- 1Shandong Key Laboratory of Mental Disorders, Department of Anatomy and Neurobiology, Shandong University, Jinan, Shandong, China
- 2Department of Neurosurgery, Laizhou City People’s Hospital, Laizhou, Shandong, China
- 3Department of Psychiatry, Shandong Provincial Mental Health Center, Jinan, Shandong, China
- 4Department of Anatomy, Changzhi Medical College, Changzhi, Shanxi, China
- 5School of Basic Medical Sciences, Cheeloo College of Medicine, Shandong University, Jinan, Shandong, China
Premenstrual syndrome (PMS), a recurrent and moderate disorder that occurs during the luteal phase of the menstrual cycle and quickly resolves after menstruation, is characterized by somatic and emotional discomfort that can be severe enough to impair daily activities. Current therapeutic drugs for PMS such as selective serotonin reuptake inhibitors are not very satisfying. As a critical pineal hormone, melatonin has increasingly been suggested to modulate PMS symptoms. In this review, we update the latest progress on PMS-induced sleep disturbance, mood changes, and cognitive impairment and provide possible pathways by which melatonin attenuates these symptoms. Moreover, we focus on the role of melatonin in PMS molecular mechanisms. Herein, we show that melatonin can regulate ovarian estrogen and progesterone, of which cyclic fluctuations contribute to PMS pathogenesis. Melatonin also modulates gamma-aminobutyric acid and the brain-derived neurotrophic factor system in PMS. Interpreting the role of melatonin in PMS is not only informative to clarify PMS etiology but also instructive to melatonin and its receptor agonist application to promote female health. As a safe interaction, melatonin treatment can be effective in alleviating symptoms of PMS. However, symptoms such as sleep disturbance, depressive mood, cognitive impairment are not specific and can be easily misdiagnosed. Connections between melatonin receptor, ovarian steroid dysfunction, and PMS are not consistent among past studies. Before final conclusions are drawn, more well-organized and rigorous studies are recommended.
Introduction
Premenstrual syndrome (PMS) is a kind of neuroendocrine disorder that threatens women’s physical and mental health. It is estimated that more than half of women complain of somatic or emotional discomfort during the luteal phase of the menstrual cycle. In general, these symptoms are mild, and some physical disturbances, such as abdominal swelling and breast tenderness, and mood changes, such as irritability and anxiety, are commonly reported (1–3). However, approximately 5.3% of women experience distress that is too severe, such as insomnia, depression and cognitive impairment, to accomplish daily activities (Table 1). This severe form of PMS is defined as premenstrual dysphoric disorder (PMDD) (5). Although the etiology of PMS is not fully illustrated, ovarian hormone fluctuations are clearly associated with PMS, as the symptoms are only observed in the luteal phase and reduced after menstruation. Consequently, in recent years, dysfunctional reproductive hormone levels and their effects on the brain neurotransmitter system have been regarded as key factors in PMS pathogenesis (5, 14, 15). In line with this notion, ovarian cyclicity disruption and brain neurotransmitter regulation have been developed as treatment methods for PMS. Serotonin reuptake inhibitors (SSRIs) are recommended as the first-line therapy for PMDD management (5). However, first-line medications fail to completely relieve symptoms in almost 75% of PMDD patients. As a result, other therapeutic drugs with proof of molecular mechanisms and clinical trials are needed (2). To our knowledge, the therapeutic effect of two or more medicine combinations is always better than that of one medicine alone. For example, oral contraceptives, such as ethinylestradiol drospirenone, can further improve the management of symptoms other than depressive symptoms by suppressing the hypothalamic-pituitary-ovarian axis in PMS and PMDD (16). Ulipristal acetate, a selective progesterone receptor modulator, may be the key to alleviating psychological symptoms such as depression (17).
Melatonin is produced from the pineal gland and serves as an internal synchronizer under the tight control of the central circadian timing system (internal clock) located in the suprachiasmatic nucleus (SCN) within the anterior hypothalamus (18). Three decades ago, Parry and his colleagues found a phase-advanced offset of melatonin secretion in PMS (19). A subsequent study demonstrates an altered phase-shift response of melatonin to light in PMDD patients, whereas the suppressive effects of light on melatonin between PMDD and healthy women are similar, indicating the contribution of circadian clock dysfunction in PMDD (20, 21). Melatonin also reciprocally modulates the circadian clock via the melatonin receptors MT1 and MT2 (22, 23). In addition, several studies also report that nocturnal melatonin changes are accompanied by sleep, emotion and ovarian hormone alterations and that an exogenous supplement of melatonin can partially correct them (24, 25). More importantly, progressive advances have shown melatonin’s improvement of reproductive functions and neuroprotection (26, 27). These studies suggest that blunted circadian melatonin might contribute to PMS and imply a potential therapeutic effect.
In this article, we review the latest literature on PMS and melatonin and their potential relationships from both behavioral and molecular perspectives. Regarding behavioral aspects, accumulating studies have demonstrated that melatonin has an antiPMS function in cell models, animal models and humans (Figure 1). Regarding molecular mechanisms, we focused on circadian genes (28), proinflammatory cytokines (IL-6, IL-1β, TNF-α, IFN-γ, and mTOR) (28–30), enzyme activity (31), ovarian hormone estrogen and progesterone (32, 33), gamma-amino butyric acid (GABA) and brain-derived neurotrophic factor (BDNF) alterations in PMS (34, 35) (Figure 2, Tables 2, 3). This work attempts to unravel the enigma of how melatonin modulates PMS symptoms through these molecule-related pathways, which may provide a theoretical foundation for melatonin-targeted treatment in PMS and PMDD.
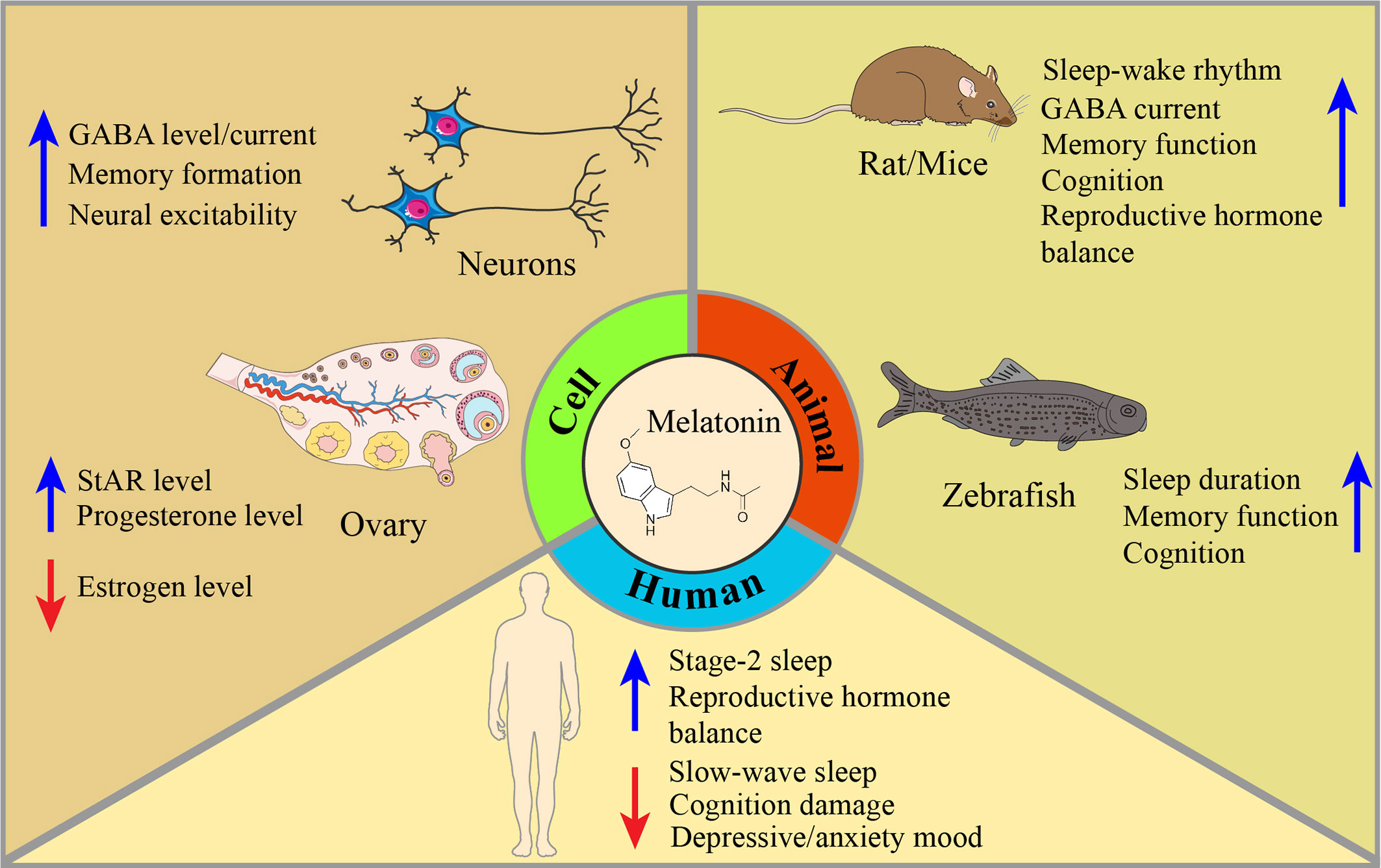
Figure 1 Mechanisms of melatonin restoration in PMS/PMDD-related symptoms, given cells, animals, and humans. Melatonin is involved in many aspects of PMS/PMDD. In cells, melatonin can upregulate GABA levels and its current among neurons, promote memory formation, and increase neuron excitability. To adjust the imbalance of the ovary’s hormones, melatonin can increase the levels of StAR and progesterone, and decrease the level of estrogen. In animals, dysfunction of sleep-wake rhythm, GABA current, memory function, cognition, and reproduction hormone balance have been modulated by melatonin intake. Meanwhile, melatonin’s improvement of sleep, memory, and cognition is also discovered in the zebrafish model. In humans, the effect of melatonin has long been applied in improving sleep quality. In PMS/PMDD-related symptoms, melatonin can restore stage-2 sleep and reproductive hormone balance and reduce slow-wake sleep, cognitive damage, and depressive/anxiety mood derived from PMS/PMDD.
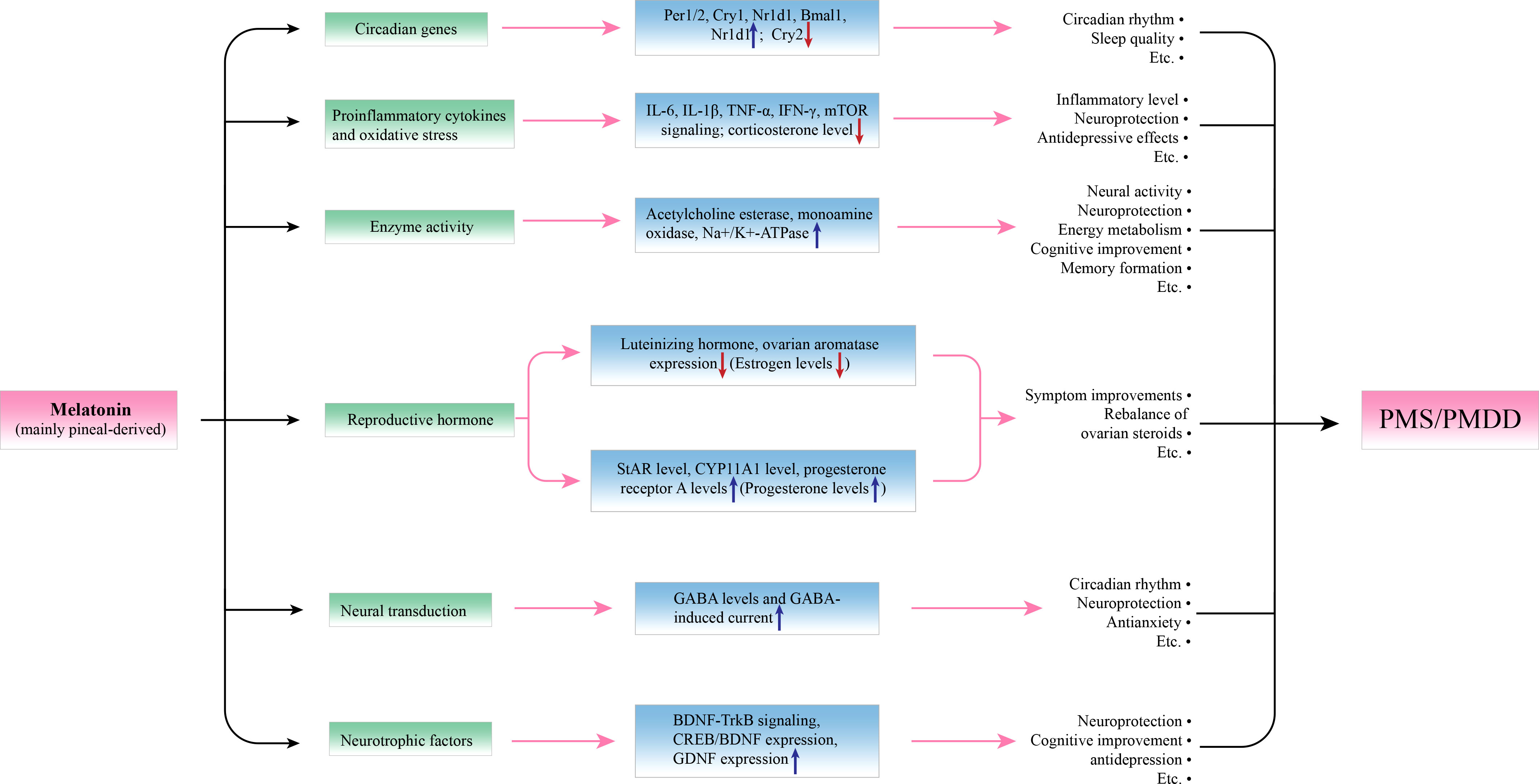
Figure 2 Potential molecular actions of melatonin in modulating premenstrual symptoms. By affecting the expression of relevant molecules, and melatonin can achieve its protective effects in PMS. Per1/2, Cry1/2, and Nr1d1 are circadian genes, melatonin can upregulate their expression to improve circadian rhythm and sleep quality. IL-6, IL-1β, TNF-α, IFN-γ, and mTOR signaling pathways and increased corticosterone levels participate in the inflammation and oxidative stress of PMS, and melatonin can downregulate these processes to play a role in neuroprotection and antidepression. Regarding enzyme activity, melatonin can upregulate the activity of acetylcholine esterase, monoamine oxidase, and Na+/K+-ATPase to promote neural activity, energy metabolism, cognition, and so on. An imbalance of reproductive hormone is seen as a pathogenesis of PMS, melatonin can upregulate the levels of StAR, CYP11A1, and progesterone receptor A and downregulate the levels of luteinizing hormone and ovarian aromatase to reverse the imbalance of the estrogen/progesterone ratio. Moreover, melatonin can promote neural transduction by increasing GABA levels and GABA-induced current, which is associated with better circadian rhythm, neuroprotection, and antianxiety effects. In addition, melatonin can act as a neurotrophic promoter by upregulating the BDNF-TrkB signaling pathway and the expression of CREB/BDNF and GDNF.
Melatonin attenuates PMS-induced sleep disturbance
Sleep disturbance in PMS
PMS women with sleep problems are commonly characterized by sleep-wake rhythm shifts, subjective sleep disturbance, and sleep electroencephalogram trait variations. In a clinical case report, patients with PMS show a delayed sleep rhythm phase in the luteal phase of the menstrual cycle, whereas the phase is advanced in the follicular phase (71). In addition, PMS-related sleep performance can be unrefreshing and insufficient, which causes daytime sleepiness (72, 73). Although the exact mechanism behind PMS and the sleep-wake cycle is still unknown, elevated progesterone levels during the luteal phase might play a key role since they can induce an increase in body temperature, which will ultimately lead to more fragmented sleep (74, 75).
Sleep disturbances during the luteal phase are often a complaint in women with PMS, including poor sleep, insomnia symptoms, and daytime sleepiness (76, 77) (Table 1). In a study of 127 medical students with PMS, 96 of them (75.6%) struggled with decreased sleep quality (78). A disrupted circadian rhythm and decreased melatonin can be found in sleep disorders (59). However, diseases such as infertility, polycystic ovary syndrome and dysfunction of the hypothalamic-pituitary-ovarian axis, and even an irregular menstrual cycle can also result in sleep disturbances and self-reported poor sleep since dysmenorrhea and accompanying mood changes (4). In a recent survey investigating the association between menstruating women and sleep quality, PMS patients shows sleep duration declines, and menstruating problems are associated with a higher incidence of insomnia and daytime sleepiness (79). As a result, sleep dysfunction is not limited to PMS, and the assessment of healthy people during the menstrual phase and criteria for those defined as PMS should be considered carefully.
Despite tremendous reports of poorer subjective sleep qualities in the late-luteal phase, women with PMS suggest few objective sleep quality alterations by polysomnographic and quantitative electroencephalogram (EEG) measures. In most cases, objective parameter changes are only found between the follicular and luteal phases. When compared to the follicular phase, shorter rapid eye movement (REM) latency and REM episodes are found during the luteal phase in both PMS patients and controls (80, 81). Interestingly, PMDD patients with depressed mood have EEG patterns similar to those of healthy women but distinguish from the EEG architecture in major depressive disorder (82, 83). Taken together, we mention 2 points here and call on more studies to get in. On the one hand, it is suggested that the absence of altered actual sleep may be a peculiarity in PMS patients with poorer perceived sleep quality. On the other hand, mood changes may not be the only contributor to PMS-induced sleep disturbance; other factors, such as circadian rhythm and melatonin dysfunction can also play a critical role.
Melatonin restores the altered circadian clock
From the viewpoint of circadian rhythm, PMS-induced sleep disturbance is a reflection of sleep-wake cycle disorder, which can also impact other biological rhythms. In return, the effects of the circadian system on sleep are well recognized, and internal clock impairment is a leading cause of circadian rhythm sleep disorders (CRSDs) (84). Nevertheless, research on the bidirectional relationship between circadian rhythm and sleep in PMS is not sufficient. Shinohara and his colleagues reported body temperature and sleep rhythm changes in a woman with PMS, indicating circadian rhythm and sleep alterations underlying PMS (71). In a laboratory-based study, sleep durations showed consistency with circadian variation (85). A recent cross-sectional study of university students, 78% of whom report social jet lag (different sleep patterns between weekends and school days), shows that circadian system misalignment due to sleep disturbance is significantly associated with menstrual symptoms (86). A reduction in REM sleep sensitive to menstrual phase changes was also found. These findings, although not direct, imply the attribution of circadian rhythm disorder to PMS-induced sleep disturbance.
In PMS, melatonin levels are decreased at both the follicular and luteal phases (24, 87). In the normal menstrual cycle, no alternation is observed in slow wave sleep (SWS). Melatonin secretion appears to be stable during the two menstrual phases. However, in women with PMDD, increased SWS, prolonged objective sleep onset latency, and reduced stage 2 sleep are functionally associated with reduced melatonin levels, and exogenous melatonin can reverse these changes and improve sleep quality (25, 88). These studies provide direct evidence of a potential interaction between melatonin and PMS-induced sleep problems. Herein, although accumulative studies have shown alterations in stage 2 sleep, SWS and REM sleep, blunted melatonin and circadian temperature rhythm in PMS, the exact mechanism of disrupted circadian clock and melatonin secretion in PMS-related sleep problems has not been elucidated.
Melatonin regulates sleep by targeting the circadian system
Recently, melatonin has been suggested to play a role in sleep circadian regulation and sleep disorder treatment. In zebrafish, melatonin is required for sleep regulation, and sleep duration is significantly reduced by blocking melatonin synthesis (36). Regarding human beings, clinical meta-analyses have shown the therapeutic effects of melatonin for patients with first-degree and secondary sleep disorders (89, 90). Specifically, in CRSDs, melatonin can accelerate entrainment in jet lag, advance phases of circadian rhythms in delayed sleep phase syndrome and increase daytime sleep in shift-work sleep disorder (37). Some melatonin receptor agonists, such as tasimelteon, have already been approved for the treatment of CRSDs (91, 92). These findings provide solid evidence of melatonin’s role in regulating sleep disorders, especially those related to circadian system interruption (Table 2).
Melatonin’s effects on sleep disturbance can be found in PMS and other diseases that need differentiation like primary sleep disorder, polycystic ovary syndrome, and primary insomnia (93, 94). For example, an exogenous supply of melatonin can improve sleep quality among women with PMDD (25). Patients with polycystic ovary syndrome, a female reproductive disease with sleep problems, show significantly increased sleep quality after 12 weeks of melatonin supplementation (95). In insomnia, one of the major complaints in women with PMS, melatonin administration can be adopted as a safe adjuvant therapy (96). The therapeutic effects of melatonin may involve the modulation of the internal clock system, as the clock 3111T/C gene polymorphism is associated with altered melatonin levels in women with insomnia (97).
Melatonin’s effects on sleep are diverse depending on the distinct functions of melatonin receptors including MT1 and MT2 (98, 99) (Table 2). In MT1 receptor knockout mice, reduced REM sleep and significantly increased non-REM (NREM) sleep are found whereas MT2 receptor knockout mice present a decrease in NREM sleep (38, 39, 100). In addition, distinct localizations of MT1 (mainly on REM-related regions such as the lateral hypothalamus) and MT2 (mainly on NREM-related areas such as the reticular thalamus) receptors also suggest that melatonin plays receptor-specific roles in sleep regulation (101). Moreover, the MT1 receptor can elevate the amplitude of the internal clock to induce the switch from wake to sleep, and the MT2 receptor may be helpful in synchronizing the sleep cycle to the circadian clock (102). However, the distribution of melatonin receptors has not been elucidated in PMS/PMDD. This evidence provides a molecular basis for melatonin, sleep and circadian clock interactions. Given the circadian rhythm changes in PMS, targeting melatonin and its receptors may relieve PMS-induced sleep problems. Well-conducted and large clinical trials are needed. Relevant mechanisms should also be further explored.
Melatonin improves mood and cognitive function
Melatonin improves depressed mood
PMS and PMDD patients are characterized by affective disorders such as anxiety and depression. In particular, psychological discomforts such as depression can be the major complaint in women with PMDD (103). As a result, alleviating emotional symptoms is crucial in PMS. Currently, anti-depressants, selective serotonin reuptake inhibitors (SSRIs), have been regarded as the preferred choice in PMS/PMDD treatment (5). However, the dose-dependency and tolerant peculiarities of traditional antidepressants limit their extensive clinical application (104). In recent years, melatonin has increasingly been suggested to regulate emotional symptoms in PMS/PMDD. In PMDD patients, altered circadian rhythms of melatonin are associated with depressed mood (24, 25, 87) (Table 2). In a double-blind study, melatonin administration attenuated premenstrual-like symptoms (41). The authors also found that women treated with melatonin exhibited less depression, anxiety, anger, and fatigue than the placebo control group. Some melatonin receptor agonists, such as agomelatine and ramelteon, have been used as novel anti-depressants and have emerged as promising prospects in depression treatment (105, 106). Together, these studies demonstrate melatonin’s regulation of negative mood, providing a basis for melatonin-mediated emotional changes in PMS.
As the synchronizer of melatonin rhythm, the circadian clock is also involved in emotion regulation. To date, major depressive disorder, bipolar disorder and other affective disorders have been associated with a dysfunctional internal clock system (107–109), which might be attributed to clock gene changes. In a mouse model of depression, Period1(Per1) levels are positively correlated with the severity of depression (110). Per2, brain and muscle ARNT-like 1(Bmal1) and nuclear receptor subfamily 1 group D member 1(Nr1d1) changes also induce depression-like behaviors via inflammation-related processes (57, 111, 112). However, whether the circadian gene Nr1d1 is associated with depressive changes, especially in females, is not fully understood (58, 111, 113). In cryptochrome circadian regulator 1/2(Cry1/2) knockout mice, elevated anxiety levels are observed compared to those in wild-type mice (114). Given that early wake therapy can improve depressive moods without melatonin alternation and that the suppression effects of light on melatonin are compatible between PMDD and healthy people (21, 87), the circadian system and its coupling pathway dysfunction may be included in the etiology of PMS mood disorders.
Apart from its direct anti-anxiety/depression effects, melatonin can also regulate core circadian genes involved in emotional disorders (Table 3). For instance, melatonin increases Per2, Cry1, and Cry2 expression within the anterior pituitary of rats (115). Phase-delayed Per1, Per2, and Cry1 levels are concomitantly reported after melatonin treatment. Moreover, in a seasonal affective disorder mouse model, melatonin supplementation elevates the rhythm amplitudes of Per1, Per2, and Bmal1 in the SCN (116). In addition, scientists have found that melatonin receptor agonists relieved patients’ depression and anxiety with decreased Per1, Per2, Cry1, Cry2 and Nr1d1 expression (28). Melatonin can upregulate Bmal1 level and promote cellular survival by PI3K/AKT signaling pathways (60). These results suggest that melatonin-induced clock gene alterations may be another potential mechanism in PMS-related affective complaints. However, there is still inconsistency inconsistence with limited recognition of the underlying pathways that govern them.
Melatonin alleviates cognitive impairment
PMS patients often complain of cognitive-related problems in the luteal phase, such as affective lability and a sense of being controlled or overwhelmed. In major depressive disorder, cognitive deficits are proverbially recognized (117), indicating potential cognitive dysfunction in PMS and PMDD. Keenan etal. (6) employed the Trail Making Test B to assess attention capacity differences between PMS and heathy women, and they found that PMS patients exhibited worse performance in the Trails B task during the luteal phase. A recent study compared working memory discrepancies in PMS participants during the follicular phase, which were detected through the N-back task (7). The results suggest that poorer working memory is correlated with increased PMS severity. In PMDD studies, women display poorer N-back task performance in the luteal phase, which is also correlated with PMDD severity, irritability, and functional impairment (118). Admittedly, current studies on PMS/PMDD cognitive alterations are not consistent (119), and some studies fail to report cognitive impairment, especially in PMS patients, which may be due to the decreased severity compared to PMDD. Further studies on cognitive changes in PMS and PMDD should be conducted.
The potential mechanisms of PMS-induced cognitive damage may be attributed to a negative emotional state and dysregulated ovarian steroid secretion. A substantial body of literature has shown that negative moods such as anxiety and depression can result in cognitive impairment (120). Given the characterized affective dysfunction in PMS/PMDD, anti-depression therapy may attenuate cognitive symptoms. Regarding ovarian hormones, estrogen supplementation enhances memory task performance, while progesterone protects cognition after brain injury (121, 122). In addition, estrogen and progesterone receptors are also distributed in brain cognition-related regions, such as the prefrontal cortex and hippocampus. In summary, current studies have demonstrated the emotion and hormone regulation of cognition, although it is plausible whether mood and steroids contribute to cognitive disorders in PMS/PMDD.
Melatonin plays an important role in cognitive regulation (Table 2). In zebrafish, melatonin treatment mitigates cognitive disorder, which results from altered circadian rhythm (47). In a 5-fluorouracil-induced cognitive deficits model, melatonin administration reversed rat spatial memory dysfunction (46). Similar effects of melatonin were discovered in an isoflurane-induced mouse model, and the improved cognitive function after melatonin treatment may be mediated through circadian clock resynchronization (29, 123). In clinical studies, melatonin alleviates cognitive disturbance resulting from breast cancer chemotherapy (124)). Recent advances have regarded melatonin as an index of cognitive impairment in elderly individuals (125). Moreover, melatonin has been shown to effectively improve cognitive deficits in AD mouse models (126). These studies from animals to humans demonstrate a direct regulation of cognition by melatonin. In particular, melatonin has been suggested to play a role in cognition through the regulation of circadian hormones such as estrogen. For instance, nonylphenol, an estrogen mimic, can induce cognitive impairment in Wistar rats, whereas melatonin treatment attenuates its neurotoxicity and adverse cognitive impact (48). Together, potential mechanisms by which melatonin can regulate emotion through circadian clock-dependent or clock-independent pathways have emerged, which may be a possible approach to melatonin regulation of PMS/PMDD-induced cognitive impairments.
Melatonin adjusts ovarian hormone levels
Decreased estrogen and elevated progesterone
PMS symptoms cyclically occur in the luteal phase and gradually vanish after menstruation, indicating the role of the menstrual cycle in PMS occurrence. In line with this viewpoint, one of the most effective treatments for PMS is ovulation suppression, and the combination of estrogen and progestogen shows a promising effect on attenuating PMS symptoms (9). ESC/E(Z) complex genes and ovarian hormone regulation genes also manifest different expression levels in PMDD patients and controls (8). With the changes in ovarian steroids and the subsequent resting regional cerebral blood flow in PMDD, ESC/E(Z) genes seem to have a greater correlation with brain function and more attention should be given (127). More importantly, extensive studies have demonstrated the effects of estrogen and progestogen on depression, anxiety and other emotional disorders in women (128, 129). As a result, ovulation-related hormones, especially estrogen and progestogen, should be considered key factors in the pathogenesis of PMS.
During the menstrual cycle, estrogen and progestogen showed distinct secretion patterns. After ovulation, estrogen levels have a slight drop and last for 1-2 days. Subsequently, both estrogen and progesterone are increased and peak in the mid-luteal phase and are significantly reduced to their lowest levels before menstruation (130). However, it seemed that the levels of ovarian hormones in PMS patients were not similar to those in normal people. Decreased estrogen and elevated progesterone levels may be characteristic of PMS, albeit with inconsistent findings. Recently, Yen etal. (131) thoroughly explored estrogen and progesterone levels in women with PMDD. They found that PMDD patients have decreased estrogen levels in the luteal phase. Moreover, in women with low estrogen levels, elevated progesterone levels are reported in PMDD patients rather than healthy controls. Other independent studies have also confirmed the role of higher progesterone and lower estrogen in PMDD (132, 133). In addition, ovarian steroid fluctuations indicate their interactions with PMDD symptoms. In premenstrual women, more severe premenstrual symptoms are correlated with a reduction in ovarian estrogen and progesterone levels (134). The estrogen and progesterone changes from low to peak are also associated with PMDD onset (135). Remarkably, estrogen receptor alpha (ERα) has gradually received wide attention in PMDD emotional symptoms. Scientists have discovered associations between ERα single nucleotide polymorphisms, the risk of PMDD and patient psychological traits (136–138). The ESR α-Xbal polymorphism also suggests its modulation of PMDD patient emotion (139). In conclusion, these studies demonstrate ovarian hormone-related changes in PMS and indicate their contribution to PMS symptoms from various perspectives. Notably, the production of reproductive hormones involves extensive sophisticated mechanisms and is under the control of the neuroendocrine system. As a result, ovarian hormone-targeted treatment does not simply correct the abnormalities in estrogen and progesterone levels, and hypothalamus-pituitary-gonadal cyclicity and circadian rhythms should also be considered.
Apart from estrogen and progesterone, PMS also induces oscillations of other endocrine factors whose secretion is dominated by hypothalamic–pituitary axes and regulated by the internal clock, such as cortisol, luteinizing hormone (LH), and follicle-stimulating hormone (FSH). Interestingly, estrogen and progesterone may also potentially contribute to these hormone circadian changes. Estrogen is known for its negative regulation of LH and FSH. Allopregnanolone, a metabolite of progesterone, is elevated in PMDD patients with decreased cortisol levels, implying the control of progesterone in cortisol diurnal secretion (140). Although there is no consensus on cortisol circadian rhythm dysfunction, the cortisol awakening response is significantly attenuated in PMS women, suggesting hypoactivity in the hypothalamus-pituitary axis (141, 142). Other hormone circadian changes are also observed in PMS patients, including elevated mean FSH, advanced FSH rhythms and reduced amplitude of LH pulses in the luteal phase of menstruation (143, 144). In summary, changes in estrogen and progesterone seem unpredictable among PMS/PMDD patients. Studies on upstream hormones of ovarian steroids may help us to understand the mechanisms of PMS/PMDD.
Melatonin ameliorates the change in ovarian steroids
Accumulating studies have demonstrated the role of melatonin in ovarian physiology and hormone regulation. In addition to the pineal gland, melatonin is also produced by granulosa cells, the cumulus oophorus, and oocytes, leading to higher concentrations of melatonin in follicular fluid than in serum, which may correlate with estradiol and progesterone levels (145). To date, melatonin has been shown to regulate estrogen and progesterone (Table 2). Normally, decreased estrogen and increased progesterone levels are commonly reported after melatonin interference (146, 147), which can exert its actions through the regulation of enzymes participating in steroid synthesis. Martínez-Campa and his colleagues found that melatonin inhibits aromatase cytochrome p450 (CYP19) mRNA expression, preventing aromatase-induced estrogen production (32). Under melatonin deprivation (pinealectomy), the expression of CYP17A1, a crucial enzyme in estrogen synthesis, is significantly increased in mice (61). However, melatonin treatment promotes steroidogenic acute regulatory protein (StAR) and CYP11A1 expression in pregnant mice, which catalyzes the synthesis of progesterone (31). Moreover, a recent in vitro study also revealed that StAR levels are upregulated after melatonin treatment, leading to elevated progesterone production (33). On the other hand, melatonin can also serve as an estrogen and progesterone receptor modulator. In rats, melatonin treatment contributes to an increase in progesterone receptor A and a decrease in ERα (49). These effects may be mediated via the MT1 receptor, as it is significantly increased in the ovary and can downregulate ERα expression (148). A complementary study was conducted by Talpur etal. (62) They demonstrated that MT1 receptor knockout mice exhibit higher estradiol and lower progesterone levels, indicating the role of the MT1 receptor in melatonin’s modulation of ovarian steroids.
Another potential mechanism involving melatonin effects on ovarian hormone levels is circadian clock regulation within both the hypothalamus and ovary. In the SCN, a timing signal from the central circadian clock is indispensable for the LH surge (149). Given melatonin’s modulation of the central clock, it is reasonable to assume that melatonin may regulate LH levels through the SCN-dependent pathway. Kisspeptin neurons within the hypothalamus also regulate LH secretion by detecting estrogen levels and internal clock signals. Although gonadotropin-releasing hormone (GnRH) neurons control reproductive hormone production, melatonin appears to synchronize ovarian hormone secretion through kisspeptin neurons (63). By targeting the kisspeptin neural system, melatonin induces hypofunction of the hypothalamus-pituitary-ovarian axis, which results in decreased LH secretion and ultimately estrogen level reduction. In the ovary, the circadian clock also suggests its modulation to steroids. Direct evidence is that Bmal1 knockout mice show blunted progesterone levels (64, 65). Moreover, critical genes in steroid synthesis, such as CYP19 and StAR, are also tightly controlled by the circadian clock (31, 66). In light of melatonin’s regulation of clock genes, clock-targeted regulation of melatonin may play an important part in estrogen and progesterone production.
In PMS, estrogen and progesterone levels show incongruent results, although the tendency of estrogen reduction and progesterone elevation is increasingly recognized. Interestingly, PMS/PMDD patients often have decreased melatonin production, which is thought to result in elevated estrogen and decreased progesterone levels. These contrarian changes indicate that the regulation of steroids involves multiple biological processes. One potential explanation is that the effects of melatonin reduction are not dominant compared with those of primary ovarian hormone changes, which can also explain the indeterminate estrogen and progesterone changes in PMS. On the other hand, although estrogen and progesterone do not show direct modulation of the circadian system, the SCN, kisspeptin neurons and ovarian circadian clock genes are clearly regulated by hormone feedback (149), indicating the participation of a disrupted internal clock and other endocrine hormones. Studies on melatonin, the circadian system and ovarian steroid interactions in PMS require further exploration.
Melatonin modulates brain GABA and BDNF
The core symptoms of PMS, such as sleep disturbance, depression, and cognition disorders, suggest the participation of neurobiological processes in PMS and PMDD. To date, the role of the serotonin system in PMS has been elucidated. Serotonin and its receptors are not only involved in the behavioral regulation of PMS but also mediate the actions of estrogen and progesterone on the brain (150). Serotonin-targeted therapy, such as SSRIs, is recommended as the first-line treatment in PMDD. However, other critical brain neurotransmitters and neuromodulators, such as GABA and BDNF, have not been fully investigated in PMS. Hence, we review recent progress on the relationships between GABA/BDNF and PMS/PMDD. Moreover, the latest studies on melatonin-GABA/BDNF interactions are also recapitulated, anticipating the potential neurological pathways of melatonin-PMS interactions.
GABA system
The GABA system participates in PMS-induced behavioral changes
GABA is the main inhibitory neurotransmitter in the central nervous system, participating in sleep, mood and synaptic plasticity regulation. In PMDD patients with mood disorders, GABA concentrations are dramatically reduced in the brain cingulate cortex, medial prefrontal cortex and left basal ganglia compared to healthy women (11). The GABA type A receptors (GABAARs) also suggest their involvement in the pathogenesis of PMS/PMDD. During the high progesterone phase, δ subunit–containing GABAAR (δGABAAR) expression is significantly increased, resulting in decreased neural excitability and anxiety (151). Diminished cyclic changes in δGABAARs conversely attenuate excitability (152). Further studies have demonstrated that ovarian cycle-linked δGABAAR changes in the hippocampus affect γ oscillations, which may contribute to cognitive and memory deficiencies in PMS/PMDD (10).
Potential mechanisms of GABAAR changes may be the response to neuroactive steroid fluctuations such as estradiol and progesterone. In an animal model of PMDD, rapid withdrawal of progesterone increases anxiety-like behaviors and elevates the expression of GABAARs α4 submit (153). Specifically, allopregnanolone, a modulator of GABAARs, is able to enhance GABA effects and is associated with the negative emotions of PMDD. Antagonizing its effects on GABAARs also yielded promising outcomes in attenuating PMS-related symptoms (154). Moreover, in a PMS rat model, a Japanese herbal medicine, Inochinohaha White, attenuates anxious behavior by upregulating GABAAR-mediated signaling via an increase in the β2 subunit (155). By targeting the GABA system, sepranolone has indicated its potential in PMDD treatment with good tolerance and safety to a certain extent (156). Taken together, these studies demonstrate the connection between the GABA system and PMS symptoms. GABA-targeted therapy may serve as a beneficial option in PMS treatment.
Melatonin regulates PMS Symptoms via the GABA System
Although the relationship between melatonin and GABA in PMS is not fully understood, melatonin and GABA system interactions are well established. Melatonin can directly affect the GABA system (Tables 2, 3). Prado and his colleagues found that melatonin disrupts the circadian changes in GABA with increased GABA levels during the daytime (50). In the hippocampus, melatonin shows distinct effects on the GABA system according to the experimental conditions and melatonin concentrations. In vitro, melatonin elevates the amplitude and frequency of GABAergic inhibitory currents and transmission (34). In contrast, melatonin can enhance neuron excitability and depress GABAAR expression via the activation of MT1 receptors in vivo (51).
In addition, the GABA system may also mediate melatonin’s regulation of sleep, emotion, circadian clock and GnRH neurons. In rats, activation of GABAARs decreases the sleep-promoting effects of melatonin (52). In addition, scientists have found that melatonin prevents anxiety-like behaviors by blocking the α2 subunit of GABAARs (53). Moreover, melatonin and circadian system interactions also involve GABA system regulation. On the one hand, by preventing GABA transmission in the SCN, daytime melatonin secretion is significantly increased, indicating that GABA controls SCN inhibition of melatonin (67). On the other hand, melatonin results in SCN neuron excitability by GABA transmission regulation (54). Similar interactions between GABA and melatonin are also found within GnRH neurons. Ishii etal. (55) found that GnRH neurons can downregulate the expression of MT1 and modify melatonin-induced GABA/GABAAR current decreases. Complementary research was conducted, and melatonin was found to modulate GABAAR-mediated GnRH excitability through melatonin receptors (56). In combination with the current literature on the interaction between melatonin and the GABA system, the hypothesis that melatonin may regulate PMS symptoms via the modulation of the GABAergic neurotransmitter system is reasonable, and further studies should be conducted.
BDNF and its signaling pathways
BDNF is involved in the etiology of PMS
BDNF, a neural growth factor in the brain, is involved in mood regulation, synaptic plasticity, neuronal growth and survival and other crucial physiological processes. To date, researchers have focused on BDNF changes in PMS patients, and the results indicate the role of BDNF in PMS symptoms and etiopathogenesis. In PMDD patients, higher serum BDNF levels are found in the luteal phase than in normal controls (12). Similar results were found by Oral etal. (13), who showed significantly elevated BDNF levels from the follicular to luteal phase. These differences between PMDD and healthy people may be attributed to compensatory BDNF increases to alleviate depression symptoms during the luteal phase. However, the results on BDNF changes in PMS are not consistent. A contradictory study found that BDNF is significantly decreased in the luteal phase compared to the control, and decreased BDNF levels are often accompanied by negative emotions such as anxiety and depression (68). In addition, BDNF levels are reduced from the follicular to luteal phase. In a PMS rat model, BDNF expression is significantly decreased with the activation of opioid receptors (157). These inconsistent results may indicate distinct neuromodulator response alternations between PMS and PMDD.
The BDNF Val66Met polymorphism, which can reduce BDNF bioactivity, has also been associated with neuropsychic disorders. In mice injected with human BDNF Val66Met, increased anxiety-like behaviors during the estrous phase are observed (158). In PMDD patients, suppressed fronto-cingulate cortex activity is observed and associated with the BDNF Val66Met allele (159). Similar to the GABA system, BDNF intersects with ovarian hormone and PMS neurological hallmarks. Steroid regulation of emotion is also dependent on BDNF and its single-nucleotide polymorphism (160).
Melatonin regulates behaviors via BDNF-related pathways
With a gradual understanding of the relationship between melatonin and BDNF, despite a paucity of direct evidence, the melatonin-BDNF pathway has been indicated as the potential mechanism of PMS pathogenesis (Table 3). According to the current literature, at least two aspects can be summarized to comprehend this possible mechanism. First, melatonin regulates depression, cognition and memory via BDNF-related molecular processes. In a mouse model of depression, melatonin displays antidepression effects with the elevation of BDNF levels in the hippocampus (35). Similar effects of melatonin are also observed when combined with fluoxetine treatment, which involves the normalization of hippocampal BDNF-tropomyosin receptor kinase B (TrkB) signaling (42). Apart from emotional changes, cognitive impairment is also common in PMS patients. To date, researchers have found that cognitive damage is attenuated after melatonin treatment by increasing BDNF and TrkB expression in the dentate gyrus, cerebral cortex and hippocampus (69, 161). Melatonin can also regulate long-term memory processes. Sung and colleagues revealed that melatonin can enhance memory formation by increasing both the cyclic adenosine monophosphate response element-binding protein (CREB) and BDNF levels (44), indicating alterations in the CREB-BDNF signaling pathway. Given the characterized depression, cognition and memory lesions in PMDD patients, melatonin regulates PMS via BDNF-related pathways.
In addition, pharmacological studies on melatonin receptor agonists have also provided new insight into melatonin-BDNF interactions in PMS. For example, agomelatine, an agonist of MT1 and MT2 receptors, can increase hippocampal BDNF expression and BDNF-positive neurons in rats (43). Agomelatine also corrects disturbed sleep-wake rhythms and sleep architecture through the elevation of MT1 receptors and BDNF levels (40). As a novel antidepressant, agomelatine reduces anxiety-like behaviors and upregulates BDNF levels in the dentate gyrus (70). Per1 and Per2 expression in the SCN is concomitantly decreased. In a chronic stress model, mice showed restored memory function and elevated CREB/BDNF expression after agomelatine application (45). Another melatonin receptor agonist, ramelteon, improves depression and anxiety symptoms by increasing BDNF levels and targeting clock genes (e.g., Per1 and Per2) (28). Taken together, these studies demonstrate BDNF pathway alterations in melatonin’s action on sleep, emotion, cognition and memory, indicating new perspectives on understanding the potential involvement of melatonin in PMS and PMDD.
Conclusion and outlook
Undoubtedly, cyclical changes in neuroendocrine factors are tightly associated with PMS and PMDD. Ovarian hormone fluctuations during the luteal phase, such as decreased estrogen and elevated progesterone, can induce brain GABA and BDNF changes, which may ultimately contribute to PMS behavioral complaints such as sleep disturbance and mood and cognitive disorders. In this review, we summarize the potential pathogenesis of PMS and the regulation of melatonin on these processes through melatonin receptors and the circadian clock. Current studies on melatonin and PMS are not sufficient. For example, symptoms such as sleep disturbance, depressive mood, and cognitive impairment are not specific and can be easily misdiagnosed with other diseases. Based on previous studies, ovarian steroids seem not specific among PMS patients, and the effect of melatonin supplementation is not clearly clarified. From the view of molecular modulation, what’s the change and relationship between melatonin receptors and PMS are still unknown. As a consequence, studies on melatonin’s role in the female reproductive system should be augmented.
Further research on the relationship between melatonin and PMS may focus on the perspectives described below. First, adequate animal models should be developed to unveil the underlying mechanisms of PMS. Recently, Bellofiore and his colleagues found that spiny mice exhibit PMS-like symptoms and can serve as a preclinical model of PMS (162). However, melatonin secretion patterns and rhythms between nocturnal animals and human beings are not similar, providing another impediment of melatonin-PMS interaction studies. Second, as functional magnetic resonance imaging reveals deficient positive emotion processing during the female luteal phase (163), functional imaging can be adopted to analyze brain activation patterns and neural circuit alterations in PMS. Third, melatonin’s modulation of the hypothalamus-pituitary-ovary (HPO) axis requires further investigation. Although current studies have shown that melatonin regulates estrogen and progesterone levels, since HPO dysfunction is characterized in PMS and regulated by the SCN, kisspeptin neurons and other endocrine factors, does melatonin participate in these regulatory effects? If it participates, what are the possible mechanisms and pathways? These questions should be taken into consideration in future research. Finally, pharmacological studies on melatonin receptor agonists and structural studies on MT1 and MT2 receptors should be conducted. To date, melatonin receptor agonists such as agomelatine and ramelteon are recommended for depression and insomnia treatments, respectively. However, these agonists are nonselective, making it difficult to target MT1 or MT2 receptors specifically to achieve satisfying therapeutic effects. Understanding structural differences between MT1 and MT2 receptors and their different affinities to some compounds may be the foundation of selective melatonin receptor agonist development and application.
Author contributions
JS contributed to the study concept and design. WY and JZ collected and sorted the literature. WY and YG drew pictures and tables. WY and JZ wrote the first draft. ZW and CD edited and approved the English version of the article. All authors contributed to the article and approved the submitted version.
Funding
This work is supported by the Chinese National Natural Science Foundation (grant numbers 81871044 and 81801316).
Conflict of interest
The authors declare that the research was conducted in the absence of any commercial or financial relationships that could be construed as a potential conflict of interest.
Publisher’s note
All claims expressed in this article are solely those of the authors and do not necessarily represent those of their affiliated organizations, or those of the publisher, the editors and the reviewers. Any product that may be evaluated in this article, or claim that may be made by its manufacturer, is not guaranteed or endorsed by the publisher.
Abbreviations
Aana2, arylalkylamine N-acetyltransferase 2; BDNF, brain-derived neurotrophic factor; Bmal1, brain and muscle ARNT-like 1; CREB, cyclic adenosine monophosphate response element-binding protein; CRSDs, circadian rhythm sleep disorders; Cry1, cryptochrome circadian regulator 1; CYP, aromatase cytochrome p450; ESR-1, estrogen receptor alpha; GABA, gamma-aminobutyric acid; GnRH, gonadotropin-releasing hormone; MT, melatonin; mTOR, mammalian target of rapamycin; Nr1d1, nuclear receptor subfamily 1 group D member 1; Per1, Period1; PMDD, premenstrual dysphoric disorder; PMS, premenstrual syndrome; PTSD, posttraumatic stress disorder; LH, luteinizing hormone; REM, rapid eye movement; SCN, suprachiasmatic nucleus; StAR, steroidogenic acute regulatory protein; TrkB, tropomyosin receptor kinase B.
References
1. Rapkin AJ, Mikacich JA. Premenstrual dysphoric disorder and severe premenstrual syndrome in adolescents. Paediatr Drugs (2013) 15(3):191–202. doi: 10.1007/s40272-013-0018-4
2. Appleton SM. Premenstrual syndrome: Evidence-based evaluation and treatment. Clin Obstet Gynecol (2018) 61(1):52–61. doi: 10.1097/grf.0000000000000339
3. Chumpalova P, Iakimova R, Stoimenova-Popova M, Aptalidis D, Pandova M, Stoyanova M, et al. Prevalence and clinical picture of premenstrual syndrome in females from Bulgaria. Ann Gen Psychiatry (2020) 19:3. doi: 10.1186/s12991-019-0255-1
4. Kloss JD, Perlis ML, Zamzow JA, Culnan EJ, Gracia CR. Sleep, sleep disturbance, and fertility in women. Sleep Med Rev (2015) 22:78–87. doi: 10.1016/j.smrv.2014.10.005
5. Lanza di Scalea T, Pearlstein T. Premenstrual dysphoric disorder. Med Clin North Am (2019) 103(4):613–28. doi: 10.1016/j.mcna.2019.02.007
6. Keenan PA, Stern RA, Janowsky DS, Pedersen CA. Psychological aspects of premenstrual syndrome. I: Cognition and memory. Psychoneuroendocrinology (1992) 17(2-3):179–87. doi: 10.1016/0306-4530(92)90056-d
7. Slyepchenko A, Lokuge S, Nicholls B, Steiner M, Hall GB, Soares CN, et al. Subtle persistent working memory and selective attention deficits in women with premenstrual syndrome. Psychiatry Res (2017) 249:354–62. doi: 10.1016/j.psychres.2017.01.031
8. Dubey N, Hoffman JF, Schuebel K, Yuan Q, Martinez PE, Nieman LK, et al. The ESC/E(Z) complex, an effector of response to ovarian steroids, manifests an intrinsic difference in cells from women with premenstrual dysphoric disorder. Mol Psychiatry (2017) 22(8):1172–84. doi: 10.1038/mp.2016.229
9. Naheed B, Kuiper JH, Uthman OA, O'Mahony F, O'Brien PM. Non-contraceptive oestrogen-containing preparations for controlling symptoms of premenstrual syndrome. Cochrane Database Syst Rev (2017) 3(3):Cd010503. doi: 10.1002/14651858.CD010503.pub2
10. Barth AM, Ferando I, Mody I. Ovarian cycle-linked plasticity of δ-GABAA receptor subunits in hippocampal interneurons affects γ oscillations in vivo. Front Cell Neurosci (2014) 8:222. doi: 10.3389/fncel.2014.00222
11. Liu B, Wang G, Gao D, Gao F, Zhao B, Qiao M, et al. Alterations of GABA and glutamate-glutamine levels in premenstrual dysphoric disorder: A 3T proton magnetic resonance spectroscopy study. Psychiatry Res (2015) 231(1):64–70. doi: 10.1016/j.pscychresns.2014.10.020
12. Oral E, Ozcan H, Kirkan TS, Askin S, Gulec M, Aydin N. Luteal serum BDNF and HSP70 levels in women with premenstrual dysphoric disorder. Eur Arch Psychiatry Clin Neurosci (2013) 263(8):685–93. doi: 10.1007/s00406-013-0398-z
13. Oral E, Kirkan TS, Yildirim A, Kotan Z, Cansever Z, Ozcan H, et al. Serum brain-derived neurotrophic factor differences between the luteal and follicular phases in premenstrual dysphoric disorder. Gen Hosp Psychiatry (2015) 37(3):266–72. doi: 10.1016/j.genhosppsych.2015.03.001
14. Matsumoto T, Asakura H, Hayashi T. Biopsychosocial aspects of premenstrual syndrome and premenstrual dysphoric disorder. Gynecol Endocrinol (2013) 29(1):67–73. doi: 10.3109/09513590.2012.705383
15. Yonkers KA, Simoni MK. Premenstrual disorders. Am J Obstet Gynecol (2018) 218(1):68–74. doi: 10.1016/j.ajog.2017.05.045
16. de Wit AE, de Vries YA, de Boer MK, Scheper C, Fokkema A, Janssen CAH, et al. Efficacy of combined oral contraceptives for depressive symptoms and overall symptomatology in premenstrual syndrome: pairwise and network meta-analysis of randomized trials. Am J Obstet Gynecol (2021) 225(6):624–33. doi: 10.1016/j.ajog.2021.06.090
17. Comasco E, Kopp Kallner H, Bixo M, Hirschberg AL, Nyback S, de Grauw H, et al. Ulipristal acetate for treatment of premenstrual dysphoric disorder: A proof-of-Concept randomized controlled trial. Am J Psychiatry (2021) 178(3):256–65. doi: 10.1176/appi.ajp.2020.20030286
18. Amaral FGD, Cipolla-Neto J. A brief review about melatonin, a pineal hormone. Arch Endocrinol Metab (2018) 62(4):472–9. doi: 10.20945/2359-3997000000066
19. Parry BL, Berga SL, Kripke DF, Klauber MR, Laughlin GA, Yen SS, et al. Altered waveform of plasma nocturnal melatonin secretion in premenstrual depression. Arch Gen Psychiatry (1990) 47(12):1139–46. doi: 10.1001/archpsyc.1990.01810240059010
20. Parry BL, Berga SL, Mostofi N, Klauber MR, Resnick A. Plasma melatonin circadian rhythms during the menstrual cycle and after light therapy in premenstrual dysphoric disorder and normal control subjects. J Biol Rhythms (1997) 12(1):47–64. doi: 10.1177/074873049701200107
21. Parry BL, Udell C, Elliott JA, Berga SL, Klauber MR, Mostofi N, et al. Blunted phase-shift responses to morning bright light in premenstrual dysphoric disorder. J Biol Rhythms (1997) 12(5):443–56. doi: 10.1177/074873049701200506
22. Tosini G, Owino S, Guillaume JL, Jockers R. Understanding melatonin receptor pharmacology: latest insights from mouse models, and their relevance to human disease. Bioessays (2014) 36(8):778–87. doi: 10.1002/bies.201400017
23. Cecon E, Oishi A, Jockers R. Melatonin receptors: molecular pharmacology and signalling in the context of system bias. Br J Pharmacol (2018) 175:3263–80. doi: 10.1111/bph.13950
24. Shechter A, Lespérance P, Ng Ying Kin NM, Boivin DB. Pilot investigation of the circadian plasma melatonin rhythm across the menstrual cycle in a small group of women with premenstrual dysphoric disorder. PloS One (2012) 7(12):e51929. doi: 10.1371/journal.pone.0051929
25. Moderie C, Boudreau P, Shechter A, Lespérance P, Boivin DB. Effects of exogenous melatonin on sleep and circadian rhythms in women with premenstrual dysphoric disorder. Sleep (2021) 44(12). doi: 10.1093/sleep/zsab171
26. Wongprayoon P, Govitrapong P. Melatonin receptor as a drug target for neuroprotection. Curr Mol Pharmacol (2021) 14(2):150–64. doi: 10.2174/1874467213666200421160835
27. Yong W, Ma H, Na M, Gao T, Zhang Y, Hao L, et al. Roles of melatonin in the field of reproductive medicine. BioMed Pharmacother (2021) 144:112001. doi: 10.1016/j.biopha.2021.112001
28. Satyanarayanan SK, Chien YC, Chang JP, Huang SY, Guu TW, Su H, et al. Melatonergic agonist regulates circadian clock genes and peripheral inflammatory and neuroplasticity markers in patients with depression and anxiety. Brain Behav Immun (2020) 85:142–51. doi: 10.1016/j.bbi.2019.03.003
29. Yuan H, Wu G, Zhai X, Lu B, Meng B, Chen J. Melatonin and rapamycin attenuate isoflurane-induced cognitive impairment through inhibition of neuroinflammation by suppressing the mTOR signaling in the hippocampus of aged mice. Front Aging Neurosci (2019) 11:314. doi: 10.3389/fnagi.2019.00314
30. Lv WJ, Liu C, Yu LZ, Zhou JH, Li Y, Xiong Y, et al. Melatonin alleviates neuroinflammation and metabolic disorder in DSS-induced depression rats. Oxid Med Cell Longev (2020) 2020:1241894. doi: 10.1155/2020/1241894
31. Guan S, Xie L, Ma T, Lv D, Jing W, Tian X, et al. Effects of melatonin on early pregnancy in mouse: Involving the regulation of StAR, Cyp11a1, and ihh expression. Int J Mol Sci (2017) 18(8). doi: 10.3390/ijms18081637
32. Martínez-Campa C, González A, Mediavilla MD, Alonso-González C, Alvarez-García V, Sánchez-Barceló EJ, et al. Melatonin inhibits aromatase promoter expression by regulating cyclooxygenases expression and activity in breast cancer cells. Br J Cancer (2009) 101(9):1613–9. doi: 10.1038/sj.bjc.6605336
33. Fang L, Li Y, Wang S, Yu Y, Li Y, Guo Y, et al. Melatonin induces progesterone production in human granulosa-lutein cells through upregulation of StAR expression. Aging (Albany NY) (2019) 11(20):9013–24. doi: 10.18632/aging.102367
34. Cheng XP, Sun H, Ye ZY, Zhou JN. Melatonin modulates the GABAergic response in cultured rat hippocampal neurons. J Pharmacol Sci (2012) 119(2):177–85. doi: 10.1254/jphs.11183fp
35. Taniguti EH, Ferreira YS, Stupp IJV, Fraga-Junior EB, Mendonça CB, Rossi FL, et al. Neuroprotective effect of melatonin against lipopolysaccharide-induced depressive-like behavior in mice. Physiol Behav (2018) 188:270–5. doi: 10.1016/j.physbeh.2018.02.034
36. Gandhi AV, Mosser EA, Oikonomou G, Prober DA. Melatonin is required for the circadian regulation of sleep. Neuron (2015) 85(6):1193–9. doi: 10.1016/j.neuron.2015.02.016
37. Spiegelhalder K, Nissen C, Riemann D. Clinical sleep-wake disorders II: Focus on insomnia and circadian rhythm sleep disorders. Handb Exp Pharmacol (2019) 253:261–76. doi: 10.1007/164_2017_40
38. Comai S, Ochoa-Sanchez R, Gobbi G. Sleep-wake characterization of double MT1/MT2 receptor knockout mice and comparison with MT1 and MT2 receptor knockout mice. Behav Brain Res (2013) 243:231–8. doi: 10.1016/j.bbr.2013.01.008
39. Ochoa-Sanchez R, Comai S, Lacoste B, Bambico FR, Dominguez-Lopez S, Spadoni G, et al. Promotion of non-rapid eye movement sleep and activation of reticular thalamic neurons by a novel MT2 melatonin receptor ligand. J Neurosci (2011) 31(50):18439–52. doi: 10.1523/jneurosci.2676-11.2011
40. Tchekalarova J, Kortenska L, Ivanova N, Atanasova M, Marinov P. Agomelatine treatment corrects impaired sleep-wake cycle and sleep architecture and increases MT(1) receptor as well as BDNF expression in the hippocampus during the subjective light phase of rats exposed to chronic constant light. Psychopharmacol (Berl) (2020) 237(2):503–18. doi: 10.1007/s00213-019-05385-y
41. Kirby AW, Clayton M, Rivera P, Comperatore CA. Melatonin and the reduction or alleviation of stress. J Pineal Res (1999) 27(2):78–85. doi: 10.1111/j.1600-079x.1999.tb00600.x
42. Li K, Shen S, Ji YT, Li XY, Zhang LS, Wang XD. Melatonin augments the effects of fluoxetine on depression-like behavior and hippocampal BDNF-TrkB signaling. Neurosci Bull (2018) 34(2):303–11. doi: 10.1007/s12264-017-0189-z
43. Lu Y, Ho CS, McIntyre RS, Wang W, Ho RC. Agomelatine-induced modulation of brain-derived neurotrophic factor (BDNF) in the rat hippocampus. Life Sci (2018) 210:177–84. doi: 10.1016/j.lfs.2018.09.003
44. Sung JY, Bae JH, Lee JH, Kim YN, Kim DK. The melatonin signaling pathway in a long-term memory in vitro study. Molecules (2018) 23(4). doi: 10.3390/molecules23040737
45. Gumuslu E, Mutlu O, Sunnetci D, Ulak G, Celikyurt IK, Cine N, et al. The antidepressant agomelatine improves memory deterioration and upregulates CREB and BDNF gene expression levels in unpredictable chronic mild stress (UCMS)-exposed mice. Drug Target Insights (2014) 8:11–21. doi: 10.4137/dti.S13870
46. Sirichoat A, Suwannakot K, Chaisawang P, Pannangrong W, Aranarochana A, Wigmore P, et al. Melatonin attenuates 5-fluorouracil-induced spatial memory and hippocampal neurogenesis impairment in adult rats. Life Sci (2020) 248:117468. doi: 10.1016/j.lfs.2020.117468
47. Giacomini A, Teixeira KH, Marcon L, Scolari N, Bueno BW, Genario R, et al. Melatonin treatment reverses cognitive and endocrine deficits evoked by a 24-h light exposure in adult zebrafish. Neurosci Lett (2020) 733:135073. doi: 10.1016/j.neulet.2020.135073
48. Tabassum H, Ashafaq M, Parvez S, Raisuddin S. Role of melatonin in mitigating nonylphenol-induced toxicity in frontal cortex and hippocampus of rat brain. Neurochem Int (2017) 104:11–26. doi: 10.1016/j.neuint.2016.12.010
49. Bondi CD, Alonso-Gonzalez C, Clafshenkel WP, Kotlarczyk MP, Dodda BR, Sanchez-Barcelo E, et al. The effect of estradiol, progesterone, and melatonin on estrous cycling and ovarian aromatase expression in intact female mice. Eur J Obstet Gynecol Reprod Biol (2014) 174:80–5. doi: 10.1016/j.ejogrb.2013.11.027
50. Marquez de Prado B, Castañeda TR, Galindo A, del Arco A, Segovia G, Reiter RJ, et al. Melatonin disrupts circadian rhythms of glutamate and GABA in the neostriatum of the aware rat: A microdialysis study. J Pineal Res (2000) 29(4):209–16. doi: 10.1034/j.1600-0633.2002.290403.x
51. Stewart LS, Leung LS. Hippocampal melatonin receptors modulate seizure threshold. Epilepsia (2005) 46(4):473–80. doi: 10.1111/j.0013-9580.2005.30204.x
52. Wang F, Li J, Wu C, Yang J, Xu F, Zhao Q. The GABA(A) receptor mediates the hypnotic activity of melatonin in rats. Pharmacol Biochem Behav (2003) 74(3):573–8. doi: 10.1016/s0091-3057(02)01045-6
53. Zhang L, Guo HL, Zhang HQ, Xu TQ, He B, Wang ZH, et al. Melatonin prevents sleep deprivation-associated anxiety-like behavior in rats: role of oxidative stress and balance between GABAergic and glutamatergic transmission. Am J Transl Res (2017) 9(5):2231–42.
54. Scott FF, Belle MD, Delagrange P, Piggins HD. Electrophysiological effects of melatonin on mouse Per1 and non-Per1 suprachiasmatic nuclei neurones in vitro. J Neuroendocrinol (2010) 22(11):1148–56. doi: 10.1111/j.1365-2826.2010.02063.x
55. Ishii H, Sato S, Yin C, Sakuma Y, Kato M. Cetrorelix, a gonadotropin-releasing hormone antagonist, induces the expression of melatonin receptor 1a in the gonadotropin-releasing hormone neuronal cell line GT1-7. Neuroendocrinology (2009) 90(3):251–9. doi: 10.1159/000231993
56. Sato S, Yin C, Teramoto A, Sakuma Y, Kato M. Sexually dimorphic modulation of GABA(A) receptor currents by melatonin in rat gonadotropin-releasing hormone neurons. J Physiol Sci (2008) 58(5):317–22. doi: 10.2170/physiolsci.RP006208
57. Gouin JP, Connors J, Kiecolt-Glaser JK, Glaser R, Malarkey WB, Atkinson C, et al. Altered expression of circadian rhythm genes among individuals with a history of depression. J Affect Disord (2010) 126(1-2):161–6. doi: 10.1016/j.jad.2010.04.002
58. Zhao C, Gammie SC. The circadian gene Nr1d1 in the mouse nucleus accumbens modulates sociability and anxiety-related behaviour. Eur J Neurosci (2018) 48(3):1924–43. doi: 10.1111/ejn.14066
59. Weissová K, Škrabalová J, Skálová K, Červená K, Bendová Z, Miletínová E, et al. Circadian rhythms of melatonin and peripheral clock gene expression in idiopathic REM sleep behavior disorder. Sleep Med (2018) 52:1–6. doi: 10.1016/j.sleep.2018.07.019
60. Beker MC, Caglayan B, Caglayan AB, Kelestemur T, Yalcin E, Caglayan A, et al. Interaction of melatonin and Bmal1 in the regulation of PI3K/AKT pathway components and cellular survival. Sci Rep (2019) 9(1):19082. doi: 10.1038/s41598-019-55663-0
61. Maganhin CC, Simões RS, Fuchs LF, Sasso GR, Simões MJ, Baracat EC, et al. Melatonin influences on steroidogenic gene expression in the ovary of pinealectomized rats. Fertil Steril (2014) 102(1):291–8. doi: 10.1016/j.fertnstert.2014.04.006
62. Talpur HS, Worku T, Rehman ZU, Dad R, Bhattarai D, Bano I, et al. Knockdown of melatonin receptor 1 and induction of follicle-stimulating hormone on the regulation of mouse granulosa cell function. Reprod Biol (2017) 17(4):380–8. doi: 10.1016/j.repbio.2017.10.005
63. Revel FG, Ansel L, Klosen P, Saboureau M, Pévet P, Mikkelsen JD, et al. Kisspeptin: a key link to seasonal breeding. Rev Endocr Metab Disord (2007) 8(1):57–65. doi: 10.1007/s11154-007-9031-7
64. Ratajczak CK, Boehle KL, Muglia LJ. Impaired steroidogenesis and implantation failure in Bmal1-/- mice. Endocrinology (2009) 150(4):1879–85. doi: 10.1210/en.2008-1021
65. Liu Y, Johnson BP, Shen AL, Wallisser JA, Krentz KJ, Moran SM, et al. Loss of BMAL1 in ovarian steroidogenic cells results in implantation failure in female mice. Proc Natl Acad Sci U.S.A. (2014) 111(39):14295–300. doi: 10.1073/pnas.1209249111
66. Chen H, Zhao L, Kumazawa M, Yamauchi N, Shigeyoshi Y, Hashimoto S, et al. Downregulation of core clock gene Bmal1 attenuates expression of progesterone and prostaglandin biosynthesis-related genes in rat luteinizing granulosa cells. Am J Physiol Cell Physiol (2013) 304(12):C1131–1140. doi: 10.1152/ajpcell.00008.2013
67. Kalsbeek A, Garidou ML, Palm IF, van der Vliet J, Simonneaux V, Pévet P, et al. Melatonin sees the light: blocking GABA-ergic transmission in the paraventricular nucleus induces daytime secretion of melatonin. Eur J Neurosci (2000) 12(9):3146–54. doi: 10.1046/j.1460-9568.2000.00202.x
68. Cubeddu A, Bucci F, Giannini A, Russo M, Daino D, Russo N, et al. Brain-derived neurotrophic factor plasma variation during the different phases of the menstrual cycle in women with premenstrual syndrome. Psychoneuroendocrinology (2011) 36(4):523–30. doi: 10.1016/j.psyneuen.2010.08.006
69. Chen BH, Park JH, Lee TK, Song M, Kim H, Lee JC, et al. Melatonin attenuates scopolamine-induced cognitive impairment via protecting against demyelination through BDNF-TrkB signaling in the mouse dentate gyrus. Chem Biol Interact (2018) 285:8–13. doi: 10.1016/j.cbi.2018.02.023
70. Cohen H, Zohar J, Carmi L. Effects of agomelatine on behaviour, circadian expression of period 1 and period 2 clock genes and neuroplastic markers in the predator scent stress rat model of PTSD. World J Biol Psychiatry (2020) 21(4):255–73. doi: 10.1080/15622975.2018.1523560
71. Shinohara K, Uchiyama M, Okawa M, Saito K, Kawaguchi M, Funabashi T, et al. Menstrual changes in sleep, rectal temperature and melatonin rhythms in a subject with premenstrual syndrome. Neurosci Lett (2000) 281(2-3):159–62. doi: 10.1016/s0304-3940(00)00826-0
72. Nicolau ZFM, Bezerra AG, Polesel DN, Andersen ML, Bittencourt L, Tufik S, et al. Premenstrual syndrome and sleep disturbances: Results from the sao paulo epidemiologic sleep study. Psychiatry Res (2018) 264:427–31. doi: 10.1016/j.psychres.2018.04.008
73. Conzatti M, Perez AV, Maciel RF, De Castro DH, Sbaraini M, Wender MCO. Sleep quality and excessive daytime sleepiness in women with premenstrual syndrome. Gynecol Endocrinol (2021) 37(10):945–9. doi: 10.1080/09513590.2021.1968820
74. Sharkey KM, Crawford SL, Kim S, Joffe H. Objective sleep interruption and reproductive hormone dynamics in the menstrual cycle. Sleep Med (2014) 15(6):688–93. doi: 10.1016/j.sleep.2014.02.003
75. Li DX, Romans S, De Souza MJ, Murray B, Einstein G. Actigraphic and self-reported sleep quality in women: associations with ovarian hormones and mood. Sleep Med (2015) 16(10):1217–24. doi: 10.1016/j.sleep.2015.06.009
76. Gupta R, Lahan V, Bansal S. Subjective sleep problems in young women suffering from premenstrual dysphoric disorder. N Am J Med Sci (2012) 4(11):593–5. doi: 10.4103/1947-2714.103326
77. Baker FC, Lee KA. Menstrual cycle effects on sleep. Sleep Med Clin (2018) 13(3):283–94. doi: 10.1016/j.jsmc.2018.04.002
78. Ozisik Karaman HI, Tanriverdi G, Degirmenci Y. Subjective sleep quality in premenstrual syndrome. Gynecol Endocrinol (2012) 28(8):661–4. doi: 10.3109/09513590.2011.650769
79. Xing X, Xue P, Li SX, Zhou J, Tang X. Sleep disturbance is associated with an increased risk of menstrual problems in female Chinese university students. Sleep Breath. (2020) 24(4):1719–27. doi: 10.1007/s11325-020-02105-1
80. Baker FC, Kahan TL, Trinder J, Colrain IM. Sleep quality and the sleep electroencephalogram in women with severe premenstrual syndrome. Sleep (2007) 30(10):1283–91. doi: 10.1093/sleep/30.10.1283
81. Baker FC, Sassoon SA, Kahan T, Palaniappan L, Nicholas CL, Trinder J, et al. Perceived poor sleep quality in the absence of polysomnographic sleep disturbance in women with severe premenstrual syndrome. J Sleep Res (2012) 21(5):535–45. doi: 10.1111/j.1365-2869.2012.01007.x
82. Parry BL, Mendelson WB, Duncan WC, Sack DA, Wehr TA. Longitudinal sleep EEG, temperature, and activity measurements across the menstrual cycle in patients with premenstrual depression and in age-matched controls. Psychiatry Res (1989) 30(3):285–303. doi: 10.1016/0165-1781(89)90020-6
83. Parry BL, Mostofi N, LeVeau B, Nahum HC, Golshan S, Laughlin GA, et al. Sleep EEG studies during early and late partial sleep deprivation in premenstrual dysphoric disorder and normal control subjects. Psychiatry Res (1999) 85(2):127–43. doi: 10.1016/s0165-1781(98)00128-0
84. Pavlova M. Circadian rhythm sleep-wake disorders. Continuum (Minneap Minn) (2017) 23(4, Sleep Neurology):1051–63. doi: 10.1212/con.0000000000000499
85. Shechter A, Varin F, Boivin DB. Circadian variation of sleep during the follicular and luteal phases of the menstrual cycle. Sleep (2010) 33(5):647–56. doi: 10.1093/sleep/33.5.647
86. Komada Y, Ikeda Y, Sato M, Kami A, Masuda C, Shibata S. Social jetlag and menstrual symptoms among female university students. Chronobiol Int (2019) 36(2):258–64. doi: 10.1080/07420528.2018.1533561
87. Parry BL, Meliska CJ, Martínez LF, López AM, Sorenson DL, Hauger RL, et al. Late, but not early, wake therapy reduces morning plasma melatonin: relationship to mood in premenstrual dysphoric disorder. Psychiatry Res (2008) 161(1):76–86. doi: 10.1016/j.psychres.2007.11.017
88. Shechter A, Lespérance P, Ng Ying Kin NM, Boivin DB. Nocturnal polysomnographic sleep across the menstrual cycle in premenstrual dysphoric disorder. Sleep Med (2012) 13(8):1071–8. doi: 10.1016/j.sleep.2012.05.012
89. Auld F, Maschauer EL, Morrison I, Skene DJ, Riha RL. Evidence for the efficacy of melatonin in the treatment of primary adult sleep disorders. Sleep Med Rev (2017) 34:10–22. doi: 10.1016/j.smrv.2016.06.005
90. Li T, Jiang S, Han M, Yang Z, Lv J, Deng C, et al. Exogenous melatonin as a treatment for secondary sleep disorders: A systematic review and meta-analysis. Front Neuroendocrinol (2019) 52:22–8. doi: 10.1016/j.yfrne.2018.06.004
91. Williams WP 3rd, McLin DE 3rd, Dressman MA, Neubauer DN. Comparative review of approved melatonin agonists for the treatment of circadian rhythm sleep-wake disorders. Pharmacotherapy (2016) 36(9):1028–41. doi: 10.1002/phar.1822
92. Nishimon S, Nishino N, Nishino S. Advances in the pharmacological management of non-24-h sleep-wake disorder. Expert Opin Pharmacother (2021) 22(8):1039–49. doi: 10.1080/14656566.2021.1876665
93. Xu H, Zhang C, Qian Y, Zou J, Li X, Liu Y, et al. Efficacy of melatonin for sleep disturbance in middle-aged primary insomnia: a double-blind, randomised clinical trial. Sleep Med (2020) 76:113–9. doi: 10.1016/j.sleep.2020.10.018
94. Fatemeh G, Sajjad M, Niloufar R, Neda S, Leila S, Khadijeh M. Effect of melatonin supplementation on sleep quality: a systematic review and meta-analysis of randomized controlled trials. J Neurol (2022) 269(1):205–16. doi: 10.1007/s00415-020-10381-w
95. Shabani A, Foroozanfard F, Kavossian E, Aghadavod E, Ostadmohammadi V, Reiter RJ, et al. Effects of melatonin administration on mental health parameters, metabolic and genetic profiles in women with polycystic ovary syndrome: A randomized, double-blind, placebo-controlled trial. J Affect Disord (2019) 250:51–6. doi: 10.1016/j.jad.2019.02.066
96. Baglioni C, Bostanova Z, Bacaro V, Benz F, Hertenstein E, Spiegelhalder K, et al. A systematic review and network meta-analysis of randomized controlled trials evaluating the evidence base of melatonin, light exposure, exercise, and complementary and alternative medicine for patients with insomnia disorder. J Clin Med (2020) 9(6). doi: 10.3390/jcm9061949
97. Semenova NV, Madaeva IM, Bairova TA, Zhambalova RM, Sholokhov LF, Kolesnikova LI. Association of the melatonin circadian rhythms with clock 3111T/C gene polymorphism in Caucasian and Asian menopausal women with insomnia. Chronobiol Int (2018) 35(8):1066–76. doi: 10.1080/07420528.2018.1456447
98. Xie Z, Chen F, Li WA, Geng X, Li C, Meng X, et al. A review of sleep disorders and melatonin. Neurol Res (2017) 39(6):559–65. doi: 10.1080/01616412.2017.1315864
99. Gobbi G, Comai S. Differential function of melatonin MT(1) and MT(2) receptors in REM and NREM sleep. Front Endocrinol (Lausanne) (2019) 10:87. doi: 10.3389/fendo.2019.00087
100. Gobbi G, Comai S. Sleep well. untangling the role of melatonin MT1 and MT2 receptors in sleep. J Pineal Res (2019) 66(3):e12544. doi: 10.1111/jpi.12544
101. Comai S, Lopez-Canul M, De Gregorio D, Posner A, Ettaoussi M, Guarnieri FC, et al. Melatonin MT(1) receptor as a novel target in neuropsychopharmacology: MT(1) ligands, pathophysiological and therapeutic implications, and perspectives. Pharmacol Res (2019) 144:343–56. doi: 10.1016/j.phrs.2019.04.015
102. Touitou Y, Bogdan A. Promoting adjustment of the sleep-wake cycle by chronobiotics. Physiol Behav (2007) 90(2-3):294–300. doi: 10.1016/j.physbeh.2006.09.001
103. American Psychiatric Association. Diagnostic and statistical manual of mental disorders (DSM-5) . Available at: https://www.psychiatry.org/psychiatrists/practice/dsm (Accessed October 1, 2022).
104. Marjoribanks J, Brown J, O'Brien PM, Wyatt K. Selective serotonin reuptake inhibitors for premenstrual syndrome. Cochrane Database Syst Rev (2013) 2013(6):Cd001396. doi: 10.1002/14651858.CD001396.pub3
105. Kishi T, Nomura I, Sakuma K, Kitajima T, Mishima K, Iwata N. Melatonin receptor agonists-ramelteon and melatonin-for bipolar disorder: a systematic review and meta-analysis of double-blind, randomized, placebo-controlled trials. Neuropsychiatr Dis Treat (2019) 15:1479–86. doi: 10.2147/ndt.S198899
106. Gabriel FC, de Melo DO, Fráguas R, Leite-Santos NC, Mantovani da Silva RA, Ribeiro E. Pharmacological treatment of depression: A systematic review comparing clinical practice guideline recommendations. PloS One (2020) 15(4):e0231700. doi: 10.1371/journal.pone.0231700
107. Johansson C, Willeit M, Smedh C, Ekholm J, Paunio T, Kieseppä T, et al. Circadian clock-related polymorphisms in seasonal affective disorder and their relevance to diurnal preference. Neuropsychopharmacology (2003) 28(4):734–9. doi: 10.1038/sj.npp.1300121
108. Liberman AR, Halitjaha L, Ay A, Ingram KK. Modeling strengthens molecular link between circadian polymorphisms and major mood disorders. J Biol Rhythms (2018) 33(3):318–36. doi: 10.1177/0748730418764540
109. Ferrer A, Costas J, Gratacos M, Martínez-Amorós È., Labad J, Soriano-Mas C, et al. Clock gene polygenic risk score and seasonality in major depressive disorder and bipolar disorder. Genes Brain Behav (2020):e12683. doi: 10.1111/gbb.12683
110. Wang XL, Wang DQ, Jiao FC, Ding KM, Ji YB, Lu L, et al. Diurnal rhythm disruptions induced by chronic unpredictable stress relate to depression-like behaviors in rats. Pharmacol Biochem Behav (2021) 204:173156. doi: 10.1016/j.pbb.2021.173156
111. Nováková M, Praško J, Látalová K, Sládek M, Sumová A. The circadian system of patients with bipolar disorder differs in episodes of mania and depression. Bipolar Disord (2015) 17(3):303–14. doi: 10.1111/bdi.12270
112. Chen X, Hu Q, Zhang K, Teng H, Li M, Li D, et al. The clock-controlled chemokine contributes to neuroinflammation-induced depression. FASEB J (2020) 34(6):8357–66. doi: 10.1096/fj.201900581RRR
113. Kishi T, Kitajima T, Ikeda M, Yamanouchi Y, Kinoshita Y, Kawashima K, et al. Association analysis of nuclear receptor rev-erb alpha gene (NR1D1) with mood disorders in the Japanese population. Neurosci Res (2008) 62(4):211–5. doi: 10.1016/j.neures.2008.08.008
114. Hühne A, Volkmann P, Stephan M, Rossner M, Landgraf D. An in-depth neurobehavioral characterization shows anxiety-like traits, impaired habituation behavior, and restlessness in male cryptochrome-deficient mice. Genes Brain Behav (2020):e12661. doi: 10.1111/gbb.12661
115. Jiménez-Ortega V, Barquilla PC, Pagano ES, Fernández-Mateos P, Esquifino AI, Cardinali DP. Melatonin supplementation decreases prolactin synthesis and release in rat adenohypophysis: correlation with anterior pituitary redox state and circadian clock mechanisms. Chronobiol Int (2012) 29(8):1021–35. doi: 10.3109/07420528.2012.705936
116. Nagy AD, Iwamoto A, Kawai M, Goda R, Matsuo H, Otsuka T, et al. Melatonin adjusts the expression pattern of clock genes in the suprachiasmatic nucleus and induces antidepressant-like effect in a mouse model of seasonal affective disorder. Chronobiol Int (2015) 32(4):447–57. doi: 10.3109/07420528.2014.992525
117. Knight MJ, Baune BT. Cognitive dysfunction in major depressive disorder. Curr Opin Psychiatry (2018) 31(1):26–31. doi: 10.1097/yco.0000000000000378
118. Yen JY, Chang SJ, Long CY, Tang TC, Chen CC, Yen CF. Working memory deficit in premenstrual dysphoric disorder and its associations with difficulty in concentrating and irritability. Compr Psychiatry (2012) 53(5):540–5. doi: 10.1016/j.comppsych.2011.05.016
119. Le J, Thomas N, Gurvich C. Cognition, the menstrual cycle, and premenstrual disorders: A review. Brain Sci (2020) 10(4). doi: 10.3390/brainsci10040198
120. Iosifescu DV. The relation between mood, cognition and psychosocial functioning in psychiatric disorders. Eur Neuropsychopharmacol (2012) 22 Suppl 3:S499–504. doi: 10.1016/j.euroneuro.2012.08.002
121. Kundu P, Neese SL, Bandara S, Monaikul S, Helferich WG, Doerge DR, et al. The effects of the botanical estrogen, isoliquiritigenin on delayed spatial alternation. Neurotoxicol Teratol (2018) 66:55–62. doi: 10.1016/j.ntt.2018.02.001
122. Lengel D, Huh JW, Barson JR, Raghupathi R. Progesterone treatment following traumatic brain injury in the 11-day-old rat attenuates cognitive deficits and neuronal hyperexcitability in adolescence. Exp Neurol (2020) 330:113329. doi: 10.1016/j.expneurol.2020.113329
123. Song J, Chu S, Cui Y, Qian Y, Li X, Xu F, et al. Circadian rhythm resynchronization improved isoflurane-induced cognitive dysfunction in aged mice. Exp Neurol (2018) 306:45–54. doi: 10.1016/j.expneurol.2018.04.009
124. Palmer ACS, Zortea M, Souza A, Santos V, Biazús JV, Torres ILS, et al. Clinical impact of melatonin on breast cancer patients undergoing chemotherapy; effects on cognition, sleep and depressive symptoms: A randomized, double-blind, placebo-controlled trial. PloS One (2020) 15(4):e0231379. doi: 10.1371/journal.pone.0231379
125. Kurowska A, Bodys-Cupak I, Staszkiewicz M, Szklarczyk J, Zalewska-Puchała J, Kliś-Kalinowska A, et al. Interleukin-6 and melatonin as predictors of cognitive, emotional and functional ageing of older people. Int J Environ Res Public Health (2020) 17(10). doi: 10.3390/ijerph17103623
126. Chen C, Yang C, Wang J, Huang X, Yu H, Li S, et al. Melatonin ameliorates cognitive deficits through improving mitophagy in a mouse model of alzheimer's disease. J Pineal Res (2021) 71(4):e12774. doi: 10.1111/jpi.12774
127. Wei SM, Baller EB, Martinez PE, Goff AC, Li HJ, Kohn PD, et al. Subgenual cingulate resting regional cerebral blood flow in premenstrual dysphoric disorder: differential regulation by ovarian steroids and preliminary evidence for an association with expression of ESC/E(Z) complex genes. Transl Psychiatry (2021) 11(1):206. doi: 10.1038/s41398-021-01328-4
129. Albert KM, Newhouse PA. Estrogen, stress, and depression: Cognitive and biological interactions. Annu Rev Clin Psychol (2019) 15:399–423. doi: 10.1146/annurev-clinpsy-050718-095557
130. Mihm M, Gangooly S, Muttukrishna S. The normal menstrual cycle in women. Anim Reprod Sci (2011) 124(3-4):229–36. doi: 10.1016/j.anireprosci.2010.08.030
131. Yen JY, Lin HC, Lin PC, Liu TL, Long CY, Ko CH. Early- and late-Luteal-Phase estrogen and progesterone levels of women with premenstrual dysphoric disorder. Int J Environ Res Public Health (2019) 16(22). doi: 10.3390/ijerph16224352
132. Thys-Jacobs S, McMahon D, Bilezikian JP. Differences in free estradiol and sex hormone-binding globulin in women with and without premenstrual dysphoric disorder. J Clin Endocrinol Metab (2008) 93(1):96–102. doi: 10.1210/jc.2007-1726
133. Li Y, Pehrson AL, Budac DP, Sánchez C, Gulinello M. A rodent model of premenstrual dysphoria: progesterone withdrawal induces depression-like behavior that is differentially sensitive to classes of antidepressants. Behav Brain Res (2012) 234(2):238–47. doi: 10.1016/j.bbr.2012.06.034
134. Ozgocer T, Ucar C, Yildiz S. Cortisol awakening response is blunted and pain perception is increased during menses in cyclic women. Psychoneuroendocrinology (2017) 77:158–64. doi: 10.1016/j.psyneuen.2016.12.011
135. Schmidt PJ, Martinez PE, Nieman LK, Koziol DE, Thompson KD, Schenkel L, et al. Premenstrual dysphoric disorder symptoms following ovarian suppression: Triggered by change in ovarian steroid levels but not continuous stable levels. Am J Psychiatry (2017) 174(10):980–9. doi: 10.1176/appi.ajp.2017.16101113
136. Huo L, Straub RE, Roca C, Schmidt PJ, Shi K, Vakkalanka R, et al. Risk for premenstrual dysphoric disorder is associated with genetic variation in ESR1, the estrogen receptor alpha gene. Biol Psychiatry (2007) 62(8):925–33. doi: 10.1016/j.biopsych.2006.12.019
137. Miller A, Vo H, Huo L, Roca C, Schmidt PJ, Rubinow DR. Estrogen receptor alpha (ESR-1) associations with psychological traits in women with PMDD and controls. J Psychiatr Res (2010) 44(12):788–94. doi: 10.1016/j.jpsychires.2010.01.013
138. Pakharenko L. Effect of estrogen receptor gene ESR1 polymorphism on development of premenstrual syndrome. Georgian Med News (2014) 235):37–41. doi: 10.1016/j.pnpbp.2017.11.013
139. Yen JY, Wang PW, Su CH, Liu TL, Long CY, Ko CH. Estrogen levels, emotion regulation, and emotional symptoms of women with premenstrual dysphoric disorder: The moderating effect of estrogen receptor 1α polymorphism. Prog Neuropsychopharmacol Biol Psychiatry (2018) 82:216–23. doi: 10.1016/j.pnpbp.2017.11.013
140. Segebladh B, Bannbers E, Moby L, Nyberg S, Bixo M, Bäckström T, et al. Allopregnanolone serum concentrations and diurnal cortisol secretion in women with premenstrual dysphoric disorder. Arch Womens Ment Health (2013) 16(2):131–7. doi: 10.1007/s00737-013-0327-1
141. Kiesner J, Granger DA. A lack of consistent evidence for cortisol dysregulation in premenstrual syndrome/premenstrual dysphoric disorder. Psychoneuroendocrinology (2016) 65:149–64. doi: 10.1016/j.psyneuen.2015.12.009
142. Hou L, Huang Y, Zhou R. Premenstrual syndrome is associated with altered cortisol awakening response. Stress (2019) 22(6):640–6. doi: 10.1080/10253890.2019.1608943
143. Reame NE, Marshall JC, Kelch RP. Pulsatile LH secretion in women with premenstrual syndrome (PMS): evidence for normal neuroregulation of the menstrual cycle. Psychoneuroendocrinology (1992) 17(2-3):205–13. doi: 10.1016/0306-4530(92)90059-g
144. Parry BL, Hauger R, LeVeau B, Mostofi N, Cover H, Clopton P, et al. Circadian rhythms of prolactin and thyroid-stimulating hormone during the menstrual cycle and early versus late sleep deprivation in premenstrual dysphoric disorder. Psychiatry Res (1996) 62(2):147–60. doi: 10.1016/0165-1781(96)02905-8
145. Reiter RJ, Tamura H, Tan DX, Xu XY. Melatonin and the circadian system: contributions to successful female reproduction. Fertil Steril (2014) 102(2):321–8. doi: 10.1016/j.fertnstert.2014.06.014
146. Chuffa LG, Seiva FR, Fávaro WJ, Amorim JP, Teixeira GR, Mendes LO, et al. Melatonin and ethanol intake exert opposite effects on circulating estradiol and progesterone and differentially regulate sex steroid receptors in the ovaries, oviducts, and uteri of adult rats. Reprod Toxicol (2013) 39:40–9. doi: 10.1016/j.reprotox.2013.04.001
147. Minguini IP, Luquetti CM, Baracat MCP, Maganhin CC, Nunes CO, Simões RS, et al. Melatonin effects on ovarian follicular cells: a systematic review. Rev Assoc Med Bras (1992) (2019) 65(8):1122–7. doi: 10.1590/1806-9282.65.8.1122
148. Molis TM, Spriggs LL, Hill SM. Modulation of estrogen receptor mRNA expression by melatonin in MCF-7 human breast cancer cells. Mol Endocrinol (1994) 8(12):1681–90. doi: 10.1210/mend.8.12.7708056
149. Sen A, Hoffmann HM. Role of core circadian clock genes in hormone release and target tissue sensitivity in the reproductive axis. Mol Cell Endocrinol (2020) 501:110655. doi: 10.1016/j.mce.2019.110655
150. Rapkin AJ, Akopians AL. Pathophysiology of premenstrual syndrome and premenstrual dysphoric disorder. Menopause Int (2012) 18(2):52–9. doi: 10.1258/mi.2012.012014
151. Maguire JL, Stell BM, Rafizadeh M, Mody I. Ovarian cycle-linked changes in GABA(A) receptors mediating tonic inhibition alter seizure susceptibility and anxiety. Nat Neurosci (2005) 8(6):797–804. doi: 10.1038/nn1469
152. Stell BM, Brickley SG, Tang CY, Farrant M, Mody I. Neuroactive steroids reduce neuronal excitability by selectively enhancing tonic inhibition mediated by delta subunit-containing GABAA receptors. Proc Natl Acad Sci U.S.A. (2003) 100(24):14439–44. doi: 10.1073/pnas.2435457100
153. Griffiths J, Lovick T. Withdrawal from progesterone increases expression of alpha4, beta1, and delta GABA(A) receptor subunits in neurons in the periaqueductal gray matter in female wistar rats. J Comp Neurol (2005) 486(1):89–97. doi: 10.1002/cne.20540
154. Bixo M, Johansson M, Timby E, Michalski L, Bäckström T. Effects of GABA active steroids in the female brain with a focus on the premenstrual dysphoric disorder. J Neuroendocrinol (2018) 30(2). doi: 10.1111/jne.12553
155. Iba H, Watanabe T, Motomura S, Harada K, Uesugi H, Shibahara T, et al. A Japanese herbal medicine attenuates anxiety-like behavior through GABA(A) receptor and brain-derived neurotrophic factor expression in a rat model of premenstrual syndrome. J Pharmacol Sci (2021) 145(1):140–9. doi: 10.1016/j.jphs.2020.11.003
156. Bixo M, Ekberg K, Poromaa IS, Hirschberg AL, Jonasson AF, Andréen L, et al. Treatment of premenstrual dysphoric disorder with the GABA(A) receptor modulating steroid antagonist sepranolone (UC1010)-a randomized controlled trial. Psychoneuroendocrinology (2017) 80:46–55. doi: 10.1016/j.psyneuen.2017.02.031
157. Song C, Xue L. Roles of the µ-opioid receptor and its related signaling pathways in the pathogenesis of premenstrual syndrome liver-qi stagnation. Exp Ther Med (2017) 13(6):3130–6. doi: 10.3892/etm.2017.4374
158. Marrocco J, Einhorn NR, Petty GH, Li H, Dubey N, Hoffman J, et al. Epigenetic intersection of BDNF Val66Met genotype with premenstrual dysphoric disorder transcriptome in a cross-species model of estradiol add-back. Mol Psychiatry (2020) 25(3):572–83. doi: 10.1038/s41380-018-0274-3
159. Comasco E, Hahn A, Ganger S, Gingnell M, Bannbers E, Oreland L, et al. Emotional fronto-cingulate cortex activation and brain derived neurotrophic factor polymorphism in premenstrual dysphoric disorder. Hum Brain Mapp (2014) 35(9):4450–8. doi: 10.1002/hbm.22486
160. Borrow AP, Cameron NM. Estrogenic mediation of serotonergic and neurotrophic systems: implications for female mood disorders. Prog Neuropsychopharmacol Biol Psychiatry (2014) 54:13–25. doi: 10.1016/j.pnpbp.2014.05.009
161. Zhang L, Zhang HQ, Liang XY, Zhang HF, Zhang T, Liu FE. Melatonin ameliorates cognitive impairment induced by sleep deprivation in rats: role of oxidative stress, BDNF and CaMKII. Behav Brain Res (2013) 256:72–81. doi: 10.1016/j.bbr.2013.07.051
162. Bellofiore N, Cousins F, Temple-Smith P, Evans J. Altered exploratory behaviour and increased food intake in the spiny mouse before menstruation: a unique pre-clinical model for examining premenstrual syndrome. Hum Reprod (2019) 34(2):308–22. doi: 10.1093/humrep/dey360
Keywords: melatonin, premenstrual syndrome, circadian rhythms, ovarian steroid, cognition, gamma-aminobutyric acid
Citation: Yin W, Zhang J, Guo Y, Wu Z, Diao C and Sun J (2023) Melatonin for premenstrual syndrome: A potential remedy but not ready. Front. Endocrinol. 13:1084249. doi: 10.3389/fendo.2022.1084249
Received: 30 October 2022; Accepted: 12 December 2022;
Published: 09 January 2023.
Edited by:
Neil James MacLusky, University of Guelph, CanadaReviewed by:
Prashant Tarale, Rutgers, The State University of New Jersey, United StatesShan-Qiang Zhang, Yuebei People’s Hospital, China
Javier Ramirez Jirano, Centro de Investigación Biomédica de Occidente (CIBO), Mexico
Copyright © 2023 Yin, Zhang, Guo, Wu, Diao and Sun. This is an open-access article distributed under the terms of the Creative Commons Attribution License (CC BY). The use, distribution or reproduction in other forums is permitted, provided the original author(s) and the copyright owner(s) are credited and that the original publication in this journal is cited, in accordance with accepted academic practice. No use, distribution or reproduction is permitted which does not comply with these terms.
*Correspondence: Jinhao Sun, c3VuamluaGFvQHNkdS5lZHUuY24=
†These authors have contributed equally to this work
‡ORCID: Wei Yin, orcid.org/0000-0002-0294-0173
Jinhao Sun, orcid.org/0000-0001-6470-4312