- 1Department of Endocrinology, Chongqing Emergency Medical Center, Chongqing University Central Hospital, Chongqing, China
- 2Department of Endocrinology, The Second Affiliated Hospital of Chongqing Medical University, Chongqing, China
- 3Department of Endocrinology, Banan People’s Hospital of Chongqing, Chongqing, China
- 4Department of Endocrinology, Chongqing Southwest Hospital, Chongqing, China
Background: The long-term clinical outcome of poor prognosis in patients with diabetic hyperglycaemic crisis episodes (HCE) remains unknown, which may be related to acute organ injury (AOI) and its continuous damage after hospital discharge. This study aimed to observe the clinical differences and relevant risk factors in HCE with or without AOI.
Methods: A total of 339 inpatients were divided into an AOI group (n=69) and a non-AOI group (n=270), and their differences and risk factors were explored. The differences in clinical outcomes and prediction models for evaluating the long-term adverse events after hospital discharge were established.
Results: The mortality among cases complicated by AOI was significantly higher than that among patients without AOI [8 (11.59%) vs. 11 (4.07%), Q = 0.034] during hospitalization. After a 2-year follow-up, the mortality was also significantly higher in patients with concomitant AOI than in patients without AOI after hospital discharge during follow-up [13 (21.31%) vs. 15 (5.8%), Q < 0.001]. The long-term adverse events in patients with concomitant AOI were significantly higher than those in patients without AOI during follow-up [15 (24.59%) vs. 31 (11.97%), Q = 0.015]. Furthermore, Blood β-hydroxybutyric acid (P = 0.003), Cystatin C (P <0.001), serum potassium levels (P = 0.001) were significantly associated with long-term adverse events after hospital discharge.
Conclusions: The long-term prognosis of HCE patients complicated with AOI was significantly worse than that of HCE patients without AOI. The laboratory indicators were closely correlated with AOI, and future studies should explore the improvement of clinical outcome in response to timely interventions.
Introduction
Hyperglycaemic crisis episodes (HCE) refer to an acute diabetic complication that is most likely to cause short-term death. Diabetic ketoacidosis (DKA) and hyperosmolar hyperglycaemia syndrome have a morbidity of approximately 2.6% and 0.2% of diabetes, respectively. The morbidity of diabetes remains high, resulting in an annually increasing number of hospitalizations because of HCE, as well as high medical expenditure and severe social burden (1, 2). HCE patients are prone to acute organ injury (AOI), which involves myocardial infarction, acute kidney injury (AKI), and neurological injury. The risk of mortality will be significantly increased in HCE patients complicated with neurological changes (3, 4). In addition, the risk of organ failure and mortality after hospital discharge will also be considerably increased in HCE patients complicated with AOI (3, 5–7). The possible reason may be related to the continuous damage caused by AOI in the hospital and its extension to hospital discharge. Although HCE has been confirmed to increase the risk of dementia, malignancy, end-stage renal disease and cerebral ischaemic injury in previous studies (4, 8, 9), the impact of different AOIs on long-term outcomes in patients with HCE remains unknown. In this work, a prospective, multicentre follow-up observational study was conducted on inpatient HCE with or without AOI, and the adverse events after hospital discharge were investigated.
Methods
Patient recruitment and exclusion criteria
A total of 339 HCE patients were enrolled from 4 tertiary teaching hospitals between August 2017 and July 2020 in accordance with the principles of the Declaration of Helsinki (2001). This study was registered at chictr.org.cn (ChiCTR1800015981) and approved by the institutional review board of Chongqing University Central Hospital. The study was approved by the Committee for Research Ethics of Chongqing University Central Hospital (NO. 202262).Written informed consent was obtained from all participants.
Inpatients aged 20 or older diagnosed with HCE were enrolled in the study. All patients met the diagnostic criteria for diabetes and HCE recommended by the American Diabetes Association (ADA) in 2009 (10). Subjects with the following conditions or diseases were excluded: 1) pregnancy; 2) history of stroke/cognitive impairment, CKD, tumour, coronary heart disease/heart failure, chronic pancreatitis, or chronic hepatitis/liver failure; and 3) other major diseases, including systemic inflammatory response syndrome or advanced malignant diseases.
AOIs included the following: 1) acute stroke or brain dysfunction; 2) acute kidney injury; 3) acute cardiovascular accident/heart failure; 4) acute pancreatitis; and 5) acute liver dysfunction/failure. According to whether HCE was concomitant with AOI during admission, the patients were divided into an AOI group and a non-AOI group, and their differences and risk factors were explored. Long-term adverse events after hospital discharge were defined as follows: 1) stroke or cognitive impairment; 2) chronic kidney disease; 3) coronary heart disease or heart failure; 4) tumour; and 5) sudden death of unknown cause. The differences in clinical outcomes and a prediction model for evaluating the long-term adverse events after discharge were established (Figure 1).
Data collection
The baseline data of patients, including age, sex, body mass index (BMI), diabetes classification, predisposing factors, and AOI at admission and at discharge, were collected. BMI was calculated as the body weight/square of height [kg/m (2)]. The diabetes classification was determined by previous medical history.
Biochemical assays
Blood glucose, blood sodium, blood potassium, C-reactive protein (CRP), procalcitonin (PCT), activated partial thromboplastin time (APTT), prothrombin activity (PTA), serum creatinine (SCR), cystatin C, creatine kinase, pH, HCO3-, base excess (BE), alanine aminotransferase (ALT), aspartate aminotransferase (AST), serum albumin, blood amylase, triglyceride (TG), total cholesterol (TC) and low density lipoprotein (LDL) were measured using an automatic biochemical analyser (AU5821, Beckman Coulter, USA). β-hydroxybutyrate was measured using a colorimetric enzymatic reaction (D-3-hydroxybutyrate kit; Ranbut, Randox Laboratories). White blood cells, neutrophils, lymphocytes, and platelets were determined by an automatic blood cell analyser (BC-6800, Mindray, Shenzhen, China). Troponin I was measured by chemiluminescence immunoassay using a DXI800 (Beckman Coulter, USA). Haemoglobin A1c was measured by high-performance liquid chromatography (BC-6800, Mindray, Shenzhen, China).
Statistical methods
Normally distributed data are expressed as the mean ± standard deviation and compared using unifactor ANOVA and the TAMHANE test. Nonnormally distributed data are expressed as percentiles (P25, P75 and P50) and analysed using the rank-sum test. Count data are expressed as percentages (%) and were analysed using the chi-square test. All data were corrected for P value by false discovery rate method. Binary logistic regression analysis was performed after selecting the relevant factors using univariate logistic regression. By calculating the variance expansion factor, we confirm that there is no unacceptable collinearity in all regression models. The ROC curves were also plotted for the related factors. The SPSS 22.2 software was used for the clinical data analysis of enrolled patients. P value < 0.05 or Q value < 0.05 were considered statistically significant.
Results
Baseline clinical and laboratory characteristics
In this study, 339 HCE patients were enrolled. Among them, 69 cases were complicated by AOI, and 270 patients were free of AOI. At admission, the age, history of diabetes, blood glucose, plasma osmotic pressure, triglycerides, blood sodium, blood chloride, SCR, urea nitrogen, cystatin C, creatine kinase, troponin I, CRP, leukocytes, HCO3-, acute infection and deaths in the AOI group were significantly higher than those in the non-AOI group (Q <0.05), while blood ketone and serum albumin in the AOI group were unexpectedly significantly lower than those in the non-AOI group (Q <0.05). Type 2 diabetes was more common in the AOI group (Q <0.05). As shown in Table 1 and Figure 2.
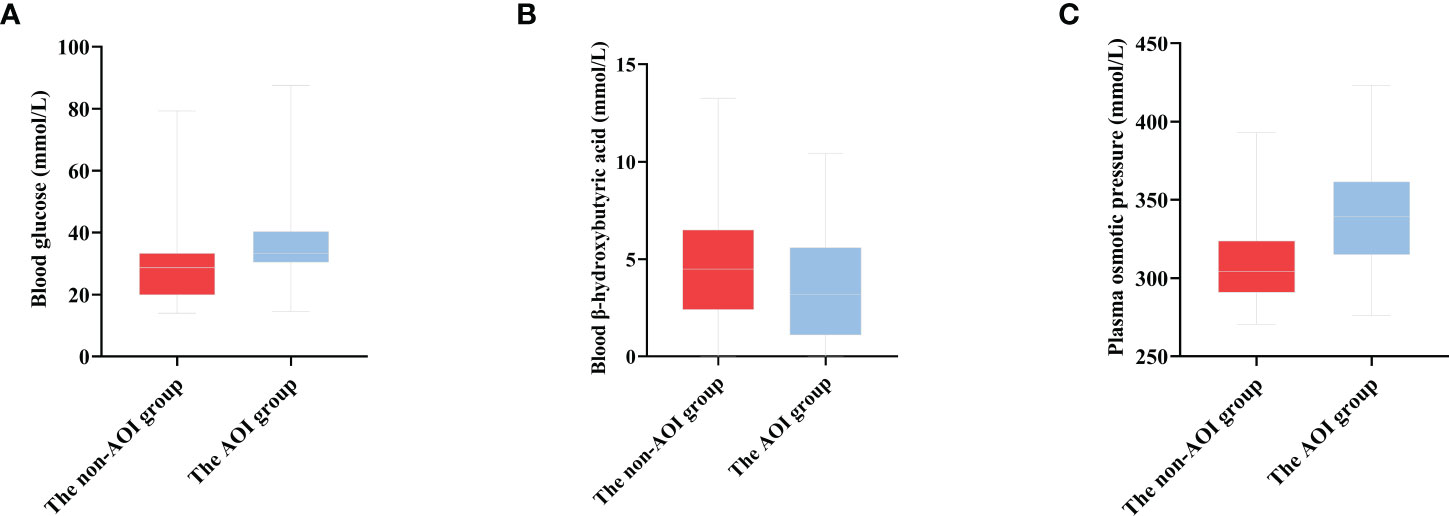
Figure 2 The distribution of blood glucose, blood ketone and plasma osmotic pressure in the two groups (A) The distribution of blood glucose in two groups [(30.43, 40.36) 33.30 vs. (19.90, 33.40) 28.70, P < 0.001]. (B) The distribution of blood β-hydroxybutyric acid in two groups [(1.10, 5.60) 3.18 vs. (2.40, 6.50) 4.50, P = 0.031]. (C) The distribution of plasma osmotic pressure in two groups [(290.83, 323.75) 304.20 vs. (315.0, 361.6) 339. 0, P < 0.001].
At the time of hospital discharge, the urea nitrogen, SCR, cystatin C, troponin I, and CRP levels in the AOI group were significantly higher than those in the non-AOI group (Q <0.05), and serum albumin was lower in the AOI group than in the non-AOI group (Q <0.05) (Table 1).
Comparison of clinical outcomes during hospitalization and hospital discharge
In this study, among the 69 HCE patients with AOI, 8 died during hospitalization, and among the 270 HCE patients without AOI, 11 died during hospitalization. The mortality among cases complicated by AOI was significantly higher than that among patients without AOI [8 (11.59%) vs. 11 (4.07%), Q =0.034] during hospitalization. Of the 61 HCE patients with concomitant AOI who recovered and were discharged from the hospital, 13 died, and 15 developed long-term adverse events during follow-up. Of the 259 HCE patients without AOI who recovered and were discharged from the hospital, 15 died, and 31 developed long-term adverse events during follow-up. The mortality was also significantly higher in patients with concomitant AOI than in patients without AOI after hospital discharge during follow-up [13 (21.31%) vs. 15 (5.8%), Q <0.001]. The long-term adverse events in patients with concomitant AOI were significantly higher than those in patients without AOI during follow-up [15 (24.59%) vs. 31 (11.97%), Q =0.015]. The short-term and long-term prognoses of cases complicated by AOI in the hospital were significantly worse than those of the group without AOI (Figure 3).
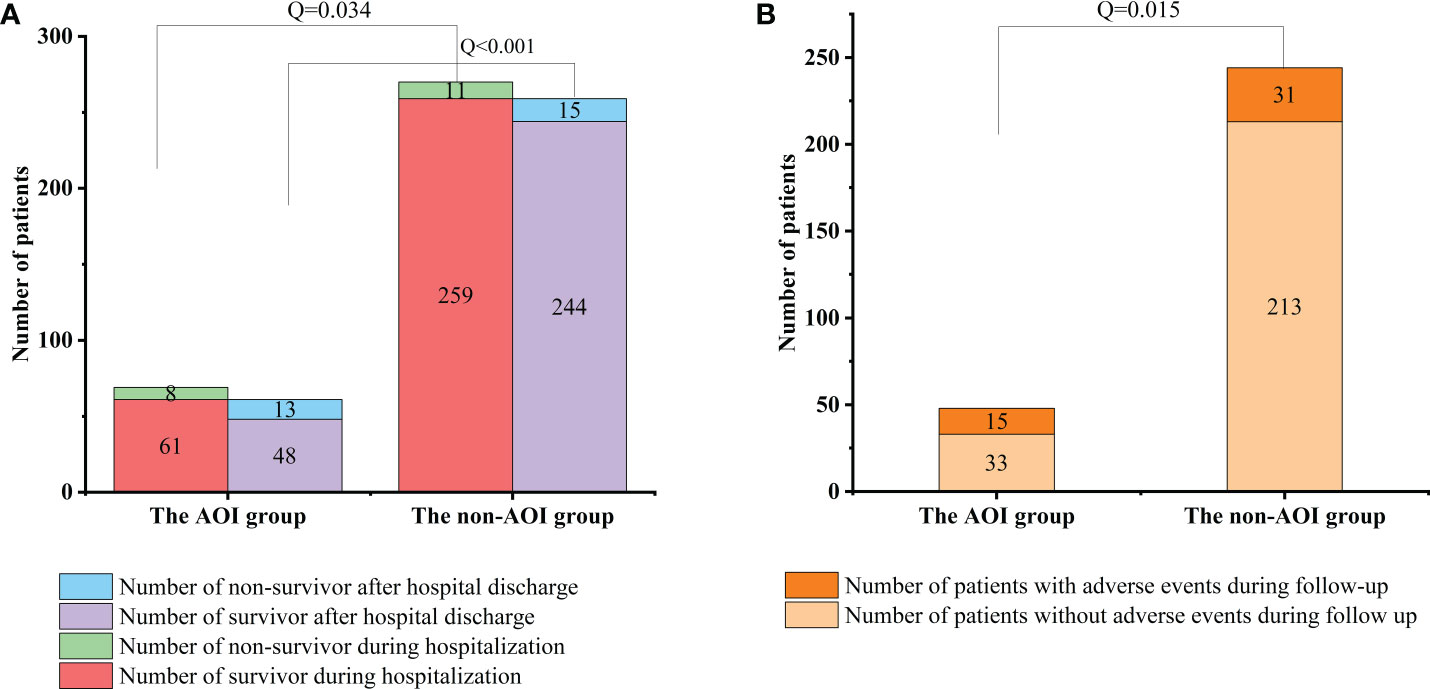
Figure 3 Comparison of mortality and follow-up between two groups during hospitalization and after discharge. (A) Comparison of mortality between the AOI group and non-AOI group during hospitalization and after discharge [8 (11.59%) vs. 11 (4.07%), P = 0.034; 13 (21.31%) vs. 15 (5.8%), P < 0.001]. (B) Comparison of follow-up between the AOI group and non-AOI group after discharge [15 (24.59%) vs. 31(11.97%), P = 0.015].
Logistic regression analysis of factors related to AOI
ROC analysis showed that the sensitivity and specificity were 81.8% and 81.1% in the acute brain injury model (AUC 0.864, P <0.001), 85.7% and 81.2% in the acute cardiac dysfunction model (AUC 0.822, P =0.004), 67.7% and 76.7% in the AKI model (AUC 0.726, P <0.001), respectively (Table S1).
Significant factors were included in logistic regression analysis after univariate screening and collinearity analysis. Plasma osmotic pressure [aOR 1.032; 95% CI 1.014, 1.051; P <0.001], albumin [aOR 0.908; 95% CI 0.835, 0.988; P =0.026], and HCO3- [aOR 1.115; 95% CI 1.029, 1.209; P =0.008] were independent factors of acute brain dysfunction in HCE. Plasma osmotic pressure [aOR 1.020; 95% CI 1.008, 1.032; P =0.001] and SCR [aOR 1.003; 95% CI 1.001, 1.005; P =0.005] were related to AKI. SCR [aOR 1.004; 95% CI 1.002, 1.007; P =0.002] and troponin I [aOR 4.307; 95% CI 1.091, 17.009; P =0.037] were associated with acute cardiac dysfunction (Table 2).
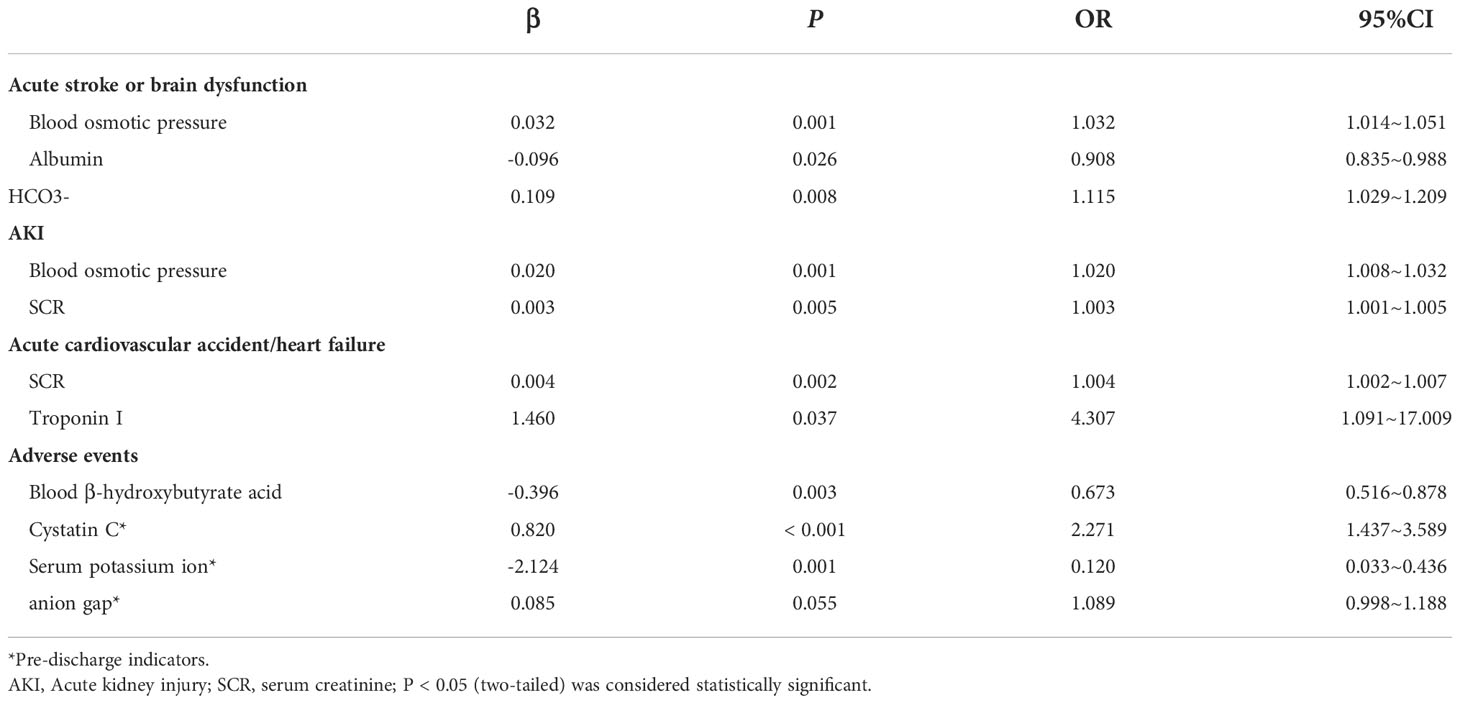
Table 2 Logistic regression analysis of acute organ injury at hospital and adverse events after hospital discharge.
Logistic regression analysis for factors related to long-term adverse events
Blood β-hydroxybutyrate acid [aOR 0.673; 95% CI 0.516, 0.878; P =0.003], Cystatin C [aOR 2.271; 95% CI 1.437, 3.589; P <0.001], serum potassium levels [aOR 0.120; 95% CI 0.033, 0.436; P =0.001] were significantly associated with long-term adverse events after hospital discharge. In addition, anion gap [aOR 1.089; 95% CI 0.998, 1.188; P =0.055] was potentially associated with long-term adverse events (Table 2). The ROC analysis of this model showed a sensitivity of 84.6% and a specificity of 80.3% (AUC 0.887, P <0.001) (Table S1).
Discussion
Our recent studies have confirmed that a high risk of mortality persisted in HCE patients with serious complications or AOI (11, 12). Age, sex, and comorbidity are regarded as important influencing factors for long-term AOI risk and mortality in HCE patients (10), which are commonly difficult to address with current clinical approaches. In addition, how to evaluate the acute and long-term AOI risk of HCE patients through laboratory indicators and how AOI affects the long-term prognosis of HCE patients remains unclear. This study demonstrated that the clinical outcomes of HCE patients with AOI were significantly worse than those of HCE patients without AOI in terms of both hospitalization and hospital discharge. Moreover, the analysis of influencing factors of AOI and long-term adverse events has laid a foundation for subsequent improved treatments. To the best of our knowledge, this model is the first in the Chinese population to predict long-term adverse events combined with AOI in HCE patients using laboratory indicators.
Acute brain injury
Plasma osmotic pressure, plasma albumin and HCO3- levels were found to be important in predicting acute brain injury in patients with HCE. Reduced body volume caused by body fluid concentration may occur in a hypertonic state, and vasoconstriction may be mediated by Rho/Rho kinase signalling (13), further leading to insufficient blood supply to support important organs. Alternatively, exposure to hypertonic saline has been confirmed in current in vitro experiments to potentially cause brain cell death (13), and brain cells can be directly damaged by the hypertonic environment. In addition, the academic community widely recognized that the rapid reduction in osmotic pressure may exacerbate the risk of cerebral oedema in HCE patients. A previous study confirmed that the water content of the cerebral cortex is significantly increased in animals with reduced plasma osmotic pressure (14). Therefore, we believe that a persistent hyperosmolar state and rapid reduction in plasma osmotic pressure may lead to acute neuropsychiatric symptoms in HCE patients via the above mechanisms.
Albumin, a highly soluble protein, can act as an indicator to roughly estimate the nutritional level of patients. Despite its crucial role in maintaining the colloid osmotic pressure of human plasma, it also possesses complex roles, including the transport of various substances, immune regulation, stabilization of endothelial function, antioxidation, and inhibition of erythrocyte sedimentation (15). The serum albumin level has been suggested as a predictor of death, hospital stay and readmission time in acute patients. In this study, the albumin level was found to be an independent influencing factor of in-hospital acute brain dysfunction in HCE patients. Low albumin levels are partly involved in blood concentration, resulting in a further increase in plasma osmotic pressure and causing acute brain dysfunction via the aforementioned effects. In addition, hypoalbuminaemia may participate in acute ischaemic changes in the brain by affecting endothelial cell dysfunction and erythrocyte sedimentation (16).
In this study, HCO3-, an indicator of the degree of body acidosis, was found to be associated with acute brain dysfunction and cognitive decline in HCE patients. Hypocapnia can reduce cerebral blood flow and cerebral oxygenation (17), and insufficient cerebral blood supply and oxygen supply may be associated with vascular dementia or Alzheimer’s disease. In an acidic environment, the altered adhesion and morphology of microglia and astrocytes, along with the continuous decrease in scavenging activity of astrocyte Aβ (18), are similarly involved in acute brain injury. In addition, the unrelieved acidosis state during reperfusion may cause more serious damage to the brain (19).
Acute kidney injury
Plasma osmotic pressure was found to be an independent factor affecting AKI in HCE patients. Similarly, in addition to acute brain injury, severe dehydration and blood concentration in the course of DKA were related to AKI. The kidney is highly vulnerable to plasma osmotic pressure. The reduced renal blood flow not only directly affects kidney function but also results in the reduction of renal oxygen supply and damage to renal cells. In addition, high osmotic pressure itself can also directly affect the kidney and induce cell apoptosis by affecting cell metabolism, nucleic acid stability and ion homeostasis (20), which is consistent with the fact that patients with isolated HHS or combined DKA had significantly more AOIs than patients with isolated DKA in this study. We speculated that this finding might be associated with the higher levels of plasma osmotic pressure. Therefore, this study concluded that higher plasma osmotic pressure indicates a higher morbidity of AKI for HCE patients. Therefore, active anti-infection and early active fluid rehydration therapy can play a positive role in protecting target organs from acute injury.
Creatinine is an endogenous marker for evaluating renal function, which is often influenced by infection, diet, and dehydration. Although its specificity and sensitivity are unsatisfactory, current guidelines suggest that serum creatinine remains an important marker for the diagnosis of AKI. Our study demonstrated a significant association between serum creatinine and AKI in patients with HCE. But because of the diagnosis of AKI is highly dependent on serum creatinine and the transient elevation of serum creatinine due to dehydration during HCE, we believe that there is not enough specificity in judging the risk of AKI by serum creatinine levels in the early stages of HCE. Similarly, due to severe dehydration, it is hard to judge the risk of AKI in HCE patients at an early stage based on their urine output. Current research indicates that Cystatin C, tissue inhibitor of metalloproteinases 2 (Timp-2) and insulin-like growth factor binding protein 7 (IGFBP7) are valuable markers for the diagnosis of AKI, but more studies are needed for HCE patients (21).
Acute cardiac dysfunction
Some studies have found that elevated serum creatinine levels are associated with cardiac microvascular injury, but because of the strong association between serum creatinine and AKI, it may be that endothelial dysfunction and inflammation during AKI contribute to microvascular damage (22). Although patients with heart failure may have mild to moderate elevated SCR, it does not affect patient outcomes significantly (23). Therefore, it is believed that the increase of SCR in this condition is not caused by the renal function damage. Our study found that SCR was associated with the development of acute cardiac dysfunction in patients with HCE, but we do not believe that SCR is directly associated with acute cardiac dysfunction in patients with HCE because of the multiple reasons mentioned above. However, it has some value in predicting the cardiac dysfunction of the HCE patients.
Troponin I is an important marker of myocardial cell injury. At present, it is considered as an important indicator for the diagnosis of myocardial infarction, with high sensitivity and specificity. Our study found that the level of troponin I was related to the occurrence of acute cardiac dysfunction in HCE patients. Previous studies found that troponin I was elevated in patients with acute decompensated diabetes without clinical acute coronary syndrom (24). Our study also confirmed that there are still some patients with non-AOI group whose troponin I level is close to the upper limit of the laboratory reference value. At present, studies have found that in patients with acute myocardial infarction, hyperglycemia is related to larger infarct size and higher troponin I (25). Hyperglycemia may cause myocardial injury by mediating endothelial cell disorder and activating inflammatory mediators. It has been confirmed by in vitro experiments that 24-48 hours of hyperglycemia exposure can stimulate the production of inflammatory mediators in myocardial cells, leading to the elevation of markers of myocardial injury (25). Therefore, we believe that elevated troponin I is significantly related to the occurrence of acute cardiac dysfunction in HCE patients, but the elevated troponin I does not represent the occurrence of acute cardiac dysfunction in HCE patients. How to accurately determine the occurrence of acute cardiac dysfunction in HCE patients still needs further research.
Long-term adverse events after hospital discharge
In this study, the levels of blood β-hydroxybutyric acid, serum potassium concentration, anion gap and cystatin C were found to be predictors for adverse events after hospital discharge in HCE patients.
Under the condition of long-term fasting or ineffective utilization of sugar for energy supply, fat decomposition will produce ketones, including acetylacetic acid, β-hydroxybutyrate and acetone, in which β-hydroxybutyrate accounts for the most. Blood-hydroxybutyrate levels are essential for the diagnosis of diabetic ketosis, and diabetic ketosis is closely associated with AKI. The increased ketone bodies were previously considered to exacerbate the body’s decompensation, resulting in an increased burden of target organs. Moreover, the increased level of blood β-hydroxybutyric acid was found to be a protective factor for HCE patients. Currently, several large-scale clinical studies have found that patients with ketosis have lower all-cause mortality during hyperglycaemic crises than those without ketosis (26, 27). Namely, HHS alone has a higher mortality than DKA alone or combined with HHS. Blood β-hydroxybutyrate can reduce renal ischemia and reperfusion injury by increasing the upstream regulator forkhead transcription factor O3 (FOXO3) and reducing caspase-1 and proinflammatory cytokines, thereby reducing cell death (28, 29). Moreover, serum β-hydroxybutyrate is also considered to be an antitumor agent (30). Exogenous ketone body intake can reduce atherosclerosis, improve heart failure and protect renal function by inhibiting inflammatory factors (31, 32) and improve cognitive function to some extent (33). Human proximal tubular cells can be directly damaged by high levels of blood β-hydroxybutyric acid (> 20 mmol/L) (34), while low concentrations of β-hydroxybutyric acid (<10 mmol/L) protect against ischaemic tissue injury. Nevertheless, blood ketones in HCE patients rarely exceed this level. Blood β-hydroxybutyrate may have no direct correlation with target organ injury in HCE patients but can reduce the risk of long-term adverse events in HCE patients. Therefore, appropriate ketogenesis can be beneficial to HCE patients. However, large-scale clinical studies are required to determine whether exogenous ketogenic supplementation is needed to improve the prognosis.
Serum potassium is involved in maintaining normal cell activity. Although malignant arrhythmia caused by severe hypokalaemia is significantly associated with the mortality of patients, studies on the relationship between hypokalaemia and the prognosis of HCE patients remain limited. Currently, studies have suggested that hypokalaemia is associated with cardiovascular events and renal adverse outcomes (35, 36), but the specific mechanism remains unclear. In this study, serum potassium concentration was found to be associated with the risk of adverse events after hospital discharge in HCE patients. Insignificant hypokalaemia is commonly associated with diuretics and poor nutritional status, and diuretics have been found to be related to long-term mortality and progression of renal disease (37). Poor nutritional status may lead to a heavy volume load and chronic inflammation by reducing plasma albumin levels, thereby resulting in higher all-cause mortality, cardiovascular events and infection-related mortality (38–40).
The anion gap is associated with coronary atherosclerotic heart disease and the risk of progression to end-stage renal disease in patients with CKD (41) and with the 30-day, 90-day, and 365-day all-cause mortality in patients with AKI (42). The anion gap mainly consists of lactic acid, phosphoric acid and ketone bodies. Lactic acid is often considered an indicator to predict the prognosis of patients with acute infection. Increased lactate levels usually indicate body hypoxia. Patients with diabetes are more likely to be infected than normal people, and aerobic glycolysis is essential for the normal immune function of cells. Conversely, lactic acid inhibits immunity in the local environment. Higher lactic acid may exacerbate the risk of reinfection or complications with diabetic foot ulcers in patients with HCE after discharge. The current study found a role for lactate in tumour occurrence and development (43), which may increase the risk of tumours in HCE patients after discharge. A previous study confirmed that compared with the normal population, DKA patients are prone to have a higher risk of developing Alzheimer’s disease after discharge (44). Considering the average age of HCE patients, acidosis should be corrected more actively than in the general population to improve the cognitive function prognosis of patients.
Cystatin C, a small protein molecule produced by nucleated cells, has been proven to be a highly sensitive biomarker of renal function. Compared with serum creatinine, cystatin C can serve as a stronger predictor for AKI. Several studies have confirmed that cystatin C can be used as a predictor of all-cause mortality in the elderly population, long-term mortality in patients with severe disease and mortality in patients with chronic kidney disease (23). Our study also proposed that cystatin C can be used as a predictor of diabetic foot ulcers (45). Cystatin C not only has a stronger ability than serum creatinine to predict mortality and the development of end-stage renal disease in diabetic patients (46, 47) but also has a strong correlation with the poor healing of diabetic foot ulcers (48) and the risk of cardiovascular disease, which seems to be associated with promoting atherosclerosis through multiple pathways (49). In this study, cystatin C was found to be not only a predictor of AKI but also an independent factor influencing adverse events after discharge in HCE patients. Although cystatin C seems to be correlated with multiple diseases, the direct involvement of cystatin C in the occurrence and development of the disease or its relationship with CKD remains unknown due to the sensitivity of cystatin C, which can be used as a diagnostic indicator of pre-CKD, and the lack of relevant clinical research.
Several limitations remain in this study. First, this work was a cross-sectional and observational study that did not include clinical intervention in HCE patients and potential improvements in clinical outcome. Second, the follow-up period was too short, and some patients could not provide detailed information, which may have led to the omission and ambiguity of a considerable portion of the positive outcomes. Therefore, we failed to conduct further analysis on the long-term outcomes and their influencing factors after discharge. Finally, we were unable to analyse DKA and HHS data due to the limited sample size and follow-up outcome.
Conclusions
In summary, we established a prediction model to observe the impact of AOI on long-term outcomes in patients with HCE. The prognosis of HCE patients with concomitant AOI was found to be significantly worse than that of HCE patients without AOI. Further study should be performed to observe whether timely intervention for AOI can improve clinical outcomes.
Data availability statement
The raw data supporting the conclusions of this article will be made available by the authors, without undue reservation.
Ethics statement
The studies involving human participants were reviewed and approved by the Committee for Research Ethics of Chongqing University Central Hospital (NO. 202262). The patients/participants provided their written informed consent to participate in this study.
Author contributions
ZD, PS and WD developed the protocol and were responsible for the study design. ZD, CY, LD, YJ and FD performed the systematic literature research and extracted data. XJ, YC and WD did data analysis and interpretation. ZD and PS wrote the first draft, and GY, YM and WD revised the article for important intellectual content. All authors contributed to the article and approved the submitted version.
Funding
This study was supported by the Joint Medical Research Programs of Chongqing Science and Technology Bureau and Health Commission Foundation awarded to Dr. Wuquan Deng in 2023. The Fundamental Research Funds for the Central Universities (2021CDJYGRH-012) and the fund of Sichuan Provincial Western Psychiatric Association’s CSPC LEADING Scientific Research Project (Grant No. WL2021002) awarded to WD.
Conflict of interest
The authors declare that the research was conducted in the absence of any commercial or financial relationships that could be construed as a potential conflict of interest.
Publisher’s note
All claims expressed in this article are solely those of the authors and do not necessarily represent those of their affiliated organizations, or those of the publisher, the editors and the reviewers. Any product that may be evaluated in this article, or claim that may be made by its manufacturer, is not guaranteed or endorsed by the publisher.
Supplementary material
The Supplementary Material for this article can be found online at: https://www.frontiersin.org/articles/10.3389/fendo.2022.1057089/full#supplementary-material
Supplementary Table 1 | Evaluation of target organ injury model in HCE patients. AKI, Acute kidney injury.
References
1. Benoit SR, Hora I, Pasquel FJ, Gregg EW, Albright AL, Imperatore G. Trends in emergency department visits and inpatient admissions for hyperglycemic crises in adults with diabetes in the U.S., 2006-2015. Diabetes Care (2020) 43(5):1057–64. doi: 10.2337/dc19-2449
2. Desai D, Mehta D, Mathias P, Menon G, Schubart UK. Health care utilization and burden of diabetic ketoacidosis in the U.S. over the past decade: A nationwide analysis. Diabetes Care (2018) 41(8):1631–8. doi: 10.2337/dc17-1379
3. Chou CH, Feng IJ, Chen YC, Chen JH, Lin HJ, Wang JJ, et al. Risk of dementia in diabetic patients with hyperglycemic crisis: A nationwide Taiwanese population-based cohort study. Neuroepidemiology (2020) 54(5):419–26. doi: 10.1159/000509754
4. Mizuguchi C, Sato Y, Imai H, Kakizawa M, Yamashita K, Aizawa T. Homonymous quadrantanopia associated with hyperosmolar hyperglycemic syndrome. J Diabetes Investig (2020) 11(5):1374–5. doi: 10.1111/jdi.13242
5. Bragg F, Holmes MV, Iona A, Guo Y, Du H, Chen Y, et al. Association between diabetes and cause-specific mortality in rural and urban areas of China. JAMA (2017) 317(3):280–9. doi: 10.1001/jama.2016.19720
6. Bai F, Jiang FF, Lu JJ, Ma SG, Peng YG, Jin Y, et al. The impact of hyperglycemic emergencies on the kidney and liver. J Diabetes Res (2013) 2013:967097. doi: 10.1155/2013/967097
7. Huang CC, Weng SF, Tsai KT, Chen PJ, Lin HJ, Wang JJ, et al. Long-term mortality risk after hyperglycemic crisis episodes in geriatric patients with diabetes: A national population-based cohort study. Diabetes Care (2015) 38(5):746–51. doi: 10.2337/dc14-1840
8. Kao Y, Feng IJ, Hsu CC, Wang JJ, Lin HJ, Huang CC. Association of hyperglycemic crisis with an increased risk of end-stage renal disease: A nationwide population-based cohort study. Diabetes Res Clin Pract (2018) 138:106–12. doi: 10.1016/j.diabres.2018.01.008
9. Yamazaki Y, Harada S, Tokuyama S. Relationship between cerebral sodium-glucose transporter and hyperglycemia in cerebral ischemia. Neurosci Lett (2015) 604:134–9. doi: 10.1016/j.neulet.2015.08.004
10. Kitabchi AE, Umpierrez GE, Miles JM, Fisher JN. Hyperglycemic crises in adult patients with diabetes. Diabetes Care (2009) 32:1335–43. doi: 10.2337/dc09-9032
11. Deng L, Xie P, Chen Y, Rui S, Yang C, Deng B, et al. Impact of acute hyperglycemic crisis episode on survival in individuals with diabetic foot ulcer using a machine learning approach. Front Endocrinol (2022) 13:974063. doi: 10.3389/fendo.2022.974063
12. Li A, Jiang X, Zhong M, Li N, Tao Y, Wu W, et al. Intractable hiccups as a rare gastrointestinal manifestation in severe endocrine and metabolic crisis: Case report and review of the literature. Ther Adv Endocrinol Metab (2020) 11:2042018820934307. doi: 10.1177/2042018820934307
13. Morland C, Pettersen MN, Hassel B. Hyperosmolar sodium chloride is toxic to cultured neurons and causes reduction of glucose metabolism and ATP levels, an increase in glutamate uptake, and a reduction in cytosolic calcium. Neurotoxicology (2016) 54:34–43. doi: 10.1016/j.neuro.2016.03.005
14. Zornow MH, Todd MM, Moore SS. The acute cerebral effects of changes in plasma osmolality and oncotic pressure. Anesthesiology (1987) 67(6):936–41. doi: 10.1097/00000542-198712000-00010
15. Herrmann FR, Safran C, Levkoff SE, Minaker KL. Serum albumin level on admission as a predictor of death, length of stay, and readmission. Arch Intern Med (1992) 152(1):125–30. doi: 10.1001/archinte.1992.00400130135017
16. Reinhart WH, Nagy C. Albumin affects erythrocyte aggregation and sedimentation. Eur J Clin Invest (1995) 25(7):523–8. doi: 10.1111/j.1365-2362.1995.tb01739.x
17. Ringer SK, Clausen NG, Spielmann N, Weiss M. Effects of moderate and severe hypocapnia on intracerebral perfusion and brain tissue oxygenation in piglets. Paediatr Anaesth (2019) 29(11):1114–21. doi: 10.1111/pan.13736
18. Eugenín J, Vecchiola A, Murgas P, Arroyo P, Cornejo F, von Bernhardi R. Expression pattern of scavenger receptors and amyloid-β phagocytosis of astrocytes and microglia in culture are modified by acidosis: Implications for alzheimer’s disease. J Alzheimers Dis (2016) 3053(3):857–73. doi: 10.3233/JAD-160083
19. Gu L, Liu X, Yang Y, Luo D, Zheng X. ASICs aggravate acidosis-induced injuries during ischemic reperfusion. Neurosci Lett (2010) 479(1):63–8. doi: 10.1016/j.neulet.2010.05.029
20. Gaut JP, Liapis H. Acute kidney injury pathology and pathophysiology: A retrospective review. Clin Kidney J (2020) 14(2):526–36. doi: 10.1093/ckj/sfaa142
21. Ronco C, Bellomo R, Kellum JA. Acute kidney injury. Lancet (2019) 394(10212):1949–64. doi: 10.1016/S0140-6736(19)32563-2
22. Reinstadler SJ, Kronbichler A, Reindl M, Feistritzer HJ, Innerhofer V, Mayr A, et al. Acute kidney injury is associated with microvascular myocardial damage following myocardial infarction. Kidney Int (2017) 92(3):743–50. doi: 10.1016/j.kint.2017.02.016
23. Metra M, Cotter G, Senger S, Edwards C, Cleland JG, Ponikowski P, et al. Prognostic significance of creatinine increases during an acute heart failure admission in patients with and without residual congestion: A Post hoc analysis of the PROTECT data. Circ Heart Fail (2018) 11(5):e004644. doi: 10.1161/CIRCHEARTFAILURE.117.004644
24. Eubanks A, Raza F, Alkhouli M, Glenn AN, Homko C, Kashem A, et al. Clinical significance of troponin elevations in acute decompensated diabetes without clinical acute coronary syndrome. Cardiovasc Diabetol (2012) 11:154. doi: 10.1186/1475-2840-11-154
25. Thakur V, Alcoreza N, Cazares J, Chattopadhyay M. Changes in stress-mediated markers in a human cardiomyocyte cell line under hyperglycemia. Int J Mol Sci (2021) 22(19):10802. doi: 10.3390/ijms221910802
26. Wachtel TJ, Silliman RA, Lamberton P. Prognostic factors in the diabetic hyperosmolar state. J Am Geriatr Soc (1987) 35(8):737–41. doi: 10.1111/j.1532-5415.1987.tb06351.x
27. Wu XY, She DM, Wang F, Guo G, Li R, Fang P, et al. Clinical profiles, outcomes and risk factors among type 2 diabetic inpatients with diabetic ketoacidosis and hyperglycemic hyperosmolar state: A hospital-based analysis over a 6-year period. BMC Endocr Disord (2020) 20(1):182. doi: 10.1186/s12902-020-00659-5
28. Lu D, Liu J, Jiao J, Long B, Li Q, Tan W, et al. Transcription factor Foxo3a prevents apoptosis by regulating calcium through the apoptosis repressor with caspase recruitment domain. J Biol Chem (2013) 288(12):8491–504. doi: 10.1074/jbc.M112.442061
29. Youm YH, Nguyen KY, Grant RW, Goldberg EL, Bodogai M, Kim D, et al. The ketone metabolite β-hydroxybutyrate blocks NLRP3 inflammasome-mediated inflammatory disease. Nat Med (2015) 21(3):263–9. doi: 10.1038/nm.3804
30. Feng S, Wang H, Wang Y, Sun R, Xie Y, Zhou Z, et al. Apatinib induces 3-hydroxybutyric acid production in the liver of mice by peroxisome proliferator-activated receptor α activation to aid its antitumor effect. Cancer Sci (2019) 110(10):3328–39. doi: 10.1111/cas.14168
31. Zhang SJ, Li ZH, Zhang YD, Chen J, Li Y, Wu FQ, et al. Ketone body 3-hydroxybutyrate ameliorates atherosclerosis via receptor Gpr109a-mediated calcium influx. Adv Sci (Weinh) (2021) 8(9):2003410. doi: 10.1002/advs.202003410
32. Monzo L, Sedlacek K, Hromanikova K, Tomanova L, Borlaug BA, Jabor A, et al. Myocardial ketone body utilization in patients with heart failure: The impact of oral ketone ester. Metabolism (2021) 115:154452. doi: 10.1016/j.metabol.2020.154452
33. Yao A, Li Z, Lyu J, Yu L, Wei S, Xue L, et al. On the nutritional and therapeutic effects of ketone body d-β-hydroxybutyrate. Appl Microbiol Biotechnol (2021) 105(16-17):6229–43. doi: 10.1007/s00253-021-11482-w
34. Tajima T, Yoshifuji A, Matsui A, Itoh T, Uchiyama K, Kanda T, et al. β-hydroxybutyrate attenuates renal ischemia-reperfusion injury through its anti-pyroptotic effects. Kidney Int (2019) 95(5):1120–37. doi: 10.1016/j.kint.2018.11.034
35. O’Donnell M, Mente A, Rangarajan S, McQueen MJ, Wang X, Liu L, et al. Urinary sodium and potassium excretion, mortality, and cardiovascular events. N Engl J Med (2014) 371(7):612–23. doi: 10.1056/NEJMoa1311889
36. Araki S, Haneda M, Koya D, Kondo K, Tanaka S, Arima H, et al. Urinary potassium excretion and renal and cardiovascular complications in patients with type 2 diabetes and normal renal function. Clin J Am Soc Nephrol (2015) 10(12):2152–8. doi: 10.2215/CJN.00980115
37. Ahmed A, Husain A, Love TE, Gambassi G, Dell'Italia LJ, Francis GS, et al. Heart failure, chronic diuretic use, and increase in mortality and hospitalization: An observational study using propensity score methods. Eur Heart J (2006) 27(12):1431–9. doi: 10.1093/eurheartj/ehi890
38. Mehrotra R, Duong U, Jiwakanon S, Kovesdy CP, Moran J, Kopple JD, et al. Serum albumin as a predictor of mortality in peritoneal dialysis: Comparisons with hemodialysis. Am J Kidney Dis (2011) 58(3):418–28. doi: 10.1053/j.ajkd.2011.03.018
39. Shi Y, Cai J, Shi C, Liu C, Zhou J, Li Z. Low serum albumin is associated with poor prognosis in patients receiving peritoneal dialysis treatment. J Healthc Eng (2022) 2022:7660806. doi: 10.1155/2022/7660806
40. Katalinic L, Premuzic V, Basic-Jukic N, Barisic I, Jelakovic B. Hypoproteinemia as a factor in assessing malnutrition and predicting survival on hemodialysis. J Artif Organs (2019) 22(3):230–6. doi: 10.1007/s10047-019-01098-3
41. Banerjee T, Crews DC, Wesson DE, McCulloch CE, Johansen KL, Saydah S, et al. Elevated serum anion gap in adults with moderate chronic kidney disease increases risk for progression to end-stage renal disease. Am J Physiol Renal Physiol (2019) 316(6):F1244–53. doi: 10.1152/ajprenal.00496.2018
42. Cheng B, Li D, Gong Y, Ying B, Wang B. Serum anion gap predicts all-cause mortality in critically ill patients with acute kidney injury: Analysis of the MIMIC-III database. Dis Markers (2020) 2020:6501272. doi: 10.1155/2020/6501272
43. Hirschhaeuser F, Sattler UG, Mueller-Klieser W. Lactate: A metabolic key player in cancer. Cancer Res (2011) 71(22):6921–5. doi: 10.1158/0008-5472.CAN-11-1457
44. Chen YL, Weng SF, Yang CY, Wang JJ, Tien KJ. Diabetic ketoacidosis further increases risk of alzheimer’s disease in patients with type 2 diabetes. Diabetes Res Clin Pract (2019) 147:55–61. doi: 10.1016/j.diabres.2018.11.013
45. Zhao J, Deng W, Zhang Y, et al. Association between serum cystatin c and diabetic foot ulceration in patients with type 2 diabetes: A cross-sectional study. J Diabetes Res (2016) 2016:8029340. doi: 10.1155/2016/8029340
46. de Boer IH, Katz R, Cao JJ, Fried LF, Kestenbaum B, Mukamal K, et al. Albuminuria, and mortality among older adults with diabetes. Diabetes Care (2009) 32(10):1833–8. doi: 10.2337/dc09-0191
47. Krolewski AS, Warram JH, Forsblom C, Smiles AM, Thorn L, Skupien J, et al. Serum concentration of cystatin c and risk of end-stage renal disease in diabetes. Diabetes Care (2012) 35(11):2311–6. doi: 10.2337/dc11-2220
48. Ai L, Hu Y, Zhang X, Zeng H, Zhao J, Zhao J, et al. High cystatin c levels predict undesirable outcome for diabetic foot ulcerations. Wound Repair Regen (2016) 24(3):560–7. doi: 10.1111/wrr.12419
Keywords: diabetes, hyperglycaemic crisis episode, acute organ injury, mortality, long-term prognosis
Citation: Duan Z, Song P, Yang C, Deng L, Jiang Y, Deng F, Jiang X, Chen Y, Yang G, Ma Y and Deng W (2022) The impact of hyperglycaemic crisis episodes on long-term outcomes for inpatients presenting with acute organ injury: A prospective, multicentre follow-up study. Front. Endocrinol. 13:1057089. doi: 10.3389/fendo.2022.1057089
Received: 29 September 2022; Accepted: 16 November 2022;
Published: 05 December 2022.
Edited by:
Pranav Kumar Prabhakar, Lovely Professional University, IndiaReviewed by:
Hilal Sipahioglu, Kayseri Education and Research Hospital, TurkeyMukesh Doble, Indian Institute of Technology Madras, India
Copyright © 2022 Duan, Song, Yang, Deng, Jiang, Deng, Jiang, Chen, Yang, Ma and Deng. This is an open-access article distributed under the terms of the Creative Commons Attribution License (CC BY). The use, distribution or reproduction in other forums is permitted, provided the original author(s) and the copyright owner(s) are credited and that the original publication in this journal is cited, in accordance with accepted academic practice. No use, distribution or reproduction is permitted which does not comply with these terms.
*Correspondence: Wuquan Deng, d3VxdWFuZGVuZ0BjcXUuZWR1LmNu
†These authors have contributed equally to this work