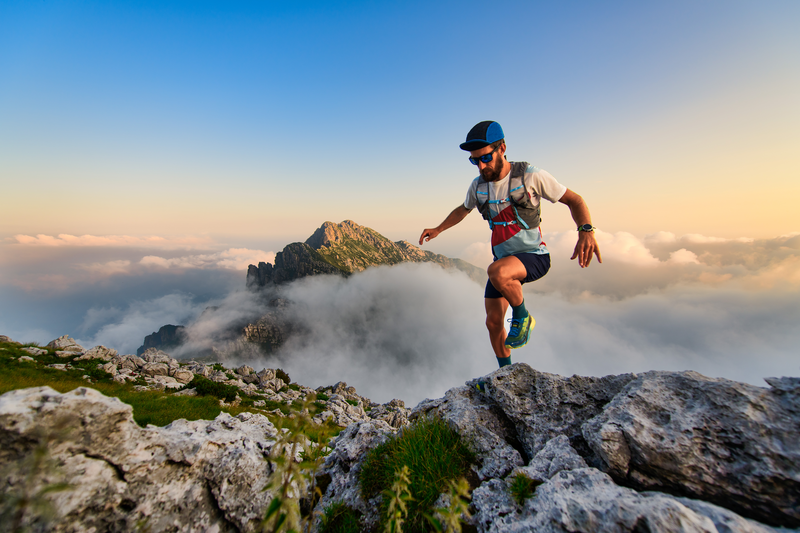
95% of researchers rate our articles as excellent or good
Learn more about the work of our research integrity team to safeguard the quality of each article we publish.
Find out more
ORIGINAL RESEARCH article
Front. Endocrinol. , 09 January 2023
Sec. Experimental Endocrinology
Volume 13 - 2022 | https://doi.org/10.3389/fendo.2022.1058298
This article is part of the Research Topic The Role of Seasonal Hormones in Plasticity of Morphology and Natural Behavior: an Integrative Perspective using Non-Traditional Model Systems View all 4 articles
In both captive and free-living birds, the emergence of the migratory phenotype is signalled by rapid and marked increases in food intake and fuelling, as well as changes in amount of nocturnality or migratory restlessness. The metabolic hormone corticosterone and, as more recently suggested, the gut-derived hormone ghrelin have been suggested to play a role in mediating such phenomenal phenotypic flexibility given that they both regulate fuel metabolism and locomotion across vertebrate taxa. Here, using the Common quail (Coturnix coturnix) as our study species, we induced autumn migration followed by a non-migratory wintering phase through controlled changes in daylight. We thus compared plasma corticosterone and ghrelin concentrations between the two sampling phases and assessed whether these hormones might reflect the migratory state. While we found no differences in plasma corticosterone between the two sampling phases and no link of this hormone with changes in body mass, levels of food intake or migratory restlessness, the migratory birds had substantially higher levels of plasma ghrelin relative to the non-migratory birds. Furthermore, while ghrelin did not correlate with the gain in body mass over the entire pre-migratory fuelling phase (over an average of nine weeks preceding blood sampling), plasma ghrelin did positively correlate with the gain in body mass observed during the final fattening stages (over an average of three weeks preceding blood sampling). Again, variation in plasma ghrelin also reflected the amount of body mass depleted over both the long- and short-time frame as birds returned to their non-migratory baseline - lower levels of plasma ghrelin consistently correlated with larger losses in body mass. Thus, while our data do not highlight a role of the hormone corticosterone in sustaining pre-migratory fattening as shown in other bird species, they do add evidence for a potential role of ghrelin in mediating migratory behaviour and further suggest that this hormone might be important in regulating the transitioning of migratory states, possibly by promoting fuel mobilisation and usage.
Most, if not all, migratory birds evolved spectacular physiological adaptations to accommodate their long-distance migratory flights between their breeding and wintering grounds reviewed in (1, 2). Extensive work has demonstrated that migratory birds use fat as the prime energy source for their migratory flights due to its higher efficiency compared to carbohydrates or proteins (3–6), reviewed by (6). The accumulation of energy reserves that many birds exhibit in preparation to their migratory flights – termed pre-migratory fuelling – is promoted by increased food intake (hyperphagia) which, subsequently, leads to rapid and remarkable gains in subcutaneous fat stores (6–9). Studies in different bird species sampled at stopover sites (i.e., where migrating birds temporarily suspend the migratory flight to rest and refuel) show that individuals with larger subcutaneous fat stores exhibit higher levels of migratory restlessness in captivity (10–14) – i.e., the urge of captive birds to migrate at night (14) and can migrate faster (13). Thus, the physiological preparations occurring over the phase of pre-migratory fuelling are likely to be central in determining the success of the subsequent active phases of the migratory cycle. While it is relatively well known that the motivation of migratory birds to fuel is controlled by changes in photoperiod (2, 15) and by endogenous programs (16–18), the exact endocrine regulators of pre-migratory fuelling remain little understood.
In vertebrates, glucocorticoid hormones (corticosterone is the primary avian glucocorticoid, while it is cortisol in most mammals and fish) play a permissive role in feeding behaviour, energy mobilisation, as well as locomotion (19–22). Thus, it was originally hypothesized that corticosterone plays roles in stimulating fattening and promoting physiological preparation to migration [reviews (23, 24)]. Various studies across different bird species have tested this hypothesis either by experimentally manipulating corticosterone signalling and examining its effect on measures of feeding behaviour and changes in body mass/fat stores e.g (25–28), or by assessing the link between naturally circulating levels of corticosterone and measures of physiological preparations in birds transitioning into the migratory state or at stopover sites e.g (29–33). As highlighted by the recent review of Bauer and Watts (24), these studies provided very limited support that corticosterone promote pre-migratory fuelling (i.e. Physiological Preparation Hypothesis). In fact, despite it is often the case that corticosterone levels are elevated in birds that had reached their peak of body mass (26, 29, 30, 34), changes in plasma corticosterone most often do not parallel with changes in body mass review (24). Thus, corticosterone might sustain enhanced body condition and maintain peak levels of fat deposits (30, 35), and/or have a role in stimulating or maintaining increased activity levels during the active migratory flights (Departure Stimulation Hypothesis, or the Flight Support Hypothesis, respectively – (24)), though fewer studies have tested the latter hypotheses.
Another endocrine candidate that has started to receive a growing attention in the field of avian migration is the recently discovered gut-derived appetite-regulating hormone ghrelin (36). Ghrelin is highly conserved in vertebrates (36) and occurs in two distinct isoforms - acylated and unacylated ghrelin (37, 38). Unacylated ghrelin was originally considered an inactive form of ghrelin because it does not bind to the growth hormone secretagogue receptor (GHSR-1a). Although the regulation of the ghrelin axis remains to be elucidated, it is now clear that unacylated ghrelin exerts a number of systemic functions likely by binding with alternative ghrelin receptors that remain to be identified [reviewed in (39)]. Knowledge about effects of ghrelin on energy metabolism comes mostly from studies in mammals (humans and rodents) and poultry (domestic chickens and Japanese quails). The effect of ghrelin on food intake and lipid metabolism appear to differ between these two taxa as in humans and rodents ghrelin promotes fat storage and feeding (40–42), while in chickens and quails it inhibits them (43–45). Furthermore, exogenous ghrelin leads to corticosterone release in chickens (46–48), suggesting that ghrelin signalling may be functionally linked with glucocorticoids. Recent studies on migratory passerines caught at stopover sites during spring migration suggested that ghrelin can influence stopover and departure decisions (49, 50), though mechanisms might differ during autumn migration (51). In free-flying garden warblers (Sylvia borin) it was first shown that migrants caught at the stopover site with completely depleted fat stores had on average lower plasma concentrations of acylated ghrelin (the only isoform that can currently be measured in avian plasma) than the birds still carrying fat stores (49). It was also shown, importantly, that the exogenous administration of ghrelin in birds that were temporarily caged altered migratory restlessness and food intake, but effects depended on the injected isoform and the birds’ nutritional conditions (49). In a follow-up study on a different passerine species – the yellow-rumped warbler (Setophaga coronata coronata)–Lupi et al. (50) showed that the experimental increase of both ghrelin isoforms in free-flying migrants caused a rapid and short-term acceleration of departure from the stopover site. Overall, these data suggested that ghrelin might promote migratory movements (50) possibly through the stimulatory effects of this hormone on fat breakdown (46). However, whether ghrelin is functionally linked with physiological adaptations during pre-migratory fuelling is currently unknown as all research so far was conducted in active migrants that had already undergone this phase and varied largely in their nutritional conditions at sampling (stopover).
Here, we experimentally controlled the migratory state of young adult Common quails (Coturnix coturnix) by simulating autumn migration followed by a non-migratory phase. The common quail is the only migratory Galliform of the western Palearctic (52). The species breeding area is broadly distributed in Eurasia, while the overwintering distribution ranges from sub-Saharan to Central/North Africa to southern India (53). Wild common quails show large variability in their migratory routes and distances with populations wintering south of the Sahara migrating longer distances compared to populations wintering north of the Sahara (between 25-35 N°). Common quails show physiological adaptations to migration similar to those found in most Passerines species, including remarkable increases in nocturnal activity and fat deposition at the onset of the migratory season in captive conditions (54–56), and the temporal interruption of their migratory flights at stopover sites (57). Furthermore, the extensive knowledge on photoperiodic control system, husbandry and breeding in captivity of the closely related Japanese quail - Coturnix japonica (58) makes the Common quail a good laboratory model for studying migratory physiology, in particular to examine the endocrine changes associated with pre-migratory fuelling while controlling for potential confounding factors such as variation in age, sex, and circadian rhythmicity of experimental animals which would be very difficult to achieve in nature. In this study, we compared plasma concentrations of corticosterone and ghrelin between quails sampled during the migratory phase (i.e. when they reached their peak of body mass), or the non-migratory phase (i.e., as they returned to their physiological baseline). We thus assessed whether these hormones reflected the migratory state of the birds estimated through measurements of food intake, migratory restlessness, and changes in body mass. As corticosterone might have a role in maintaining peak levels of fuel in migratory birds ready to depart (30, 35), we predicted that plasma corticosterone would be higher in the birds sampled during the migratory phase than the non-migratory phase. It is difficult to make exact predictions about the relationships between migratory state and ghrelin given the limited research so far. However, the use of a migratory Galliform as our study system is particularly advantageous in that it allowed us to cross check function of ghrelin known for the few studied migratory Passerines and the domestic Galliformes. Given the inhibitory effects of ghrelin on food intake and its stimulatory effect on lipid breakdown in the domestic Galliformes (citations above), we predicted that plasma ghrelin would reflect changes in body mass as birds were transitioning to a migratory, or non-migratory state. Furthermore, as the hormonal measurements were performed within the same individual birds we also tested whether circulating levels of ghrelin were positively correlated with measures of corticosterone.
This study was performed on a subset of subjects used for a larger experiment published elsewhere (full details on experimental design in (56). Briefly, eggs of Common quail were obtained from our breeding stock kept at the Konrad Lorenz Institute of Ethology (Vetmeduni Vienna, Austria). The stock quails originated from wild funders captured along the coast of Palermo (Sicily, Italy) that showed no signs of admixture with Japanese quails (59). The eggs were artificially incubated (incubator: MG 70/100 F, FIEM srl, Italia) and hatchlings reared under a 16:8 hrs light:dark cycle until they were 7 weeks of age. When they could be sexed (4 weeks of age), the birds were housed in sex-mixed groups of 11-12 in each enclosure (6 enclosures in total). Food (turkey starter, Lagerhaus, Austria) and water were always provided ad libitum throughout the course of the experiment. All birds were housed within a single room and the temperature was always maintained between 20-24°C. The experiment was performed in compliance with the Austria legislation with approval of the Ethics Committee of the University of Veterinary Medicine Vienna, and the Federal Ministry of Science, Research and Economy (BMWFW-68.205/0037-WF/V/3b/2017).
At seven weeks of age (indicated as experimental day 0), all birds part of the main experiment (n=68) were exposed to a gradual reduction of day length (30 min/week) until the photoperiod reached 12:12 hrs light:dark (experimental day 49) and was maintained constant until the end of the experiment. This light:dark schedule simulated autumn migration to (sub)tropical regions followed by a sedentary stage at the wintering grounds. As reported elsewhere (56), we determined the migratory state of the birds by regularly monitoring changes in body mass and subcutaneous fat scores over the entire experimental period. We found that quails increased their body mass (on average 22%) due to accumulation of body fat stores as the days were getting shorter (experimental days 0-56); while the birds rapidly lost body mass (on average 13%) due to a depletion of fat stores when the photoperiod was maintained constant – experimental days 70-105 (Figures 1A, B and Table S1 in Supplementary Material). We further assessed the migratory state of the birds 1-3 days prior blood sampling (when birds housed within a same enclosure where singly housed) via measurements of food intake and nocturnal activity levels or migratory restlessness (full methodological details in Supplementary Material). Briefly, food intake was estimated in each individually housed bird by measuring the amount of food eaten from the start of the single housing (10:00-11:00 h) until the following 24 hours; while migratory restlessness was estimated by an infrared sensor system connected with an activity recorder that registered locomotor activity within each cage at 1-min intervals. As expected, migratory birds showed higher levels of food intake and migratory restlessness compared the non-migratory birds (Figures 1C, D, Supplementary Material and Table S2).
Figure 1 Assessment of physiological migratory state in the captive population of common quails used in the main experiment (56) – birds aged seven weeks old when the experiment started (experimental day 0). (A) Changes in body mass and (B) total subcutaneous fat scores (assessed in the furcular, scapular and abdomen following Boswell et al. (55) over the experimental period; the arrow in both figures indicates the weekly reduction in daylight (experimental days 0-49); sample size from experimental days 0-56: 68 birds, sample size from experimental days 70-105: 35 birds. (C) Food intake (g) and (D) migratory restlessness (i.e. average nocturnal activity levels) separately by sampling group (n=33 migratory and 35 non-migratory) assessed when the birds were singly housed, 1-3 days prior blood sampling in the migratory group (experimental days 56-70) and the non-migratory group (experimental days 105-119). Data are presented as means ± se; * indicates p < 0.05.
Upon experimental day 56, 33 out of 68 birds were randomly chosen and allocated to the migratory sampling phase (experimental days 56-70); of this, 16 birds were sampled at 9:00 h (three hours after lights on), and 17 were sampled at 21:00 h (three hours after lights off). The remaining birds were allocated to the non-migratory sampling phase (experimental days 105-119); of this, 18 were sampled at 9:00 h and 17 were sampled at 21:00 h. We performed the hormonal measurements only on the subsets of birds sampled at 9:00h; samples collected at 21:00 h were meant to be used for other purposes (to be published elsewhere). Within each sampling phase, blood samples were taken over a consecutive period of twelve days in singly housed birds. We took blood samples (up to 400 µl) from a maximum of two birds in each sampling day to collect samples as close to the defined collection time as possible to control for variation due to circadian rhythms (60, 61) and to ensure reliable estimation of baseline physiological measurements (62). Thus, we collected blood samples within 2 min from entering the experimental room (mean ± se: 61.97 ± 1.38 sec, n=34; as expected, bleed time was not correlated with either corticosterone or ghrelin, -0.29 < Pearson’s r > 0.15, p ≥ 0.1) and immediately placed them on ice. Within 30 min from collection, blood samples were centrifuged to separate plasma from red blood cells. For each bird, we made two separate plasma aliquots; one aliquot (70-100 µl) was acidified using 1M HCL (dilution 1:10) before storing the samples at -80C for stabilization of acylated ghrelin in plasma as described in Goymann et al. (49).
Corticosterone was extracted two times in 1 ml of diethyl ether (Carl Roth, Austria) from plasma aliquots (~20 μl). After extraction, corticosterone concentrations (ng/ml) were measured using an enzyme immunoassay following the manufacturer instructions (Assay Designs Corticosterone Kit 901-097, Enzo Life Sciences). Samples were run in two plates (equally spread in relation to sampling group) and the average intra-assay and inter-assay variation calculated using a plasma quail pool run in both plates were 6.5% and 11.1%, respectively; the detection limit of the assay was 0.2 ng/ml.
Plasma ghrelin levels were measured using a radioimmunoassay (RIA) that has been described previously (63, 64). Acidified plasma was validated to use directly for the RIA by serial dilution of the plasma without extraction similar to tilapia (65). A primary antibody that recognizes the N-terminal portion of octanoylated rat ghrelin (Gly1–Arg11) was used at a final dilution of 1/5,000,000. Octanoylated chicken ghrelin (chicken ghrelin-26-C8), synthesized at Daiichi Suntory Pharma Co. Ltd., Institute for Medicinal Research and Development (Gunma, Japan), was used as the standard peptide instead of rat ghrelin. 125I-(Tyr29)- rat ghrelin was used as a tracer (15,000 cpm per tube). All samples were run in one unique assay and the intra-assay coefficients of variation was 5.4%; the detection limit of the assay was 0.5 fmol per tube. Due to insufficient plasma volumes, we lack corticosterone and ghrelin measurements in two samples (corticosterone: two males of each sampling phase, ghrelin: 2 non-migratory females).
Statistical analyses were performed in R v3.6.2 (66) integrated in RStudio v1.3.1093 (67). We first assessed the association between plasma ghrelin and plasma corticosterone using Pearson’s tests regardless of sampling group and separately by sampling group. We then performed separate General Linear Models (GLMs, built-in function in the R environment) to assess for differences in plasma ghrelin or plasma corticosterone concentrations in relation to the sampling group (migratory or non-migratory), sex and their interaction. Corticosterone data were log-transformed to improve normality of model residuals. As in the main study population the migratory birds were on average fatter, ingested more food, and were more active at night than non-migratory birds (Figure 1), in order to avoid covariation among these variables and sampling group we performed separate GLMs for each sampling group to assess for links between each hormone with respect to food intake and migratory restlessness. As body mass dynamics is an integrative tissue measure of migratory behavior (24), we also used similarly structured GLMs to test whether plasma ghrelin and plasma corticosterone predicted either the total amount of body mass gained until the birds reached their fuelling peak (i.e., body mass change between experimental day 0 and the day in which the birds allocated to the migratory group were singly housed, herein referred as “total body mass gained”) or the total amount of body mass depleted as they entered the non-migratory status (i.e., body mass change between experimental day 70 and the day in which birds allocated to the non-migratory group were singly housed – herein referred as “total body mass lost”). As both corticosterone and ghrelin can also induce short-term changes in fuel metabolism e.g., (68, 69), we performed two additional models to explore whether levels of plasma ghrelin and plasma corticosterone would reflect within-individual changes in body mass detected over the last two-four weeks preceding blood sampling (migratory birds: body mass gained between experimental day 42 and the day of single housing – herein referred as “recently gained body mass”; non-migratory birds: body mass lost between experimental day 91 and the day of single housing – herein referred as “recently lost body mass”). We note that there was no association between either plasma ghrelin or corticosterone and variability in sampling date within each sampling group (migratory: -0.31< Pearson’s r < 0.36, p ≥ 0.17, non-migratory: -0.15 < Pearson’s r < -0.02, p ≥ 0.59) and thus we did not enter sampling date in the GLMs. In all models, non-significant interactions (p > 0.05) were sequentially dropped from the final models. All models met the assumptions of normality and homogeneity, which were assessed via graphical diagnostics of the residuals (70). Unless otherwise specified, descriptive statistics are provided as means ± se.
We found no correlation between plasma acylated ghrelin levels and plasma corticosterone (Pearson r = -0.12, p = 0.5; separately by sampling group: Pearson r > -0.11, p > 0.3). Migratory quails of both sexes had remarkably higher plasma ghrelin concentrations compared to quails sampled during the non-migratory phase (p = 0.004, Table 1A and Figure 2A), while we found no effect of sampling phase nor sex on plasma corticosterone (Table 1B; Figure 2B). Within each sampling group, we found no association of plasma ghrelin or plasma corticosterone with either food intake or migratory restlessness (Tables 2A, B). In the migratory sampling phase, the total amount of body mass gained was not associated with either plasma corticosterone or plasma ghrelin (Table 2A and Figure 3A), though plasma ghrelin but not plasma corticosterone did correlate with the recently gained body mass (Table 2A and Figure 3B). In the non-migratory sampling phase, the total amount of body mass lost was negatively associated with plasma ghrelin (p = 0.03, Table 2B and Figure 3C), but not with plasma corticosterone (Table 2B). Similarly, lower levels of plasma ghrelin also reflected larger recent losses in body mass (p = 0.02, Table 2B and Figure 3D).
Table 1 Results of GLMs assessing the effects of sampling phase and sex on plasma concentrations of (a) ghrelin or (b) corticosterone.
Figure 2 Concentrations of plasma ghrelin (A) and plasma corticosterone (B) in relation to sampling phase. Data are shown as mean ± se; * indicates p < 0.05.
Table 2 Results of GLMs, separately by sampling group, assessing the effects of plasma corticosterone, plasma ghrelin and sex on migratory status assessed through changes in body mass, levels of food intake and migratory restlessness (for full details on study design see Material and Methods).
Figure 3 Correlation plots between concentrations of plasma ghrelin and changes in body mass during the migratory phase (A, B) or the non-migratory phase (C, D). In (A) the total body mass gain was calculated as the difference in body mass between the time in which migratory birds were singly housed for blood sampling (experimental days 56-70) and experimental day 0; in (B) the recent body mass gain was calculated as the difference in body mass between the day of single housing and experimental day 42; in (C) the total body mass loss is the difference between the time in which non-migratory birds were singly housed for blood sampling (experimental days 105-119) and experimental day 70, and in (D) the recent body mass loss was calculated as the difference in body mass between the day of single housing and experimental day 91. Grey area in the graphs represents 95% confidence interval.
In this study, we exposed Common quails to controlled changes in day length to simulate autumn migration followed by a non-migratory wintering phase. We compared plasma corticosterone and ghrelin concentrations between the two sampling phases and assessed whether these two metabolic hormones vary between migratory states. In accordance with our predictions, we found that the emergence of the migratory phenotype was associated with higher levels of plasma ghrelin and that plasma ghrelin was associated with changes in body mass as birds transitioned into the autumnal migratory state, or the wintering non-migratory state. Contrary to our predictions, we observed no correlation between ghrelin and circulating levels of the metabolic hormone corticosterone, which also did not differ between migratory and non-migratory birds and was not associated with changes in body mass, food intake or migratory restlessness that accompanied the switching of the migratory phenotype.
This study allowed us to cross check function of ghrelin known for domestic Galliformes with the recent findings on few migratory Passerines by studying a migratory Galliform in controlled laboratory conditions. The elevation of plasma ghrelin in the fattened migratory quails relative to the leaner non-migratory quails we found is in line with the garden warbler study by Goymann et al. (49) showing a positive relationship between plasma ghrelin and fat stores in spring migrating birds caught at a stopover site. On the other hand, our results are in contrast with the study by Eikenaar et al. (51) in common blackbirds (Turdus merula) in which such association was not found in birds sampled at a stopover during autumn migration. However, it is difficult to draw a direct comparison between the previous studies on ghrelin in migratory birds, which were all performed in actively migrating birds, and ours that instead involved long-term housing of birds and manipulation of photoperiod conditions to induce the transitioning of the migratory state. Regardless, an important caveat of our study design is that the nutritional and migratory state of the birds were inter-connected given that all migratory birds were sampled at a standardised time, when they reached their peak in body mass/fat stores. We cannot, therefore, rule out the possibility that plasma ghrelin might simply reflect the birds’ nutritional conditions without necessarily having a role in the mediation of migratory behaviour. However, this possibility appears somewhat unlikely given that we also found associations between plasma ghrelin and changes in body mass within the two sampling phases. Specifically, while plasma ghrelin did not covary with the total amount of body mass gained since the beginning until completion of the fattening process, it did positively covary with the body mass gained over its intermediate/final stages (i.e., recent body mass gain) in partially fattened birds. Despite this finding is based on a correlative approach, it raises the intriguing possibility that elevated levels of ghrelin might contribute to inhibit further pre-migratory fuelling as the injection of chicken ghrelin induces short-term anorectic effects by stimulating the breakdown of lipids (46, 64) and rapidly stimulates migratory movements in the wild (50). This possibility is further supported by the associations we found between ghrelin and the rapid depletion in body mass observed within the birds sampled during the non-migratory phase, when we observed lower levels of ghrelin now reflecting larger losses in body mass both over the long- and short-time frame (i.e., over an average of six or three weeks preceding blood sampling, respectively). These results suggest that ghrelin may regulate body mass via a negative feedback loop with high circulating concentrations of ghrelin exerting inhibitory effects on further increases in fattening. Nevertheless, the exact mechanisms underlying the regulation of food intake and avian migratory fattening by the ghrelin axis remain to be fully elucidated. The lack of correlation of plasma ghrelin with both food intake and levels of migratory restlessness that we assessed in close proximity of blood sampling (1-3 days earlier) could be interpreted as contrasting with the latter hypothesis. However, this apparent contrast could be due to limited variance within the two sampling groups as all migratory birds were sampled at their peak of body mass and when migratory restlessness levels were high, while all non-migratory birds were sampled when most of the accumulated fuel reserves had been depleted and when migratory restlessness levels were low. In future work, a repeated sampling design within the same individual birds will be one among the key necessary steps to experimentally verify whether ghrelin has a functional/causative role in mediating fuelling dynamics typical of phenotypic transitions between migratory states.
Contrary to our prediction, circulating levels of corticosterone were not elevated in migratory birds compared to non-migratory birds. This prediction was mostly based on previous work in captive birds reporting a relatively large up-regulation in corticosterone secretion, especially detected in migrants that had reached stable and high levels of body mass at completion of pre-migratory fuelling (24, 26, 29, 30, 34). It is possible that the variance due to inter-individual variation in baseline levels of corticosterone (71) has masked the emergence of potential differences in corticosterone between migratory and non-migratory quails. However, we did not observe in our data a large variance in corticosterone levels, which were always very low and in a range comparable with baseline values reported for the closely related Japanese quail e.g., (72, 73). We also did not find associations between corticosterone levels and variability in sampling date within each sampling group. The neat study by Piersma et al. (30) in the Red knots demonstrated a rapid decline in plasma levels of corticosterone as soon as the fattened migrants started depleting body mass over the first seven days preceding blood sampling. As we performed blood sampling in the migratory birds over two consecutive weeks (experimental days 56-70), it could be argued that the depletion in energy reserves had already started in some birds, thus reducing our statistical power to detect an up-regulation in corticosterone secretion. However, we can exclude this potential confounding factor because body mass remained high, or even slightly increased, during this time-frame, and the signs of body mass depletion were only observed after experimental day 70. Thus, in our study species it seems unlikely that corticosterone up-regulation (at least of the magnitude reported in previous work) contribute in sustaining high levels of pre-migratory fuelling. On the other hand, in accordance with many previous studies [reviewed in (24); e.g (30, 32, 74, 75)], we found that circulating levels of corticosterone did not reflect amounts of body mass gained or depleted before blood sampling as birds transitioned to the autumnal migratory state or to the wintering non-migratory state, respectively. These results provide further support to the idea that corticosterone has a very limited, if any, role in stimulating physiological preparations for migration (24). Corticosterone could, however, have a role in promoting or sustaining migratory departure as studies conducted in the wild suggested (76–79). Here, we did not find a correlation between plasma corticosterone and migratory restlessness, despite the latter being higher in the migratory birds. This apparent discrepancy could be due to several aspects of study design associated to our temporal sampling scale. Importantly, we did not perform a long-term tracking of activity levels over the transitioning phases and we did not take corticosterone measurements during or just before the hours in which migratory restlessness is actually expressed (i.e., afternoon, or night given that quails are nocturnal migrants (54) when corticosterone levels would be predicted to be higher.
We did not find a relationship between circulating levels of plasma ghrelin and plasma corticosterone. We predicted such a relationship to be positive because the peripheral administration of ghrelin induces corticosterone release in the closely related chickens (46–48). Despite plasma ghrelin was relatively high in the migratory birds, it is possible that such increase was not large enough to induce a positive feedback on the Hypothalamic-Pituitary-Adrenal axis (HPA axis) and further manipulative experiments would be needed to clarify if ghrelin has glucocorticoid stimulatory effects. We cannot exclude the possibility that a potential relationship between ghrelin and corticosterone was missed due to a lag in the response time, as it would happen if peaks in ghrelin and corticosterone secretion occur at different times respect to changes in body mass associated to different migratory states. Again, a repeated-measure design experiment would be extremely useful to test this possibility. Nevertheless, our results are consistent with the only previous study that tested a functional relationship between ghrelin and corticosterone in a migratory bird, which reported no correlation between plasma levels of the two hormones in migrants close to depart at stopover (51). Further studies will be needed to clarify whether there is (or not) a functional interplay between ghrelin and corticosterone in relation to modulation of migratory behaviour.
The original contributions presented in the study are included in the article/Supplementary Material. Further inquiries can be directed to the corresponding author.
The animal study was reviewed and approved by Ethics Committee of the University of Veterinary Medicine Vienna, and the Federal Ministry of Science, Research and Economy (BMWFW-68.205/0037-WF/V/3b/2017).
Conceptualization: VM and LF; Methodology: VM, HK, GP, and LF; Formal analysis: VM; Resources: VM, HK, and LF; Writing – original draft: VM; Writing – review & editing: VM, HK, and LF; Project administration: VM; Funding acquisition: VM and LF. All authors contributed to the article and approved the submitted version.
The work was funded by a Marie Sklodowska-Curie Individual Fellowship to VM (grant Nr. 704582), and by intramural funds and an Austrian Science Fund (FWF) (grant Nr. 31947-B29) to LF. V.M. was additionally supported by a FWF Lise Meitner Fellowship (grant Nr. M2520-B29).
We thank Wolfgang Pegler and Christa Grabmayer for assistance with the animal husbandry, Roland Sasse for help in building the animal cages, Aryan Havrest for help during the animal experiment, Renate Hengsberger for her support with the formatting of the references.
HK was employed by Grandsoul Research Institute for Immunology, Inc.
The remaining authors declare that the research was conducted in the absence of any commercial or financial relationships that could be construed as a potential conflict of interest.
All claims expressed in this article are solely those of the authors and do not necessarily represent those of their affiliated organizations, or those of the publisher, the editors and the reviewers. Any product that may be evaluated in this article, or claim that may be made by its manufacturer, is not guaranteed or endorsed by the publisher.
The Supplementary Material for this article can be found online at: https://www.frontiersin.org/articles/10.3389/fendo.2022.1058298/full#supplementary-material
1. McWilliams SR, Karasov WH. Phenotypic flexibility in digestive system structure and function in migratory birds and its ecological significance. Comp Biochem Physiol A (2001) 128(3):579–93. doi: 10.1016/S1095-6433(00)00336-6
2. Cornelius JM, Boswell T, Jenni-Eiermann S, Breuner CW, Ramenofsky M. Contributions of endocrinology to the migration life history of birds. Gen Comp Endocrinol (2013) 190:47–60. doi: 10.1016/j.ygcen.2013.03.027
3. Blem CR. Avian energy storage. In: Power DM, editor. Current ornithology, vol. 7. New York Plenum Press (1990). p. 59–113.
4. McWilliams SR, Guglielmo C, Pierce B, Klaassen M. Flying, fasting, and feeding in birds during migration: A nutritional and physiological ecology perspective. J Avian Biol (2004) 35(5):377–93. doi: 10.1111/j.0908-8857.2004.03378.x
5. Jenni L, Jenni-Eiermann S. Fuel supply and metabolic constraints in migrating birds. J Avian Biol (1998) 29(4):521–8. doi: 10.2307/3677171
6. Guglielmo CG. Obese super athletes: Fat-fueled migration in birds and bats. J Exp Biol (2018) 221:jeb165753. doi: 10.1242/jeb.165753
7. Jenni-Eiermann S, Jenni L. High plasma triglyceride levels in small birds during migratory flight: A new pathway for fuel supply during endurance locomotion at very high mass-specific metabolic rates? Physiol Zool (1992) 65(1):112–23. doi: 10.1086/physzool.65.1.30158242
8. Bairlein F. How to get fat: Nutritional mechanisms of seasonal fat accumulation in migratory songbirds. Naturwissenschaften (2002) 89:1–10. doi: 10.1007/s00114-001-0279-6
9. Jenni L, Schaub M. Behavioural and physiological reactions to environmental variation in bird migration: A review. In: Berthold P, Gwinner E, Sonnenschein E, editors. Avian migration. Berlin Heidelberg New York: Springer (2003). p. 155–71. doi: 10.1007/978-3-662-05957-9_10
10. Fusani L, Cardinale M, Carere C, Goymann W. Stopover decision during migration: Physiological conditions predict nocturnal restlessness in wild passerines. Biol Lett (2009) 5(3):302–5. doi: 10.1098/rsbl.2008.0755
11. Eikenaar C, Schläfke JL. Size and accumulation of fuel reserves at stopover predict nocturnal restlessness in a migratory bird. Biol Lett (2013) 9(6):20130712. doi: 10.1098/rsbl.2013.0712
12. Lupi S, Goymann W, Cardinale M, Fusani L. Physiological conditions influence stopover behaviour of short-distance migratory passerines. J Ornithol (2016) 157(2):583–9. doi: 10.1007/s10336-015-1303-5
13. Sjöberg S, Alerstam T, Åkesson S, Schulz A, Weidauer A, Coppack T, et al. Weather and fuel reserves determine departure and flight decisions in passerines migrating across the Baltic Sea. Anim Behav (2015) 104:59–68. doi: 10.1016/j.anbehav.2015.02.015
14. Farner DS. The annual stimulus for migration: Experimental and physiologic aspects. In: Wolfson A, editor. Recent studies in avian biology. Urbana, IL: University of Illinois Press (1955). p. 198–237.
15. King JR, Farner DS. Bioenergetic basis of light-induced fat deposition in the white-crowned sparrow. Proc Soc Exp Biol Med (1956) 93(2):354–9. doi: 10.3181/00379727-93-22755
16. Gwinner E. Circadian and circannual programmes in avian migration. J Exp Biol (1996) 199(Pt 1):39–48. doi: 10.1242/jeb.199.1.39
17. Helm B, Gwinner E. Migratory restlessness in an equatorial nonmigratory bird. PloS Biol (2006) 4(4):e110. doi: 10.1371/journal.pbio.0040110
18. Dawson A. Seasonality in a temperate zone bird can be entrained by near equatorial photoperiods. Proc R Soc Lond B (2007) 274(1610):721–5. doi: 10.1098/rspb.2006.0067
19. Wingfield JC, Maney DL, Breuner CW, Jacobs JD, Sharon L, Ramenofsky M, et al. Ecological bases of hormone-behavior interactions: The "Emergency life history stage". Am Zool (1998) 38(1):191–206. doi: 10.1093/icb/38.1.191
20. Breuner CW, Wingfield JC, Hahn TP. Corticosterone and irruptive migration in free-living mountain white-crowned sparrows (Zonotrichia ieucophrys oriantha). Am Zool (1998) 38(5):23A. https://www.jstor.org/stable/40783821
21. Sapolsky RM, Romero LM, Munck AU. How do glucocorticoids influence stress responses? integrating permissive, suppressive, stimulatory, and preparative actions. Endocr Rev (2000) 21(1):55–89. doi: 10.1210/edrv.21.1.0389
22. Angelier F, Clement-Chastel C, Gabrielsen GW, Chastel O. Corticosterone and time-activity budget: An experiment with black-legged kittiwakes. Horm Behav (2007) 52(4):482–91. doi: 10.1016/j.yhbeh.2007.07.003
23. Ramenofsky M. Hormones in migration and reproductive cycles of birds. In: Norris DO, Lopez KH, editors. Hormones and reproduction of vertebrates. 4 - birds. London: Academic Press (2011). p. 205–37. doi: 10.1016/B978-0-12-374929-1.10008-3
24. Bauer CM, Watts HE. Corticosterone's roles in avian migration: Assessment of three hypotheses. Horm Behav (2021) 135:105033. doi: 10.1016/j.yhbeh.2021.105033
25. Holberton RL, Wilson CM, Hunter MJ, Cash WB, Sims CG. The role of corticosterone in supporting migratory lipogenesis in the dark-eyed junco, Junco hyemalis: A model for central and peripheral regulation. Physiol Biochem Zool (2007) 80(1):125–37. doi: 10.1086/508816
26. Landys MM, Piersma T, Ramenofsky M, Wingfield JC. Role of low-affinity glucocorticoid receptor in the regulation of behavior and energy metabolism in the migratory red knot calidris canutus Islandica Physiol Biochem Zool (2004) 77(4):658–68. doi: 10.1086/420942
27. Landys MM, Ramenofsky M, Guglielmo CG, Wingfield JC. The low-affinity glucocorticoid receptor regulates feeding and lipid breakdown in the migratory gambel's white-crowned sparrow Zonotrichia leucophrys gambelii J Exp Biol (2004) 207(1):143–54. doi: 10.1242/jeb.00734
28. Eikenaar C. Endocrine regulation of fueling by hyperphagia in migratory birds. J Comp Physiol A (2017) 203(6-7):439–45. doi: 10.1007/s00359-017-1152-1
29. Holberton RL, Boswell T, Hunter MJ. Circulating prolactin and corticosterone concentrations during the development of migratory condition in the dark-eyed junco Junco hyemalis Gen Comp Endocrinol (2008) 155(3):641–9. doi: 10.1016/j.ygcen.2007.11.001
30. Piersma T, Reneerkens J, Ramenofsky M. Baseline corticosterone peaks in shorebirds with maximal energy stores for migration: A general preparatory mechanism for rapid behavioral and metabolic transitions? Gen Comp Endocrinol (2000) 120(1):118–26. doi: 10.1006/gcen.2000.7543
31. Fudickar AM, Greives TJ, Atwell JW, Stricker CA, Ketterson ED. Reproductive allochrony in seasonally sympatric populations maintained by differential response to photoperiod: Implications for population divergence and response to climate change. Am Nat (2016) 187(4):436–46. doi: 10.1086/685296
32. Ramenofsky M, Campion AW, Pérez JH, Krause JS, Németh Z. Behavioral and physiological traits of migrant and resident white-crowned sparrows: A common garden approach. J Exp Biol (2017) 220(7):1330–40. doi: 10.1242/jeb.148171
33. Eikenaar C, Müller F, Rüppel G, Stöwe M. Endocrine regulation of migratory departure from stopover: Evidence from a longitudinal migratory restlessness study on northern wheatears. Horm Behav (2018) 99:9–13. doi: 10.1016/j.yhbeh.2018.01.008
34. Holberton RL. Changes in patterns of corticosterone secretion concurrent with migratory fattening in a Neotropical migratory bird. Gen Comp Endocrinol (1999) 116(1):49–58. doi: 10.1006/gcen.1999.7336
35. Eikenaar C, Fritzsch A, Bairlein F. Corticosterone and migratory fueling in northern wheatears facing different barrier crossings. Gen Comp Endocrinol (2013) 186:181–6. doi: 10.1016/j.ygcen.2013.02.042
36. Kojima M, Hosoda H, Date Y, Nakazato M, Matsuo H, Kangawa K. Ghrelin is a growth-Hormone-Releasing acylated peptide from stomach. Nature (1999) 402(6762):656–60. doi: 10.1038/45230
37. Hosoda H, Kojima M, Kangawa K. Biological, physiological, and pharmacological aspects of ghrelin. J Pharmacol Sci (2006) 100(5):398–410. doi: 10.1254/jphs.CRJ06002X
38. Kaiya H, Miyazato M, Kangawa K, Peter RE, Unniappan S. Ghrelin: A multifunctional hormone in non-mammalian vertebrates. Comp Biochem Physiol A (2008) 149(2):109–28. doi: 10.1016/j.cbpa.2007.12.004
39. Chopin LK, Seim I, Walpole CM, Herington AC. The ghrelin axis–does it have an appetite for cancer progression? Endocr Rev (2012) 33(6):849–91. doi: 10.1210/er.2011-1007
40. Wren AM, Seal LJ, Cohen MA, Brynes AE, Frost GS, Murphy KG, et al. Ghrelin enhances appetite and increases food intake in humans. J Clin Endocrinol Metab (2001) 86(12):5992–. doi: 10.1210/jcem.86.12.8111
41. Cummings DE. Ghrelin and the short- and long-term regulation of appetite and body weight. Physiol Behav (2006) 89(1):71–84. doi: 10.1016/j.physbeh.2006.05.022
42. Wang Q, Liu C, Uchida A, Chuang J-C, Walker A, Liu T, et al. Arcuate agrp neurons mediate orexigenic and glucoregulatory actions of ghrelin. Mol Metab (2014) 3(1):64–72. doi: 10.1016/j.molmet.2013.10.001
43. Furuse M, Tachibana T, Ohgushi A, Ando R, Yoshimatsu T, Denbow DM. Intracerebroventricular injection of ghrelin and growth hormone releasing factor inhibits food intake in neonatal chicks. Neurosci Lett (2001) 301(2):123–6. doi: 10.1016/S0304-3940(01)01621-4
44. Shousha S, Nakahara K, Kojima M, Miyazato M, Hosoda H, Kangawa K, et al. Different effects of peripheral and central ghrelin on regulation of food intake in the Japanese quail. Gen Comp Endocrinol (2005) 141(2):178–83. doi: 10.1016/j.ygcen.2004.12.021
45. Kaiya H, Kangawa K, Miyazato M. Update on ghrelin biology in birds. Gen Comp Endocrinol (2013) 190:170–5. doi: 10.1016/j.ygcen.2013.04.014
46. Buyse J, Janssen S, Geelissen S, Swennen Q, Kaiya H, Darras VM, et al. Ghrelin modulates fatty acid synthase and related transcription factor mrna levels in a tissue-specific manner in neonatal broiler chicks. Peptides (2009) 30(7):1342–7. doi: 10.1016/j.peptides.2009.04.015
47. Kaiya H, van der Geyten S, Kojima M, Hosoda H, Kitajima Y, Matsumoto M, et al. Chicken ghrelin: Purification, cdna cloning, and biological activity. Endocrinology (2002) 143(9):3454–63. doi: 10.1210/en.2002-220255
48. Saito ES, Kaiya H, Tachibana T, Tomonaga S, Denbow DM, Kangawa K, et al. Inhibitory effect of ghrelin on food intake is mediated by the corticotropin-releasing factor system in neonatal chicks. Regul Pept (2005) 125(1-3):201–8. doi: 10.1016/j.regpep.2004.09.003
49. Goymann W, Lupi S, Kaiya H, Cardinale M, Fusani L. Ghrelin affects stopover decisions and food intake in a long-distance migrant. Proc Natl Acad Sci USA (2017) 1148(8):1946–51. doi: 10.1073/pnas.1619565114
50. Lupi S, Morbey YE, MacDougall-Shackleton SA, Kaiya H, Fusani L, Guglielmo CG. Experimental ghrelin administration affects migratory behaviour in a songbird. Horm Behav (2022) 141:105139. doi: 10.1016/j.yhbeh.2022.105139
51. Eikenaar C, Hessler S, Ballstaedt E, Schmaljohann H, Kaiya H. Ghrelin, corticosterone and the resumption of migration from stopover, an automated telemetry study. Physiol Behav (2018) 194:450–5. doi: 10.1016/j.physbeh.2018.06.036
52. Perennou C. European Union management plan 2009-2011. Common quail Coturnix coturnix. Luxembourg European Commission (2009). 71 p.
53. Cramp S, Simmons KEL. Handbook of the birds of Europe, the middle East and north Africa. Birds Western Palaearctic vol. 2 Hawks to Bustards Oxford (1980). 695 p
54. Bertin A, Houdelier C, Richard-Yris MA, Guyomarc'h C, Lumineau S. Stable individual profiles of daily timing of migratory restlessness in European quail. Chronobiol Int (2007) 24(2):253–67. doi: 10.1080/07420520701283685
55. Boswell T, Hall MR, Goldsmith AR. Annual cycles of migratory fattening, reproduction and moult in European quail (Coturnix coturnix). J Zool (1993) 231(4):627–44. doi: 10.1111/j.1469-7998.1993.tb01943.x
56. Marasco V, Sebastiano M, Costantini D, Pola G, Fusani L. Controlled expression of the migratory phenotype affects oxidative status in birds. J Exp Biol (2021) 224(6):jeb233486. doi: 10.1242/jeb.233486
57. Nadal J, Sáez D, Margalida A. Crossing artificial obstacles during migration: The relative global ecological risks and interdependencies illustrated by the migration of common quail Coturnix coturnix Sci Total Environ (2022) 808:152173. doi: 10.1016/j.scitotenv.2021.152173
58. Ball GF, Balthazart J. Japanese Quail as a model system for studying the neuroendocrine control of reproductive and social behaviors. ILAR J (2010) 51(4):310–25. doi: 10.1093/ilar.51.4.310
59. Smith S, Fusani L, Boglarka B, Sanchez-Donoso I, Marasco V. Lack of introgression of Japanese quail in a captive population of common quail. Eur J Wildl Res (2018) 64(5):51. doi: 10.1007/s10344-018-1209-7
60. Breuner CW, Wingfield JC, Romero LM. Diel rhythms of basal and stress-induced corticosterone in a wild, seasonal vertebrate, gambel’s white-crowned sparrow. J Exp Zool (1999) 284(3):334–42. doi: 10.1002/(SICI)1097-010X(19990801)284:3<334::AID-JEZ11>3.0.CO;2-%23
61. Bodosi B, Gardi J, Hajdu I, Szentirmai E, Obal F, Krueger JM. Rhythms of ghrelin, leptin, and sleep in rats: Effects of the normal diurnal cycle, restricted feeding, and sleep deprivation. Am J Physiol Reg Int Comp Physiol (2004) 287(5):R1071–R9. doi: 10.1152/ajpregu.00294.2004
62. Wingfield JC, Smith JP, Farner DS. Endocrine responses of white-crowned sparrows to environmental stress. Condor (1982) 84(4):399–409. doi: 10.2307/1367443
63. Hosoda H, Kojima M, Matsuo H, Kangawa K. Ghrelin and des-acyl ghrelin: Two major forms of rat ghrelin peptide in gastrointestinal tissue. Biochem Biophys Res Commun (2000) 279(3):909–13. doi: 10.1006/bbrc.2000.4039
64. Kaiya H, Saito ES, Tachibana T, Furuse M, Kangawa K. Changes in ghrelin levels of plasma and proventriculus and ghrelin mrna of proventriculus in fasted and refed layer chicks. Domest Anim Endocr (2007) 32(4):247–59. doi: 10.1016/j.domaniend.2006.03.006
65. Riley LG, Fox BK, Breves JP, Kaiya H, Dorough CP, Hirano T, et al. Absence of effects of short-term fasting on plasma ghrelin and brain expression of ghrelin receptors in the tilapia, Oreochromis mossambicus. Zoolog Sci (2008) 25(8):821–7. doi: 10.2108/zsj.25.821
66. R Core Team. R: A language and environment for statistical computing. Vienna, Austria: R Foundation for Statistical Computing (2022). Available at: http://www.R-project.org/.
67. R Studio Team. Rstudio: Integrated development for R. PBC, Boston, MA: RStudio (2022). Available at: http://www.rstudio.com/.
68. Simon J. Effects of daily corticosterone injections upon plasma glucose, insulin, uric acid and electrolytes and food intake pattern in the chicken. Diabete Metab (1984) 10(3):211–7. http://europepmc.org/abstract/MED/6386560
69. Al Massadi O, López M, Tschöp M, Diéguez C, Nogueiras R. Current understanding of the hypothalamic ghrelin pathways inducing appetite and adiposity. Trends Neurosci (2017) 40(3):167–80. doi: 10.1016/j.tins.2016.12.003
70. Zuur AF, Ieno EN, Walker NJ, Saveliev AA, Smith GM. Mixed efffects models and extensions in ecology with R. New York Springer (2009).
71. Ouyang JQ, Hau M, Bonier F. Within seasons and among years: When are corticosterone levels repeatable? Horm Behav (2011) 60(5):559–64. doi: 10.1016/j.yhbeh.2011.08.004
72. Marasco V, Robinson J, Herzyk P, Spencer KA. Pre- and post-natal stress in context: Effects on the stress physiology in a precocial bird. J Exp Biol (2012) 215(22):3955–64. doi: 10.1242/jeb.071423
73. Zimmer C, Boogert NJ, Spencer KA. Developmental programming: Cumulative effects of increased pre-hatching corticosterone levels and post-hatching unpredictable food availability on physiology and behaviour in adulthood. Horm Behav (2013) 64(3):494–500. doi: 10.1016/j.yhbeh.2013.07.002
74. Henshaw I, Fransson T, Jakobsson S, Jenni-Eiermann S, Kullberg C. Information from the geomagnetic field triggers a reduced adrenocortical response in a migratory bird. J Exp Biol (2009) 212(18):2902–7. doi: 10.1242/jeb.033332
75. Robart AR, Morado MI, Watts HE. Declining food availability, corticosterone, and migratory response in a nomadic, irruptive migrant. Horm Behav (2019) 110:56–67. doi: 10.1016/j.yhbeh.2019.02.007
76. Lõhmus M, Sandberg R, Holberton RL, Moore FR. Corticosterone levels in relation to migratory readiness in red-eyed vireos (Vireo olivaceus). Behav Ecol Sociobiol (2003) 54:233–9. doi: 10.1007/s00265-003-0618-z
77. Eikenaar C, Klinner T, Stöwe M. Corticosterone predicts nocturnal restlessness in a long-distance migrant. Horm Behav (2014) 66(2):324–9. doi: 10.1016/j.yhbeh.2014.06.013
78. Eikenaar C, Müller F, Leutgeb C, Hessler S, Lebus K, Taylor PD, et al. Corticosterone and timing of migratory departure in a songbird. Proc R Soc Lond B (2017) 284(1846):20162300. doi: 10.1098/rspb.2016.2300
Keywords: phenotypic flexibility, avian migration, migratory state, migratory fuelling, ghrelin, corticosterone
Citation: Marasco V, Kaiya H, Pola G and Fusani L (2023) Ghrelin, not corticosterone, is associated with transitioning of phenotypic states in a migratory Galliform. Front. Endocrinol. 13:1058298. doi: 10.3389/fendo.2022.1058298
Received: 30 September 2022; Accepted: 28 November 2022;
Published: 09 January 2023.
Edited by:
Tracy A. Larson, University of Virginia, United StatesReviewed by:
Melissa Lin Grunst, Université de la Rochelle, FranceCopyright © 2023 Marasco, Kaiya, Pola and Fusani. This is an open-access article distributed under the terms of the Creative Commons Attribution License (CC BY). The use, distribution or reproduction in other forums is permitted, provided the original author(s) and the copyright owner(s) are credited and that the original publication in this journal is cited, in accordance with accepted academic practice. No use, distribution or reproduction is permitted which does not comply with these terms.
*Correspondence: Valeria Marasco, dmFsZXJpYS5tYXJhc2NvQHZldG1lZHVuaS5hYy5hdA==
Disclaimer: All claims expressed in this article are solely those of the authors and do not necessarily represent those of their affiliated organizations, or those of the publisher, the editors and the reviewers. Any product that may be evaluated in this article or claim that may be made by its manufacturer is not guaranteed or endorsed by the publisher.
Research integrity at Frontiers
Learn more about the work of our research integrity team to safeguard the quality of each article we publish.