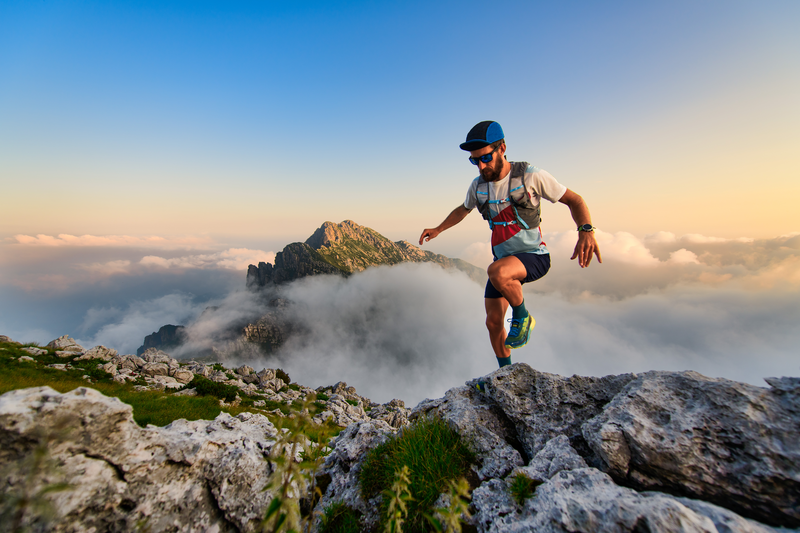
95% of researchers rate our articles as excellent or good
Learn more about the work of our research integrity team to safeguard the quality of each article we publish.
Find out more
MINI REVIEW article
Front. Endocrinol. , 11 January 2023
Sec. Clinical Diabetes
Volume 13 - 2022 | https://doi.org/10.3389/fendo.2022.1074568
This article is part of the Research Topic Clinical and Genetic Determinants of Diabetes and Complications View all 31 articles
Wound healing is a programmed process of continuous events which is impaired in the case of diabetic patients. This impaired process of healing in diabetics leads to amputation, longer hospitalisation, immobilisation, low self-esteem, and mortality in some patients. This problem has paved the way for several innovative strategies like the use of nanotechnology for the treatment of wounds in diabetic patients. The use of biomaterials, nanomaterials have advanced approaches in tissue engineering by designing multi-functional nanocomposite scaffolds. Stimuli-responsive scaffolds that interact with the wound microenvironment and controlled release of bioactive molecules have helped in overcoming barriers in healing. The use of different types of nanocomposite scaffolds for faster healing of diabetic wounds is constantly being studied. Nanocomposites have helped in addressing specific issues with respect to healing and improving angiogenesis. Method: A literature search was followed to retrieve the articles on strategies for wound healing in diabetes across several databases like PubMed, EMBASE, Scopus and Cochrane database. The search was performed in May 2022 by two researchers independently. They keywords used were “diabetic wounds, nanotechnology, nanocomposites, nanoparticles, chronic diabetic wounds, diabetic foot ulcer, hydrogel”. Exclusion criteria included insulin resistance, burn wound, dressing material.
Nanomedicine is one of the fastest-growing fields offering several avenues for therapy, diagnostics, delivery systems and improving efficiency (1). The superior properties of the ‘nano’ components have been used in tissue engineering for the repair and regeneration of several organs and tissues. Wound healing is a normal process involving a series of steps; however it is affected by a number of variables like age, obesity, stress, diseases, habits, infections, trauma etc. (2). But in certain conditions, healing is halted at the second phase, i.e., the inflammatory phase which could be due to chronic conditions. Many diseases that cause impaired blood flow, such as in the case of diabetic foot ulcers or pressure ulcers are the contributing factors. Common wound pathogens, nosocomial infections are also known to hinder the progression of healing to the third phase, which is proliferation. Several factors are attributed to patients with diabetes mellitus such as the improper function of macrophages and growth factors, and low blood circulation; the major factor for delayed wound healing (3). The incidence of diabetes is seen to be increasing at a steady rate globally, with a mortality rate of 1.5 million deaths in 2019. The indirect death due to diabetes was 460,000 due to kidney disease, and 20% due to cardiovascular complications (4). Diabetic patients are prone to develop diabetic foot ulcers and the percentage affected is more than 20% (5). To treat such chronic wounds, newer therapies such as cell/gene therapy, and engineered biomaterials are sought after due to unsuccessful treatment modalities. Tissue engineering has led researchers to explore several new skin substitutes using natural, synthetic, and semi-synthetic polymers. They are often used in combination with biomolecules, proteins, and polysaccharides (6). To overcome the existing limitations, they have been combined with nanomaterial to form a highly functional, multi-modal, smart nanocomposite to treat chronic wounds such as in the case of diabetes (7). The major advantages of nanotherpay are due to the charge, and large surface area to volume ratio that enhances the interaction with the target area (8). The ability to encapsulate and control the drug release by attaining a sustained release of the desired biomolecules leads to accelerated healing (9). Figure 1 represents the types of nanocomposites and its advantages in wound healing.
Various factors, including pH, temperature, blood sugar level, and oxygen saturation, are important in the healing of wounds. Scaffolds have attracted interest recently as a novel dressing and provide an innovative perspective on tissue regeneration (10, 11). Researchers state that the design of the dressing material spatially is of utmost importance for a biomaterial to function as an effective regenerative scaffold, which is now possible through nanocomposites which have been summarised in this review.
While researchers are experimenting with effective and scarless wound healing strategies, wound management in diabetes itself is a tedious process for the patients and the healthcare sector. So, several advanced techniques and technology have been employed in the remedial measures for diabetic wounds.
Several types of metal nanoparticles, metal oxide nanoparticles, nanotubes, and polymeric nanoparticles have been used in wound healing. Because of their innate antimicrobial property, the silver nanoparticle has been extensively used in wound care management. It is strikingly important to note that silver nanoparticles are effective against nosocomial infections and multidrug-resistant pathogens (12, 13). At a concentration of 50 mg/ml silver nanoparticles were observed to destruct the bacterial cell membrane and kill S. aureus and E. coli (14).
A nanocomposite was fabricated with polyethylene glycol diacrylate, silica, bioactive glass nanoparticles, sodium alginate and copper. This silica-based nanocomposite was found to be an excellent injectable with elastomeric, biomimetic, and antibacterial properties. The regeneration of blood vessels was observed with high collagen deposition, and VEGF expression in a full-thickness diabetic wound model (15). Table 1 indicates the different types of nanocomposites employed for diabetic wound healing.
Nitric oxide (NO) induces the formation of blood vessels and the migration of endothelial cells by eNOS or MAPk pathways. Zinc oxide is known to induce NO production, hence several scaffolds for wound healing have incorporated ZnO NP’s nanofibers fabricated using poly-caprolactone with ZnO NP’s which exhibited high proliferation of fibroblast cells. A higher rate of vascular regeneration was observed because of the expression of VEGF and FGF (27). Cerium oxide nanoparticles were used in combination with microRNA (miR-146a) for faster healing in diabetic wounds. The synergistic role of scavenging the free radicals and modulating the inflammatory pathway proved to increase the synthesis of collagen, thereby higher rate of angiogenesis and low inflammation; this aided in a significantly higher rate of wound closure (28).
Poly-N-acetyl-glucosamine based nanofibrous scaffold was prepared to overcome the limitations in treating a diabetic wound. This bioactive scaffold was found to enhance cell metabolism, and migration of endothelial cells with a higher rate of wound closure in a full- thickness diabetic mice model. The gene expression of uPAR, VEGF, Il-1 and MMP responsible for migration, angiogenesis, inflammatory activity, and matrix remodelling was observed (29). Another study found that short-fibre poly-N-acetyl glucosamine nanofibers were used alongside the vacuum-assisted closure of complex wounds. This aided in controlling the blood loss by acting as a hemostatic agent activating platelets and better granulation. The presence of collagen I and the wound contraction rate was significant in the treated groups (30).
The ulcers in diabetic wounds are caused by oxidative stress, so researchers prepared Prussian blue nanoparticles (PBNP’s) to scavenge the free radicals generated at the wound site. This PBNP was encapsulated in a heat-sensitive gel using poly (d, L-lactide)-poly (ethylene glycol)-poly (d, L-lactide) (PDLLA-PEG-PDLLA) hydrogel (PLEL). It was confirmed that the nanoparticle was able to protect the cells and mitochondria against reactive oxygen species (ROS). In an animal model, it was found to progress diabetic wound healing at a faster rate, reduce ROS production, and enhance cell survival and growth simultaneously reducing the interleukin and tumor necrosis factor (31).
A pH-responsive scaffold was developed which aided in faster healing with less scar formation. This injectable scaffold was prepared with polysaccharides and exhibited antibacterial activity against multi-drug resistant bacteria. In vivo studies showed that the exosome released promoted angiogenesis in the full-thickness wound (32). A dual responsive scaffold that modulates the release based on pH and metformin release was prepared using PEG. The active components encapsulated were phenylboronic acid, benzaldehyde, L-arginine, and chitosan which exhibited anti-inflammatory effects and promoted angiogenesis. The synergistic healing of metformin and graphene oxide was observed in a rat model with type II diabetic foot ulcer. Based on the stimulus it was found to release the drug, metformin which was faster healing in chronic diabetic athletic wounds (33).
Silver nanoclusters were conjugated with vancomycin in a gelatin-based hydrogel along with nimesulide that is pH sensitive. This complete biomaterial containing phenylboronic acid and polyvinyl alcohol also contained ROS and exhibited anti-inflammatory action. It was found to be biocompatible, with excellent cell-adhesive behaviour and aided healing in wounds with infection. Because of its sensitive and dual-responsive properties, hydrogel was found to be good for treating chronic diabetic wounds (34). A thermos-responsive scaffold that is skin-friendly and designed for infants and diabetic patients with sensitive skin was attempted by researchers. This non-irritable hydrogel patch was designed with a protein-polyphenol complex that was activated upon reaction to the body temperature upon application. This was found to be skin-friendly and gentle even for a prolonged period of use because of its immune-modulatory action (35).
Hydrogels are the most preferred dressing agent for wound healing owing to their capability to retain moisture at the site of wounds, agent because hydrogels are designed to hold moisture at the wound surface, and create the best setting for healing, balancing skin hydration and in the removal of necrotic tissue. They could be prepared with ease providing sustained drug release. Both natural and synthetic polymers could be used in the preparation of hydrogels. These may include, fibrin, hyaluronic acid, cellulose derivatives, copolymers and others (36).
Hydrogels are exceptional in providing a humid atmosphere for the healing of wounds and ensure permeable water vapours with microbial entry prevention at the wound site. A heparinised PVA-based hydrogel formulated demonstrated significant antibacterial activity without any cellular toxic effects (37). Another hydrogel containing coumestrol/hydroxypropyl-β-cyclodextrin was developed using hydroxypropyl methylcellulose. The insoluble coumestrol (helps with photoaging; improves elasticity of skin during menopause) was solubilized using hydroxypropyl-β-cyclodextrin to obtain a hydrogel which led to faster wound healing process through the better propagation of cells. This also demonstrated good cell adhesion and compatibility as observed through Wistar rats (38). A gel-based hydrogel was formulated using adipose-derived stem cells as a suitable wound healing agent that was obtained from both mouse and porcine models. These in vivo models demonstrated excellent healing of wounds (39). Topic nitric oxide helps in the healing process of acute and chronic wounds. An antibacterial peptide was developed based on this, which could self-assemble with respect to changes in pH, and could lead to the development of hydrogel with improved bactericidal activity (40). A ZnO-based nanocomposite hydrogel demonstrated significant antibacterial properties and was found biocompatible and safe with a faster rate of wound healing (41). Though there are many ongoing research on hydrogels related to skin repair, there is another group of researchers who developed hydrogels containing HA and carboxylated CS that mimics skin with high mechanical strength. The in vitro studies on L929 cells demonstrate superior biocompatibility with improved cell proliferation. Further, in vivo studies also demonstrated a faster healing process and suggested that this hydrogel as an ideal candidate suited for wound recovery and healing (42). Though there are many ongoing research on hydrogels used as wound dressing agent, we would like to identify the importance of hydrogels as a potential wound dressing agent with reference to diabetic wounds.
Diabetes being a chronic disease is yet challenging to cure and the medical requirements are inadequate (43). The skin wounds caused by diabetes do not get completely healed due to limited blood supply and deprived antimicrobial capability with the poor inflammatory response (44). Among 750,000 emerging cases of diabetic foot ulcer in America, nearly 10% of cases involved amputation of limbs every year (45). Many measures are taken for treating wound healing due to diabetes such as growth factor and cellular-based therapy but the cost was too high (46–48). So, there has been an increasing interest in bioactive biomaterials as a potent wound dressing agent for treating in case of diabetic-based wounds (49). Some of the biomaterials have progressed to clinics such as biomedical hydrogels, films and ointments, and others (50). On the other hand, multifunctional biomaterials are developed with potent antioxidants, antibacterial activity and hemostasis (51–53). Hydrogel biomaterial-based dressings are also developed with their property similar to that of the extracellular matrix and this demonstrated good wound healing (54–58).
Due to vascular impairment, diabetes-related wound healing and skin regrowth remain a major concern. To overcome this, a silica-based nanocomposite hydrogel scaffold that could promote both wound healing and skin regeneration in diabetic conditions was developed by enhancing early angiogenesis with no bioactive factors. This injectable nanocomposite exhibits an excellent healing pattern with superior antibacterial properties. Also, enables viability, growth, and angiogenesis of endothelial progenitor cells through in vitro studies. In vivo studies demonstrated restoration of blood vessels through HIF-1α/VEGF and collagen deposition in diabetic wound. It was also suggested to have its application in regenerative medicine (15). Several tissue engineering strategies using nanobiomaterials for vascular regeneration have been reported (59). A multifunctional sprayable cross-linking bioadhesive hydrogel-based nanocomposite was developed for diabetic wound healing. Here, Kappa-carrageenan being the hydrogel matrix, different concentrations of modified ZnO nanoparticles were incorporated to improve their mechanical properties with good antibacterial activity. To this, L-glutamic acid was also loaded into this network to enhance the rate of wound healing. This biocompatible nanocomposite also demonstrated elasticity similar to human skin with adhesive nature and clotting capability. The in vivo studies further demonstrated significant wound healing at a faster rate without any infection (59). A 2-D nanoclay (Laponite RD)/polymer-based nanocomposite hydrogels were developed as a substitute for treating foot ulcers due to diabetes. It was also suggested that enzymes or active compounds loaded to the hydrogel could help in the healing of diabetic foot ulcers through their antibacterial activity (60). Another research on zwitterionic poly (sulfobetaine acrylamide) nanocomposite that was composed of hectorite nanoclay demonstrated as a potent chronic wound dressing agent. This hydrogel exhibited insignificant cytotoxicity against NIH-3T3 fibroblast and was resistant against the adsorption of BSA and certain bacterial strains. In vivo studies on both normal and diabetic wounds were conducted in mice in comparison with commercially available dressings. Histology confirmed significant re-epithelialization and faster healing of diabetic wounds than the commercial products (61). A bioactive HQB nanocomposite hydrogel was developed through the cross-linking of modified hyaluronic acid with quaternized chitosan coated with bioactive glasses. This demonstrated superior wound healing properties in diabetic-induced rats and suggested it to have a good prospect in clinical application (62). A cost-effective and simple dual-network hydrogel comprised of MnO2 nanosheets was developed from silk fibroin and carboxymethyl cellulose. This helped in angiogenesis, reduced inflammation, and had remarkable healing rates comparable to commercial dressing through in vivo studies (63). An alginate and Eudragit nanoparticle-based nanocomposite hydrogel comprising edaravone was produced for the highest ROS sequestration to overcome chronic inflammation and delayed wound healing in diabetes. A lower dosage of this hydrogel enhanced wound healing, and a higher dosage impeded the healing process in diabetic mice and suggested dosage levels played a key role in the healing process (64). Some examples of nanoparticle-based wound dressing materials for which clinical trials are undertaken is listed in Table 2.
The major risk associated with patients affected with diabetes includes delayed wound healing and amputation. This is mainly due to the reduced tissue blood circulation causing hypoxia and the associated risks. A PVA/Chitosan-based nano fibre wound dressing was developed with high antimicrobial activity, improved vapour transmission rate, good odour-absorbing capacity and no cytotoxic effects; and was proved to accelerate the diabetic wound healing when tested in both diabetic and non-diabetic rats (72). A safe, cyto-compatible, epidermal growth factor-modified curcumin-incorporated chitosan nano-spray was developed that demonstrated accelerated wound healing properties, improved angiogenesis, and re-epithelialization with superior antibacterial effects in rats. It was further suggested that this nano-spray could help in the treatment of diabetic wounds and other skin injuries (73). A formulation composed of poly lactic acid/chitosan nanoscaffolds encapsulating cod liver oil was developed and characterized which demonstrated significant wound healing property to be used in the treatment of the most complicated disorder, diabetic foot ulcers, seen in diabetic patients (74). A topical formulation of lecithin-chitosan nanoparticles incorporated with melatonin was developed with desirable properties such as fibroblast induction, collagen deposition and promotion of angiogenesis. The formulation demonstrated4 accelerated wound closure in diabetic rats (75). A nanocomposite sponge comprised of chitosan, hyaluronic acid and nano-silver was developed against many antibiotic-resistant bacteria including methicillin-resistant S. aureus. The excellent antibacterial action exhibited by this nanocomposite sponge made it a suitable dressing agent for diabetic foot ulcers with mild toxicity towards mammalian cells (76). A hydrogel membrane composed of polyvinyl alcohol, starch, chitosan and nano zinc oxide was prepared and was found effective as a potent wound dressing agent in initial wound healing stages through in vivo studies in rats and exhibited wide-spectrum antibacterial action through in vitro studies (77). An injectable nanocomposite composed of curcumin, chitosan and alginate was identified as a promising wound dressing agent for wound recovery. The in vivo studies in rats demonstrated that the nano-curcumin based nanocomposite showed significant collagen deposition and epidermis re-epithelialization in wounds (78).
Although there is a gap in the translation of nanomedicine, the use of computer-aided analysis has become evident in this area. This has led researchers get a clear vision on the behaviour and application of nanoparticle- based therapy in reproductive biology (79), transporting drugs across biological barriers like the blood-brain barrier (80, 81). Another important aspect with respect to the design and use of nanotherapeutics is the toxicity; the understanding of which has been highly enhanced using computational biology (82). Several machine-learning approaches have been explored by the researchers that gives a magnified view of the interaction of the nanomaterial with the cells. This enables tailor-made and non-toxic application of nanomedicine to improve healthcare (83).
Several impeding factors in healing chronic wounds exist using conventional treatment methods. Novel strategies have been designed to overcome them using nanotechnology has proved to be promising. The advanced biomaterials developed with cellular and acellular scaffolds in conjunction with nanomaterials of suitable nature would prove to be an efficient wound care management in diabetic ulcers. The ability to modulate and control the release of active compounds and drugs has added advantage of controlling infection in these wounds significantly shortening the stay at the hospital for patients. An in-depth analysis of the factors that promote angiogenesis and wound closure at a faster rate by the use of nanocomposite biomaterials would help in translating these products to patients. Production of such tailor-made biomaterial constructs with specific factors and design would be the desired wound treatment strategy specifically in chronic wounds. With the recent advancements in the field of artificial intelligence the design of scaffolds could be customised to evade toxicity and meet the scrupulous needs that exist in regenerative therapy. This would help researchers validate and predict the outcome of their research without the sacrifice of many animals, avoid the strain of extraneous tasks involving the toxicological assessment. So, by means of integrating artificial intelligence and the lab-scale studies will yield effective translation of nanotherapeutics in wound care management.
RRR and DV conceptualized the manuscript and performed the literature search and AJ and SY drafted the manuscript. RR, DU and AS reviewed and modified the manuscript. All authors contributed to the article and approved the submitted version.
The authors declare that the research was conducted in the absence of any commercial or financial relationships that could be construed as a potential conflict of interest.
All claims expressed in this article are solely those of the authors and do not necessarily represent those of their affiliated organizations, or those of the publisher, the editors and the reviewers. Any product that may be evaluated in this article, or claim that may be made by its manufacturer, is not guaranteed or endorsed by the publisher.
AgNP, Silver Nanoparticle; PVA, Polyvinyl alcohol; HA, Hydroxyapatite; VEGF, Vascular Endothelial Growth Factor; CD31, Cluster of differentiation 31; CuNP, Copper nanoparticle; MnO2, Manganese dioxide; PCL, Polycaprolactone; ROS, Reactive Oxygen Secies; MMP, Matrix Metalloproteinases; MAPk, Mitogen-Activated Protein kinases.
1. Li Z, Yang Y, Wei H, Shan X, Wang X, Ou M, et al. Charge-reversal biodegradable MSNs for tumor synergetic chemo/photothermal and visualized therapy. J Control Release (2021) 338:719–30. doi: 10.1016/j.jconrel.2021.09.005
2. Guo S, DiPietro LA. Factors affecting wound healing. J Dent Res (2010) 89:219–29. doi: 10.1177/0022034509359125
3. Anderson K, Hamm RL. Factors that impair wound healing. J Am Coll Clin Wound Spec (2012) 4:84–91. doi: 10.1016/j.jccw.2014.03.001
4. Unnikrishnan R, Mohan V. Newer antidiabetic agents: At what price will they be cost effective? The Lancet Diabetes & Endocrinology (2021) 9(12):801–3. doi: 10.1016/S2213-8587(21)00264-3
5. Everett E, Mathioudakis N. Update on management of diabetic foot ulcers. Ann N Y Acad Sci (2018) 1411:153–65. doi: 10.1111/nyas.13569
6. Davison-Kotler E, Sharma V, Kang NV, García-Gareta E. A universal classification system of skin substitutes inspired by factorial design. Tissue Eng Part B Rev (2018) 24:279–88. doi: 10.1089/ten.teb.2017.0477
7. Richmond NA, Vivas AC, Kirsner RS. Topical and biologic therapies for diabetic foot ulcers. Med Clin (2013) 97:883–98. doi: 10.1016/j.mcna.2013.03.014
8. Kalashnikova I, Das S, Seal S. Nanomaterials for wound healing: Scope and advancement. Nanomedicine (2015) 10:2593–612. doi: 10.2217/nnm.15.82
9. Parani M, Lokhande G, Singh A, Gaharwar AK. Engineered nanomaterials for infection control and healing acute and chronic wounds. ACS Appl Mater Interfaces (2016) 8:10049–69. doi: 10.1021/acsami.6b00291
10. Mordorski B, Rosen J, Friedman A. Nanotechnology as an innovative approach for accelerating wound healing in diabetes. Diabetes Manag (2015) 5:329–33. doi: 10.2217/dmt.15.28
11. Awasthi A, Gulati M, Kumar B, Kaur J, Vishwas S, Khursheed R, et al. Recent progress in development of dressings used for diabetic wounds with special emphasis on scaffolds. BioMed Res Int (2022). doi: 10.1155/2022/1659338
12. Chaloupka K, Malam Y, Seifalian AM. Nanosilver as a new generation of nanoproduct in biomedical applications. Trends Biotechnol (2010) 28:580–8. doi: 10.1016/j.tibtech.2010.07.006
13. Salomoni R, Léo P, Montemor AF, Rinaldi BG, Rodrigues MFA. Antibacterial effect of silver nanoparticles in pseudomonas aeruginosa. Nanotechnol Sci Appl (2017) 10:115. doi: 10.2147/NSA.S133415
14. Gomaa EZ. Silver nanoparticles as an antimicrobial agent: A case study on staphylococcus aureus and escherichia coli as models for gram-positive and gram-negative bacteria. J Gen Appl Microbiol (2017) 63:36–43. doi: 10.2323/jgam.2016.07.004
15. Li Y, Xu T, Tu Z, Dai W, Xue Y, Tang C, et al. Bioactive antibacterial silica-based nanocomposites hydrogel scaffolds with high angiogenesis for promoting diabetic wound healing and skin repair. Theranostics (2020) 10:4929. doi: 10.7150/thno.41839
16. Liu X, Wong KKY. Application of nanomedicine in wound healing. In: Nasir A, Friedman A, Wang S (eds.) Nanotechnology in dermatology. Springer (2013). p. 221–9. doi: 10.1007/978-1-4614-5034-4_21
17. Chen S-A, Chen H-M, Yao Y-D, Hung C-F, Tu C-S, Liang Y-J. Topical treatment with anti-oxidants and au nanoparticles promote healing of diabetic wound through receptor for advance glycation end-products. Eur J Pharm Sci (2012) 47:875–83. doi: 10.1016/j.ejps.2012.08.018
18. Li J, Zhai D, Lv F, Yu Q, Ma H, Yin J, et al. Preparation of copper-containing bioactive glass/eggshell membrane nanocomposites for improving angiogenesis, antibacterial activity and wound healing. Acta Biomater (2016) 36:254–66. doi: 10.1016/j.actbio.2016.03.011
19. Zhai W, Lu H, Chen L, Lin X, Huang Y, Dai K, et al. Silicate bioceramics induce angiogenesis during bone regeneration. Acta Biomater (2012) 8:341–9. doi: 10.1016/j.actbio.2011.09.008
20. Weng L, Boda SK, Teusink MJ, Shuler FD, Li X, Xie J. Binary doping of strontium and copper enhancing osteogenesis and angiogenesis of bioactive glass nanofibers while suppressing osteoclast activity. ACS Appl Mater Interfaces (2017) 9:24484–96. doi: 10.1021/acsami.7b06521
21. Borkow G, Gabbay J, Dardik R, Eidelman AI, Lavie Y, Grunfeld Y, et al. Molecular mechanisms of enhanced wound healing by copper oxide-impregnated dressings. Wound Repair Regener (2010) 18:266–75. doi: 10.1111/j.1524-475X.2010.00573.x
22. Zhou W, Zi L, Cen Y, You C, Tian M. Copper sulfide nanoparticles-incorporated hyaluronic acid injectable hydrogel with enhanced angiogenesis to promote wound healing. Front Bioeng Biotechnol (2020) 8:417. doi: 10.3389/fbioe.2020.00417
23. Ranjbar-Mohammadi M, Rabbani S, Bahrami SH, Joghataei MT, Moayer F. Antibacterial performance and in vivo diabetic wound healing of curcumin loaded gum tragacanth/poly (ϵ-caprolactone) electrospun nanofibers. Mater Sci Eng C (2016) 69:1183–91. doi: 10.1016/j.msec.2016.08.032
24. Abdel-Mohsen AM, Jancar J, Abdel-Rahman RM, Vojtek L, Hyršl P, Dušková M, et al. A novel in situ silver/hyaluronan bio-nanocomposite fabrics for wound and chronic ulcer dressing: In vitro and in vivo evaluations. Int J Pharm (2017) 520:241–53. doi: 10.1016/j.ijpharm.2017.02.003
25. Zheng Z, Liu Y, Huang W, Mo Y, Lan Y, Guo R, et al. Neurotensin-loaded PLGA/CNC composite nanofiber membranes accelerate diabetic wound healing. Artif Cells Nanomed Biotechnol (2018) 46:493–501. doi: 10.1080/21691401.2018.1460372
26. Chen H, Jia P, Kang H, Zhang H, Liu Y, Yang P, et al. Upregulating hif-1α by hydrogel nanofibrous scaffolds for rapidly recruiting angiogenesis relative cells in diabetic wound. Adv Healthc Mater (2016) 5:907–18. doi: 10.1002/adhm.201501018
27. Augustine R, Dominic EA, Reju I, Kaimal B, Kalarikkal N, Thomas S. Electrospun polycaprolactone membranes incorporated with ZnO nanoparticles as skin substitutes with enhanced fibroblast proliferation and wound healing. Rsc Adv (2014) 4:24777–85. doi: 10.1039/c4ra02450h
28. Dewberry LC, Niemiec SM, Hilton SA, Louiselle AE, Singh S, Sakthivel TS, et al. Cerium oxide nanoparticle conjugation to microRNA-146a mechanism of correction for impaired diabetic wound healing. Nanomed Nanotechnol Biol Med (2022) 40:102483. doi: 10.1016/j.nano.2021.102483
29. Scherer SS, Pietramaggiori G, Matthews J, Perry S, Assmann A, Carothers A, et al. Poly-n-acetyl glucosamine nanofibers: A new bioactive material to enhance diabetic wound healing by cell migration and angiogenesis. Ann Surg (2009) 250:322–30. doi: 10.1097/SLA.0b013e3181ae9d45
30. Erba P, Adini A, Demcheva M, Valeri CR, Orgill DP. Poly-n-acetyl glucosamine fibers are synergistic with vacuum-assisted closure in augmenting the healing response of diabetic mice. J Trauma Acute Care Surg (2011) 71:S187–93. doi: 10.1097/TA.0b013e318225583c
31. Xu Z, Liu Y, Ma R, Chen J, Qiu J, Du S, et al. Thermosensitive hydrogel incorporating Prussian blue nanoparticles promotes diabetic wound healing via ROS scavenging and mitochondrial function restoration. ACS Appl Mater Interfaces (2022) 14:14059–71. doi: 10.1021/acsami.1c24569
32. Wang M, Wang C, Chen M, Xi Y, Cheng W, Mao C, et al. Efficient angiogenesis-based diabetic wound healing/skin reconstruction through bioactive antibacterial adhesive ultraviolet shielding nanodressing with exosome release. ACS Nano (2019) 13:10279–93. doi: 10.1021/acsnano.9b03656
33. Liang Y, Li M, Yang Y, Qiao L, Xu H, Guo B. pH/glucose dual responsive metformin release hydrogel dressings with adhesion and self-healing via dual-dynamic bonding for athletic diabetic foot wound healing. ACS Nano (2022) 16:3194–207. doi: 10.1021/acsnano.1c11040
34. Wang Y, Wu Y, Long L, Yang L, Fu D, Hu C, et al. Inflammation-responsive drug-loaded hydrogels with sequential hemostasis, antibacterial, and anti-inflammatory behavior for chronically infected diabetic wound treatment. ACS Appl Mater Interfaces (2021) 13:33584–99. doi: 10.1021/acsami.1c09889
35. Jiang Y, Zhang X, Zhang W, Wang M, Yan L, Wang K, et al. Infant skin friendly adhesive hydrogel patch activated at body temperature for bioelectronics securing and diabetic wound healing. ACS Nano (2022), 16:8662–76. doi: 10.1021/acsnano.2c00662
36. Bhattacharya D, Ghosh B, Mukhopadhyay M. Development of nanotechnology for advancement and application in wound healing: A review. IET Nanobiotechnol (2019) 13:778–85. doi: 10.1049/iet-nbt.2018.5312
37. Khorasani MT, Joorabloo A, Moghaddam A, Shamsi H, MansooriMoghadam Z. Incorporation of ZnO nanoparticles into heparinised polyvinyl alcohol/chitosan hydrogels for wound dressing application. Int J Biol Macromol (2018) 114:1203–15. doi: 10.1016/j.ijbiomac.2018.04.010
38. Bianchi SE, Machado BEK, da Silva MGC, da Silva MMA, Dal Bosco L, Marques MS, et al. Coumestrol/hydroxypropyl-β-cyclodextrin association incorporated in hydroxypropyl methylcellulose hydrogel exhibits wound healing effect: In vitro and in vivo study. Eur J Pharm Sci (2018) 119:179–88. doi: 10.1016/j.ejps.2018.04.019
39. Hsu L, Peng B, Chen M, Thalib B, Ruslin M, Tung TDX, et al. The potential of the stem cells composite hydrogel wound dressings for promoting wound healing and skin regeneration: in vitro and in vivo evaluation. J BioMed Mater Res Part B Appl Biomater (2019) 107:278–85. doi: 10.1002/jbm.b.34118
40. Durão J, Vale N, Gomes S, Gomes P, Barrias CC, Gales L. Nitric oxide release from antimicrobial peptide hydrogels for wound healing. Biomolecules (2018) 9:4. doi: 10.3390/biom9010004
41. Khorasani MT, Joorabloo A, Adeli H, Mansoori-Moghadam Z, Moghaddam A. Design and optimization of process parameters of polyvinyl (alcohol)/chitosan/nano zinc oxide hydrogels as wound healing materials. Carbohydr Polym (2019) 207:542–54. doi: 10.1016/j.carbpol.2018.12.021
42. Zhu C, Lei H, Fan D, Duan Z, Li X, Li Y, et al. Novel enzymatic crosslinked hydrogels that mimic extracellular matrix for skin wound healing. J Mater Sci (2018) 53:5909–28. doi: 10.1007/s10853-017-1956-y
43. Nathan DM. Diabetes: Advances in diagnosis and treatment. Jama (2015) 314:1052–62. doi: 10.1001/jama.2015.9536
44. Mudge EJ. Recent accomplishments in wound healing. Int Wound J (2015) 12:4–9. doi: 10.1111/iwj.12230
45. Castleberry SA, Almquist BD, Li W, Reis T, Chow J, Mayner S, et al. Self-assembled wound dressings silence MMP-9 and improve diabetic wound healing in vivo. Adv Mater (2016) 28:1809–17. doi: 10.1002/adma.201503565
46. Lim JZM, Ng NSL, Thomas C. Prevention and treatment of diabetic foot ulcers. J R Soc Med (2017) 110:104–9. doi: 10.1177/0141076816688346
47. Laiva AL, O’Brien FJ, Keogh MB. Innovations in gene and growth factor delivery systems for diabetic wound healing. J Tissue Eng Regener Med (2018) 12:e296–312. doi: 10.1002/term.2443
48. Yan W, Liu H, Deng X, Jin Y, Wang N, Chu J. Acellular dermal matrix scaffolds coated with connective tissue growth factor accelerate diabetic wound healing by increasing fibronectin through PKC signalling pathway. J Tissue Eng Regener Med (2018) 12:e1461–73. doi: 10.1002/term.2564
49. Xi Y, Ge J, Guo Y, Lei B, Ma PX. Biomimetic elastomeric polypeptide-based nanofibrous matrix for overcoming multidrug-resistant bacteria and enhancing full-thickness wound healing/skin regeneration. ACS Nano (2018) 12:10772–84. doi: 10.1021/acsnano.8b01152
50. Mir M, Ali MN, Barakullah A, Gulzar A, Arshad M, Fatima S, et al. Synthetic polymeric biomaterials for wound healing: A review. Prog Biomater (2018) 7:1–21. doi: 10.1007/s40204-018-0083-4
51. Xi Y, Ge J, Wang M, Chen M, Niu W, Cheng W, et al. Bioactive anti-inflammatory, antibacterial, antioxidative silicon-based nanofibrous dressing enables cutaneous tumor photothermo-chemo therapy and infection-induced wound healing. ACS Nano (2020) 14:2904–16. doi: 10.1021/acsnano.9b07173
52. Zhou L, Xi Y, Xue Y, Wang M, Liu Y, Guo Y, et al. Injectable self-healing antibacterial bioactive polypeptide-based hybrid nanosystems for efficiently treating multidrug resistant infection, skin-tumor therapy, and enhancing wound healing. Adv Funct Mater (2019) 29:1806883. doi: 10.1002/adfm.201806883
53. Lee Y-H, Chang J-J, Chien C-T, Yang M-C, Chien H-F. Antioxidant sol-gel improves cutaneous wound healing in streptozotocin-induced diabetic rats. Exp Diabetes Res (2012). doi: 10.1155/2012/504693
54. Han L, Wang M, Li P, Gan D, Yan L, Xu J, et al. Mussel-inspired tissue-adhesive hydrogel based on the polydopamine–chondroitin sulfate complex for growth-factor-free cartilage regeneration. ACS Appl Mater Interfaces (2018) 10:28015–26. doi: 10.1021/acsami.8b05314
55. Saldin LT, Cramer MC, Velankar SS, White LJ, Badylak SF. Extracellular matrix hydrogels from decellularized tissues: Structure and function. Acta Biomater (2017) 49:1–15. doi: 10.1016/j.actbio.2016.11.068
56. Zhao X, Sun X, Yildirimer L, Lang Q, Lin ZYW, Zheng R, et al. Cell infiltrative hydrogel fibrous scaffolds for accelerated wound healing. Acta Biomater (2017) 49:66–77. doi: 10.1016/j.actbio.2016.11.017
57. Naahidi S, Jafari M, Logan M, Wang Y, Yuan Y, Bae H, et al. Biocompatibility of hydrogel-based scaffolds for tissue engineering applications. Biotechnol Adv (2017) 35:530–44. doi: 10.1016/j.biotechadv.2017.05.006
58. Zhang YS, Khademhosseini A. Advances in engineering hydrogels. Science (2017) 356:eaaf3627. doi: 10.1126/science.aaf3627
59. Tavakoli S, Mokhtari H, Kharaziha M, Kermanpur A, Talebi A, Moshtaghian J. A multifunctional nanocomposite spray dressing of kappa-carrageenan-polydopamine modified ZnO/L-glutamic acid for diabetic wounds. Mater Sci Eng C (2020) 111:110837. doi: 10.1016/j.msec.2020.110837
60. Villalba-Rodríguez AM, Martínez-González S, Sosa-Hernández JE, Parra-Saldívar R, Bilal M, Iqbal HMN. Nanoclay/Polymer-based hydrogels and enzyme-loaded nanostructures for wound healing applications. Gels (2021) 7:59. doi: 10.3390/gels7020059
61. Huang K-T, Fang Y-L, Hsieh P-S, Li C-C, Dai N-T, Huang C-J. Zwitterionic nanocomposite hydrogels as effective wound dressings. J Mater Chem B (2016) 4:4206–15. doi: 10.1039/C6TB00302H
62. Zhu S, Dai Q, Yao L, Wang Z, He Z, Li M, et al. Engineered multifunctional nanocomposite hydrogel dressing to promote vascularization and anti-inflammation by sustained releasing of Mg2+ for diabetic wounds. Compos Part B Eng (2022) 231:109569. doi: 10.1016/j.compositesb.2021.109569
63. Pu Y, Wang P, Yang R, Tan X, Shi T, Ma J, et al. Bio-fabricated nanocomposite hydrogel with ROS scavenging and local oxygenation accelerates diabetic wound healing. J Mater Chem B (2022), 10:4083–95. doi: 10.1039/D2TB00343K
64. Fan Y, Wu W, Lei Y, Gaucher C, Pei S, Zhang J, et al. Edaravone-loaded alginate-based nanocomposite hydrogel accelerated chronic wound healing in diabetic mice. Mar Drugs (2019) 17:285. doi: 10.3390/md17050285
65. Yahia EA, El-Sharkawey AE, Bayoumi MM. Quantitative evaluation of diabetic foot wound healing using hydrogel nanosilver based dressing vs. traditional dressing: A prospective randomized control study. Pak J Med Heal Sci (2021) 15:1571–4. doi: 10.53350/pjmhs211561571
66. Koivuniemi R, Hakkarainen T, Kiiskinen J, Kosonen M, Vuola J, Valtonen J, et al. Clinical study of nanofibrillar cellulose hydrogel dressing for skin graft donor site treatment. Adv Wound Care (2020) 9:199–210. doi: 10.1089/wound.2019.0982
67. Adhya A, Bain J, Ray O, Hazra A, Adhikari S, Dutta G, et al. Healing of burn wounds by topical treatment: A randomized controlled comparison between silver sulfadiazine and nano-crystalline silver. J Basic Clin Pharm (2014) 6:29. doi: 10.4103/0976-0105.145776
68. Krieger BR, Davis DM, Sanchez JE, Mateka JJL, Nfonsam VN, Frattini JC, et al. The use of silver nylon in preventing surgical site infections following colon and rectal surgery. Dis Colon Rectum (2011) 54:1014–9. doi: 10.1097/DCR.0b013e31821c495d
69. Silver GM, Robertson SW, Halerz MM, Conrad P, Supple KG, Gamelli RL. A silver-coated antimicrobial barrier dressing used postoperatively on meshed autografts: A dressing comparison study. J Burn Care Res (2007) 28:715–9. doi: 10.1097/BCR.0B013E318148C9E4
70. Oram Y, Kahraman F, Karιncaoğlu Y, Koyuncu E. Evaluation of 60 patients with pilonidal sinus treated with laser epilation after surgery. Dermatol Surg (2010) 36:88–91. doi: 10.1111/j.1524-4725.2009.01387.x
71. Miller CN, Newall N, Kapp SE, Lewin G, Karimi L, Carville K, et al. A randomized-controlled trial comparing cadexomer iodine and nanocrystalline silver on the healing of leg ulcers. Wound Repair Regener (2010) 18:359–67. doi: 10.1111/j.1524-475X.2010.00603.x
72. Majd SA, Khorasgani MR, Moshtaghian SJ, Talebi A, Khezri M. Application of Chitosan/PVA nano fiber as a potential wound dressing for streptozotocin-induced diabetic rats. Int J Biol Macromol (2016) 92:1162–8. doi: 10.1016/j.ijbiomac.2016.06.035
73. Li Y, Leng Q, Pang X, Shi H, Liu Y, Xiao S, et al. Therapeutic effects of EGF-modified curcumin/chitosan nano-spray on wound healing. Regener Biomater (2021) 8:rbab009. doi: 10.1093/rb/rbab009
74. Khazaeli P, Alaei M, Khaksarihadad M, Ranjbar M. Preparation of PLA/chitosan nanoscaffolds containing cod liver oil and experimental diabetic wound healing in male rats study. J Nanobiotechnol (2020) 18:1–9. doi: 10.1186/s12951-020-00737-9
75. Correa VLR, Martins JA, de Souza TR, Rincon G de CN, Miguel MP, de Menezes LB, et al. Melatonin loaded lecithin-chitosan nanoparticles improved the wound healing in diabetic rats. Int J Biol Macromol (2020) 162:1465–75. doi: 10.1016/j.ijbiomac.2020.08.027
76. Anisha BS, Biswas R, Chennazhi KP, Jayakumar R. Chitosan–hyaluronic acid/nano silver composite sponges for drug resistant bacteria infected diabetic wounds. Int J Biol Macromol (2013) 62:310–20. doi: 10.1016/j.ijbiomac.2013.09.011
77. Baghaie S, Khorasani MT, Zarrabi A, Moshtaghian J. Wound healing properties of PVA/starch/chitosan hydrogel membranes with nano zinc oxide as antibacterial wound dressing material. J Biomater Sci Polym Ed (2017) 28:2220–41. doi: 10.1080/09205063.2017.1390383
78. Li X, Chen S, Zhang B, Li M, Diao K, Zhang Z, et al. In situ injectable nano-composite hydrogel composed of curcumin, n, O-carboxymethyl chitosan and oxidized alginate for wound healing application. Int J Pharm (2012) 437:110–9. doi: 10.1016/j.ijpharm.2012.08.001
79. Chandrasekar V, Singh AV, Maharjan RS, Dakua SP, Balakrishnan S, Dash S, et al. Perspectives on the technological aspects and biomedical applications of virus-like Particles/Nanoparticles in reproductive biology: Insights on the medicinal and toxicological outlook. Adv NanoBiomed Res (2022), 2200010. doi: 10.1002/anbr.202200010
80. Singh AV, Chandrasekar V, Janapareddy P, Mathews DE, Laux P, Luch A, et al. Emerging application of nanorobotics and artificial intelligence to cross the BBB: Advances in design, controlled maneuvering, and targeting of the barriers. ACS Chem Neurosci (2021) 12:1835–53. doi: 10.1021/acschemneuro.1c00087
81. Singh AV, Chandrasekar V, Laux P, Luch A, Dakua SP, Zamboni P, et al. Micropatterned neurovascular interface to mimic the blood–brain barrier’s neurophysiology and micromechanical function: A BBB-on-CHIP model. Cells (2022) 11:2801. doi: 10.3390/cells11182801
82. Singh AV, Ansari MHD, Rosenkranz D, Maharjan RS, Kriegel FL, Gandhi K, et al. Artificial intelligence and machine learning in computational nanotoxicology: Unlocking and empowering nanomedicine. Adv Healthc Mater (2020) 9:1901862. doi: 10.1002/adhm.201901862
Keywords: nanocomposites, scaffolds, hydrogen-based scaffolds, Chitosan-based scaffolds, diabetic wound healing
Citation: Renuka RR, Julius A, Yoganandham ST, Umapathy D, Ramadoss R, Samrot AV and Vijay DD (2023) Diverse nanocomposites as a potential dressing for diabetic wound healing. Front. Endocrinol. 13:1074568. doi: 10.3389/fendo.2022.1074568
Received: 19 October 2022; Accepted: 16 December 2022;
Published: 11 January 2023.
Edited by:
Ramkumar Kunka Mohanram, SRM Institute of Science and Technology, IndiaReviewed by:
Sachin Kumar Singh, Lovely Professional University, IndiaCopyright © 2023 Renuka, Julius, Yoganandham, Umapathy, Ramadoss, Samrot and Vijay. This is an open-access article distributed under the terms of the Creative Commons Attribution License (CC BY). The use, distribution or reproduction in other forums is permitted, provided the original author(s) and the copyright owner(s) are credited and that the original publication in this journal is cited, in accordance with accepted academic practice. No use, distribution or reproduction is permitted which does not comply with these terms.
*Correspondence: Remya Rajan Renuka, cmVteWEucHJhdmVlbjVAZ21haWwuY29t; Danis D. Vijay, ZGFuaXNodmlqYXlAa2ltcy5lZHUuaW4=
Disclaimer: All claims expressed in this article are solely those of the authors and do not necessarily represent those of their affiliated organizations, or those of the publisher, the editors and the reviewers. Any product that may be evaluated in this article or claim that may be made by its manufacturer is not guaranteed or endorsed by the publisher.
Research integrity at Frontiers
Learn more about the work of our research integrity team to safeguard the quality of each article we publish.