- 1State Key Laboratory Cultivation Base, Eye Institute of Shandong First Medical University, Qingdao, China
- 2Shandong Provincial Key Laboratory of Ophthalmology, Eye Institute of Shandong First Medical University, Qingdao, China
With the global prevalence of diabetes mellitus over recent decades, more patients suffered from various diabetic complications, including diabetic ocular surface diseases that may seriously affect the quality of life and even vision sight. The major diabetic ocular surface diseases include diabetic keratopathy and dry eye. Diabetic keratopathy is characterized with the delayed corneal epithelial wound healing, reduced corneal nerve density, decreased corneal sensation and feeling of burning or dryness. Diabetic dry eye is manifested as the reduction of tear secretion accompanied with the ocular discomfort. The early clinical symptoms include dry eye and corneal nerve degeneration, suggesting the early diagnosis should be focused on the examination of confocal microscopy and dry eye symptoms. The pathogenesis of diabetic keratopathy involves the accumulation of advanced glycation end-products, impaired neurotrophic innervations and limbal stem cell function, and dysregulated growth factor signaling, and inflammation alterations. Diabetic dry eye may be associated with the abnormal mitochondrial metabolism of lacrimal gland caused by the overactivation of sympathetic nervous system. Considering the important roles of the dense innervations in the homeostatic maintenance of cornea and lacrimal gland, further studies on the neuroepithelial and neuroimmune interactions will reveal the predominant pathogenic mechanisms and develop the targeting intervention strategies of diabetic ocular surface complications.
Introduction
Diabetes mellitus (DM) is an endemic disease that occurs all over the world, imposing extensive health burden on society (1, 2). Diabetics with prolonged periods of hyperglycemia suffer from numerous complications affecting almost every organ system, including the ocular tissues (3, 4). DM-related ocular complications are the leading cause of blindness, especially in developed countries. Although diabetic retinopathy is the most common and well-known ophthalmic complication, DM also has profound clinically relevant effects on the ocular surface (5, 6).
The corneal tissue composes five stratified layers: the epithelium, Bowman’s layer, stroma, Descemet’s membrane and the endothelium (7, 8). Corneal epithelium is the cornea’s outermost layer, whose integrity is essential to maintaining healthy vision. Corneal stroma, which is populated by keratocytes, represents almost 90% of the thickness of the cornea. Corneal endothelium, a single cell layer between the corneal stroma and anterior chamber, exhibits barrier and ‘pump’ functions to maintain corneal dehydration. In addtition, to maintain a healthy ocular surface, the lacrimal gland and meibomian glands produce tears and lipids to prevent excessive evaporation of the tear film. Dysfunctions of these glands will cause dry eye disease (9, 10).
Although the structure of the ocular surface is relatively uncomplicated, problems with either component may have serious consequences. For DM-related ocular complications, various primary pathological manifestations occur, such as decreased corneal sensitivity, delayed epithelialization after corneal abrasions, basement membrane abnormality, corneal neuropathy, and endothelial decompensation (11, 12). Generally, these changes are referred to as diabetic keratopathy or diabetic neurotrophic keratopathy. Another common diabetic complication in the ocular surface is dry eye, with the involvement of lacrimal functional unit dysfunction (LFUD) (13). These complications drastically influence on the quality of life of patients and are frequently underdiagnosed and underestimated.
Current therapies for DK mainly include topical lubricants, antibiotic ointments, patching, bandage soft contact lenses, and corneal transplantation (14). Nevertheless, these treatments are usually incurable for serious DK, even if in combination. For the treatment of dry eye, identifying effective therapeutics remains an urgent challenge. Thus, research on novel drug targets is vital to the prevention and treatment for diabetic complications on the ocular surface.
Herein, we review recent advances in the pathogenesis of diabetic keratopathy and dry eye. We also evaluated the progress in diagnosis and treatment. These novel findings will shed new light on potential intervention strategies for diabetic ocular surface complications.
Diabetic keratopathy
Diabetic keratopathy is the most common clinical disease in which diabetes affects the ocular surface. It is a potential vision threatening disease, mainly including epitheliopathy, neuropathy and endotheliopathy.
Diabetic corneal epitheliopathy
The corneal epithelium consists of 5-7 layers of non-keratinized stratified squamous epithelium, which plays a key role in maintaining corneal transparency and stability. Because the cornea has no blood vessels, and the level of tear glucose level is far less than that of aqueous humor and serum in diabetic patients (15, 16), it is believed that the glucose in corneal epithelial cells is mainly transported from aqueous humor (17). The level of glycosylation in the corneas of diabetic patients increased significantly (18), and the accumulation of glycogen granules was observed in diabetic corneal epithelial cells (19). In diabetic patients, corneal epithelial cells are exposed to persistent high levels of glucose, resulting in various clinical epithelial abnormalities.
Several studies have found that corneal epithelium in diabetic patients tends to have increased fragility, lower cell density, thinner thickness and reduced barrier function (20–22). An electron-microscopic examination of corneal epithelium showed an increased epithelial fragility in specimens of diabetic patients (23). Saini and Khandalavla measured the corneal epithelial fragility of healthy people and diabetic patients using an esthesiometer (20). The results revealed that the average corneal epithelial fragility of diabetic patients was significantly higher than that of healthy people, and that the epithelial fragility of diabetic retinopathy patients increased more significantly. Increased corneal epithelial fragility was also found in Goto Kakizaki rats with type 2 DM (24). A few studies reported that there was no statistical significance in the reduction of corneal basal epithelial cell density in diabetic patients (25, 26). However, more clinical studies have demonstrated that the density of corneal basal epithelial cells was significantly reduced in type 1 and type 2 diabetic patients (21, 27–29), which may be related to the reduction of corneal innervation, impaired of basement membrane and higher turnover rate (21). In the diabetic patients, the mean corneal epithelium thickness was thinner (22, 30) which is associated with the stage of the disease. Similarly, Cai et al. verified the characteristics of the thinning of corneal epithelium and the decreasing density of basal epithelial cells in the rodent model of type 1 diabetes induced by streptozotocin (31). The changes of corneal epithelial density and thickness reflect the imbalance between cell proliferation, differentiation, migration and death. The corneal epithelium has a strong barrier function, making it the first line of defense for the eyeball to resist the external environment. It has long been found that the barrier function of diabetic corneal epithelium is weakened (32–34) which is related to the increase of glycosylated hemoglobin level (34), and correspondingly, diabetic corneas are more prone to infection than healthy people (35–39). In vitro studies have proven that high glucose exposure leads to the impairment of the human corneal epithelial cell barrier function, but this change was not caused by the reduced expression of tight junction protein (40).
Clinically, epitheliopathy is characterized by superficial punctate keratitis, recurrent epithelial erosion, persistent epithelial defect and delayed and often incomplete wound healing. In our previous review, according to Semeraro’s classification criteria (41), we summarized the manifestations of mild, moderate and severe diabetic corneal epithelial lesions found in our hospital (4). Corneal abrasions in diabetic patients can cause more serious damage, in some cases leading to basement membrane detachment, and in other cases leading to recurrent corneal erosion (42). Epithelial wound healing is critical for restoring corneal barrier function after injury. Corneal epithelial damage in diabetic patients often takes longer to heal, even does not heal, which is also the main reason why diabetic corneal erosion is difficult to treat (14).
The surgical treatment on diabetic patients will more often lead to subsequent epithelial lesions, such as long-term erosion of epithelial cells and poor healing of epithelial cell defects. It has been confirmed that patients with diabetes who have undergone corneal refractive surgery are at greater risk of developing various epithelial diseases (43–45). Therefore, some ophthalmologists suggest that refractive surgery for diabetic patients should be carefully considered, especially for patients with poor blood glucose control (44–47). A recent study showed that DM is an important risk factor of corneal epithelial defect after vitreoretinal surgery (48). Frequently, diabetic patients with epithelial keratitis after cataract surgery have the characteristics of rapid development, severe epithelial damage, and slow corneal epithelial repair (49). Patients with diabetes are at a greater risk of epithelial debridement due to impaired epithelial wound healing (50).
Diabetic corneal neuropathy
Corneal nerves, a branch of the ophthalmic division of the trigeminal nerve, enter the peripheral cornea in a radial fashion parallel and then penetrate Bowman’s layer to form the corneal sub-basal nerve plexus, which terminate in free nerve endings in the corneal epithelium and comprises the outermost layer of the cornea and protects cornea from microbial invasion (51, 52). Diabetic peripheral neuropathy (DPN) is the most common complication of diabetes, affecting up to 50% of diabetic patients (53). Recent study reported that the density of corneal nerve fiber and branch, and the corneal nerve fiber length are significantly decreased in diabetic patients (12). Moreover, the loss of 6% or more of corneal nerve fibers per year has been found in 17% of diabetic patients (54, 55). Approximately 39% of diabetic patients experience painful DPN when left untreated (56).
In type 1 and type 2 diabetic patients and animal models, the length, branch and density of corneal nerve fibers in the sub-basal nerve plexus near the corneal epithelium have been found to be reduced, which relates to the severity of diabetic polyneuropathy (24, 31, 57–63). Detailed examination by in vivo confocal microscopy has revealed increased corneal nerve tortuosity and thickness in diabetic patients (60, 64–68). Moreover, reduced corneal sensitivity is observed in diabetic patients and animals, and the degree is correlated with the severity of diabetes (60, 63, 67, 69–71). Pritchard et al. reported that corneal sensation threshold was significantly higher for patients with neuropathy compared to those without neuropathy and controls (72). Recent studies have identified corneal sensitivity as a potential marker of diabetic neuropathy (73). In addition, the regeneration of corneal sub-basal nerves is significantly slower in diabetic animals during corneal epithelial wound healing (24, 74). Importantly, the reduction of sub-basal nerve plexus density and corneal sensitivity, which precedes other clinical and electrophysiology tests, could be used as markers for DPN assessment (75, 76). In addition, patients with diabetes often have burning, dryness or painful feeling in the eye (77).
Pathologic mechanisms
The pathogenesis is difficult to investigate through human epidemiological studies due to too many confounding factors. Therefore, researchers often use animal diabetes models and in vitro cell models to study pathogenesis (78, 79). The changes in growth factors, immune cells and signal pathways in diabetic keratopathy have been elaborated in previous reviews (4, 14, 17, 78, 80). Here, we mainly discuss the following aspects.
Chronic inflammation
As a significant characteristic of DM, low-grade chronic inflammation is regarded as an important mechanism for the development of DM and its complications, including diabetic nephropathy, diabetic retinopathy, and diabetic cardiomyopathy (81, 82). These chronic inflammatory scenarios was triggered and sustained by immune cells and structural cells of specific organs/tissues, which activated innate immunity mainly through pattern recognition receptors (PRRs), such as Toll-like receptors (TLRs) and nucleotide-binding oligomerization domain (NOD)-like receptors (NLRs) (82, 83). Therefore, chronic inflammation theoretically also contributes to the development of DK. Several compelling evidence we found consolidated the pathogenic involvement of chronic inflammation in the development of DK (Figure 1).
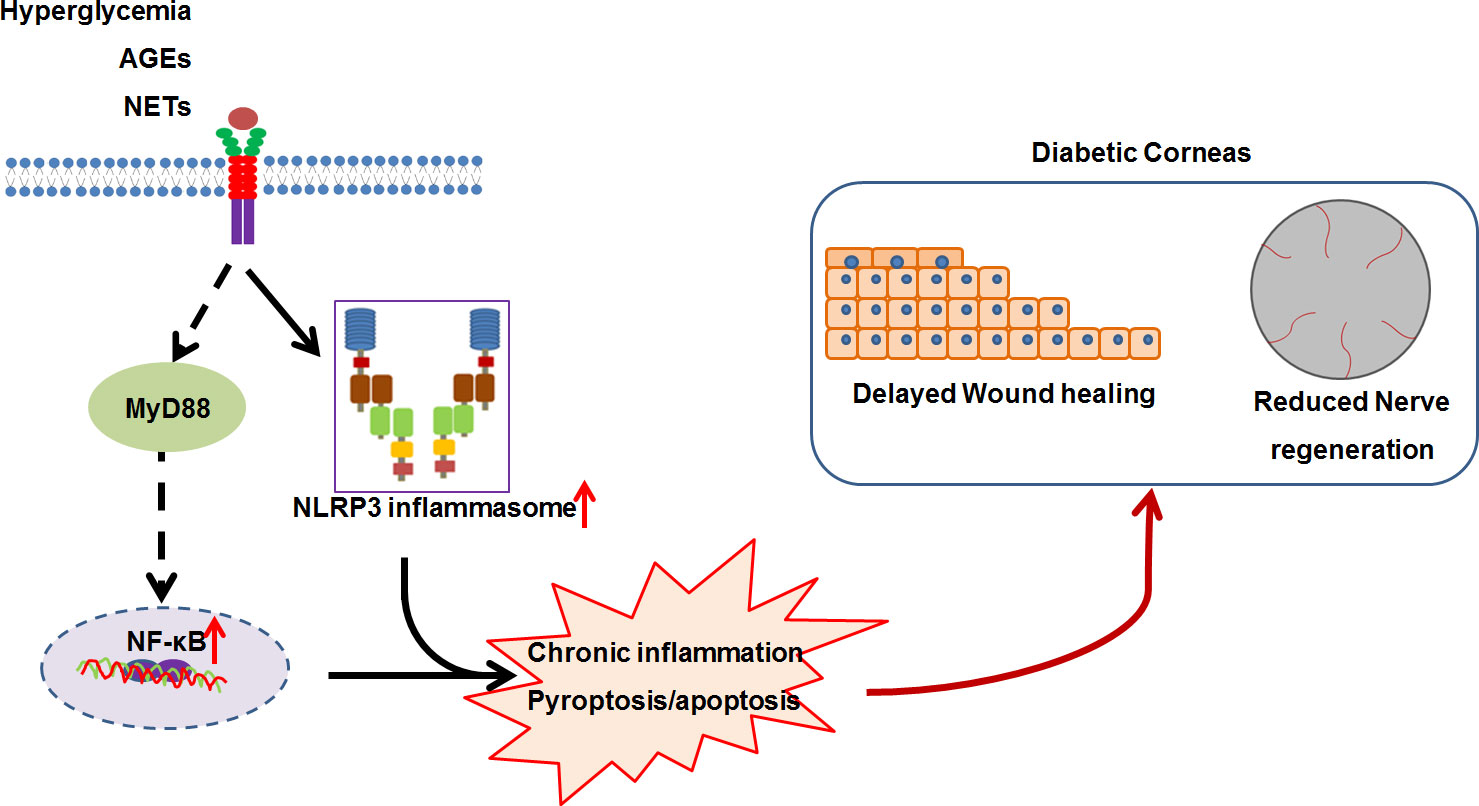
Figure 1 The working model for the low-grade chronic inflammation contributing to diabetic keratopathy. In diabetic mellitus, numerous diabetes-associated danger molecules (such as hyperglycemia, AGEs and NETs), persistently activate NF-κB signaling and NLRP3 inflammasome, resulting in chronic inflammation and pyroptosis, which ultimately postpones corneal epithelial wound healing and impairs re-generation.
NOD-like receptor protein 3 (NLRP3) inflammasome, a fully characterized inflammasome, contains NLRP3, adaptor protein ASC, and pro-caspase-1(pro-CASP1), and can be activated by various stimuli, including pathogenic molecules, sterile insults, and metabolic products (84, 85). NLRP3 inflammasome-mediated inflammation plays key roles in the development and progression of DM and its complications, such as diabetic nephropathy (83), diabetic retinopathy (86, 87), and diabetic cardiomyopathy (88). Using genetic and pharmacological approach, we revealed that persistent activation of NLRP3 inflammasome resulted in delayed diabetic corneal wound healing and impaired re-innervation (89, 90). This was supported by the findings of hyper-activation of NLRP3 inflammasome responsible for delayed diabetic skin wound healing and diabetic foot ulcer closure (91–93). Furthermore, we mechanistically revealed that the accumulated advanced glycation end-products (AGEs) promoted hyperactivation of NLRP3 inflammasome through ROS production, ultimately resulting in impaired corneal wound healing and nerve regeneration (89). The findings of AGEs accumulation on the basement membrane of corneal epithelium and Descemet’s membrane in diabetic patients (94–96) were therefore mirrored the possibility of AGEs involving in the DK progression via NLRP3 inflammasome signaling. Generally, the assembly and activation of NLRP3 inflammasome results in the CASP1-dependent secretion of interleukin (IL)-1β and IL-18, as well as gasdermin D (GSDMD)-mediated pyroptosis (97). Yan et al. found that the imbalance of IL-1β and IL-1RA (IL-1 receptor antagonist) in DM corneas inhibited epithelial proliferation and promoted apoptosis, further delaying corneal epithelial healing and re-innervation (98). Inhibition of IL-1β signaling using recombinant IL-1RA and IL-1β neutralizing antibody significantly reversed the postponed diabetic corneal epithelial closure and restored re-innervation (90, 99). In addition to the elevated matured form of IL-1β, the activated form of GSDMD in diabetic corneas after abrasion was also significantly increased (89), which suggested that the GSDMD-executed pyroptosis could be also probably responsible for the excessive inflammation and the impaired corneal would healing and nerve regeneration. Therefore, NLRP3 inflammasome-mediated chronic inflammation is one of important contributors to the pathogenesis of DK, and targeting NLRP3 inflammasome could a promising for DK treatment. Moreover, we also found that blocking TLR4 signaling via TAK-242 expedited diabetic corneal re-epithelialization and nerve regeneration. In addition to receptor of AGEs (RAGE), AGEs also elicit inflammatory response through TLR4 and myeloid differentiation 2 (MD2) (100). AGEs/TLR4 mediated inflammatory response could be another factor attributed to the postponed diabetic corneal wound healing and impaired nerve regeneration.
Under normal conditions, the cornea is endowed with a heterogeneous resident population of antigen-presenting cells, including dendritic cells and macrophages (101–103). Several lines of evidence revealed that specific deletion of dendritic cells or macrophages results in a delayed corneal wound healing in healthy or DM corneas (74, 104–106). Although accumulative evidence indicates the essential role of macrophages and dendritic cells in the pathogenesis and development of DM and its complications (107–110), whether chronic inflammation triggered by macrophages and dendritic cells contributes to DK pathogenesis and progression remains elusive. Fewer neutrophils are usually distributed in normal corneas, but more are recruited after tissue injury or infection. During diabetic corneal wound closure, the number of neutrophils was significantly heightened (111), suggesting a pathogenic role for postponed corneal wound healing and impaired nerve regeneration.
As a component of innate immune system, neutrophils carry out numerous functions, including wound repair (112). During normal wound healing, neutrophils undergo apoptosis after accomplishing their functions, and are subsequently engulfed by macrophages to resolve inflammation (112). However, the DM triggered the neutrophils to NETosis (99, 113). During NETosis, the neutrophils die through releasing web-like chromatin structures loaded with cytotoxic proteins, which is termed as neutrophil extracellular traps (NET) (114). A series of evidence has revealed that NETosis primed by DM resulted in the delayed wound healing and sterile inflammation (99, 113). During diabetic corneal wound healing, NETs production was pronouncedly elevated, and blockade of NETs formation using DNase I or Cl-amidine not only improved inflammation resolution, but also promoted corneal epithelial wound healing and mechanical sensation restoration (115). Besides its crucial role in innate host defense, NETs also fuel inflammatory and autoimmune response, including NLRP3 inflammasome (92, 93, 116, 117). In this regard, NETs would be an essential driver for chronic inflammation during DK pathogenesis and progression.
Neurotrophic function
The relationship between corneal nerves and epithelium has been found interdependency and mutual support. The corneal nerves maintain the integrity of corneal epithelium by releasing neurotrophic factors (118). Our laboratory has been committed to studying the role and mechanism of neurotrophic functions in diabetic keratopathy. We found that the levels of many neuropeptides, neurotrophic factors and axon guidance molecules in diabetic corneas were lower than in normal corneas, suggesting that the imbalance of neurotrophic function may be among the critical mechanisms of diabetic keratopathy (4).
Neuropeptides released from the sensory nerve terminals, such as substance P (SP), vasoactive-intestinal peptide (VIP), calcitonin gene-related peptide (CGRP), neuropeptide Y (NPY), and insulin–like growth factor -1 (IGF-1), play important roles in maintenance and nutrition of the corneal epithelium by promoting migration and proliferation (111, 119–127). Substance P (SP) is an 11-amino acid (ARG-PRO-LYS-PRO-GLN-GLN-PHE-PHE-GLY-LEU-MET) neuropeptide expressed in the corneal nerves, cornealepithelium and stromal keratocytes in the cornea (128–131). However, there has been no report on the expression of SP in the resident immune cell population of cornea. We found that SP content in cornea of type 1 diabetic mice decreased significantly. Exogenous SP supplementation markedly promoted epithelial wound healing and cornealsensation recovery by augmenting mitochondrial function, which was blocked by the antagonist of its NK-1R receptor, indicating that SP-NK-1R signaling played a notable role in regulating diabetic epithelial repair (Figure 2) (126). Many studies have illustraed that SP-NK-1R pathway can activate multiple signal pathways that promote epithelial growth, migration and adhesion (122, 132–135). Moreover, administration of eye drops containing SP and IGF-1 ameliorated the barrier function by promoting corneal wound healing in rats and rabbits with neurophic keratopathy (121, 136, 137). FGLM, a SP-derivedpeptide (PHE-GLY-LEU-MET), combined with IGF-1, promoted the corneal epithelialwound healing and has been used for the neurotrophic keratopathy treatment in clinical successfully (138, 139). In addition, a tetrapeptide (SSSR) derived from the IGF-1 combination with FGLM also has the synergy in corneal epithelial wound healing (140, 141).
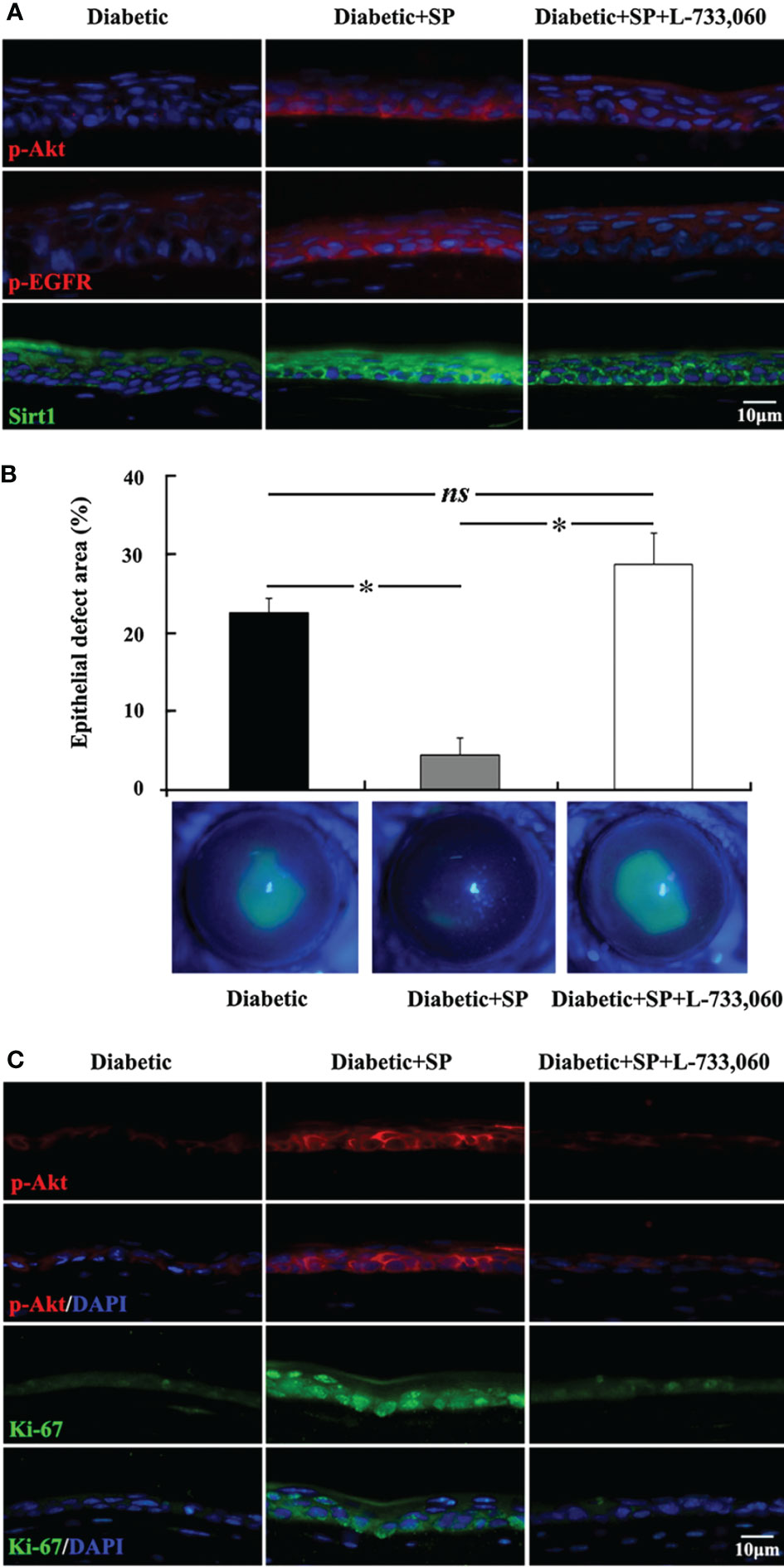
Figure 2 SP-NK-1R signaling regulates diabetic corneal epithelial wound healing. (A) In the unwounded corneal epithelium, the elevation of p-Akt, p-EGFR, and Sirt1 level by SP application was attenuated in NK-1 receptor antagonist L-733,060-injected SP-treated diabetic mice. (B) L-733,060 injection reversed the promotion of SP on diabetic corneal epithelial wound healing. (C) L-733,060 treatment reversed the promotion of SP on p-Akt activation and proliferation in the regenerated corneal epithelium. ns, no significance; *p< 0.05. (ref 126).
Neurotrophin (NT) is a kind of protein molecule produced by tissues and astrocytes dominated by nerves and necessary for the growth and survival of neurons. Nerves can nourish corneal epithelium, and neurotrophic factors derived from corneal epithelium can also nourish nerves by promoting the growth and survival of nerves. Hyperglycemia attenuates the expression of nerve growth factor (NGF) and glial cell-derived nerve growth factor (GDNF) in the corneal epithelium, while exogenous NGF and GDNF increased the sub-basal nerve fiber density and corneal sensitivity (142). In diabetic mellitus, the content of CNTF and netrin-1 is lessened in diabetic mouse corneas, and we have demonstrated exogenous CNTF improves the corneal epithelial wound healing and nerve regeneration markedly (143). Gao et al. pointed out that dendritic cells are also the main source of CNTF. The reduction of CNTF levels caused by the decrease in dendritic cells during diabetic corneal wound healing is the potential mechanism of diabetic corneal neuropathy (106).
Mesencephalic astrocyte-derived neurotrophic factor (MANF), first discovered as secreted proteins with trophic activity, was expressed in the neuronal and non-neuronal systems especially in high metabolic tissues (144–146). MANF also plays an important role in diabetes. Notably, mice with the konckout of MANF developed diabetes due to increasing apoptotic cell death and reduced proliferation of pancreatic β cells, while recombinant MANF could promote proliferation and prevent cell death (146, 147). In addition, MANF has anti-inflammatory abilities in human pancreatic β cells that protect cells from cell death by repressing the NF-κB signaling pathway (148). MANF has been newly identified in corneas and is reduced in both unwounded and wounded corneal epithelium of diabetic mice. Moreover, recombinant MANF significantly promoted the wound healing of epithelium and nerve regeneration by inhibiting hyperglycemia-induced ER stress and ER-stress related apoptosis (149). Hence, MANF might be a potential therapeutic target for treating diabetic keratopathy.
Besides neuropeptides and neurotrophic factors, there is also a class of factors that play a key role in the repair of nerve innervation, namely axon guidance molecules. These molecules mainly include the Slits family, Netrins family, Ephrins family, Semaphorins family, etc (150). We found that hyperglycemia downregulates netrin-1 expression in corneal epithelium, and the subconjunctival injection of netrin-1 promotes corneal epithelial wound healing and nerve regeneration in diabetic mice. Netrin-1 facilitates the proliferation and migration of corneal epithelial cells under high-glucose conditions. Furthermore, we revealed that netrin-1 inhibited neutrophil infltration, enhanced M2 macrophage transition, and attenuated the expression of pro-infammatory factors in diabetic mouse corneal epithelium via adenosine 2B receptor (151). Bettahi et al. revealed that diabetes inhibited the upregulation of Sema3c induced by corneal epithelial injury, but had no effect on Sema3a (152). The diabetic corneal epithelium and nerve regeneration can be promoted by exogenous supplementation with Sema3c (153). The above-mentioned studies suggest that the reduction of axon guidance factors, such as netrin and Sema3c, is partly responsible for diabetic keratopathy.
Some growth factors and metabolites also have neuroprotective effects. The expression of vascular endothelial growth factor (VEGF)-B is decreased in the regenerated diabetic corneal epithelium, and exogenous VEGF-B promotes the regeneration of diabetic corneal nerve fibers by reactivating the PI-3K/Akt-Gsk3ß-mTOR signaling (154). Moreover, VEGF-B also elevates the corneal content of pigment epithelial-derived factor (PEDF). He et al. found PEDF plus docosahexaenoic acid (DHA) could accelerate corneal nerve regeneration in diabetic mice (155). Nicotinamide adenine dinucleotide (NAD) is involved in glycolysis, gluconeogenesis, tricarboxylic acid cycle, and other cellular metabolic reactions, and has essential biological functions (156). Our group demonstrated that NAD+ biosynthesis plays an important role in maintaining corneal homeostasis and innervation (157). In diabetic corneas, NAD+ content was decreased, and elevated the levels of NAD+ and its precursors NMN and nicotinamide riboside (NR) markedly promoted epithelial and nerve repair by activating SIRT1 and pEGFR, pAKT, and pERK1/2 signaling (158). Another study found that nicotinamide mononucleotide is helpful in improving cell viability and tight junctions in high glucose treated human corneal epithelial cells through the SIRT1/Nrf2/HO-1 pathway (159).
Neural ion channels changes
Corneal neurons express a range of membrane channels, including chemical or polymodal nociceptors, mechanonociceptors, and thermal or cold receptors (160, 161). Among corneal afferent neurons, approximately 45% expressed TRPV1, 28% expressed Piezo2, and 8% expressed TRPM8, with 6% of TRPV1 neurons co-expressing TRPM8 (162). The transient receptor potential (TRP) family is thought to transduce environmental and endogenous stimuli to electrophysiological signals. TRPV1 is a well-characterised channel expressed by a subset of peripheral sensory neurons, and canonically mediates inflammatory and neuropathic pain (163). TRPV1 sensitization can be induced by capsaicin. Nowadays, capsaicin 8% patch has been used to alleviate pain in patients with peripheral neuropathic pain, which induced fewer systemic side effects (164–169). Corneal TRPV1 is involved in the maintenance of the corneal structure, re-epithelialization, and inflammation in corneal injury (170). In addition, blinking behavior in guinea pigs related to ocular discomfort is reversed by treatment with the TRPV1 blocker, capsazepine (171). Therefore, corneal TRPV1 may be important for healing corneal tissue, and alleviating the pain in inflammatory disorders of the ocular surface. The depletion of TRPV1+ sensory nerves delayed corneal wound healing by enhancing the recruitment of neutrophils and γδ T cells, increasing the number and TNF-α expression of CCR2+ macrophages and decreasing the number of CCR2– macrophages and IL-10 expression (172). In diabetic conditions, the TRPV1 expression in trigeminal ganglia is increased and the integrity of TRPV1 neurons is important for avoiding alveolar bone resorption and inflammation (173).
Recently, cold receptors have come under greater scrutiny. TRPM8, which is activated by temperatures lower than 25-28°C and menthol, is widely expressed in corneal afferent fibers (174–176). We found that in Trpm8-deficient mice, corneal wound healing is accelerated, while squamous metaplasia occurred in the central corneal opacity after multiple injuries (unpublished data). TRPV1-dependent neuronal sensitization facilitates the release of SP from TRPM8+ cold-sensing neurons to signal nociception in response to cold (177, 178). Overexpression of TRPV1 in TRPM8+ sensory neurons leads to cold allodynia in both corneal and non-corneal tissues without affecting their thermal sensitivity (177). Type 1 diabetic mice exhibit heightened sensitivity to both heat and cold. In diabetic hyperalgesic mice, the thermal hyperalgesia induced by an increase in TRPV1 function is further aggravated by decreased TRPM8 function (179). Abdulhakeem S. Alamri et al. found that the density of corneal nerve fibers in mice fed a high-fat and high-cholesterol diet and those with hyperglycemia had a similar reduction. The reduction of nerve fibers expressing TRPM8 receptors in the corneas of the two models was more significant than that of TrpV1 positive nerve fibers (180).
Diabetic autonomic neuropathy
Several influencing factors have been implicated in the pathogenesis of diabetic neuropathy. The hyperglycemic activation of the polyol pathway and protein kinase C may reduce the neuronal blood flow causing direct neuronal damage (181–183). In addition, the increased oxidative stress induced excess nitric oxide production may result in the formation of peroxynitrite and damage to neurons (184, 185). Moreover, the reduction of neurotrophic growth factors, the deficiency of essential fatty acids, and the accumulation of advanced glycosylation end products may also cause less endoneurial blood flow and nerve hypoxia which altered nerve function (183, 186–188). Diabetic neuropathy has been classified as diabetic peripheral and autonomic neuropathies based on pathophysiological characteristics (127). However, few studies have focused on the changes of autonomic nervous system in diabetes keratopathy and its regulatory mechanism.
Diabetic autonomic neuropathy (DAN) is a serious and common complication which has negative impact on the survival and quality of life in patients’ with diabetes (189). DAN may affect many organ systems throughout the body, such as gastrointestinal, genitourinary, and cardiovascular (190). The autonomic nervous system is divided into the sympathetic and the parasympathetic nervous systems. In mammalian corneas, the density of the sympathetic innervations which are from the superior cervical ganglion, vary among species (191). The sympathetic innervations compose about 10–15% of corneal innervations in rabbit, mouse, rat and cat, whereas in primates, they are rarely reported (52, 104, 192). The activation of the sympathetic nervous system has been found in type 1 and type 2 diabetic mice (193–196). In cornea, the activation of sympathetic nervous system may inhibit the wound healing of corneal epithelium and induce the expression of proinflammatory genes in the CD64+CCR2+ macrophages through the β-2 adrenergic receptor (ADRB2) (104). Moreover, we found that the abnormal activation of sympathetic nerve in diabetic mice resulted in the partial depletion of multiple neurotrophins in corneal epithelial cells and dysfunction of limbal stem cells through ADRB2, which further delayed the corneal sensory nerve regeneration and epithelial wound healing (Unpublished data).
The parasympathetic innervations, which are from the ciliary ganglion, exist in different species and vary among in rats, cats, and mice (52, 104, 197). Conversely, the activation of parasympathetic nerves promotes the wound healing of corneal epithelium and enhances the expression of the anti-inflammatory genes in CD64+CCR2- macrophages through α-7 nicotinic acetylcholine receptor (α7nAChR) (104). VIP is secreted predominantly by parasympathetic nervous system. The distinct local macrophages have been found to be activated by VIP, which further modulated inflammation and epithelial renewal. Recently, we found VIP and its receptor are decreased in diabetic corneas in the process of wound healing compared with normal, while exogenous VIP attenuates the wound healing of DM corneas by regulating the wounding inflammatory response and nerve regeneration through Sonic Hedgehog signaling pathway (111).
miRNAs and long noncoding RNAs
Generally, miRNA has been proven to be a key regulator of gene expression and can target a variety of molecules that affect cell physiology and disease development. Numerous reports have shown that miRNA relates to the pathology of the diabetic corneal epithelium and nerve damage, making miRNA becoming a promising therapeutic approach for the treatment of diabetic keratopathy.
As the source of corneal nerve fibers, changes in the trigeminal ganglion (TG) caused by diabetes may contribute to corneal neuropathy. Through RNA sequencing, our group found that 68 miRNAs and 114 mRNAs in the TG tissues of diabetic mice diverged from those in normal TG tissues. We predicted that the interaction of miR-350-5p and Mup20, miR-592-5p and Angptl7, and miR-351-5p and Elovl6 may be related to diabetic corneal neuropathy (198). Jianzhang Hu et al. found that inhibiting the expression of miR-181a and miR-34c in TG of diabetic mice promoted the growth of trigeminal sensory neural cells and the regeneration of corneal nerve fibers by regulating autophagic activation (199, 200). Our study revealed that the expression of miR-182 was downregulated in the TG tissue of diabetic mice, which was a key molecule downstream of the endogenous protective gene Sirt1 in TG. And NOX4 was a key target gene for miR-182 to regulate diabetic corneal epithelial and nerve repair (201). Targeting NOX4 and Sirt1 could effectively mitigate the severity of diabetic keratopathy (201, 202).
We also screened differentially expressed miRNAs in the regenerated corneal epithelium of normal and type 1 diabetic mice, and found that miR-223-5p was significantly upregulated, which may be involved in regulating the delay of diabetic corneal wound healing. In the next validation experiment, we confirmed that inhibition of miR-223-5p accelerated the regeneration of diabetic corneal epithelium and nerves, which mediates inflammation response and epithelial cell proliferation through its target gene Hpgds (203). In 2016, our group also found that miR-204-5p, which can directly regulate sirt1, has increased expression in diabetic corneas, and inhibition of miR-204-5p promotes corneal epithelial regeneration by accelerating cell cycle (204).
Compared with normal diabetic mice, diabetic miR-146a KO mice had significantly delayed epithelial wound healing of cornea and skin, and increased neutrophil infiltration. The potential mechanism was that miR-146a KO induced an imbalance in the IL-1β, TNF-α, IRAK1, TRAF6 and NF-κB signaling pathways. Interestingly, there was no difference in corneal wound healing between miR-146a KO and normal mice with normal blood glucose (205). Subsequently, another group’s research in cultured human limbal epithelial cells showed that overexpression of miR-146a reduced the expression of proinflammatory TRAF6, IRAK1 and downstream target NF-κB; and inhibited the expression of cytokine IL-1α, IL-1β, IL-6 and IL-8 and chemokines CXCL1, CXCL2, and CXCL5, which were significantly upregulated in diabetic corneal limbal epithelial cells (206). These studies indicate that miR-146a plays an important role in the regulation of corneal epithelial homeostasis and regeneration under diabetic conditions.
lncRNAs are a class of noncoding RNA molecules with a length of more than 200 nucleotides, which have been reported to play a regulatory role in diabetic complications, retinopathy, pterygium and other eye diseases. Xiaxue Chen and Jianzhang Hu analyzed the differentially expressed lncRNAs (DELs) in the regenerated corneal epithelium of type 1 diabetic and normal corneas. In the diabetic group, 111 upregulated DELs and 117 downregulated DELs were detected. The authors conducted in-depth research on lncRNAs Rik, which is significantly downregulated in diabetes, and found that Rik can be combined with miR-181a-5p as a ceRNA, thus promoting the healing of diabetic corneal epithelial wounds (207).
Limbal stem cell dysfunction
The corneal epithelium is self-renewed and regenerated by limbal stem cells (LSCs) that reside in the basal epithelial layer of the limbus, which plays a key role in corneal epithelial wound healing (208–211). A study based on the alteration of LSCs in patients with diabetes found that the expression of markers of LSCs such as ΔNp63α, ATP-binding cassette sub-family G member 2 (ABCG2), N-cadherin, K15, K17, K19, and β1 integrin was decreased significantly in the diabetic limbus (212). In vitro cultured LSCs from healthy and diabetic patients were subjected to immunofluorescence staining with LSC markers, and it was also found that the expression of LSCs markers ΔNp63α, PAX6, ABCG2, K15 and K17 in diabetic patients was reduced markedly, especially K15 and K17 (213). Similarly, type 1 and type 2 diabetic mice also showed a significant reduction of LSCs markers in corneal limbus (143, 214). Thus, the loss or dysfunction of the resident LSCs could be responsible for clinically observed delayed corneal epithelial wound healing in diabetic corneas. Therefore, improving the function of diabetic LSCs through genes or growth factors is expected to be an effective means to promote diabetic corneal epithelial wound healing.
We found that the expression of neurotrophic factor CNTF was significantly reduced in corneal epithelium of STZ-induced type 1 diabetic mice. Studies in cultured mouse corneal epithelial stem/progenitor cells found that CNTF increases the efficiency of clone formation, promotes cell proliferation, and upregulates the expression level of corneal epithelial stem/progenitor cell-related transcription factors by activating Stat3 signal (143). It can also upregulate MMPs by activating Akt signal to promote the migration of corneal epithelial stem/progenitor cells (215). CNTF supplementation by subconjunctival injection can promote the corneal epithelial would healing both in normal and diabetic mice, and is accompanied by the enhancement of corneal epithelial stem/progenitor cell proliferation activity (Figure 3). In contrast, the application of CNTF neutralizing antibody significantly impairs the normal repair function of corneal epithelium. Hiroki Ueno et al. reported that insulin-like growth factor-I (IGF-I) is capable of protecting against corneal stem/progenitor cells and nerve damage in diabetes (214). Taken together, growth factors, such as CNTF and IGF-1, have potential effects in ameliorating limbal stem cell deficiency and treating diabetic keratopathy by enhancing LSCs functions.
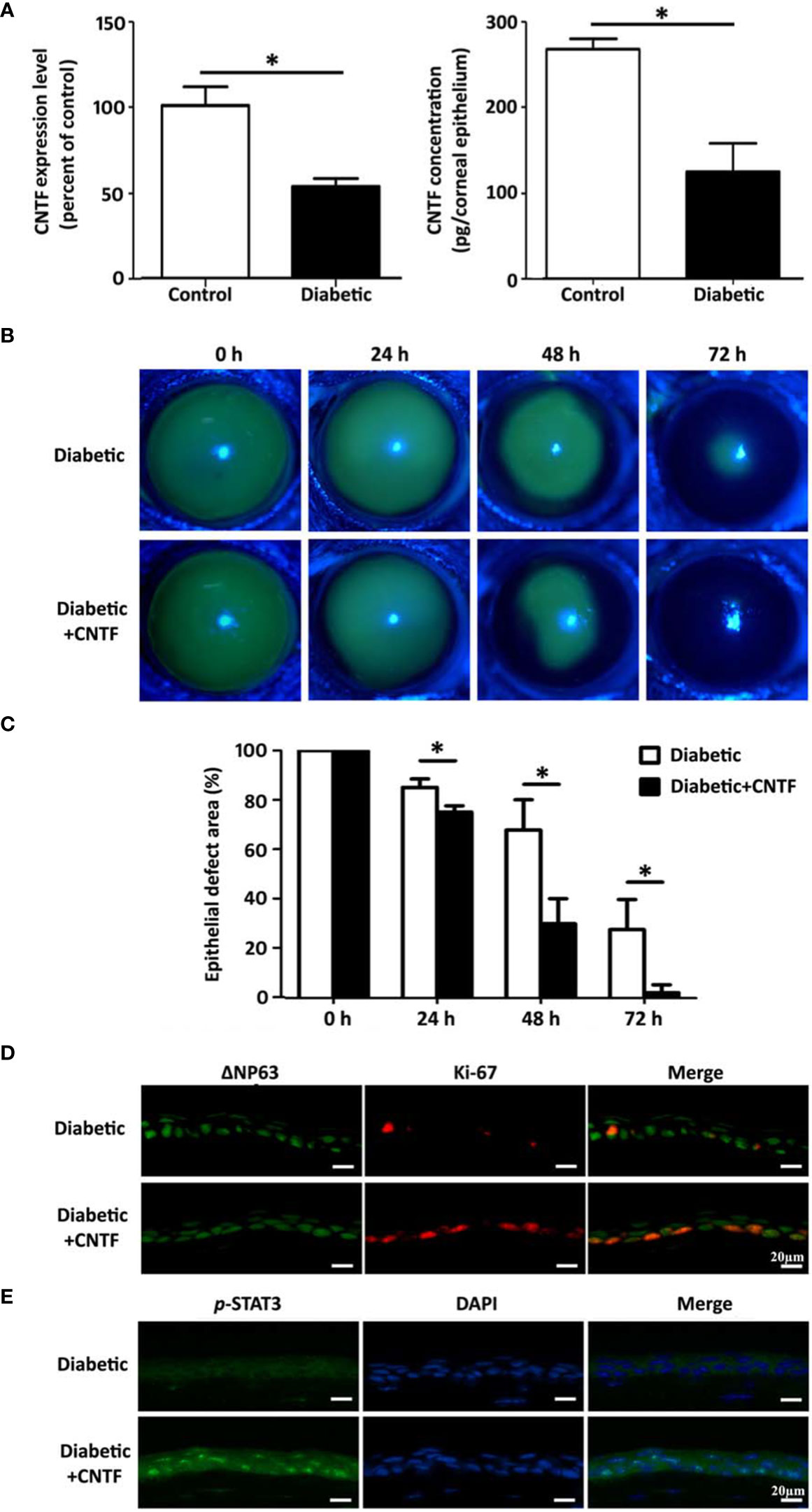
Figure 3 CNTF promotes corneal epithelial wound healing in diabetic mice. (A) CNTF is decreased in diabetic corneas both in mRNA and in protein level. (B, C) Subconjunctival injection of 50 ng CNTF significantly promotes the corneal epithelial wound healign in diabetic mice. (D) The expression of ΔNp63 and Ki-67 in the regenerating corneal epithelium is upregulated after CNTF treatment. (E) CNTF activated Stat3 signaling in diabetic wounded corneas. "*p< 0.05. (ref 215).
Some compounds also have the effect of enhancing the stemness of limbal stem cells, such as ascorbic acid (216), ROCK inhibitor Y-27632 (217), and pluripotin (218). Recently, we found that the proinflammatory cytokines IL-1β and TNF-α were overexpressed during diabetic corneal epithelial wound healing (219). Proinflammatory cytokines can suppress the LSCs markers expression and the colony-forming capacity of corneal epithelial stem cells, as well as destroy the normal ability for corneal epithelial wound healing in a mouse model (220). Proinflammatory cytokines regulate corneal epithelial wound healing through p16Ink4a-STAT3 signaling, and knockdown of p16Ink4a partially restores diabetic corneal epithelial repair defects (221). Yuka Okada et al. confirmed that the sensory nerve TRPV4 is essential for maintaining the stemness of LSCs and is one of the main mechanisms for maintaining corneal epithelial homeostasis (221). Thus, controlling inflammation and maintaining sensory nerve function are beneficial to diabetic corneal epithelial wound healing.
Diabetic corneal endotheliopathy
Clinical manifestation
Corneal endothelial cells (CECs) can be characterized according to the percentage of hexagonal cells (HEX) and the coefficient of variation (CV) (222–225). The previous researches are inconsistent regarding the effect of DM on CEC pleomorphism and polymegathism. Many studies report that the CECs of diabetic patients have a decreased HEX and an increased CV compared to healthy controls (226–230), whereas other studies show no differences (224, 225, 231, 232). Most studies support the hypothesis that DM is associated with worsening CEC pleomorphism and polymegathism. Especially, studies comparing patients with type-1 and type-2 DM (T1DM and T2DM, respectively) found that individuals with T1DM had more remarkable changes in CEC morphology (230, 233, 234).
The rate of cell density loss stabilizes to approximately 0.5% per year (235). Endothelial cell density (ECD) is an indirect marker of endothelial health and function (223–225, 235–239). The rate of CEC loss and the subsequent decrease in ECD speed up in patients with DM (225, 230, 234, 237, 238, 240–244). It should be noted that patients with T1DM (compared to T2DM) and those with a longer disease duration sustain a more severe decline in ECD.
It is widely known that an increase in central corneal thickness (CCT) could serve as one of the earliest signs of CEC dysfunction (245). Many researchers found that T1DM subjects have a higher CCT (238, 245–247). In fact, there have also been reports of a difference in CCT between T1DM and T2DM while few studies have found CCT and DM are unrelated.
Pathologic mechanisms
The pathogenesis of corneal endotheliopathy in diabetes is still less studied. The reported mechanisms mainly include mitophagy impairment, endoplasmic reticulum (ER) stress and pyroptosis.
Mitophagy is a highly selective form of autophagy that eliminates dysfunctional or excess mitochondria under stressful conditions, such as hypoxia (248). In our recent study, we demonstrated that hyperglycemia causes abnormal endothelial cell morphology and impaired mitophagy, leading to the accumulation of damaged mitochondria. In vivo data also confirmed that increased mitophagy had a protective effect on the CE of diabetic mice. Our results suggest that regulating mitophagy may be a promising strategy for the treatment of diabetic corneal endothelial dysfunction (249).
The ER stress response is a vital regulatory mechanism that maintains intracellular homeostasis (250, 251). The overactivation of the ER stress response and mitochondrial dysfunction are prominent etiological factors in the development of diabetes (252). We observed ER stress response activation in diabetic mice and diabetic human corneal endothelial cells, which induced CEC-specific morphological changes. Persistent ER stress response activation can cause CEC loss and corneal endothelial dysfunction (Figure 4). Consequently, in DM, the inhibition of ER stress could mitigate endothelial cell loss and corneal edema via the mitochondrial pathway (253).
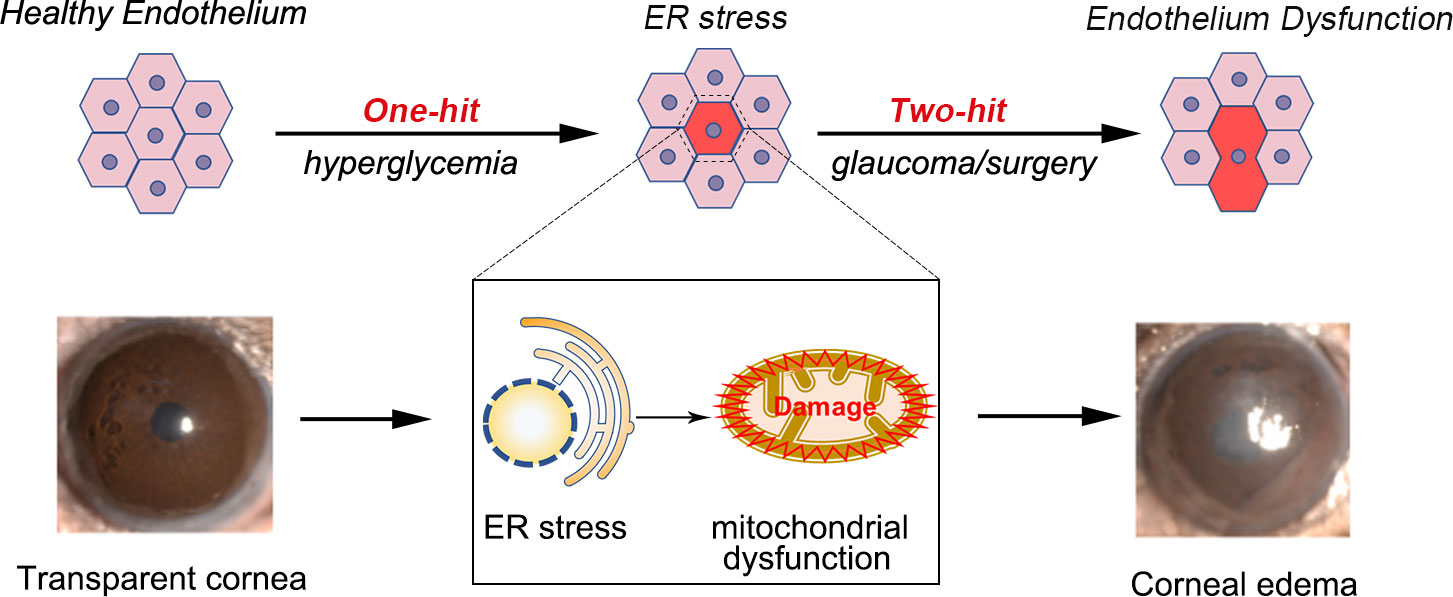
Figure 4 Proposed mechanism of endothelium dysfunction in the diabetic cornea. Endothelial cells from diabetic mice exhibit high levels of ER stress and ER stress appears before morphological changes of the endothelium. Activation of ER stress can promote corneal endothelial dysfunction by triggering mitochondrial dysfunction. Acute injuries, such as glaucoma, can lead to corneal edema and endothelial cell loss.
Pyroptosis is a recently discovered form of programmed cell death that is related to inflammation (254–256). Zhang et al. unraveled the novel role of long non-coding (lnc) RNA KCNQ1OT1 in pyroptosis, whereby KCNQ1OT1-repressed micro-RNA (miR)-214 expression upregulated the expression of the inflammatory molecule Caspase-1 and promoted pyroptosis in vitro and in vivo. Additionally, KCNQ1OT1 acts as a competing endogenous (ce)RNA that competitively binds miR-214 to regulate Caspase-1 activity, thus promoting diabetic corneal endothelium dysfunction. Further study of the role of KCNQ1OT1 will be critical for understanding the pathogenesis of diabetic corneal endothelium dysfunction and will help identify new biomarkers or potential therapeutic targets to treat this debilitating condition (257).
Diabetic related dry eye
Diabetic lacrimal gland disorder
Characteristics of diabetic lacrimal gland
Patients with DM may have a higher prevalence of dry eye than the healthy population (258). It has been reported that dry eye disease affects about one-fifth of patients with T2DM and reduces the patients’ quality of life (13, 259, 260). Dry eye may be caused by impaired tear production or excessive tear evaporation and is associated with photophobia, red eyes, vision impairment, local pain, and pruritus. The tear film is the interface between the ocular surface and the environment, and it contains a tightly controlled complement of water, proteins and lipids. LG secretion of proteins and fluid into the tear film is essential for maintaining the health of the ocular surface.
DM impairs tear secretion and induces LG changes. Early studies have identified the involvement of insulin in disorder of LG, such as impaired secretion and a reduction in protein secretion (261, 262). Subsequent studies validated that lipid accumulation in the LG acinar increased with age in a non-obese diabetic (NOD) mouse model. This change is along with lymphocytic infiltration and destruction of the acini. In addition, LG cholesteryl esters obviously increased in these mice (263). Similarly, the polyol pathway was triggered by hyperglycemia in type-2 diabetes, and the accumulation of sorbitol within cells led to cellular edema and dysfunction, which finally resulted in LG dysfunction and decreased tear secretion (264). Recently, He et al. reported that hyperlipidemia affects LG function, including the inhibition of tear secretion, rising lipid accumulation, inflammation, and oxidative stress levels (265). Nakata et al. demonstrated that diabetes suppresses hemodialysis-induced increases in tear fluid secretion, which suggests that the autonomic control of the LG function may be compromised by neuropathy in patients with DM (266). Most recently, our results suggested that streptozotocin-induced type-1 diabetic mice exhibited the early onset of reduced tear secretion and LG weight compared to the symptoms of diabetic keratopathy (267).
Pathogenesis of diabetic lacrimal gland
Hyperglycemia, oxidative stress, nerve alterations may play an important role in the development of LG impairment (268) in DM. The detailed mechanisms have become clearer.
Mitochondria is the major source of intracellular reactive oxygen species and the target of oxidative damage (269–271). Previous studies had confirmed the existence of oxidative stress and mitochondrial dysfunction in the LG of dry eye mice (272, 273). In the type-1 diabetic model, oxygen consumption rate and basal extracellular acidification rate detection results suggested that the early onset of diabetic dry eye may be due to the susceptibility to a mitochondrial bioenergetic deficit in diabetic LG (Figure 5), while the application of mitochondria-targeted antioxidant SKQ1 may ameliorate diabetic dry eye and keratopathy.
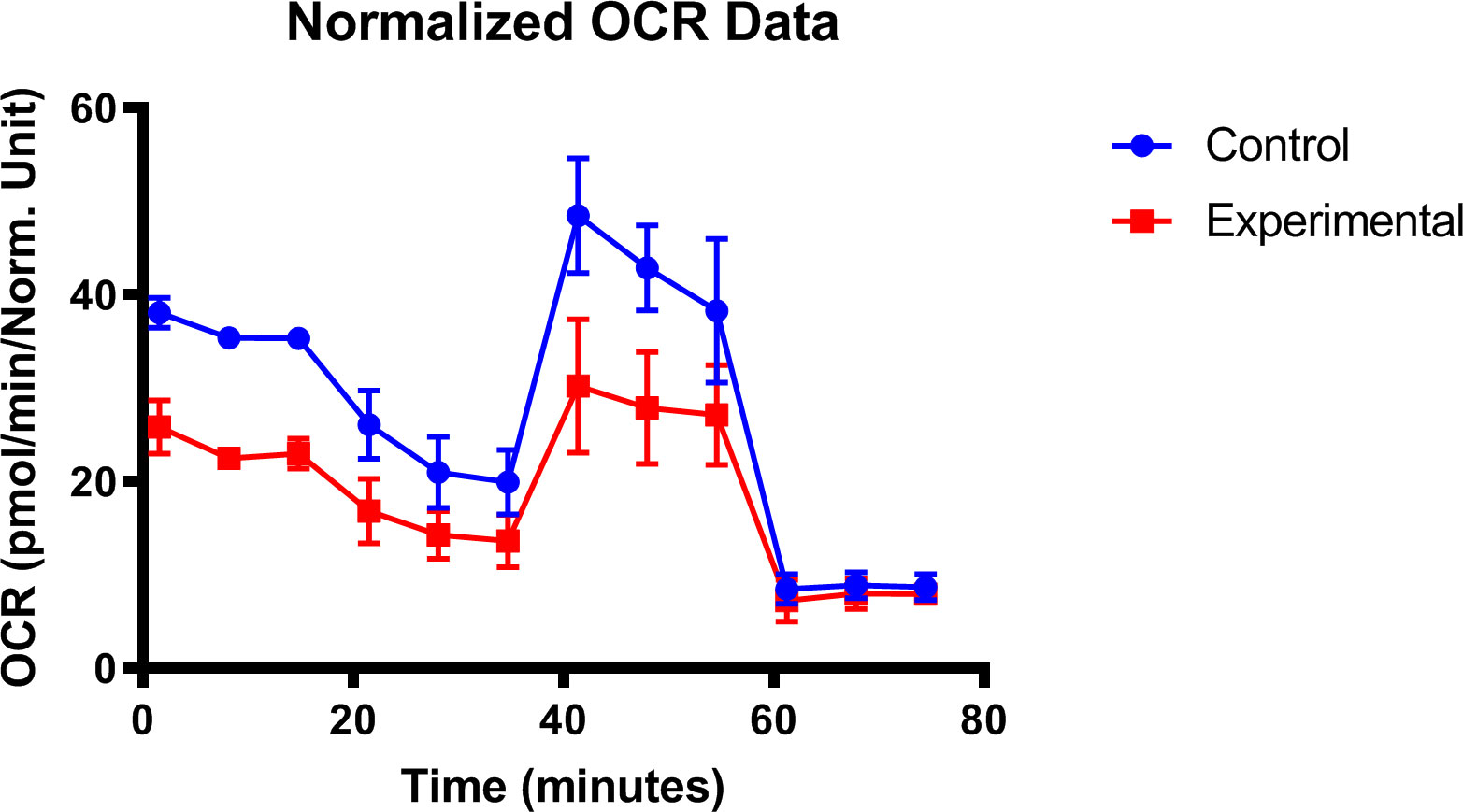
Figure 5 Mitochondrial dysfunction in diabetic lacrimal gland. Lacrimal gland cells from the diabetic mice after 16 weeks were evaluated with the seahorse XFp analyzer (ref. 267).
It is recognized that inflammation plays a prominent role in the development and propagation of dry eye. Hyperglycemia initiates an inflammatory cascade that generates the innate, adaptive immune responses of the lacrimal functional unit (LFU). The downstream immune-inflammatory regulators have been identified as matrix metalloproteinase-9 (MMP-9), immature antigen-presenting cells (APCs), CD4+ helper T cells (TH) subtype 1, and TH17 cell subsets, interferon-γ (IFN-γ) chemokines, chemokine receptors, cell adhesion molecules (CAMs), and interleukin-17 (IL-17) (274).
The neural response that regulates LG fluid secretion is an integral part of the LFU, which consists of the sensory afferent nerves of the cornea and conjunctiva, the efferent parasympathetic and sympathetic nerves that innervate the LG, the LG secretory cells, and the LG excretory ducts (275). Both anatomically and functionally, the parasympathetic system predominates, with overwhelming evidence indicating that the loss of parasympathetic innervation blocks LG functioning (276–283). Research has demonstrated that different densities of sympathetic innervation in glandular areas and the sympathetic denervation of the rabbit LG by ablating the superior cervical ganglion did not alter the LG acinar morphology and induced the denervation supersensitivity of protein secretion (284, 285). In addition, researchers have reported that the electrostimulation of the superior cervical ganglion increased tear secretion (286). However, the involvement of sympathetic stimulation in LG in DM remains poorly understood. Recently, we illustrated that the sympathetic pathway is activated in the pathogenesis of diabetic dry eye and may provide a potential strategy to counteract diabetic dry eye by interfering with sympathetic activity (Unpublished data).
Diabetic meibomian gland dysfunction
Clinical manifestation
Meibomian gland dysfunction (MGD) is an important cause of dry eye, and diabetes may be a risk factor. Studies reveal a high incidence of MGD in patients with diabetes (287–289), especially long-lasting diabetes (290, 291).Yu et al. (287) observed 132 eyes to assess the changes of Meibomian Glands (MGs) in type 2 DM. As the diabetes progressed, they found more MGs dropouts and absence of MGs in the DM group, and MG bubbles density were decreased with shape alterations, such as atrophy, fibrosis, expansion. The opening of glandular duct appeared to be atrophic and cornified. Additionally, lipid layer thickness (LLT), lid margin abnormalities, and tear breakup time (BUT) were significantly changed in diabetic patients; interestingly, the results of LLT were varied in different investigations (289, 290), which deserves further research. More importantly, some studies suggested that diabetes was associated with asymptomatic MGD, and it may be an early sign of ocular discomfort in T2D (290, 292). These findings suggest a lack of association between signs and symptoms. Therefore, it is alert to notice the signs of MGD in the absence of symptoms and perhaps the necessary treatment should be taken to prevent the progression of complications.
While MGD in type 2 DM has been widely investigated in the literature, studies on type 1 DM were very limited. Previous studies reported that BUT were lower in the Type 1 DM group and significantly associated with the duration of DM (293, 294). Semer et al. (295) evaluated the changes of MGs with Type 1 DM and found that in diabetic children, a higher secretion score and total eyelid score appeared. The thinning and shortening of MGs and presence of ghost areas were more common. In Type 1 DM animal model established by streptozotocin (STZ), more signs were founded, such as acini dropout, condensed lipid deposition at the orifice of the MG, disorganized acini and ducts, lipid metabolism disorder compared to those of non-diabetic controls (296, 297). Previous studies have documented peroxisome proliferator activator receptor-γ (PPARγ) plays a dominant role in regulating meibocyte differentiation and lipid synthesis (298, 299). Recent study has confirmed the reduced PPARγ in diabetic MGs, and upregulation of PPARγ could improve the production of lipid (300). Taken together, these indicated that pathological process of MGD could be observed in diabetic model induced by STZ, so, it may be used as vital tool for studying the physiopathology of MGD resulting from hyperglycemia. Generally, the pathogenesis of Type 1 DM differs from that of Type 2 DM, and distinctions in the presentation and progression of MGs between DM types has been seldom reported. Hence, future comparative investigations are necessary.
Pathogenesis of diabetic meibomian gland dysfunction
Unlike other sebaceous glands, the lipid secretion of MG is controlled by various neurotransmitter-neuromodulator mechanisms, and disparate neuropeptides/neurotransmitters play a role in the functioning of MG cells (301–303). The continuous proliferation and differentiation of MG cells are the basis for maintaining the secretion of lipids. Neuropathy, one of the most common complications of DM, may lead to MG dysfunction by disrupting the function of MG cells. Peripheral neuropathy may also alter meibum delivery to the ocular surface. Clinical studies have revealed that peripheral neuropathy causes a decline in nerve impulses emanating from the brain and corneal hypoesthesia, which leads to reduced blink rates (69, 70, 304). During blink movement, the muscle could produce a compression force to the tarsal plate and facilitate the delivery of the lipid from the MGs. Therefore, it is speculated that neuropathy leads to a decline in the blinking rate and meibum delivery forces, and ultimately leading to greater MGD prevalence in diabetes patients.
In diabetic patients, laser scanning confocal microscopy (LSCM) displayed the infiltration of inflammatory cells in the interstitial of gland bubble (305). In STZ-induced diabetic mouse model, more CD45 positive cells, such as macrophage and neutrophils, accumulated in MGs (297). Similarly, Yuli at al. found more inflammatory cells and overexpressed inflammatory factors in MG of diabetic rat. Genomic analysis techniques revealed that inflammation-related genes were upregulated in type 2 diabetic mice (306). In addition, more studies have demonstrated that the lipid homeostasis is related to the inflammation (307, 308). Many lipid species could regulate inflammatory responses. In turn, inflammation can alter the lipid metabolism. As a systemic metabolic disease, DM is closely associated with the lipid metabolism, and it has been recognized that diabetes induces the disruption of lipid homeostasis in MGs (297, 309). It was suggested that phospholipids (PLs) may play a key role in the inflammatory reaction. A higher level of PLs was observed in the meibum with DM, and the overexpression of PLs could release more inflammatory mediators, such as free fatty acid (FFA). FFA was considered to be toxic hydrolysate generated by microbial lipases from normal lipids, which would conversely induce inflammation and hyperkeratinization, thus damaging the ocular surface and MGs (310).
Potential treatment options
Diabetic ocular surface diseases is treated by local symptomatic treatment (such as the use of steroids to treat epithelial defect) on the premise of systemic control of blood glucose (such as insulin injection). However, the existing primary treatment methods cannot fully meet the treatment needs of diabetic ocular surface diseases, so it is necessary to find alternative treatment targets.
Stem cells therapy have been proposed as an emerging treatment option for diabetic keratopathy. Mesenchymal stem cells (MSCs) are a good choice for stem cell therapy due to their pluripotency and regenerative potential (311–314). MSCs exist in various tissues, including bone marrow, peripheral blood, adipose tissue, placenta, nervous tissue and so on. MSCs are known to play an important role in regulating tissue repair and immune inflammation through direct or indirect mechanisms. Our study based on bone marrow mesenchymal stem cells (BM-MSCs) on diabetic corneal wound healing found that the local transplantation of BM-MSCs significantly promoted the repair of corneal epithelium in type 1 diabetic mice. In mechanism, BM-MSCs alleviate diabetic corneal impairment by promoting the activation of corneal epithelial stem/progenitor cells and accelerating the polarization of macrophages to anti-inflammatory M2 phenotypes by secreting tumor necrosis factor-a–stimulated gene/protein-6 (TSG-6) (315).
Based on its ability to self-renew and promote regeneration, hemopoeitic stem cell (HSC) is another potential adult stem cell for disease therapy. Maha et al. assessed the possible effect of HSC therapy on STZ-induced diabetic keratopathy in albino rat and found that a tail vein injection of HSC ameliorated the changes of cornea and conjunctival epithelium caused by diabetic keratopathy (316).
Many studies in stem cell therapy have been conducted to restore corneal functioning, including autologous/allogeneic limbal stem cell transplantation (317), embryonic stem cells (ES)/induced pluripotent stem cells (iPS)-induced corneal cells (318, 319) and various adult stem cell (320, 321) treatments. Some have entered clinical trials; however, stem cell therapy in the field of diabetic keratopathy is still in its early stages. Although MSC and HSC transplantations have certain application prospects at the animal level, they are still far from clinical application, and further exploration is needed in the future.
Considering that cornea, lacrimal gland and meibomian gland are densely innervated, and neuropathy is one of the most common, complex and serious complications of diabetes patients, treatment based on neural regulation has also been emphasized. Exogenous supplementation of sensory neuropeptide SP, CGRP and parasympathetic neuropeptide VIP has been proven to effectively promote the regeneration of corneal epithelium and nerves in the experimental stage. As mentioned above, the therapeutic effects of various neurotrophic factors and axon guidance molecules on diabetic ocular surface diseases have also been successively verified in diabetic animal models. It is worth mentioning that Cenegermin (Oxervate™), an ophthalmic eye drops mainly composed of recombinant human NGF, was recently approved by the FDA for the treatment of neurotrophic keratopathy (322). In addition, our latest study found that sympathetic overactivation caused by diabetes also participated in the pathogenesis of diabetes keratopathy and diabetes related dry eye (Unpublished data). Sympathetic nerve-targeting regulation may also be a potential therapeutic target for diabetic ocular surface disease.
In addition, recent research has also revealed many other new methods to treat corneal epithelial defects, including the application of natural Chinese medicine (such as lycium barbarum polysaccharide) (323), various cell derived exosomes (324), and biological materials (such as hydrogel) (325). The mechanism revealed by these studies has something in common with the pathogenesis of DK, and maybe also used for developing new DK treatment methods, which may eventually open up a new way for developing new treatment methods to improve corneal wound healing.
Conclusion
With increasing clinical evidences of ocular surface damage in diabetic patients, ophthalmologists have gradually recognized the harm of diabetic ocular surface complications, and more basic ophthalmic research has focused on the disclosure of the pathogenesis and potential therapeutic targets of diabetic ocular surface complications.
The defined pathogenesis of diabetes keratopathy includes the accumulation of advanced glycation end products, the imbalance of growth factors and signaling pathways, the occurrence of persistent inflammation, the decline of neurotrophic function, the dysfunction of stem cells, the impairment of mitochondrial function, excessive oxidative stress, etc. Therefore, controlling inflammation and excessive oxidative stress, improving the function of stem cells and mitochondria, and targeting relevant growth factors, neurotrophic factors and signal pathways will be the direction of developing new targets for DK treatment, and guiding the clinical treatment of DK.
Diabetic dry eye was found to be closely associated with the abnormal mitochondrial function of lacrimal gland and the abnormal lipid metabolism of meibomian gland. For the treatment of dry eyes in diabetes, attention should be paid to improving tear secretion and meibomian gland lipid metabolism. Animal experiments have confirmed that promoting mitochondrial function has a good therapeutic effect on diabetic dry eyes, providing a basis for future clinical applications.
Clinical prospective studies have discerned that the early clinical symptoms of diabetic ocular surface complication are dry eye and corneal nerve degeneration, suggesting that early diagnosis should first examine corneal nerves changes using confocal microscopy and examine dry eye related clinical indicators. Further study on the interaction between neuro-epithelium and neuro-immunity will help to reveal the key pathogenic mechanism and formulate targeted intervention strategies for ocular surface complications of diabetes.
Author contributions
LX and QZ contributed to the manuscript design, discussion and revision. LY, QW, YL and CW contributed to the manuscript preparation and writing. All authors contributed to the article and approved the submitted version.
Funding
This work was supported by grants from the National Natural Science Foundation of China (82070927), Shandong Provincial key research and development program (2021ZDSYS14) and Academic Promotion Program and Innovation Project of Shandong First Medical University (2019ZL001 and 2019RC008).
Conflict of interest
The authors declare that the research was conducted in the absence of any commercial or financial relationships that could be construed as a potential conflict of interest.
Publisher’s note
All claims expressed in this article are solely those of the authors and do not necessarily represent those of their affiliated organizations, or those of the publisher, the editors and the reviewers. Any product that may be evaluated in this article, or claim that may be made by its manufacturer, is not guaranteed or endorsed by the publisher.
References
1. Khan MAB, Hashim MJ, King JK, Govender RD, Mustafa H, Al Kaabi J. Epidemiology of type 2 diabetes - global burden of disease and forecasted trends. J Epidemiol Glob Health (2020) 10(1):107–11. doi: 10.2991/jegh.k.191028.001
2. Cho NH, Shaw JE, Karuranga S, Huang Y, da Rocha Fernandes JD, Ohlrogge AW, et al. Idf diabetes atlas: Global estimates of diabetes prevalence for 2017 and projections for 2045. Diabetes Res Clin Pract (2018) 138:271–81. doi: 10.1016/j.diabres.2018.02.023
3. Purushothaman I, Zagon IS, Sassani JW, McLaughlin PJ. Ocular surface complications in diabetes: The interrelationship between insulin and enkephalin. Biochem Pharmacol (2021) 192:114712. doi: 10.1016/j.bcp.2021.114712
4. Yu FX, Lee PSY, Yang L, Gao N, Zhang Y, Ljubimov AV, et al. The impact of sensory neuropathy and inflammation on epithelial wound healing in diabetic corneas. Prog Retin Eye Res (2022) 89:101039. doi: 10.1016/j.preteyeres.2021.101039
5. Han SB, Yang HK, Hyon JY. Influence of diabetes mellitus on anterior segment of the eye. Clin Interv Aging (2019) 14:53–63. doi: 10.2147/CIA.S190713
6. Sayin N, Kara N, Pekel G. Ocular complications of diabetes mellitus. World J Diabetes (2015) 6(1):92–108. doi: 10.4239/wjd.v6.i1.92
7. Meek KM, Knupp C. Corneal structure and transparency. Prog Retin Eye Res (2015) 49:1–16. doi: 10.1016/j.preteyeres.2015.07.001
8. Wilson SE, Mohan RR, Mohan RR, Ambrósio R Jr., Hong J, Lee J. The corneal wound healing response: Cytokine-mediated interaction of the epithelium, stroma, and inflammatory cells. Prog Retin Eye Res (2001) 20(5):625–37. doi: 10.1016/s1350-9462(01)00008-8
9. Veernala I, Jaffet J, Fried J, Mertsch S, Schrader S, Basu S, et al. Lacrimal gland regeneration: The unmet challenges and promise for dry eye therapy. Ocular Surface (2022) 25:129–41. doi: 10.1016/j.jtos.2022.06.005
10. Chhadva P, Goldhardt R, Galor A. Meibomian gland disease: The role of gland dysfunction in dry eye disease. Ophthalmology (2017) 124(11s):S20–s6. doi: 10.1016/j.ophtha.2017.05.031
11. Misra SL, Patel DV, McGhee CN, Pradhan M, Kilfoyle D, Braatvedt GD, et al. Peripheral neuropathy and tear film dysfunction in type 1 diabetes mellitus. J Diabetes Res (2014) 2014:848659. doi: 10.1155/2014/848659
12. Asiedu K, Dhanapalaratnam R, Krishnan AV, Kwai N, Poynten A, Markoulli M. Impact of peripheral and corneal neuropathy on markers of ocular surface discomfort in diabetic chronic kidney disease. Optom Vis Sci (2022) 99(11):807–16. doi: 10.1097/opx.0000000000001955
13. Zhang X, Zhao L, Deng S, Sun X, Wang N. Dry eye syndrome in patients with diabetes mellitus: Prevalence, etiology, and clinical characteristics. J Ophthalmol (2016) 2016:8201053. doi: 10.1155/2016/8201053
14. Priyadarsini S, Whelchel A, Nicholas S, Sharif R, Riaz K, Karamichos D. Diabetic keratopathy: Insights and challenges. Surv Ophthalmol (2020) 65(5):513–29. doi: 10.1016/j.survophthal.2020.02.005
15. Lane JD, Krumholz DM, Sack RA, Morris C. Tear glucose dynamics in diabetes mellitus. Curr Eye Res (2006) 31(11):895–901. doi: 10.1080/02713680600976552
16. Sen DK, Sarin GS. Tear glucose levels in normal people and in diabetic patients. Br J Ophthalmol (1980) 64(9):693–5. doi: 10.1136/bjo.64.9.693
17. Zhu L, Titone R, Robertson DM. The impact of hyperglycemia on the corneal epithelium: Molecular mechanisms and insight. Ocular Surface (2019) 17(4):644–54. doi: 10.1016/j.jtos.2019.06.007
18. Sady C, Khosrof S, Nagaraj R. Advanced maillard reaction and crosslinking of corneal collagen in diabetes. Biochem Biophys Res Commun (1995) 214(3):793–7. doi: 10.1006/bbrc.1995.2356
19. Rehany U, Ishii Y, Lahav M, Rumelt S. Ultrastructural changes in corneas of diabetic patients: An electron-microscopy study. Cornea (2000) 19(4):534–8. doi: 10.1097/00003226-200007000-00026
20. Saini JS, Khandalavla B. Corneal epithelial fragility in diabetes mellitus. Can J Ophthalmol (1995) 30(3):142–6.
21. Quadrado MJ, Popper M, Morgado AM, Murta JN, Van Best JA. Diabetes and corneal cell densities in humans by in vivo confocal microscopy. Cornea (2006) 25(7):761–8. doi: 10.1097/01.ico.0000224635.49439.d1
22. Yusufoğlu E, Güngör Kobat S, Keser S. Evaluation of central corneal epithelial thickness with anterior segment Oct in patients with type 2 diabetes mellitus. Int Ophthalmol (2022). doi: 10.1007/s10792-022-02384-5
23. Meller D, Augustin AJ, Koch FH. A modified technique of impression cytology to study the fine structure of corneal epithelium. Ophthalmic Res (1996) 28(2):71–9. doi: 10.1159/000267877
24. Wang F, Gao N, Yin J, Yu FS. Reduced innervation and delayed re-innervation after epithelial wounding in type 2 diabetic goto-kakizaki rats. Am J Pathol (2012) 181(6):2058–66. doi: 10.1016/j.ajpath.2012.08.029
25. Frueh BE, Körner U, Böhnke M. Confocal microscopy of the cornea in patients with diabetes. Klin Monbl Augenheilkd (1995) 206(5):317–9. doi: 10.1055/s-2008-1035450
26. Deák EA, Szalai E, Tóth N, Malik RA, Berta A, Csutak A. Longitudinal changes in corneal cell and nerve fiber morphology in young patients with type 1 diabetes with and without diabetic retinopathy: A 2-year follow-up study. Invest Ophthalmol Vis Sci (2019) 60(2):830–7. doi: 10.1167/iovs.18-24516
27. Liu T, Sun DP, Li DF, Bi WJ, Xie LX. Observation and quantification of diabetic keratopathy in type 2 diabetes patients using in vivo laser confocal microscopy. Zhonghua Yan Ke Za Zhi (2020) 56(10):754–60. doi: 10.3760/cma.j.cn112142-20200108-00012
28. Chang PY, Carrel H, Huang JS, Wang IJ, Hou YC, Chen WL, et al. Decreased density of corneal basal epithelium and subbasal corneal nerve bundle changes in patients with diabetic retinopathy. Am J Ophthalmol (2006) 142(3):488–90. doi: 10.1016/j.ajo.2006.04.033
29. Ishibashi F, Kawasaki A, Yamanaka E, Kosaka A, Uetake H. Morphometric features of corneal epithelial basal cells, and their relationship with corneal nerve pathology and clinical factors in patients with type 2 diabetes. J Diabetes Investig (2013) 4(5):492–501. doi: 10.1111/jdi.12083
30. Rosenberg ME, Tervo TMT, Immonen IJ, Müller LJ, Grönhagen–Riska C, Vesaluoma MH. Corneal structure and sensitivity in type 1 diabetes mellitus. Invest Ophthalmol Vis Sci (2000) 41:2915–21. doi: 10.1016/s0002-9394(00)00839-4
31. Cai D, Zhu M, Petroll WM, Koppaka V, Robertson DM. The impact of type 1 diabetes mellitus on corneal epithelial nerve morphology and the corneal epithelium. Am J Pathol (2014) 184(10):2662–70. doi: 10.1016/j.ajpath.2014.06.016
32. Göbbels M, Spitznas M, Oldendoerp J. Impairment of corneal epithelial barrier function in diabetics. Graefes Arch Clin Exp Ophthalmol (1989) 227(2):142–4. doi: 10.1007/bf02169787
33. Chang SW, Hsu HC, Hu FR, Chen MS. Corneal autofluorescence and epithelial barrier function in diabetic patients. Ophthalmic Res (1995) 27(2):74–9. doi: 10.1159/000267600
34. Gekka M, Miyata K, Nagai Y, Nemoto S, Sameshima T, Tanabe T, et al. Corneal epithelial barrier function in diabetic patients. Cornea (2004) 23(1):35–7. doi: 10.1097/00003226-200401000-00006
35. Dan J, Zhou Q, Zhai H, Cheng J, Wan L, Ge C, et al. Clinical analysis of fungal keratitis in patients with and without diabetes. PloS One (2018) 13(5):e0196741. doi: 10.1371/journal.pone.0196741
36. Keay L, Edwards K, Stapleton F. Signs, symptoms, and comorbidities in contact lens-related microbial keratitis. Optom Vis Sci (2009) 86(7):803–9. doi: 10.1097/OPX.0b013e3181ae1b69
37. Stapleton F, Carnt N. Contact lens-related microbial keratitis: How have epidemiology and genetics helped us with pathogenesis and prophylaxis. Eye (Lond) (2012) 26(2):185–93. doi: 10.1038/eye.2011.288
38. Ting DSJ, Ho CS, Deshmukh R, Said DG, Dua HS. Infectious keratitis: An update on epidemiology, causative microorganisms, risk factors, and antimicrobial resistance. Eye (Lond) (2021) 35(4):1084–101. doi: 10.1038/s41433-020-01339-3
39. Wang B, Yang S, Zhai HL, Zhang YY, Cui CX, Wang JY, et al. A comparative study of risk factors for corneal infection in diabetic and non-diabetic patients. Int J Ophthalmol (2018) 11(1):43–7. doi: 10.18240/ijo.2018.01.08
40. Alfuraih S, Barbarino A, Ross C, Shamloo K, Jhanji V, Zhang M, et al. Effect of high glucose on ocular surface epithelial cell barrier and tight junction proteins. Invest Ophthalmol Vis Sci (2020) 61(11):3. doi: 10.1167/iovs.61.11.3
41. Semeraro F, Forbice E, Romano V, Angi M, Romano MR, Filippelli ME, et al. Neurotrophic keratitis. Ophthalmologica (2014) 231(4):191–7. doi: 10.1159/000354380
42. Skarbez K, Priestley Y, Hoepf M, Koevary SB. Comprehensive review of the effects of diabetes on ocular health. Expert Rev Ophthalmol (2010) 5(4):557–77. doi: 10.1586/eop.10.44
43. Fraunfelder FW, Rich LF. Laser-assisted in situ keratomileusis complications in diabetes mellitus. Cornea (2002) 21(3):246–8. doi: 10.1097/00003226-200204000-00002
44. Jabbur NS, Chicani CF, Kuo IC, O’Brien TP. Risk factors in interface epithelialization after laser in situ keratomileusis. J Refract Surg (2004) 20(4):343–8. doi: 10.3928/1081-597x-20040701-07
45. Cobo-Soriano R, Beltrán J, Baviera J. Lasik outcomes in patients with underlying systemic contraindications: A preliminary study. Ophthalmology (2006) 113(7):1118. doi: 10.1016/j.ophtha.2006.02.023
46. Halkiadakis I, Belfair N, Gimbel HV. Laser in situ keratomileusis in patients with diabetes. J Cataract Refract Surg (2005) 31(10):1895–8. doi: 10.1016/j.jcrs.2005.03.075
47. Mohammadpour M. Lasik and systemic contraindications. Ophthalmology (2007) 114(5):1032–3. doi: 10.1016/j.ophtha.2007.02.009
48. Sarici K, Petkovsek D, Martin A, Yuan A, Goshe JM, Srivastava SK, et al. Corneal epithelial defects following vitreoretinal surgery: Incidence and outcomes from the discover study. Int J Ophthalmol (2022) 15(1):83–8. doi: 10.18240/ijo.2022.01.13
49. Wang Y, Li D, Su W, Dai Y. Clinical features, risk factors, and therapy of epithelial keratitis after cataract surgery. J Ophthalmol (2021) 2021:6636228. doi: 10.1155/2021/6636228
50. Yeung A, Dwarakanathan S. Diabetic keratopathy. Dis Mon (2021) 67(5):101135. doi: 10.1016/j.disamonth.2021.101135
51. Patel DV, McGhee CN. Mapping of the normal human corneal Sub-basal nerve plexus by in vivo laser scanning confocal microscopy. Invest Ophthalmol Vis Sci (2005) 46(12):4485–8. doi: 10.1167/iovs.05-0794
52. Müller LJ, Marfurt CF, Kruse F, Tervo TM. Corneal nerves: Structure, contents and function. Exp Eye Res (2003) 76(5):521–42. doi: 10.1016/s0014-4835(03)00050-2
53. Bikbova G, Oshitari T, Baba T, Bikbov M, Yamamoto S. Diabetic corneal neuropathy: Clinical perspectives. Clin Ophthalmol (2018) 12:981–7. doi: 10.2147/opth.S145266
54. Lewis EJH, Lovblom LE, Ferdousi M, Halpern EM, Jeziorska M, Pacaud D, et al. Rapid corneal nerve fiber loss: A marker of diabetic neuropathy onset and progression. Diabetes Care (2020) 43(8):1829–35. doi: 10.2337/dc19-0951
55. Bu Y, Shih KC, Tong L. The ocular surface and diabetes, the other 21st century epidemic. Exp Eye Res (2022) 220:109099. doi: 10.1016/j.exer.2022.109099
56. Snyder MJ, Gibbs LM, Lindsay TJ. Treating painful diabetic peripheral neuropathy: An update. Am Fam Physician (2016) 94(3):227–34.
57. Ziegler D, Papanas N, Zhivov A, Allgeier S, Winter K, Ziegler I, et al. Early detection of nerve fiber loss by corneal confocal microscopy and skin biopsy in recently diagnosed type 2 diabetes. Diabetes (2014) 63(7):2454–63. doi: 10.2337/db13-1819
58. Petropoulos IN, Alam U, Fadavi H, Asghar O, Green P, Ponirakis G, et al. Corneal nerve loss detected with corneal confocal microscopy is symmetrical and related to the severity of diabetic polyneuropathy. Diabetes Care (2013) 36(11):3646–51. doi: 10.2337/dc13-0193
59. Davidson EP, Coppey LJ, Kardon RH, Yorek MA. Differences and similarities in development of corneal nerve damage and peripheral neuropathy and in diet-induced obesity and type 2 diabetic rats. Invest Ophthalmol Vis Sci (2014) 55(3):1222–30. doi: 10.1167/iovs.13-13794
60. De Cillà S, Ranno S, Carini E, Fogagnolo P, Ceresara G, Orzalesi N, et al. Corneal subbasal nerves changes in patients with diabetic retinopathy: An in vivo confocal study. Invest Ophthalmol Vis Sci (2009) 50(11):5155–8. doi: 10.1167/iovs.09-3384
61. He J, Bazan HE. Mapping the nerve architecture of diabetic human corneas. Ophthalmology (2012) 119(5):956–64. doi: 10.1016/j.ophtha.2011.10.036
62. Stem MS, Hussain M, Lentz SI, Raval N, Gardner TW, Pop-Busui R, et al. Differential reduction in corneal nerve fiber length in patients with type 1 or type 2 diabetes mellitus. J Diabetes Complications (2014) 28(5):658–61. doi: 10.1016/j.jdiacomp.2014.06.007
63. Zhivov A, Winter K, Hovakimyan M, Peschel S, Harder V, Schober HC, et al. Imaging and quantification of subbasal nerve plexus in healthy volunteers and diabetic patients with or without retinopathy. PloS One (2013) 8(1):e52157. doi: 10.1371/journal.pone.0052157
64. Kallinikos P, Berhanu M, O’Donnell C, Boulton AJ, Efron N, Malik RA. Corneal nerve tortuosity in diabetic patients with neuropathy. Invest Ophthalmol Vis Sci (2004) 45(2):418–22. doi: 10.1167/iovs.03-0637
65. Malik RA, Kallinikos P, Abbott CA, van Schie CH, Morgan P, Efron N, et al. Corneal confocal microscopy: A non-invasive surrogate of nerve fibre damage and repair in diabetic patients. Diabetologia (2003) 46(5):683–8. doi: 10.1007/s00125-003-1086-8
66. Mocan MC, Durukan I, Irkec M, Orhan M. Morphologic alterations of both the stromal and subbasal nerves in the corneas of patients with diabetes. Cornea (2006) 25(7):769–73. doi: 10.1097/01.ico.0000224640.58848.54
67. Roszkowska AM, Licitra C, Tumminello G, Postorino El, Colonna MR, Aragona P. Corneal nerves in diabetes-The role of the in vivo corneal confocal microscopy of the subbasal nerve plexus in the assessment of peripheral small fiber neuropathy. Surv Ophthalmol (2021) 66(3):493–513. doi: 10.1016/j.survophthal.2020.09.003
68. Szalai E, Deák E, Módis L Jr., Németh G, Berta A, Nagy A, et al. Early corneal cellular and nerve fiber pathology in young patients with type 1 diabetes mellitus identified using corneal confocal microscopy. Invest Ophthalmol Vis Sci (2016) 57(3):853–8. doi: 10.1167/iovs.15-18735
69. Cousen P, Cackett P, Bennett H, Swa K, Dhillon B. Tear production and corneal sensitivity in diabetes. J Diabetes Complications (2007) 21(6):371–3. doi: 10.1016/j.jdiacomp.2006.05.008
70. Saito J, Enoki M, Hara M, Morishige N, Chikama T, Nishida T. Correlation of corneal sensation, but not of basal or reflex tear secretion, with the stage of diabetic retinopathy. Cornea (2003) 22(1):15–8. doi: 10.1097/00003226-200301000-00004
71. Tavakoli M, Kallinikos PA, Efron N, Boulton AJ, Malik RA. Corneal sensitivity is reduced and relates to the severity of neuropathy in patients with diabetes. Diabetes Care (2007) 30(7):1895–7. doi: 10.2337/dc07-0175
72. Pritchard N, Edwards K, Dehghani C, Fadavi H, Jeziorska M, Marshall A, et al. Longitudinal assessment of neuropathy in type 1 diabetes using novel ophthalmic markers (Landmark): Study design and baseline characteristics. Diabetes Res Clin Pract (2014) 104(2):248–56. doi: 10.1016/j.diabres.2014.02.011
73. Sitompul R. Corneal sensitivity as a potential marker of diabetic neuropathy. Acta Med Indones (2017) 49(2):166–72.
74. Gao N, Yan C, Lee P, Sun H, Yu FS. Dendritic cell dysfunction and diabetic sensory neuropathy in the cornea. J Clin Invest (2016) 126(5):1998–2011. doi: 10.1172/jci85097
75. Edwards K, Pritchard N, Vagenas D, Russell A, Malik RA, Efron N. Utility of corneal confocal microscopy for assessing mild diabetic neuropathy: Baseline findings of the landmark study. Clin Exp Optom (2012) 95(3):348–54. doi: 10.1111/j.1444-0938.2012.00740.x
76. So WZ, Qi Wong NS, Tan HC, Yu Lin MT, Yu Lee IX, Mehta JS, et al. Diabetic corneal neuropathy as a surrogate marker for diabetic peripheral neuropathy. Neural Regener Res (2022) 17(10):2172–8. doi: 10.4103/1673-5374.327364
77. Pesavento F, Lovato A, Cappello S, Postiglione M. Acupuncture in the treatment of dry eye syndrome with anxiety symptoms. a case report. Eur J Transl Myol (2022) 32(2):10482. doi: 10.4081/ejtm.2022.10482
78. Ljubimov AV. Diabetic complications in the cornea. Vision Res (2017) 139:138–52. doi: 10.1016/j.visres.2017.03.002
79. Bu Y, Shih KC, Kwok SS, Chan YK, Lo AC, Chan TCY, et al. Experimental modeling of cornea wound healing in diabetes: Clinical applications and beyond. BMJ Open Diabetes Res Care (2019) 7(1):e000779. doi: 10.1136/bmjdrc-2019-000779
80. Chen K, Li Y, Zhang X, Ullah R, Tong J, Shen Y. The role of the Pi3k/Akt signalling pathway in the corneal epithelium: Recent updates. Cell Death Dis (2022) 13(5):513. doi: 10.1038/s41419-022-04963-x
81. Donath MY, Dinarello CA, Mandrup-Poulsen T. Targeting innate immune mediators in type 1 and type 2 diabetes. Nat Rev Immunol (2019) 19(12):734–46. doi: 10.1038/s41577-019-0213-9
82. Tang SCW, Yiu WH. Innate immunity in diabetic kidney disease. Nat Rev Nephrol (2020) 16(4):206–22. doi: 10.1038/s41581-019-0234-4
83. Wada J, Makino H. Innate immunity in diabetes and diabetic nephropathy. Nat Rev Nephrol (2016) 12(1):13–26. doi: 10.1038/nrneph.2015.175
84. Swanson KV, Deng M, Ting JP. The Nlrp3 inflammasome: Molecular activation and regulation to therapeutics. Nat Rev Immunol (2019) 19(8):477–89. doi: 10.1038/s41577-019-0165-0
85. Sharma BR, Kanneganti TD. Nlrp3 inflammasome in cancer and metabolic diseases. Nat Immunol (2021) 22(5):550–9. doi: 10.1038/s41590-021-00886-5
86. Chen H, Zhang X, Liao N, Mi L, Peng Y, Liu B, et al. Enhanced expression of Nlrp3 inflammasome-related inflammation in diabetic retinopathy. Invest Ophthalmol Vis Sci (2018) 59(2):978–85. doi: 10.1167/iovs.17-22816
87. Raman KS, Matsubara JA. Dysregulation of the Nlrp3 inflammasome in diabetic retinopathy and potential therapeutic targets. Ocul Immunol Inflammation (2022) 30(2):470–8. doi: 10.1080/09273948.2020.1811350
88. Yang F, Qin Y, Wang Y, Meng S, Xian H, Che H, et al. Metformin inhibits the Nlrp3 inflammasome Via Ampk/Mtor-dependent effects in diabetic cardiomyopathy. Int J Biol Sci (2019) 15(5):1010–9. doi: 10.7150/ijbs.29680
89. Wan L, Bai X, Zhou Q, Chen C, Wang H, Liu T, et al. The advanced glycation end-products (Ages)/Ros/Nlrp3 inflammasome axis contributes to delayed diabetic corneal wound healing and nerve regeneration. Int J Biol Sci (2022) 18(2):809–25. doi: 10.7150/ijbs.63219
90. Wang Y, Wan L, Zhang Z, Li J, Qu M, Zhou Q. Topical calcitriol application promotes diabetic corneal wound healing and reinnervation through inhibiting Nlrp3 inflammasome activation. Exp Eye Res (2021) 209:108668. doi: 10.1016/j.exer.2021.108668
91. Mirza RE, Fang MM, Weinheimer-Haus EM, Ennis WJ, Koh TJ. Sustained inflammasome activity in macrophages impairs wound healing in type 2 diabetic humans and mice. Diabetes (2014) 63(3):1103–14. doi: 10.2337/db13-0927
92. Liu D, Yang P, Gao M, Yu T, Shi Y, Zhang M, et al. Nlrp3 activation induced by neutrophil extracellular traps sustains inflammatory response in the diabetic wound. Clin Sci (Lond) (2019) 133(4):565–82. doi: 10.1042/cs20180600
93. Huang W, Jiao J, Liu J, Huang M, Hu Y, Ran W, et al. Mfg-E8 accelerates wound healing in diabetes by regulating “Nlrp3 inflammasome-neutrophil extracellular traps” axis. Cell Death Discovery (2020) 6:84. doi: 10.1038/s41420-020-00318-7
94. Kaji Y, Usui T, Oshika T, Matsubara M, Yamashita H, Araie M, et al. Advanced glycation end products in diabetic corneas. Invest Ophthalmol Vis Sci (2000) 41(2):362–8.
95. Zou C, Wang S, Huang F, Zhang YA. Advanced glycation end products and ultrastructural changes in corneas of long-term streptozotocin-induced diabetic monkeys. Cornea (2012) 31(12):1455–9. doi: 10.1097/ICO.0b013e3182490907
96. Kaji Y, Amano S, Usui T, Suzuki K, Tanaka S, Oshika T, et al. Advanced glycation end products in descemet’s membrane and their effect on corneal endothelial cell. Curr Eye Res (2001) 23(6):469–77. doi: 10.1076/ceyr.23.6.469.6968
97. Newton K, Dixit VM, Kayagaki N. Dying cells fan the flames of inflammation. Science (2021) 374(6571):1076–80. doi: 10.1126/science.abi5934
98. Yan C, Gao N, Sun H, Yin J, Lee P, Zhou L, et al. Targeting imbalance between il-1beta and il-1 receptor antagonist ameliorates delayed epithelium wound healing in diabetic mouse corneas. Am J Pathol (2016) 186(6):1466–80. doi: 10.1016/j.ajpath.2016.01.019
99. Fadini GP, Menegazzo L, Rigato M, Scattolini V, Poncina N, Bruttocao A, et al. Netosis delays diabetic wound healing in mice and humans. Diabetes (2016) 65(4):1061–71. doi: 10.2337/db15-0863
100. Wang Y, Luo W, Han J, Khan ZA, Fang Q, Jin Y, et al. Md2 activation by direct age interaction drives inflammatory diabetic cardiomyopathy. Nat Commun (2020) 11(1):2148. doi: 10.1038/s41467-020-15978-3
101. Ramke M, Zhou X, Materne EC, Rajaiya J, Chodosh J. Resident corneal c-fms(+) macrophages and dendritic cells mediate early cellular infiltration in adenovirus keratitis. Exp Eye Res (2016) 147:144–7. doi: 10.1016/j.exer.2016.05.016
102. Hamrah P, Liu Y, Zhang Q, Dana MR. The corneal stroma is endowed with a significant number of resident dendritic cells. Invest Ophthalmol Vis Sci (2003) 44(2):581–9. doi: 10.1167/iovs.02-0838
103. Wu M, Hill LJ, Downie LE, Chinnery HR. Neuroimmune crosstalk in the cornea: The role of immune cells in corneal nerve maintenance during homeostasis and inflammation. Prog Retin Eye Res (2022), 101105. doi: 10.1016/j.preteyeres.2022.101105
104. Xue Y, He J, Xiao C, Guo Y, Fu T, Liu J, et al. The mouse autonomic nervous system modulates inflammation and epithelial renewal after corneal abrasion through the activation of distinct local macrophages. Mucosal Immunol (2018) 11(5):1496–511. doi: 10.1038/s41385-018-0031-6
105. Liu J, Xue Y, Dong D, Xiao C, Lin C, Wang H, et al. Ccr2(-) and Ccr2(+) corneal macrophages exhibit distinct characteristics and balance inflammatory responses after epithelial abrasion. Mucosal Immunol (2017) 10(5):1145–59. doi: 10.1038/mi.2016.139
106. Gao N, Yin J, Yoon GS, Mi QS, Yu FS. Dendritic cell-epithelium interplay is a determinant factor for corneal epithelial wound repair. Am J Pathol (2011) 179(5):2243–53. doi: 10.1016/j.ajpath.2011.07.050
107. Meshkani R, Vakili S. Tissue resident macrophages: Key players in the pathogenesis of type 2 diabetes and its complications. Clin Chim Acta (2016) 462:77–89. doi: 10.1016/j.cca.2016.08.015
108. Maschalidi S, Mehrotra P, Keçeli BN, De Cleene HKL, Lecomte K, van der Cruyssen R, et al. Targeting Slc7a11 improves efferocytosis by dendritic cells and wound healing in diabetes. Nature (2022) 606(7915):776–84. doi: 10.1038/s41586-022-04754-6
109. Kraakman MJ, Murphy AJ, Jandeleit-Dahm K, Kammoun HL. Macrophage polarization in obesity and type 2 diabetes: Weighing down our understanding of macrophage function? Front Immunol (2014) 5:470. doi: 10.3389/fimmu.2014.00470
110. Kim H, Kim M, Lee HY, Park HY, Jhun H, Kim S. Role of dendritic cell in diabetic nephropathy. Int J Mol Sci (2021) 22(14):7554. doi: 10.3390/ijms22147554
111. Zhang Y, Gao N, Wu L, Lee PSY, Me R, Dai C, et al. Role of vip and sonic hedgehog signaling pathways in mediating epithelial wound healing, sensory nerve regeneration, and their defects in diabetic corneas. Diabetes (2020) 69(7):1549–61. doi: 10.2337/db19-0870
112. Wang J, Hossain M, Thanabalasuriar A, Gunzer M, Meininger C, Kubes P. Visualizing the function and fate of neutrophils in sterile injury and repair. Science (2017) 358(6359):111–6. doi: 10.1126/science.aam9690
113. Wong SL, Demers M, Martinod K, Gallant M, Wang Y, Goldfine AB, et al. Diabetes primes neutrophils to undergo netosis, which impairs wound healing. Nat Med (2015) 21(7):815–9. doi: 10.1038/nm.3887
114. Papayannopoulos V. Neutrophil extracellular traps in immunity and disease. Nat Rev Immunol (2018) 18(2):134–47. doi: 10.1038/nri.2017.105
115. Zhang J, Dai Y, Wei C, Zhao X, Zhou Q, Xie L. Dnase I improves corneal epithelial and nerve regeneration in diabetic mice. J Cell Mol Med (2020) 24(8):4547–56. doi: 10.1111/jcmm.15112
116. Herre M, Cedervall J, Mackman N, Olsson AK. Neutrophil extracellular traps in the pathology of cancer and other inflammatory diseases. Physiol Rev (2023) 103(1):277–312. doi: 10.1152/physrev.00062.2021
117. Barnes BJ, Adrover JM, Baxter-Stoltzfus A, Borczuk A, Cools-Lartigue J, Crawford JM, et al. Targeting potential drivers of covid-19: Neutrophil extracellular traps. J Exp Med (2020) 217(6):e20200652. doi: 10.1084/jem.20200652
118. Lambiase A, Rama P, Bonini S, Caprioglio G, Aloe L. Topical treatment with nerve growth factor for corneal neurotrophic ulcers. N Engl J Med (1998) 338(17):1174–80. doi: 10.1056/nejm199804233381702
119. Lambiase A, Micera A, Sacchetti M, Cortes M, Mantelli F, Bonini S. Alterations of tear neuromediators in dry eye disease. Arch Ophthalmol (2011) 129(8):981–6. doi: 10.1001/archophthalmol.2011.200
120. Nishida T, Nakamura M, Ofuji K, Reid TW, Mannis MJ, Murphy CJ. Synergistic effects of substance p with insulin-like growth factor-1 on epithelial migration of the cornea. J Cell Physiol (1996) 169(1):159–66. doi: 10.1002/(SICI)1097-4652(199610)169:1<159::AID-JCP16>3.0.CO;2-8
121. Nakamura M, Ofuji K, Chikama T, Nishida T. Combined effects of substance p and insulin-like growth factor-1 on corneal epithelial wound closure of rabbit in vivo. Curr Eye Res (1997) 16(3):275–8. doi: 10.1076/ceyr.16.3.275.15409
122. Nakamura M, Chikama T, Nishida T. Up-regulation of integrin alpha 5 expression by combination of substance p and insulin-like growth factor-1 in rabbit corneal epithelial cells. Biochem Biophys Res Commun (1998) 246(3):777–82. doi: 10.1006/bbrc.1998.8704
123. Reid TW, Murphy CJ, Iwahashi CK, Foster BA, Mannis MJ. Stimulation of epithelial cell growth by the neuropeptide substance p. J Cell Biochem (1993) 52(4):476–85. doi: 10.1002/jcb.240520411
124. Garcia-Hirschfeld J, Lopez-Briones LG, Belmonte C. Neurotrophic influences on corneal epithelial cells. Exp Eye Res (1994) 59(5):597–605. doi: 10.1006/exer.1994.1145
125. Mikulec AA, Tanelian DL. Cgrp increases the rate of corneal re-epithelialization in an in vitro whole mount preparation. J Ocul Pharmacol Ther (1996) 12(4):417–23. doi: 10.1089/jop.1996.12.417
126. Yang L, Di G, Qi X, Qu M, Wang Y, Duan H, et al. Substance p promotes diabetic corneal epithelial wound healing through molecular mechanisms mediated Via the neurokinin-1 receptor. Diabetes (2014) 63(12):4262–74. doi: 10.2337/db14-0163
127. Markoulli M, Flanagan J, Tummanapalli SS, Wu J, Willcox M. The impact of diabetes on corneal nerve morphology and ocular surface integrity. Ocular Surface (2018) 16(1):45–57. doi: 10.1016/j.jtos.2017.10.006
128. Jones MA, Marfurt CF. Peptidergic innervation of the rat cornea. Exp Eye Res (1998) 66(4):421–35. doi: 10.1006/exer.1997.0446
129. Tran MT, Lausch RN, Oakes JE. Substance p differentially stimulates il-8 synthesis in human corneal epithelial cells. Invest Ophthalmol Vis Sci (2000) 41(12):3871–7.
130. Słoniecka M, Le Roux S, Zhou Q, Danielson P. Substance p enhances keratocyte migration and neutrophil recruitment through interleukin-8. Mol Pharmacol (2016) 89(2):215–25. doi: 10.1124/mol.115.101014
131. Watanabe M, Nakayasu K, Iwatsu M, Kanai A. Endogenous substance p in corneal epithelial cells and keratocytes. Jpn J Ophthalmol (2002) 46(6):616–20. doi: 10.1016/s0021-5155(02)00617-2
132. Nakamura M, Ofuji K, Chikama T, Nishida T. The Nk1 receptor and its participation in the synergistic enhancement of corneal epithelial migration by substance p and insulin-like growth factor-1. Br J Pharmacol (1997) 120(4):547–52. doi: 10.1038/sj.bjp.0700923
133. Ofuji K, Nakamura M, Nishida T. Signaling regulation for synergistic effects of substance p and insulin-like growth factor-1 or epidermal growth factor on corneal epithelial migration. Jpn J Ophthalmol (2000) 44(1):1–8. doi: 10.1016/s0021-5155(99)00168-9
134. Nakamura M, Chikama T, Nishida T. Participation of P38 map kinase, but not P44/42 map kinase, in stimulation of corneal epithelial migration by substance p and igf-1. Curr Eye Res (2005) 30(10):825–34. doi: 10.1080/02713680591006129
135. Nakamura M, Nagano T, Chikama T, Nishida T. Up-regulation of phosphorylation of focal adhesion kinase and paxillin by combination of substance p and igf-1 in sv-40 transformed human corneal epithelial cells. Biochem Biophys Res Commun (1998) 242(1):16–20. doi: 10.1006/bbrc.1997.7899
136. Nagano T, Nakamura M, Nakata K, Yamaguchi T, Takase K, Okahara A, et al. Effects of substance p and igf-1 in corneal epithelial barrier function and wound healing in a rat model of neurotrophic keratopathy. Invest Ophthalmol Vis Sci (2003) 44(9):3810–5. doi: 10.1167/iovs.03-0189
137. Nakamura M, Kawahara M, Nakata K, Nishida T. Restoration of corneal epithelial barrier function and wound healing by substance p and igf-1 in rats with capsaicin-induced neurotrophic keratopathy. Invest Ophthalmol Vis Sci (2003) 44(7):2937–40. doi: 10.1167/iovs.02-0868
138. Chikama T, Fukuda K, Morishige N, Nishida T. Treatment of neurotrophic keratopathy with substance-P-Derived peptide (Fglm) and insulin-like growth factor I. Lancet (1998) 351(9118):1783–4. doi: 10.1016/s0140-6736(98)24024-4
139. Nakamura M, Chikama T, Nishida T. Synergistic effect with phe-Gly-Leu-Met-Nh2 of the c-terminal of substance p and insulin-like growth factor-1 on epithelial wound healing of rabbit cornea. Br J Pharmacol (1999) 127(2):489–97. doi: 10.1038/sj.bjp.0702550
140. Yamada N, Yanai R, Kawamoto K, Nagano T, Nakamura M, Inui M, et al. Promotion of corneal epithelial wound healing by a tetrapeptide (Sssr) derived from igf-1. Invest Ophthalmol Vis Sci (2006) 47(8):3286–92. doi: 10.1167/iovs.05-1205
141. Yamada N, Matsuda R, Morishige N, Yanai R, Chikama TI, Nishida T, et al. Open clinical study of eye-drops containing tetrapeptides derived from substance p and insulin-like growth factor-1 for treatment of persistent corneal epithelial defects associated with neurotrophic keratopathy. Br J Ophthalmol (2008) 92(7):896–900. doi: 10.1136/bjo.2007.130013
142. Di G, Qi X, Zhao X, Zhang S, Danielson P, Zhou Q. Corneal epithelium-derived neurotrophic factors promote nerve regeneration. Invest Ophthalmol Vis Sci (2017) 58(11):4695–702. doi: 10.1167/iovs.16-21372
143. Zhou Q, Chen P, Di G, Zhang Y, Wang Y, Qi X, et al. Ciliary neurotrophic factor promotes the activation of corneal epithelial Stem/Progenitor cells and accelerates corneal epithelial wound healing. Stem Cells (2015) 33(5):1566–76. doi: 10.1002/stem.1942
144. Petrova P, Raibekas A, Pevsner J, Vigo N, Anafi M, Moore MK, et al. Manf: A new mesencephalic, astrocyte-derived neurotrophic factor with selectivity for dopaminergic neurons. J Mol Neurosci (2003) 20(2):173–88. doi: 10.1385/jmn:20:2:173
145. Lindholm P, Peränen J, Andressoo JO, Kalkkinen N, Kokaia Z, Lindvall O, et al. Manf is widely expressed in mammalian tissues and differently regulated after ischemic and epileptic insults in rodent brain. Mol Cell Neurosci (2008) 39(3):356–71. doi: 10.1016/j.mcn.2008.07.016
146. Danilova T, Galli E, Pakarinen E, Palm E, Lindholm P, Saarma M, et al. Mesencephalic astrocyte-derived neurotrophic factor (Manf) is highly expressed in mouse tissues with metabolic function. Front Endocrinol (Lausanne) (2019) 10:765. doi: 10.3389/fendo.2019.00765
147. Lindahl M, Danilova T, Palm E, Lindholm P, Võikar V, Hakonen E, et al. Manf is indispensable for the proliferation and survival of pancreatic β cells. Cell Rep (2014) 7(2):366–75. doi: 10.1016/j.celrep.2014.03.023
148. Hakonen E, Chandra V, Fogarty CL, Yu NY, Ustinov J, Katayama S, et al. Manf protects human pancreatic beta cells against stress-induced cell death. Diabetologia (2018) 61(10):2202–14. doi: 10.1007/s00125-018-4687-y
149. Wang X, Li W, Zhou Q, Li J, Wang X, Zhang J, et al. Manf promotes diabetic corneal epithelial wound healing and nerve regeneration by attenuating hyperglycemia-induced endoplasmic reticulum stress. Diabetes (2020) 69(6):1264–78. doi: 10.2337/db19-0835
150. Dun XP, Parkinson DB. Classic axon guidance molecules control correct nerve bridge tissue formation and precise axon regeneration. Neural Regener Res (2020) 15(1):6–9. doi: 10.4103/1673-5374.264441
151. Zhang Y, Chen P, Di G, Qi X, Zhou Q, Gao H. Netrin-1 promotes diabetic corneal wound healing through molecular mechanisms mediated Via the adenosine 2b receptor. Sci Rep (2018) 8(1):5994. doi: 10.1038/s41598-018-24506-9
152. Bettahi I, Sun H, Gao N, Wang F, Mi X, Chen W, et al. Genome-wide transcriptional analysis of differentially expressed genes in diabetic, healing corneal epithelial cells: Hyperglycemia-suppressed Tgfβ3 expression contributes to the delay of epithelial wound healing in diabetic corneas. Diabetes (2014) 63(2):715–27. doi: 10.2337/db13-1260
153. Lee PS, Gao N, Dike M, Shkilnyy O, Me R, Zhang Y, et al. Opposing effects of neuropilin-1 and -2 on sensory nerve regeneration in wounded corneas: Role of Sema3c in ameliorating diabetic neurotrophic keratopathy. Diabetes (2019) 68(4):807–18. doi: 10.2337/db18-1172
154. Di G, Zhao X, Qi X, Zhang S, Feng L, Shi W, et al. Vegf-b promotes recovery of corneal innervations and trophic functions in diabetic mice. Sci Rep (2017) 7:40582. doi: 10.1038/srep40582
155. He J, Pham TL, Kakazu A, Bazan HEP. Recovery of corneal sensitivity and increase in nerve density and wound healing in diabetic mice after pedf plus dha treatment. Diabetes (2017) 66(9):2511–20. doi: 10.2337/db17-0249
156. Katsyuba E, Auwerx J. Modulating nad(+) metabolism, from bench to bedside. EMBO J (2017) 36(18):2670–83. doi: 10.15252/embj.201797135
157. Li Y, Ma X, Li J, Yang L, Zhao X, Qi X, et al. Corneal denervation causes epithelial apoptosis through inhibiting nad+ biosynthesis. Invest Ophthalmol Vis Sci (2019) 60(10):3538–46. doi: 10.1167/iovs.19-26909
158. Li Y, Li J, Zhao C, Yang L, Qi X, Wang X, et al. Hyperglycemia-reduced nad(+) biosynthesis impairs corneal epithelial wound healing in diabetic mice. Metabolism (2021) 114:154402. doi: 10.1016/j.metabol.2020.154402
159. Pu Q, Guo XX, Hu JJ, Li AL, Li GG, Li XY. Nicotinamide mononucleotide increases cell viability and restores tight junctions in high-Glucose-Treated human corneal epithelial cells Via the Sirt1/Nrf2/Ho-1 pathway. BioMed Pharmacother (2022) 147:112659. doi: 10.1016/j.biopha.2022.112659
160. Belmonte C, Acosta MC, Gallar J. Neural basis of sensation in intact and injured corneas. Exp Eye Res (2004) 78(3):513–25. doi: 10.1016/j.exer.2003.09.023
161. Mansoor H, Tan HC, Lin MT, Mehta JS, Liu YC. Diabetic corneal neuropathy. J Clin Med (2020) 9(12):3956. doi: 10.3390/jcm9123956
162. Alamri A, Bron R, Brock JA, Ivanusic JJ. Transient receptor potential cation channel subfamily V member 1 expressing corneal sensory neurons can be subdivided into at least three subpopulations. Front Neuroanat (2015) 9:71. doi: 10.3389/fnana.2015.00071
163. Asiedu K. Role of ocular surface neurobiology in neuronal-mediated inflammation in dry eye disease. Neuropeptides (2022) 95:102266. doi: 10.1016/j.npep.2022.102266
164. Kamei J, Zushida K, Morita K, Sasaki M, Tanaka S. Role of vanilloid Vr1 receptor in thermal allodynia and hyperalgesia in diabetic mice. Eur J Pharmacol (2001) 422(1-3):83–6. doi: 10.1016/s0014-2999(01)01059-7
165. Backonja M, Wallace MS, Blonsky ER, Cutler BJ, Malan P Jr., Rauck R, et al. Ngx-4010, a high-concentration capsaicin patch, for the treatment of postherpetic neuralgia: A randomised, double-blind study. Lancet Neurol (2008) 7(12):1106–12. doi: 10.1016/s1474-4422(08)70228-x
166. Irving GA, Backonja MM, Dunteman E, Blonsky ER, Vanhove GF, Lu SP, et al. A multicenter, randomized, double-blind, controlled study of ngx-4010, a high-concentration capsaicin patch, for the treatment of postherpetic neuralgia. Pain Med (2011) 12(1):99–109. doi: 10.1111/j.1526-4637.2010.01004.x
167. Simpson DM, Brown S, Tobias J. Controlled trial of high-concentration capsaicin patch for treatment of painful hiv neuropathy. Neurology (2008) 70(24):2305–13. doi: 10.1212/01.wnl.0000314647.35825.9c
168. Haanpää M, Cruccu G, Nurmikko TJ, McBride WT, Docu Axelarad A, Bosilkov A, et al. Capsaicin 8% patch versus oral pregabalin in patients with peripheral neuropathic pain. Eur J Pain (2016) 20(2):316–28. doi: 10.1002/ejp.731
169. Simpson DM, Robinson-Papp J, Van J, Stoker M, Jacobs H, Snijder RJ, et al. Capsaicin 8% patch in painful diabetic peripheral neuropathy: A randomized, double-blind, placebo-controlled study. J Pain (2017) 18(1):42–53. doi: 10.1016/j.jpain.2016.09.008
170. Okada Y, Reinach PS, Shirai K, Kitano-Izutani A, Miyajima M, Yamanaka O, et al. Transient receptor potential channels and corneal stromal inflammation. Cornea (2015) 34 Suppl 11:S136–41. doi: 10.1097/ico.0000000000000602
171. Acosta CM, Luna C, Quirce S, Belmonte C, Gallar J. Changes in sensory activity of ocular surface sensory nerves during allergic keratoconjunctivitis. Pain (2013) 154(11):2353–62. doi: 10.1016/j.pain.2013.07.012
172. Liu J, Huang S, Yu R, Chen X, Li F, Sun X, et al. Trpv1(+) sensory nerves modulate corneal inflammation after epithelial abrasion Via Ramp1 and Sstr5 signaling. Mucosal Immunol (2022) 15(5):867–81. doi: 10.1038/s41385-022-00533-8
173. Zhang B, Yang Y, Yi J, Zhao Z, Ye R. Ablation of transient receptor potential vanilloid subtype 1-expressing neurons in rat trigeminal ganglia aggravated bone resorption in periodontitis with diabetes. Arch Oral Biol (2022) 133:105293. doi: 10.1016/j.archoralbio.2021.105293
174. Andersson DA, Chase HW, Bevan S. Trpm8 activation by menthol, icilin, and cold is differentially modulated by intracellular ph. J Neurosci (2004) 24(23):5364–9. doi: 10.1523/jneurosci.0890-04.2004
175. Liu B, Qin F. Functional control of cold- and menthol-sensitive Trpm8 ion channels by phosphatidylinositol 4,5-bisphosphate. J Neurosci (2005) 25(7):1674–81. doi: 10.1523/jneurosci.3632-04.2005
176. Premkumar LS, Raisinghani M, Pingle SC, Long C, Pimentel F. Downregulation of transient receptor potential melastatin 8 by protein kinase c-mediated dephosphorylation. J Neurosci (2005) 25(49):11322–9. doi: 10.1523/jneurosci.3006-05.2005
177. Li F, Yang W, Jiang H, Guo C, Huang AJW, Hu H, et al. Trpv1 activity and substance p release are required for corneal cold nociception. Nat Commun (2019) 10(1):5678. doi: 10.1038/s41467-019-13536-0
178. Situ P, Begley CG, Simpson TL. Effects of tear film instability on sensory responses to corneal cold, mechanical, and chemical stimuli. Invest Ophthalmol Vis Sci (2019) 60(8):2935–41. doi: 10.1167/iovs.19-27298
179. Pabbidi MR, Premkumar LS. Role of transient receptor potential channels Trpv1 and Trpm8 in diabetic peripheral neuropathy. J Diabetes Treat (2017) 2017(4):029.
180. Alamri AS, Brock JA, Herath CB, Rajapaksha IG, Angus PW, Ivanusic JJ. The effects of diabetes and high-fat diet on polymodal nociceptor and cold thermoreceptor nerve terminal endings in the corneal epithelium. Invest Ophthalmol Vis Sci (2019) 60(1):209–17. doi: 10.1167/iovs.18-25788
181. Greene DA, Lattimer SA. Impaired rat sciatic nerve sodium-potassium adenosine triphosphatase in acute streptozocin diabetes and its correction by dietary myo-inositol supplementation. J Clin Invest (1983) 72(3):1058–63. doi: 10.1172/jci111030
182. Greene DA, Lattimer SA, Sima AA. Are disturbances of sorbitol, phosphoinositide, and na+-K+-Atpase regulation involved in pathogenesis of diabetic neuropathy? Diabetes (1988) 37(6):688–93. doi: 10.2337/diab.37.6.688
183. Veves A, King GL. Can vegf reverse diabetic neuropathy in human subjects? J Clin Invest (2001) 107(10):1215–8. doi: 10.1172/jci13038
184. Hoeldtke RD, Bryner KD, McNeill DR, Hobbs GR, Riggs JE, Warehime SS, et al. Nitrosative stress, uric acid, and peripheral nerve function in early type 1 diabetes. Diabetes (2002) 51(9):2817–25. doi: 10.2337/diabetes.51.9.2817
185. Vinik AI, Erbas T, Park TS, Stansberry KB, Scanelli JA, Pittenger GL. Dermal neurovascular dysfunction in type 2 diabetes. Diabetes Care (2001) 24(8):1468–75. doi: 10.2337/diacare.24.8.1468
186. Brownlee M. Glycation products and the pathogenesis of diabetic complications. Diabetes Care (1992) 15(12):1835–43. doi: 10.2337/diacare.15.12.1835
187. Vinik AI. Diagnosis and management of diabetic neuropathy. Clin Geriatr Med (1999) 15(2):293–320. doi: 10.1016/S0749-0690(18)30061-2
188. Cameron NE, Cotter MA. Metabolic and vascular factors in the pathogenesis of diabetic neuropathy. Diabetes (1997) 46 Suppl 2:S31–7. doi: 10.2337/diab.46.2.s31
189. Vinik AI, Erbas T. Recognizing and treating diabetic autonomic neuropathy. Cleve Clin J Med (2001) 68(11):928–30. doi: 10.3949/ccjm.68.11.928
190. Vinik AI, Maser RE, Mitchell BD, Freeman R. Diabetic autonomic neuropathy. Diabetes Care (2003) 26(5):1553–79. doi: 10.2337/diacare.26.5.1553
191. Marfurt CF, Ellis LC. Immunohistochemical localization of tyrosine hydroxylase in corneal nerves. J Comp Neurol (1993) 336(4):517–31. doi: 10.1002/cne.903360405
192. Marfurt CF, Kingsley RE, Echtenkamp SE. Sensory and sympathetic innervation of the mammalian cornea. a retrograde tracing study. Invest Ophthalmol Vis Sci (1989) 30(3):461–72.
193. Vasamsetti SB, Florentin J, Coppin E, Stiekema LCA, Zheng KH, Nisar MU, et al. Sympathetic neuronal activation triggers myeloid progenitor proliferation and differentiation. Immunity (2018) 49(1):93–106.e7. doi: 10.1016/j.immuni.2018.05.004
194. Esler M, Rumantir M, Wiesner G, Kaye D, Hastings J, Lambert G. Sympathetic nervous system and insulin resistance: From obesity to diabetes. Am J Hypertens (2001) 14(11 Pt 2):304s–9s. doi: 10.1016/s0895-7061(01)02236-1
195. Seals DR, Bell C. Chronic sympathetic activation: Consequence and cause of age-associated obesity? Diabetes (2004) 53(2):276–84. doi: 10.2337/diabetes.53.2.276
196. Thorp AA, Schlaich MP. Relevance of sympathetic nervous system activation in obesity and metabolic syndrome. J Diabetes Res (2015) 2015:341583. doi: 10.1155/2015/341583
197. Marfurt CF, Jones MA, Thrasher K. Parasympathetic innervation of the rat cornea. Exp Eye Res (1998) 66(4):437–48. doi: 10.1006/exer.1997.0445
198. Zhang Y, Jiang H, Dou S, Zhang B, Qi X, Li J, et al. Comprehensive analysis of differentially expressed micrornas and mrnas involved in diabetic corneal neuropathy. Life Sci (2020) 261:118456. doi: 10.1016/j.lfs.2020.118456
199. Hu J, Huang Y, Lin Y, Lin J. Protective effect inhibiting the expression of mir-181a on the diabetic corneal nerve in a mouse model. Exp Eye Res (2020) 192:107925. doi: 10.1016/j.exer.2020.107925
200. Hu J, Hu X, Kan T. Mir-34c participates in diabetic corneal neuropathy Via regulation of autophagy. Invest Ophthalmol Vis Sci (2019) 60(1):16–25. doi: 10.1167/iovs.18-24968
201. Wang Y, Zhao X, Wu X, Dai Y, Chen P, Xie L. Microrna-182 mediates Sirt1-induced diabetic corneal nerve regeneration. Diabetes (2016) 65(7):2020–31. doi: 10.2337/db15-1283
202. Wang Y, Zhao X, Shi D, Chen P, Yu Y, Yang L, et al. Overexpression of Sirt1 promotes high glucose-attenuated corneal epithelial wound healing Via P53 regulation of the Igfbp3/Igf-1r/Akt pathway. Invest Ophthalmol Vis Sci (2013) 54(5):3806–14. doi: 10.1167/iovs.13-12091
203. Zhang Y, Dou S, Qi X, Zhang Z, Qiao Y, Wang Y, et al. Transcriptional network analysis reveals the role of mir-223-5p during diabetic corneal epithelial regeneration. Front Mol Biosci (2021) 8:737472. doi: 10.3389/fmolb.2021.737472
204. Gao J, Wang Y, Zhao X, Chen P, Xie L. Microrna-204-5p-Mediated regulation of Sirt1 contributes to the delay of epithelial cell cycle traversal in diabetic corneas. Invest Ophthalmol Vis Sci (2015) 56(3):1493–504. doi: 10.1167/iovs.14-15913
205. Bi X, Zhou L, Liu Y, Gu J, Mi QS. Microrna-146a deficiency delays wound healing in normal and diabetic mice. Adv Wound Care (New Rochelle) (2022) 11(1):19–27. doi: 10.1089/wound.2020.1165
206. Poe AJ, Shah R, Khare D, Kulkarni M, Phan H, Ghiam S, et al. Regulatory role of mir-146a in corneal epithelial wound healing Via its inflammatory targets in human diabetic cornea. Ocular Surface (2022) 25:92–100. doi: 10.1016/j.jtos.2022.06.001
207. Chen X, Hu J. Long noncoding rna 3632454l22rik contributes to corneal epithelial wound healing by sponging mir-181a-5p in diabetic mice. Invest Ophthalmol Vis Sci (2021) 62(14):16. doi: 10.1167/iovs.62.14.16
208. Tseng SC. Concept and application of limbal stem cells. Eye (Lond) (1989) 3(Pt 2):141–57. doi: 10.1038/eye.1989.22
209. Joe AW, Yeung SN. Concise review: Identifying limbal stem cells: Classical concepts and new challenges. Stem Cells Transl Med (2014) 3(3):318–22. doi: 10.5966/sctm.2013-0137
210. Di Girolamo N. Moving epithelia: Tracking the fate of mammalian limbal epithelial stem cells. Prog Retin Eye Res (2015) 48:203–25. doi: 10.1016/j.preteyeres.2015.04.002
211. Amitai-Lange A, Altshuler A, Bubley J, Dbayat N, Tiosano B, Shalom-Feuerstein R. Lineage tracing of stem and progenitor cells of the murine corneal epithelium. Stem Cells (2015) 33(1):230–9. doi: 10.1002/stem.1840
212. Saghizadeh M, Soleymani S, Harounian A, Bhakta B, Troyanovsky SM, Brunken WJ, et al. Alterations of epithelial stem cell marker patterns in human diabetic corneas and effects of c-met gene therapy. Mol Vis (2011) 17:2177–90.
213. Kramerov AA, Saghizadeh M, Maguen E, Rabinowitz YS, Ljubimov AV. Persistence of reduced expression of putative stem cell markers and slow wound healing in cultured diabetic limbal epithelial cells. Mol Vis (2015) 21:1357–67.
214. Ueno H, Hattori T, Kumagai Y, Suzuki N, Ueno S, Takagi H. Alterations in the corneal nerve and Stem/Progenitor cells in diabetes: Preventive effects of insulin-like growth factor-1 treatment. Int J Endocrinol (2014) 2014:312401. doi: 10.1155/2014/312401
215. Chen J, Chen P, Backman LJ, Zhou Q, Danielson P. Ciliary neurotrophic factor promotes the migration of corneal epithelial Stem/Progenitor cells by up-regulation of mmps through the phosphorylation of akt. Sci Rep (2016) 6:25870. doi: 10.1038/srep25870
216. Chen J, Lan J, Liu D, Backman LJ, Zhang W, Zhou Q, et al. Ascorbic acid promotes the stemness of corneal epithelial Stem/Progenitor cells and accelerates epithelial wound healing in the cornea. Stem Cells Transl Med (2017) 6(5):1356–65. doi: 10.1002/sctm.16-0441
217. Zhou Q, Duan H, Wang Y, Qu M, Yang L, Xie L. Rock inhibitor y-27632 increases the cloning efficiency of limbal Stem/Progenitor cells by improving their adherence and ros-scavenging capacity. Tissue Eng Part C Methods (2013) 19(7):531–7. doi: 10.1089/ten.TEC.2012.0429
218. Duan H, Wang Y, Yang L, Qu M, Wang Q, Shi W, et al. Pluripotin enhances the expansion of rabbit limbal epithelial Stem/Progenitor cells in vitro. Exp Eye Res (2012) 100:52–8. doi: 10.1016/j.exer.2012.04.012
219. Wang X, Zhang S, Dong M, Li Y, Zhou Q, Yang L. The proinflammatory cytokines il-1β and tnf-α modulate corneal epithelial wound healing through P16(Ink4a) suppressing Stat3 activity. J Cell Physiol (2020) 235(12):10081–93. doi: 10.1002/jcp.29823
220. Yang L, Zhang S, Duan H, Dong M, Hu X, Zhang Z, et al. Different effects of pro-inflammatory factors and hyperosmotic stress on corneal epithelial Stem/Progenitor cells and wound healing in mice. Stem Cells Transl Med (2019) 8(1):46–57. doi: 10.1002/sctm.18-0005
221. Okada Y, Sumioka T, Ichikawa K, Sano H, Nambu A, Kobayashi K, et al. Sensory nerve supports epithelial stem cell function in healing of corneal epithelium in mice: The role of trigeminal nerve transient receptor potential vanilloid 4. Lab Invest (2019) 99(2):210–30. doi: 10.1038/s41374-018-0118-4
222. González-Méijome JM, Jorge J, Queirós A, Peixoto-de-Matos SC, Parafita MA. Two single descriptors of endothelial polymegethism and pleomorphism. Graefes Arch Clin Exp Ophthalmol (2010) 248(8):1159–66. doi: 10.1007/s00417-010-1337-6
223. Larsson LI, Bourne WM, Pach JM, Brubaker RF. Structure and function of the corneal endothelium in diabetes mellitus type I and type ii. Arch Ophthalmol (1996) 114(1):9–14. doi: 10.1001/archopht.1996.01100130007001
224. Storr-Paulsen A, Singh A, Jeppesen H, Norregaard JC, Thulesen J. Corneal endothelial morphology and central thickness in patients with type ii diabetes mellitus. Acta Ophthalmol (2014) 92(2):158–60. doi: 10.1111/aos.12064
225. Sudhir RR, Raman R, Sharma T. Changes in the corneal endothelial cell density and morphology in patients with type 2 diabetes mellitus: A population-based study, Sankara nethralaya diabetic retinopathy and molecular genetics study (Sn-dreams, report 23). Cornea (2012) 31(10):1119–22. doi: 10.1097/ICO.0b013e31823f8e00
226. Itoi M, Nakamura T, Mizobe K, Kodama Y, Nakagawa N, Itoi M. Specular microscopic studies of the corneal endothelia of Japanese diabetics. Cornea (1989) 8(1):2–6. doi: 10.1097/00003226-198903000-00002
227. Urban B, Peczyńska J, Głowińska-Olszewska B, Urban M, Bakunowicz-Lazarczyk A, Kretowska M. Evaluation of central corneal thickness in children and adolescents with type I diabetes mellitus. Klin Oczna (2007) 109(10-12):418–20. doi: 10.1155/2013/913754
228. Leelawongtawun W, Surakiatchanukul B, Kampitak K, Leelawongtawun R. Study of corneal endothelial cells related to duration in diabetes. J Med Assoc Thai (2016) 99 Suppl 4:S182–8.
229. Meyer LA, Ubels JL, Edelhauser HF. Corneal endothelial morphology in the rat. effects of aging, diabetes, and topical aldose reductase inhibitor treatment. Invest Ophthalmol Vis Sci (1988) 29(6):940–8.
230. Roszkowska AM, Tringali CG, Colosi P, Squeri CA, Ferreri G. Corneal endothelium evaluation in type I and type ii diabetes mellitus. Ophthalmologica (1999) 213(4):258–61. doi: 10.1159/000027431
231. Inoue K, Tokuda Y, Inoue Y, Amano S, Oshika T, Inoue J. Corneal endothelial cell morphology in patients undergoing cataract surgery. Cornea (2002) 21(4):360–3. doi: 10.1097/00003226-200205000-00006
232. Islam QU, Mehboob MA, Amin ZA. Comparison of corneal morphological characteristics between diabetic and non diabetic population. Pak J Med Sci (2017) 33(6):1307–11. doi: 10.12669/pjms.336.13628
233. Goldstein AS, Janson BJ, Skeie JM, Ling JJ, Greiner MA. The effects of diabetes mellitus on the corneal endothelium: A review. Surv Ophthalmol (2020) 65(4):438–50. doi: 10.1016/j.survophthal.2019.12.009
234. Módis L Jr., Szalai E, Kertész K, Kemény-Beke A, Kettesy B, Berta A. Evaluation of the corneal endothelium in patients with diabetes mellitus type I and ii. Histol Histopathol (2010) 25(12):1531–7. doi: 10.14670/hh-25.1531
235. Tuft SJ, Coster DJ. The corneal endothelium. Eye (Lond) (1990) 4(Pt 3):389–424. doi: 10.1038/eye.1990.53
236. Aldrich BT, Schlötzer-Schrehardt U, Skeie JM, Burckart KA, Schmidt GA, Reed CR, et al. Mitochondrial and morphologic alterations in native human corneal endothelial cells associated with diabetes mellitus. Invest Ophthalmol Vis Sci (2017) 58(4):2130–8. doi: 10.1167/iovs.16-21094
237. Anbar M, Ammar H, Mahmoud RA. Corneal endothelial morphology in children with type 1 diabetes. J Diabetes Res (2016) 2016:7319047. doi: 10.1155/2016/7319047
238. El-Agamy A, Alsubaie S. Corneal endothelium and central corneal thickness changes in type 2 diabetes mellitus. Clin Ophthalmol (2017) 11:481–6. doi: 10.2147/opth.S126217
239. Gao F, Lin T, Pan Y. Effects of diabetic keratopathy on corneal optical density, central corneal thickness, and corneal endothelial cell counts. Exp Ther Med (2016) 12(3):1705–10. doi: 10.3892/etm.2016.3511
240. Calvo-Maroto AM, Cerviño A, Perez-Cambrodí RJ, García-Lázaro S, Sanchis-Gimeno JA. Quantitative corneal anatomy: Evaluation of the effect of diabetes duration on the endothelial cell density and corneal thickness. Ophthalmic Physiol Opt (2015) 35(3):293–8. doi: 10.1111/opo.12191
241. Chocron IM, Rai DK, Kwon JW, Bernstein N, Hu J, Heo M, et al. Effect of diabetes mellitus and metformin on central corneal endothelial cell density in eye bank eyes. Cornea (2018) 37(8):964–6. doi: 10.1097/ico.0000000000001623
242. Choo M, Prakash K, Samsudin A, Soong T, Ramli N, Kadir A. Corneal changes in type ii diabetes mellitus in Malaysia. Int J Ophthalmol (2010) 3(3):234–6. doi: 10.3980/j.issn.2222-3959.2010.03.12
243. Liaboe CA, Aldrich BT, Carter PC, Skeie JM, Burckart KA, Schmidt GA, et al. Assessing the impact of diabetes mellitus on donor corneal endothelial cell density. Cornea (2017) 36(5):561–6. doi: 10.1097/ico.0000000000001174
244. Urban B, Raczyńska D, Bakunowicz-Łazarczyk A, Raczyńska K, Krętowska M. Evaluation of corneal endothelium in children and adolescents with type 1 diabetes mellitus. Mediators Inflammation (2013) 2013:913754. doi: 10.1155/2013/913754
245. Busted N, Olsen T, Schmitz O. Clinical observations on the corneal thickness and the corneal endothelium in diabetes mellitus. Br J Ophthalmol (1981) 65(10):687–90. doi: 10.1136/bjo.65.10.687
246. Lee JS, Oum BS, Choi HY, Lee JE, Cho BM. Differences in corneal thickness and corneal endothelium related to duration in diabetes. Eye (Lond) (2006) 20(3):315–8. doi: 10.1038/sj.eye.6701868
247. Saini JS, Mittal S. In vivo assessment of corneal endothelial function in diabetes mellitus. Arch Ophthalmol (1996) 114(6):649–53. doi: 10.1001/archopht.1996.01100130641001
248. Lemasters JJ. Selective mitochondrial autophagy, or mitophagy, as a targeted defense against oxidative stress, mitochondrial dysfunction, and aging. Rejuvenation Res (2005) 8(1):3–5. doi: 10.1089/rej.2005.8.3
249. Chen C, Zhou Q, Li Z, et al. Hyperglycemia induces corneal endothelial dysfunction through attenuating mitophagy . Exp Eye Res (2022) 215:108903. doi: 10.1016/j.exer.2021.108903
250. Livezey M, Huang R, Hergenrother PJ, Shapiro DJ. Strong and sustained activation of the anticipatory unfolded protein response induces necrotic cell death. Cell Death Differ (2018) 25(10):1796–807. doi: 10.1038/s41418-018-0143-2
251. Xiong Z, Yuan C, Shi J, Xiong W, Huang Y, Xiao W, et al. Restoring the epigenetically silenced Pck2 suppresses renal cell carcinoma progression and increases sensitivity to sunitinib by promoting endoplasmic reticulum stress. Theranostics (2020) 10(25):11444–61. doi: 10.7150/thno.48469
252. Lim H, Lim YM, Kim KH, Jeon YE, Park K, Kim J, et al. A novel autophagy enhancer as a therapeutic agent against metabolic syndrome and diabetes. Nat Commun (2018) 9(1):1438. doi: 10.1038/s41467-018-03939-w
253. Chen C, Zhang B, Xue J, Li Z, Dou S, Chen H, et al. Pathogenic role of endoplasmic reticulum stress in diabetic corneal endothelial dysfunction. Invest Ophthalmol Vis Sci (2022) 63(3):4. doi: 10.1167/iovs.63.3.4
254. Lamkanfi M, Dixit VM. Mechanisms and functions of inflammasomes. Cell (2014) 157(5):1013–22. doi: 10.1016/j.cell.2014.04.007
255. You ZP, Zhang YL, Li BY, Zhu XG, Shi K. Bioinformatics analysis of weighted genes in diabetic retinopathy. Invest Ophthalmol Vis Sci (2018) 59(13):5558–63. doi: 10.1167/iovs.18-25515
256. Bergsbaken T, Fink SL, Cookson BT. Pyroptosis: Host cell death and inflammation. Nat Rev Microbiol (2009) 7(2):99–109. doi: 10.1038/nrmicro2070
257. Zhang Y, Song Z, Li X, Xu S, Zhou S, Jin X, et al. Long noncoding rna Kcnq1ot1 induces pyroptosis in diabetic corneal endothelial keratopathy. Am J Physiol Cell Physiol (2020) 318(2):C346–c59. doi: 10.1152/ajpcell.00053.2019
258. De Freitas GR, Ferraz GAM, Gehlen M, Skare TL. Dry eyes in patients with diabetes mellitus. Prim Care Diabetes (2021) 15(1):184–6. doi: 10.1016/j.pcd.2020.01.011
259. Uchino M, Schaumberg DA. Dry eye disease: Impact on quality of life and vision. Curr Ophthalmol Rep (2013) 1(2):51–7. doi: 10.1007/s40135-013-0009-1
260. Su YC, Hung JH, Chang KC, Sun CC, Huang YH, Lee CN, et al. Comparison of sodium-glucose cotransporter 2 inhibitors vs glucagonlike peptide-1 receptor agonists and incidence of dry eye disease in patients with type 2 diabetes in Taiwan. JAMA Netw Open (2022) 5(9):e2232584. doi: 10.1001/jamanetworkopen.2022.32584
261. Hann LE, Kelleher RS, Sullivan DA. Influence of culture conditions on the androgen control of secretory component production by acinar cells from the rat lacrimal gland. Invest Ophthalmol Vis Sci (1991) 32(9):2610–21.
262. Dias AC, Batista TM, Roma LP, Módulo CM, Malki LT, Dias LC, et al. Insulin replacement restores the vesicular secretory apparatus in the diabetic rat lacrimal gland. Arq Bras Oftalmol (2015) 78(3):158–63. doi: 10.5935/0004-2749.20150041
263. Wu K, Joffre C, Li X, MacVeigh-Aloni M, Hom M, Hwang J, et al. Altered expression of genes functioning in lipid homeostasis is associated with lipid deposition in nod mouse lacrimal gland. Exp Eye Res (2009) 89(3):319–32. doi: 10.1016/j.exer.2009.03.020
264. Ramos-Remus C, Suarez-Almazor M, Russell AS. Low tear production in patients with diabetes mellitus is not due to sjögren’s syndrome. Clin Exp Rheumatol (1994) 12(4):375–80.
265. He X, Zhao Z, Wang S, Kang J, Zhang M, Bu J, et al. High-fat diet-induced functional and pathologic changes in lacrimal gland. Am J Pathol (2020) 190(12):2387–402. doi: 10.1016/j.ajpath.2020.09.002
266. Nakata M, Okada Y, Kobata H, Shigematsu T, Reinach PS, Tomoyose K, et al. Diabetes mellitus suppresses hemodialysis-induced increases in tear fluid secretion. BMC Res Notes (2014) 7:78. doi: 10.1186/1756-0500-7-78
267. Qu M, Wan L, Dong M, Wang Y, Xie L, Zhou Q. Hyperglycemia-induced severe mitochondrial bioenergetic deficit of lacrimal gland contributes to the early onset of dry eye in diabetic mice. Free Radic Biol Med (2021) 166:313–23. doi: 10.1016/j.freeradbiomed.2021.02.036
268. Alves M, Calegari VC, Cunha DA, Saad MJ, Velloso LA, Rocha EM. Increased expression of advanced glycation end-products and their receptor, and activation of nuclear factor kappa-b in lacrimal glands of diabetic rats. Diabetologia (2005) 48(12):2675–81. doi: 10.1007/s00125-005-0010-9
269. Kubota M, Shui YB, Liu M, Bai F, Huang AJ, Ma N, et al. Mitochondrial oxygen metabolism in primary human lens epithelial cells: Association with age, diabetes and glaucoma. Free Radic Biol Med (2016) 97:513–9. doi: 10.1016/j.freeradbiomed.2016.07.016
270. Czajka A, Malik AN. Hyperglycemia induced damage to mitochondrial respiration in renal mesangial and tubular cells: Implications for diabetic nephropathy. Redox Biol (2016) 10:100–7. doi: 10.1016/j.redox.2016.09.007
271. Shao Y, Chen J, Dong LJ, He X, Cheng R, Zhou K, et al. A protective effect of pparα in endothelial progenitor cells through regulating metabolism. Diabetes (2019) 68(11):2131–42. doi: 10.2337/db18-1278
272. Seo Y, Ji YW, Lee SM, Shim J, Noh H, Yeo A, et al. Activation of hif-1α (Hypoxia inducible factor-1α) prevents dry eye-induced acinar cell death in the lacrimal gland. Cell Death Dis (2014) 5(6):e1309. doi: 10.1038/cddis.2014.260
273. Uchino Y, Kawakita T, Ishii T, Ishii N, Tsubota K. A new mouse model of dry eye disease: Oxidative stress affects functional decline in the lacrimal gland. Cornea (2012) 31 Suppl 1:S63–7. doi: 10.1097/ICO.0b013e31826a5de1
274. Stevenson W, Chauhan SK, Dana R. Dry eye disease: An immune-mediated ocular surface disorder. Arch Ophthalmol (2012) 130(1):90–100. doi: 10.1001/archophthalmol.2011.364
275. Dartt DA. Neural regulation of lacrimal gland secretory processes: Relevance in dry eye diseases. Prog Retin Eye Res (2009) 28(3):155–77. doi: 10.1016/j.preteyeres.2009.04.003
276. Ruskell GL. Changes in nerve terminals and acini of the lacrimal gland and changes in secretion induced by autonomic denervation. Z Zellforsch Mikrosk Anat (1969) 94(2):261–81. doi: 10.1007/bf00339361
277. Ruskell GL. The distribution of autonomic post-ganglionic nerve fibres to the lacrimal gland in monkeys. J Anat (1971) 109(Pt 2):229–42.
278. Dartt DA, Baker AK, Vaillant C, Rose PE. Vasoactive intestinal polypeptide stimulation of protein secretion from rat lacrimal gland acini. Am J Physiol (1984) 247(5 Pt 1):G502–9. doi: 10.1152/ajpgi.1984.247.5.G502
279. Dartt DA. Dysfunctional neural regulation of lacrimal gland secretion and its role in the pathogenesis of dry eye syndromes. Ocular Surface (2004) 2(2):76–91. doi: 10.1016/s1542-0124(12)70146-5
280. Nakamura S, Imada T, Jin K, Shibuya M, Sakaguchi H, Izumiseki F, et al. The oxytocin system regulates tearing. (2022). doi: 10.1101/2022.03.08.483433
281. Jin K, Imada T, Hisamura R, Ito M, Toriumi H, Tanaka KF, et al. Identification of lacrimal gland postganglionic innervation and its regulation of tear secretion. Am J Pathol (2020) 190(5):1068–79. doi: 10.1016/j.ajpath.2020.01.007
282. Dias-Teixeira K. Lacrimal gland postganglionic innervation: Unveiling the role of parasympathetic and sympathetic nerves in stimulating tear secretion. Am J Pathol (2020) 190(5):968–9. doi: 10.1016/j.ajpath.2020.03.001
283. Nguyen DH, Toshida H, Schurr J, Beuerman RW. Microarray analysis of the rat lacrimal gland following the loss of parasympathetic control of secretion. Physiol Genomics (2004) 18(1):108–18. doi: 10.1152/physiolgenomics.00011.2004
284. Ding C, Walcott B, Keyser KT. Sympathetic neural control of the mouse lacrimal gland. Invest Ophthalmol Vis Sci (2003) 44(4):1513–20. doi: 10.1167/iovs.02-0406
285. Meneray MA, Bennett DJ, Nguyen DH, Beuerman RW. Effect of sensory denervation on the structure and physiologic responsiveness of rabbit lacrimal gland. Cornea (1998) 17(1):99–107. doi: 10.1097/00003226-199801000-00015
286. Tangkrisanavinont V. Stimulation of lacrimal secretion by sympathetic nerve impulses in the rabbit. Life Sci (1984) 34(24):2365–71. doi: 10.1016/0024-3205(84)90423-5
287. Yu T, Shi WY, Song AP, Gao Y, Dang GF, Ding G. Changes of meibomian glands in patients with type 2 diabetes mellitus. Int J Ophthalmol (2016) 9(12):1740–4. doi: 10.18240/ijo.2016.12.06
288. Shamsheer RP, Arunachalam C. A clinical study of meibomian gland dysfunction in patients with diabetes. Middle East Afr J Ophthalmol (2015) 22(4):462–6. doi: 10.4103/0974-9233.167827
289. Lin X, Xu B, Zheng Y, Coursey TG, Zhao Y, Li J, et al. Meibomian gland dysfunction in type 2 diabetic patients. J Ophthalmol (2017) 2017:3047867. doi: 10.1155/2017/3047867
290. Wu H, Fang X, Luo S, Shang X, Xie Z, Dong N, et al. Meibomian glands and tear film findings in type 2 diabetic patients: A cross-sectional study. Front Med (Lausanne) (2022) 9:762493. doi: 10.3389/fmed.2022.762493
291. Sandra Johanna GP, Antonio LA, Andrés GS. Correlation between type 2 diabetes, dry eye and meibomian glands dysfunction. J Optom (2019) 12(4):256–62. doi: 10.1016/j.optom.2019.02.003
292. Viso E, Rodríguez-Ares MT, Abelenda D, Oubiña B, Gude F. Prevalence of asymptomatic and symptomatic meibomian gland dysfunction in the general population of Spain. Invest Ophthalmol Vis Sci (2012) 53(6):2601–6. doi: 10.1167/iovs.11-9228
293. Inanc M, Kiziltoprak H, Hekimoglu R, Tekin K, Ozalkak S, Koc M, et al. Alterations of tear film and ocular surface in children with type 1 diabetes mellitus. Ocul Immunol Inflammation (2020) 28(3):362–9. doi: 10.1080/09273948.2019.1571212
294. Akinci A, Cetinkaya E, Aycan Z. Dry eye syndrome in diabetic children. Eur J Ophthalmol (2007) 17(6):873–8. doi: 10.1177/112067210701700601
295. Koca S, Koca SB, İnan S. Ocular surface alterations and changes of meibomian glands with meibography in type 1 diabetic children. Int Ophthalmol (2022) 42(5):1613–21. doi: 10.1007/s10792-021-02155-8
296. Guo Y, Zhang H, Zhao Z, Luo X, Zhang M, Bu J, et al. Hyperglycemia induces meibomian gland dysfunction. Invest Ophthalmol Vis Sci (2022) 63(1):30. doi: 10.1167/iovs.63.1.30
297. Wang H, Zhou Q, Wan L, Guo M, Chen C, Xue J, et al. Lipidomic analysis of meibomian glands from type-1 diabetes mouse model and preliminary studies of potential mechanism. Exp Eye Res (2021) 210:108710. doi: 10.1016/j.exer.2021.108710
298. Jester JV, Potma E, Brown DJ. Pparγ regulates mouse meibocyte differentiation and lipid synthesis. Ocular Surface (2016) 14(4):484–94. doi: 10.1016/j.jtos.2016.08.001
299. Kim SW, Xie Y, Nguyen PQ, Bui VT, Huynh K, Kang JS, et al. Pparγ regulates meibocyte differentiation and lipid synthesis of cultured human meibomian gland epithelial cells (Hmgec). Ocular Surface (2018) 16(4):463–9. doi: 10.1016/j.jtos.2018.07.004
300. Zou Z, Wang H, Zhang B, Zhang Z, Chen R, Yang L. Inhibition of Gli1 suppressed hyperglycemia-induced meibomian gland dysfunction by promoting pparγ expression. BioMed Pharmacother (2022) 151:113109. doi: 10.1016/j.biopha.2022.113109
301. Kam WR, Sullivan DA. Neurotransmitter influence on human meibomian gland epithelial cells. Invest Ophthalmol Vis Sci (2011) 52(12):8543–8. doi: 10.1167/iovs.11-8113
302. Chung CW, Tigges M, Stone RA. Peptidergic innervation of the primate meibomian gland. Invest Ophthalmol Vis Sci (1996) 37(1):238–45.
303. Bründl M, Garreis F, Schicht M, Dietrich J, Paulsen F. Characterization of the innervation of the meibomian glands in humans, rats and mice. Ann Anat (2021) 233:151609. doi: 10.1016/j.aanat.2020.151609
304. Tavakoli M, Petropoulos IN, Malik RA. Corneal confocal microscopy to assess diabetic neuropathy: An eye on the foot. J Diabetes Sci Technol (2013) 7(5):1179–89. doi: 10.1177/193229681300700509
305. Yu T, Han XG, Gao Y, Song AP, Dang GF. Morphological and cytological changes of meibomian glands in patients with type 2 diabetes mellitus. Int J Ophthalmol (2019) 12(9):1415–9. doi: 10.18240/ijo.2019.09.07
306. Yıldız E, Zibandeh N, Özer B, Şahin A. Effects of type 2 diabetes mellitus on gene expressions of mouse meibomian glands. Curr Eye Res (2020) 45(1):72–80. doi: 10.1080/02713683.2019.1656750
307. Yan J, Horng T. Lipid metabolism in regulation of macrophage functions. Trends Cell Biol (2020) 30(12):979–89. doi: 10.1016/j.tcb.2020.09.006
308. Glass CK, Olefsky JM. Inflammation and lipid signaling in the etiology of insulin resistance. Cell Metab (2012) 15(5):635–45. doi: 10.1016/j.cmet.2012.04.001
309. Yang Q, Li B, Sheng M. Meibum lipid composition in type 2 diabetics with dry eye. Exp Eye Res (2021) 206:108522. doi: 10.1016/j.exer.2021.108522
310. Knop E, Knop N, Millar T, Obata H, Sullivan DA. The international workshop on meibomian gland dysfunction: Report of the subcommittee on anatomy, physiology, and pathophysiology of the meibomian gland. Invest Ophthalmol Vis Sci (2011) 52(4):1938–78. doi: 10.1167/iovs.10-6997c
311. Han JW, Choi D, Lee MY, Huh YH, Yoon YS. Bone marrow-derived mesenchymal stem cells improve diabetic neuropathy by direct modulation of both angiogenesis and myelination in peripheral nerves. Cell Transplant (2016) 25(2):313–26. doi: 10.3727/096368915x688209
312. Monfrini M, Donzelli E, Rodriguez-Menendez V, Ballarini E, Carozzi VA, Chiorazzi A, et al. Therapeutic potential of mesenchymal stem cells for the treatment of diabetic peripheral neuropathy. Exp Neurol (2017) 288:75–84. doi: 10.1016/j.expneurol.2016.11.006
313. He D, Xu Y, Xiong X, Yin C, Lei S, Cheng X. The bone marrow-derived mesenchymal stem cells (Bmscs) alleviate diabetic peripheral neuropathy induced by stz Via activating gsk-3β/β-Catenin signaling pathway. Environ Toxicol Pharmacol (2020) 79:103432. doi: 10.1016/j.etap.2020.103432
314. Zhou JY, Zhang Z, Qian GS. Mesenchymal stem cells to treat diabetic neuropathy: A long and strenuous way from bench to the clinic. Cell Death Discovery (2016) 2:16055. doi: 10.1038/cddiscovery.2016.55
315. Di G, Du X, Qi X, Zhao X, Duan H, Li S, et al. Mesenchymal stem cells promote diabetic corneal epithelial wound healing through tsg-6-Dependent stem cell activation and macrophage switch. Invest Ophthalmol Vis Sci (2017) 58(10):4344–54. doi: 10.1167/iovs.17-21506
316. Zickri MB, Ahmad NA, Maadawi ZM, Mohamady YK, Metwally HG. Effect of stem cell therapy on induced diabetic keratopathy in albino rat. Int J Stem Cells (2012) 5(1):57–64. doi: 10.15283/ijsc.2012.5.1.57
317. Jackson CJ, Myklebust Ernø IT, Ringstad H, Tønseth KA, Dartt DA, Utheim TP. Simple limbal epithelial transplantation: Current status and future perspectives. Stem Cells Transl Med (2020) 9(3):316–27. doi: 10.1002/sctm.19-0203
318. Hayashi R, Ishikawa Y, Sasamoto Y, Katori R, Nomura N, Ichikawa T, et al. Co-Ordinated ocular development from human ips cells and recovery of corneal function. Nature (2016) 531(7594):376–80. doi: 10.1038/nature17000
319. He J, Ou S, Ren J, Sun H, He X, Zhao Z, et al. Tissue engineered corneal epithelium derived from clinical-grade human embryonic stem cells. Ocular Surface (2020) 18(4):672–80. doi: 10.1016/j.jtos.2020.07.009
320. Bandeira F, Goh TW, Setiawan M, Yam GH, Mehta JS. Cellular therapy of corneal epithelial defect by adipose mesenchymal stem cell-derived epithelial progenitors. Stem Cell Res Ther (2020) 11(1):14. doi: 10.1186/s13287-019-1533-1
321. Shukla S, Mittal SK, Foulsham W, Elbasiony E, Singhania D, Sahu SK, et al. Therapeutic efficacy of different routes of mesenchymal stem cell administration in corneal injury. Ocular Surface (2019) 17(4):729–36. doi: 10.1016/j.jtos.2019.07.005
322. Pflugfelder SC, Stern ME. Biological functions of tear film. Exp Eye Res (2020) 197:108115. doi: 10.1016/j.exer.2020.108115
323. Wong HL, Bu Y, Chan YK, Shih KC. Lycium barbarum polysaccharide promotes corneal re-epithelialization after alkaline injury. Exp Eye Res (2022) 221:109151. doi: 10.1016/j.exer.2022.109151
324. Desjardins P, Berthiaume R, Couture C, Le-Bel G, Roy V, Gros-Louis F, et al. Impact of exosomes released by different corneal cell types on the wound healing properties of human corneal epithelial cells. Int J Mol Sci (2022) 23(20):12201. doi: 10.3390/ijms232012201
Keywords: diabetic keratopathy, dry eye, neuropathy, epitheliopathy, lacrimal gland, pathogenesis
Citation: Zhou Q, Yang L, Wang Q, Li Y, Wei C and Xie L (2022) Mechanistic investigations of diabetic ocular surface diseases. Front. Endocrinol. 13:1079541. doi: 10.3389/fendo.2022.1079541
Received: 25 October 2022; Accepted: 02 December 2022;
Published: 16 December 2022.
Edited by:
Xinyuan Zhang, Beijing Tongren Hospital, Capital Medical University, ChinaReviewed by:
Yashan Bu, The University of Hong Kong, Hong Kong SAR, ChinaKofi Asiedu, University of New South Wales, Australia
Copyright © 2022 Zhou, Yang, Wang, Li, Wei and Xie. This is an open-access article distributed under the terms of the Creative Commons Attribution License (CC BY). The use, distribution or reproduction in other forums is permitted, provided the original author(s) and the copyright owner(s) are credited and that the original publication in this journal is cited, in accordance with accepted academic practice. No use, distribution or reproduction is permitted which does not comply with these terms.
*Correspondence: Lixin Xie, bGl4aW5feGllQGhvdG1haWwuY29t