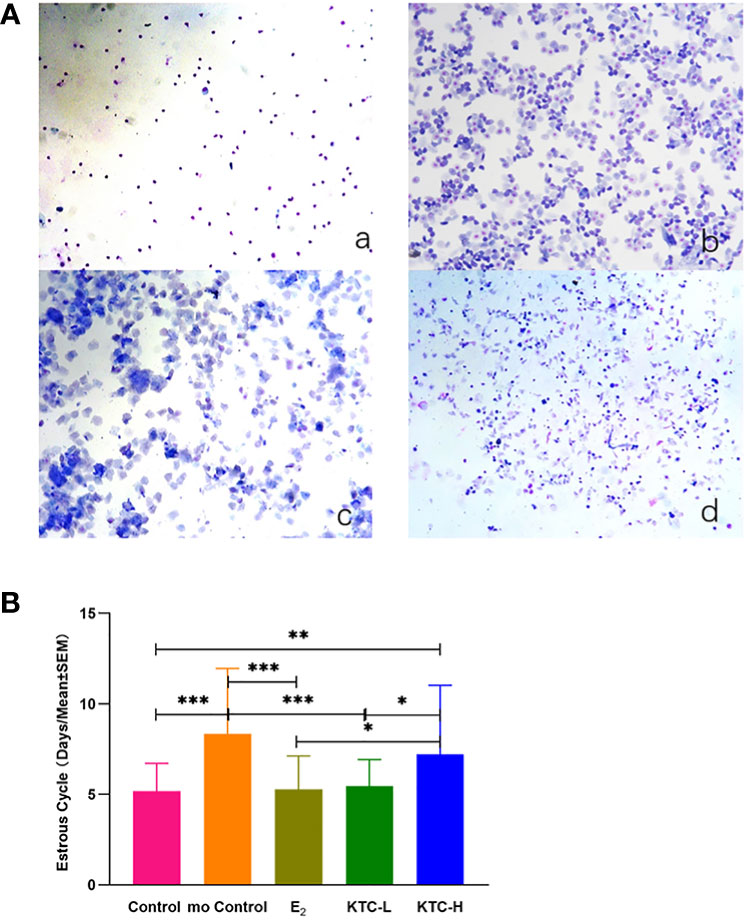
Figure 2 (A) The typical pictures of smears of vaginal cells at different stages of the estrous cycle in rats (×100). a Diestrus; b Proestrus; c Estrus; d Metestrus. (B) The estrous cycle in five groups. * P<0.05; ** P<0.01; *** P<0.001.
3.2 Serum E2, FSH, and AMH levels after establishing the POI model and final administration
As shown in Figure 3A, the AMH and E2 levels of the POI model group were significantly lower than the Control group (4.15 ± 0.92 vs. 3.34 ± 0.42, P=0.044; 378.12 ± 142.77 vs. 129.19 ± 33.31, P=0.005), while the FSH levels of the POI model group were significantly higher (17.64 ± 3.74 vs. 10.43 ± 2.09, P=0.019), thus indicating the successful establishment of cisplatin-induced POI rat model.
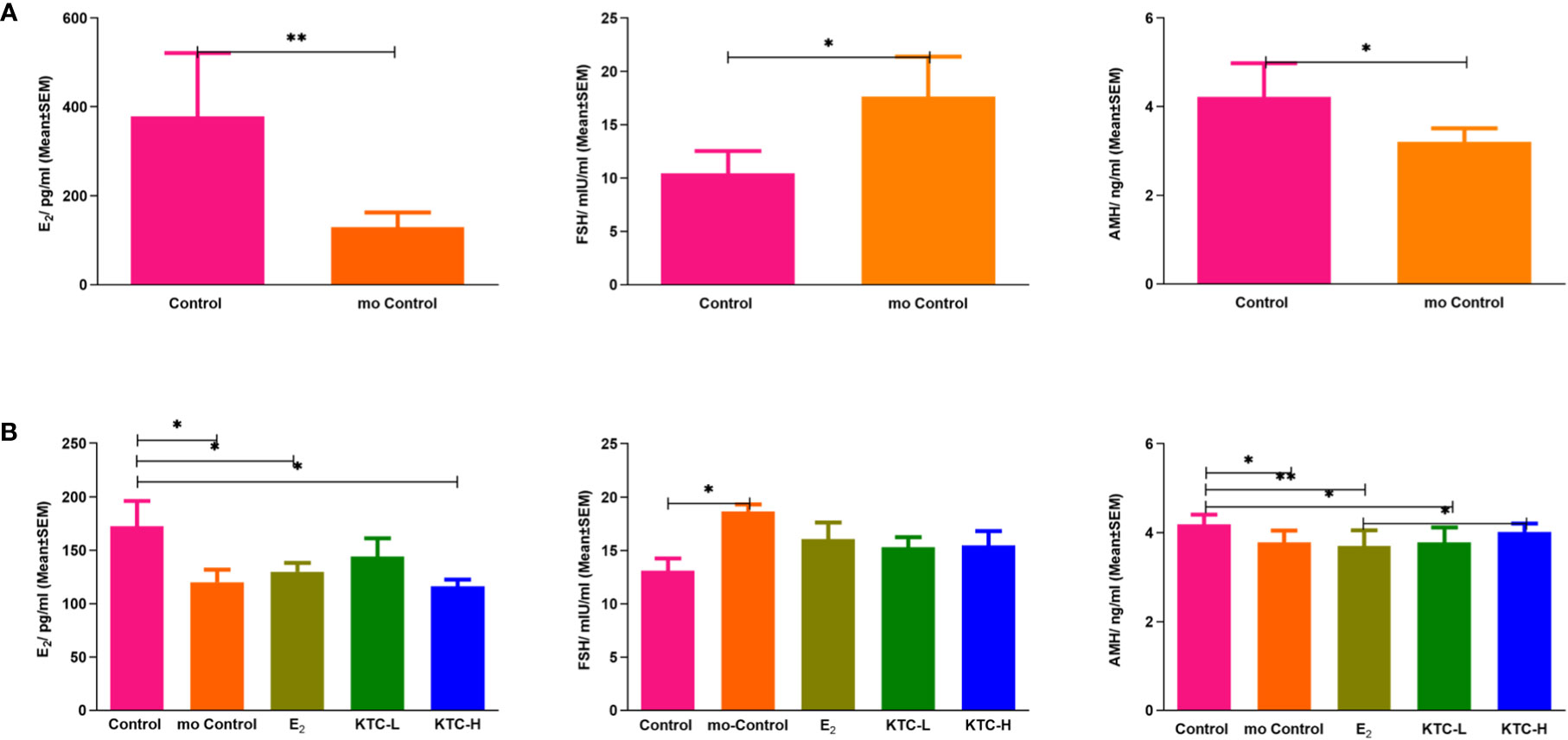
Figure 3 (A) Serum E2, FSH, and AMH levels after establishing the POI model. * P<0.05; ** P<0.01 (B) Serum E2, FSH, and AMH levels in the five groups after administration for 28 days. * P<0.05; ** P<0.01.
As shown in Figure 3B, the E2 level of the Control group (172.69 ± 23.39) was significantly higher than the mo Control, E2, and KTC-H groups (120.12 ± 25.92, P=0.028; 129.76 ± 25.37, P=0.035; 116.36 ± 19.90, P=0.011, respectively). However, the E2 level of the KTC-L group (136.77 ± 38.78) was not significantly different from the Control group (P=0.082). The FSH level of the Control group (13.10 ± 2.32) was significantly lower than the mo Control group (18.65 ± 1.31, P=0.038) but not significantly different from the E2, KTC-L, and KTC-H groups (16.05 ± 4.72, P=0.185; 15.30 ± 2.12, P=0.370; 15.46 ± 3.87, P=0.295; respectively). The AMH level of the Control group (4.18 ± 0.22) was significantly higher than the mo Control, E2, and KTC-L groups (3.78 ± 0.27, 3.70 ± 0.36, 3.78 ± 0.34) (P=0.006, P=0.001, P=0.009), but not significantly different from the KTC-H group (4.02 ± 0.19, P=0.228). The AMH level of the KTC-H group was significantly higher than the E2 group (P=0.020).
3.3 Ovarian coefficient
As shown in Figure 4, the ovarian coefficient of the mo Control group (0.035 ± 0.004) was significantly lower than the Control (0.045 ± 0.007), KTC-L (0.045 ± 0.004), and KTC-H groups (0.044 ± 0.008) (P=0.003, P=0.003, P=0.009, respectively), with no statistical differences observed among the latter three groups. The E2 group (0.040 ± 0.004) was not significantly different from the KTC-L and KTC-H groups (P=0.087, P=0.196, respectively).
3.4 Resting and growing follicles
As shown in Figure 5A, regarding the resting follicles numbers, the Control group (15 ± 8) were significantly higher than the mo Control, E2, KTC-L, and KTC-H groups (3 ± 3, P=0.000; 8 ± 4, P=0.004; 6 ± 4, P=0.001; 7 ± 5, P=0.000; respectively). The E2, and KTC-H groups were significantly higher than the mo Control group (P=0.012, P=0.032, respectively).
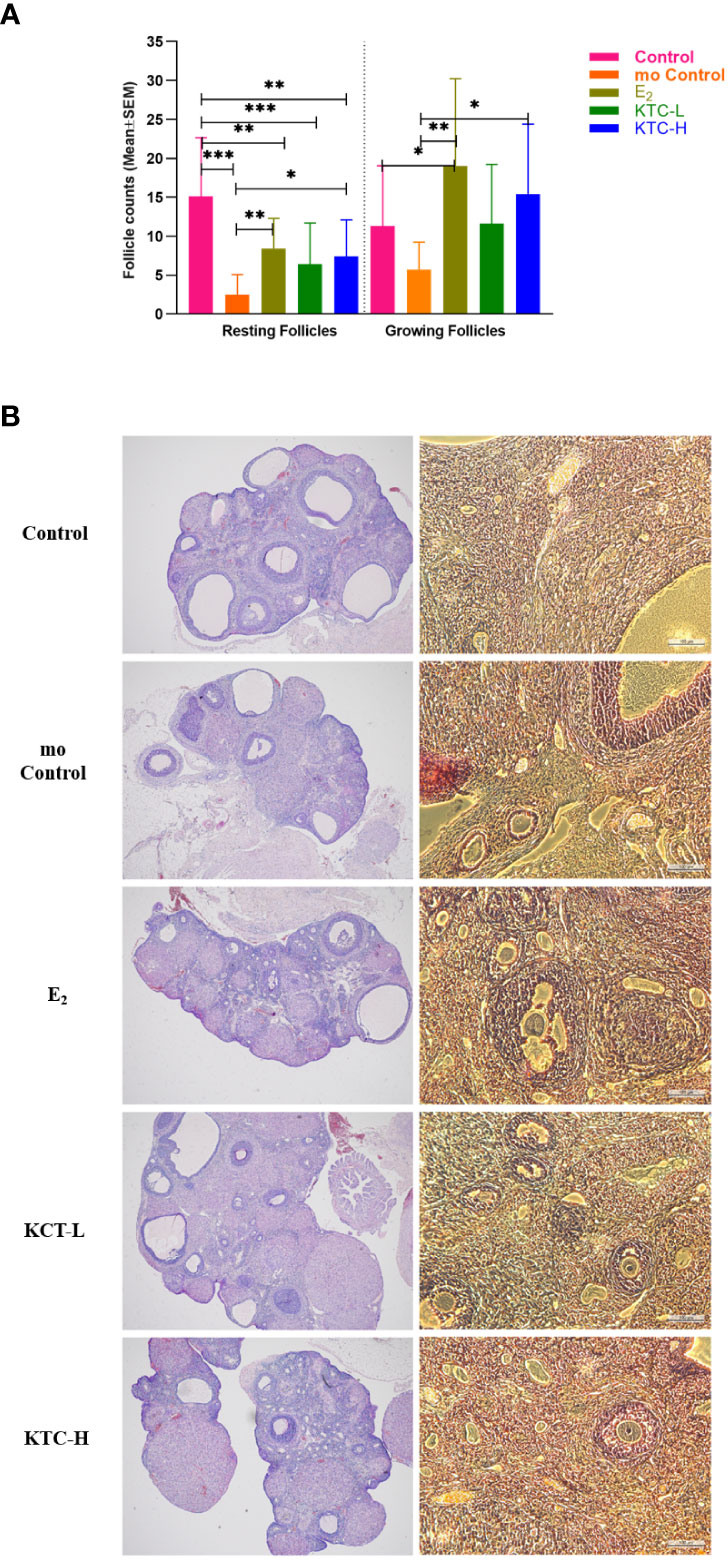
Figure 5 (A) The number of Resting and Growing follicles among the five groups is compared. (B) Typical HE pictures of the five groups. * P<0.05, ** P<0.01, *** P<0.001.
Regarding the numbers of the growing follicles, the mo Control group (6 ± 4) was significantly lower than the E2, and KTC-H groups (19 ± 12, P=0.002; 15 ± 9, P=0.017; respectively). Figure 5B is the typical HE pictures of follicles of the five groups.
3.5 Protein expression of GDF9, LC3A-II, and Beclin 1
As shown in Figure 6, regarding the relative protein expression of GDF9, both the KTC-L (0.985 ± 0.188) and KTC-H groups (0.705 ± 0.038) were significantly higher than the Control (0.530 ± 0.061) and mo Control groups (0.551 ± 0.035) (P=0.000, P=0.000; P=0.013, P=0.027, respectively).
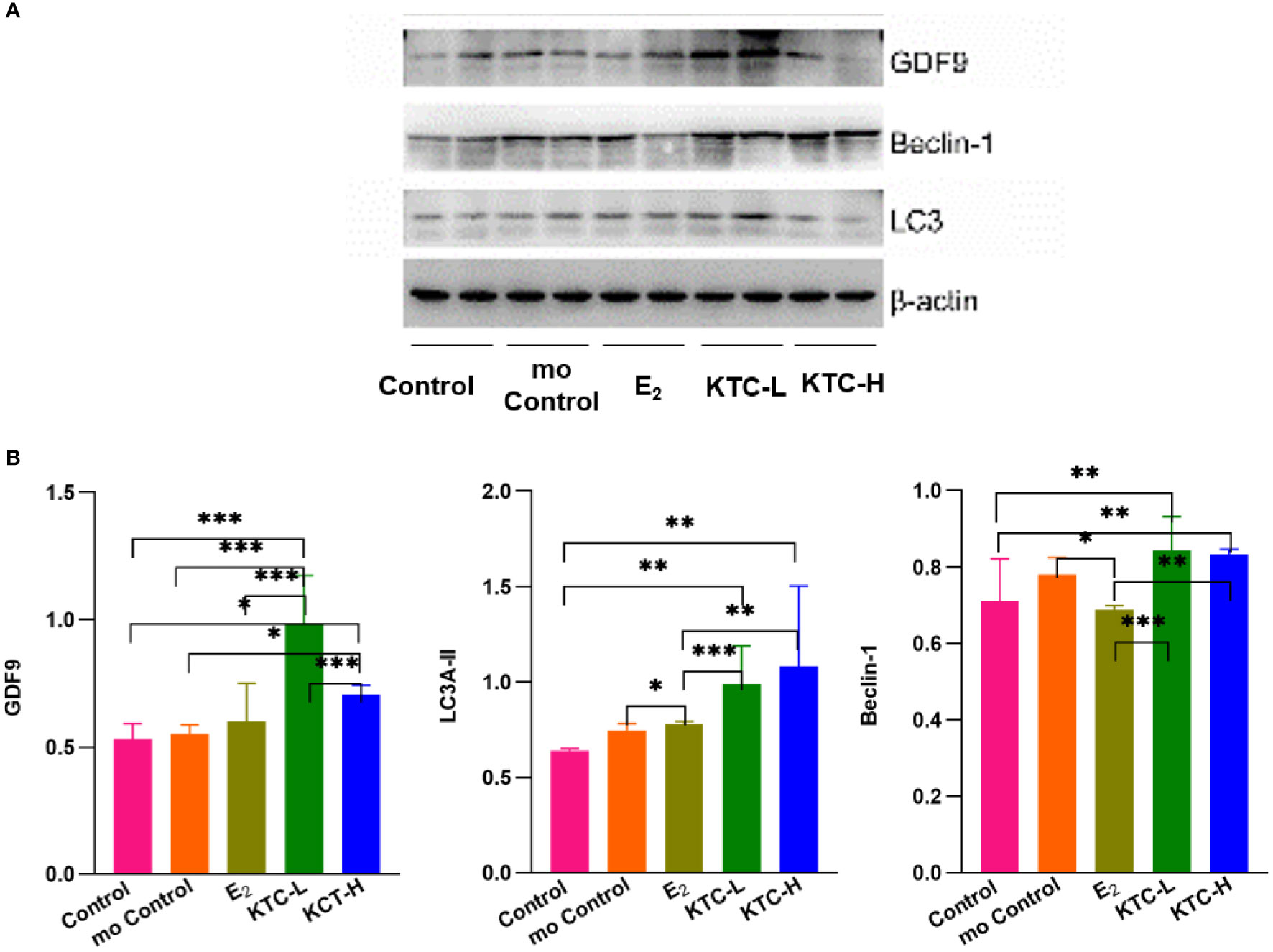
Figure 6 (A) Western blotting of GDF9, LC3A-II, Beclin-1 protein analysis. (B) The relative protein expression of GDF9, LC3A-II, Beclin-1 in the five groups. * P<0.05, ** P<0.01, *** P<0.001.
About the relative protein expression of LC3A-II, the KTC-H group (1.080 ± 0.424) was significantly higher than the Control, mo Control, and E2 groups (0.641 ± 0.010, P=0.001; 0.746 ± 0.035, P=0.011; 0.780 ± 0.013, P=0.021; respectively). The KTC-L group (0.988 ± 0.201) was significantly higher than the Control group (P=0.009).
About the relative protein expression of Beclin 1, both the KTC-H (0.832 ± 0.014) and KTC-L groups (0.843 ± 0.088) were significantly higher than the Control (0.711 ± 0.110) and E2 groups (0.688 ± 0.011) (P=0.004, P=0.001; P=0.002, P=0.000; respectively). The mo Control group (0.781 ± 0.044) was significantly higher than the E2 group (P=0.023).
4 Discussion
Cisplatin has been one of the most widely used anti-tumor drugs in clinical practice in the past 20 years. Some scholars have confirmed that Cisplatin can induce cell apoptosis in the ovary and tissue necrosis, leading to POI (15). This study used Cisplatin to establish a reliable model of POI rats. The model was then administered with different doses of Kuntai capsule to observe its effect on rats’ ovarian function and explore ways of preserving ovarian function in young female patients receiving chemotherapy.
4.1 Follicle development
GDF9 is a member of the Transforming Growth Factor β (TGFβ) family. In addition to promoting pre-antral follicles growth, antral follicles growth, and granulosa cells proliferation, GDF9 also stimulates the granulosa cells’ basal secretion of progesterone and Estradiol of small antral follicles of female rats (16–19). In this study, GDF9 expression in both Kuntai groups was significantly higher than in the Control and mo Control groups. This indicates that Kuntai capsule has a positive effect on the development of granulosa cells in small follicles, which is consistent with the results of follicle counts.
4.2 Autophagy
Microtubule-associated protein 1A/1B-LC3 is a soluble protein widely distributed in mammalian tissues and cultured cells. The expression of LC3A-II in the mammalian ovaries is relatively higher than other isoforms, thus making it a suitable marker for monitoring autophagy (20–24).
The results of this study revealed the expression of LC3A-II protein in the following descending order: KTC-H > KTC-L > E2 > mo Control > Control. In other words, all four POI model groups had a higher LC3A-II expression than the Control group, with the KTC-H and KTC-L groups showing the most apparent autophagic responses.
The Beclin 1 gene is the mammalian ortholog of yeast autophagy-related gene-6 (ATG6) and the most widely studied autophagy-related gene. Beclin 1 acts as a platform for binding with proteins like Phosphoinositide 3-kinase (PI3KC3), ultraviolet radiation resistance-associated gene (UVRAG), activating molecule in Beclin 1-regulated autophagy (Ambra1), and Bax interacting factor-1 (Bif-1) to promote autophagy (25–29).
In this study, the expression of Beclin-1 is in the following descending order: KTC-L > KTC-H > mo Control > Control > E2. The results showed that although autophagy is common among the five groups, Beclin-1 expression in both the KTC-L and KTC-H groups was significantly higher, indicating that Kuntai Capsule induces stronger autophagy than the other administration.
Autophagy plays dual roles in cell survival and death by inhibiting or partnering with apoptosis. Autophagy helps the body eliminate cancerous cells, and damaged organelles facilitate cellular adaptation to the changing internal and external environment and promote cell survival by delaying aging and cell death (30, 31).
4.3 Traditional Chinese medicine network pharmacology and Kuntai capsule
Unlike modern medicine, which has a single active compound, Traditional Chinese Medicine integrates many plants into formulae of compatible ingredients. Each ingredient contains various compounds, with complex interactions among these compounds forming a network. This also gave rise to the development of the discipline ‘Traditional Chinese Medicine Network Pharmacology’ (32–35), which updates the research paradigm from the current ‘one target, one drug’ mode to a new ‘network target, multi-components mode’ (36).
Research on the network pharmacology of Kuntai Capsule has been carried out recently. Kuntai Capsule consists of 6 herb components: Rehmannia glutinosa, Coptis, Paeonia, Scutellaria, Equus asinus, and Poria cocos. Li DX (37) utilized the Traditional Chinese Medicine Systems Pharmacology Database and Analysis Platform (TCMSP) to find out 21 active compounds corresponding to the six herb components, which are mainly flavonoids and alkaloids, including verbascoside, 5-hydroxymethylfurfural, baicalein, wogonin, skullcapflavone II, berberine, columbamine, magnoflorine, jatrorrhizine, and palmatine, etc. Wang LJ and Chen YM (38, 39) did further research and acquired the corresponding 186 gene targets in the cells of the human body of these 21 compounds via TCM-mesh (http://mesh.tcm.microbioinformatics.org) and COSMIC (http://cancer.sanger.ac.uk/cosmic). These gene targets are highly enriched in the central nervous system, breast, endometrium, and ovary. This result indicates that the Kuntai capsule can treat related diseases caused by ovarian insufficiency. Besides, Kyoto Encyclopedia of Genes and Genomes (KEGG) results found that these targets were involved in the PI3K-Akt, mTOR, and insulin signaling pathways, which participate in follicular development in the follicle recruitment stage (38–40). Signaling pathways may become one of the directions in further research.
4.4 Mortality rate in establishing the POI rat model
Several studies used different doses of cisplatin to establish the POI rat model, the median lethal dose (LD50) of cisplatin in rats ranging from 6 to 20 mg/kg (41–43). A dose less than 6 mg/kg can reduce death, but the POI model would not be successful enough to carry out further experiments; doses higher than 6 mg/kg would ensure a higher success of modeling, but it would have adverse effects such as decreased appetite, weight loss, and myelosuppression, and especially a higher risk of death (9). In a study (10) comparing lower doses of cisplatin (1.5 mg/kg, 2.0 mg/kg, or 2.5 mg/kg once a day for five consecutive days) with higher dose of cisplatin (4 mg/kg on Day 1 and 6 mg/kg on Day 8), it was concluded that the lower dose regimes were not effective to establish the POI model by examining the body mass, follicle count, and serum AMH.Therefore, in our pre-experiment study, we did not adopt the lower-dose cisplatin method to establish the POI model and followed the higher-dose modeling method (9).
Our mortality rate, at 41%, was about in the range of other studies as far as mortality rates have been reported (41–43). Contrary to those studies that do not mention their mortality rate in the modeling process, we intended to list out the number of rats that died in the modeling process to act as a reference for researchers planning to use cisplatin-induced POI rat models and a reminder that other means need to be explored to reduce mortality rate while ensuring the modeling effectiveness, such as caudal injection of cisplatin or subcutaneous injection of saline to reduce dehydration. Our intention was to stress the need and the possibilities to reduce the mortality due to the toxic cisplatin.
5 Limitation
There are still limitations to this study. The indicators in our research are not comprehensive enough. In further research, we will add TUNEL staining to measure apoptosis, Western blot analysis of SQSTM1/P62 to ensure the role of Kuntai Capsule-induced autophagy, perform GDF9, LC3A-II, and Beclin1 immunohistochemistry and immunofluorescence stainings, and culture primary ovarian granulosa cells in vitro to further ensure the role of Kuntai Capsule in E2 secretion and activation of autophagy.
6 Conclusion
Traditional Chinese medicine Kuntai capsule can improve POI rats’ estrous cycle and ovarian coefficient, increase the numbers of resting and growing follicles, and up-regulate the protein expression of GDF9, LC3A-II, and Beclin 1 of the ovary. Further research needs to be done to have an in-depth understanding of the mechanisms involved.
Data availability statement
The data presented in the study are deposited in the Figshare repository, accession number: https://doi.org/10.6084/m9.figshare.21896502.
Ethics statement
The animal study was reviewed and approved by The Ethics Committee of Capital Medical University (ethics code: AEEI-2018-163).
Author contributions
All authors qualify for authorship by contributing substantially to this article. SL: Performed the experiment, wrote the original draft, and revised the manuscript. XR: Project leader, project supervisor, and revised the first draft. AM: Experimental supervision, interpretation of results, and article revision. All authors contributed to the article and approved the submitted version.
Funding
This study was supported by Beijing Natural Science Foundation (7202047), Capital’s Funds for Health Improvement and Research (2020-2-2112), and Beijing Municipal Administration of Hospitals’ Ascent Plan (DFL20181401), China International Talent Exchange Association Medical health technology innovation and talent training special fund (2017041900004, 2018042000001).
Conflict of interest
The authors declare that the research was conducted without any commercial or financial relationships that could be construed as a potential conflict of interest.
Publisher’s note
All claims expressed in this article are solely those of the authors and do not necessarily represent those of their affiliated organizations, or those of the publisher, the editors and the reviewers. Any product that may be evaluated in this article, or claim that may be made by its manufacturer, is not guaranteed or endorsed by the publisher.
References
1. Webber L, Davies M, Anderson R, Bartlett J, Braat D, Cartwright B, et al. ESHRE guideline: Management of women with premature ovarian insufficiency. Hum Reprod (2016) 31:926–37. doi: 10.1093/humrep/dew027
2. Torrealday S, Kodaman P, Pal L. Premature ovarian insufficiency - an update on recent advances in understanding and management. F1000Research (2017) 6:2069. doi: 10.12688/f1000research.11948.1
3. Salama M, Woodruff T. Anticancer treatments and female fertility: clinical concerns and role of oncologists in oncofertility practice. Expert Rev Anticancer Ther (2017) 17:687–92. doi: 10.1080/14737140.2017.1335199
4. Ferlay J, Soerjomataram I, Dikshit R, Sultan E, Colin M, Marise R, et al. Cancer incidence and mortality worldwide: Sources, methods and major patterns in GLOBOCAN 2012. Int J Cancer (2014) 136:E359–86. doi: 10.1002/ijc.29210
5. Ward E, DeSantis C, Robbins A, Kohler B, Jemal A. Childhood and adolescent cancer statistics, 2014. CA Cancer J Clin (2014) 64:83–103. doi: 10.3322/caac.21219
6. Stroud JS, Mutch D, Rader J, Powell M, Thaker PH, Grigsby PW. Effects of cancer treatment on ovarian function. Fertil Steril (2009) 92:417–27. doi: 10.1016/j.fertnstert.2008.07.1714
7. Eldani M, Luan Y, Xu PC, Bargar T, Kim SY. Continuous treatment with cisplatin induces the oocyte death of primordial follicles without activation. FASEB J (2020) 34:13885–99. doi: 10.1096/fj.202001461RR
8. Spears N, Lopes F, Stefansdottir A, Rossi V, De Felici M, Anderson RA. Ovarian damage from chemotherapy and current approaches to its protection. Hum Reprod Update (2019) 25:673–93. doi: 10.1093/humupd/dmz027
9. Zhang LJ, Tao SY, Zhao PW, Mao XL. Experimental study of cisplatin in establishment of premature ovarian failure model. World Chin Med (2015) 10:1563–1565+1569. doi: 10.3969/j.issn.1673-7202.2015.10.029
10. Dong R, Zhu X, Fan BZ, Xu W. Intraperitoneal injection in different dosages of cisplatin to establish a rat model of premature ovarian failure induced by chemotherapy. Lab Anim Comp Med (2020) 40:104–9. doi: 10.3969/j.issn.1674-5817.2020.02.003
11. Yeh J, Kim BS, Peresie J, Page C. Declines in levels of hyperpolarization-activated cation (HCN) channels in the rat ovary after cisplatin exposure. Reprod Sci (2009) 16:986–94. doi: 10.1177/1933719109339217
12. Yeh J, Kim B, Liang Y, Peresie J. Mullerian inhibiting substance as a novel biomarker of cisplatin-induced ovarian damage. Biochem Biophys Res Commun (2006) 348:337–44. doi: 10.1016/j.bbrc.2006.06.195
13. Yeh J, Kim B, Liang Y, Peresie J. Gonadotropin stimulation as a challenge to calibrate cisplatin induced ovarian damage in the female rat. Reprod Toxicol (2009) 28:556–62. doi: 10.1016/j.reprotox.2009.08.003
14. Shi XY. Modern medical experimental zoology [M] Vol. 334. . Beijing: People's Military Medical Press (2000).
15. Nozaki Y, Furubo E, Matsuno T, Fukui R, Kizawa K, Kozaki T, et al. Collaborative work on evaluation of ovarian toxicity. 6) two- or four-week repeated-dose studies and fertility study of cisplatin in female rats. J Toxicol Sci (2009) 34(Suppl 1):SP73–81. doi: 10.2131/jts.34.s73
16. Liu MN, Zhang K, Xu TM. The role of BMP15 and GDF9 in the pathogenesis of primary ovarian insufficiency. Hum Fertil (2021) 24:325–32. doi: 10.1080/14647273.2019.1672107
17. Qin Y, Jiao X, Simpson JL, Chen ZJ. Genetics of primary ovarian insufficiency: New developments and opportunities. Hum Reprod Update (2015) 21:787–808. doi: 10.1093/humupd/dmv036
18. Persani L, Rossetti R, Di Pasquale E, Cacciatore C, Fabre S. The fundamental role of bone morphogenetic protein 15 in ovarian function and its involvement in female fertility disorders. Hum Reprod Update (2014) 20:869–83. doi: 10.1093/humupd/dmu036
19. Meireles A, Bilibio JP, Lorenzzoni PL, Conto ED, Nascimento FC, Cunha-Filho JS. Association of FSHR, LH, LHR, BMP15, GDF9, AMH, and AMHR polymorphisms with poor ovarian response in patients undergoing in vitro fertilization. JBRA assisted Reprod (2021) 25:439–46. doi: 10.5935/1518-0557.20210004
20. Heckmann BL, Green DR. LC3-associated phagocytosis at a glance. J Cell Sci (2019) 132:jcs222984. doi: 10.1242/jcs.222984
21. Romao S, Münz C. LC3-associated phagocytosis. Autophagy (2014) 10:526–8. doi: 10.4161/auto.27606
22. Yefimova MG, Ravel C, Rolland AD, et al. MERTK-mediated LC3-associated phagocytosis (LAP) of apoptotic substrates in blood-separated tissues: Retina, testis, ovarian follicles. Cells. (2021) 10:1443. doi: 10.3390/cells10061443
23. Herb M, Gluschko A, Schramm M. LC3-associated phagocytosis-the highway to hell for phagocytosed microbes. Semin Cell Dev Biol (2019) 101:68–76. doi: 10.1016/j.semcdb.2019.04.016
24. Klionsky DJ, Abdel-Aziz AK, Abdelfatah S, Abdellatif M, Abdoli A, Abel S, et al. Guidelines for the use and interpretation of assays for monitoring autophagy (4th edition)1. Autophagy (2021) 17:1–382. doi: 10.1080/15548627.2020.1797280
25. Liang XH, Jackson S, Seaman M, Brown K, Kempkes B, et al. Induction of autophagy and inhibition of tumorigenesis by beclin 1. Nature (1999) 402:672–6. doi: 10.1038/45257
26. Xu HD, Qin ZH. Beclin 1, bcl-2 and autophagy. Adv Exp Med Biol (2019) 1206:109–26. doi: 10.1007/978-981-15-0602-4_5
27. Levine B, Liu R, Dong XN, Zhong Q. Beclin orthologs: integrative hubs of cell signaling, membrane trafficking, and physiology. Trends Cell Biol (2015) 25:533–44. doi: 10.1016/j.tcb.2015.05.004
28. Gaytán M, Morales C, Sánchez-Criado JE, Criado S, Gaytán F. Immunolocalization of beclin 1, a bcl-2-binding, autophagy-related protein, in the human ovary: possible relation to life span of corpus luteum. Cell Tissue Res (2008) 331(2):509–17. doi: 10.1007/s00441-007-0531-2
29. Fernández ÁF, Sebti S, Wei YJ, Zou ZJ, Shi MJ, McMillan KL, et al. Disruption of the beclin 1-BCL2 autophagy regulatory complex promotes longevity in mice. Nature (2018) 558:136–40. doi: 10.1038/s41586-018-0270-4
30. Levine B, Kroemer G. Autophagy in the pathogenesis of disease. Cell (2008) 132:27–42. doi: 10.1016/j.cell.2007.12.018
31. Escobar ML, Echeverria OM, Martínez SP, Vázquez-Nin GH, Chavero SJ, Gerardo H, et al. Beclin 1 interacts with active caspase-3 and bax in oocytes from atretic follicles in the rat ovary. J Histochem Cytochem (2019) 67:873–89. doi: 10.1369/0022155419881127
32. Li S. Network pharmacology evaluation method guidance-draft. World J Tradit Chin Med (2021) 4:527–32. doi: 10.4103/wjtcm.wjtcm_11_21
34. Wang ZY, Wang X, Li S. Traditional Chinese medicine network pharmacology development in new era under guidance of network pharmacology evaluation method guidance. China J Chin Materia Med (2022) 01:7–17. doi: 10.19540/j.cnki.cjcmm.20210914.702
35. Zhang YQ, Li S. Progress in network pharmacology for modern research of traditional Chinese medicine. Chin J Pharmacol Toxicol (2015) 06:883–92. doi: 10.3867/j.issn.1000-3002.2015.06.002
36. Li S, Zhang B. Traditional Chinese medicine network pharmacology: theory, methodology and application. Chin J Natural Medicines (2013) 02:0110–20. doi: 10.1016/S1875-5364(13)60037-0
37. Li DX, Wang Z, Zhang J. Constituents determination of kuntai capsule by LC-ESI-MS / MS. Chin J Exp Traditional Med Formulae (2017) 19:90–3. doi: 10.13422/j.cnki.syfjx.2017190090
38. Wang LJ, Yang Q, Zhu P. Investigation for molecular network and biological functions of kuntai capsule based on bioinformatics analysis. Chin Traditional Herbal Drugs (2018) 11:2601–5. doi: 10.7501/j.issn.0253-2670.2018.11.017
39. Chen YM, Zhu QH, Gao Q. Mechanism and experimental verification of antioxidant action of heyan kuntai capsule based on systematic pharmacology. Chin Traditional Herbal Drugs (2020) 17:4506–12. doi: 10.7501/j.issn.0253-2670.2020.17.019
40. Hsueh AJW, Kawamura K, Cheng Y, Bart C. Intraovarian control of early folliculogenesis. Endocr Rev (2015) 36:1–24. doi: 10.1210/er.2014-1020
41. Ramírez-Camacho R, García-Berrocal JR, Buján J, Marero AM, Trinidad A. Supporting cells as a target of cisplatin-induced inner ear damage: therapeutic implications. Laryngoscope (2004) 114:533–7. doi: 10.1097/00005537-200403000-00027
42. Kociba RJ, Sleight SD. Acute toxicologic and pathologic effects of cis-diamminedichloroplatinum (NSC-119875) in the male rat. Cancer Chemother Rep (1971) 55:1–8.
Keywords: Kuntai capsule, cisplatin, premature ovarian insufficiency, rats, protein expression, ovarian function
Citation: Luo S, Ruan X and Mueck AO (2023) The effect of Kuntai capsule on ovarian function in cisplatin-induced premature ovarian insufficiency rats. Front. Endocrinol. 13:1097165. doi: 10.3389/fendo.2022.1097165
Received: 13 November 2022; Accepted: 29 December 2022;
Published: 19 January 2023.
Edited by:
Abdel Halim Harrath, King Saud University, Saudi ArabiaCopyright © 2023 Luo, Ruan and Mueck. This is an open-access article distributed under the terms of the Creative Commons Attribution License (CC BY). The use, distribution or reproduction in other forums is permitted, provided the original author(s) and the copyright owner(s) are credited and that the original publication in this journal is cited, in accordance with accepted academic practice. No use, distribution or reproduction is permitted which does not comply with these terms.
*Correspondence: Xiangyan Ruan, ruanxiangyan@ccmu.edu.cn