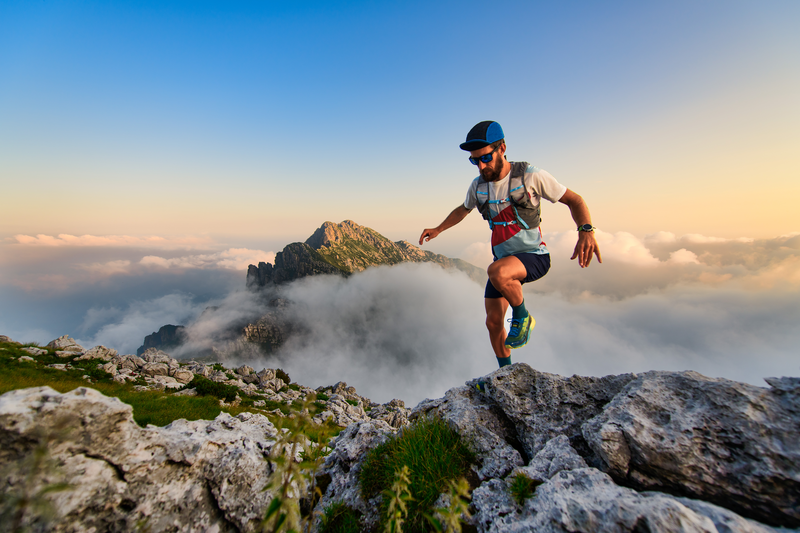
94% of researchers rate our articles as excellent or good
Learn more about the work of our research integrity team to safeguard the quality of each article we publish.
Find out more
ORIGINAL RESEARCH article
Front. Endocrinol. , 04 January 2023
Sec. Diabetes: Molecular Mechanisms
Volume 13 - 2022 | https://doi.org/10.3389/fendo.2022.1077514
This article is part of the Research Topic Novel Insights into the Pathophysiology of Diabetes-related Complications: Implications for Improved Therapeutic Strategies View all 11 articles
Diabetes is a global disease with huge impacts on patients due to its complications, among which non-healing wounds and depression are common and challenging. The neurokinin 1 receptor (NK1R) inhibitor, aprepitant has been broadly applied for an antidepressant effect in depressive patients. Recent literature has indicated a therapeutic effect of downregulation in NK1R to diabetes-related fracture, cardiomyopathy, gastroparesis, and ocular surface disorders. In this study, differential expression genes in diabetes and depression were analyzed based on several RNA sequencing datasets from the GEO database to confirm NK1R in the overlapping set. Interaction network and gene set enrichment analysis were subsequently conducted. As a result, NK1R-related genes took part in angiogenesis, epithelial-mesenchymal transition (EMT), collagen deposition, and inflammation in diabetes and depression. In vivo, the downregulation of NK1R was proved to promote vascular proliferation and enhance diabetic wound healing, which provides a potential therapeutic target for the management of diabetic non-healing wounds and depression.
Diabetes mellitus (DM) is a worldwide health problem associated with severe complications that influence both the patient’s quality of life and survival (1). As one of the major categories, Type 1 DM (T1DM) exhibits hyperglycemia, significant microvascular injury and a chronic inflammatory response thus resulting in peripheral nerve damage and impaired wound healing, especially at sacrococcygeal region and extremities (2, 3). Moreover, patients with T1DM tend to develop psychiatric morbidity (4). It has been stated that more than thirty percent of patients with T1DM may be suffered from depression (5). Although the exact mechanism remains unclear, treatment with insulin cannot simply manage the aforementioned conundrums simultaneously.
Recent studies have demonstrated the key role of neurogenic inflammation (NI) caused by the secretion of the proinflammatory neuropeptides from peripheral neurons in diabetes and depression (6, 7). As the specific receptor of neuropeptide Substance P (SP), the neurokinin-1 receptor (NK1R) is found expressed on neurons, epithelial cells, fibroblasts, and immune cells, thus mediating NI and contributes to inflammatory diseases and psychosomatic disorders (8). Aprepitant, the NK1R antagonist which originally developed for the blockage of the SP-NK1R pathway, has been broadly proved effective and beneficial for varies of depressions by both fundamental researches and clinical trials (9–13). On the other hand, NK1R antagonist demonstrated potential therapeutic effects for diabetic fractures, gastroparesis, and ocular surface diseases (14–16).
Therapeutic targets of diabetes have been widely studied. However, potential effects of aprepitant in diabetic non-healing wound were still lack of investigation. In this study, we hypothesized that NK1R played a vital role through inflammation in the development of T1DM-related non-healing wounds and depression. Moreover, inhibition of NK1R activation and subsequent pro-inflammatory initiation would possess a therapeutic effect in diabetic non-healing wound. The GSE37450 and GSE198597 dataset were used to explore the potential therapeutic effect of aprepitant for T1DM-related non-healing wounds and depression, respectively.
The RNA sequencing data of T1DM and depression were downloaded from the Gene Expression Omnibus (GEO) database (https://www.ncbi.nlm.nih.gov/geo/). GSE37450 was used to analyze T1DM gene expression and GSE198597 was used to analyze depression gene expression.
After log2 transformation, we used R (version 4.1.2) to calculate the differential expression of spleen leukocytes between normal and NOD mice in GSE37450. We performed lmFit and eBays functions in the limma package for multiple linear regression and statistical tests to obtain the significance of each differential expressed gene. The same methods were applied to GSE198597 for differential expression of the hypothalamus between normal and depressed C57BL/6N mice.
We performed Gene set enrichment analysis (GSEA) using the GSEA software (GSEA version: 4.2.3) from Broad Institute (https://www.gsea-msigdb.org/gsea/index.jsp). We downloaded Gene Ontology gene sets containing BP, CC, and MF subsets as well as hallmark gene sets in the Molecular Signatures Database (MSigDB). The minimum number of the gene set was 5, and the maximum number of the gene set was 5000. After a thousand resampling, we obtained the related pathways and molecular mechanisms in MSigDB.
Protein interaction data were derived from the STRING database (https://string-db.org). The interaction network was depicted in Cytoscape (version 3.4.1). Then, Pearson correlation analysis by the corr. test function of the psych package was performed for the NK1R-related genes in overlapped gene sets. In addition, the heatmaps of overlapped genes in each disease were obtained from a complex heatmap package.
C57BL/6 mice (male, 6 weeks old) were supplied by ShengChang Biotech (Shanghai, China). All animal experiments were approved by the Animal Experimentation Ethics Committee of the School of Medicine, Shanghai Jiao Tong University. C57BL/6 mice were accommodated in a controlled habitat and provided with water and rodent food for 1 week to be familiarized with their habitat.
After a week of adaptive feeding, C57BL/6 mice were singly intraperitoneally injected with streptozotocin (STZ, 150mg/kg, Sigma-Aldrich, S0130) in a freshly prepared citrate buffer (0.1 M, pH 4.5). In the next 5 days, the blood glucose was measured by a blood glucose monitor (Supplementary Materials, Table S1). The mice that showed blood glucose concentrations above 16.7 mM were considered diabetic and used for further experimentation.
After diabetes induction, a total of 24 diabetic mice were anesthetized by intraperitoneal injection of 10% chloral hydrate (3.5 mL per 1 kg body weight). Before wound fabrication, the dorsal side of the mice was shaved, depilated, and disinfected. Then, an oval wound (about 6 mm in diameter) was created on the dorsum of the mouse. The NK-1R antagonist aprepitant (Med Chem Express, CAS No. 170729-80-3, 15uL/wound) was injected around the wounds immediately. The 24 mice were randomly divided into 4 groups: 0uM (PBS, control) group; 200uM-aprepitant group; 500uM-aprepitant group and 1000uM-aprepitant group. Treatments were performed every day until Day 15. Digital photographs were taken at day 0, 5, 10, and 15. A circular marker (6mm in diameter) was placed around the wound to standardize the measurements. ImageJ software was used to calculate the wound area of each photographic image. Besides, body weight was also established at day 0, 5, 10, and 15.
After 15 days, all remaining mice were sacrificed, and the surrounding tissues of wounds were obtained for histological analysis. The wounds were fixed with 4% paraformaldehyde for tissue fixation. After being dehydrated with a series of graded ethanol, the tissues were embedded in paraffin and cut into 5-μm-thick longitudinal section. Then, the sections were stained with hematoxylin and eosin (H&E) and Masson’s trichrome stain. The image was taken by a microscope (Nikon, Japan).
The expression of CD31 in wounds was examined using an anti-CD31 antibody (Abcam, ab28364) by a standard immunohistochemistry protocol. Dewaxed sections were incubated with primary anti-CD31 antibody (Abcam, ab28364) at a dilution of 1:300 for 1 h at room temperature and then incubated with goat anti-mouse immunoglobulin (HRP, Abcam, ab6789) secondary antibody for 30 min at room temperature. The image was taken by a microscope (Nikon, Japan).
After 15 days, all remaining mice were sacrificed, and the surrounding tissues of wounds were obtained for qRT-PCR. Wound skin tissues were stored in liquid nitrogen and total RNA was extracted using TRIzol reagent (Invitrogen, USA) according to the manufacturer’s protocol. Then, 1 µg of total RNA was reverse transcribed into cDNA using the FastKing cDNA synthesis kit (Tiangen Biotech, China). qRT-PCR was performed with TB Green Premix (Takara, Japan). The mRNA expression levels of GAD1, ALDH1B1, FOXP2 were detected. PCR primers were designed based on sequences from the corresponding genes (Supplementary Materials, Table S2). All data were normalized using GAPDH as the internal control by the Δ-CT method.
Total proteins were extracted using RIPA lysis buffer containing phosphatase and protease inhibitors (Beyotime Biotechnology, China). The concentration of total protein was detected with a BCA Protein Assay kit (Beyotime Biotechnology, China). Equal amounts (20 µg) of protein were separated using 4–20% SDS-PAGE gels. The proteins were then transferred to nitrocellulose membranes (0.45µm; Millipore, USA). The membranes were blocked with 5% non-fat milk for 1 h at room temperature and incubated with primary antibodies (Supplementary Materials, Table S3) overnight at 4°C. After washing, the membranes were incubated with goat anti-rabbit secondary antibodies (Table S2). Finally, the membranes were visualized using the Odyssey CLx Infrared Imaging System (LI-COR Biosciences, Lincoln,Nebraska, USA). GAPDH and β-actin protein intensities were used as internal controls.
Data analysis was performed using GraphPad Prism (9.0.0, America). Continuous variables are presented as the mean ( ± standard deviation, SD). One-way analysis of variance (ANOVA) was performed to compare the means of multiple groups, and if significant, a post hoc Tukey’s test was used to explore the pairwise differences between groups. P-values< 0.05 were considered statistically significant.
By differential expression analysis of GSE37450, NK1R was found up-regulated in T1DM. Among all of the differentially expressed genes, NK1R belonged to the top-ranking sets. In hallmark gene sets, we found that differentially expressed genes in T1DM were significantly correlated with epithelial-mesenchymal transition (EMT), inflammatory response, myogenesis, apical junction, and angiogenesis (Figure 1).
Figure 1 (A) Volcano plot of differential expression analysis in GSE37450 dataset. (B) Rank of differentially expressed genes in GSE37450 dataset. (C)Heatmap of differential expression analysis in GSE37450 dataset. (D) Enrichment score and the rank in ordered dataset of GSEA hallmark gene sets in GSE37450 dataset. (E) The distribution of gene expression in significant hallmark gene sets in GSE37450. X axis represents log2(Fold Change) of enriched genes.
In GSE198597, we found NK1R was significantly up-regulated in depression and belonged to the top-ranking sets as well. These differently expressed genes in depression were enriched in EMT, interferon response, bile acid metabolism, oxidative phosphorylation and angiogenesis, which was similar to the enrichment analysis of T1DM (Figure 2).
Figure 2 (A) Volcano plot of differential expression analysis in GSE198597 dataset. (B) Rank of differentially expressed genes in GSE198597 dataset. (C)Heatmap of differential expression analysis in GSE198597 dataset. (D) Enrichment score and the rank in ordered dataset of GSEA hallmark gene sets in GSE198597 dataset. (E) The distribution of gene expression in significant hallmark gene sets in GSE198597. X axis represents log2(Fold Change) of enriched genes.
After differential expression analysis of GSE37450 and GSE198597, we found 97 overlapped genes that are differently expressed in both datasets (Figure 3A). The interaction network of these overlapped genes from STRING was depicted, and NK1R-related genes (Gal, Gad1, Foxp2, Lhx9, and Aldh1b1) in the network were extracted (Figures 3B, C). Then, Pearson analysis was performed to show the correlation among NK1R-related genes (Figures 3D, E). In addition, we constructed a heatmap of overlapped genes in each disease to show the expression pattern respectively (Figures 3F, G).
Figure 3 (A) Overlapped genes of differential expression analysis in both GSE37450 and GSE198597 dataset. (B) Interaction network of overlapped genes. (C) Interaction of NK1R-related genes in overlapped gene sets. (D) Pearson analysis of NK1R-related genes in overlapped gene sets in GSE37450 dataset. (E) Pearson analysis of NK1R-related genes in overlapped gene sets in GSE198597 dataset. (F) Heatmap of NK1R-related genes in overlapped gene sets in GSE37450 dataset. (G) Heatmap of NK1R-related genes in overlapped gene sets in GSE198597 dataset.
Furtherly, we performed GO enrichment analysis for overlapped genes. In the biological process, these genes were enriched in the regulation of cardiocyte differentiation, fat cell differentiation, and organ morphogenesis. In the cellular component, they were enriched in the adherens junction, basal plasma membrane, and extracellular matrix. As for molecule function, GDP binding, kinase binding, nuclear receptor activity, and transcription factor activity were found enriched by overlapped genes (Figures 4A–C). Then, we combined the expression matrix and GO enrichment analysis, constructing the interaction network between overlapped genes and GO terms (Figures 4D–I).
Figure 4 (A–C) GO enrichment analysis of overlapped gene sets. (D–F) GO enrichment analysis of overlapped gene sets based on GSE37450 expression matrix. (G–I) GO enrichment analysis of overlapped gene sets based on GSE198597 expression matrix.
To explore the potential therapeutic effect of NK-1R antagonist aprepitant for clinical treatment, we established a full-thickness diabetic wound model and used PBS, 200uM aprepitant, 500uM aprepitant, 1000uM aprepitant to treat the diabetic mice (Figure 5A). The unclosed wound rate in mice treated with 1000uM aprepitant was the lowest in these different treated groups at days 0, 5, 10, and 15 post-wounding (Figures 5B, C). Moreover, the aprepitant-treated groups resulted in the recovery of body weights compared with controled group (Figure 5D). Besides, histological analyses were performed to investigate the wound repair efficiency in different treatment groups at day 15 post-operation. H&E staining analysis(5X,20X,40X) showed that 1000uM aprepitant-treated group exhibited the highest re-epithelialization rate among other groups (Figure 5E). We also observed the Masson staining analysis which demonstrated that the collagen deposition in the 1000uM aprepitant-treated group was significantly increased when compared to other groups (Figure 5F). CD31 is an indicator of blood vessels and very few blood vessels were observed in the wound tissues of the PBS group, while the treatment of 200uM, 500uM, and 1000uM groups improved vascular network formation (Figure 5G). Notably, the most CD31-positive blood vessels per field in CD31 immunostaining photographs (40X) was achieved in the 1000uM group (Figure 5H). On Day 15, we measured the mRNA level of GAD1, ALDH1B1, and FOXP2 in the wound skins. The results showed that the levels of GAD1, ALDH1B1, and FOXP2 in the 1000uM aprepitant group were reduced compared to the PBS, and 200uM groups (Figure 5I). The results of western blotting showed similar concentration-related trend as qRT-PCR (Figure 5J). These data indicated that aprepitant significantly accelerated diabetic wound healing by promoting wound re-epithelialization, collagen deposition and angiogenesis, with down-regulated GAD1, ALDH1B1, and FOXP2 expression in wounds.
Figure 5 (A) Schematic timeline of diabetic wound formation and the following treatments. (B, C) Representative images and quantitative measurement of wounds on days 0, 5, 10, and 15 days. (D) Changes of body weights of mice after different treatments. (E, F) Representative H&E staining images and Masson staining images. (G, H) Representative CD31 immunostaining images after different treatments and the corresponding quantitative data of the CD31-positive blood vessels’ numbers from respective staining images (40X). (I) qRT-PCR analysis of relative ALDH1B1, FOXP2, and GAD1 levels in different treated groups. (J) Expression of ALDH1B1, FOXP2, and GAD1 levels in different treated groups detected by western blotting analysis. Scale bars: 500μm for 5X, 200μm for 20X, and 100μm for 40X. n=6, ns: no significant, *p <.05, **p <.01, ***p <.001, ****p <.0001. Data were presented as mean ± SD. One-way ANOVA with Tukey post-hoc test was used.
It is commonly acknowledged that T1DM results from the inflammatory response, exhibiting microvascular injury and damage to peripheral nerves (17). As a critical mediator between neurons and inflammation, NK1R is responsible for the release of proinflammatory cytokines such as CCL4, CXCL2, MCP-1, CCL5, and IL-8, which recruit monocytes, macrophages, and lymphocytes thus leading to the pathogenesis of various inflammatory diseases (18). Neuroimmune interactions with macrophages controlled islet destruction, indicating the control of neuroimmune interactions may represent a new therapeutic target for T1DM (19). Recent reports showed that targeting NK1R was effective in several diabetes-related diseases. Carlin et al. (15) found that the application of NK1R antagonist, and tradipitant clinically improved the patients with diabetic gastroparesis. Jeong et al. (20) described the effects of selective NK1R antagonist RP-67580 in diabetic atria. Although there are studies illustrating the association between NK1R and diabetic complications, e.g., corneal wound healing, fracture, and limb ischemia, evidence-based insight into the exact mechanisms were still lacking (14, 21, 22). As diabetic non-healing wounds and depression are highly correlated with chronic inflammation while NK1R plays a central role in the development of inflammation via angiogenesis, we hypothesized NK1R as a potential therapeutic target for both diseases (23).
Based on the results of the bioinformatics analysis, differential gene expression showed that NK1R was significantly upregulated in diabetes and depression, indicating a key role of NK1R in both diseases. According to hallmark gene sets in GSEA, differently expressed genes in diabetes were enriched in EMT, inflammatory response, and angiogenesis, which was also found highly correlated with depression, indicating the potentially shared mechanisms between the two diseases. Then, we performed GO analysis on overlapped genes to clarify the enrichment relationship between diabetes and depression. The results showed that these genes were enriched in organ morphogenesis, fat cell and cardiocyte differentiation, adherens junction, basal plasma membrane, extracellular matrix, GDP and kinase binding, nuclear receptor activity as well as a transcription factor.
To further explore the key proteins related to NK1R which affected the development of diabetes and depression, we conducted PPI analysis and identified the five NK1R-related genes: aldehyde dehydrogenase 1B1 (ALDH1B1), forkhead box P2 (FOXP2), galanin (GAL), glutamate decarboxylase 1 (GAD1) and LIM homeobox 9 (LHX9). Among the five aforementioned genes, GAL has been broadly studied along with the NK1R in neurons and found to interact with life stresses thus mediating depression and anxiety (24–26). Moreover, GAL also regulated insulin release, which was associated with T1DM development (27). ALDH1B1 was found upregulated in the injured pancreas after streptozotocin paradigms and showed an association with diabetes (28, 29). Li T et al. revealed the relationship between FOXP2 and depression (30). As to LHX9, the hypothalamic marker has been proven highly correlated with FOXP2 in patients with depression (30–33). Combined with the enrichment analysis, we provoked the hypothesis that the GAD1, ALDH1B1, and FOXP2 genes functioned with NK1R in terms of angiogenesis, EMT, collagen deposition, and inflammation, while blockade of NK1R may be therapeutic not only for depression but also for the diabetic non-healing wound.
To further verify the hypothesis, C57BL/6 mice were generated as a murine model of a T1DM non-healing wound. Strikingly, we found that topically applied NK1R antagonist, aprepitant could promote the healing of the refractory diabetic wound in a concentration-related manner. qRT-PCR and western blotting were then used for the verification of selected related genes and proteins in the diabetic non-healing wound model. The mRNA level of GAD1, ALDH1B1 and FOXP2 was observed to downregulated in aprepitant-treated mice in a concentration-related and negatively-correlated manner. The expression of these proteins was proved to be downregulated with aprepitant and showed the same concentration-related manner as qRT-PCR. Versus the control group, the thickening epithelial layer and increased collagen deposition could be observed by H&E and Masson staining. CD31 immunohistochemistry showed that a high concentration of aprepitant could potentially increase neovascularization. These phenomenons provided compelling pieces of evidence that aprepitant could effectively promote the healing process of T1DM non-healing wounds through regulation of the aforementioned NK1R-related genes. The underlying mechanism is still controversial. Momen (34) contributed the aprepitant-related angiogenesis to MMP-2, MMP-9, VEGF, and VEGFR. On the other hand, regulation of EGFR, Akt, and SIRT1 by NK1R blockade was also considered beneficial in terms of re-epithelialization and collagen deposition (35–37).
To the best of our knowledge, our study was the first to utilize bioinformatics methods in detecting potential therapeutic targets in diabetic non-healing wound and depression. We explored the common mechanisms of the two diseases, analyzed roles of NK1R-related genes and verified the therapeutic effect of aprepitant. Although the related pathways and key molecules remain not entirely clear, our work provided promising evidences that blockage of the SP-NK1R pathway will downregulate the expression of ALDH1B1, FOXP2 and GAD1, which may be therapeutic for both diseases.
Besides, the neuroinflammation network plays an important role in both diseases, while NK1R could initiate neurogenic inflammation thus leading to neuronal damage (38–40). The release of inflammatory cytokines and the activation of CD8+ T cells contribute to pancreas injury, followed by vasodilation and increased permeability of the microvasculature caused by NK1R (2, 18). Consequently, rescuing the disrupted vascular endothelial cells and relieving neurogenic inflammation through the NK1R pathway stands as a promising therapeutic strategy. Our study also has some limitations. Due to lacking in feasible animal models with both diseases, further in vivo study for depression in T1DM mice is yet to be verified. Moreover, in-depth researches need to be carried out to reveal the underlying mechanisms and complex interplay between the two diseases.
The datasets presented in this study can be found in online repositories. The names of the repository/repositories and accession number(s) can be found in the article/Supplementary Material.
The animal study was reviewed and approved by Animal Experimentation Ethics Committee of the School of Medicine, Shanghai Jiao Tong University.
ML and HM performed data processing, animal experiments as well as statistical analysis, and were major contributors to writing the manuscript. SZ conducted the experiments and YP took part in the statistical analysis. LD helped in the data collection. YZ revised the manuscript. PM conceived and designed this study and revised the manuscript. All the authors read and approved the final manuscript.
This study was supported by the National Natural Science Foundation of China (grant number: 82172222, 81801918), the Outstanding Professional and Technical Leader Program of the Shanghai Municipal Science and Technology Commission(18XD1423700), the Shanghai Health Industry Clinical Research Special Project (20204Y0443), and the Cross Research Project of Ninth People’s Hospital, Shanghai Jiao Tong University School of Medicine (JYJC202009), Shanghai Municipal Education Commission-Gaofeng Clinical Medicine Grant Support (Grant number:20152227).
The authors declare that the research was conducted in the absence of any commercial or financial relationships that could be construed as a potential conflict of interest.
All claims expressed in this article are solely those of the authors and do not necessarily represent those of their affiliated organizations, or those of the publisher, the editors and the reviewers. Any product that may be evaluated in this article, or claim that may be made by its manufacturer, is not guaranteed or endorsed by the publisher.
The Supplementary Material for this article can be found online at: https://www.frontiersin.org/articles/10.3389/fendo.2022.1077514/full#supplementary-material
1. Siddiqui AA, Siddiqui SA, Ahmad S, Siddiqui S, Ahsan I, Sahu K. Diabetes: Mechanism, pathophysiology and management-a review. Int J Drug Dev Res (2013) 5(2):0–.
2. Baltzis D, Eleftheriadou I, Veves A. Pathogenesis and treatment of impaired wound healing in diabetes mellitus: new insights. Adv Ther (2014) 31(8):817–36.
4. Jacobson AM, Samson JA, Weinger K, Ryan CM. Diabetes, the brain, and behavior: is there a biological mechanism underlying the association between diabetes and depression? Int Rev Neurobiol (2002) 51:455–79.
5. Prabhakar V, Gupta D, Kanade P, Radhakrishnan M. Diabetes-associated depression: the serotonergic system as a novel multifunctional target. Indian J Pharmacol (2015) 47(1):4.
6. Duarte-Silva E, de Melo MG, Maes M, Chaves Filho AJM, Macedo D, Peixoto CA. Shared metabolic and neuroimmune mechanisms underlying type 2 diabetes mellitus and major depressive disorder. Prog Neuropsychopharmacol Biol Psychiatry (2021) 111:110351. doi: 10.1016/j.pnpbp.2021.110351
7. Tsui H, Winer S, Chan Y, Truong D, Tang L, Yantha J, et al. Islet glia, neurons, and β cells: The neuroimmune interface in the pathogenesis of type 1 diabetes. Ann New York Acad Sci (2008) 1150(1):32–42. doi: 10.1196/annals.1447.033
8. Muñoz M, Coveñas R, Esteban F, Redondo M. The substance P/NK-1 receptor system: NK-1 receptor antagonists as anti-cancer drugs. J biosciences. (2015) 40(2):441–63.
9. Ebner K, Singewald GM, Whittle N, Ferraguti F, Singewald N. Neurokinin 1 receptor antagonism promotes active stress coping via enhanced septal 5-HT transmission. Neuropsychopharmacology (2008) 33(8):1929–41. doi: 10.1038/sj.npp.1301594
10. Schank JR, Heilig M. Chapter six - substance p and the neurokinin-1 receptor: The new CRF. In: Thiele TE, editor. International review of neurobiology. 136: Academic Press (2017) 151–75. doi: 10.1016/bs.irn.2017.06.008
11. Hargreaves R. Imaging substance p receptors (NK1) in the living human brain using positron emission tomography. J Clin Psychiatry (2002) 63(11):18–24.
12. Kramer MS, Cutler N, Feighner J, Shrivastava R, Carman J, Sramek JJ, et al. Distinct mechanism for antidepressant activity by blockade of central substance p receptors. Science (1998) 281(5383):1640–5. doi: 10.1126/science.281.5383.1640
13. Krishnan KRR. Clinical experience with substance p receptor (NK1) antagonists in depression. J Clin Psychiatry (2002) 63(11):25–9.
14. Wang X, Su N. Neurokinin-1-tachykinin receptor agonist promotes diabetic fracture healing in rats with type 1 diabetes via modulation of wnt/β-catenin signalling axis. Saudi J Biol Sci (2021) 28(4):2139–45. doi: 10.1016/j.sjbs.2021.02.026
15. Carlin JL, Lieberman VR, Dahal A, Keefe MS, Xiao C, Birznieks G, et al. Efficacy and safety of tradipitant in patients with diabetic and idiopathic gastroparesis in a randomized, placebo-controlled trial. Gastroenterology (2021) 160(1):76–87.e4. doi: 10.1053/j.gastro.2020.07.029
16. Zhu L, Titone R, Robertson DM. The impact of hyperglycemia on the corneal epithelium: molecular mechanisms and insight. Ocular Surface. (2019) 17(4):644–54. doi: 10.1016/j.jtos.2019.06.007
17. Egan AM, Dinneen SF. What is diabetes? Medicine (2019) 47(1):1–4. doi: 10.1016/j.mpmed.2018.10.002
18. O'Connor TM, O'Connell J, O'Brien DI, Goode T, Bredin CP, Shanahan F. The role of substance p in inflammatory disease. J Cell Physiol (2004) 201(2):167–80. doi: 10.1002/jcp.20061
19. Christoffersson G, Ratliff SS, von Herrath MG. Interference with pancreatic sympathetic signaling halts the onset of diabetes in mice. Sci Adv (2020) 6(35):eabb2878. doi: 10.1126/sciadv.abb2878
20. Jeong Y, Lee S, Kim W. Substance P/neurokinin1 receptor is associated with the expression of cardiac stem/pluripotency-associated genes in diabetic right and left atria. Research Square (2021). doi: 10.21203/rs.3.rs-254561/v1
21. Dang Z, Avolio E, Albertario A, Sala-Newby GB, Thomas AC, Wang N, et al. Nerve growth factor gene therapy improves bone marrow sensory innervation and nociceptor-mediated stem cell release in a mouse model of type 1 diabetes with limb ischaemia. Diabetologia (2019) 62(7):1297–311. doi: 10.1007/s00125-019-4860-y
22. Yang L, Di G, Qi X, Qu M, Wang Y, Duan H, et al. Substance p promotes diabetic corneal epithelial wound healing through molecular mechanisms mediated via the neurokinin-1 receptor. Diabetes (2014) 63(12):4262–74. doi: 10.2337/db14-0163
23. Esteban F, Ramos-García P, Muñoz M, González-Moles MÁ. Substance p and neurokinin 1 receptor in chronic inflammation and cancer of the head and neck: A review of the literature. Int J Environ Res Public Health (2022) 19(1):375.
24. Spirovski D, Li Q, Pilowsky PM. Brainstem galanin-synthesizing neurons are differentially activated by chemoreceptor stimuli and represent a subpopulation of respiratory neurons. J Comp Neurology. (2012) 520(1):154–73. doi: 10.1002/cne.22723
25. Rana T, Behl T, Sehgal A, Singh S, Sharma N, Abdeen A, et al. Exploring the role of neuropeptides in depression and anxiety. Prog Neuropsychopharmacol Biol Psychiatry (2022) 114:110478. doi: 10.1016/j.pnpbp.2021.110478
26. Juhasz G, Hullam G, Eszlari N, Gonda X, Antal P, Anderson IM, et al. Brain galanin system genes interact with life stresses in depression-related phenotypes. Proc Natl Acad Sci (2014) 111(16):E1666–E73. doi: 10.1073/pnas.1403649111
27. Fang P, Yu M, Shi M, Bo P, Zhang Z. Galanin peptide family regulation of glucose metabolism. Front Neuroendocrinology. (2020) 56:100801. doi: 10.1016/j.yfrne.2019.100801
28. Ioannou M, Serafimidis I, Arnes L, Sussel L, Singh S, Vasiliou V, et al. ALDH1B1 is a potential stem/progenitor marker for multiple pancreas progenitor pools. Dev Biol (2013) 374(1):153–63. doi: 10.1016/j.ydbio.2012.10.030
29. Singh S, Chen Y, Matsumoto A, Orlicky DJ, Dong H, Thompson DC, et al. ALDH1B1 links alcohol consumption and diabetes. Biochem Biophys Res Commun (2015) 463(4):768–73. doi: 10.1016/j.bbrc.2015.06.011
30. Li T, Zeng Z, Zhao Q, Wang T, Huang K, Li J, et al. FoxP2 is significantly associated with schizophrenia and major depression in the Chinese han population. World J Biol Psychiatry (2013) 14(2):146–50. doi: 10.3109/15622975.2011.615860
31. Liu J, Merkle FT, Gandhi AV, Gagnon JA, Woods IG, Chiu CN, et al. Evolutionarily conserved regulation of hypocretin neuron specification by Lhx9. Development (2015) 142(6):1113–24. doi: 10.1242/dev.117424
32. Homman-Ludiye J, Kwan WC, de Souza MJ, Bourne JA. Full: Ontogenesis and development of the nonhuman primate pulvinar. J Comp Neurology. (2018) 526(17):2870–83. doi: 10.1002/cne.24534
33. Dalal J, Roh JH, Maloney SE, Akuffo A, Shah S, Yuan H, et al. Translational profiling of hypocretin neurons identifies candidate molecules for sleep regulation. Genes Dev (2013) 27(5):565–78. doi: 10.1101/gad.207654.112
34. Momen Razmgah M, Ghahremanloo A, Javid H, AlAlikhan A, Afshari A-R, Hashemy SI. The effect of substance p and its specific antagonist (aprepitant) on the expression of MMP-2, MMP-9, VEGF, and VEGFR in ovarian cancer cells. Mol Biol Rep (2022) 49(10):9307–14. doi: 10.1007/s11033-022-07771-w
35. Zhang S, Li K, Yu Z, Jun C, Zhang Z, Zhang Y, et al. Dramatic effect of botulinum toxin type a on hypertrophic scar: A promising therapeutic drug and its mechanism through the SP-NK1R pathway in cutaneous neurogenic inflammation. Front Med (2022) 520. doi: 10.3389/fmed.2022.820817
36. Backman LJ, Eriksson DE, Danielson P. Substance p reduces TNF-α-induced apoptosis in human tenocytes through NK-1 receptor stimulation. Br J sports Med (2014) 48(19):1414–20. doi: 10.1136/bjsports-2013-092438
37. Ceci L, Francis H, Zhou T, Giang T, Yang Z, Meng F, et al. Knockout of the tachykinin receptor 1 in the Mdr2–/–(Abcb4–/–) mouse model of primary sclerosing cholangitis reduces biliary damage and liver fibrosis. Am J Pathology. (2020) 190(11):2251–66. doi: 10.1016/j.ajpath.2020.07.007
38. Singh RB, Naderi A, Cho W, Ortiz G, Musayeva A, Dohlman TH, et al. Modulating the tachykinin: Role of substance p and neurokinin receptor expression in ocular surface disorders. Ocular Surface (2022) 25:142–53. doi: 10.1016/j.jtos.2022.06.007
39. Jayasimhan A, Mariño E. Dietary SCFAs, IL-22, and GFAP: The three musketeers in the gut–Neuro–Immune network in type 1 diabetes. Front Immunol (2019) 10:2429. doi: 10.3389/fimmu.2019.02429
40. Morris G, Berk M F, Carvalho A, Caso J R, Sanz Y, Maes M. The role of microbiota and intestinal permeability in the pathophysiology of autoimmune and neuroimmune processes with an emphasis on inflammatory bowel disease type 1 diabetes and chronic fatigue syndrome. Curr Pharm design. (2016) 22(40):6058–75. doi: 10.2174/1381612822666160914182822
Keywords: NK1R, aprepitant, T1DM, depression, wound healing
Citation: Li M, Ma H, Zhang S, Peng Y, Ding L, Zhang Y and Min P (2023) Potential therapeutic effect of NK1R antagonist in diabetic non-healing wound and depression. Front. Endocrinol. 13:1077514. doi: 10.3389/fendo.2022.1077514
Received: 23 October 2022; Accepted: 13 December 2022;
Published: 04 January 2023.
Edited by:
Jian Ma, Harbin Medical University, ChinaReviewed by:
Chen Li, Free University of Berlin, GermanyCopyright © 2023 Li, Ma, Zhang, Peng, Ding, Zhang and Min. This is an open-access article distributed under the terms of the Creative Commons Attribution License (CC BY). The use, distribution or reproduction in other forums is permitted, provided the original author(s) and the copyright owner(s) are credited and that the original publication in this journal is cited, in accordance with accepted academic practice. No use, distribution or reproduction is permitted which does not comply with these terms.
*Correspondence: Yixin Zhang, emhhbmd5aXhpbjY2ODhAMTYzLmNvbQ==; Peiru Min, YXJ1X3JlbkBtc24uY29t
†These authors have contributed equally to this work and share first authorship
Disclaimer: All claims expressed in this article are solely those of the authors and do not necessarily represent those of their affiliated organizations, or those of the publisher, the editors and the reviewers. Any product that may be evaluated in this article or claim that may be made by its manufacturer is not guaranteed or endorsed by the publisher.
Research integrity at Frontiers
Learn more about the work of our research integrity team to safeguard the quality of each article we publish.