- 1Department of Pediatrics, University of Chieti, Chieti, Italy
- 2Paediatric Outpatient Department, Moscow, Russia
In our modern society, where highly palatable and calorie-rich foods are readily available, and sedentary lifestyle is common among children and adolescents, we face the pandemic of obesity, nonalcoholic fatty liver disease, hypertension, atherosclerosis, and T2D. Insulin resistance (IR) is known to be the main underlying mechanism of all these associated health consequences; therefore, the early detection of IR is fundamental for preventing them.A Consensus Statement, internationally supported by all the major scientific societies in pediatric endocrinology, was published in 2010, providing all the most recent reliable evidence to identify the definition of IR in children, its measurement, its risk factors, and the effective strategies to prevent and treat it. However, the 2010 Consensus concluded that further research was necessary to assess some of the discussed points, in particular the best way to measure insulin sensitivity, standardization of insulin measurements, identification of strong surrogate biomarkers of IR, and the effective role of lifestyle intervention and medications in the prevention and treatment of IR.The aim of this review is to update each point of the consensus with the most recent available studies, with the goal of giving a picture of the current state of the scientific literature regarding IR in children, with a particular regard for issues that are not yet fully clarified.
Insulin resistance refers to reduced whole body glucose uptake
Intake of carbohydrates and, therefore, elevated blood glucose levels lead to an activation of complex regulatory pathways, which are responsible for the clearance of glucose from the bloodstream and homeostatic control of blood glucose. The increased secretion of insulin by pancreatic beta cells is a direct response leading to enhanced glucose transport into peripheral tissues (1–3). Insulin resistance (IR) is the inverse state of insulin sensitivity (IS) (4) and the relative unresponsiveness of peripheral tissues to the effects of the hormone (2, 5). It can be described as impaired signal transduction and biological actions in response to increasing amounts of insulin in the circulation (6).
Peripheral IR contributes to the impairment of glucose disposition and IGT (7). IR is associated with reduced insulin-stimulated glucose utilization (8), primarily by the skeletal muscle and adipose tissue (1, 2, 4). In patients with type 2 diabetes (T2D), muscle IR is responsible for 85%–90% weakened total body glucose disposal (7).
IR is developed during some physiological conditions, such as pregnancy or puberty, as well as during intercurrent infections (8).
Insulin resistance is a continuum
IR is a continuum from the stage of impaired IS to impaired glucose tolerance, followed by T2D (4, 7, 9). It is the earliest metabolic abnormality of T2D (7).
Insulin resistance is commonly associated with obesity
The prevalence of IR is higher in overweight and obese children compared with normal-weight children (10).
Obesity is characterized by an excessive accumulation of adipose tissue in the body (3, 11). IR is the main factor for many of the complications of obesity (11). According to the World Health Organization (WHO), the number of obese children and adolescents has increased tenfold over the past four decades (12).
One of the consequences of insulin resistance is chronic compensatory hyperinsulinemia
In patients with IR, greater concentrations of insulin are needed to achieve the same effects that were earlier elicited by lower hormone levels (13). Primarily, pancreatic beta cells try to compensate metabolic dysregulated processes by increasing the secretion of insulin (8, 9, 14), but chronic hyperinsulinemia may play the crucial role in the pathology and progression of metabolic disorders (15). Hyperinsulinemia is accompanied by increased appetite and body weight gain (9). Ultimately, the pancreatic beta cell function declines because of glucolipotoxicity and genetic factors (14), and the secretion of insulin becomes insufficient (8, 9).
Standards for insulin resistance in children, with definitions for normal and abnormal levels, are nonexistent
There is no standard definition of IS/IR in children (4, 13, 15, 16). The assessment of IS requires accurate measurement of insulin (17). However, there is not any standardized technique for the measurement of plasma insulin, and that makes it difficult to compare results between different laboratories (13).
Van der Aa et al. conducted a systematic review of all population-based studies reporting on the incidence of IR in children. In 18 population-based studies, six different methods were used to determine IR: homeostasis model assessment insulin resistance (HOMA-IR), fasted plasma insulin (FPI), the quantitative insulin sensitivity check index (QUICKI), the fasted glucose/insulin ratio (FGIR), HOMA2, and the McAuley index. The cutoff values used to define IR also varied (10). There is also no agreement regarding the necessity of using the age-adjusted cutoff points when determining IR in children (15, 18).
The euglycemic hyperinsulinemic clamp is the “gold standard” for measuring insulin sensitivity; the frequently sampled IV glucose tolerance test and steady-state plasma glucose methods are also valid measurements
The HE-clamp, proposed by Andres et al. in 1966 and developed by DeFronzo et al. in 1979 (19), is the gold standard for the measurement of IS. This method evaluates insulin-mediated glucose utilization under steady-state conditions (20). However, the clamp method is invasive, time-consuming, and expensive, and, therefore, it cannot be used routinely in clinical practice (21).
In 1979, Bergman et al. proposed the minimal model methodology as a simple alternative to the HE-clamp. During the frequently sampled intravenous glucose tolerance test (FSIGT1), the dynamics of glucose and insulin are analyzed via a computer (22). Further studies showed that the IS index derived by the minimal model reliably correlates with the results of the clamp method (23).
The homeostasis model assessment and the quantitative insulin-sensitivity check index do not offer any advantages over fasting insulin in euglycemic children
As the clamp method is not feasible on a large scale, several surrogate tools have been developed to evaluate IS. The most widely used surrogate markers are fasting surrogate indices such as fasting plasma insulin (FPI), HOMA-IR, and QUICKI (24). Several studies showed high correlation between these markers in children (15, 25–27).
George et al. evaluated the correlations between the clamp-measured IS and the surrogate estimates in 188 obese and overweight adolescents with normal glucose tolerance, prediabetes, and diabetes. The results showed that fasting indices correlated significantly with clamp IS. OGTT-derived surrogates did not demonstrate any advantage over the fasting indices when compared with clamp methodology. Furthermore, HOMA or QUICKI did not show any advantage over the 1/IF when compared with the clamp-method results (25).
Schwartz et al. compared the surrogate measures of IR (fasting insulin, HOMA, QUICKI, and FGIR) with the insulin clamp measure of IR in a large cohort of adolescents. The results showed that other surrogate measures seem not to have any advantage over fasting insulin (26).
Fasting insulin is a poor measure of whole-body insulin sensitivity in an individual child
Despite the aforementioned, fasting insulin is a poor marker of IS, as it does not always correlate well in children with the gold-standard clamp-measured IS (26, 27). This index may reveal compensatory hyperinsulinemia but could not be used as a marker of IR in a large clinical practice (24).
The two most important biological conditions associated with insulin resistance in childhood are ethnicity and puberty
Transient reduction in whole-body IS and a temporary increase in IR are observed during puberty (7–9, 13, 28). During puberty, IS decreases by 25%–50% and recovers when pubertal development ends (29). Impaired IS during puberty is one of the main factors increasing the frequency of glucose metabolism disorders (30). The changes in hormonal profile during puberty are well known to affect IS, but the exact mechanisms of this are not fully understood so far (28). Increased growth hormone and IGF-1 secretion, accompanied by increased lipid breakdown and increased free fatty acids (FFAs) in the circulation, as well as rising concentrations of the sex hormone, contribute in developing IR during puberty (7, 28).
Another biological condition strongly associated with IR in children is race/ethnicity. Several studies compared IS in different ethnic groups and found ethnicity being an important risk factor for IR in children. It has been demonstrated that Hispanic, African-American, Asian, and Pima Indian children are more insulin-resistant compared with white children (31–33).
Based on current screening criteria and methodology, there is no justification for screening children for insulin resistance, including obese children
It is well known that IR in children and adolescents may predict cardiovascular risk in the future (34). A strong association between IR and several chronic diseases makes the screening program for an early identification of at-risk children highly important. At the same time, the reliable methods of the measurement of IR are not available tools in a large clinical practice. There is no agreement regarding a uniform definition and cutoff value of IR (10), as well as no recommended pharmacological treatment for isolated IR in children (4); that is why screening programs for IR in children do not exist even in obese patients (4, 35).
Obesity, particularly increased abdominal visceral adiposity, and nonalcoholic fatty liver disease are associated with insulin resistance in children
Nonalcoholic fatty liver disease (NAFLD) is associated with IR in children (4) and is the hepatic manifestation of metabolic syndrome (MetS) (36). It is the most common pediatric liver disease (37). NAFLD is the fatty infiltration of the liver in the absence of other causes of liver damage, such as genetic/metabolic disorders, alcohol consumption, infections, medications, or malnutrition (38). The pathogenesis of NAFLD is multicomponent (2, 39, 40). Hepatic triglyceride accumulation leads to steatosis (2). Excessive hepatic FFA accumulation or ‘lipotoxicity’ causes the activation of intracellular signaling pathways. Further hepatocellular damage is mediated by multiple factors: altered gut flora, stellate cell activation, increased mitochondrial permeability, adipocytokines, apoptosis, etc. (39, 40). IR plays a crucial role in the pathogenesis of NAFLD by facilitating lipogenesis in hepatocytes, impairing the inhibition of lipolysis, and stimulating the secretion of adipokines and other cytokines (40).
Polycystic ovary syndrome, independent of weight, is characterized by insulin resistance in childhood
PCOS is a heterogeneous disorder. The diagnostic criteria for adolescent girls of PCOS are menstrual irregularity, hyperandrogenemia, and/or clinical features of hyperandrogenism (41). PCOS is often associated with IR. The severity of IR is more pronounced in obese patients than in lean ones, both in adolescent girls (42, 43) and in women (44).
Genetics and heritability play a role in childhood insulin resistance
Genome-wide association studies (GWASs) have identified several loci associated with IR (Table 1) (60).
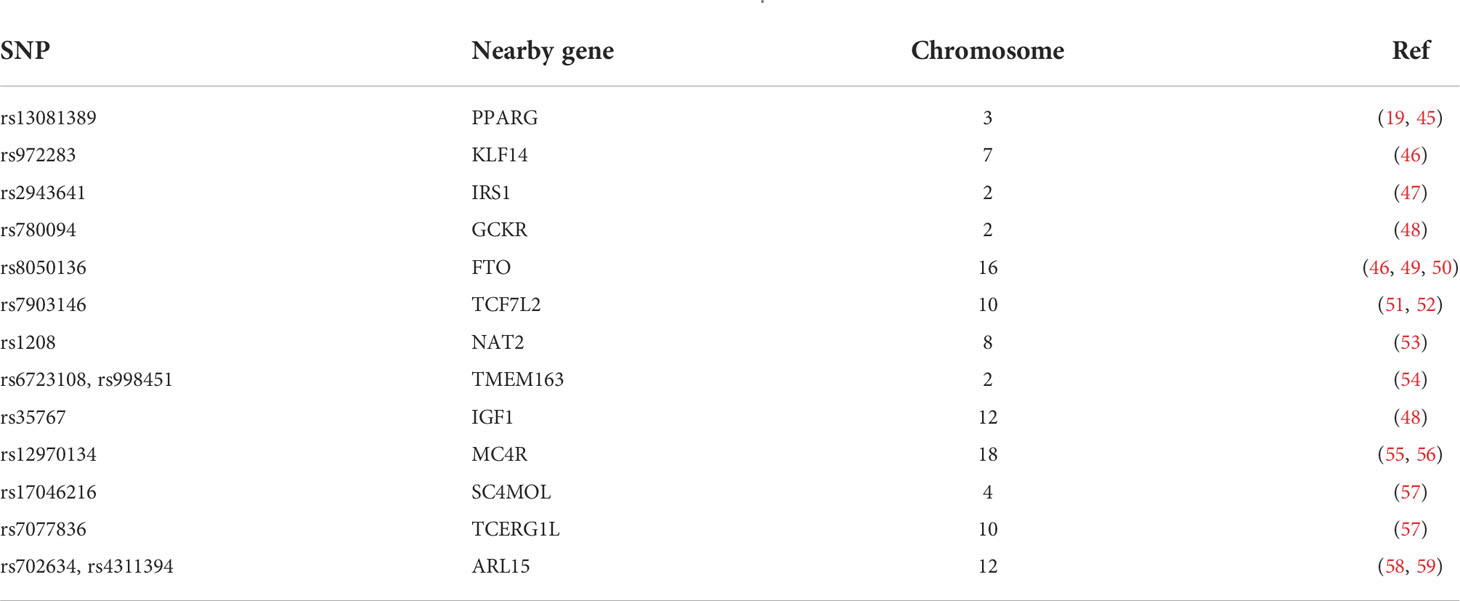
Table 1 Variants associated with IR. Adapted from Brown and Yanovski (17).
The PPARγ (peroxisome proliferator-activated receptor gamma) variant Pro12Ala was first shown to be associated with a decreased risk of developing T2D (19, 45). rs972283 near KLF14 (kruppel-like factor 14) was identified through large-scale association analysis of a European cohort (46) associated with reduced insulin action (61). IRS1 (insulin receptor substrate 1) is a fundamental component of the insulin signaling pathway initiating the activation of PI3K in response to insulin (47). GCKR (glucokinase regulator) encodes glucokinase regulatory protein (GKRP), which inhibits the activity of glucokinase, an enzyme involved in the regulation of hepatic glucose disposal and storage (62). Studies on TCF7L2 (transcription factor 7-like 2) have not demonstrated its role in insulin-dependent tissues despite the most consistent association with the risk of developing T2D of any gene variants identified so far (51, 52, 63, 64). Moreover, a variant in NAT2 (N-acetyltransferase 2) was recently found to be a direct measure of IS (53).
Two loci near TMEM163 (transmembrane protein 163) were demonstrated to be associated with reduced plasma insulin and HOMA-IR (65). Low plasma levels of IGF-1 are known to be associated with a reduction in IS (54), and analysis of the rs35767 polymorphism indicates that carriers of the G allele have lower circulating levels of IGF-1 compared with A allele carriers (66). MC4R (melanocortin 4 receptor) was shown in a GWAS of a UK cohort of Indian-Asian and European ancestry to be associated with both IR and waist circumference, with higher risk-allele frequencies being found in the Indian-Asian cohort (67).
A GWAS of an African American cohort identified an SNP near TCERG1L (transcription elongation regulator 1-like) and one near SC4MOL (sterol-C4- methyl oxidase-like) as being associated with both fasting insulin and HOMA-IR; this finding was also present in a West African cohort (55). The exact function of ARL15 (ADP ribosylation factor like GTPase 15), involved in intracellular vesicle trafficking, is still unknown. Variants of these genes are associated with decreased adiponectin levels and associated with the risk of T2D, coronary heart disease, and higher HOMA-IR (57).
Intrauterine exposure to poorly controlled maternal diabetes increases the risk of obesity, insulin resistance, and IGT in childhood
The offspring risk of T2D is more closely associated with maternal than paternal diabetes (68). The risk of T2D in the offspring is strongly associated with the diagnosis of diabetes in mothers before they reach the age of 50. This could be due both to genetic transmission of diabetes susceptibility and the intrauterine environment. In fact, women with GDM have been shown to have increased serum leukocyte count and levels of inflammatory cytokines, including interleukin (IL)-6 and tumor necrosis factor (TNF)-α, in comparison with control subjects. Exposure to a proinflammatory environment within the uterus may increase the fetus diabetes risk by influencing the fetal epigenome at this early stage; however, more studies are needed (69).
By now, there are no accepted treatments for GDM except for lifestyle intervention and insulin therapy in selected cases, whose effectiveness is limited due to frequent IR. Results regarding glyburide and metformin are promising; however, their long-term safety needs to be deepened (70).
Postnatal and childhood weight gain increase the risk of insulin resistance in normal-birth-weight and small-for-gestational-age children
It is well known that postnatal weight gain is associated with IR risk and greater adiposity in children and young adults (4, 71–75) and predicts IR-related outcomes in adults (76, 77).
Ong et al. (78) showed that rapid weight gain from age 0 to 2 years, whether with or without prior fetal growth deceleration, was associated with increased HOMA-IR and systolic and diastolic blood pressure, manifesting as early as 3 years of age.
Adolescents are another category at risk of developing IR (7) because, during puberty, there is a surge in growth hormone and insulin-like growth factor-I (IGF-1), which causes IR increase through increased lipid breakdown and increased FFAs (79). Girls are more likely to develop T2D than boys (80): estrogen overstimulates insulin receptors on β-cells resulting in excessive insulin signaling and β-cell exhaustion (81). Obese children who develop T2D around puberty and continue to gain weight have a higher risk of persistent IR even post-puberty.
Insulin resistance is a risk factor for prediabetes and T2D in childhood
According to Consensus 2020, IR and impaired-cell function are the two key components in the pathogenesis of T2D in youth (4). To support this statement, Kim et al. (82) conducted a cross-sectional study on 205 youth investigating the adipose IR index (adipose-IR) (calculated as fasting insulin × free fatty acids [FFAs]) across the spectrum from normal glucose tolerance (NGT) to IGT to T2D and the predictive power of adipose-IR for determining dysglycemia in youth. What emerged is that adipose-IR increases progressively along the spectrum of glucose tolerance from NGT to IGT to T2D and that adipose-IR is a significant predictor of dysglycemia.
Insulin resistance is associated with the metabolic syndrome and cardiometabolic risk factors
IR is one of the main elements that define the MetS (83) and contribute to cardiovascular risk. Recently, some studies have directly demonstrated the association between IR and atherosclerotic abnormalities in children. Miniello et al. (84) showed a clear association between increased HOMA-IR and impaired endothelial function since childhood, correlating HOMA-IR with flow-mediated dilatation, carotid intima-media thickness, and the anteroposterior diameter of infrarenal abdominal aorta in children.
Moreover, the results from the Pune Children’s Study suggest that prepubertal glucose-insulin metabolism is associated with high markers of atherosclerosis in adulthood (85).
Diet and weight loss drugs improve insulin sensitivity in adolescents through weight loss and other mechanisms
Although diet is well known to affect IS, it is unclear what dietary macronutrient composition could contribute more to the development of IR (86, 87).
High fiber intake contributes to reducing body weight and improving IS by slow gastric emptying and absorption of dietary carbohydrates and fats, increased satiety, and regulatory effect on inflammatory markers and gut hormones (88–90).
Fat intake is associated with lower IS, and this association in adolescents seems to be independent of body fat (91). Arslanian et al. demonstrated that increased fat/carbohydrate ratio is negatively correlated with IS and insulin clearance also in prepubertal children (32). In addition, in non-obese adolescents, consumption of a high-fat/low-carbohydrate diet, even during a short time (7 days), was shown to impair IS (92). Regarding the fat quality, a consistent differential effect has not been found (93).
IS could be impaired also by high-glycemic-index food intake (94); however, data regarding the efficacy of low-glycemic-index diet in reducing IR are controversial (95).
Exercise and fitness improve insulin sensitivity through weight loss and also mechanisms independent of weight loss in adolescents
Exercise contributes to the improvement of IS through other mechanisms besides the effect of weight loss (88, 96–99). Randomized studies have clearly shown the beneficial effect of physical activity on fasting insulin levels in overweight children before weight loss was documented (100, 101).
Regarding the type of exercise to recommend, several studies have demonstrated significant beneficial effects of both aerobic and combined aerobic and resistance exercises in overweight children independently of changes in body weight and percent body fat (102–105). However, data regarding the efficiency of resistance training on IR in children and adolescents are debatable (106, 107).
Multicomponent lifestyle intervention improves insulin sensitivity more than individual lifestyle components in adolescents
A multicomponent lifestyle approach could be more effective in improving IS than a simpler approach (88, 108, 109). Dietary intake, physical activity, and sleeping habits can affect IS, and each is individually linked to IR (110). Androutsos et al. (110) demonstrated that a lifestyle pattern consisting of high moderate-vigorous physical activity and frequent eating habit was inversely related to HOMA-IR. Patterns combining different lifestyle behaviors may be more practical for translating into recommendations and thus more useful for public health.
Metformin improves insulin sensitivity in adolescence
Metformin reduces IR by decreasing fasting plasma glucose and insulin concentrations in adults. Moreover, it is an insulin sensitizer and likely acts in the gut lumen through multiple mechanisms. In non-diabetic obese adults, it reduces food intake, determining weight loss and the reduction of fasting plasma glucose, cholesterol, and insulin concentrations. Furthermore, metformin has been shown to improve the BMI, body fat composition, fasting glucose, insulin, glycated hemoglobin (HbA1c), IR expressed by the HOMA-IR, blood pressure, and lipid profile in short trials on small sample sizes of children and adolescents (111).
Metformin is approved by the US Food and Drug Administration (FDA) for the treatment of T2D in 10-year-old or older children, and it is the only treatment evaluated in children with prediabetes.
The 2017 Pediatric Obesity Clinical Guidelines by The Endocrine Society recommend using it only in selected patients, since its long-term benefits in insulin-resistant children are still to be analyzed (112).
Maternal obesity, gestational diabetes, and smoking in pregnancy should be targeted to lessen obesity and insulin resistance in children
Infants of women with gestational diabetes (GDM) have an increased risk of becoming overweight or obese during adolescence and are more likely to develop type II diabetes later in life. Furthermore, according to several studies, epigenetic alterations of different genes of the fetus of a GDM mother in utero could result in the transgenerational transmission of GDM and type II diabetes (113).
A cross-sectional study conducted on 976 children and adolescents showed a significant association between maternal weight and cigarette consumption during pregnancy and the subsequent development of MetS in children (114).
Given these promises, the reinforcement of screening and prevention methods is a must.
Breast-feeding should be promoted through public health interventions as a contributing factor to reducing the prevalence of obesity and potential insulin resistance later in life; in addition, ongoing dietary advice starting from weaning has the potential to prevent insulin resistance in the long term
Wisnieski et al. (115) conducted a systematic review on the effects of breastfeeding on the risk of MetS showing a protective role of breastfeeding on the development of MetS. Some studies also showed an inverse relationship between duration of breastfeeding and MetS (114, 116), whereas others did not find a temporal association (117, 118).
It has been postulated that, during pregnancy and the next 2 years of life, correct maternal eating habits, breastfeeding, and then weaning with an intake of correct doses of the components of diet are essential for reducing the risk of MetS (119).
Identification of infants and preschool children at risk for obesity combined with intervention programs to prevent excessive weight gain should be developed and evaluated
Obesity is one of the leading causes of IR in childhood. Preventive strategies are the effective public health intervention in fighting against this pandemic. A multicomponent approach has been found beneficial in preventing obesity. All the environments where the child lives have a crucial role; therefore, family, school, and community should collaborate in the child’s healthy lifestyle. Furthermore, a structured weight reduction program individualized for every child is needed. Anti-obesity drugs are indicated only in rare specific cases, and bariatric surgery is recommended only in older adolescents, but data about its long-term effectiveness in this age group are limited (120).
Conclusions
Since 2010, many further studies have been conducted to better explain the underlying mechanisms of IR and the characteristics of children with impaired IS. Although some concepts, such as the timing of rapid weight gain with respect to future IR and the role of IR in predicting the development of impaired glucose tolerance and T2D, have been better clarified, a validated definition of IR is still lacking. Further research is still needed to best measure IS and to find strong surrogate biomarkers of IR.
Author contributions
VT and SS drafted the manuscript. FC provided critical feedback and helped in shaping the manuscript. All authors contributed to the article and approved the submitted version.
Conflict of interest
The authors declare that the research was conducted in the absence of any commercial or financial relationships that could be construed as a potential conflict of interest.
Publisher’s note
All claims expressed in this article are solely those of the authors and do not necessarily represent those of their affiliated organizations, or those of the publisher, the editors and the reviewers. Any product that may be evaluated in this article, or claim that may be made by its manufacturer, is not guaranteed or endorsed by the publisher.
References
1. Chadt A, Al-Hasani H. Glucose transporters in adipose tissue, liver, and skeletal muscle in metabolic health and disease. Pflugers Arch (2020) 472(9):1273–98. doi: 10.1007/s00424-020-02417-x
2. Bussler S, Penke M, Flemming G, Elhassan YS, Kratzsch J, Sergeyev E, et al. Novel insights in the metabolic syndrome in childhood and adolescence. Horm Res Paediatr (2017) 88:181–93. doi: 10.1159/000479510
3. Kojta I, Chacińska M, Błachnio-Zabielska A. Obesity, bioactive lipids, and adipose tissue inflammation in insulin resistance. Nutrients (2020) 12(5):1305. doi: 10.3390/nu12051305
4. Levy-Marchal C, Arslanian S, Cutfield W, Sinaiko A, Druet C, Marcovecchio ML, et al. Insulin resistance in children: consensus, perspective, and future directions. J Clin Endocrinol Metab (2010) 95(12):5189–98. doi: 10.1210/jc.2010-1047
5. Güneş H, Güneş H, Temiz F. The relationship between epicardial adipose tissue and insulin resistance in obese children. Arq Bras Cardiol (2020) 114(4):675–82. doi: 10.36660/abc.20190197
6. Yang Q, Vijayakumar A, Kahn BB. Metabolites as regulators of insulin sensitivity and metabolism. Nat Rev Mol Cell Biol (2018) 19(10):654–72. doi: 10.1038/s41580-018-0044-8
7. Valaiyapathi B, Gower B, Ashraf AP. Pathophysiology of type 2 diabetes in children and adolescents. Curr Diabetes Rev (2020) 16(3):220–9. doi: 10.2174/1573399814666180608074510
8. Lauterbach MA, Wunderlich FT. Macrophage function in obesity-induced inflammation and insulin resistance. Pflugers Arch (2017) 469(3-4):385–96. doi: 10.1007/s00424-017-1955-5
9. Temneanu OR, Trandafir LM, Purcarea MR. Type 2 diabetes mellitus in children and adolescents: a relatively new clinical problem within pediatric practice. J Med Life (2016) 9(3):235–9.
10. van der Aa MP, Fazeli Farsani S, Knibbe CA, de Boer A, van der Vorst MM. Population-based studies on the epidemiology of insulin resistance in children. J Diabetes Res (2015) 2015:362375. doi: 10.1155/2015/362375
11. Güngör NK. Overweight and obesity in children and adolescents. J Clin Res Pediatr Endocrinol (2014) 6(3):129–43. doi: 10.4274/Jcrpe.1471
12. World Health Organization. Tenfold increase in childhood and adolescent obesity in four decades: New study by imperial college London and WHO . Available at: https://www.who.int/news/item/11-10-2017-tenfold-increase-in-childhood-and-adolescent-obesity-in-four-decades-new-study-by-imperial-college-london-and-who (Accessed 21 October 2020).
13. Ighbariya A, Weiss R. Insulin resistance, prediabetes, metabolic syndrome: What should every pediatrician know? J Clin Res Pediatr Endocrinol (2017) 9(Suppl 2):49–57. doi: 10.4274/jcrpe.2017.S005
14. Samuel VT, Shulman GI. The pathogenesis of insulin resistance: integrating signaling pathways and substrate flux. J Clin Invest. (2016) 126(1):12–22. doi: 10.1172/JCI77812
15. Lee SH, Ahn MB, Choi YJ, Kim SK, Kim SH, Cho WK, et al. Comparison of different criteria for the definition of insulin resistance and its relationship to metabolic risk in children and adolescents. Ann Pediatr Endocrinol Metab (2020) 25(4):227–33. doi: 10.6065/apem.2040002.001
16. Rupérez FJ, Martos-Moreno GÁ, Chamoso-Sánchez D, Barbas C, Argente J. Insulin resistance in obese children: What can metabolomics and adipokine modelling contribute? Nutrients (2020) 12(11):3310. doi: 10.3390/nu12113310
17. Brown RJ, Yanovski JA. Estimation of insulin sensitivity in children: methods, measures and controversies. Pediatr Diabetes. (2014) 15(3):151–61. doi: 10.1111/pedi.12146
18. Nogueira-de-Almeida CA, de Mello ED. Different criteria for the definition of insulin resistance and its relation with dyslipidemia in overweight and obese children and adolescents. Pediatr Gastroenterol Hepatol Nutr (2018) 21(1):59–67. doi: 10.5223/pghn.2018.21.1.59
19. Altshuler D, Hirschhorn JN, Klannemark M, Lindgren CM, Vohl MC, Nemesh J, et al. The common PPARgamma Pro12Ala polymorphism is associated with decreased risk of type 2 diabetes. Nat Genet (2000) 26(1):76–80. doi: 10.1038/79216
20. Muniyappa R, Lee S, Chen H, Quon MJ. Current approaches for assessing insulin sensitivity and resistance in vivo: advantages, limitations, and appropriate usage. Am J Physiol Endocrinol Metab (2008) 294(1):E15–26. doi: 10.1152/ajpendo.00645.2007
21. Ciresi A, Guarnotta V, Pizzolanti G, Giordano C. Comparison between euglycemic hyperinsulinemic clamp and surrogate indices of insulin sensitivity in children with growth hormone deficiency. Growth Horm IGF Res (2018) 39:40–4. doi: 10.1016/j.ghir.2017.12.007
22. Bergman RN, Ider YZ, Bowden CR, Cobelli C. Quantitative estimation of insulin sensitivity. Am J Physiol (1979) 236(6):E667–77. doi: 10.1152/ajpendo.1979.236.6.E667
23. Bergman RN, Prager R, Volund A, Olefsky JM. Equivalence of the insulin sensitivity index in man derived by the minimal model method and the euglycemic glucose clamp. J Clin Invest. (1987) 79(3):790–800. doi: 10.1172/JCI112886
24. Tagi VM, Giannini C, Chiarelli F. Insulin resistance in children. Front Endocrinol (Lausanne). (2019) 10:342. doi: 10.3389/fendo.2019.00342
25. George L, Bacha F, Lee S, Tfayli H, Andreatta E, Arslanian S. Surrogate estimates of insulin sensitivity in obese youth along the spectrum of glucose tolerance from normal to prediabetes to diabetes. J Clin Endocrinol Metab (2011) 96(7):2136–45. doi: 10.1210/jc.2010-2813
26. Schwartz B, Jacobs DR Jr, Moran A, Steinberger J, Hong CP, Sinaiko AR. Measurement of insulin sensitivity in children: comparison between the euglycemic-hyperinsulinemic clamp and surrogate measures. Diabetes Care (2008) 31(4):783–8. doi: 10.2337/dc07-1376
27. Rasmussen-Torvik LJ, Pankow JS, Jacobs DR, Steffen LM, Moran AM, Steinberger J, et al. Heritability and genetic correlations of insulin sensitivity measured by the euglycaemic clamp. Diabetes Med (2007) 24(11):1286–9. doi: 10.1111/j.1464-5491.2007.02271.x
28. Haymond MW, Chung ST. Commentary on the impact of obesity and puberty on insulin sensitivity. J Clin Endocrinol Metab (2020) 105(5):e2082–3. doi: 10.1210/clinem/dgaa104
29. Goran MI, Gower BA. Longitudinal study on pubertal insulin resistance. Diabetes (2001) 50(11):2444–50. doi: 10.2337/diabetes.50.11.2444
30. Marwitz SE, Gaines MV, Brady SM, Mi SJ, Broadney MM, Yanovski SZ, et al. Cross-sectional and longitudinal examination of insulin sensitivity and secretion across puberty among non-Hispanic black and white children. Endocrinol Metab (Seoul). (2020) 35(4):847–57. doi: 10.3803/EnM.2020.771
31. Goran MI, Bergman RN, Cruz ML, Watanabe R. Insulin resistance and associated compensatory responses in African- American and Hispanic children. Diabetes Care (2002) 25:2184 –2190. doi: 10.2337/diacare.25.12.2184
32. Arslanian SA, Saad R, Lewy V, Danadian K, Janosky J. Hyperinsulinemia in African-American children: decreased insulin clearance and increased insulin secretion and its relationship to insulin sensitivity. Diabetes (2002) 51:3014–9. doi: 10.2337/diabetes.51.10.3014
33. Whincup PH, Gilg JA, Papacosta O, Seymour C, Miller GJ, Alberti KG, et al. Early evidence of ethnic differences in cardiovascular risk: cross sectional comparison of British south Asian and white children. BMJ (2002) 324:635. doi: 10.1136/bmj.324.7338.635
34. DeBoer MD. Assessing and managing the metabolic syndrome in children and adolescents. Nutrients (2019) 11(8):1788. doi: 10.3390/nu11081788
35. Lee S, Bacha F, Gungor N, Arslanian S. Comparison of different definitions of pediatric metabolic syndrome: relation to abdominal adiposity, insulin resistance, adiponectin, and inflammatory biomarkers. J Pediatr (2008) 152:177–84. doi: 10.1016/j.jpeds.2007.07.053
36. Patton HM, Yates K, Unalp-Arida A, Behling CA, Huang TT, Rosenthal P, et al. Association between metabolic syndrome and liver histology among children with nonalcoholic fatty liver disease. Am J Gastroenterol (2010) 105(9):2093–102. doi: 10.1038/ajg.2010.152
37. Barshop NJ, Sirlin CB, Schwimmer JB, Lavine JE. Review article: epidemiology, pathogenesis and potential treatments of paediatric non-alcoholic fatty liver disease. Aliment Pharmacol Ther (2008) 28(1):13–24. doi: 10.1111/j.1365-2036.2008.03703.x
38. Vos MB, Abrams SH, Barlow SE, Caprio S, Daniels SR, Kohli R, et al. NASPGHAN clinical practice guideline for the diagnosis and treatment of nonalcoholic fatty liver disease in children: Recommendations from the expert committee on NAFLD (ECON) and the north American society of pediatric gastroenterology, hepatology and nutrition (NASPGHAN). J Pediatr Gastroenterol Nutr (2017) 64(2):319–34. doi: 10.1097/MPG.0000000000001482
39. Draijer L, Benninga M, Koot B. Pediatric NAFLD: an overview and recent developments in diagnostics and treatment. Expert Rev Gastroenterol Hepatol (2019) 13(5):447–61. doi: 10.1080/17474124.2019.1595589
40. Buzzetti E, Pinzani M, Tsochatzis EA. The multiple-hit pathogenesis of non-alcoholic fatty liver disease (NAFLD). Metabolism (2016) 65(8):1038–48. doi: 10.1016/j.metabol.2015.12.012
41. Witchel SF, Oberfield SE, Peña AS. Polycystic ovary syndrome: Pathophysiology, presentation, and treatment with emphasis on adolescent girls. J Endocr Soc (2019) 3(8):1545–73. doi: 10.1210/js.2019-00078
42. Arslanian SA, Lewy VD, Danadian K. Glucose intolerance in obese adolescents with polycystic ovary syndrome: roles of insulin resistance and b-cell dysfunction and risk of cardiovascular dis-ease. J Clin Endocrinol Metab (2001) 86:66 –71. doi: 10.1210/jcem.86.1.7123
43. Silfen ME, Denburg MR, Manibo AM, Lobo RA, Jaffe R, Ferin M, et al. Early endocrine, metabolic, and sonographic characteristics of polycystic ovary syndrome (PCOS): comparison between nonobese and obese adolescents. J Clin Endocrinol Metab (2003) 88:4682–8. doi: 10.1210/jc.2003-030617
44. Dahan MH, Reaven G. Relationship among obesity, insulin resistance, and hyperinsulinemia in the polycystic ovary syndrome. Endocrine (2019) 64(3):685–9. doi: 10.1007/s12020-019-01899-9
45. Deeb SS, Fajas L, Nemoto M, Pihlajamaki J, Mykkanen L, Kuusisto J, et al. A Pro12Ala substitution in PPARgamma2 associated with decreased receptor activity, lower body mass index and improved insulin sensitivity. Nat Genet (1998) 20(3):284–7. doi: 10.1038/3099
46. Voight BF, Scott LJ, Steinthorsdottir V, Morris AP, Dina C, Welch RP, et al. Twelve type 2 diabetes susceptibility loci identified through large-scale association analysis. Nat Genet (2010) 42(7):579–89. doi: 10.1038/ng.609
47. Rung J, Cauchi S, Albrechtsen A, Shen L, Rocheleau G, Cavalcanti-Proenca C, et al. Genetic variant near IRS1 is associated with type 2 diabetes, insulin resistance and hyperinsulinemia. Nat Genet (2009) 41(10):1110–5. doi: 10.1038/ng.443
48. Do R, Bailey SD, Desbiens K, Belisle A, Montpetit A, Bouchard C, et al. Genetic variants of FTO influence adiposity, insulin sensitivity, leptin levels, and resting metabolic rate in the Quebec family study. Diabetes (2008) 57(4):1147–50. doi: 10.2337/db07-1267
49. Zeggini E, Scott LJ, Saxena R, Voight BF, Marchini J, Hu T, et al. Meta-analysis of genome-wide association data and large-scale replication identifies additional sus ceptibility loci for type 2 diabetes. Nat Genet (2008) 40(5):638–45. doi: 10.1038/ng.120
50. Morris AP, Voight BF, Teslovich TM, Ferreira T, Segre' AV, Steinthorsdottir V, et al. Large-Scale association analysis provides insights into the genetic architecture and patho physiology of type 2 diabetes. Nat Genet (2012) 44(9):981–90. doi: 10.1038/ng.2383
51. Damcott CM, Pollin TI, Reinhart LJ, Ott SH, Shen H, SIlver KD, et al. Polymorphisms in the transcription factor 7-like 2 (TCF7L2) gene are associated with type 2 diabetes in the Amish: replication and evidence for a role in both insulin secretion and insulin resistance. Diabetes (2006) 55(9):2654–9. doi: 10.2337/db06-0338
52. Elbein SC, Chu WS, Das SK, Borengasser A, Hasstedt SJ, Wang H, et al. Transcription factor 7-like 2 polymorphisms and type 2 diabetes, glucose homeostasis traits and gene expression in US participants of European and African descent. Diabetologia (2007) 50(8):1621–30. doi: 10.1007/s00125-007-0717-x
53. Knowles JW, Xie W, Zhang Z, Chennamsetty I, Assimes TL, Paanen J, et al. Identification and validation of n-acetyltransferase 2 as an insulin sensitivity gene. J Clin Invest. (2015) 125(4):1739–51. doi: 10.1172/JCI74692
54. Succurro E, Andreozzi F, Marini MA, Hribal ML, Petricone F, Sesti G, et al. Low plasma insulin-like growth factor-1 levels are associated with reduced insulin sensitivity and increased insulin secretion in nondiabetic subjects. Nutr Metab Cardiovasc Dis (2009) 19(10):713–9. doi: 10.1016/j.numecd.2008.12.011
55. Chen G, Bentley A, Adeyemo A, Shriner D, Zhou J, Doumatey, et al. Genome-wide association study identifies novel loci association with fasting insulin and insulin resistance in African americans. Hum Mol Genet (2012) 21(20):4530–6. doi: 10.1093/hmg/dds282
56. Mahajan A, Go MJ, Zhang W, Below JE, Gaulton KJ, Ferreira T, et al. Genome-wide trans-ancestry meta-analysis provides insight into the genetic architecture of type 2 diabetes susceptibility. Nat Genet (2014) 46(3):234–44. doi: 10.1038/ng.2897
57. Richards JB, Waterworth D, O’Rahilly S, Hivert MF, Loos RJF, Perry JRB, et al. A genome-wide association study reveals variants in ARL15 that influence adiponectin levels. PloS Genet (2009) 5(12):e1000768. doi: 10.1371/journal.pgen.1000768
58. Dupuis J, Langenberg C, Prokopenko I, Saxena R, Soranzo N, Jackson AU, et al. New genetic loci implicated in fasting glucose homeostasis and their impact on type 2 diabetes risk. Nat Genet (2010) 42(2):105–16. doi: 10.1038/ng.520
59. Shu L, Sauter NS, Schulthess FT, Matveyenko AV, Oberholzer J, Maedles K, et al. Transcription factor 7-like 2 regulates beta-cell survival and function in human pancreatic islets. Diabetes (2008) 57(3):645–53. doi: 10.2337/db07-0847
60. Brown AE, Walker M. Genetics of insulin resistance and the metabolic syndrome. Curr Cardiol Rep (2016) 18(8):75. doi: 10.1007/s11886-016-0755-4
61. Yang M, Ren Y, Lin Z, Tang C, Jia Y, Lai Y, et al. Kruppel-like factor 14 increases insulin sensitivity through activation of PI3K/Akt signal pathway. Cell Signal (2015) 27(11):2201–8. doi: 10.1016/j.cellsig.2015.07.019
62. Van Schaftingen E. A protein from rat liver confers to glucokinase the property of being antagonistically regulated by fructose 6-phosphate and fructose 1-phosphate. Eur J Biochem (1989) 179(1):179–84. doi: 10.1111/j.1432-1033.1989.tb14538.x
63. Liu PH, Chang YC, Jiang YD, Chen WJ, Chang TJ, Kuo SS, et al. Genetic variants of TCF7L2 are associated with insulin resistance and related metabolic phenotypes in Taiwanese adolescents and Caucasian young adults. J Clin Endocrinol Metab (2009) 94(9):3575–82. doi: 10.1210/jc.2009-0609
64. Bailey KA, Savic D, Zielinski M, Wang LJ, Witkowski P, Brady M, et al. Evidence of non-pancreatic beta cell-dependent roles of Tcf7l2 in the regulation of glucose metabolism in mice. Hum Mol Genet (2015) 24(6):1646–54. doi: 10.1093/hmg/ddu577
65. Tabassum R, Chauhan G, Dwivedi OP, Mahajan A, Jaiswal A, Kaur I, et al. Genome-wide association study for type 2 diabetes in indians identifies a new susceptibility locus at 2q21. Diabetes (2013) 62(3):977–86. doi: 10.2337/db12-0406
66. Mannino GC, Greco A, De Lorenzo C, Marini MA, Perticone F, Sesti G, et al. A fasting insulin-raising allele at IGF1 locus is associated with circulating levels of IGF-1 and insulin sensitivity. PloS One (2013) 8(12):e85483. doi: 10.1371/journal.pone.0085483
67. Chambers JC, Elliott P, Zabaneh D, Zhang W, Li Y, Froguel P. Common genetic variation near MC4R is associated with waist circumference and insulin resistance. Nat Genet (2008) 40(6):716–8. doi: 10.1038/ng.156
68. Plows JF, Stanley JL, Baker PN, Reynolds CM, Vickers MH. The pathophysiology of gestational diabetes mellitus. Int J Mol Sci (2018) 19(11):3342. doi: 10.3390/ijms19113342
69. Feig DS, Moses RG. Metformin therapy during pregnancy good for the goose and good for the gosling too? Diabetes Care (2011) 34:2329–30. doi: 10.2337/dc11-1153
70. Camelo Castillo W, Boggess K, Stürmer T, Brookhart MA, Benjamin DK, Jonsson Funk M. Association of adverse pregnancy outcomes with glyburide vs insulin in women with gestational diabetes. JAMA Pediatr (2015) 169:452–8. doi: 10.1001/jamapediatrics.2015.74
71. Mericq V, Ong KK, Bazaes R, Pena V, Avila A, Salazar T, et al. Longitudinal changes in insulin sensitivity and secretion from birth to age three years in small- and appropriate-for-gestational-age children. Diabetologia (2005) 48:2609–14. doi: 10.1007/s00125-005-0036-z
72. Finken MJ, Keijzer-Veen MG, Dekker FW, Frolich M, Hille ET, Romijn JA, et al. Preterm birth and later insulin resistance: effects of birth weight and postnatal growth in a population based longitudinal study from birth into adult life. Diabetologia (2006) 49:478–85. doi: 10.1007/s00125-005-0118-y
73. Sinaiko AR, Donahue RP, Jacobs DR Jr., Prineas RJ. Relation of weight and rate of increase in weight during childhood and adolescence to body size, blood pressure, fasting insulin, and lipids in young adults. Minneapolis Children’s Blood Pressure Study Circ (1999) 99:1471–6. doi: 10.1161/01.CIR.99.11.1471
74. Eriksson JG, Forsen T, Tuomilehto J, Osmond C, Barker DJ. Early adiposity rebound in childhood and risk of type 2 diabetes in adult life. Diabetologia (2003) 46:190–4. doi: 10.1007/s00125-002-1012-5
75. Ong KK, Petry CJ, Emmett PM, Sandhu MS, Kiess W, Hales CN, et al. Insulin sensitivity and secretion in normal children related to size at birth, postnatal growth, and plasma insulin-like growth factor-I levels. Diabetologia (2004) 47:1064–70. doi: 10.1007/s00125-004-1405-8
76. Leunissen RW, Kerkhof GF, Stijnen T, Hokken-Koelega A. Timing and tempo of first-year rapid growth in relation to cardiovascular and metabolic risk profile in early adulthood. JAMA (2009) 301:2234–42. doi: 10.1001/jama.2009.761
77. Barker DJ. The origins of the developmental origins theory. J Intern Med (2007) 261:412–7. doi: 10.1111/j.1365-2796.2007.01809.x
78. Ong YY, Sadananthan SA, Aris IM, Tint MT, Yuan WL, Huang JY, et al. Mismatch between poor fetal growth and rapid postnatal weight gain in the first 2 years of life is associated with higher blood pressure and insulin resistance without increased adiposity in childhood: the GUSTO cohort study. Int J Epidemiol. (2020) 49(5):1591–603. doi: 10.1093/ije/dyaa143
79. Flint A, Arslanian S. Treatment of type 2 diabetes in youth. Diabetes Care (2011) 34(Suppl. 2):S177–83. doi: 10.2337/dc11-s215
80. Mayer-Davis EJ, Lawrence JM, Dabelea D, Divers J, Isom S, Dolan L, et al. Search for diabetes in youth study. incidence trends of type 1 and type 2 diabetes among youths, 2002-2012. N Engl J Med (2017) 376(15):1419–29. doi: 10.1056/NEJMoa1610187
81. Nadal A, Alonso-Magdalena P, Soriano S, Quesada I, Ropero AB. The pancreatic beta-cell as a target of estrogens and xenoestrogens: Implications for blood glucose homeostasis and diabetes. Mol Cell Endocrinol (2009) 304(1-2):63–8. doi: 10.1016/j.mce.2009.02.016
82. Kim JY, Bacha F, Tfayli H, Michaliszyn SF, Yousuf S, Arslanian S. Adipose tissue insulin resistance in youth on the spectrum from normal weight to obese and from normal glucose tolerance to impaired glucose tolerance to type 2 diabetes. Diabetes Care (2019) 42(2):265–72. doi: 10.2337/dc18-1178
83. Ahrens W, Moreno LA, Mårild S, Molnár D, Siani A, De Henauw S, et al. Metabolic syndrome in young children: definitions and results of the IDEFICS study. Int J Obes (2014) 38(Suppl 2):S4–14. doi: 10.1038/ijo.2014.130
84. Miniello VL, Faienza MF, Scicchitano P, Cortese F, Gesualdo M, Zito A, et al. Insulin resistance and endothelial function in children and adolescents. Int J Cardiol (2014) 174(2):343–7. doi: 10.1016/j.ijcard.2014.04.115
85. Yajnik CS, Katre PA, Joshi SM, Kumaran K, Bhat DS, Lubree HG, et al. Higher glucose, insulin and insulin resistance (HOMA-IR) in childhood predict adverse cardiovascular risk in early adulthood: the pune children’s study. Diabetologia (2015) 58(7):1626–36. doi: 10.1007/s00125-015-3602-z
86. Tagi VM, Samvelyan S, Chiarelli F. Metabolic syndrome in children. Minerva Pediatr (2020) 72(4):312–25. doi: 10.23736/S0026-4946.20.05834-X
87. Gow ML, Ho M, Burrows TL, Baur LA, Stewart L, Hutchesson MJ, et al. Impact of dietary macronutrient distribution on BMI and cardiometabolic outcomes in overweight and obese children and adolescents: a systematic review. Nutr Rev (2014) 72:453–70. doi: 10.1111/nure.12111
88. Steffen LM, Jacobs DR Jr, Murtaugh MA, Moran A, Steinberger J, Hong CP, et al. Whole grain intake is associated with lower body mass and greater insulin sensitivity among adolescents. Am J Epidemiol (2003) 158:243–50. doi: 10.1093/aje/kwg146
89. Carlson JJ, Eisenmann JC, Norman GJ, Ortiz KA, Young PC. Dietary fiber and nutrient density are inversely associated with the metabolic syndrome in US adolescents. J Am Diet Assoc (2011) 111:1688–95. doi: 10.1016/j.jada.2011.08.008
90. Kynde I, Johnsen NF, Wedderkopp N, Bygbjerg IB, Helge JW, Heitmann BL. Intake of total dietary sugar and fibre is associated with insulin resistance among Danish 8-10- and 14-16-year-old girls but not boys. European youth heart studies I and II. Public Health Nutr (2010) 13:1669–74. doi: 10.1017/S1368980010000285
91. Weigensberg MJ, Ball GD, Shaibi GQ, Cruz ML, Gower BA, Goran MI. Dietary fat intake and insulin resistance in black and white children. Obes Res (2005) 13:1630–7. doi: 10.1038/oby.2005.200
92. Sunehag AL, Toffolo G, Treuth MS, Butte NF, Cobelli C, Bier DM, et al. Effects of dietary macronutrient content on glucose metabolism in children. J Clin Endocrinol Metab (2002) 87:5168–78. doi: 10.1210/jc.2002-020674
93. Galgani JE, Uauy RD, Aguirre CA, Díaz EO. Effect of the dietary fat quality on insulin sensitivity. Br J Nutr (2008) 100:471–9. doi: 10.1017/S0007114508894408
94. Ludwig DS. The glycemic index: physiological mechanisms relating to obesity, diabetes, and cardiovascular disease. JAMA (2002) 287:2414–23. doi: 10.1001/jama.287.18.2414
95. Kong AP, Chan RS, Nelson EA, Chan JC. Role of low glycemic index diet in management of childhood obesity. Obes Rev (2011) 12:492–8. doi: 10.1111/j.1467-789X.2010.00768.x
96. Thomas DE, Elliott EJ, Baur L. Low glycaemic index or low glycaemic load diets for overweight and obesity. Cochrane Database Syst Rev (2007) 2007:CD005105. doi: 10.1002/14651858.CD005105.pub2
97. Allen DB, Nemeth BA, Clark RR, Peterson SE, Eickhoff J, Carrel AL. Fitness is a stronger predictor of fasting insulin levels than fatness in overweight male middle-school children. J Pediatr (2007) 150:383–7. doi: 10.1016/j.jpeds.2006.12.051
98. Martínez-Gómez D, Eisenmann JC, Moya JM, Gómez Martínez S, Marcos A, Veiga OL. The role of physical activity and fitness on the metabolic syndrome in adolescents: effect of different scores. the AFINOS study. J Physiol Biochem (2009) 65:277–89. doi: 10.1007/BF03180580
99. Lätt E, Mäestu J, Rääsk T, Jürimäe T, Jürimäe J. Cardiovascular fitness, physical activity, and metabolic syndrome risk factors among adolescent estonian boys: A longitudinal study. Am J Hum Biol (2016) 28:782–8. doi: 10.1002/ajhb.22866
100. Rizzo NS, Ruiz JR, Oja L, Veidebaum T, Sjöström M. Associations between physical activity, body fat, and insulin resistance (homeostasis model assessment) in adolescents: the European youth heart study. Am J Clin Nutr (2008) 87:586–92. doi: 10.1093/ajcn/87.3.586
101. Ferguson MA, Gutin B, Le NA, Karp W, Litaker M, Humphries M, et al. Effects of exercise training and its cessation on components of the insulin resistance syndrome in obese children. Int J Obes Relat Metab Disord (1999) 23:889–95. doi: 10.1038/sj.ijo.0800968
102. Bell LM, Watts K, Siafarikas A, Thompson A, Ratnam N, Bulsara M, et al. Exercise alone reduces insulin resistance in obese children independently of changes in body composition. J Clin Endocrinol Metab (2007) 92:4230–5. doi: 10.1210/jc.2007-0779
103. Mendelson M, Michallet AS, Monneret D, Perrin C, Estève F, Lombard PR, et al. Impact of exercise training without caloric restriction on inflammation, insulin resistance and visceral fat mass in obese adolescents. Pediatr Obes (2015) 10:311–9. doi: 10.1111/ijpo.255
104. García-Hermoso A, Saavedra JM, Escalante Y, Sánchez López M, Martínez-Vizcaíno V. Endocrinology and adolescence: aerobic exercise reduces insulin resistance markers in obese youth: a meta-analysis of randomized controlled trials. Eur J Endocrinol (2014) 171:R163–71. doi: 10.1530/EJE-14-0291
105. Chae HW, Kwon YN, Rhie YJ, Kim HS, Kim YS, Paik IY, et al. Effects of a structured exercise program on insulin resistance, inflammatory markers and physical fitness in obese Korean children. J Pediatr Endocrinol Metab (2010) 23:1065–72. doi: 10.1515/jpem.2010.168
106. Van Der Heijden GJ, Wang ZJ, Chu Z, Toffolo G, Manesso E, Sauer PJ, et al. Strength exercise improves muscle mass and hepatic insulin sensitivity in obese youth. Med Sci Sports Exerc (2010) 42:1973–80. doi: 10.1249/MSS.0b013e3181df16d9
107. Shaibi GQ, Cruz ML, Ball GD, Weigensberg MJ, Salem GJ, Crespo NC, et al. Effects of resistance training on insulin sensitivity in overweight Latino adolescent males. Med Sci Sports Exerc (2006) 38:1208–15. doi: 10.1249/01.mss.0000227304.88406.0f
108. Savoye M, Shaw M, Dziura J, Tamborlane WV, Rose P, Guandalini C, et al. Effects of a weight management program on body composition and metabolic parameters in overweight children: a randomized controlled trial. JAMA (2007) 297:2697–704. doi: 10.1001/jama.297.24.2697
109. Park TG, Hong HR, Lee J, Kang HS. Lifestyle plus exercise intervention improves metabolic syndrome markers without change in adiponectin in obese girls. Ann Nutr Metab (2007) 51:197–203. doi: 10.1159/000104137
110. Androutsos O, Moschonis G, Mavrogianni C, Roma-Giannikou E, Chrousos GP, Kanaka-Gantenbein C, et al. Identification of lifestyle patterns, including sleep deprivation, associated with insulin resistance in children: the healthy growth study. Eur J Clin Nutr (2014) 68:344–9. doi: 10.1038/ejcn.2013.280
111. Rena G, Hardie DG, Pearson ER. The mechanisms of action of metformin. Diabetologia (2017) 60(9):1577–85. doi: 10.1007/s00125-017-4342-z
112. Tagi VM, Samvelyan S, Chiarelli F. Treatment of metabolic syndrome in children. Horm Res Paediatr (2020) 93(4):215–25. doi: 10.1159/000510941
113. Kc K, Shakya S, Zhang H. Gestational diabetes mellitus and macrosomia: a literature review. Ann Nutr Metab (2015) 66 Suppl 2:14–20. doi: 10.1159/000371628
114. González-Jiménez E, Montero-Alonso MA, Schmidt-RioValle J, García-García CJ, Padez C. Metabolic syndrome in Spanish adolescents and its association with birth weight, breastfeeding duration, maternal smoking, and maternal obesity: a cross-sectional study. Eur J Nutr (2015) 54(4):589–97. doi: 10.1007/s00394-014-0740-x
115. Wisnieski L, Kerver J, Holzman C, Todem D, Margerison-Zilko C. Breastfeeding and risk of metabolic syndrome in children and adolescents: A systematic review. J Hum Lact. (2018) 34(3):515–25. doi: 10.1177/0890334417737038
116. Wang J, Zhu Y, Cai L, Jing J, Chen Y, Mai J, et al. Metabolic syndrome and its associated early-life factors in children and adolescents: A cross-sectional study in guangzhou, China. Public Health Nutr (2015) 19:1147–54. doi: 10.1017/S1368980015002542
117. Yakubov R, Nadir E, Stein R and Klein-Kremer A. The duration of breastfeeding and its association with metabolic syndrome among obese children. Sci World J (2015) 114:E4. doi: 10.1155/2015/731319
118. Izadi V, Kelishadi R, Qorbani M, EsmaeilMotlagh M, Taslimi M, Heshmat R, et al. Duration of breastfeeding and cardiovascular risk factors among Iranian children and adolescents: The CASPIAN III study. Nutrition (2013) 29:744–751. doi: 10.1016/j.nut.2012.10.016
119. Agosti M, Tandoi F, Morlacchi L, Bossi A. Nutritional and metabolic programming during the first thousand days of life. Pediatr Med Chir. (2017) 39(2):157. doi: 10.4081/pmc.2017.157
Keywords: insulin resistance, children, obesity, metabolic syndrome, genetics, diagnosis, treatment
Citation: Tagi VM, Samvelyan S and Chiarelli F (2022) An update of the consensus statement on insulin resistance in children 2010. Front. Endocrinol. 13:1061524. doi: 10.3389/fendo.2022.1061524
Received: 04 October 2022; Accepted: 14 October 2022;
Published: 16 November 2022.
Edited by:
Maurizio Delvecchio, Giovanni XXIII Children’s Hospital, ItalyReviewed by:
Sonia Caprio, Yale University, United StatesCopyright © 2022 Tagi, Samvelyan and Chiarelli. This is an open-access article distributed under the terms of the Creative Commons Attribution License (CC BY). The use, distribution or reproduction in other forums is permitted, provided the original author(s) and the copyright owner(s) are credited and that the original publication in this journal is cited, in accordance with accepted academic practice. No use, distribution or reproduction is permitted which does not comply with these terms.
*Correspondence: Veronica Maria Tagi, dmVyb25pY2EudGFnaUBnbWFpbC5jb20=