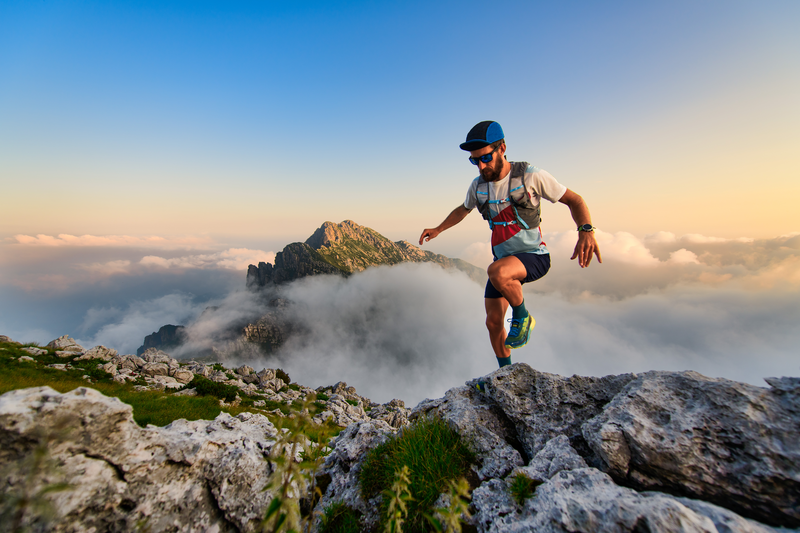
95% of researchers rate our articles as excellent or good
Learn more about the work of our research integrity team to safeguard the quality of each article we publish.
Find out more
ORIGINAL RESEARCH article
Front. Endocrinol. , 09 December 2022
Sec. Experimental Endocrinology
Volume 13 - 2022 | https://doi.org/10.3389/fendo.2022.1051797
This article is part of the Research Topic Central and Peripheral Mechanism Interfering in Metabolic Syndrome View all 5 articles
A commentary has been posted on this article:
Commentary: Gut microbiota and its derived SCFAs regulate the HPGA to reverse obesity-induced precocious puberty in female rats
The intestinal microbiota and its derived short-chain fatty acids (SCFAs) can reverse obesity and obesity-related metabolic diseases, but whether it has an effect on obesity complicated by precocious puberty and its potential mechanism need to be further understood. The purpose of this study was to investigate the effect of the gut microbiota and its derived short-chain fatty acids (SCFAs) on obesity-induced precocious puberty rats and their regulatory mechanisms. We constructed obesity-induced precocious puberty rats using a high-fat diet (HFD) had notable similarity to precocious puberty caused by obesity due to overeating in children. We then added acetate, propionate, butyrate or their mixture to the HFD, and investigated the effect of intestinal microbiota and its derived SCFAs on the hypothalamic-pituitary-gonadal axis (HPGA) in rats with obesity-induced precocious puberty. We found that obesity-induced precocious puberty rats had an early first estrous cycle, increased hypothalamic mRNA expression of Kiss1, GPR54 and GnRH, and early gonadal maturation. Meanwhile, the intestinal microbiota imbalance and the main SCFAs production decreased in the colon. The addition of acetate, propionate, butyrate or their mixture to the HFD could significantly reverse the precocious puberty of rats, reduce GnRH release from the hypothalamus and delay the development of the gonadal axis through the Kiss1–GPR54–PKC–ERK1/2 pathway. Our findings suggest that gut microbiota-derived SCFAs are promising therapeutic means for the prevention of obesity-induced precocious puberty and provide new therapeutic strategies with clinical value.
Overweight and obesity among children has nearly doubled since 1980, making children with obesity a global epidemic and China become the country with the largest number of children with obesity now (1, 2). Several factors contribute to obesity, such as genetic and dietary factors, but the root cause lie in the imbalance between energy intake and consumption that leads to fat accumulation (3, 4). However, children with obesity, the incidence of metabolic diseases such as hyperinsulinemia, dyslipidemia, disturbances of sex hormones, which are significantly related to childhood obesity, are also increasing. The risk of cancer in adulthood is also significantly increased by childhood obesity (5, 6). In recent years, the incidence of precocious puberty in children with obesity has gradually increased. Several studies have shown that high body mass index (BMI) is associated with earlier pubertal maturation in children (7–10). However, it is still unclear whether obesity contributes to precocious puberty. Regarding the relationship between childhood obesity and precocious puberty, researchers believe the key metabolic peptide leptin and genetic factors play important roles (11, 12).
Over the past few years, there has been increasing recognition that the gut microbiota is responsible for regulating obesity and endocrine via the gut-brain axis (13–16). A disorder of the gut microbiota and a high proportion of Firmicutes to Bacteroides contributed to childhood obesity (17, 18). By altering their gut microbiota, HFD mothers can promote obesity and precocious puberty in their children (19). Similarly, recent studies have reported that gut microbiota may affect sex hormones and participate in the pathogenesis of precocious puberty in children and HFD mice (18, 20, 21). It is generally known that the initiation of the HPGA and the release of gonadotropin-releasing hormone (GnRH) are necessary for the physiological initiation of precocious puberty. During the activation of GnRH neurons, the gene product kisspeptin encoded by Kiss1 binds to its receptor GPR54 (Kiss1R) stimulate hypothalamic neurons to release GnRH, which promotes the secretion of luteinizing hormone (LH) and follicle stimulating hormone (FSH) in the pituitary (22, 23). However, the specific mechanism by which the gut microbiota regulates the HPGA is still unknown.
Gut microbiota-derived SCFAs also regulate obesity and related metabolic disorders. Biologically, SCFAs are formed from the fermentation of indigestible starch and dietary fiber by intestinal microbes. In colon fecal, SCFAs were primarily composed of acetate, propionate and butyrate at molar ratios of 60:20:20 (24). SCFAs target multiple organs and tissues and regulate related gene expression and tissue metabolism (25, 26). Studies have confirmed that high levels of dietary fiber and SCFAs supplementation in rodents can alleviate HFD-induced obesity (27). However, the specific regulatory role of the gut microbiota and its derived SCFAs on HPGA in obesity-induced rats remains unknown. Herein, we provide evidence that supplementation with SCFAs can reverse and delay the onset of obesity-induced precocious puberty rats by regulating the expression of HPGA related genes.
Pregnant Sprague−Dawley rats were acquired from Chongqing Medical University (Chongqing, China), which were housed under standard conditions: light and dark cycles for 12 hours and temperature 22 °C. Upon birth, the female rats were weaned on postnatal day (PND) 21. Subsequently, all the female animals were randomly assigned to different experimental groups after PND21 (n= 6-8). The control group was allowed free access to pelleted food (CTR; Diet D12450B, 10% fat content, Keao Xieli, China) and tap water. The HF group was fed a high-fat diet (HF; Diet D12451, 45% fat content, Keao Xieli, Beijing, China). The rats in the SCFAs groups received supplementation with 5% sodium acetate (HF-A, S2289, Sigma-Aldrich, USA), sodium propionate (HF-P, P1880, Sigma-Aldrich, USA) or sodium butyrate (HF-B, 303410, Sigma-Aldrich, USA), and the mixed group (HF-SCFA) received sodium acetate, sodium propionate or sodium butyrate at a ratio of 3:1:1 in the high-fat diet (Diet D12451, 45% fat content, Keao Xieli, Beijing, China). Body weight was monitored daily. All experiments were approved by the Animal Ethics Committee of Children’s Hospital of Chongqing Medical University.
Indicators for evaluating the maturity of female pubertal rats. Monitoring puberty by observing the vaginal opening (VO) after PND21. Exfoliated cells from the rats with vaginal opening were examined by smear to confirm first estrus. The rats were sacrificed at diestrus stage of the estrous cycle, while the remaining rats were sacrificed at the same time. We used histological analysis and scoring method of ovary (Pub-Score) to analyze the development of follicles and corpus luteum (CL) according to the reference (28, 29). For non-ovulating animals, determine the most advanced healthy antral follicle class from small follicles to antral follicles (F1-F5), thus determining the time of prepubertal maturity from -5 to-1 (representing the time of the first ovulation). For rats that ovulated, the CL was used as the morphological sign of ovulation, allowing puberty to be divided from +1 (equivalent to CL1) to +5 (equivalent to CL5) at intervals of 1 day.
All rats were anesthetized by intraperitoneal injection of 2% pentobarbital sodium. Blood samples were collected from the abdominal aorta, and the serum was separated after centrifugation (3500 rpm, 20 min, 4°C) and stored at -80°C. The serum hormone and blood lipid levels were measured. After sacrifice, the hypothalamus, pituitary, uterus, ovary, colon and fecal were removed, frozen in liquid nitrogen and preserved at -80°C. The wet weight of the uterus and ovary was determined, relative uterus weight (UW) and ovary weight (OW) (mg/100 g) were calculated. The ovary and uterine tissues were stained with formalin for hematoxylin and eosin (H&E) staining.
The concentrations of serum FSH, LH and GnRH were measured by ELISA kits (Jianglaibio, China). The minimum detection concentrations of FSH and LH were less than 0.38 mIU/ml and 0.074 mIU/ml, respectively. Hypothalamic tissue was weighed, and precooled PBS was added according to the weight–volume ratio (1:9). After the homogenate was fully ground on ice, the supernatant of the homogenate was centrifuged (5000 g, 10 min). The minimum detection concentration of GnRH was less than 7.9 pg/ml. All samples were measured in duplicate. For all hormones, the intra-and inter-batch coefficients were less than 10%.
All samples were measured for triglycerides, total cholesterol, low-density lipoprotein cholesterol (LDL-C), and high-density lipoprotein cholesterol (HDL-C) by standard laboratory techniques using a Hitachi automatic analyzer (Hitachi 7600, Japan).
Using TRIzol reagent (Takara, Japan), total RNA was obtained from the rat hypothalamus, pituitary, colon and ovary. One microgram of total RNA was reverse transcribed into cDNA using Superscript II reverse transcription kit (Takara, Japan). Then, cDNA obtained by reverse transcription and gene-specific primers and a SYBR Green dye kit (Takara, Japan) were used for amplification. Table 1 shows the forward and reverse primers used for the polymerase chain reaction. β-actin was used as the endogenous control. The amount of mRNA relative to the endogenous control was calculated by the 2-△△CT method.
Rat hypothalamus proteins were extracted using protein extraction kit (Beyotime, China). Protein samples were separated to 10% SDS−PAGE gels. After electrophoresis, the proteins were transferred to polyvinylidene fluoride (PVDF) membranes (Millipore, USA). The membranes were blocking for 1 hour, then incubated overnight at 4°C with primary antibodies against GPR54 (A2967, ABclonal, Shanghai, China), PKC (2056T, Cell Signaling, USA), phospho-ERK1/2 (9101s, Cell Signaling, USA), ERK1/2 (9102S, Cell Signaling, USA), and β-actin (TA-09, China). Next, the membranes were incubated with the corresponding secondary antibody (ZSGB-BIO, China) at 37°C for 1 hour. The membranes were visualized by chemiluminescent substrate (Millipore, USA) under the ChemiDOC Touch Imaging System (Bio-Rad, USA). The results were analyzed by ImageJ software.
Fecal DNA was extracted from different samples using OMEGA stool DNA Kit according to the manufacturer’s instructions. The V3-V4 regions per sample were amplified using primers by a PCR system (GeneAmp 9700, ABI, USA). Amplicons were paired-end sequenced with the Illumina NovaSeq 6000 (Illumina, USA) by LC-Bio Technology Co., Ltd. (Hangzhou, China). Raw fastq files were merged using FLASH and high-quality filtered using fqtrim. Chimeric sequences were filtered by Vsearch. After dereplication using DADA2, we obtained a feature table and feature sequence. Alpha-diversity and β-diversity were calculated by QIIME. The ASVs were annotated by aligned feature sequences with the SILVA database. Other diagrams were implemented using the R package.
Detection and analysis of SCFAs in rat fecal samples. Briefly, acetic acid, propanoic acid, butyric acid, isobutyric acid, isovaleric acid, hexanoic acid and valeric acid were mixed with ether at different volumes to prepare the standard concentration gradient. Then, 50 mg of each sample was obtained, the internal standard acetic-d4 was added, and methanol was added to 0.5 ml. The samples were ultrasonicated for 10 min in an ice bath, vortexed and centrifuged (12,000 rpm, 4°C, 5 min). A total of 1μl was injected and detected by gas chromatography-mass spectrometry (Thermo Trace1610, USA).
All the results are presented as the mean ± standard deviation. Two-paired Student’s t-test was used for two group, one-way analysis of variance (ANOVA) followed by Duncan or Turkey comparison was used for multiple group comparisons. The Wilcoxon rank-sum test was used for the analysis of microbial difference. And the data were plotted by GraphPad Prism 8.0 Software (GraphPad Software Inc., USA). P < 0.05 indicated a statistically significant difference.
Female rats fed a HFD to construct an obesity model had an increased body weight (BW) during the peripubertal period (Figure 1A), and female adolescent rats had increases in total cholesterol, triglycerides, LDL-C and HDL-C (Figures 1B–E). Furthermore, precocious puberty was induced in obese rats, as characterized by the earlier age VO (Figure 1F) and first estrous (Figure 1G). At the same time, endometrial thickness (Figure 1H) and UW (Figure 1I) were increased. We found that the HF group had a higher percentage undergone ovulation according to the histological score of the ovary (66.66% HF versus 16.6% CTR) (Figure 1J) and OW (Figure 1K). Our results also showed that the relative release of serum LH (Figure 1L) and FSH (Figure 1M) and the secretion of GnRH from the hypothalamus (Figure 1N) were significantly increased in the HF group. Altogether, these results support that HFD leads to obesity and consequently induces precocious puberty in rats.
Figure 1 HFD induces obesity and precocious puberty in female rats. (A) Evolution of body weight. (B) Serum total cholesterol. (C) Serum triglyceride. (D) Serum low density lipoprotein cholesterol (LDL-C). (E) Serum high density lipoprotein cholesterol (HDL-C). (F) Cumulative percentage of vaginal opening. (G) Cumulative percentage of first estrus. (H) Uterine wall thickness. (I) Relative uterus weight. (J) Histological score of follicular development and ovulation. (K) Relative ovary weight. (L) Serum LH levels. (M) Serum FSH levels. (N) GnRH secretion in the hypothalamus. n = 6 per group for rat samples. *P<0.05; **P<0.01.
To further explore the effects of HFD-induced obesity on the HPGA, we first detected the key genes regulating the gonadal development pathway. The results showed that the expression of Kiss1, GPR54, GnRH and ERα in the hypothalamus were higher than in the CTR group (Figures 2A–D), which in turn activated the gonadotropin-releasing hormone receptor (GnRHR, Figure 2E) in the pituitary, and the luteinizing receptor (LHR, Figure 2F), follicle-stimulating hormone receptor (FSHR, Figure 2G) and estrogen receptor α (ERα, Figure 2H) in the ovary. The above results showed that obesity-induced precocious puberty promotes the gene expression of the HPGA.
Figure 2 HFD promotes the gene expression of the HPGA in rats with obesity-induced precocious puberty. (A) The gene expression of Kiss1 in the hypothalamus. (B) The gene expression of GPR54 in the hypothalamus. (C) The gene expression of GnRH in the hypothalamus. (D) The gene expression of ERα in the hypothalamus. (E) The gene expression of GnRHR in the pituitary. (F) The gene expression of LHR in the ovary. (G) The gene expression of FSR in the ovary. (H) The gene expression of ERα in the ovary. n = 6 per group for rat samples. *P<0.05; **P<0.01.
To explore the changes in the gut microbiome in rats with obesity-induced precocious puberty, we analyzed the feces between the HF group and CTR group. The composition of the microbiota in the feces of the HF group was significantly different from that of the CTR group (Figure 3). Based on Bray−Curtis, Jaccard and unweighted Uni-Frac PCoA, there was a significant difference in the β-diversity of the feces between the HF group and CTR group (Figures 3A–C). At the phylum level, compared with those in the CTR group, the HF group showed increased levels of Bacteroidota, decreased levels of Firmicutes, and increased Proteobacteria and Verrucomicrobiota(Figure 3D). However, the proportion of Patescibacteria was significantly lower and the levels of Campylobacterota and Proteobacteria were higher than that in the CTR group (P < 0.05, Figure 3G). At the family level, the levels of Lactobacillaceae and Lachnospiraceae were decreased in the HF group, while the level of Enterobacteriaceae was increased (Figure 3E). The proportions of Clostridia, Selenomonadaceae and Bifidobacteriaceae in the HF group were significant higher. And the level of Saccharimonadaceae, Helicobacteraceae and Clostridaceae were significantly lower than CTR group (P < 0.05, Figure 3H). At the genus level, the HFD induced a decrease in Lactobacillus and an increase in Chlamydia in HF group (Figure 3F). The proportions of Bacteroides, Helicobacter and Klebsiella showed a significant increase, and the proportions of Bifidobacterium, Quinella showed a significant decrease (P < 0.05, Figure 3I). These data suggest that the abundance of gut microbes in obesity-induced precocious puberty rats was significantly different from that in CTR group.
Figure 3 HFD alters gut microbiota in obesity-induced precocious puberty female rats. During postnatal day (PND) 21, female rats were fed a HFD and a normal diet until the rats were sacrificed during diestrus after completing the estrous cycle. Microbial 16S rDNA genes were subsequently sequenced. (A) PCoA plot of Bray-Curtis distance of the gut microbiota. (B) PCoA plot Jaccard distance of gut microbiota. (C) PCoA plot of unweighted Uni-Frac distance of gut microbiota. (D–F) Community composition distribution at the phylum, family and genus level. (G–I) The most differentially abundant proportions between the HF and CTR group at the phylum, family and genus levels. n=6 per group for gut microbiota analysis. Significance was tested with the Wilcoxon rank-sum test.
It is widely recognized that the intestinal microbiota affects the endocrine system by synthesizing neurotransmitters and active metabolites, such as SCFAs (30). In our study, the SCFAs levels in the feces of obesity-induced precocious puberty rats were significantly changed. Our results showed that the levels of SCFAs in the HF group were significantly lower in acetic, propionic and hexanoic acid. (Figures 4A, B, Figure 4E). Although the level of butyric acids was not statistically different, there was a trend to decrease (Figure 4C). Correspondingly, the levels of valeric acid, isobutyric acid and isovaleric acids increased (Figures 4D–G). Furthermore, the mRNA expression of GPR43, GPR41 and GPR109a in the colon (Figures 4H–J) was significantly increased in the HF group.
Figure 4 HFD alters the levels of fecal SCFAs and their related receptors in the colon in the rats with obesity-induced precocious puberty. (A) The content of acetic acid in fecal. (B) The content of propanoic acid in fecal. (C) The content of butyric acid in fecal. (D) The content of valeric acid in fecal. (E) The content of hexanoic acid in fecal. (F) The content of isobutyric acid in fecal. (G) The content of isovaleric acid in fecal. (H) The gene expression of GPR43 in the colon. (I) The gene expression of GPR41 in the colon. (J) The gene expression of GPR109a in the colon. Fecal SCFAs levels were detected by GC-MS. n = 6 per group for rat samples. *P<0.05; **P<0.01; ***P<0.001. ns, not significant.
To further explore whether obesity-induced precocious puberty is caused by intestinal microbiota and SCFAs, we added 5% sodium acetate, sodium propionate or sodium butyrate or the three together as a mixture to the HFD. After adding the SCFAs sodium acetate, sodium propionate or sodium butyrate or their mixture, the body weight of immature female adolescent rats was significantly lower than that of the HF group (Figure 5A). Compared to the HF group, supplementation with SCFAs attenuated HFD-induced total cholesterol, triglyceride, LDL-C and HDL-C levels (Figures 5B–E). In addition, the age of VO and the first estrous cycle were significantly delayed (Figures 5F, G). In HFD rats that received diet supplementation with SCFAs, there was a significant decrease in serum LH and FSH (Figures 5H, I).
Figure 5 SCFAs reverse obesity and precocious puberty in obesity-induced precocious puberty rats. (A) Evolution of body weight. (B) Serum total cholesterol. (C) Serum triglyceride. (D) Serum low density lipoprotein cholesterol (LDL-C). (E) Serum high density lipoprotein cholesterol (HDL-C). (F) Cumulative percentage of vaginal opening. (G) Cumulative percentage of first estrus. (H) Serum LH levels. (I) Serum FSH levels. (J) Morphological and histopathological changes in uterine and uterine wall thickness after addition of SCFAs. (K) Relative uterus weight. (L) Histological score of follicular development and ovulation after addition of SCFAs diet. (M) Relative ovary weight. n = 6 per group for rat samples. *P<0.05; **P<0.01.
After supplementation with 5% SCFAs in HFD, histopathological and morphological changes in the uterus showed that the thickness of the uterine endometrium (Figure 5J) in the SCFAs treated groups was obviously lower than that in the HF group, as was the relative uterus weight (UW) (Figure 5K). To further assess the ovarian physiology of different groups, we evaluated the histological score of follicular development and ovulation. As shown in Figures 5L, M, SCFAs reduced the percentage of rats that ovulated by the HFD that reached ovulation (HF 83.3% versus HF-A 16.6% versus HF-P 16.6% versus HF-B 50% versus HF-SCFA 16.6%), as well as the relative ovary weight (OW). The above studies show that SCFAs can significantly affect the development of sexual organs in rats.
We observed the effect of SCFAs on the gene expression of the HPGA. Compared with the HF group, 5% sodium acetate, sodium propionic, sodium butyrate and their mixtures significantly reduced the mRNA expression of the Kiss1, GPR54, GnRH and ERα genes in the hypothalamus (Figures 6A–D). Considering the pituitary responsiveness to hypothalamic neuropeptides, the levels of GnRHR were found to significantly decline with the addition of SCFAs (Figure 6E). LH, FSH and estrogen control the overall activity of the female reproductive tract by binding to their receptors on ovarian cells (31). To this end, we detected LHR, FSHR and ERα in the ovary. Consistent with the development of the ovary, SCFAs reduced the expression of the gonadotropin receptors LHR, FSHR and ERα in the ovary (Figures 6F–H). The above results indicate that SCFAs significantly reduce HFD-mediated changes in the expression of genes related to HPGA, thereby restoring its level to that of a normal diet.
Figure 6 SCFAs reverse the expression of genes related to the HPGA in rats with obesity-induced precocious puberty. (A)The gene expression of Kiss1 in the hypothalamus. (B) The gene expression of GPR54 in the hypothalamus. (C) The gene expression of GnRH in the hypothalamus. (D) The gene expression of ERα in the hypothalamus. (E) Gene expression of GnRHR in the pituitary. (F) The gene expression of FSR in the ovary. (G) The gene expression of LHR in the ovary. (H) The gene expression of ERα in the ovary. n = 6 per group for rat samples. *P<0.05; **P<0.01.
We further examined the intestinal microbiota of rats in the HF the group and SCFAs intervention groups. We found that the fecal α-diversity analysis based on Chao1, Shannon and Simpson indexes in the SCFAs intervention groups revealed a significant decrease compared with the HF group (Figure 7A). Analysis of β-diversity based on the Bray−Curtis, Jaccard and unweighted UniFrac PCoA analyses showed that the microbiota of the SCFAs added groups were significantly distinct (Figures 7B–D). This was consistent with previous studies showing that HFD increases gut microbiota biodiversity (20). SCFAs reverse the biodiversity of HFD. Notably, at the phylum level, there were significant differences in Firmicutes, Bacteroidota, Verrucomicrobiota, Proteobacteria, Actinobacteriota and Campylobacterota (Figures 7E, G). Interestingly, after adding the SCFAs mixture, compared with that in the HF group, the bacterial change in relative abundance was the most significant. At the genus level, there were significant differences among Chamydia and Lactobacillus, Escherichia-Shigella, Bacteroidetes, Romboutsia, Lactococcus and Alloprevotella (Figures 7F, H). The above data showed that compared with the HF group, the abundance of gut microbiota changed significantly after SCFAs intervention. Considering the protective effect of SCFAs on the metabolic parameters studied, as well as on the change in gut microbiota, we evaluated the SCFAs binding and activation the orphan G-protein-coupled receptors GPR43, GPR41 and GPR109a in the colon. The elevated mRNA levels of GPR43, GPR41 and GPR109a (Figures 7I–K) showed a significant decrease in SCFAs-supplemented groups compared to the HF group.
Figure 7 SCFAs regulate microbiota and its receptors change in obesity-induced precocious puberty rats. During PND21, female rats were fed HFD, and 5% acetate, propionate, butyrate diet and their mixtures were added until the rats were sacrificed during diestrus after completing in estrous cycle. Microbial 16S rDNA genes were subsequently sequenced. (A) α-diversity of the chao1, shannon and simpon index in different groups. (B) PCoA plot of Bray-Curtis distance of the gut microbiota. (C) PCoA plot Jaccard distance of gut microbiota. (D) PCoA plot of unweighted Uni-Frac distance of gut microbiota. (E, F) Community composition distribution at the phylum and genus levels. (G, H) The most differentially abundant proportions between the HF, CTR, HF-A, HF-P, HF-B and HF-SCFA groups at the phylum and genus levels. n=6-8 per group for gut microbiota analysis. Significances was tested with the Wilcoxon rank-sum test. (I) The gene expression of GPR43 in the colon. (J) The gene expression of GPR41 in the colon. (K) The gene expression of GPR109a in the colon. n = 6 per group for rat samples. *P<0.05; **P<0.01.
Studies have reported that GnRH release may be related to PKC and phosphorylated ERK1/2, as phosphorylation of these proteins is considered the main cellar signaling event in the activation of the GPR54-GnRH pathway (32, 33). To further explore the potential molecular regulatory mechanism of gut microbiota-derived SCFAs of the GPR54-GnRH signaling pathway in the hypothalamus, we measured the protein levels of GPR54, PKC, and ERK1/2 in the hypothalamus (Figure 8A) and examined the secretion of GnRH in the hypothalamus between the HF group and the SCFAs supplement groups. Compared with the HFD, SCFAs significantly reduced the protein expression of GPR54 and PKC and the phosphorylation of ERK (Figures 8B–D) and the secretion of GnRH (Figure 8E) in the hypothalamus. These data suggest that GPR54, PKC and ERK1/2 may be the key mediators of SCFAs in obesity-induced precocious puberty (Figure 8F).
Figure 8 SCFAs regulate hypothalamic GnRH release through GPR54-PKC-ERK1/2 pathway. (A) Representative Western blot images showing GPR54, PKC, p-ERK1/2, t-ERK1/2 and β-actin expression in rats added with SCFAs. (B–D) Statistical analysis of GPR54, PKC and p-ERk1/2 expression in each group. (E) GnRH secretion in the hypothalamus. (F) HFD led to a decrease in SCFAs in the gut and promoted the expression of the GPR54-PKC-ERK1/2 pathway. Supplementation with SCFAs with a HFD reversed the high expression of the GPR54-PKC-ERK1/2 pathway and further decreased the secretion of GnRH, weakening the activation of the pituitary gland and inhibiting gonadal development. n = 6 per group for rat samples. *P<0.05; **P<0.01; ***P<0.001.
Central precocious puberty (CPP) refers to the appearance of secondary sexual characteristics before the age of 8 in girls and 9 in boys. The underlying reason is the premature activation of the HPGA (34). However, the incidence of CPP tends to higher in children with obesity, and accumulative evidence has shown that the intestinal microbiota can change the expression of genes and their related metabolites through SCFAs, which play an important role in weight regulation in children with obesity (35, 36). The concentrations of propionic acid and butyric acid in the feces of children with obesity are lower than those of normal-weight children. This change is related to an imbalance in the abundance of phylum Firmicutes (37). Consistent with previous reports, we found that the obesity-induced precocious puberty rats showed gut microbiota imbalance. Correspondingly, the proportion of gut microbiota-derived SCFAs is also dysregulated (20, 38). In our study, acetic acid, propionic acid and butyric acid in the intestinal tract decreased in obesity-induced precocious puberty rats, and the changes in SCFAs may be related to the changes of Bacteroides, Bifidobacterium, Quinella, Helicobacter, Klebsiella.
Acetic acid, propionic acid and butyric acid are important components of SCFAs, accounting for more than 95% of SCFAs. Acetate, propionate and butyrate act as synthetic metabolic substrates and signal molecules in cell function (39–42). To further explore the effect of SCFAs on obesity-induced precocious puberty, we used a HFD containing 5% SCFAs to interfere with an obesity-induced precocious puberty rat model and found that SCFAs could significantly reverse the phenotype of obesity, which was consistent with previous results (25). At the same time, we found that SCFAs could also reverse the age of VO and the first estrous cycle as well as endometrial thickness and premature ovarian maturation in obesity-induced precocious puberty rats. The above results imply that SCFAs can alleviate HFD induced obesity and precocious puberty, and that SCFAs supplementation may play a role in the prevention of obesity and precocious puberty.
During the process of puberty induction, hypothalamic Kiss1 and its receptor GPR54 function as important regulators of GnRH nerve cell activation and GnRH secretion and play an important role in puberty maturation (22). Our results confirmed that SCFAs can significantly decrease the gene expression of Kiss1, GPR54, GnRH and ERα in the hypothalamus of obesity-induced precocious puberty rats. In our study, under the low expression of the Kiss1 gene and GPR54, which ultimately downregulated GnRHR activation and secretion of LH and FSH in the pituitary gland. Furthermore, the expression of LHR and FSHR in the ovaries was reduced, which further delayed the maturation of the ovaries and uterus, reversed the development of the gonadal axis and alleviated the symptoms of precocious puberty in obesity-induced precocious puberty rats. The above results suggest that the interdependence of gut microbiota with SCFAs may be the reason for precocious puberty in HFD.
After adding 5% SCFAs to the HFD, we found that the gut microbiota also changed, accompanied by reversing the symptoms of precocious puberty. SCFAs increased the relative abundance of Chlamydia and Lactobacillus and decreased the relative abundance of Bacteroides, Romboutsia, Lactococcus and Alloprevotella. Consistent with previous studies, the increase in Chlamydia is associated with obesity-related metabolic syndrome, and the increase in Lactobacillus can reverse obesity induced by HFD and regulate the microbiological SCFAs hormone pathway to regulate metabolism (43, 44). The increase in Bacteroides abundance is related to the level of estradiol (18). Bacteroides, Romboutsia, Lactococcus and Alloprevotella are related to obesity and related metabolic syndrome and showed a positive correlation with Lactococcus and GnRH, and the above bacteria may play a role in promoting sexual development (20, 45, 46). In our results, the increase in Lactobacillus and the decrease in Bcateroides, Romboutsia, Lactococcus and Alloprevotella may be the reasons why SCFAs reverse HFD-induced precocious puberty in rats. However, changes in intestinal microbiota and SCFAs led to changes of GPR43, GPR41 and GPR109a in the colon. It was found that GPR43, GPR41 and GPR109a in the intestinal tract of obesity-induced precocious puberty rats were significantly increased, and SCFAs supplementation decreased the expression of GPR43, GPR41 and GPR109a in the intestine. The above conclusions are consistent with the conclusions reported in the literature on obesity in mice (25). SCFAs alter the bacterial community structure in the gut and may be responsible for the changes in the expression of GPR43, GPR41 and GPR109a (25).
Human GPR54 plays a role in the regulation of endocrine function. Kisspeptin, the product of Kiss1 neurons, binds to GPR54 and stimulates PIP2 hydrolysis, Ca2+ mobilization, arachidonic acid release, ERK1/2 and p38 MAP kinase phosphorylation and inhibits cell proliferation (22, 47). To confirm the exact effect of SCFAs on the GPR54-GnRH pathway in obesity-induced precocious puberty rats induced by HFD, we studied its effect on related proteins. We found that the SCFAs in HFD could significantly downregulate the levels of GPR54, reduce the expression of PKC, inhibit phosphorylated ERK1/2 signal pathway, and reduce the secretion of hypothalamic GnRH, thus reducing the secretion of pituitary LH and FSH, thus inhibiting the development of the gonadal axis and relieving the symptoms of precocious puberty.
These findings indicate the complexity of the mechanism behind obesity-induced precocious puberty. We demonstrated that the gut microbiota and its derived SCFAs play a certain role in the pathogenesis and prevention of obesity-induced precocious puberty. In this complex context, our findings focus on the fact that we act on Kiss1 neurons and their receptor GPR54 through SCFAs derived from intestinal microbiota, and then reduce the release of hypothalamic GnRH and pituitary LH and FSH through the PKC-ERK1/2 pathway and delay the development of the ovary and uterus. As discussed here, the opportunity and improvement of SCFAs for obesity-induced precocious puberty promise to provide new strategies and open avenues for treatment.
The datasets generated for this study are included in the article. The data presented in the study are deposited in the NCBI repository, accession number PRJNA893237.
The animal study was reviewed and approved by Ethics Committee of Children’s Hospital of Chongqing Medical University (No. CHCMU-IACU20211028002).
LW carried out all experiments and data analysis and prepared the manuscripts. JZ, JT, BT, and HX designed the experiments, review, and revised the manuscripts. QY and RW contributed to reagent management. HL, YC and HD edit the chart. All authors approved the final manuscript.
This work was supported by the National Natural Science Foundation of China (grant No. 81700250 and 81670270).
The authors declare that the research was conducted in the absence of any commercial or financial relationships that could be construed as a potential conflict of interest.
All claims expressed in this article are solely those of the authors and do not necessarily represent those of their affiliated organizations, or those of the publisher, the editors and the reviewers. Any product that may be evaluated in this article, or claim that may be made by its manufacturer, is not guaranteed or endorsed by the publisher.
1. Castaneda D, Gabani M, Choi SK, Nguyen QM, Chen C, Mapara A, et al. Targeting autophagy in obesity-associated heart disease. Obes (Silver Spring) (2019) 27:1050–8. doi: 10.1002/oby.22455
2. Collaborators GBDO, Afshin A, Forouzanfar MH, Reitsma MB, Sur P, Estep K, et al. Health effects of overweight and obesity in 195 countries over 25 years. N Engl J Med (2017) 377:13–27. doi: 10.1056/NEJMoa1614362
3. David LA, Maurice CF, Carmody RN, Gootenberg DB, Button JE, Wolfe BE, et al. Diet rapidly and reproducibly alters the human gut microbiome. Nature (2014) 505:559–63. doi: 10.1038/nature12820
4. Fung TC, Olson CA, Hsiao EY. Interactions between the microbiota, immune and nervous systems in health and disease. Nat Neurosci (2017) 20:145–55. doi: 10.1038/nn.4476
5. Karra P, Winn M, Pauleck S, Bulsiewicz-Jacobsen A, Peterson L, Coletta A, et al. Metabolic dysfunction and obesity-related cancer: Beyond obesity and metabolic syndrome. Obes (Silver Spring) (2022) 30:1323–34. doi: 10.1002/oby.23444
6. Weihrauch-Bluher S, Schwarz P, Klusmann JH. Childhood obesity: Increased risk for cardiometabolic disease and cancer in adulthood. Metabolism (2019) 92:147–52. doi: 10.1016/j.metabol.2018.12.001
7. Shearrer GE, Sadler JR, Papantoni A, Burger KS. Earlier onset of menstruation is related to increased body mass index in adulthood and altered functional correlations between visual, task control and somatosensory brain networks. J Neuroendocrinol (2020) 32:e12891. doi: 10.1111/jne.12891
8. Reinehr T, Roth CL. Is there a causal relationship between obesity and puberty? Lancet Child Adolesc Health (2019) 3:44–54. doi: 10.1016/s2352-4642(18)30306-7
9. Huang A, Roth CL. The link between obesity and puberty: what is new? Curr Opin Pediatr (2021) 33:449–57. doi: 10.1097/MOP.0000000000001035
10. Bell JA, Carslake D, Wade KH, Richmond RC, Langdon RJ, Vincent EE, et al. Influence of puberty timing on adiposity and cardiometabolic traits: A mendelian randomisation study. PloS Med (2018) 15:e1002641. doi: 10.1371/journal.pmed.1002641
11. Nieuwenhuis D, Pujol-Gualdo N, Arnoldussen IAC, Kiliaan AJ. Adipokines: A gear shift in puberty. Obes Rev (2020) 21:e13005. doi: 10.1111/obr.13005
12. Ghaemi N, Ghahraman M, Noroozi Asl S, Vakili R, Fardi Golyan F, Moghbeli M, et al. Novel DNA variation of GPR54 gene in familial central precocious puberty. Ital J Pediatr (2019) 45:10. doi: 10.1186/s13052-019-0601-6
13. Torres-Fuentes C, Schellekens H, Dinan TG, Cryan JF. The microbiota–gut–brain axis in obesity. Lancet Gastroenterol Hepatol (2017) 2:747–56. doi: 10.1016/s2468-1253(17)30147-4
14. Silva YP, Bernardi A, Frozza RL. The role of short-chain fatty acids from gut microbiota in gut-brain communication. Front Endocrinol (Lausanne) (2020) 11:25. doi: 10.3389/fendo.2020.00025
15. Wang T, Sha L, Li Y, Zhu L, Wang Z, Li K, et al. Dietary alpha-linolenic acid-rich flaxseed oil exerts beneficial effects on polycystic ovary syndrome through sex steroid hormones-Microbiota-Inflammation axis in rats. Front Endocrinol (Lausanne) (2020) 11:284. doi: 10.3389/fendo.2020.00284
16. Dong G, Zhang J, Yang Z, Feng X, Li J, Li D, et al. The association of gut microbiota with idiopathic central precocious puberty in girls. Front Endocrinol (Lausanne) (2019) 10:941. doi: 10.3389/fendo.2019.00941
17. Indiani C, Rizzardi KF, Castelo PM, Ferraz LFC, Darrieux M, Parisotto TM. Childhood obesity and Firmicutes/Bacteroidetes ratio in the gut microbiota: A systematic review. Child Obes (2018) 14:501–9. doi: 10.1089/chi.2018.0040
18. Li Y, Shen L, Huang C, Li X, Chen J, Li SC, et al. Altered nitric oxide induced by gut microbiota reveals the connection between central precocious puberty and obesity. Clin Transl Med (2021) 11:e299. doi: 10.1002/ctm2.299
19. Wang M, Zhang Y, Miller D, Rehman NO, Cheng X, Yeo JY, et al. Microbial reconstitution reverses early female puberty induced by maternal high-fat diet during lactation. Endocrinology (2020) 161:bqz041. doi: 10.1210/endocr/bqz041
20. Bo T, Liu M, Tang L, Lv J, Wen J, Wang D. Effects of high-fat diet during childhood on precocious puberty and gut microbiota in mice. Front Microbiol (2022) 13:930747. doi: 10.3389/fmicb.2022.930747
21. Aghaee S, Deardorff J, Greenspan LC, Quesenberry CP Jr., Kushi LH, Kubo A. Breastfeeding and timing of pubertal onset in girls: a multiethnic population-based prospective cohort study. BMC Pediatr (2019) 19:277. doi: 10.1186/s12887-019-1661-x
22. Trevisan CM, Montagna E, de Oliveira R, Christofolini DM, Barbosa CP, Crandall KA, et al. Kisspeptin/GPR54 system: What do we know about its role in human reproduction? Cell Physiol Biochem (2018) 49:1259–76. doi: 10.1159/000493406
23. Xie Q, Kang Y, Zhang C, Xie Y, Wang C, Liu J, et al. The role of kisspeptin in the control of the hypothalamic-Pituitary-Gonadal axis and reproduction. Front Endocrinol (Lausanne) (2022) 13:925206. doi: 10.3389/fendo.2022.925206
24. Binder HJ. Role of colonic short-chain fatty acid transport in diarrhea. Annu Rev Physiol (2010) 72:297–313. doi: 10.1146/annurev-physiol-021909-135817
25. Lu Y, Fan C, Li P, Lu Y, Chang X, Qi K. Short chain fatty acids prevent high-fat-diet-induced obesity in mice by regulating G protein-coupled receptors and gut microbiota. Sci Rep (2016) 6:37589. doi: 10.1038/srep37589
26. Liu X, Li X, Xia B, Jin X, Zou Q, Zeng Z, et al. High-fiber diet mitigates maternal obesity-induced cognitive and social dysfunction in the offspring via gut-brain axis. Cell Metab (2021) 33:923–938.e926. doi: 10.1016/j.cmet.2021.02.002
27. Machate DJ, Figueiredo PS, Marcelino G, Guimaraes RCA, Hiane PA, Bogo D, et al. Fatty acid diets: Regulation of gut microbiota composition and obesity and its related metabolic dysbiosis. Int J Mol Sci (2020) 21:4093. doi: 10.3390/ijms21114093
28. Gaytan F, Morales C, Leon S, Heras V, Barroso A, Avendano MS, et al. Development and validation of a method for precise dating of female puberty in laboratory rodents: The puberty ovarian maturation score (Pub-score). Sci Rep (2017) 7:46381. doi: 10.1038/srep46381
29. Heras V, Castellano JM, Fernandois D, Velasco I, Rodriguez-Vazquez E, Roa J, et al. Central ceramide signaling mediates obesity-induced precocious puberty. Cell Metab (2020) 32:951–966.e958. doi: 10.1016/j.cmet.2020.10.001
30. Qi X, Yun C, Pang Y, Qiao J. The impact of the gut microbiota on the reproductive and metabolic endocrine system. Gut Microbes (2021) 13:1–21. doi: 10.1080/19490976.2021.1894070
31. Mehl NS, Srisuwatanasagul S, Swangchan-Uthai T, Sirivaidyapong S, Khalid M. GnRH-agonist implants suppress reproductive function and affects ovarian LHR and FSHR expression in prepubertal female cats. Theriogenology (2017) 87:250–8. doi: 10.1016/j.theriogenology.2016.09.003
32. Novaira HJ, Ng Y, Wolfe A, Radovick S. Kisspeptin increases GnRH mRNA expression and secretion in GnRH secreting neuronal cell lines. Mol Cell Endocrinol (2009) 311:126–34. doi: 10.1016/j.mce.2009.06.011
33. Bai GL, Hu KL, Huan Y, Wang X, Lei L, Zhang M, et al. The traditional Chinese medicine fuyou formula alleviates precocious puberty by inhibiting GPR54/GnRH in the hypothalamus. Front Pharmacol (2020) 11:596525. doi: 10.3389/fphar.2020.596525
34. Latronico AC BV, Causes CJC. Diagnosis, and treatment of central precocious puberty. Lancet Diabetes Endocrinol (2016) 4(3):265–74. doi: 10.1016/S2213-8587(15)00380-0
35. Dalile B, Van Oudenhove L, Vervliet B, Verbeke K. The role of short-chain fatty acids in microbiota-gut-brain communication. Nat Rev Gastroenterol Hepatol (2019) 16:461–78. doi: 10.1038/s41575-019-0157-3
36. Nirmalkar K, Murugesan S, Pizano-Zarate ML, Villalobos-Flores LE, Garcia-Gonzalez C, Morales-Hernandez RM, et al. Gut microbiota and endothelial dysfunction markers in obese Mexican children and adolescents. Nutrients (2018) 10:2009. doi: 10.3390/nu10122009
37. Murugesan S, Ulloa-Martinez M, Martinez-Rojano H, Galvan-Rodriguez FM, Miranda-Brito C, Romano MC, et al. Study of the diversity and short-chain fatty acids production by the bacterial community in overweight and obese Mexican children. Eur J Clin Microbiol Infect Dis (2015) 34:1337–46. doi: 10.1007/s10096-015-2355-4
38. Meyer RK, Lane AI, Weninger SN, Martinez TM, Kangath A, Laubitz D, et al. Oligofructose restores postprandial short-chain fatty acid levels during high-fat feeding. Obes (Silver Spring) (2022) 30:1442–52. doi: 10.1002/oby.23456
39. Al-Lahham SH, Peppelenbosch MP, Roelofsen H, Vonk RJ, Venema K. Biological effects of propionic acid in humans; metabolism, potential applications and underlying mechanisms. Biochim Biophys Acta (2010) 1801:1175–83. doi: 10.1016/j.bbalip.2010.07.007
40. Hong YH, Nishimura Y, Hishikawa D, Tsuzuki H, Miyahara H, Gotoh C, et al. Acetate and propionate short chain fatty acids stimulate adipogenesis via GPCR43. Endocrinology (2005) 146:5092–9. doi: 10.1210/en.2005-0545
41. den Besten G, van Eunen K, Groen AK, Venema K, Reijngoud DJ, Bakker BM. The role of short-chain fatty acids in the interplay between diet, gut microbiota, and host energy metabolism. J Lipid Res (2013) 54:2325–40. doi: 10.1194/jlr.R036012
42. Salvi PS, Cowles RA. Butyrate and the intestinal epithelium: Modulation of proliferation and inflammation in homeostasis and disease. Cells (2021) 10:1775. doi: 10.3390/cells10071775
43. Walenna NF, Kurihara Y, Chou B, Ishii K, Soejima T, Hiromatsu K. Chlamydia pneumoniae infection-induced endoplasmic reticulum stress causes fatty acid-binding protein 4 secretion in murine adipocytes. J Biol Chem (2020) 295:2713–23. doi: 10.1074/jbc.RA119.010683
44. Song W, Song C, Li L, Wang T, Hu J, Zhu L, et al. Lactobacillus alleviated obesity induced by high-fat diet in mice. J Food Sci (2021) 86:5439–51. doi: 10.1111/1750-3841.15971
45. Zeng Q, Li D, He Y, Li Y, Yang Z, Zhao X, et al. Discrepant gut microbiota markers for the classification of obesity-related metabolic abnormalities. Sci Rep (2019) 9:13424. doi: 10.1038/s41598-019-49462-w
46. Kong C, Gao R, Yan X, Huang L, Qin H. Probiotics improve gut microbiota dysbiosis in obese mice fed a high-fat or high-sucrose diet. Nutrition (2019) 60:175–84. doi: 10.1016/j.nut.2018.10.002
Keywords: obesity, puberty, microbiota, short chain fatty acids (SCFAs), high-fat diet
Citation: Wang L, Xu H, Tan B, Yi Q, Liu H, Deng H, Chen Y, Wang R, Tian J and Zhu J (2022) Gut microbiota and its derived SCFAs regulate the HPGA to reverse obesity-induced precocious puberty in female rats. Front. Endocrinol. 13:1051797. doi: 10.3389/fendo.2022.1051797
Received: 23 September 2022; Accepted: 21 November 2022;
Published: 09 December 2022.
Edited by:
Luisa M. Seoane, Servicio Gallego de Salud, SpainReviewed by:
Yuying Li, Institute of Bast Fiber Crops, Chinese Academy of Agricultural Sciences, ChinaCopyright © 2022 Wang, Xu, Tan, Yi, Liu, Deng, Chen, Wang, Tian and Zhu. This is an open-access article distributed under the terms of the Creative Commons Attribution License (CC BY). The use, distribution or reproduction in other forums is permitted, provided the original author(s) and the copyright owner(s) are credited and that the original publication in this journal is cited, in accordance with accepted academic practice. No use, distribution or reproduction is permitted which does not comply with these terms.
*Correspondence: Jing Zhu, amluZ3podUBjcW11LmVkdS5jbg==
Disclaimer: All claims expressed in this article are solely those of the authors and do not necessarily represent those of their affiliated organizations, or those of the publisher, the editors and the reviewers. Any product that may be evaluated in this article or claim that may be made by its manufacturer is not guaranteed or endorsed by the publisher.
Research integrity at Frontiers
Learn more about the work of our research integrity team to safeguard the quality of each article we publish.