- 1Key Laboratory of Freshwater Fisheries and Germplasm Resources Utilization, Ministry of Agriculture and Rural Affairs, Freshwater Fisheries Research Center, Chinese Academy of Fishery Sciences, Wuxi, China
- 2Wuxi Fisheries College, Nanjing Agricultural University, Wuxi, China
Polo-like kinase 1 (Plk1) has multiple functions in the cell cycle, including in the maturation of centrosomes during the G2/M transition, the separation of centrosomes, and the activation of cyclin-dependent kinase 1 expression and spindle assembly. In this study, we investigated the potential regulatory roles of Plk1 in the reproductive development of the male oriental river prawn (Machrobrachium nipponense). The full cDNA sequence of Mn-Plk1 was 2360 base pairs long, with an open reading frame of 1836 base pairs encoding 611 amino acids. Protein sequence alignment identified a conserved serine/threonine kinase domain and two Polo-boxes. Phylogenetic tree analysis revealed that Mn-Plk1 had the closest evolutionary distance with Plk1s of freshwater prawns and then with those of crustacean species, whereas the evolutionary distance with mollusks was much more distant. Quantitative PCR analysis predicted that Mn-Plk1 plays essential roles in the regulation of gonad development. RNA interference analysis and histological observations showed that expression of insulin-like androgenic gland hormone decreased as the expression of Mn-Plk1 decreased, and fewer than 5% of cells were sperm cells at day 14 in the dsPlk1 injected prawns. This result indicated that Plk1 positively regulated testis development in M. nipponense by affecting the expression of this hormone. Our results highlight the functions of Plk1 in M. nipponense and provide valuable information that can be applied to establish artificial techniques to regulate testis development in this species.
Introduction
The X-organ–SG complex (XO–SG) is a principal neuroendocrine gland in crustaceans, and it is located in the eyestalk. It stores and releases many neurosecretory hormones (1) that play essential roles in the regulation of many biological functions in crustacean species (2–12). The neurosecretory hormones secreted by the XO–SG have been reported to have negatively regulatory effects on both testis and ovarian development of the oriental river prawn (Macrobrachium nipponense) (13–15). For example, Qiao et al. (2015) showed that knockdown of the expression of the gonad-inhibiting hormone gene from female M. nipponense promoted ovarian development, as verified by RNA interference (RNAi) (13). Testis development was induced by stimulating the expression of the insulin-like androgenic gland hormone (IAG) gene in M. nipponense when eyestalks were ablated in male prawns (14, 15). IAG is a well-studied gene among crustacean species. It promotes male sex differentiation and the establishment of the male sex characteristics, especially testis development (16, 17). In Macrobrachium rosenbergii, decreased IAG expression caused by RNAi inhibited male sex differentiation and development of secondary sexual characteristics, especially spermatogenesis (18). Similar functions of IAG are well known in many other crustacean species (19–23).
Researchers have conducted transcriptome profiling analysis of the androgenic gland after the ablation of eyestalks from male M. nipponense in order to identify metabolic pathways and genes that are involved in the reproductive development of male M. nipponense (14). They proposed that the cell cycle plays essential roles in the regulation of male reproductive development in M. nipponense because it was the main metabolic pathway enriched in differentially expressed genes (14). The cell cycle ensures correct cell proliferation and plays crucial regulatory roles in the prevention and/or correction of damaged DNA, mutations, and genetic abnormalities (24, 25). Thus, the up-regulated and enriched genes in the metabolic pathway of the cell cycle were predicted to regulate the process of reproductive development in male M. nipponense based on the negative relationship between the neurosecretory hormones secreted by the XO–SG and reproductive development of male prawns.
Previously, polo-like kinase 1 (Plk1) was found to be enriched in the metabolic pathway of the cell cycle, and its expression was significantly up-regulated in the androgenic gland after eyestalk ablation in male M. nipponense (14). This finding suggested that Plk1 may promote the reproductive development of male M. nipponense. The cell cycle process is regulated by a variety of kinases, including those in the Plk family. Plk1 was the first and continues to be the most studied Plk, and it is involved in regulation of the cell cycle. It can promote the maturation of centrosomes during the G2/M transition and the separation of centrosomes, and it can regulate the activation of cyclin-dependent kinase 1 and spindle assembly (26, 27). Plk1 proteins are reported to be expressed during the late G2/M phase, regulating the process of mitosis in all animal species (28). Previous studies have shown that Plk1 plays essential roles in therapies designed to treat a variety of cancers, including primary colorectal cancer (29), prostate and pancreatic cancers (30), and rectal cancer (31). However, studies of the effects of Plk1 on gonad development are rare. Knockdown of the expression of Plk1 was shown to inhibit female meiosis in the fruit fly Drosophila melanogaster, indicating that Plk1 is involved in the process of oogenesis (32). Additionally, the Plk1 of the blood fluke Schistosoma mansoni was reported to be a key mitotic kinase that is involved in the process of parasite reproduction (33).
M. nipponense is widely distributed in freshwater and low-salinity estuarine regions in China and other Asian countries. It is a commercially important species in China, providing huge economic benefits (34). The rapid gonad development of hatchlings has restricted the sustainable development of the M. nipponense industry, as newly hatched male and female M. nipponense can reach sexual maturity within 40 days after hatching during the reproductive season (35). Rapid gonad development affects the market size of M. nipponense because it has negative regulatory effects on growth performance (36, 37). Thus, an artificial technique to regulate the process of gonad development in this species is urgently needed.
The goal of this study was to investigate the potential functions of Plk1 in male reproductive development of M. nipponense using quantitative real-time PCR (qPCR), RNAi, and histological observations. Our results can be applied to development of an artificial technique to regulate the process of gonad development in M. nipponense. They also provide a basis for further studies of the mechanism of male reproductive development in other crustacean species.
Methods and materials
Ethics approval
Permission to conduct the experiments was obtained from the the Freshwater Fisheries Research Center (2021JBFM02, 12-06-2021). All experiments were performed in accordance with Jiangsu Laboratory’s Animal Management Guidelines (014000319/2008-00079). All M. nipponense used in this study were collected from the Dapu M. nipponense Breeding Base (120°13′44″E, 31°28′ 22″N; Wuxi, China). All prawns were maintained for 3 days in aerated freshwater with dissolved oxygen content ≥ 6 mg/L prior to tissue collection. An ice bath was used to anesthetize the prawns when tissues were collected (38).
Tissue collection
Testis were collected for the synthesis of templates and used for rapid amplification of cDNA ends (RACE) cloning. Different tissues collected for qPCR analysis included eyestalks, brain, heart, hepatopancreas, muscle, gonad, and gills from both male (body weight of 2.87-4.12 g) and female (body weight of 1.84-2.12 g) M. nipponense. Specimens at different developmental stages were collected from a full-sib population every 5 days during their maturation process. The body weights of the full-sib population were from 0.003-0.021 g. Testis and androgenic gland were sampled during both the reproductive season in July (water temperature of 30 ± 2°C, illumination time ≥ 16 h) (body weight of 3.12-4.47 g) and the non-reproductive season in January (water temperature of 13 ± 2°C, illumination time ≤ 12 h) (body weight of 2.15-3.97 g). For each tissue, sex, and time point, five samples were pooled together to form a biological replicate, and three biological replicates were used for qPCR analysis. All collected tissues were immediately preserved in liquid nitrogen until used for qPCR analysis.
RACE
Table 1 lists the specific primers used for Mn-Plk1 cloning. The primers were designed using the Primer-BLAST tool from NCBI (http://www.ncbi.nlm.nih.gov/tools/primer-blast/) based on the open reading frame (ORF) of Mn-Plk1. The full-length cDNA of Mn-Plk1 was cloned using the RACE technique following the methods described in previous studies (39, 40). Briefly, the total RNA from the testis was extracted using RNAiso Plus Reagent (Takara, Shiga, Japan), and the extracted total RNA was then used to synthesize the templates for 3′cDNA and 5′cDNA cloning using the 3′-Full RACE Core Set Ver.2.0 kit and the 5′-Full RACE kit (Takara), respectively. After the assembling, two pairs of primer were designed based on the full-length cDNA sequence of Mn-Plk1, in order to verify the accuracy of Mn-Plk1 cDNA sequence (Table 1).
Structural analysis of Mn-Plk1
The structural characteristics of Mn- Plk1 were analysed using the BLASTx and BLASTN search program (http://www.ncbi.nlm.nih.gov/BLAST/) and the ORF Finder tool (http://www.ncbi.nlm.nih.gov/gorf/gorf.html). The theoretical isoelectric point and the molecular weight of Mn-Plk1 protein were measured using the ComputepI/Mwtool software (http://ca.expasy.org/tools/pi_tool.html). Table 2 lists the accession numbers of amino acid sequences of different species, which were used to construct the phylogenetic tree of Plk1 using MEGA X, the maximum-likelihood method, and the bootstrap method with 1000 replications.
qPCR analysis
qPCR was used to determine the relative mRNA expression of Mn-Plk1 in the different tissues. Table 1 lists the primers used for qPCR analysis, which was performed following previously described methods (39, 40). Briefly, RNAiso Plus Reagent was used to extract the total RNA from each tissue following the manufacturer’s protocol. After removing genomic DNA by gDNA Eraser, the total RNA was used to synthesize the cDNA template using PrimeScript™ RT reagent Kit (Takara) based on the manufacturer’s protocol. The UltraSYBR Mixture (CWBIO, Beijing, China) with the SYBR Green RT-qPCR assay was used to determine the expression level of Mn-Plk1 in each tissue, and the Bio-Rad iCycler iQ5 Real-Time PCR System (Bio-Rad, Hercules, CA, USA) was used to perform the qPCR analyses. The amplification protocols for qPCR analysis were 95°C for 10 min, followed by 37 cycles of 95°C for 10 s and 60°C for 1 min. All primers for qPCR analysis were listed in Table 3. The eukaryotic translation initiation factor 5A is a stable reference gene for qPCR analysis in M. nipponense, and it was used in the present study (41). The relative mRNA expression levels of Mn-Plk1 were calculated using on the 2−ΔΔCT comparative CT method (42).
RNAi analysis
The potential function of Plk1 in reproductive development of male M. nipponense was investigated by RNAi, which has been widely used in gene functional analysis in this species. Table 1 lists the specific RNAi primer with a T7 promoter site, which was designed using Snap Dragon tools (http://www.flyrnai.org/cgibin/RNAifind_primers.pl) based on the ORF of Mn-Plk1. The Mn-Plk1 dsRNA (dsPlk1) was synthesized using the Transcript Aid™ T7 High Yield Transcription kit (Fermentas, Waltham, MA, USA) following the manufacturer’s protocol, and the green fluorescent protein dsRNA (dsGFP) was also synthesized and used as the negative control (43).
Six hundred male M. nipponense were collected from the Dapu M. nipponense Breeding Base and randomly divided into the dsPlk1 group (RNAi) and dsGFP group (control) (N = 300). These prawns were collected at approximately 5 months after hatching and had a body weight of 3.14−4.32 g. The dsPlk1 and dsGFP were injected into the prawns through the cardio coelom with the injected dose of 4 μg/g according to previously described methods (44). dsPlk1 and dsGFP were injected once and then again with the same dose after 7 days. Androgenic gland samples (N=15) were collected from both the control group and the RNAi group on days 1, 7, and 14 after dsGFP or dsPlk1 injection. The Mn-Plk1 mRNA expression levels were measured by qPCR to confirm the silencing efficiency. The mRNA expression of Mn-IAG was also measured using the same cDNA templates to analyse the regulatory relationship between Plk1 and IAG in M. nipponense.
Haematoxylin and eosin (HE) staining
The morphological differences between testis samples taken from the dsPlk1- and dsGFP-injected prawns were assessed by histological observations of tissues stained with HE. The histological observations were performed following previously described methods (45, 46). The slides were observed under an Olympus SZX16 microscope (Olympus Corporation, Tokyo, Japan). The cell types were labelled according to the previous studies (14, 15), and the numbers of each cell type were counted through the whole testis after the treatment of dsGFP and dsPlk1.
Statistical analysis
SPSS Statistics 23.0 (IBM, Armonk, NY, USA) was used to evaluate differences in Mn-Plk1 expression levels, followed the independent samples t-test and analysis of ANOVA variance (least significant difference and Duncan’s multiple range tests). Quantitative data were expressed as mean ± standard deviation. P < 0.05 and P < 0.01 were considered to be differentially expressed and significantly differentially expressed, respectively.
Results
Sequence analysis
The full-length cDNA sequence of Mn-Plk1 was 2,360 base pairs (bp) long, with a 5′ and a 3′ untranslated region of 92 bp and 432 bp, respectively. The ORF of Mn-Plk1 was 1,836 bp long and encoded 611 amino acids (Figure 1). The cDNA sequence of Mn-Plk1 was submitted to NCBI with the accession number OP379748. The theoretical isoelectric point of Mn-Plk1 was 4.90, and the molecular weight of the protein was 18.466 kDa.
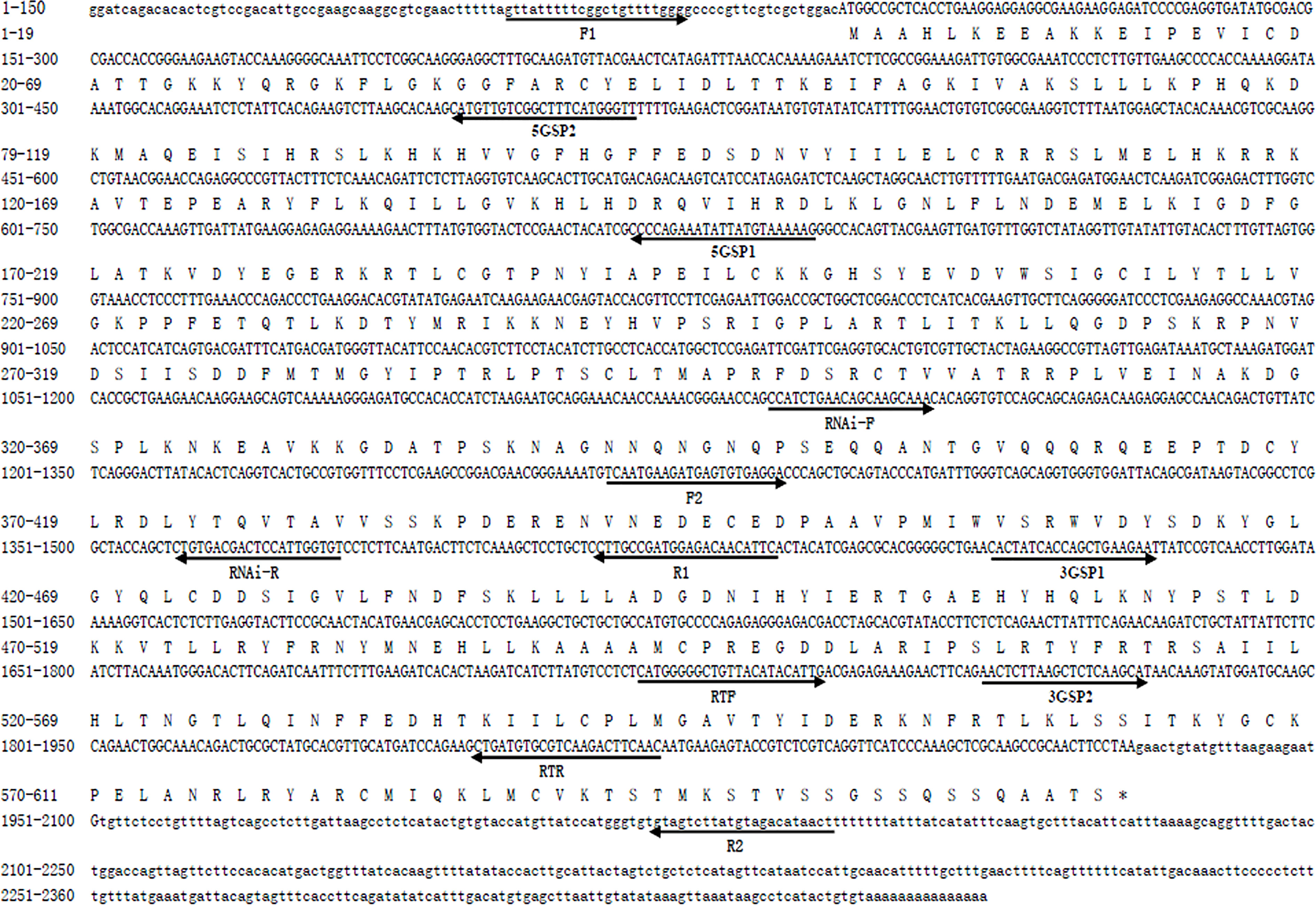
Figure 1 Nucleotide and deduced amino acid sequence of Mn-Plk1. The nucleotide sequence is displayed in the 5′–3′ directions and numbered at the left. The deduced amino acid sequence is shown in a single capital letter amino acid code. Arrows indicated the location and direction of each primer. 3′ UTR and 5′ UTR are shown with lowercase letters. Codons are numbered at the left with the methionine (ATG) initiation codon, an asterisk denotes the termination codon (TGA).
The protein sequence of Mn-Plk1 shared over 80% identity with the Plk1 sequences from other crustacean species in NCBI based on the BLASTx analysis. The species included Procambarus clarkii (83.03%, XP_045623220.1), Penaeus monodon (82.42%, XP_037785985.1), Penaeus chinensis (82.42%, XP_047493190.1), Penaeus japonicus (82.06%, XP_042864001.1), and Penaeus vannamei (81.20%, XP_027211725.1) (Figure 2). Protein sequence alignment of Plk1 from different species revealed that Mn-Plk1 contains the common structure of Polo members, including a highly conserved N-terminal kinase and C-terminal protein binding domain (PBD). The conserved N-terminal kinase is composed of a serine/threonine kinase domain, of which the sub-domains I to XI have been labelled (Figure 2). The C-terminal PBD is composed of two Polo-boxes called Polo-box 1 and Polo-box 2 (Figure 2).
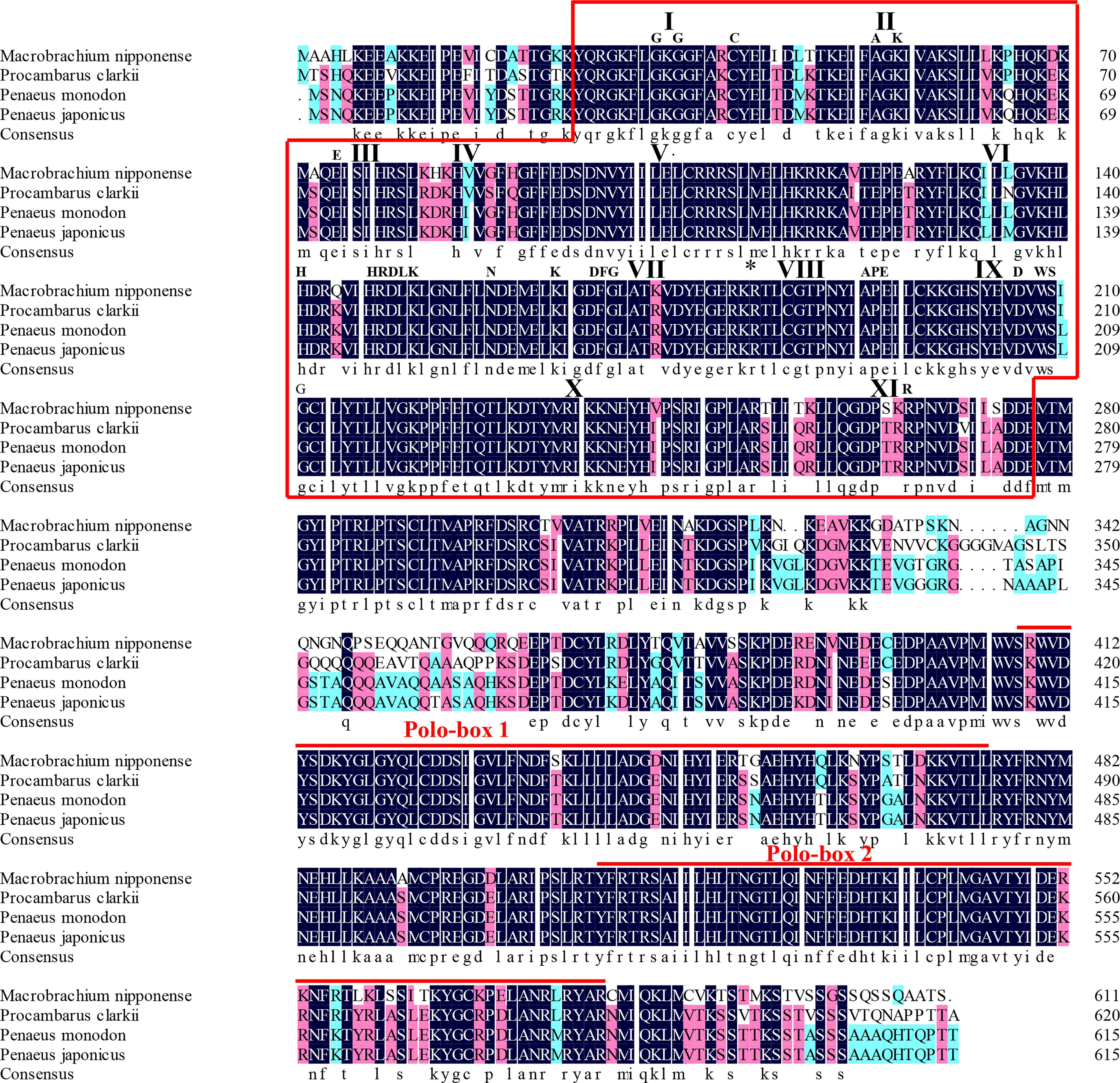
Figure 2 The similarity identity of amino acid sequences of Plk1 between different species. The Serine/Threonine (Ser/Thr) kinase domain composed of sub-domains I to XI is boxed. Important motifs and residues conserved in Ser/Thr kinases (HRDLKxN, KxxDFG, APE, DxWSxG and R) are indicated above the alignment. The asterisk indicates the position of the conserved T182 residue essential for kinase activation and the bullet point indicates the L104 residue involved in BI 2536 binding. The two Polo-box domains (PBD) are over-lined.
Phylogenetic tree analysis
The phylogenetic tree analysis revealed that the protein sequences of Plk1 from different species were mainly divided into two branches: crustacean species and mollusks species (Figure 3). Mn-Plk1 was clustered into the crustacean branch and had the closest evolutionary distance with that of crayfish, and then it clustered with the Plk1s of marine shrimp, followed by that of crabs. The evolutionary distance of Mn-Plk1 with Plk1s of mollusks species was much more distant.
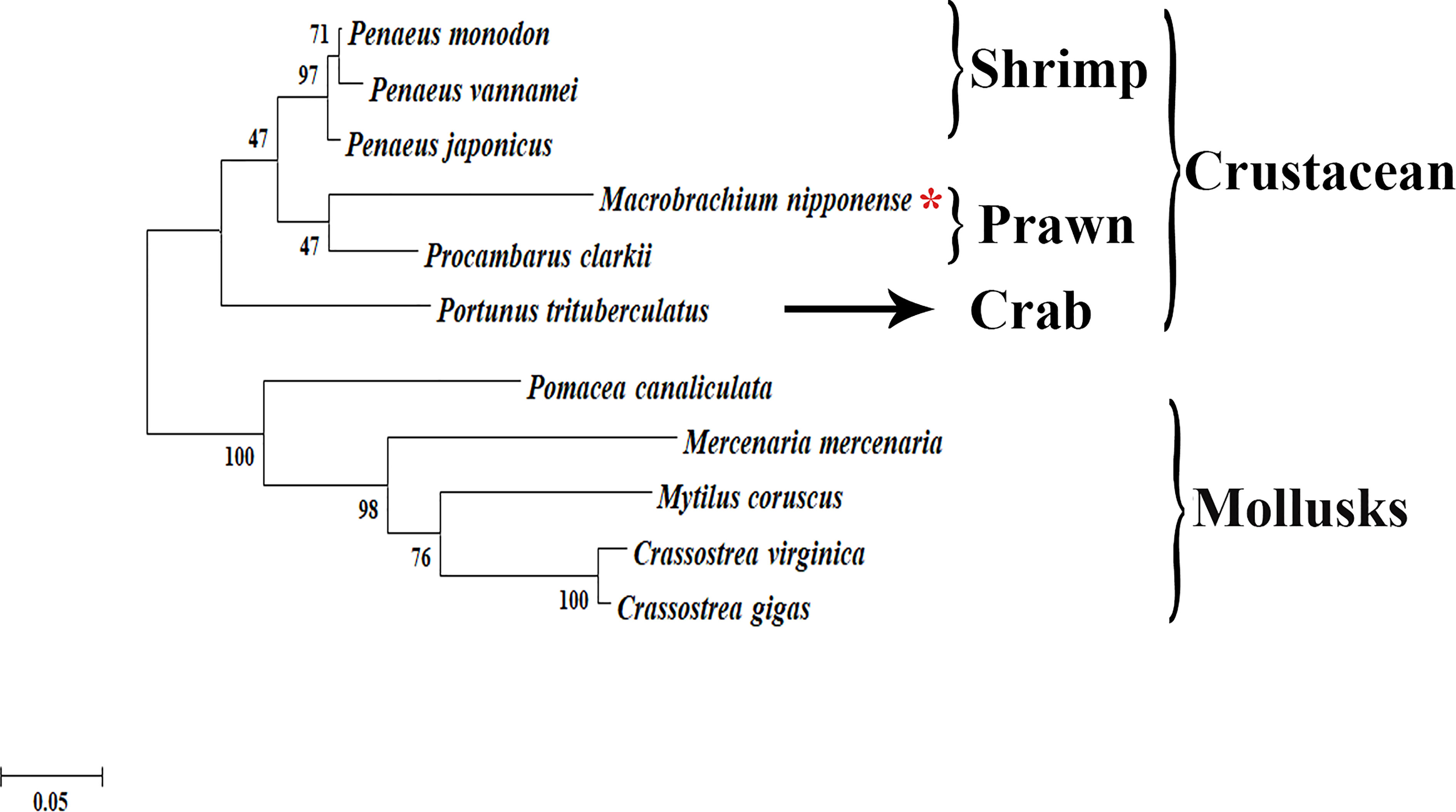
Figure 3 The phylogenetic tree of Plk1 from different organisms based on amino acid sequence comparisons. Species names of Plk1 are listed on the right of the tree. Red asterisk indicated M. nipponense.
qPCR analysis
The qPCR analysis revealed that the highest expression of Mn-Plk1 occurred in the testis and ovary of male and female prawns, respectively, and it was significantly higher than the expression in the other tested tissues (P < 0.01). The expression levels in the testis and ovary were 505.56-fold and 840.39-fold higher than that of the female eyestalk, which had the lowest expression of all of the tested tissues. Mn-Plk1 expression levels were higher in the eyestalk, brain, heart, and hepatopancreas of male prawns than of female prawns, whereas the expression levels in the muscle, gonad, and gill showed the opposite pattern (Figure 4A).
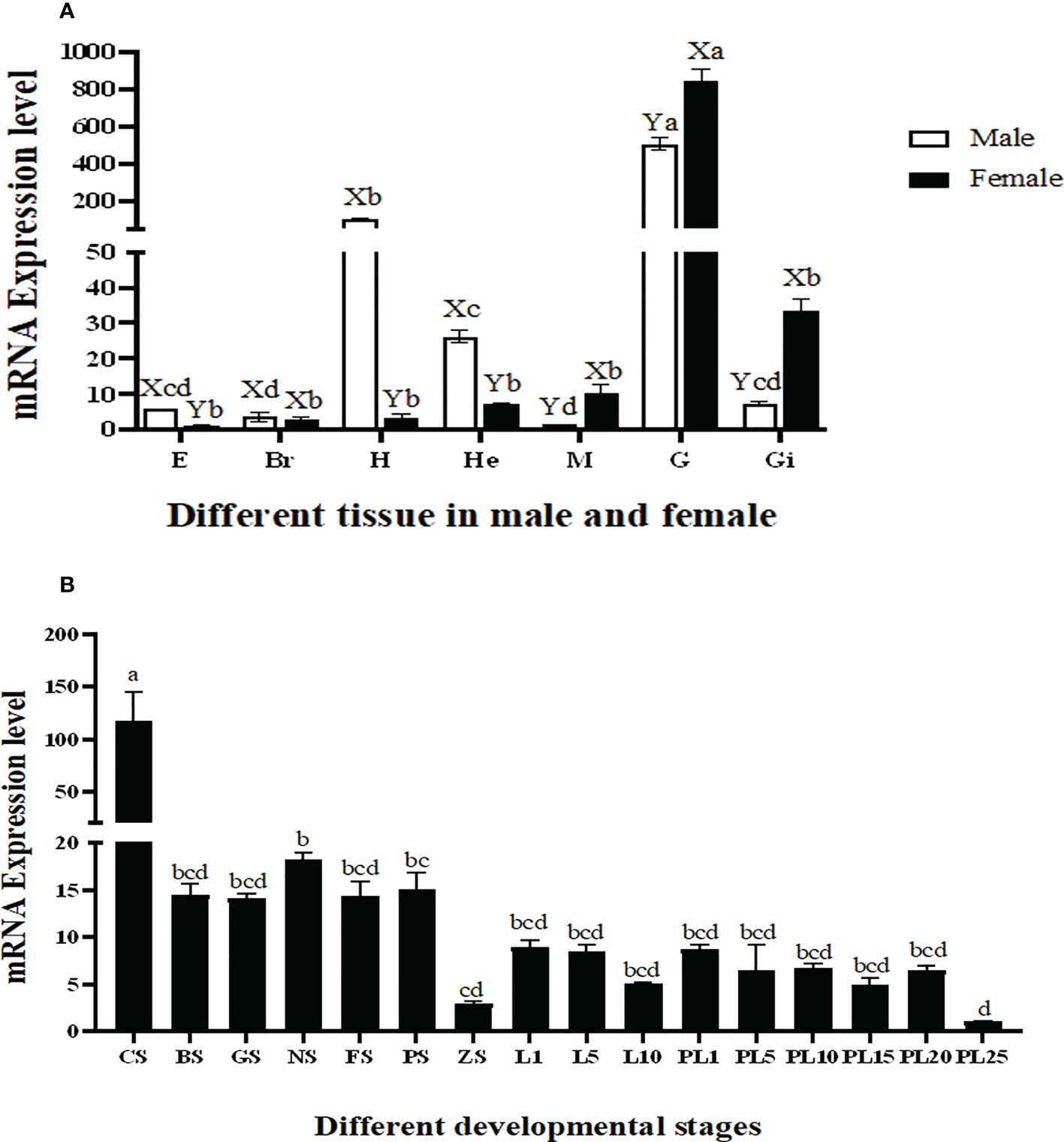
Figure 4 Measurement of the Mn-Plk1 expression in different mature tissues and developmental stages by qPCR. The amount of Mn-Plk1 mRNA was normalized to the EIF transcript level. Data are shown as mean ± SD (standard deviation) of tissues from three separate individuals. Lowercases (a-d) on the bars indicate expression differences among all tissues from both sex (ANOVA). Capital letters (X, Y) on the bars indicate the expression differences in the same tissue between male and female prawns (independent sample T-test). (A) Mn-Plk1 expression in different mature tissues. (B) Mn-Plk1 expression in different developmental stages. E, eyestalk; Br, brain; H, heart; He, hepatopancreas; M, muscle; G, gonad; Gi, gill; CS, cleavage stage; BS, blastula stage, GS, gastrula stage; NS, nauplius stage; FS, posterior nauplius stage; PS, protozoea stage; GS, zoea stage; L, larval developmental stage; PL, post-larval developmental stage.
Mn-Plk1 expression was widely detected during the whole developmental process of juvenile prawns, but peak expression occurred at the cleavage stage and was significantly higher than that at the other tested stages (P < 0.01). The expression levels during the larval and post-larval developmental stages (PL) generally remained stable and showed no significant differences (P > 0.05) (Figure 4B). The lowest expression of Mn-Plk1 was observed at PL25, and the value at cleavage stage was 117.57 folds higher than that of PL25.
The qPCR analysis also revealed that Mn-Plk1 expression was higher in the testis and androgenic gland from samples collected during the reproductive season than during the non-reproductive season. The values in the testis and androgenic gland from the reproductive season were 2.69-fold and 3.36-fold higher than those from the non-reproductive season, respectively, and the differences were statistically significant (P < 0.01) (Figure 5).
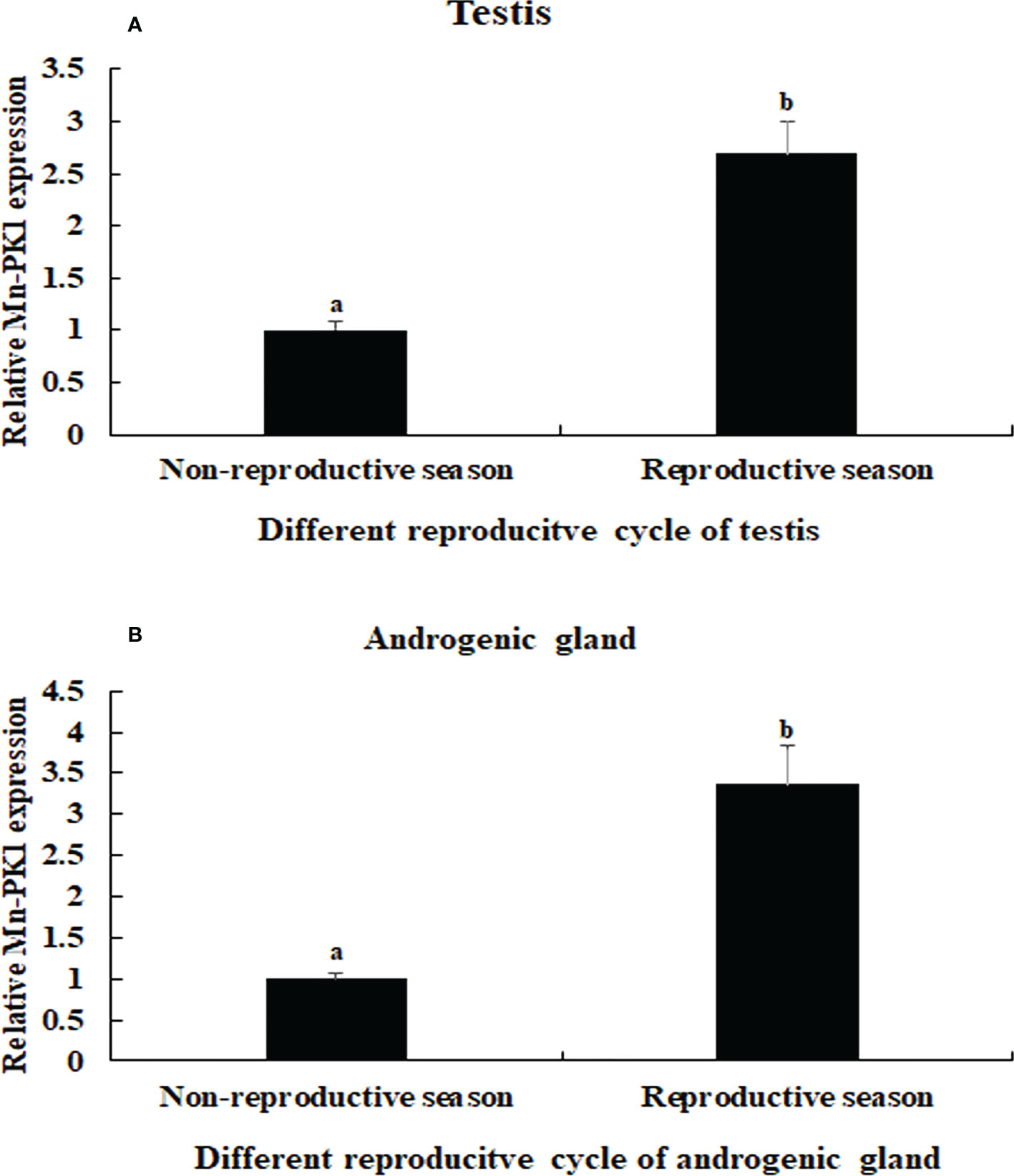
Figure 5 Measurement of the Mn-Plk1 expression in the testis and androgenic gland taken from different reproductive season. The amount of Mn-Plk1 mRNA was normalized to the EIF transcript level. Data are shown as mean ± SD (standard deviation) of tissues from three separate individuals. Lowercases (a, b) on the bars indicate expression differences between different samples (independent sample T-test). (A) Mn-Plk1 expression in the testis taken from different reproductive season. (B) Mn-Plk1 expression in the androgenic gland taken from different reproductive season.
RNAi analysis
In the RNAi experiment, qPCR analysis revealed that the Mn-Plk1 expression remained at a stable level in the androgenic gland after the injection of dsGFP (P > 0.05), whereas it was significantly decreased after the injection of dsPlk1. The decrease reached 94.8% and 88.9% at days 7 and 14, respectively, after the injection of dsPlk1 compared with the injection of dsGFP, and the values were significantly different (P < 0.01) (Figure 6A).
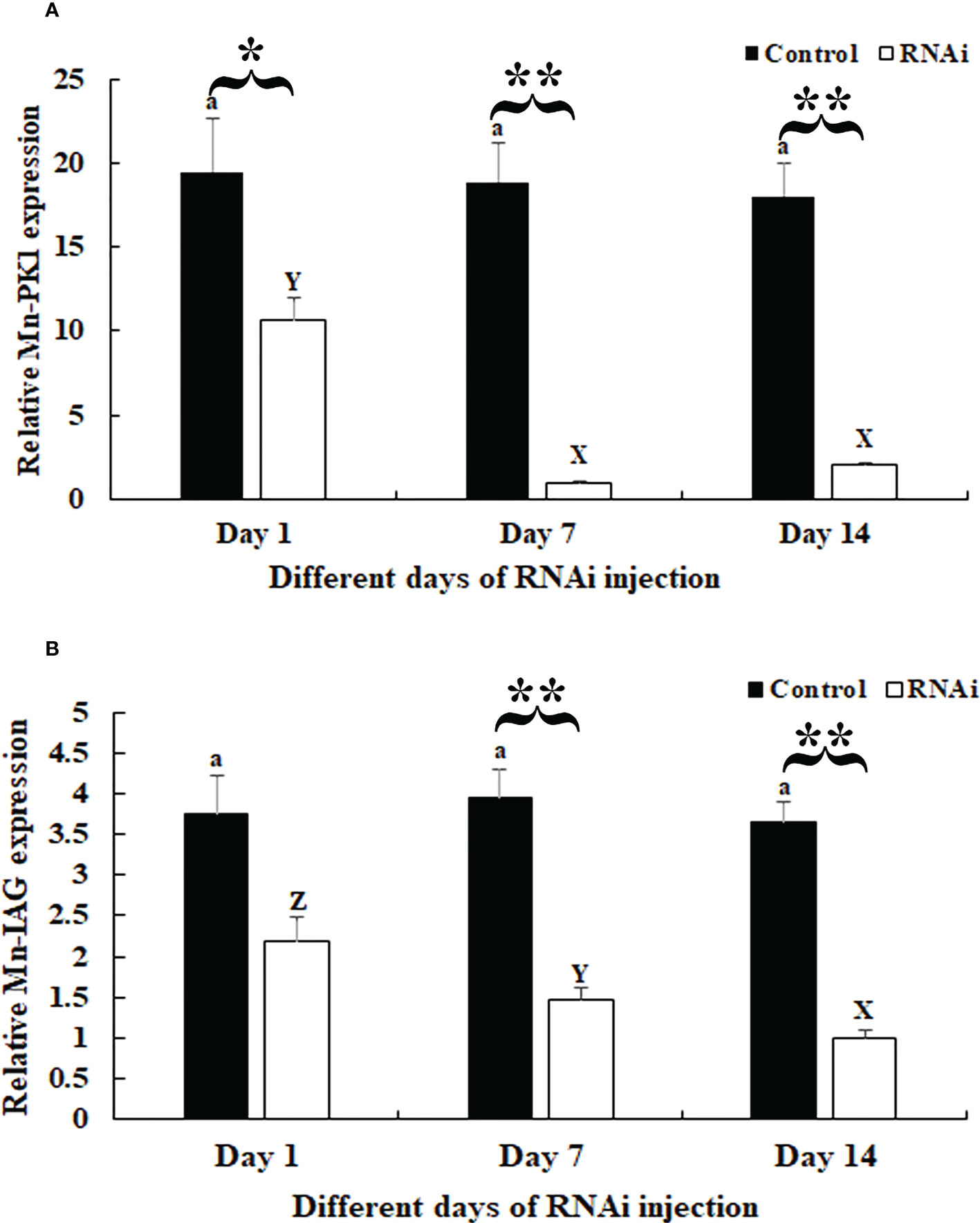
Figure 6 Measurement of Mn-Plk1 and Mn-IAG expression at different days after dsPlk1 and dsGFP injection. The amount of Mn-Plk1 and Mn-IAG mRNA was normalized to the EIF transcript level. Data are shown as mean ± SD (standard deviation) of tissues from three separate individuals. Lowercases (a and b) on the bars indicate the expression differences between different days in the dsGFP injected prawns, while capital letters (X, Y and Z) on the bars indicate expression differences between different days in the dsPlk1 prawns (ANOVA). *(P < 0.05) and **(P < 0.01) indicates significant expression difference between the RNAi group and control group at the sample day (independent sample T-test). (A) Measurement of Mn-Plk1 expression at different days after dsGFP and dsPlk1 injection. (B) Measurement of Mn-IAG expression at different days after dsGFP and dsPlk1 injection.
The expression of Mn-IAG in the same cDNA templates also remained at a stable level in the dsGFP-injected prawns (P > 0.05), but it decreased in the dsPlk-injected prawns. Decreases of > 55% and 60% were observed at days 7 and 14, respectively, in the dsPlk1 group compared to the dsGFP group (P < 0.01) (Figure 6B).
Histological observation revealed significant morphological differences between the testis from dsGFP- and dsPlk1-injected prawns. The cells in the testis were almost the same on different days after dsGFP injection, and sperm were the dominant cells, which were dramatically more than that of spermatogonia and spermatocyte (Figures 7A, B). However, the number of sperm decreased with time after dsPlk1 injection, while the percentages of spermatogonia and spermatocytes increased. Sperm (approximate 5%) were rarely observed on day 14 in dsPlk1-injected prawns, while over 80% cells were observed as spermatogonia (Figures 7A, C).
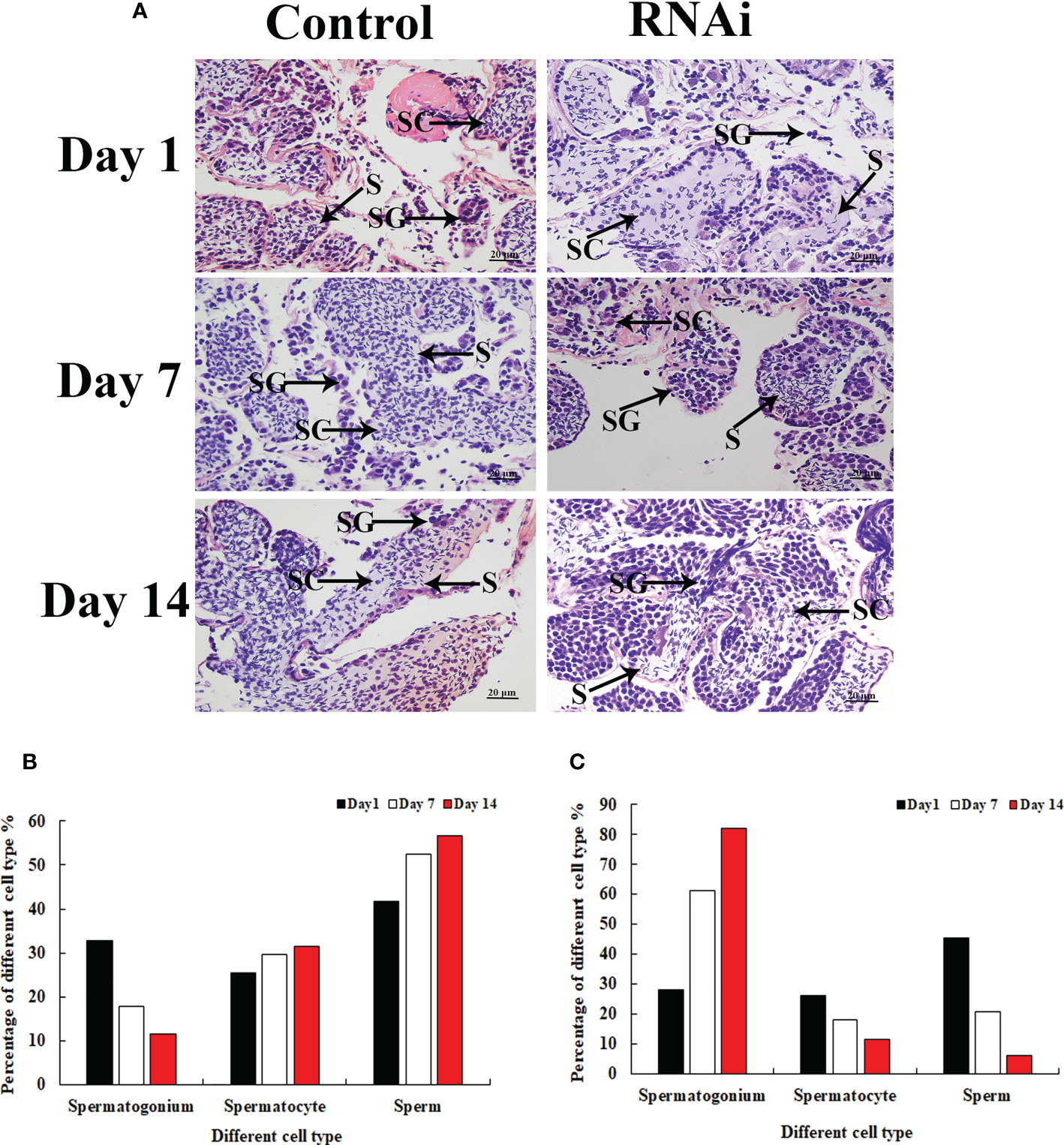
Figure 7 The histological observations of testis between RNAi and control group. SG, Spermatogonia; SC, spermatocyte; S, sperm. Scale bars = 20 μm. (A) The histological observations of testis taken from the dsGFP and dsPlk1 injected prawns. (B) The percentage of different cell types in the testis of dsGFP injected prawns. (C) The percentage of different cell types in the testis of dsPlk1 injected prawns..
Discussion
Plk1 plays vital roles in the regulation of maturation of centrosomes during the G2/M transition, the separation of centrosomes, and the activation of cyclin-dependent kinase 1 expression and spindle assembly during the cell cycle (26, 27). Plk1 was tested in therapies for primary colorectal cancer (29), prostate and pancreatic cancers (30), and rectal cancer (31). However, studies of the role of Plk1 in gonad development are scarce. A previous study predicted that Plk1 has potential functions in the reproductive development of male M. nipponense (14). In the present study, we investigated the regulatory functions of Plk1 in testis development of M. nipponense using qPCR, RNAi analysis, and histological observations.
BLASTx analysis revealed that the protein sequence of Mn-Plk1 shared over 80% identity with the Plk1 protein sequences from other crustacean species. Furthermore, protein sequence alignment analysis of Plk1 identified some common structures of Polo members, including a highly conserved N-terminal kinase with a serine/threonine kinase domain and a C-terminal PBD with two Polo-boxes, which was consistent with a previous report (33). These results indicated that the cDNA sequence obtained in this study was the Plk1 sequence of M. nipponense. The phylogenetic tree analysis showed a close evolutionary distance between Mn-Plk1 and the Plk1s of other crustacean species but a more distant relationship with those of mollusks species. Mn-Plk1 has the closest evolutionary distance with the Plk1 of P. clarkii, which is the only freshwater prawn with a Plk1 sequence in NCBI. Further studies should identify more Plk1 sequences from freshwater prawns in order to promote the evolutionary analysis of Mn-Plk1.
In humans, overexpression of Plk1 can promote mitosis and normal bipolar spindle assembly, while inhibition the Plk1 expression delays mitosis (47). However, no study to date has reported qPCR analysis of Plk1 in any aquatic species. In the present study, the highest expression of Mn-Plk1 mRNA occurred in the testis and ovary of male and female prawns, respectively, and it was significantly higher than expression levels in the other tested tissues (P < 0.01). This result suggests that Plk1 plays essential roles in the regulation of gonad development in M. nipponense and that the process of mitosis is much vigorous in the gonad than in the other tissues. In addition, Mn-Plk1 expression levels in the testis and androgenic gland collected during the reproductive season were 2.69-fold and 3.36-fold higher than those taken during the non-reproductive season, respectively. Previous studies identified significant morphological differences in these organs between the reproductive and non-reproductive seasons, with much greater development during the former than the latter (48, 49). These results indicate that Plk1 is a strong candidate gene for involvement in the reproductive development of male M. nipponense. Our data showed that the peak expression of Plk1 occurred at the cleavage stage, indicating the process of mitosis was strongest during this stage.
Knockdown of the expression of Plk1 by RNAi has been shown to inhibit the proliferation of pancreatic cancer (50) and colorectal cancer (51) cells. RNAi has also been widely used to identify the functions of male reproductive genes in M. nipponense (52, 53). In the present study, a > 85% decrease of Mn-Plk1 expression was observed in the androgenic gland of dsPlk1-injected prawns on days 7 and 14 compared to that of dsGFP-injected prawns, indicating that the synthesized dsPlk1 in the present study efficiently knocked down the expression of Plk1 in M. nipponense. Interestingly, the decreased Mn-Plk1 expression led to a significant decrease of Mn-IAG expression, indicating that Plk1 positively regulates IAG expression in M. nipponense. IAG has been proven to promote male sex differentiation and reproduction in crustacean species, especially testis development (16–18). Histological observation revealed morphological differences between the testis of dsPlk1- and dsGFP-injected prawns. For example, on day 14 approximate 5% of cells were identified as sperm in the prawns injected with dsPlk1, and this number was much lower than that in dsGFP-injected prawns. This result indicated that dsPlk1 had a significant inhibitory effect on testis development in M. nipponense.
In conclusion, our results indicate that Plk1 regulates testis development by affecting IAG expression in M. nipponense. These results provide valuable information that can be applied to establish artificial techniques to regulate testis development in this species through editing the gene sequence of Mn-Plk1 by CRISPR or knockdown the expression levels of Mn-Plk1 during the sex-differentiation sensitive period by RNAi.
Data availability statement
The original contributions presented in the study are included in the article/supplementary material. Further inquiries can be directed to the corresponding authors.
Ethics statement
Permission to conduct the experiments was obtained from the the Freshwater Fisheries Research Center (2021JBFM02, 12-06-2021). All experiments were performed in accordance with Jiangsu Laboratory’s Animal Management Guidelines (014000319/2008-00079). Written informed consent was obtained from the owners for the participation of their animals in this study.
Author contributions
ShJ wrote the manuscript. PW performed the RACE cloning, WZ analyzed the sequence characteristics. SuJ provided the experimental prawns. HQ performed the qPCR analysis. YG performed the RNAi analysis. YW performed the histological observations. YX revised the manuscript. HF supervised the manuscript. All authors contributed to the article and approved the submitted version.
Funding
This research was supported by grants from the Natural Science Foundation of Jiangsu Province (BK20221207), Central Public-Interest Scientific Institution Basal Research Fund, CAFS (2021JBFM02 and 2020TD36), National Key R&D Program of China (2018YFD0900201), Jiangsu Agricultural Industry Technology System (JATS[2020]461), China Agriculture Research System-48 (CARS-48), and New Cultivar Breeding Major Project of Jiangsu Province (PZCZ201745).
Conflict of interest
The authors declare that the research was conducted in the absence of any commercial or financial relationships that could be construed as a potential conflict of interest.
Publisher’s note
All claims expressed in this article are solely those of the authors and do not necessarily represent those of their affiliated organizations, or those of the publisher, the editors and the reviewers. Any product that may be evaluated in this article, or claim that may be made by its manufacturer, is not guaranteed or endorsed by the publisher.
Supplementary material
The Supplementary Material for this article can be found online at: https://www.frontiersin.org/articles/10.3389/fendo.2022.1084802/full#supplementary-material
References
1. Hopkins PM. The eyes have it: A brief history of crustacean neuroendocrinology. Gen Comp Endocrinol (2012) 175:357–66. doi: 10.1016/j.ygcen.2011.12.002
2. Revathi P, Iyapparaj P, Vasanthi LA, Jeyanthi S, Krishnan M. Impact of eyestalk ablation on the androgenic gland activity in the freshwater prawn Macrobrachium rosenbergii (De man). World (2013) 5:373–81. doi: 10.5829/idosi.wjfms.2013.05.04.731
3. Treerattrakool S, Panyim S, Udomkit A. A induction of ovarian maturation and spawning in penaeus monodon broodstock by double-stranded RNA. Mar Biotechnol (2011) 13:163–9. doi: 10.1007/s10126-010-9276-0
4. Treerattrakool S, Chartthai C, Phromma-in N, Panyim S, Udomkit A. Silencing of gonad-inhibiting hormone gene expression in penaeus monodon by feeding with GIH dsRNA-enriched artemia. Aquaculture (2013) 404:116–21. doi: 10.1016/j.aquaculture.2013.04.024
5. Pamuru RR, Rosen O, Manor R, Chung JS, Zmora N, Glazer L, et al. Stimulation of molt by RNA interference of the molt inhibiting hormone in the crayfish Cherax quadricarinatus. Gen Comp Endocrinol (2012) 178:227–36. doi: 10.1016/j.ygcen.2012.05.007
6. Salma U, Uddowla MH, Kim M, Kim JM, Bo KK, Baek HJ, et al. Five hepatopancreatic and one epidermal chitinases from a pandalid shrimp (Pandalopsis japonica): Cloning and effects of eyestalk ablation on gene expression. Comp Biochem Phys B (2012) 161:197–207. doi: 10.1016/j.cbpb.2011.11.005
7. Shen H, Zhou X, Bai A, Ren X, Zhang Y. Ecdysone receptor gene from the freshwater prawn Macrobrachium nipponense: identification of different splice variants and sexually dimorphic expression, fluctuation of expression in the molt cycle and effect of eyestalk ablation. Gen Comp Endocrinol (2013) 193:86–94. doi: 10.1016/j.ygcen.2013.07.014
8. Santos EA, Eduardo L, Nery M, Goncalves AA, Keller R. Evidence for the involvement of the crustacean hyperglycemic hormone in the regulation of lipid metabolism. Physiol Biochem Zool (1997) 70:415–20. doi: 10.1086/515846
9. Almeida EA, Petersen RL, Andreatta ER, Bainy AC. Effects of captivity and eyestalk ablation on antioxidant status of shrimps (Farfantepenaeus paulensis). Aquaculture (2004) 238:523–8. doi: 10.1016/j.aquaculture.2004.04.010
10. Tiu SHK, Chan SM. The use of recombinant protein and RNA interference approaches to study the reproductive functions of a gonad-stimulating hormone from the shrimp metapenaeus ensis. FEBS J (2007) 274:4385–95. doi: 10.1111/j.1742-4658.2007.05968.x
11. Sainz-Hernandez JC, Racotta IS, Dumas S, Hernandez-Lopez J. Effect of unilateral and bilateral eyestalk ablation in litopenaeus vannamei male and female on several metabolic and immunologic variables. Aquaculture (2008) 283:188–93. doi: 10.1016/j.aquaculture.2008.07.002
12. Diarte-Plata G, Sainz-Hernández JC, Aguiñaga-Cruz JA, Fierro-Coronado JA, Polanco-Torres A, Puente-Palazuelos C. Eyestalk ablation procedures tominimize pain in the freshwater prawn Macrobrachium americanum. Appl Anim Behav Sci (2012) 140:172–8. doi: 10.1016/j.applanim.2012.06.002
13. Qiao H, Xiong YW, Zhang WY, Fu HT, Jiang SF, Sun SM, et al. Characterization, expression, and function analysis of gonad-inhibiting hormone in oriental river prawn, Macrobrachium nipponense and its induced expression by temperature. Comp Biochem Phys A (2015) 185:1–8. doi: 10.1016/j.cbpa.2015.03.005
14. Jin SB, Fu Y, Hu YN, Fu HT, Jiang SF, Xiong YW, et al. Identification of candidate genes of male sexual development from androgenic gland in Macrobrachium nipponense through performing long-reads and next generation transcriptome sequencing after eyestalk ablation. Sci Rep (2021) 11:19855. doi: 10.1038/s41598-021-99022-4
15. Jin S, Fu Y, Hu Y, Fu H, Jiang S, Xiong Y, et al. Transcriptome profiling analysis of the testis after eyestalk ablation for selection of the candidate genes involved in the Male sexual development in Macrobrachium nipponense. Front Genet (2021) 12:675928. doi: 10.3389/fgene.2021.675928
16. Sagi A, Cohen D, Wax Y. Production of Macrobrachium rosenbetgii in momosex population: Yield characteristes under intensive monoculture conditions in cages. Aquaculture (1986) 51:265–75. doi: 10.1016/0044-8486(86)90318-2
17. Sagi A, Cohen D, Milner Y. Effect of androgenic gland ablation on morphotypic differentiation and sexual characteristics of male freshwater prawns Macrobrachium rosenbergii. Gen Comp Endocr (1990) 77:15–22. doi: 10.1016/0016-6480(90)90201-V
18. Ventura T, Manor R, Aflalo ED, Weil S, Rosen O, Sagi A. Timing sexual differentiation: full functional sex reversal achieved through silencing of a single insulin-like gene in the prawn, Macrobrachium rosenbergii. Biol Reprod (2012) 86(3):90. doi: 10.1095/biolreprod.111.097261
19. Li SH, Li FH, Sun Z, Xiang JH. Two spliced variants of insulin-like androgenic gland hormone gene in the Chinese shrimp, Fenneropenaeus chinensis. Gen Comp Endocrinol (2012) 177(2):246–55. doi: 10.1016/j.ygcen.2012.04.010
20. Huang XS, Ye HH, Huang HY, Yang YN, Gong J. An insulin-like androgenic gland hormone gene in the mud crab, Scylla paramamosain, extensively expressed and involved in the processes of growth and female reproduction. Gen Comp Endocrinol (2014) 204:229–38. doi: 10.1016/j.ygcen.2014.06.002
21. Liu F, Shi W, Ye H, Liu A, Zhu Z. RNAi reveals role of insulin-like androgenic gland hormone 2 (IAG2) in sexual differentiation and growth in Hermaphrodite shrimp. Front Mar Sci (2021) 8:666763. doi: 10.3389/fmars.2021.666763
22. Ma KY, Li JL, Qiu GF. Identification of putative regulatory region of insulin-like androgenic gland hormone gene (IAG) in the prawn Macrobrachium nipponense and proteins that interact with IAG by using yeast two-hybrid system. Gen Comp Endocr (2016) 229:112–8. doi: 10.1016/j.ygcen.2016.03.019
23. Zhou TT, Wang W, Wang CG, Sun CB, Shi LL, Chan SF. Insulin-like androgenic gland hormone from the shrimp Fenneropenaeus merguiensis: Expression, gene organization and transcript variants. Gene (2021) 782:145529. doi: 10.1016/j.gene.2021.145529
24. Kamb AA. Cell cycle regulator potentially involved in genesis of many tumour types. Trend Genet (1994) 10:228. doi: 10.1016/0168-9525(94)90162-7
25. Spellman PT, Sherlock G, Zhang MQ, Iyer VR, Anders K, Eisen MB, et al. Comprehensive identification of cell cycle-regulated genes of the yeast saccharomyces cerevisiae by microarray hybridization. Mol Biol Cell (1998) 9:3273–97. doi: 10.1091/mbc.9.12.3273
26. Glover DM, Hagan IM, Tavares AA. Polo-like kinases: a team that plays throughout mitosis. Genes. Dev (1998) 12:3777–87. doi: 10.1101/gad.12.24.3777
27. Nigg EA. Polo-like kinases: positive regulators of cell division from start to finish. Curr Opin Cell Biol (1998) 10:776–83. doi: 10.1016/S0955-0674(98)80121-X
28. Archambault V, Glover DM. Polo-like kinases: conservation and divergence in their functions and regulation. Nat Rev Mol Cell Biol (2009) 10:265–75. doi: 10.1038/nrm2653
29. Takahashi T, Sano B, Nagata T, Kato H, Sugiyama Y, Kunieda K, et al. Polo-like kinase 1 (PLK1) is overexpressed in primary colorectal cancers. Cancer Sci (2003) 94(2):148–52. doi: 10.1111/j.1349-7006.2003.tb01411.x
30. Garland LL, Taylor C, Pilkington DL, Cohen JL, Hoff DDV. A phase I pharmacokinetic study of HMN-214, a novel oral stilbene derivative with polo-like kinase-1-interacting properties, in patients with advanced solid tumors. Clin Cancer Res (2006) 12(17):5182–9. doi: 10.1158/1078-0432.CCR-06-0214
31. Rödel F, Keppner S, Capalbo G, Bashary R, Kaufmann M, Rödel C, et al. Polo-like kinase 1 as predictive marker and therapeutic target for radiotherapy in rectal cancer. Am J Pathol (2010) 177(2):918–29. doi: 10.2353/ajpath.2010.100040
32. Bonner AM, Hughes SE, Hawley RS. Regulation of polo kinase by matrimony is required for cohesin maintenance during drosophila melanogaster female meiosis. Curr Boil. (2020) 30(4):715–22. doi: 10.1016/j.cub.2019.12.027
33. Long T, Cailliau K, Beckmann S, Browaeys E, Trolet J, Grevelding CG, et al. Schistosoma mansoni polo-like kinase 1: A mitotic kinase with key functions in parasite reproduction. Int J Parasitol (2010) 40(9):1075–86. doi: 10.1016/j.ijpara.2010.03.002
34. Zhang XL, Cui LF, Li SM, Liu XZ, Han X, Jiang KY, et al. Bureau of fisheries, ministry of agriculture, P.R.C. fisheries economic statistics. In: China Fishery yearbook, vol. 24. Beijing: Beijing China Agricultural Press (2020).
35. Jin SB, Zhang Y, Guan HH, Fu HT, Jiang SF, Xiong YW, et al. Histological observation of gonadal development during post-larva in oriental river prawn, Macrobrachium nipponense. Chin J Fish (2016) 29(4):11–6. doi: 10.3969/j.issn.1005-3832.2016.04.003
36. Li M, Zhang F, Ding J, Zuo R, Chang Y. Effects of lipid sources on the growth performance, gonad development, fatty acid profile and transcription of related genes in juvenile sea urchin (Strongylocentrotus intermedius). Aquacult Nutr (2020) 27(1):28–38. doi: 10.1111/anu.13162
37. Park I, Nam YK, Dong SK. Growth performance, morphometric traits and gonad development of induced reciprocal diploid and triploid hybrids between the mud loach (Misgurnus mizolepis günther) and cyprinid loach (Misgurnus anguillicaudatus cantor). Aquac Res (2006) 37(12):1246–53. doi: 10.1111/j.1365-2109.2006.01556.x
38. Feng WR, Feng W, Ge JC, Li JL, Su SY, Jia R. Alterations of amino acid metabolism and intestinal microbiota in Chinese mitten crab (Eriocheir sinensis) fed on formulated diet and iced trash fish. Comp Biochem Phys D (2021) 40:100924. doi: 10.1016/j.cbd.2021.100924
39. Jin SB, Jiang SF, Xiong YW, Qiao H, Sun SM, Zhang WY, et al. Molecular cloning of two tropomyosin family genes and expression analysis during development in oriental river prawn, Macrobrachium nipponense. Gene (2014) 546(2):390–7. doi: 10.1016/j.gene.2014.05.014
40. Jin SB, Fu HT, Jiang SF, Xiong YW, Sun SM, Qiao H, et al. Molecular cloning, expression, and In situ hybridization analysis of forkhead box protein L2 during development in Macrobrachium nipponense. J World Aquacul. Soc (2018) 49(10):429–40. doi: 10.1111/jwas.12510
41. Hu YN, Fu HT, Qiao H, Sun SM, Zhang WY, Jin SB, et al. Validation and evaluation of reference genes for quantitative real-time PCR in Macrobrachium nipponense. Int J Mol Sci (2018) 19:2258. doi: 10.3390/ijms19082258
42. Livak KJ, Schmittgen TD. Analysis of relative gene expression data using real-time quantitative PCR and the 2–ΔΔCT method. Methods (2001) 25:402–8. doi: 10.1006/meth.2001.1262
43. Zhang SB, Jiang P, Wang ZQ, Long SR, Liu RD, Zhang X, et al. Dsrna-mediated silencing of nudix hydrolase in trichinella spiralis inhibits the larval invasion and survival in mice. Exp Parasitol (2016) 162:35–42. doi: 10.1016/j.exppara.2016.01.005
44. Jiang FW, Fu HT, Qiao H, Zhang WY, Jiang SF, Xiong YW, et al. The RNA interference regularity of transformer-2 gene of oriental river prawn Macrobrachium nipponense. Chin Agricul. Sci Bul. (2014) 30(32):32–7.
45. Ma XK, Liu XZ, Wen HS, Xu YJ, Zhang LJ. Histological observation on gonadal sex differentiation in Cynoglossus semilaevis günther. Mar Fish. Res (2006) 27(2):55–61. doi: CNKI:SUN:HYSC.0.2006-02-007
46. ShangGuan BM, Liu ZZ, Li SQ. Histological studies on ovarian development in Scylla serrata. J Fish. Sci China (1991) 15(2):96–103.
47. Mundt KE, Golsteyn RM, Lane HA, Nigg EA. On the regulation and function of human polo-like kinase 1 (PLK1): effects of overexpression on cell cycle progression. Biochem Bioph Res Co (1997) 239(2):377–85. doi: 10.1006/bbrc.1997.7378
48. Jin SB, Hu YN, Fu HT, Jiang S, Xiong Y, Qiao H, et al. Analysis of testis metabolome and transcriptome from the oriental river prawn (Macrobrachium nipponense) in response to different temperatures and illumination times. Comp Biochem Phys D (2020) 34:100662. doi: 10.1016/j.cbd.2020.100662
49. Jin S, Zhang W, Xiong Y, Jiang SF, Qiao H, Gong YS, et al. Genetic regulation of male sexual development in the oriental river prawn Macrobrachium nipponense during reproductive vs. non-reproductive season. Aquacult Int (2022) 30:2059–79. doi: 10.1007/s10499-022-00887-7
50. Zhang YL, Fan Y, Wu Y, Zhang YC, Zhang W. Effects of polo-like kinase-1 gene silence by RNA interference on proliferation of human pancreatic cancer cells. World Chin J Digestol (2007) 15(3):3484–8. doi: 10.3969/j.issn.1009-3079.2007.33.006
51. Ni GH, Fan Y, Chen J, Qian LP, Lin GJ, Chen GX, et al. Effects of RNA interference polo-like kinase-1 gene on telomerase activity of human colon cancer cell. Fudan Uni J Med Sci (2009) 36(1):14–8. doi: CNKI:SUN:SHYK.0.2009-01-005
52. Jin S, Hu Y, Fu H, Jiang S, Xiong Y, Qiao H, et al. Identification and characterization of the pyruvate dehydrogenase E1 gene in the oriental river prawn, Macrobrachium nipponense. Front Endocrinol (2021) 12:752501. doi: 10.3389/fendo.2021.752501
Keywords: Macrobrachium nipponense, polo-like kinase 1, RNAi analysis, histological observations, testis development
Citation: Jin S, Zhang W, Wang P, Jiang S, Qiao H, Gong Y, Wu Y, Xiong Y and Fu H (2022) Identification of potential functions of polo-like kinase 1 in male reproductive development of the oriental river prawn (Macrobrachium nipponense) by RNA interference analysis. Front. Endocrinol. 13:1084802. doi: 10.3389/fendo.2022.1084802
Received: 31 October 2022; Accepted: 17 November 2022;
Published: 05 December 2022.
Edited by:
Ardavan Farhadi, Hainan University, ChinaReviewed by:
Alejandra García-Gasca, Centro de Investigación en Alimentación y Desarrollo, Consejo Nacional de Ciencia y Tecnología (CONACYT), MexicoApinunt Udomkit, Mahidol University, Thailand
Copyright © 2022 Jin, Zhang, Wang, Jiang, Qiao, Gong, Wu, Xiong and Fu. This is an open-access article distributed under the terms of the Creative Commons Attribution License (CC BY). The use, distribution or reproduction in other forums is permitted, provided the original author(s) and the copyright owner(s) are credited and that the original publication in this journal is cited, in accordance with accepted academic practice. No use, distribution or reproduction is permitted which does not comply with these terms.
*Correspondence: Yiwei Xiong, xiongyw@ffrc.cn; Hongtuo Fu, fuht@ffrc.cn
†ORCID: Yiwei Xiong, orcid.org/000-0001-5644-213x
Hongtuo Fu, orcid.org/000-0003-2974-9464