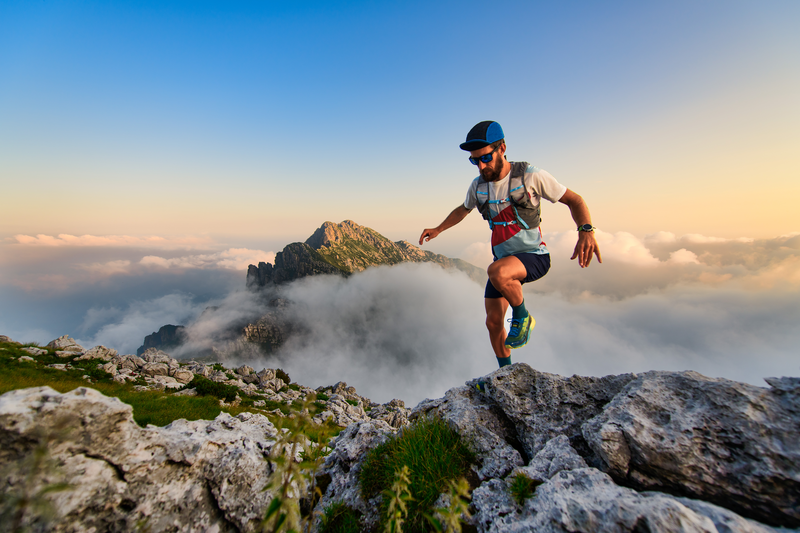
94% of researchers rate our articles as excellent or good
Learn more about the work of our research integrity team to safeguard the quality of each article we publish.
Find out more
REVIEW article
Front. Endocrinol. , 08 December 2022
Sec. Bone Research
Volume 13 - 2022 | https://doi.org/10.3389/fendo.2022.1081185
This article is part of the Research Topic Underlying Mechanisms and Treatment for Intervertebral Disc Disease View all 14 articles
Low back pain (LBP) is one of the most common musculoskeletal symptoms and severely affects patient quality of life. The majority of people may suffer from LBP during their life-span, which leading to huge economic burdens to family and society. According to the series of the previous studies, intervertebral disc degeneration (IDD) is considered as the major contributor resulting in LBP. Furthermore, non-coding RNAs (ncRNAs), mainly including microRNAs (miRNAs), long noncoding RNAs (lncRNAs) and circular RNAs (circRNAs), can regulate diverse cellular processes, which have been found to play pivotal roles in the development of IDD. However, the potential mechanisms of action for ncRNAs in the processes of IDD are still completely unrevealed. Therefore, it is challenging to consider ncRNAs to be used as the potential therapeutic targets for IDD. In this paper, we reviewed the current research progress and findings on ncRNAs in IDD: i). ncRNAs mainly participate in the process of IDD through regulating apoptosis of nucleus pulposus (NP) cells, metabolism of extracellular matrix (ECM) and inflammatory response; ii). the roles of miRNAs/lncRNAs/circRNAs are cross-talk in IDD development, which is similar to the network and can modulate each other; iii). ncRNAs have been attempted to combat the degenerative processes and may be promising as an efficient bio-therapeutic strategy in the future. Hence, this review systematically summarizes the principal pathomechanisms of IDD and shed light on the therapeutic potentials of ncRNAs in IDD.
Currently, low back pain (LBP) serves as one of the most prevalent musculoskeletal symptoms and has severe effects on the quality of life of individuals in the worldwide (1–3). Previous studies indicated that almost all people may have suffered from LBP during their life-span, resulting in substantial distress and economic burden (3, 4). With regard to the causes for LBP, it is still not completely unraveled, but intervertebral disc degeneration (IDD) is considered as the major contributor (5). IDD is a series of physiological and pathological changes occurring in the aging and degeneration of the intervertebral disc (IVD). A cross-sectional study indicated that almost 40% individuals suffered from IDD are less than 30 years, which is as high as 90% between 50 and 55 years (6). Nonetheless, there is lack of effective bio-therapeutic strategies for IDD and surgical intervention is hard to avoid in the final stage (7). Therefore, it is greatly necessary to clarify the underlying mechanisms of IDD at a cellular and molecular level.
IDD is a long and chronic process accompanying structural failure and progressive aging of the normal intervertebral disc (IVD), which is attributed to series of factors including lifestyle, aging, genetic predispositions and excessively mechanical loading (8, 9). IVD is the fibrocartilage tissue structure between each two vertebrae and serve as crucial role in maintaining the stability of the spine. In terms of anatomical structure, IVD is composed of nucleus pulposus (NP) cells, annulus fibrosus (AF) and cartilaginous endplate (CEP) (Figure 1). Accumulating evidences demonstrate that genetic and environmental factors contribute to IDD, but the exact molecular mechanisms are still largely unclear (10). The pathophysiological processes of IDD are mainly characterized by cells apoptosis, imbalance of extracellular matrix (ECM) and inflammatory response (Figure 1).
Figure 1 Schematic illustration of the anatomical structure of normal IVD and main pathophysiological features of IDD. IVD is composed of the central NP tissue, AF surrounding the NP, and CEP adhering the upper and lower vertebrae.The pathophysiological features of IDD are mainly characterized by cells apoptosis, imbalance of ECM and inflammatory response. IVD, intervertebral disc; IDD, intervertebral disc degeneration; CEP, cartilaginous endplate; AF, annulus fibrosus; NP, nucleus pulposus; ECM, extracellular matrix.
Noncoding RNAs (ncRNAs), primarily including microRNAs (miRNAs), long ncRNAs (lncRNAs) and circular RNAs (circRNAs), have been found to exert extensive effects on biological processes such as cell proliferation, apoptosis and ECM metabolism (4, 11, 12). Furthermore, compelling evidences supported that the expression of ncRNAs are significantly different between IDD and control samples, implicating ncRNAs play crucial roles in the development of IDD (13–15). Notably, novel ncRNAs have been constantly identified through microarray, RNA sequencing and reverse transcription-quantitative PCR (RT-qPCR) (16, 17), which has attracted a large number of interesting researcher’s attentions on the functions and specific molecular mechanisms of ncRNAs in IDD. There is no doubt that these works provide excellent value of understanding the precise roles of ncRNAs and display promising future for IDD bio-therapeutic strategy.
In this review, we systematically summarized the literature of past five years on ncRNAs in the pathophysiological processes of IDD, mainly involving miRNAs, lncRNAs and circRNAs. In addition, the application prospects of ncRNAs as bio-therapeutic strategy for effective treatment of IDD are also discussed.
MiRNAs, a group of endogenous ncRNAs with 20–24bp nucleotides in length, can regulate gene expression through recognizing and targeting the complementary 3′untranslated regions (3′UTRs) of particular mRNAs. Previous studies demonstrated that miRNAs were significantly differentially expressed between IDD and non-IDD, and acted as pivotal roles in the development of IDD (11, 18).
The apoptosis of NP cells is a typical feature of IDD and a myriad of studies reported that miRNAs mediated the NP cells apoptosis through regulating specific gene expression (18). Previously, Ji et al. firstly confirmed that miR-141 causes spontaneous progression of IDD by means of a study in miR-141 KO and wild-type mice (19). Mechanistically, miR-141 induced NP cell apoptosis and facilitated IDD progress via the regulation of downstream SIRT1/NF-κB axis (19). In addition, silencing of miR-141 had a protective effect on IDD mice, while upregulating miR-141 accelerated the development of IDD. Similar to miR-141, a large body of studies demonstrated that miRNAs were up-regulated in DD and aggravated NP cells apoptosis, such as miR-96/FRS2 (20), miR-4478/MTH1 (21) and miR-328-5p/WWP2 (22). On the contrary, there were substantial numbers of miRNAs were down-regulated and most likely exert anti-apoptotic effects in IDD, mainly involving miR-129-5p/BMP2 (23), miR-623/CXCL12 (24) and miR-155-3p/KDM3A/HIF1α (25). Therefore, miRNA-mediated apoptosis of NP cells affected the pathological process of IDD via downstream hub proteins or signal pathway. Notably, apart from anti-apoptosis, miR-623/CXCL12 axis inhibited senescence in LPS-induced NP cells (24), which suggested that miRNAs likely play an important role in IDD development by affecting cellular senescence.
Given the particular structure of IVD, CEP is essential for NP cells to acquire nutrition and prevent damaging factors into the disc. Previous studies showed that CEP cells apoptosis or calcification induced CEP degeneration, which resulted in nutrient loss and rendered IDD progress (26, 27). In a previous experiment, overexpression of miR-34a was evidenced to promote Fas-induced CEP cells apoptosis through inhibiting Bcl-2 (28). Additionally, miR-20a/ANKH-mediated stiff matrix enhanced calcification of CEP, whereas suppression of miR-20a alleviated the gene expression of calcification (29). Likewise, miR-221 positively regulated CEP cells apoptosis by targeting estrogen receptor α (ERα) (30). Based on the findings above, miRNAs have been shown to contribute to CEP cells apoptosis and calcification, which is closely pertinent to the development of IDD. However, recent literature demonstrated that miR-142-3p knockdown promoted apoptosis and autophagy of CEP cells by HMGB1 (31), implying that miR-142-3p is likely to have potential in protecting IDD. Apart from the foregoing mechanisms, miR-106a-5p also mediates AF cells apoptosis, which can be suppressed by melatonin (32).
Taken together, miRNAs-mediated cells apoptosis (NP, CEP and AF cells) regulates the onset of IDD, which provides potential targets for intervening IDD. Nevertheless, the roles of miRNAs in NP cells apoptosis attracted more attention. In the future, the related-studies should pay more attention to the effects of miRNAs on CEP and AF cells apoptosis.
ECM, a non-cellular three-dimensional macromolecular network predominantly composed of collagens and proteoglycan, is indispensable to maintain normal IVD cell function. With regard to the pathological process of IDD, the imbalance of ECM metabolism is a biological hallmark for IDD, which is characterized by synthesis decrease and degradation increase (9, 33). The metabolism of ECM is determined by proteolytic enzymes, such as matrix metalloproteinases (MMPs) and growth differentiation factor 5 (GDF5). miRNAs have been evidenced to play crucial roles in ECM degradation through modulating the activity of essential enzymes.
MMPs have acted as an essential factor for modulating metabolism of ECM. It has been reported that miR-127-5p was downregulated in degenerated NP tissues and inhibited the expression of type II collagen (34). Further experiment confirmed that miR-127-5p mediated the degradation of ECM components through enhancing MMP-13 expression. Another study found that the level of miR-210 remarkably increased in human degenerated NP cells, resulting in suppression of autophagy-related gene 7 (ATG7) and elevation of MMP-13 and MMP-3 (35). Notably, ATG7 knock-down seriously undermined the influences of miR-210 inhibitor on MMP-13 and MMP-3. These findings indicated that miR-210-induced ECM degradation was attributed to the proteolytic activity of upregulated MMP-13 and MMP-3 by directly targeting ATG7. Similarly, Wang et al. (36) also evidenced that miR-21 contributed to ECM catabolism by inhibiting autophagy through PTEN/Akt/mTOR signal pathway and elicited upregulation of MMP-9 and MMP-3 expression. Collectively, miRNAs play critical roles in MMPs-mediated metabolism of ECM via modulating autophagy.
GDF5, a crucial member of bone morphogenetic protein family, has been found to exert vital effects in musculoskeletal physiological process (37, 38). More importantly, recent publications also revealed that GDF5 provided protective effects against IDD through inhibiting ECM catabolism and promoting ECM anabolism (39, 40). Specifically, miR-132 contributed to the degradation of ECM by inhibiting GDF5, whereas antagomiR-132 protected ECM from degradation in IDD rats (39). Likewise, upregulation of miR-665 enhanced expression of catabolic genes (MMP13 and ADAMTS4) via specifically binding to GDF5, leading to ECM degradation (40). Consequently, GDF5 is likely to be an important target in ECM degradation caused by miRNAs.
It has been evidenced that SRY-related high mobility group box (SOX) is involved in the process of IDD (41). MiR-30d was found to be upregulated in degenerative NP tissues, and suppression of miR-30d resulted in hypoactive catabolism of ECM in vitro (42). Notably, bioinformatics analysis demonstrated that SOX9, an important transcription factor, was a direct target regulated by miR-30d. Interestingly, overexpression of miR-499a-5p is propitious to prevent NP cells apoptosis and ECM decomposition through repressing SOX4 expression (43). Although SOX9 and SOX4 belong to the transcription factors, they show opposite effects on ECM in the development of IDD. In addition, there are some miRNAs mediated ECM metabolism, such as miR-154/FGF14 (44), miR-145/ADAM17 (45) and miR-1260b/TCF7L2 (46) axis. This implicates a wide biofunction of miRNAs in the IDD process.
In summary, ECM metabolism mediated by miRNAs has affected the progress of IDD. Targeting miRNAs may be a promising approach for maintenance of the proper balance of ECM catabolism and anabolism, thereby delaying the pathological change of IDD.
Inflammation is widely envisioned as one of pathological features accompanying with IDD (47, 48). Up to now, accumulating evidence have also shown that multiple miRNAs are involved in regulating the inflammatory response of IDD (49–51).
Nuclear factor κB (NF-κB) is a key signal pathway in inflammatory response. The activation of NF-κB promotes the inflammatory cascade, leading to adverse environment for NP cells and impetus to degeneration of IVD (48). With regard to miRNAs-related to modulating inflammation, miR-16 was confirmed to negatively regulate the inflammation-related genes in LPS-induced NP cells, including COX-2, iNOS and PGE2 (51). Subsequently, target prediction found that TAB3 was directly regulated by miR-16, which was experimentally validated by a miRNA luciferase reporter assay. Besides, miR-16 attenuated the inflammation in LPS-mediated NP cells via inhibiting NF-κB and MAPK signal pathway. Likewise, MiR-223 was identified to share similar roles in LPS-treated NP cells through Irak1-mediated suppression of NF-κB (52). Apart from the above-mentioned, miR-15a-5p was found to aggravate the inflammation and apoptosis of NP cells by modulating NF-κB pathway (53). Hence, miRNAs can alleviate inflammation in NP cells by suppressing NF-κB. On the contrary, Dong et al. (54) demonstrated that miR-640 showed overt pro-inflammatory effects by enforcing activation of NF-κB, resulting in NP cells degeneration, conversely, inhibition of miR-640 displayed the opposite effects. Extracellular signal-regulated kinase (ERK) pathway has been evidenced to play key role in inhibiting inflammation (55). MiR-181a suppressed the expression of inflammatory factors through blocking ERK pathway in IDD mice (56), indicating that miR-181a affords protective effects in IDD.
Apart from mediated-apoptosis in IDD process, ERα is reported to a key player in modulating inflammation (57, 58). Specifically, the expression of miR-203-3p was positively correlated with the severity of IDD and negatively with Erα (58). Further evidence showed that ERα was the specific target of miR-203-3p and can be inhibited in LPS-stimulated NP cells, indicating that miR-203-3p was likely to aggravate intervertebral disc inflammation and degeneration through targeting ERα. These findings revealed that the same gene could exert different roles due to different miRNAs, implying the complexity and diversity of miRNA in regulation of IDD.
Additionally, other miRNAs were also demonstrated to link with inflammation in IDD progress. For example, miR-194-5p actively contributed to human IDD by targeting CUL4A and CUL4B and significantly decreased in inflammatory environment of IDD, indicating a negative regulation of miR-194-5p in the progression of IDD (59). In contrast, miR-125b-5p expression was found to be enforced in IL-1β-induced NP cells and human degenerating NP samples, which contributed to inflammation and NP cells apoptosis by monitoring TRIAP1 (60).
In summary, current studies demonstrate that miRNAs are key regulators and have important effects on the pathological cascades of IDD through intervening cells apoptosis, ECM metabolism and inflammation. In fact, the roles of miRNAs in the pathogenesis of IDD are not limited to the above-mentioned aspects. Latest studies indicate that miRNAs-mediated autophagy and ferroptosis are intimately linked with pathological progression of IDD. MiR-202-5p suppressed autophagy in degenerating NP cells through targeting ATG7 (61). Notably, Wu and colleagues (62) provided sufficient evidence that downregulation of miR-130b-3p promoted autophagy in NP cells and ameliorated IDD through ATG14/PRKAA1 in vivo and vitro. Ferroptosis, an iron-dependent type of programmed cell death, is associated with the pathogenesis of IDD. Overexpression of miR-10a-5p partially reversed IL-6-mediated ferroptosis in cartilage cells (63). In addition, latest evidence showed that inhibition of miR-874-3p positively modulated ferroptosis in NP cells by targeting activation transcription factor 3 (ATF3) (64). Undoubtedly, increasing studies revealed that miRNAs can mediate the initiation and progress of IDD (Table 1), indicating miRNAs-based therapy for IDD may be a promising strategy.
LncRNAs are a class of long noncoding RNAs whose transcript length exceeds 200 nucleotides in length. In general, lncRNAs are lack of capacity to code proteins, but share excellent regulation of gene expression through genetic, transcriptional and post-transcriptional modifications (65). Recently, emerging studies indicated that lncRNAs were associated with musculoskeletal degeneration diseases, such as osteoarthritis and IDD (4, 66). LncRNAs have exerted critical effects on the IDD by regulating cellular phenotype (cells proliferation, apoptosis, autophagy and ECM metabolism) through directly targeting hub gene expression and miRNAs.
It is well known that lncRNAs participate in the pathological changes of degenerative diseases by sponging specific miRNAs. LncRNA PART1 was verified to be significantly increased in NP cells isolated from IDD patients, indicating that PART1 probably impacted the degeneration of NP cells (67). Then, the expression of genes responsible for cells apoptosis, proliferation and ECM metabolism were evaluated. Results showed that lncRNA PART1 promoted NP cells apoptosis and ECM degradation, whereas cells proliferation and ECM synthesis were suppressed. Mechanistically, lncRNA PART1 competitively sponged miR-93, causing the degradation of NP cells by MMP2. Based on these findings, it can be inferred that lncRNAs play key role in IDD, albeit lack of in vivo evidence to support the potential role of PART1 for IDD. Zhong and team (68) found that lncRNA ADIRF-AS1/miR-214-3p/SERPINA1 pathway was negatively correlated with the severity of IDD, suggesting the protective role of ADIRF-AS1. Specifically, upregulation of ADIRF-AS1 enhanced NP cells viability and suppressed cellular senescence and apoptosis. Strikingly, latest study reported by Yu et al. (69) uncovered that lncRNA GAS5 was a principal contributor to NP cells apoptosis and catabolism of ECM through miR-17-3p/Ang-2 axis, eliciting the occurrence and progress of IDD. In addition, both inhibition of GAS5 and upregulation of miR-17-3p ameliorated IVDD in mice models. Collectively, in vitro and vivo studies afforded abundant supports that the lncRNA GAS5 might participate in IDD progress by miR-17-3p/Ang-2-mediated NP cells apoptosis and ECM degradation. Besides, inflammation and oxidative stress destroyed the homeostasis of IVD, enhancing the development of IDD. LncRNA MT1DP was found to mitigate anti-oxidation through miR-365/NRF-2 signal pathway, leading to NP cells apoptosis and IDD (70). Notably, LncRNA FAM83H-AS1 alleviated inflammatory response and promoted NP cells proliferation through miR-22-3p, preventing further deterioration of IDD in rat models caused by advanced glycation end products (71).
LncRNAs play important roles in regulating IDD through direct modulation of hub gene or signal pathway, ultimately triggering downstream cascades. Within the event, nuclear factor E2-related factor 2 (Nrf2) was reported to facilitate protecting NP cells from oxidative injury and preventing IDD deterioration (72, 73). Kang and colleagues (74) evidenced that upregulation of lncRNA ANPODRT attenuated oxidative stress and reduced tert-butyl hydroperoxide-stimulated apoptosis in NP cells, which could be attributed to the activation of Nrf2. As previously mentioned, miRNAs-mediated autophagy influenced the progress of IDD. As a consequence, lncRNAs may affect the process of IDD through autophagic pathway. HOTAIR, a novel lncRNA, was found to associate with autophagy and highly expressed in human NP tissue suffering from IDD (75). Furthermore, upregulation of HOTAIR potentiated autophagy via AMPK/mTOR/ULK1 signaling pathway in human NP cells, leading to NP cells apoptosis, senescence, and ECM degradation. More importantly, blocking HOTAIR attenuated the adverse effects in IDD rats. Interestingly, lncRNA HOTAIR could activate Wnt/β-catenin pathway and exert similar functions except for modulating AMPK/mTOR/ULK1 (76), implying lncRNA may act as key regulators of multiple downstream pathways.
At the present, an increasing number of lncRNAs have been found to involve in the pathological process of IDD, such as lncRNA SNHG6/miR-101-3p (77), lncRNA MIR155HG/miR-223-3p (78) and lncRNA H19/miR-139-3p/CXCR4/NF-κB (79) axes. In summary, these important roles of lncRNAs in IDD have been comprehensively verified (Table 2). Helpfully, these findings further elucidate the underlying mechanisms of IDD and shed light on lncRNAs as potential therapeutic target for treatment of IDD.
CircRNAs, another particular type of ncRNAs with the covalently closed loops, have attracted substantial attentions because of their excellent biological properties. Currently, there are a series of circRNAs have been evidenced to be pertinent to the underlying mechanisms of IDD (15, 80). As naturally formed endogenous non−coding RNAs, circRNAs serve as a competing endogenous RNAs and modulate the pathological process of IDD, mainly involving in cellular apoptosis, ECM metabolism and inflammation (80).
In the light of pathological mechanism of IDD, NP cells apoptosis is primary factor accelerating the pathological progression of IDD. Cheng and colleagues (81) observed that circVMA21 could depress the expression of apoptotic and catabolic genes, and enhance the collagen II and aggrecan expression in NP cells treated by inflammatory cytokines through miR-200c/XIAP pathway. In vivo injection of circVMA21 ameliorated the degeneration of NP tissues in rat model, suggesting the protective role of circVMA21/miR-200c/XIAP against IDD. Subsequently, Guo et al. (82) also verified that circGRB10 provided the beneficial effects in preventing IDD by reducing NP cell apoptosis through miR-328-5p/ERBB2 axis. Similarly, other studies demonstrated that cicrRNAs also afforded protection against IDD development, mainly including circRNA-CIDN/miR-34a-5p/SIRT1 (83), circGLCE/miR-587/STAP1 (84) and circARL15/miR-431-5p/DISC1 (85) pathway. On the contrary, recent studies showed that circRNAs facilitated apoptosis of NP cells. For instance, circ_001653 was significantly upregulated in degenerated NP tissues, which promoted the NP cells apoptosis and ECM degradation by miR-486-3p/CEMIP axis (86). In addition, a series of evidence indicated that circITCH induced apoptosis and mediated IDD through miR-17-5p/SOX4 axis (87).
As is known to all, the imbalance of ECM metabolism is detrimental for IDD. Considering that miRNAs play important role in modulating anabolism and catabolism of ECM, circRNAs also have been reported to associate with ECM metabolism through sponging miRNAs. Upregulation of circ-4099 promoted the ECM (collagen II and aggrecan) synthesis and restricted expression of pro-inflammatory factors (IL-1β, TNF-α, and PGE2) through targeting miR-616-5p/SOX9 (88). Likewise, circSEMA4B could alleviate ECM catabolism in IL-1β-induced NP cells via miR-431/GSK-3β/SFRP1 axis (89). Accordingly, these results support that circRNAs can prevent IDD through facilitating the anabolism of ECM. Nevertheless, circRNAs also share unfavorable roles in ECM metabolism and can induce ECM degradation. For instance, growing evidence showed that circRNA_104670 enhanced the expression of MMP-2 known to be associated with ECM catabolism, through miRNA-17-3p/MMP2 pathway, potentiating ECM degradation (90). Furthermore, circRNA_104670 and miRNA-17-3p was found to have excellent diagnostic significance for IDD based on the outcome of the receiver-operating characteristic curve.
Recently, accumulating studies found that circRNAs play vital roles in modulating inflammation response. It is well known that NF-κB is a classical inflammation-related pathway. Guo et al. (91) evidenced that circ-FAM169A mediated inflammatory cytokines (IL-1β and TNF-α) expression through BTRC/NF-κB axis, which induced NP cells apoptosis and ECM degradation. Based on above, there is no doubt that circRNAs play a crucial role in the regulation of miRNAs. Notwithstanding, whether multiple circRNAs can interplay and impact the biological process remain unclear. Strikingly, latest publication showed that circ_0040039 and circ_0004354 competitively regulated miR-345-3p/FAF1/TP73 pathway in IDD, initiating inflammation, ECM catabolism and pro-inflammation in NP cells (92). In detail, circ_0004354 showed stronger capacity to bind miR-345-3p when inflammatory cytokines reached lower level at the early phase of IDD. Subsequently, circ_0004354 was suppressed through negative feedback due to increase of inflammatory cytokines. Meanwhile, increased inflammatory cytokines induced circ_0040039 expression, which in turn inhibited the binding of circ_0004354 with miR-345-3p and augmented circ_0040039 ability to target miR-345-3p. Collectively, circ_0004354 and circ_0040039 showed different capacity to bind miR-345-3p relying on different concentration of inflammatory cytokines, eventually triggering inflammatory cascades and accelerating IDD progression.
Taken together, circRNAs display excellent ability to modulate the development of IDD through cells apoptosis, ECM metabolism and inflammation (Table 3). In addition, circRNAs also take important roles in affecting cellular senescence. For instance, latest research published by Wang et al. (93) reported that circ_7042 could prevent the IDD progression through inhibiting NP cells apoptosis, senescence and ECM degradation by absorption of miR-369-3p/BMP2/PI3K/Akt axis. Apart from sponging miRNAs, the interaction of different circRNAs may have impacts on the downstream pathway, which urgently needs more evidence to further verify.
In terms of current findings, dysregulation of ncRNAs mediates the onset and progression of IDD. More importantly, the exertion of functions regarding ncRNAs appears to be not completely independent of modulating the pathological process of IDD. LncRNAs and circRNAs, as particular ncRNAs, can directly sponge miRNAs and initiate a series of gene expression to regulate cells apoptosis, ECM metabolism and inflammation. Furthermore, there is a competitive relationship to bind miRNAs among multiple circRNAs. Collectively, the cross-talk of miRNAs/lncRNAs/circRNAs orchestrates IDD development (Figure 2), which is similar to the network and can modulate each other.
Figure 2 Schematic diagram of the cross-talk of miRNAs/lncRNAs/circRNAs orchestrating IDD development. LncRNAs/circRNAs directly sponge miRNAs and initiate a series of gene expression relating to IDD. Besides, miRNAs are able to modulate IDD independently through targeting key genes.
On the basis of understanding functions of ncRNAs, emerging evidence demonstrate that multiple strategies show excellent efficacy on treatment IDD through ncRNAs, mainly including stem cell therapy, exosomes, biomaterials and pharmacologic strategy (Figure 3).
Figure 3 A simplified scheme of ncRNA-based therapeutics for treatment of IDD. Stem cell, exosome, biomaterial and pharmacologic strategy, as carries, deliver loading endogenous and exogenous ncRNAs to degenerative intervertebral disc tissue. TCM, traditional Chinese medicine.
With more profound studies on stem cells, stem cell therapy is considered as a promising approach to intervene IDD owing to their capacity to release ncRNAs (94, 95). Shi and colleagues (96) found that bone marrow mesenchymal stem cells (BMSCs) enhanced the expression of autophagy-related genes and suppressed apoptosis-related genes in OGD NP cells through secreting miR-155, reducing NP cells apoptosis. Although they indicated that miRNA-derived from BMSCs probably afforded protective effects on NP cells in vitro, there is currently no in vivo evidence. Therefore, whether stem cells can improve IDD by ncRNAs needs further study. Intriguingly, a recent study demonstrated that BMSC-derived extracellular vesicles (BMSC-EVs) supported survival of NP cells and reduced ECM catabolism through circ_0050205/miR-665/GPX4 pathway in IDD mice (97). Hence, stem cell therapy may also be an effective strategy for treatment of IDD through ncRNAs.
Exosome, a kind of extracellular vesicles secreted by the majority of cell types, contains numerous bioactive components involved in intercellular communication. Accumulating studies have paid attention to the role of exosomal ncRNAs in treatment of IDD (98, 99). For instance, Cheng and colleagues (100) demonstrated that MSC-derived exosomes (MSC-exosomes) could be taken by NP cells and exerted cytoprotective effects against NP cells apoptosis. This was likely to be mainly ascribed to activation of miR-21/PI3K/Akt signal pathway. In rat IDD model, the injection of MSC-exosomes reduced NP cells apoptosis and delayed IDD deterioration, showing the therapeutic potential of MSC exosomes in IDD. Recently, Chen et al. (101) also extracted exosomes from cartilage endplate stem cells (CESC-exosomes) and found that CESC-exosomes can suppress NP cells apoptosis and ECM degradation through delivery of miR-125-5p. Although exosomal ncRNAs show greatly therapeutic potential in treatment of IDD, obtaining highly purified exosomes in large quantities still requires further investigation. Hence, further study needs to be conducted to bail out current dilemma for clinical application.
Biomaterial-based strategies have attracted increasing attention in the field of disc pro-regeneration (102, 103). Existing studies indicate that biomaterials have shown remarkable potential to arrest IDD owing to their unique biological properties including excellent biocompatibility and mechanical properties (103). Hydrogel, a particular biomaterial, is similar to the natural extracellular matrix and has been widely used to clinical trials. Feng and colleagues (104) designed a kind of polyplex micelle-encapsulated hydrogel that could encapsulate miR-29a and prevent miR-29a from spillage and degradation in vivo. In rabbit IDD models, the injection of miR-29a/polyplex reversed IDD through the suppression of MMP-2/β-catenin signal pathway. Lipid nanocapsules (LNC), as a particular carrier, can load and release certain miRNAs into cells. Regarding the point, miR-155-loaded LNC (miR-155 LNC) has been devised to evaluate the potential for treatment of IDD in vitro and in vivo (105). Intriguingly, their results showed that miR-155 LNC could be internalized in NP cells and maintain bioactivity. Moreover, in vivo experiments, injection of miR-155 LNC was proven to be safe and feasible. Unfortunately, they didn’t further investigate the specific effects of miR-155 LNC on IDD progress. Collectively, biomaterials show great potential for treatment of IDD through delivery of ncRNAs.
At the present, pharmacological intervention is also considered as adjuvant therapy alternatives for IDD, such as traditional Chinese medicine (TCM) and natural products (106, 107). Notably, Yang et al. (108) found that aucubin, an active ingredient of eucommia ulmoides, could prevent the ECM degradation in human NP cells treated by IL‐1β or TNF‐α. Concomitantly, further experiments verified that miR-140/CREB1 signal pathway participated in aucubin-mediated protection against IDD (108). In addition, latest study demonstrated that arctigenin shared multiple roles in anti-IDD through up-regulating miR-483-3p, including reduction of NP cells apoptosis, ECM catabolism and inflammation-related genes expression (109). Consequently, these vitro findings afford a novel pharmacological strategy based on ncRNAs for treatment IDD. Although ncRNAs can be envisioned as pharmacological targets for the management of IDD, whether pharmacologic strategy-linked with ncRNAs exerts positive effects in vivo due to the complicated internal environment. Therefore, future study should focus on the in vivo effects and screen the determined ncRNAs for potential treatment of IDD.
Up to now, ncRNA-based therapeutics have made enormous progress, but present studies focused only on NP cells or rodents with IDD models. In fact, the biomechanical properties of intervertebral disc are obviously different between human and rodents. Therefore, it is essential to investigate the efficacy of ncRNA-based therapy in the IDD models conforming to the human biomechanical properties.
Intervertebral disc degeneration is a main contributor to chronic low back pain. With regard to the pathological features, many factors involved in the progression of IDD, mainly focused on cells apoptosis, ECM metabolism and inflammation (9, 11). Increasing evidence have indicated that ncRNAs involve in the initiation and development of IDD, which hints the important potential of ncRNAs for IDD of treatment (110). In present review, we summarized the functions of ncRNAs in IDD and found that ncRNAs acted as pivotal regulators in the pathological process of IDD. On one hand, ncRNAs play positive roles in delaying or reversing IDD progression through inhibiting cells apoptosis, ECM catabolism and inflammatory cascades. On the other hand, ncRNAs also cause IDD deterioration by promoting cells apoptosis, ECM degradation and pro-inflammatory cytokines secretion. In addition, ncRNAs have exerted effects on IDD by regulating autophagy and ferroptosis. In fact, the ncRNAs to IDD is a double-edge sword, which depends on the downstream hub gene or signal pathway. Critically, multiple ncRNAs can regulate the same target genes or the different targets can be modulated by the same ncRNAs, like network, which jointly participates in IDD. Based on the existing evidence, ncRNAs have been attempted to manage the degenerative processes and shown positive efficacy though delivery of ncRNAs.
To our best knowledge, it is requisite for clinical application to develop drug targeting ncRNAs and conduct large-scale studies. Current studies demonstrate that a variety of ncRNAs are involved in the process of IDD (15, 80), but the decisive ncRNAs in IDD remain unclear. Furthermore, most studies still focused on the NP cells or IDD models. Therefore, ncRNAs-based therapy is still in the preclinical stage. Apart from clinical treatment, whether ncRNAs can be considered as clinical biomarkers for diagnosis is not fully elucidated, diagnosis of IDD at early stage is still challenging. In the future, research should pay more attention to the following aspects: i). the roles of ncRNAs should be explored in depth to better understand the underlying mechanisms of IDD; ii). screening of the ncRNAs with diagnostic value; iii). design of the IDD models that are similar to human biomechanical properties.
In conclusion, the present studies have demonstrated that ncRNAs are hub regulators mediating the onset and progression of IDD. In this review, we have systematically reviewed recent advances in related fields and summarized the role of ncRNAs in IDD. Further, we have discussed the ncRNAs-based strategy for treatment of IDD, which sheds light on the preface of switching theoretical strategy toward actually clinical application. Lastly, considering the current research advance in ncRNAs for treatment of IDD, we have analyzed the issues that need to be paid more attention in future research, producing meaningful ideas for next studies. Based on the aforementioned, ncRNAs, as novel therapeutic targets for IDD, may possess an excellent prospect. Comprehensive understanding the function of ncRNAs in IDD is critical for exploring biological therapies for treatment of IDD.
HY and DH conceived of the review and supervised the project. CJ, ZC and XW wrote the manuscript and drew figures. YZ and XG contributed to literature review and editing. ZX contributed to compiled table. All authors contributed to the article and approved the submitted version.
This work was supported by the National Natural Science Foundation of China (Grants No. 82071551 and 81830077).
The authors declare that the research was conducted in the absence of any commercial or financial relationships that could be construed as a potential conflict of interest.
All claims expressed in this article are solely those of the authors and do not necessarily represent those of their affiliated organizations, or those of the publisher, the editors and the reviewers. Any product that may be evaluated in this article, or claim that may be made by its manufacturer, is not guaranteed or endorsed by the publisher.
1. Suman A, Schaafsma F, Elders P, van Tulder M, Anema J. Cost-effectiveness of a multifaceted implementation strategy for the Dutch multidisciplinary guideline for nonspecific low back pain: Design of a stepped-wedge cluster randomised controlled trial. BMC Public Health (2015) 15:522. doi: 10.1186/s12889-015-1876-1
2. Vos T, Abajobir AA, Abbafati C, Abbas KM, Abate KH, Abd-Allah F, et al. Global, regional, and national incidence, prevalence, and years lived with disability for 328 diseases and injuries for 195 countries, 1990-2016: A systematic analysis for the global burden of disease study 2016. Lancet (2017) 390(10100):1211–59. doi: 10.1016/s0140-6736(17)32154-2
3. Guo H, Guo M, Wan Z, Song F, Wang H. Emerging evidence on noncoding-rna regulatory machinery in intervertebral disc degeneration: A narrative review. Arthritis Res Ther (2020) 22(1):270. doi: 10.1186/s13075-020-02353-2
4. Li Z, Li X, Chen C, Li S, Shen J, Tse G, et al. Long non-coding rnas in nucleus pulposus cell function and intervertebral disc degeneration. Cell proliferation (2018) 51(5):e12483. doi: 10.1111/cpr.12483
5. Luoma K, Riihimäki H, Luukkonen R, Raininko R, Viikari-Juntura E, Lamminen A. Low back pain in relation to lumbar disc degeneration. Spine (2000) 25(4):487–92. doi: 10.1097/00007632-200002150-00016
6. Cheung K, Karppinen J, Chan D, Ho D, Song Y, Sham P, et al. Prevalence and pattern of lumbar magnetic resonance imaging changes in a population study of one thousand forty-three individuals. Spine (2009) 34(9):934–40. doi: 10.1097/BRS.0b013e3181a01b3f
7. Jiang C, Yin S, Wei J, Zhao W, Wang X, Zhang Y, et al. Full-endoscopic posterior lumbar interbody fusion with epidural anesthesia: Technical note and initial clinical experience with one-year follow-up. J Pain Res (2021) 14:3815–26. doi: 10.2147/jpr.S338027
8. Setton L, Chen J. Mechanobiology of the intervertebral disc and relevance to disc degeneration. J Bone Joint Surg Am volume (2006) 88-A(Suppl 2):52–7. doi: 10.2106/jbjs.F.00001
9. Cazzanelli P, Wuertz-Kozak K. Micrornas in intervertebral disc degeneration, apoptosis, inflammation, and mechanobiology. Int J Mol Sci (2020) 21(10):3601. doi: 10.3390/ijms21103601
10. Kadow T, Sowa G, Vo N, Kang J. Molecular basis of intervertebral disc degeneration and herniations: What are the important translational questions? Clin orthopaedics related Res (2015) 473(6):1903–12. doi: 10.1007/s11999-014-3774-8
11. Wang C, Wang WJ, Yan YG, Xiang YX, Zhang J, Tang ZH, et al. Micrornas: New players in intervertebral disc degeneration. Clin Chim Acta (2015) 450:333–41. doi: 10.1016/j.cca.2015.09.011
12. Li Z, Chen X, Xu D, Li S, Chan M, Wu W. Circular rnas in nucleus pulposus cell function and intervertebral disc degeneration. Cell proliferation (2019) 52(6):e12704. doi: 10.1111/cpr.12704
13. Li Y, Zhou S, Peng P, Wang X, Du L, Huo Z, et al. Emerging role of circular rna in intervertebral disc degeneration: Knowns and unknowns (Review). Mol Med Rep (2020) 22(4):3057–65. doi: 10.3892/mmr.2020.11437
14. Zheng Y, Song G, Guo J, Su X, Chen Y, Yang Z, et al. Interactions among Lncrna/Circrna, mirna, and mrna in musculoskeletal degenerative diseases. Front Cell Dev Biol (2021) 9:753931. doi: 10.3389/fcell.2021.753931
15. Zou F, Ding Z, Jiang J, Lu F, Xia X, Ma X. Confirmation and preliminary analysis of circrnas potentially involved in human intervertebral disc degeneration. Mol Med Rep (2017) 16(6):9173–80. doi: 10.3892/mmr.2017.7718
16. Beermann J, Piccoli MT, Viereck J, Thum T. Non-coding rnas in development and disease: Background, mechanisms, and therapeutic approaches. Physiol Rev (2016) 96(4):1297–325. doi: 10.1152/physrev.00041.2015
17. Yao R-W, Wang Y, Chen L-L. Cellular functions of long noncoding rnas. Nat Cell Biol (2019) 21(5):542–51. doi: 10.1038/s41556-019-0311-8
18. Sherafatian M, Abdollahpour HR, Ghaffarpasand F, Yaghmaei S, Azadegan M, Heidari M. Microrna expression profiles, target genes, and pathways in intervertebral disk degeneration: A meta-analysis of 3 microarray studies. World Neurosurg (2019) 126:389–97. doi: 10.1016/j.wneu.2019.03.120
19. Ji ML, Jiang H, Zhang XJ, Shi PL, Li C, Wu H, et al. Preclinical development of a microrna-based therapy for intervertebral disc degeneration. Nat Commun (2018) 9(1):5051. doi: 10.1038/s41467-018-07360-1
20. Yang X, Liu H, Zhang Q, Liu K, Yu D, Zhang Y, et al. Mir-96 promotes apoptosis of nucleus pulpous cells by targeting Frs2. Hum Cell (2020) 33(4):1017–25. doi: 10.1007/s13577-020-00389-9
21. Zhang J, Liu R, Mo L, Liu C, Jiang J. Mir-4478 accelerates nucleus pulposus cells apoptosis induced by oxidative stress by targeting Mth1. Spine (2022). doi: 10.1097/brs.0000000000004486
22. Yan J, Wu LG, Zhang M, Fang T, Pan W, Zhao JL, et al. Mir-328-5p induces human intervertebral disc degeneration by targeting Wwp2. Oxid Med Cell Longev (2022) 2022:3511967. doi: 10.1155/2022/3511967
23. Yang W, Sun P. Downregulation of microrna-129-5p increases the risk of intervertebral disc degeneration by promoting the apoptosis of nucleus pulposus cells Via targeting Bmp2. J Cell Biochem (2019) 120(12):19684–90. doi: 10.1002/jcb.29274
24. Zhong H, Zhou Z, Guo L, Liu F, Zheng B, Bi S, et al. The mir-623/Cxcl12 axis inhibits lps-induced nucleus pulposus cell apoptosis and senescence. Mech Ageing Dev (2021) 194:111417. doi: 10.1016/j.mad.2020.111417
25. Zhou X, Li J, Teng J, Liu Y, Zhang D, Liu L, et al. Microrna-155-3p attenuates intervertebral disc degeneration Via inhibition of Kdm3a and Hif1α. Inflammation Res (2021) 70(3):297–308. doi: 10.1007/s00011-021-01434-5
26. Zhao X, Xu B, Duan W, Chang L, Tan R, Sun Z, et al. Insights into exosome in the intervertebral disc: Emerging role for disc homeostasis and normal function. Int J Med Sci (2022) 19(11):1695–705. doi: 10.7150/ijms.75285
27. Grant MP, Epure LM, Bokhari R, Roughley P, Antoniou J, Mwale F. Human cartilaginous endplate degeneration is induced by calcium and the extracellular calcium-sensing receptor in the intervertebral disc. Eur Cell Mater (2016) 32:137–51. doi: 10.22203/ecm.v032a09
28. Chen H, Wang J, Hu B, Wu X, Chen Y, Li R, et al. Mir-34a promotes fas-mediated cartilage endplate chondrocyte apoptosis by targeting bcl-2. Mol Cell Biochem (2015) 406(1-2):21–30. doi: 10.1007/s11010-015-2420-4
29. Liu MH, Sun C, Yao Y, Fan X, Liu H, Cui YH, et al. Matrix stiffness promotes cartilage endplate chondrocyte calcification in disc degeneration Via mir-20a targeting ankh expression. Sci Rep (2016) 6:25401. doi: 10.1038/srep25401
30. Sheng B, Yuan Y, Liu X, Zhang Y, Liu H, Shen X, et al. Protective effect of estrogen against intervertebral disc degeneration is attenuated by mir-221 through targeting estrogen receptor α. Acta Biochim Biophys Sin (Shanghai) (2018) 50(4):345–54. doi: 10.1093/abbs/gmy017
31. Wang B, Ji D, Xing W, Li F, Huang Z, Zheng W, et al. Mir-142-3p and Hmgb1 are negatively regulated in proliferation, apoptosis, migration, and autophagy of cartilage endplate cells. Cartilage (2021) 13(2_suppl):592s–603s. doi: 10.1177/19476035211012444
32. Hai B, Ma Y, Pan X, Yong L, Liang C, He G, et al. Melatonin benefits to the growth of human annulus fibrosus cells through inhibiting mir-106a-5p/Atg7 signaling pathway. Clin Interv Aging (2019) 14:621–30. doi: 10.2147/cia.S193765
33. Wang XQ, Tu WZ, Guo JB, Song G, Zhang J, Chen CC, et al. A bioinformatic analysis of micrornas' role in human intervertebral disc degeneration. Pain Med (2019) 20(12):2459–71. doi: 10.1093/pm/pnz015
34. Hua WB, Wu XH, Zhang YK, Song Y, Tu J, Kang L, et al. Dysregulated mir-127-5p contributes to type ii collagen degradation by targeting matrix metalloproteinase-13 in human intervertebral disc degeneration. Biochimie (2017) 139:74–80. doi: 10.1016/j.biochi.2017.05.018
35. Wang C, Zhang ZZ, Yang W, Ouyang ZH, Xue JB, Li XL, et al. Mir-210 facilitates ecm degradation by suppressing autophagy Via silencing of Atg7 in human degenerated Np cells. BioMed Pharmacother (2017) 93:470–9. doi: 10.1016/j.biopha.2017.06.048
36. Wang WJ, Yang W, Ouyang ZH, Xue JB, Li XL, Zhang J, et al. Mir-21 promotes ecm degradation through inhibiting autophagy Via the Pten/Akt/Mtor signaling pathway in human degenerated Np cells. BioMed Pharmacother (2018) 99:725–34. doi: 10.1016/j.biopha.2018.01.154
37. Chujo T, An HS, Akeda K, Miyamoto K, Muehleman C, Attawia M, et al. Effects of growth differentiation factor-5 on the intervertebral disc–in vitro bovine study and in vivo rabbit disc degeneration model study. Spine (Phila Pa 1976) (2006) 31(25):2909–17. doi: 10.1097/01.brs.0000248428.22823.86
38. Lv B, Gan W, Cheng Z, Wu J, Chen Y, Zhao K, et al. Current insights into the maintenance of structure and function of intervertebral disc: A review of the regulatory role of growth and differentiation factor-5. Front Pharmacol (2022) 13:842525. doi: 10.3389/fphar.2022.842525
39. Liu W, Xia P, Feng J, Kang L, Huang M, Wang K, et al. Microrna-132 upregulation promotes matrix degradation in intervertebral disc degeneration. Exp Cell Res (2017) 359(1):39–49. doi: 10.1016/j.yexcr.2017.08.011
40. Tan H, Zhao L, Song R, Liu Y, Wang L. Microrna-665 promotes the proliferation and matrix degradation of nucleus pulposus through targeting Gdf5 in intervertebral disc degeneration. J Cell Biochem (2018) 119(9):7218–25. doi: 10.1002/jcb.26888
41. Gruber HE, Norton HJ, Ingram JA, Hanley EN Jr. The Sox9 transcription factor in the human disc: Decreased immunolocalization with age and disc degeneration Spine (2005) 30(6):625–30 doi: 10.1097/01.brs.0000155420.01444.c6
42. Lv J, Li S, Wan T, Yang Y, Cheng Y, Xue R. Inhibition of microrna-30d attenuates the apoptosis and extracellular matrix degradation of degenerative human nucleus pulposus cells by up-regulating Sox9. Chem Biol Interact (2018) 296:89–97. doi: 10.1016/j.cbi.2018.09.010
43. Sun JC, Zheng B, Sun RX, Meng YK, Wang SM, Yang HS, et al. Mir-499a-5p suppresses apoptosis of human nucleus pulposus cells and degradation of their extracellular matrix by targeting Sox4. BioMed Pharmacother (2019) 113:108652. doi: 10.1016/j.biopha.2019.108652
44. Wang J, Liu X, Sun B, Du W, Zheng Y, Sun Y. Upregulated mir-154 promotes ecm degradation in intervertebral disc degeneration. J Cell Biochem (2019). 120(7):11900–11907 doi: 10.1002/jcb.28471
45. Zhou J, Sun J, Markova DZ, Li S, Kepler CK, Hong J, et al. Microrna-145 overexpression attenuates apoptosis and increases matrix synthesis in nucleus pulposus cells. Life Sci (2019) 221:274–83. doi: 10.1016/j.lfs.2019.02.041
46. Chen S, Shi G, Zeng J, Li PH, Peng Y, Ding Z, et al. Mir-1260b protects against lps-induced degenerative changes in nucleus pulposus cells through targeting Tcf7l2. Hum Cell (2022) 35(3):779–91. doi: 10.1007/s13577-021-00655-4
47. Navone SE, Marfia G, Giannoni A, Beretta M, Guarnaccia L, Gualtierotti R, et al. Inflammatory mediators and signalling pathways controlling intervertebral disc degeneration. Histol Histopathol (2017) 32(6):523–42. doi: 10.14670/hh-11-846
48. Wuertz K, Vo N, Kletsas D, Boos N. Inflammatory and catabolic signalling in intervertebral discs: The roles of nf-κb and map kinases. Eur Cell Mater (2012) 23:103–19. doi: 10.22203/ecm.v023a08
49. O'Connell RM, Rao DS, Baltimore D. Microrna regulation of inflammatory responses. Annu Rev Immunol (2012) 30:295–312. doi: 10.1146/annurev-immunol-020711-075013
50. Liang H, Yang X, Liu C, Sun Z, Wang X. Effect of nf-kb signaling pathway on the expression of mif, tnf-α, il-6 in the regulation of intervertebral disc degeneration. J Musculoskelet Neuronal Interact (2018) 18(4):551–6.
51. Du K, He X, Deng J. Microrna-16 inhibits the lipopolysaccharide-induced inflammatory response in nucleus pulposus cells of the intervertebral disc by targeting Tab3. Arch Med Sci (2021) 17(2):500–13. doi: 10.5114/aoms.2018.74950
52. Wang H, Hao P, Zhang H, Xu C, Zhao J. Microrna-223 inhibits lipopolysaccharide-induced inflammatory response by directly targeting Irak1 in the nucleus pulposus cells of intervertebral disc. IUBMB Life (2018) 70(6):479–90. doi: 10.1002/iub.1747
53. Zhang S, Song S, Zhuang Y, Hu J, Cui W, Wang X, et al. Role of microrna-15a-5p/Sox9/Nf-κb axis in inflammatory factors and apoptosis of murine nucleus pulposus cells in intervertebral disc degeneration. Life Sci (2021) 277:119408. doi: 10.1016/j.lfs.2021.119408
54. Dong W, Liu J, Lv Y, Wang F, Liu T, Sun S, et al. Mir-640 aggravates intervertebral disc degeneration Via nf-κb and wnt signalling pathway. Cell Prolif (2019) 52(5):e12664. doi: 10.1111/cpr.12664
55. Zhang HJ, Liao HY, Bai DY, Wang ZQ, Xie XW. Mapk /Erk signaling pathway: A potential target for the treatment of intervertebral disc degeneration. BioMed Pharmacother (2021) 143:112170. doi: 10.1016/j.biopha.2021.112170
56. Sun Y, Shi X, Peng X, Li Y, Ma H, Li D, et al. Microrna-181a exerts anti-inflammatory effects Via inhibition of the erk pathway in mice with intervertebral disc degeneration. J Cell Physiol (2020) 235(3):2676–86. doi: 10.1002/jcp.29171
57. Jia M, Dahlman-Wright K, Gustafsson J. Estrogen receptor alpha and beta in health and disease. Best Pract Res Clin Endocrinol Metab (2015) 29(4):557–68. doi: 10.1016/j.beem.2015.04.008
58. Cai Z, Li K, Yang K, Luo D, Xu H. Suppression of mir-203-3p inhibits lipopolysaccharide induced human intervertebral disc inflammation and degeneration through upregulating estrogen receptor α. Gene Ther (2020) 27(9):417–26. doi: 10.1038/s41434-019-0118-z
59. Chen Z, Han Y, Deng C, Chen W, Jin L, Chen H, et al. Inflammation-dependent downregulation of mir-194-5p contributes to human intervertebral disc degeneration by targeting Cul4a and Cul4b. J Cell Physiol (2019) 234(11):19977–89. doi: 10.1002/jcp.28595
60. Jie J, Xu X, Li W, Wang G. Regulation of apoptosis and inflammatory response in interleukin-1β-Induced nucleus pulposus cells by mir-125b-5p Via targeting Triap1. Biochem Genet (2021) 59(2):475–90. doi: 10.1007/s10528-020-10009-8
61. Chen J, Sun Q, Liu GZ, Zhang F, Liu CY, Yuan QM, et al. Effect of mir-202-5p-Mediated Atg7 on autophagy and apoptosis of degenerative nucleus pulposus cells. Eur Rev Med Pharmacol Sci (2020) 24(2):517–25. doi: 10.26355/eurrev_202001_20027
62. Wu T, Jia X, Zhu Z, Guo K, Wang Q, Gao Z, et al. Inhibition of mir-130b-3p restores autophagy and attenuates intervertebral disc degeneration through mediating Atg14 and Prkaa1. Apoptosis (2022) 27(5-6):409–25. doi: 10.1007/s10495-022-01725-0
63. Bin S, Xin L, Lin Z, Jinhua Z, Rui G, Xiang Z. Targeting mir-10a-5p/Il-6r axis for reducing il-6-Induced cartilage cell ferroptosis. Exp Mol Pathol (2021) 118:104570. doi: 10.1016/j.yexmp.2020.104570
64. Li Y, Pan D, Wang X, Huo Z, Wu X, Li J, et al. Silencing Atf3 might delay tbhp-induced intervertebral disc degeneration by repressing npc ferroptosis, apoptosis, and ecm degradation. Oxid Med Cell Longev (2022) 2022:4235126. doi: 10.1155/2022/4235126
65. Brosnan CA, Voinnet O. The long and the short of noncoding rnas. Curr Opin Cell Biol (2009) 21(3):416–25. doi: 10.1016/j.ceb.2009.04.001
66. Zhao B, Lu M, Wang D, Li H, He X. Genome-wide identification of long noncoding rnas in human intervertebral disc degeneration by rna sequencing. BioMed Res Int (2016) 2016:3684875. doi: 10.1155/2016/3684875
67. Gao D, Hao L, Zhao Z. Long non-coding rna Part1 promotes intervertebral disc degeneration through regulating the mir−93/Mmp2 pathway in nucleus pulposus cells. Int J Mol Med (2020) 46(1):289–99. doi: 10.3892/ijmm.2020.4580
68. Zhong H, Zhou Z, Guo L, Liu FS, Wang X, Li J, et al. Serpina1 is a hub gene associated with intervertebral disc degeneration grade and affects the nucleus pulposus cell phenotype through the adirf-As1/Mir-214-3p axis. Transl Res (2022) 245:99–116. doi: 10.1016/j.trsl.2022.02.006
69. Yu X, Liu Q, Wang Y, Bao Y, Jiang Y, Li M, et al. Depleted long noncoding rna Gas5 relieves intervertebral disc degeneration Via microrna-17-3p/Ang-2. Oxid Med Cell Longev (2022) 2022:1792412. doi: 10.1155/2022/1792412
70. Liao ZW, Fan ZW, Huang Y, Liang CY, Liu C, Huang S, et al. Long non-coding rna Mt1dp interacts with mir-365 and induces apoptosis of nucleus pulposus cells by repressing nrf-2-Induced anti-oxidation in lumbar disc herniation. Ann Transl Med (2021) 9(2):151. doi: 10.21037/atm-20-8123
71. Jiang X, Chen D. Lncrna Fam83h-As1 maintains intervertebral disc tissue homeostasis and attenuates inflammation-related pain Via promoting nucleus pulposus cell growth through mir-22-3p inhibition. Ann Transl Med (2020) 8(22):1518. doi: 10.21037/atm-20-7056
72. Zhang CY, Hu XC, Zhang GZ, Liu MQ, Chen HW, Kang XW. Role of Nrf2 and ho-1 in intervertebral disc degeneration. Connect Tissue Res (2022) 63(6):559–76. doi: 10.1080/03008207.2022.2089565
73. Xiang Q, Zhao Y, Lin J, Jiang S, Li W. The Nrf2 antioxidant defense system in intervertebral disc degeneration: Molecular insights. Exp Mol Med (2022) 54(8):1067–75. doi: 10.1038/s12276-022-00829-6
74. Kang L, Tian Y, Guo X, Chu X, Xue Y. Long noncoding rna anpodrt overexpression protects nucleus pulposus cells from oxidative stress and apoptosis by activating Keap1-Nrf2 signaling. Oxid Med Cell Longev (2021) 2021:6645005. doi: 10.1155/2021/6645005
75. Zhan S, Wang K, Xiang Q, Song Y, Li S, Liang H, et al. Lncrna hotair upregulates autophagy to promote apoptosis and senescence of nucleus pulposus cells. J Cell Physiol (2020) 235(3):2195–208. doi: 10.1002/jcp.29129
76. Zhan S, Wang K, Song Y, Li S, Yin H, Luo R, et al. Long non-coding rna hotair modulates intervertebral disc degenerative changes Via Wnt/β-catenin pathway. Arthritis Res Ther (2019) 21(1):201. doi: 10.1186/s13075-019-1986-8
77. Gao ZX, Lin YC, Wu ZP, Zhang P, Cheng QH, Ye LH, et al. Lncrna Snhg6 can regulate the proliferation and apoptosis of rat degenerate nucleus pulposus cells Via regulating the expression of mir-101-3p. Eur Rev Med Pharmacol Sci (2020) 24(16):8251–62. doi: 10.26355/eurrev_202008_22621
78. Yang W, Huang XD, Zhang T, Zhou YB, Zou YC, Zhang J. Lncrna Mir155hg functions as a cerna of mir-223-3p to promote cell pyroptosis in human degenerative Np cells. Clin Exp Immunol (2022) 207(2):241–52. doi: 10.1093/cei/uxab030
79. Sun Z, Tang X, Wang H, Sun H, Chu P, Sun L, et al. Lncrna H19 aggravates intervertebral disc degeneration by promoting the autophagy and apoptosis of nucleus pulposus cells through the mir-139/Cxcr4/Nf-κb axis. Stem Cells Dev (2021) 30(14):736–48. doi: 10.1089/scd.2021.0009
80. Xu D, Ma X, Sun C, Han J, Zhou C, Wong SH, et al. Circular rnas in intervertebral disc degeneration: An updated review. Front Mol Biosci (2021) 8:781424. doi: 10.3389/fmolb.2021.781424
81. Cheng X, Zhang L, Zhang K, Zhang G, Hu Y, Sun X, et al. Circular rna Vma21 protects against intervertebral disc degeneration through targeting mir-200c and X linked inhibitor-of-Apoptosis protein. Ann Rheum Dis (2018) 77(5):770–9. doi: 10.1136/annrheumdis-2017-212056
82. Guo W, Zhang B, Mu K, Feng SQ, Dong ZY, Ning GZ, et al. Circular rna Grb10 as a competitive endogenous rna regulating nucleus pulposus cells death in degenerative intervertebral disk. Cell Death Dis (2018) 9(3):319. doi: 10.1038/s41419-017-0232-z
83. Xiang Q, Kang L, Wang J, Liao Z, Song Y, Zhao K, et al. Circrna-cidn mitigated compression loading-induced damage in human nucleus pulposus cells Via mir-34a-5p/Sirt1 axis. EBioMedicine (2020) 53:102679. doi: 10.1016/j.ebiom.2020.102679
84. Chen Z, Zhang W, Deng M, Li Y, Zhou Y. Circglce alleviates intervertebral disc degeneration by regulating apoptosis and matrix degradation through the targeting of mir-587/Stap1. Aging (Albany NY) (2020) 12(21):21971–91. doi: 10.18632/aging.104035
85. Wang H, Zhu Y, Cao L, Guo Z, Sun K, Qiu W, et al. Circarl15 plays a critical role in intervertebral disc degeneration by modulating mir-431-5p/Disc1. Front Genet (2021) 12:669598. doi: 10.3389/fgene.2021.669598
86. Cui S, Zhang L. Circ_001653 silencing promotes the proliferation and ecm synthesis of npcs in idd by downregulating mir-486-3p-Mediated cemip. Mol Ther Nucleic Acids (2020) 20:385–99. doi: 10.1016/j.omtn.2020.01.026
87. Zhang F, Lin F, Xu Z, Huang Z. Circular rna itch promotes extracellular matrix degradation Via activating Wnt/β-catenin signaling in intervertebral disc degeneration. Aging (2021) 13(10):14185–97. doi: 10.18632/aging.203036
88. Wang H, He P, Pan H, Long J, Wang J, Li Z, et al. Circular rna circ-4099 is induced by tnf-α and regulates ecm synthesis by blocking mir-616-5p inhibition of Sox9 in intervertebral disc degeneration. Exp Mol Med (2018) 50(4):1–14. doi: 10.1038/s12276-018-0056-7
89. Wang X, Wang B, Zou M, Li J, Lü G, Zhang Q, et al. Circsema4b targets mir-431 modulating il-1β-Induced degradative changes in nucleus pulposus cells in intervertebral disc degeneration Via wnt pathway. Biochim Biophys Acta Mol Basis Dis (2018) 1864(11):3754–68. doi: 10.1016/j.bbadis.2018.08.033
90. Song J, Wang HL, Song KH, Ding ZW, Wang HL, Ma XS, et al. Circularrna_104670 plays a critical role in intervertebral disc degeneration by functioning as a cerna. Exp Mol Med (2018) 50(8):1–12. doi: 10.1038/s12276-018-0125-y
91. Guo W, Mu K, Zhang B, Sun C, Zhao L, Dong ZY, et al. The circular rna Fam169a functions as a competitive endogenous rna and regulates intervertebral disc degeneration by targeting mir-583 and btrc. Cell Death Dis (2020) 11(5):315. doi: 10.1038/s41419-020-2543-8
92. Li Y, Wu X, Li J, Du L, Wang X, Cao J, et al. Circ_0004354 might compete with Circ_0040039 to induce npcs death and inflammatory response by targeting mir-345-3p-Faf1/Tp73 axis in intervertebral disc degeneration. Oxid Med Cell Longev (2022) 2022:2776440. doi: 10.1155/2022/2776440
93. Wang Z, Zhao Y, Liu Y, Qu Z, Zhuang X, Song Q, et al. Circ0007042 alleviates intervertebral disc degeneration by adsorbing mir-369 to upregulate Bmp2 and activate the Pi3k/Akt pathway. Arthritis Res Ther (2022) 24(1):214. doi: 10.1186/s13075-022-02895-7
94. Vadalà G, Ambrosio L, Russo F, Papalia R, Denaro V. Interaction between mesenchymal stem cells and intervertebral disc microenvironment: From cell therapy to tissue engineering. Stem Cells Int (2019) 2019:2376172. doi: 10.1155/2019/2376172
95. Zhang W, Sun T, Li Y, Yang M, Zhao Y, Liu J, et al. Application of stem cells in the repair of intervertebral disc degeneration. Stem Cell Res Ther (2022) 13(1):70. doi: 10.1186/s13287-022-02745-y
96. Shi M, Zhao Y, Sun Y, Xin D, Xu W, Zhou B. Therapeutic effect of Co-culture of rat bone marrow mesenchymal stem cells and degenerated nucleus pulposus cells on intervertebral disc degeneration. Spine J (2021) 21(9):1567–79. doi: 10.1016/j.spinee.2021.05.007
97. Yu XJ, Liu QK, Lu R, Wang SX, Xu HR, Wang YG, et al. Bone marrow mesenchymal stem cell-derived extracellular vesicles carrying Circ_0050205 attenuate intervertebral disc degeneration. Oxid Med Cell Longev (2022) 2022:8983667. doi: 10.1155/2022/8983667
98. Widjaja G, Jalil AT, Budi HS, Abdelbasset WK, Efendi S, Suksatan W, et al. Mesenchymal Stromal/Stem cells and their exosomes application in the treatment of intervertebral disc disease: A promising frontier. Int Immunopharmacol (2022) 105:108537. doi: 10.1016/j.intimp.2022.108537
99. Li Z, Wu Y, Tan G, Xu Z, Xue H. Exosomes and exosomal mirnas: A new therapy for intervertebral disc degeneration. Front Pharmacol (2022) 13:992476. doi: 10.3389/fphar.2022.992476
100. Cheng X, Zhang G, Zhang L, Hu Y, Zhang K, Sun X, et al. Mesenchymal stem cells deliver exogenous mir-21 Via exosomes to inhibit nucleus pulposus cell apoptosis and reduce intervertebral disc degeneration. J Cell Mol Med (2018) 22(1):261–76. doi: 10.1111/jcmm.13316
101. Chen D, Jiang X. Exosomes-derived mir-125-5p from cartilage endplate stem cells regulates autophagy and ecm metabolism in nucleus pulposus by targeting Suv38h1. Exp Cell Res (2022) 414(1):113066. doi: 10.1016/j.yexcr.2022.113066
102. Bowles RD, Setton LA. Biomaterials for intervertebral disc regeneration and repair. Biomaterials (2017) 129:54–67. doi: 10.1016/j.biomaterials.2017.03.013
103. Li C, Bai Q, Lai Y, Tian J, Li J, Sun X, et al. Advances and prospects in biomaterials for intervertebral disk regeneration. Front Bioeng Biotechnol (2021) 9:766087. doi: 10.3389/fbioe.2021.766087
104. Feng G, Zha Z, Huang Y, Li J, Wang Y, Ke W, et al. Sustained and bioresponsive two-stage delivery of therapeutic mirna Via polyplex micelle-loaded injectable hydrogels for inhibition of intervertebral disc fibrosis. Adv Healthc Mater (2018) 7(21):e1800623. doi: 10.1002/adhm.201800623
105. Le Moal B, Lepeltier É, Rouleau D, Le Visage C, Benoit JP, Passirani C, et al. Lipid nanocapsules for intracellular delivery of microrna: A first step towards intervertebral disc degeneration therapy. Int J Pharm (2022) 624:121941. doi: 10.1016/j.ijpharm.2022.121941
106. Zhu L, Yu C, Zhang X, Yu Z, Zhan F, Yu X, et al. The treatment of intervertebral disc degeneration using traditional Chinese medicine. J Ethnopharmacol (2020) 263:113117. doi: 10.1016/j.jep.2020.113117
107. Chen HW, Zhang GZ, Liu MQ, Zhang LJ, Kang JH, Wang ZH, et al. Natural products of pharmacology and mechanisms in nucleus pulposus cells and intervertebral disc degeneration. Evid Based Complement Alternat Med (2021) 2021:9963677. doi: 10.1155/2021/9963677
108. Yang S, Li L, Zhu L, Zhang C, Li Z, Guo Y, et al. Aucubin inhibits il-1β- or tnf-α-Induced extracellular matrix degradation in nucleus pulposus cell through blocking the mir-140-5p/Creb1 axis. J Cell Physiol (2019) 234(8):13639–48. doi: 10.1002/jcp.28044
109. Ji Z, Guo R, Ma Z, Li H. Arctigenin inhibits apoptosis, extracellular matrix degradation, and inflammation in human nucleus pulposus cells by up-regulating mir-483-3p. J Clin Lab Anal (2022) 36(7):e24508. doi: 10.1002/jcla.24508
Keywords: non-coding RNAs, microRNAs, long noncoding RNAs, circular RNAs, intervertebral disc degeneration
Citation: Jiang C, Chen Z, Wang X, Zhang Y, Guo X, Xu Z, Yang H and Hao D (2022) The potential mechanisms and application prospects of non-coding RNAs in intervertebral disc degeneration. Front. Endocrinol. 13:1081185. doi: 10.3389/fendo.2022.1081185
Received: 27 October 2022; Accepted: 23 November 2022;
Published: 08 December 2022.
Edited by:
Shibao Lu, Xuanwu Hospital, Capital Medical University, ChinaReviewed by:
Chang Qing Zhao, Shanghai Jiao Tong University, ChinaCopyright © 2022 Jiang, Chen, Wang, Zhang, Guo, Xu, Yang and Hao. This is an open-access article distributed under the terms of the Creative Commons Attribution License (CC BY). The use, distribution or reproduction in other forums is permitted, provided the original author(s) and the copyright owner(s) are credited and that the original publication in this journal is cited, in accordance with accepted academic practice. No use, distribution or reproduction is permitted which does not comply with these terms.
*Correspondence: Hao Yang, eWFuZ2hhbzcxXzk5QHllYWgubmV0; Dingjun Hao, aGFvZGluZ2p1bkBtYWlsLnhqdHUuZWR1LmNu
†These authors have contributed equally to this work
Disclaimer: All claims expressed in this article are solely those of the authors and do not necessarily represent those of their affiliated organizations, or those of the publisher, the editors and the reviewers. Any product that may be evaluated in this article or claim that may be made by its manufacturer is not guaranteed or endorsed by the publisher.
Research integrity at Frontiers
Learn more about the work of our research integrity team to safeguard the quality of each article we publish.